- 1Yinshanbeilu Grassland Eco-hydrology National Observation and Research Station, China Institute of Water Resources and Hydropower Research, Beijing, China
- 2Institute of Water Resources for Pastoral Area Ministry of Water Resources, Hohhot, China
- 3Faculty of Environmental Sciences and Natural Resource Management, Norwegian University of Life Sciences, Aas, Norway
- 4School of Ecology and Environment, Inner Mongolia University, Hohhot, China
Soil aggregate is extremely important for soil health and sustainable land management. Overgrazing has caused serious degeneration of grassland in the past decades and how to restore the degraded soil through grazing management is urgently needed. In this research, we investigated effects of long-term grazing exclusion and short-term rotational grazing with different grazing intensities on aggregate stability in the upper 10 cm of soil at two grazing sites in Xilinhot, Inner Mongolia. Treatments included long-term (34 years) exclusion, moderate-term (21 years) exclusion, and continuous grazing at adjacent reference plots. In addition, effects of rotational grazing under different grazing intensity [i.e., no grazing (0 days/month), light grazing (3 days/month), moderate grazing (6 days/month) and high grazing (12 days/month)] were investigated after 5 years. Stability of aggregate fractions were determined using wet sieving. Our results showed that the stable aggregates fraction were significantly increased under grazing exclusion for both fine (0.25–1 mm) and coarse (1–2 mm) size fractions. At the rotational grazing site, stability of fine aggregates was significantly enhanced under grazing compared with no grazing, while there was little influence on stability of coarse aggregates. Our results showed that grazing exclusion significantly increased soil aggregate stability and the peak appeared in moderate-term exclusion, meanwhile, rotational grazing had little influence on aggregate stability. We suggest that rotational grazing rather than long-term grazing exclusion is a better way for soil aggregate stability and soil health, and current grazing prohibition policies may need to be adjusted.
Introduction
The steppe in arid and semi-arid of northern China is among the largest remaining natural grassland in the world (Kawamura et al., 2005). However, overgrazing has caused serious grassland soil deterioration in the past decades (Tong et al., 2004). Researchers have shown that grazing exclusion is effective to restore the degraded grassland soil (Mekuria et al., 2007; Cheng et al., 2011). Recently, several policies (e.g., Prohibiting Grazing Policy and Returning Grazing Lands to Grasslands) were implemented to restore the degraded grassland in northern China. When grazing is excluded, as the input of organic matter increases, it may have a beneficial effect on soil organic carbon (SOC) and physical structure, but excessive litter accumulation on the surface soil immobilizes organic carbon (Reeder and Schuman, 2002) and inhibits plant growth (Xiong and Nilsson, 1999; Dong et al., 2021). So, long-term absence of grazing may inhibit important ecosystem functions. The positive effects of controlled livestock grazing and prohibiting grazing on grassland ecosystems are widely reported, however, its potential negative effects on soil quality is rarely mentioned in Inner Mongolia.
Rotational grazing, also known as multi-paddock grazing, has been proposed as a potential alternative grazing management option to maintain ecosystem sustainability and enhance grassland productivity (Sanjari et al., 2010; Teague et al., 2011). Compared with continuous grazing, rotational grazing provides a short period of high intensity grazing, followed by a long recovery period, which is particularly important for herbs (Teague et al., 2011), and which could supply higher forage production (Gourlez et al., 2018). Rotational grazing has been well studied in Oceania and North America and was found to be valuable in practice (Sovell et al., 2000; Chan et al., 2010; Sanjari et al., 2010). In Inner Mongolia, however, previous studies are more focused on continuous grazing or seasonal grazing (e.g., Steffens et al., 2008; Kölbl et al., 2011; Reszkowska et al., 2011). The influence of rotational grazing has received little attention resulting in limited information about the effects of rotational grazing on soil in Inner Mongolia. Aggregate stability is of special importance for soil health and the stability of the SOC pool (Herrick et al., 2001), as it determines soil’s resistance to degradation and erosion (Six et al., 2000). Moreover, increased aggregate stability reduces SOC loss by hindering microbe accessibility and increasing interaction between mineral surfaces and SOC (Wu et al., 2012).
In this study, we assess the impact of 1) 34- and 21-years grazing exclusion vs ambient grazing and 2) 5 years rotational grazing with different grazing intensities on grasslands soils of Inner Mongolia on aggregate stability. The goal of this research is to evaluate the effect of grazing exclusion and rotational grazing on soil aggregate stability. We hypothesize that rotational grazing enhances aggregate stability, while both long-term grazing exclusion and continuous grazing tend to exert negative effects. This research will provide theoretical basis for grassland management and grazing system arrangements.
