- 1Environmental Engineering Program, University of Northern British Columbia, Prince George, BC, Canada
- 2Ecosystem Science, Fisheries and Oceans Canada, Ottawa, ON, Canada
- 3School of Life and Environmental Sciences, Wenzhou University, Wenzhou, China
This study investigated the preparation of stable conventional heavy crude oil-in-water (O/W) emulsions by mechanical homogenization with the addition of a non-ionic surfactant, Tween-20. A four-factor, five-level central composite design was carried out to investigate the effects of four independent variables, including mixing intensity (4,000–10,000 rpm), mixing duration (5–45 min), water salinity (0–40 g/L), and the concentration of emulsifier (0.1–2.1 wt%) on the emulsion stability. Emulsion stability was determined by quantification of creaming index, turbidity change rate, and average oil droplet size. The results demonstrated that the salinity of 30 g/L, mixing intensity of 8,500 rpm, mixing duration of 35 min, and emulsifier concentration of 1.6 wt% led to the formation of the most stable emulsion.
1 Introduction
A liquid emulsion can be defined as a mixture of two immiscible liquids where one liquid is dispersed in the form of droplets in another continuous phase (Jha et al., 2014). An emulsion can be classified as oil/water (O/W), water/oil (W/O), and multiple emulsions: water/oil/water (W/O/W) or oil/water/oil (O/W/O) (Abed et al., 2019). These emulsions are essential components of many commercial products, and they have been used in different applications, including foods, cosmetics, personal care products, agrochemicals, supplements, and pharmaceuticals (Wong et al., 2015; Yukuyama et al., 2016). Emulsions are also generated during the production of crude oil (Khan et al., 2015). The occurrences of accidental and operational releases of oil in the marine environment during exploration, extraction, and transportation are well documented (Carpenter, 2019). In many cases, oil emulsions are formed from marine oil spills under the turbulent action of waves (Li et al., 2016). Similarly, oil spills in other water bodies with different salinity, such as freshwater and brackish water, also lead to the generation of emulsions (Tansel and Pascual, 2011).
Emulsions are not formed under normal conditions by contacting water and oil. The presence of interfacial barriers known as interfacial tension (IFT) prevents the complete mixing of two immiscible liquids, thus preventing the formation of the emulsion. Therefore, emulsion formation requires energy input: a mechanical mixing force such as shaking and stirring (Nour, 2018). During the production of crude oils, various turbulent sources of mixing energy can create shear forces. Generally, the more significant the amount of shear, the smaller the droplet size of the dispersed phase and the tighter the emulsion (Liu et al., 2016). In the ocean, waves and tides can provide adequate mixing energy to produce stable emulsions with many types of crude oils (Doerffer, 2013). Different researchers have used various equipment and methods to prepare O/W emulsions in the laboratory, including ultrasound, high shear homogenizer, high-pressure homogenizer, microfluidizer, colloid mill, and membrane emulsification (Silva et al., 2016; Taha et al., 2020).Although emulsion generation using a mechanical homogenizer is more complex and requires more energy than other processes such as sonication (Gavahian et al., 2018), mechanical homogenization is one of the commonly used techniques for preparing emulsions as it generates a large shearing and cutting force leading to the formation of fine emulsions (Yang et al., 2012; Mayer et al., 2013).
Emulsions are thermodynamically unstable, and they have a natural tendency to break down into oil and water phases over time through several processes, including gravitational separation, coalescence (or flocculation), and Ostwald ripening (Israelachvili, 2011). Gravitational separation in emulsion occurs due to the difference in density between dispersed and continuous phases. When droplets float up to the top, it is referred to as creaming, and when they sink to the bottom of the vessel, it is called sedimentation. Likewise, droplet aggregation (also known as flocculation), occurs when droplets attract each other. Lastly, Ostwald ripening is the phenomenon in which smaller particles attach and dissolve on the surface of the larger particles to reach a more thermodynamically stable state, leading to the formation of larger droplets which reduces the stability of emulsions (Costa et al., 2019; Mal et al., 2021).
To increase the stability of emulsions, surfactants, also known as emulsifiers, have been widely used due to their special amphiphilic nature. A surfactant molecule consists of a hydrophilic head that interacts with water and a hydrophobic tail that is attracted by the oil (Esmaeili et al., 2019). Surfactants migrate to the oil-water interface; an energy barrier is thereby generated around the dispersed droplets to prevent them from coalescing as shown in Figure 2. In addition, surfactants can reduce the interfacial tension between oil and water phases so that the energy required for droplet dispersion is reduced, facilitating the emulsification process (Kumar and Mahto, 2017). Tween 20, a widely used non-ionic surfactant, is suitable for preparing O/W emulsions (Sartomo et al., 2020). The salient features of these non-ionic surfactants are, they are not affected by the salinity of the water, have a relatively low price (Saad et al., 2020), non-toxic (Alahmer et al., 2010), and lastly, emulsions prepared by these surfactants can be easily separated (Kumar and Mahto, 2017).