Method and Materials
Site Description and Experimental Design
The Exclusion Site and Rotational Grazing Site are both located in Xilinhot, Inner Mongolia (Figure 1). The climatic condition is a semiarid temperate continental climate, with more than 60% of precipitation occurring from July to September. Soils are classified as Kastanozem (IUSS Working Group, 2014).
The Exclusion Site (ES) is located near the Inner Mongolia Grassland Ecosystem Research Station (IMGERS, 43°38′ N, 116°42′ E). The mean annual precipitation and mean annual temperature are about 343 mm and 0.7°C, respectively (Steffens et al., 2008). In 1983, a fenced area of 24 ha was set up to exclude grazing (long-term exclusion, LTE). In 1996, an additional fenced area of 2 ha was established adjacent to the former (medium-term exclusion, MTE). The area outside the fences had continuous free grazing by sheep from local herdsmen (ambient grazing, AG) (Kölbl et al., 2011). The grassland was moderately to heavily degraded. At the time of sampling, in autumn 2017, the plots had been enclosed 34 and 21 years, respectively.
The Rotational Grazing Site (RGS) is located at the Xilinhot National Climate Observatory (44°08′ N, 116°20′ E). The mean annual precipitation and mean annual temperature are about 281 mm and 2.4°C, respectively. For the rotational grazing experiment, fenced plots with four treatments according to grazing gradients were set up in 2012, with three replications for each treatment. Since 2013, the grazing experiment has been conducted in growing season (i.e., from June to September). Each plot covers an area of 1.44 ha (120 m × 120 m) where 28 sheep grazed for 0 days (no grazing, NG), 3 days (light grazing, LG), 6 days (moderate grazing, MG) and 12 days per month (high grazing, HG), respectively (Figure 2). The unfenced (free grazing) area keeps continuous free grazing with low intensity. Soil samples were collected in July 2017, the 5th year of grazing experiment.
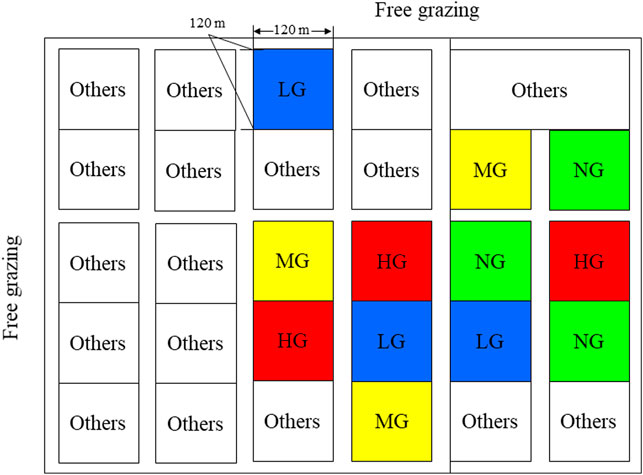
FIGURE 2. Experimental design at the rotational grazing site (NG: No grazing. LG: Light grazing. MG: Moderate grazing. HG: High grazing. Others: Other experiments which were not relevant to this research. Ambient grazing: Free ambient grazing).
An overview of belowground biomass and soil characteristics tested by related researchers at the same experimental plots is shown in Table 1.
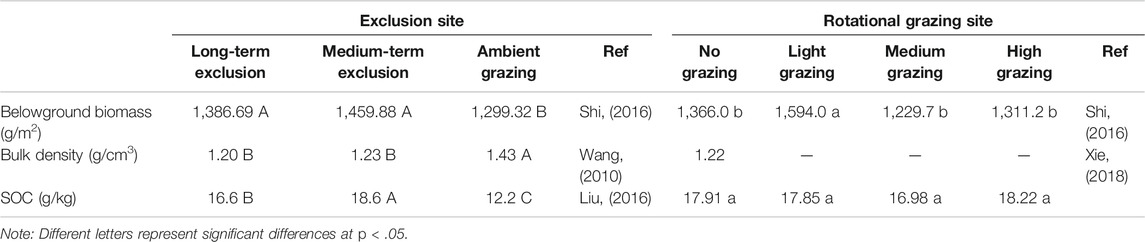
TABLE 1. Vegetation and soil characteristics (0–20 cm) at grazing exclusion sites and rotational grazing sites.