A better understanding of O/W emulsion formation is crucial because of the oil spills in the aquatic environment, and the properties of the generated emulsions are essential for predicting, controlling, and mitigating the environmental impacts of such emulsions in different water bodies (Payne and Phillips, 2018). Likewise, selecting appropriate technologies for treating such emulsions requires an understanding of different factors that affect the formation and stability of the emulsions (Goodarzi and Zendehboudi, 2019). The current study investigates the optimum operating conditions to prepare stable conventional heavy crude O/W emulsions using a mechanical homogenizer and an emulsifier, Tween 20. Effects of mixing intensity, mixing duration, pH, and Tween 20 concentration were studied on the stability of the prepared O/W emulsions. The stability of the prepared O/W emulsion was determined by different methods, including creaming stability test, turbidity measurement, and optical microscopy. The results from the experiments can be used to prepare stable emulsions. The prepared emulsion can then be used to assess the performance of different technologies and processes in treating stable emulsions, as treatment of stable emulsions is one of the most significant challenges in oil spill response operations.
2 Materials and Methods
2.1 Materials
All chemicals were of analytical grade and purchased from Sigma-Aldrich (Oakville, ON, Canada). A conventional heavy crude oil recovered from the Western Canada Sedimentary Basin (WCSB) provided by Multi-Partner Oil Spill Research Initiative (MPRI) was used as the oil phase, and its physical properties are listed in Table 1. Test water phase samples of different salinity were prepared with ultrapure water (UPW, Milli-Q ® Advantage A10) and sodium chloride (NaCl, ≥99.0%). A synthetic surfactant (Tween 20, ≥40%) was used as an emulsifier to form O/W emulsions.
2.2 Equipment
A 700 watts high-speed homogenizer (Ultra Turrax T50, IKA®-Werke GmbH and Co.) fitted with a dispersing tool (IKA Works model: S50N-G45G) were used for generating O/W emulsions. A UV-Visible spectrophotometer (Lambda 465 UV/VIS Spectrophotometer, Perkin Elmer) with the wavelength range of 190–1,100 and 1 nm optical resolution was used to measure the turbidity of the prepared emulsion samples in quartz cuvettes (10 mm pathlength and 3.5 ml capacity). A compound microscope (Fisherbrand™ AX800) equipped with a digital camera (Fisherbrand™ C-Mount Digital Camera) was used to observe the microstructure of the emulsions.
2.3 Experimental Procedures
2.3.1 Preparation of Emulsion
Homogeneous test mixtures of saline water were prepared with 0–40 g of NaCl (detailed values shown in Table 3) in 1 L of UPW with a magnetic stirrer. For experiments, oil concentration was fixed at 3,000 ppm, and Tween 20 concentration was varied from 0.1–2.1 wt% (details shown in Table 3). Tween 20 was added to the saline test solutions and mixed thoroughly by the stirrer at 1,000 rpm, 30°C for 2 min before the addition of 3 g of the fresh conventional heavy crude oil. The IKA mechanical homogenizer and its dispersing tool attachment were used for the emulsification of the crude oil under various mixing intensities (4,000–10,000 rpm) with different mixing durations (5–45 min). The crude oil was dispersed throughout the water phase by high shearing forces and mixing energy. All the experiments were conducted at room temperature. The homogenizer was turned off for 20 min after 10 min of operation to prevent the heating of the prepared emulsions when high mixing intensities and long mixing durations were applied.
2.3.2 Emulsion Stability Analysis
The stability of the emulsion was investigated by three different techniques described in the sections below.
2.3.2.1 Creaming Stability Test
An 8 ml O/W emulsion was transferred into a 10 ml graduated cylinder immediately after preparation, and it was tightly capped to avoid evaporation and stored under room temperature for 24 h (Patil and Benjakul, 2017). Emulsion stability was estimated by measuring the thickness of the creaming layer (separated oil layer). The creaming index (CI) was expressed as the percentage of the total height of the cream layer at the top over the total height of the emulsion sample according to Eq. 1 (Campelo et al., 2017; Ferreira et al., 2010):
where CH is the height of a cream layer, EH is the total height of an emulsion sample. A larger CI indicates that the emulsion has lower stability.