Soil Sampling and Pretreatment
At each plot, five points were chosen randomly and the distance between each two points was greater than 15 m. About 250 g soil from 0 to 10 cm was sampled at each point using a small spade and the five soil samples were bulked prior to drying and further treatment. After air-drying for 2 weeks, the soil samples were sieved at 2 mm. All samples were stored at room temperature until analysis.
Aggregate Stability
About 200 g air-dried soil was added to a set of nested sieves with sieve openings of 1 and 0.25 mm, respectively, to obtain aggregate fractions of 1–2 mm (coarse aggregates), 0.25–1 mm (fine aggregates).
The aggregate content was calculated according to:
where %A is the percentage of coarse/fine aggregate in bulk soil, ma is the mass of coarse/fine aggregate, m is the total mass of all size fractions.
Aggregate stability of the coarse and fine aggregate fractions was determined using wet sieving (Five Star Scientific, Twin Falls, United States). Both aggregate size classes (4 g), placed on the sieve (mesh 0.25 mm), were pre-moistened with distilled water for 5 min. Next, the sieve moved up and down for 3 min (stroke is 1.3 cm, at about 34 strokes/min), while immersed in a container with 80 ml distilled water. Unstable aggregates disintegrated and were collected in the container. Subsequently, the container with distilled water was replaced with a container having 80 ml dispersing solution (2 g L−1 sodium hexametaphosphate (NaPO3)6). The material remaining on the sieve was wet-sieved, while immersed in (NaPO3)6 solution, until only roots and mineral particles >0.25 mm were left on the sieve. The (NaPO3)6 container (with accumulated sediment from the stable aggregates, now disintegrated in the (NaPO3)6 solution) and the distilled water container (with unstable aggregates) were dried in an oven (at 65°C, for about 20 h) after which, the weights were determined. More details about the procedure are presented in Kemper and Rosenau (1986).
The aggregate stability of both the coarse and fine fractions was calculated according to:
where %WSA is the percentage of water stable aggregate in coarse/fine aggregate size fractions, ws is the mass of coarse/fine stable aggregate, wus is the mass of coarse/fine unstable aggregate.
The percentage of both the coarse and fine water stable aggregate in bulk soil was calculated according to:
where %WSAB is the percentage of coarse/fine water stable aggregate in bulk soil.
Statistics
Statistical analyses were done using R version 3.4.5. To compare the significance of differences between different treatments, Duncan’s multiple comparison (significance level p < .05) was calculated using Agricolae package (De Mendiburu, 2014).
Results
At the exclusion site, after 34- and 21-years grazing exclusion, the fraction of coarse aggregate was significantly greater in the exclusion than under ambient grazing. On the contrary, the fraction of fine aggregates decreased significantly by 22.89 and 14.34%, respectively, compared with ambient grazing (Table 2; Figure 3A). Rotational grazing had little influence on aggregate content except that light grazing enhanced the content of coarse aggregate (Table 2; Figure 4A).
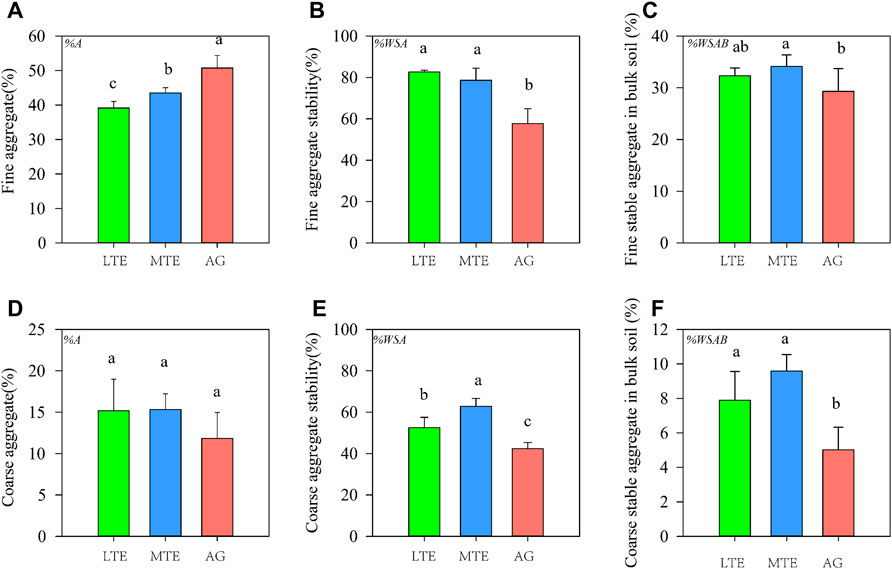
FIGURE 3. Aggregate content and aggregate stability (mean ± SD) in response to grazing exclusion in the fine fraction (A–C) and the coarse fraction (D–F). Different letters represent significant differences at p < .05. NOTE: LTE: Long-term exclusion; MTE: Medium-term exclusion; AG: Ambient grazing. %A: the percentage of coarse/fine aggregate in bulk soil; %WSA: the percentage of water stable aggregate in coarse/fine aggregate size fractions; %WSAB: the percentage of coarse/fine water stable aggregate in bulk soil.
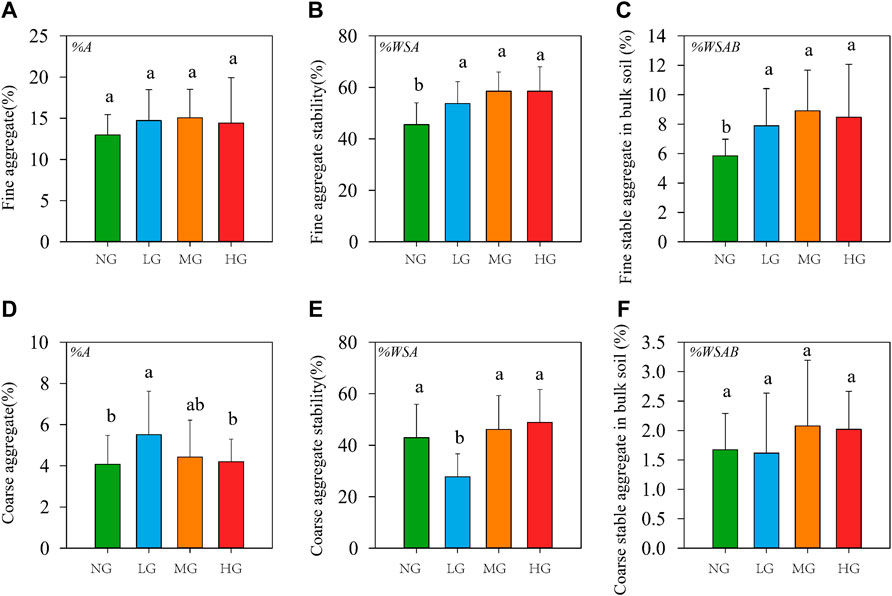
FIGURE 4. Aggregate content and aggregate stability (mean ± SD) in response to rotational grazing in the fine fraction (A–C) and the coarse fraction (D–F). Different letters represent significant differences at p < .05. NOTE: NG: No grazing; LG: Light grazing; MG: Medium grazing; HG: High grazing. %A: the percentage of coarse/fine aggregate in bulk soil; %WSA: the percentage of water stable aggregate in coarse/fine aggregate size fractions; %WSAB: the percentage of coarse/fine water stable aggregate in bulk soil.
Aggregate stability of both size fractions increased significantly after 34- and 21-years of grazing exclusion as compared to continuous grazing (Figures 3B,E). There was no significant difference in the percentage of stable aggregates (i.e., %WSA) in the fine size fractions between long-term exclusion and medium-term exclusion (Figures 3B,C). Yet, the stability of the coarse fraction was significantly greater under medium-term than under long-term grazing exclusion (Figure 3E). Meanwhile, the difference between the percentage of stable aggregates (i.e., %WSAB) in the coarse fractions was not significant (Figure 3F).
Relative to no grazing, rotational grazing significantly enhanced the fine aggregate stability (i.e., %WSA) irrespective of grazing density (Figure 4B) and further increased the proportion of fine stable aggregates (i.e., %WSAB) in the bulk soil (Figure 4C). On the other hand, in the coarse size fraction, the aggregate stability (i.e., %WSA) decreased significantly under light grazing compared to no grazing (Figure 4E), however, light grazing enhanced the percentage of coarse aggregate in the bulk soil (i.e., %A), as a result, the proportion of coarse stable aggregates in the bulk soil (i.e., %WSAB) did not change (Figure 4F and Eq. 3).
Discussion
Effect of Grazing Exclusion on Grassland Soil
Our results showed that, both medium-term and long-term grazing exclusion significantly increased the number of soil aggregates and the soil aggregate stability. Grassland soil is tightly related to plant productivity (Wang, 2010). Increased above- and belowground biomass, mainly functioning as plant litter inputs, may have multiple beneficial effects on soil physical and chemical properties (McSherry and Ritchie, 2013) in semi-arid grassland (Steffens et al., 2011). Previous researches showed that aggregate stability was highly related to root activity (Obia et al., 2016), and that soil organic matter (SOM) might function as a binding agent in the formation of aggregates (Bronick and Lal, 2005). Belowground biomass and SOC at long-term and medium-term exclusion were significantly higher than that at ambient grazing at the end of the 2016 growing season (Table 1). Higher primary productivity produces more root exudates (Wilson et al., 2018) and enhances aggregate stability. Combined with destruction of soil structure by livestock trampling in ambient grazing, which resulted in a negative effect on soil physical and chemical properties (i.e., higher bulk density, lower aggregate stability and SOC content; Table 1) (Steffens et al., 2008).
It is worth to note that, although livestock exclusion is commonly found to be positive to soil aggregate stability in Inner Mongolia (Su et al., 2005; Steffens et al., 2008; Kölbl et al., 2011), our results suggest that coarse aggregate stability shows a non–linear response to the length of exclusion period (Figure 3E). Specifically, we found that there was a peak in coarse aggregate stability after medium-term exclusion, whereas these properties declined in long-term exclusion experiments. Wu et al. (2008) found that aboveground biomass and SOC were the highest after 24 years of grazing exclusion (although not significant when compared with 28 years grazing exclusion and ambient grazing). This was because excess litter accumulating on the ground might influence plant growth, and litter accumulation decreased rainfall infiltration resulting in reduced soil water availability (Wu et al., 2008). In addition, Reeder and Schuman (2002) suggested that the accumulation of litter on the soil surface might restrict litter decomposition and thus C cycling and nutrient turnover in the ecosystem. Lower SOC content in long-term exclusion site [See (Dong et al., 2021)] decreased aggregate stability, and lower proportions of aggregate-occluded SOC in turn enhanced the risk of organic matter degradation and further decreased the aggregate stability (Wu et al., 2012).
Effect of Rotational Grazing on Grassland Soil
Our results showed that rotational grazing significantly increased the fine aggregate stability but had little influence on stability of coarse aggregates with the exception of a significant reduction under light grazing (Figure 4E). Due to the increased belowground biomass (see Table 1), light grazing significantly increased the total content of coarse aggregates in bulk soil, but it had little influence on the percentage of coarse stable aggregates in the bulk soil (Figure 4F). The reason might be that light grazing increased the unstable coarse aggregates rather than stable coarse aggregates, then, the percentage of coarse stable aggregates was relatively decreased (Figure 4E). By contrast, medium and high grazing did not increase the percentage of coarse aggregates (Table 2 and Figure 4D). This might be because higher grazing pressure under medium and high grazing also accompanied by more disturbances especially trampling, which could crush the aggregates. As a result, medium and high grazing offset the increased aggregates stimulated by grazing. Our result is consistent with Wang et al. (2020) who also found light grazing promoted the formation of coarse aggregates and higher grazing intensity caused the disintegration of soil aggregates. Our results also showed that coarse aggregate stability was lower than fine aggregate stability (Figures 3B,E, Figures 4B,E). Puget et al. (1998) showed that coarse aggregates (>1 mm) were more sensitive to grazing interference. As a result, more coarse aggregates were crushed by trampling, which might offset the enhancement of belowground biomass increase and lead to no increase in coarse aggregate stability (Wu et al., 2012).
In addition, rotational grazing did not result in significant differences between grazing intensities. Badgery et al. (2017) found that rotational grazing supported 7–22% higher stocking rate compared with continuous grazing. This was supported by Fang and Xie’s earlier findings at the same study site suggesting that rotational grazing had a slight influence on aggregates and organic C content (Fang, 2017; Xie, 2018). Considering that grassland had higher capacity under rotational grazing than continuous grazing of herbivores (Gourlez et al., 2018), even high grazing in this study was still within the carrying capacity of the ecosystem. This may suggest that grassland under rotational grazing has a higher resistance to the impact of grazing. In general, our results provided some evidence that rotational grazing, at least in a short-term, has little, if not positive, influence on aggregate stability.
Conclusion
Our results showed that grazing exclusion significantly increased soil aggregate stability, compared with continuous grazing. However, the peak appeared in moderate-term exclusion, whereas long-term exclusion tended to decrease these indicators compared with moderate-term exclusion (although the differences were not significant in some indicators). To restore the degenerated grassland, 2 decades of grazing exclusion was the best period. Five years of rotational grazing increased fine aggregate stability. Rotational grazing intensities had slight impact on soil. We suggest that a sustainable utilization (e.g., rotational grazing) rather than long-term exclusion is a better way to maintain soil health and livelihoods of the local herdsmen.
Data Availability Statement
The original contributions presented in the study are included in the article/Supplementary Material, further inquiries can be directed to the corresponding author.
Author Contributions
Conceived the ideas: LD, VM, CL and JM. Analysis and interpretation of data: LD and YZ. Drafting of the manuscript: LD and YZ. Critical revision of the manuscript for important intellectual content: JM and VM.
Funding
This study was funded by the Special Project of Basic Scientific Research Business Expenses of China Institute of Water Resources and Hydropower Research (MK 2021J11), the National Key Research and Development Plan of China (2016YFC0500503) and SIU project UTF-2016-long-term/10089.
Conflict of Interest
The authors declare that the research was conducted in the absence of any commercial or financial relationships that could be construed as a potential conflict of interest.
Publisher’s Note
All claims expressed in this article are solely those of the authors and do not necessarily represent those of their affiliated organizations, or those of the publisher, the editors, and the reviewers. Any product that may be evaluated in this article, or claim that may be made by its manufacturer, is not guaranteed or endorsed by the publisher.
Acknowledgments
The authors are indebted to the members of the Inner Mongolia Grassland Ecosystem Research Station (IMGERS) and Xilinhot National Climate Observatory (XNCO) who helped collect the samples. We also thanks laboratory engineers at the soil science laboratory of the Norwegian University of Life for help with lab work. LD also thanks the China Scholarship Council (CSC) for fellowship support.
References
Badgery, W. B., Millar, G. D., Michalk, D. L., Cranney, P., and Broadfoot, K. (2017). The Intensity of Grazing Management Influences Lamb Production from Native Grassland. Anim. Prod. Sci. 57, 1837–1848. doi:10.1071/An15866
Bronick, C. J., and Lal, R. (2005). Soil Structure and Management: a Review. Geoderma 124, 3–22. doi:10.1016/j.geoderma.2004.03.005
Chan, K. Y., Oates, A., Li, G. D., Conyers, M. K., Prangnell, R. J., Poile, G., et al. (2010). Soil Carbon Stocks under Different Pastures and Pasture Management in the Higher Rainfall Areas of South-Eastern Australia. Soil Res. 48, 7–15. doi:10.1071/Sr09092
Cheng, J., Wu, G. L., Zhao, L. P., Li, Y., Li, W., and Cheng, J. M. (2011). Cumulative Effects of 20-year Exclusion of Livestock Grazing on above- and Belowground Biomass of Typical Steppe Communities in Arid Areas of the Loess Plateau, China. Plant Soil Environ. 57, 40–44. doi:10.17221/153/2010-Pse
De Mendiburu, F. (2014). Agricolae: Statistical Procedures for Agricultural Research. R Package version1.2–8. Available at: https://CRAN.R-project.org/package=agricolae.
Dong, L., Martinsen, V., Wu, Y., Zheng, Y., Liang, C., Liu, Z., et al. (2021). Effect of Grazing Exclusion and Rotational Grazing on Labile Soil Organic Carbon in north China. Eur. J. Soil Sci. 72, 372–384. doi:10.1111/ejss.12952
Fang, J. (2017). Effects of Grazing on Productivity, Nutrient Content and Ecological Stoichiometry Feature of the Typical Grassland Communities. (In Chinese with English abstract). Inner Mongolia University, 13–20.
Gourlez de la Motte, L., MamadouMamadou, O., Beckers, Y., Bodson, B., Heinesch, B., and Aubinet, M. (2018). Rotational and Continuous Grazing Does Not Affect the Total Net Ecosystem Exchange of a Pasture Grazed by Cattle but Modifies CO2 Exchange Dynamics. Agric. Ecosyst. Environ. 253, 157–165. doi:10.1016/j.agee.2017.11.011
Herrick, J. E., Whitford, W. G., De Soyza, A. G., Van Zee, J. W., Havstad, K. M., Seybold, C. A., et al. (2001). Field Soil Aggregate Stability Kit for Soil Quality and Rangeland Health Evaluations. Catena 44, 27–35. doi:10.1016/S0341-8162(00)00173-9
Iuss Working Group, W. (2014). World Reference Base for Soil Resources 2014 International Soil Classification System for Naming Soils and Creating Legends for Soil Maps. Rome: FAO.
Kawamura, K., Akiyama, T., Yokota, H.-o., Tsutsumi, M., Yasuda, T., Watanabe, O., et al. (2005). Quantifying Grazing Intensities Using Geographic Information Systems and Satellite Remote Sensing in the Xilingol Steppe Region, Inner Mongolia, China. Agric. Ecosyst. Environ. 107, 83–93. doi:10.1016/j.agee.2004.09.008
Kölbl, A., Steffens, M., Wiesmeier, M., Hoffmann, C., Funk, R., Krümmelbein, J., et al. (2011). Grazing Changes Topography-Controlled Topsoil Properties and Their Interaction on Different Spatial Scales in a Semi-arid Grassland of Inner Mongolia, P.R. China. Plant Soil 340, 35–58. doi:10.1007/s11104-010-0473-4
Liu, J. (2016). “Research on Nitrogen Froms and Microbial Community Structure of Grassland Soil in Inner Mongolia,” in Chinese with English Abstract) (Zhejiang University), 16–17.
McSherry, M. E., and Ritchie, M. E. (2013). Effects of Grazing on Grassland Soil Carbon: a Global Review. Glob. Change Biol. 19, 1347–1357. doi:10.1111/gcb.12144
Mekuria, W., Veldkamp, E., Haile, M., Nyssen, J., Muys, B., and Gebrehiwot, K. (2007). Effectiveness of Exclosures to Restore Degraded Soils as a Result of Overgrazing in Tigray, Ethiopia. J. Arid Environments 69, 270–284. doi:10.1016/j.jaridenv.2006.10.009
Obia, A., Mulder, J., Martinsen, V., Cornelissen, G., and Børresen, T. (2016). In Situ effects of Biochar on Aggregation, Water Retention and Porosity in Light-Textured Tropical Soils. Soil Tillage Res. 155, 35–44. doi:10.1016/j.still.2015.08.002
Puget, P., Angers, D. A., and Chenu, C. (1998). Nature of Carbohydrates Associated with Water-Stable Aggregates of Two Cultivated Soils. Soil Biol. Biochem. 31, 55–63. doi:10.1016/S0038-0717(98)00103-5
Reeder, J. D., and Schuman, G. E. (2002). Influence of Livestock Grazing on C Sequestration in Semi-arid Mixed-Grass and Short-Grass Rangelands. Environ. Pollut. 116, 457–463. doi:10.1016/S0269-7491(01)00223-8
Reszkowska, A., Krümmelbein, J., Peth, S., Horn, R., Zhao, Y., and Gan, L. (2011). Influence of Grazing on Hydraulic and Mechanical Properties of Semiarid Steppe Soils under Different Vegetation Type in Inner Mongolia, China. Plant Soil 340, 59–72. doi:10.1007/s11104-010-0405-3
Sanjari, G., Ghadiri, H., Yu, B., and Ciesiolka, C. (2010). “Increase in Ground Cover under a Paddock Scale Rotational Grazing experiment in South-East Queensland,” in Proceedings of the 19th World Congress of Soil Science: Soil Solutions for a Changing World (International Union of Soil Sciences (IUSS), 52–55.
Shi, Y. (2016). Effects of Grazing on Underground Biomass and Individual Plant Root Traits of a Typical Grassland Community (In Chinese with English Abstract). Inner Mongolia Universituy, 12–17.
Six, J., Elliott, E. T., and Paustian, K. (2000). Soil Macroaggregate Turnover and Microaggregate Formation: a Mechanism for C Sequestration under No-Tillage Agriculture. Soil Biol. Biochem. 32, 2099–2103. doi:10.1016/S0038-0717(00)00179-6
Sovell, L. A., Vondracek, B., Frost, J. A., and Mumford, K. G. (2000). Impacts of Rotational Grazing and Riparian Buffers on Physicochemical and Biological Characteristicsof southeastern Minnesota, USA, Streams. Environ. Manage. 26, 629–641. doi:10.1007/s002670010121
Steffens, M., Kölbl, A., Schörk, E., Gschrey, B., and Kögel-Knabner, I. (2011). Distribution of Soil Organic Matter between Fractions and Aggregate Size Classes in Grazed Semiarid Steppe Soil Profiles. Plant Soil 338, 63–81. doi:10.1007/s11104-010-0594-9
Steffens, M., Kölbl, A., Totsche, K. U., and Kögel-Knabner, I. (2008). Grazing Effects on Soil Chemical and Physical Properties in a Semiarid Steppe of Inner Mongolia (P.R. China). Geoderma 143, 63–72. doi:10.1016/j.geoderma.2007.09.004
Teague, W. R., Dowhower, S. L., Baker, S. A., Haile, N., DeLaune, P. B., and Conover, D. M. (2011). Grazing Management Impacts on Vegetation, Soil Biota and Soil Chemical, Physical and Hydrological Properties in Tall Grass Prairie. Agric. Ecosyst. Environ. 141, 310–322. doi:10.1016/j.agee.2011.03.009
Tong, C., Wu, J., Yong, S., Yang, J., and Yong, W. (2004). A Landscape-Scale Assessment of Steppe Degradation in the Xilin River Basin, Inner Mongolia, China. J. Arid Environments 59, 133–149. doi:10.1016/j.jaridenv.2004.01.004
Wang, H. (2010). “The Study of Soil Physical Properties and Infiltration Capacity of Typical Grassland,” in Chinese with English Abstract (Inner Mongolia University), 17–23.
Wang, J., Zhao, C., Zhao, L., Wen, J., and Li, Q. (2020). Effects of Grazing on the Allocation of Mass of Soil Aggregates and Aggregate-Associated Organic Carbon in an alpine Meadow. Plos one 15, e0234477. doi:10.1371/journal.pone.0234477
Wilson, C. H., Strickland, M. S., Hutchings, J. A., Bianchi, T. S., and Flory, S. L. (2018). Grazing Enhances Belowground Carbon Allocation, Microbial Biomass, and Soil Carbon in a Subtropical Grassland. Glob. Change Biol. 24, 2997–3009. doi:10.1111/gcb.14070
Wu, H., Wiesmeier, M., Yu, Q., Steffens, M., Han, X., and Kögel-Knabner, I. (2012). Labile Organic C and N Mineralization of Soil Aggregate Size Classes in Semiarid Grasslands as Affected by Grazing Management. Biol. Fertil. Soils 48, 305–313. doi:10.1007/s00374-011-0627-4
Wu, L., He, N., Wang, Y., and Han, X. (2008). Storage and Dynamics of Carbon and Nitrogen in Soil after Grazing Exclusion in Leymus Chinensis Grasslands of Northern China. J. Environ. Qual. 37, 663–668. doi:10.2134/jeq2007.0196
Xie, D. S. (2018). Study on the Change Process of Soil Aggregates and Organic Carbon under Different Grazing Gradient in Typical Steppe. (In Chinese with English abstract). Inner Mongolia University.
Xiong, S., and Nilsson, C. (1999). The Effects of Plant Litter on Vegetation: a Meta-Analysis. J. Ecol. 87, 984–994. doi:10.1046/j.1365-2745.1999.00414.x
Keywords: aggregation, grazing management, long-term exclusion, steppe, suitability
Citation: Dong L, Zheng Y, Martinsen V, Liang C and Mulder J (2022) Effect of Grazing Exclusion and Rotational Grazing on Soil Aggregate Stability in Typical Grasslands in Inner Mongolia, China. Front. Environ. Sci. 10:844151. doi: 10.3389/fenvs.2022.844151
Received: 27 December 2021; Accepted: 19 January 2022;
Published: 11 February 2022.
Edited by:
Huawei Pi, Henan University, ChinaReviewed by:
Xiaoyu Meng, Henan University, ChinaBaicheng Niu, Northwest Institute of Eco-Environment and Resources (CAS), China
Copyright © 2022 Dong, Zheng, Martinsen, Liang and Mulder. This is an open-access article distributed under the terms of the Creative Commons Attribution License (CC BY). The use, distribution or reproduction in other forums is permitted, provided the original author(s) and the copyright owner(s) are credited and that the original publication in this journal is cited, in accordance with accepted academic practice. No use, distribution or reproduction is permitted which does not comply with these terms.
*Correspondence: Jan Mulder, amFuLm11bGRlckBubWJ1Lm5v