2.3.2.2 Turbidity Measurement
Turbidity measurement for the emulsion stability offers the advantage of rapid and accurate determination of emulsion stability (Alade et al., 2021). Kundu et al. (2019) the concentration of oil droplets in the emulsion. The emulsion turbidity will decrease when there is less oil droplets with larger droplet size present in the emulsion due to droplet coalescence (Kundu et al., 2013). The turbidity was measured immediately after the preparation of the emulsion and after 24 h of storage (Zhang et al., 2016). A glass pipette was used to transfer 3 ml of prepared emulsion into a 3.5 ml quartz cuvette. The turbidity was measured at a wavelength of 260 nm by the transmission of light using a UV-Visible spectrophotometer. Each measurement was conducted in triplicate and the average transmittance reading was recorded. UPW was used as a blank. The turbidity was calculated using the equation below (Sezer, 2019; Mureșan and Dănilă, 2020):
where τ is the turbidity of the emulsion, L is the path length of the light (1 cm), I is the intensity of the light coming out of the sample, I0 is the intensity of the initial incident light beam.
The turbidity change rate was applied to indicate the emulsion stability in terms of the following equation:
where τi is the initial turbidity of the freshly prepared emulsion, τf is the turbidity measured after 24 h. The higher turbidity change rate indicates that the emulsion is less stable since more oil droplets float to the top.
2.3.2.3 Microscopy Analysis
Microscopic analysis was conducted to determine the mean diameter of oil droplets and droplet size distribution to investigate the emulsion stability. The smaller oil droplet size indicates the emulsion is more stable (Abbasi et al., 2020). After the homogenization of the emulsion, a drop of each sample was placed on a glass microscope slide. A compound microscope with a 10× ocular lens and 20× objective lens was used to visualize the dispersed oil droplets in the water phase (the image being viewed is 200 times its actual size). Images from the digital camera, captured on a computer using SeBaView software (Laxco Inc., Bothell, WA, United States) were processed with ImageJ (National Institutes of Health, Bethesda, MD, United States) image analysis software for particle analysis. For statistical analysis, the diameter of at least 300 oil droplets was measured for each sample.
2.4 Experimental Design
The experiments were designed using the Design Expert 12.0 (Stat-Ease Inc., Minneapolis, MN, United States). Response Surface Methodology (RSM) with Central Composite Design (CCD) was used to investigate the effects of different factors, including mixing duration, mixing intensity, salinity, Tween 20 concentration on the emulsion stability (Table 2). The full factorial design considered four factors at five levels, and a total of 27 runs (3 replicates at the center point to evaluate the error) were employed as shown in Table 3.
3 Results and Discussion
Table 4 shows the obtained results of the response variables, including creaming index, turbidity change rate, and average oil droplet size, in each experiment.
3.1 Creaming Index
The creaming Index in this study was best described by the regression equation provided (Table 5), after sequential omission of the non-significant factors. The model also had a non-significant lack of fit (p = 0.195 1). To predict a quadratic polynomial model, multiple regression coefficients were made by least squares technique, and concerning the coefficient significance, the following model was proposed:
where A is the salinity (g/L), B is the mixing intensity (rpm), C represents the mixing duration (minute), and D represents the Tween 20 concentration (wt%).
Results from the experiment indicated that the creaming stability was directly related to salinity, mixing intensity, mixing duration, and emulsifier concentration. Likewise, the mutual interaction between these parameters also was significant and affected the creaming Index.
It can be observed from Figure 1 that an increase in salinity led to a decrease in CI. The experiment results were in alignment with Ling et al. (2018). They investigated the effect of salinity on the stability of W/O emulsions by measuring droplet size distribution using nuclear magnetic resonance, and demonstrated that an increase in salinity resulted in the formation of a more stable emulsion. This result is contrary to that obtained by Maaref and Ayatollahi (2018), where they prepared the W/O (medium crude oil) emulsions using a magnetic stirrer and they indicated that an increase in salt concentration led to the formation of a less stable solution due to the increased rate of aggregation and coalescence. Moreover, as the mixing duration and mixing intensity increased, the CI gradually decreased. This could be due to the production of oil droplets with smaller sizes by higher shear force, finally increasing the emulsion stability (Kundu et al., 2016). The results were also found in alignment with the results from Ashrafizadeh and Kamran (2010). Besides, it was shown from Figure 3 that the increase in emulsifier concentration reduced CI, leading to the formation of a stable emulsion. Among all the different emulsifier concentrations used in this experiment, 1.6 wt% was the most favorable concentration for generating a stable O/W emulsion.
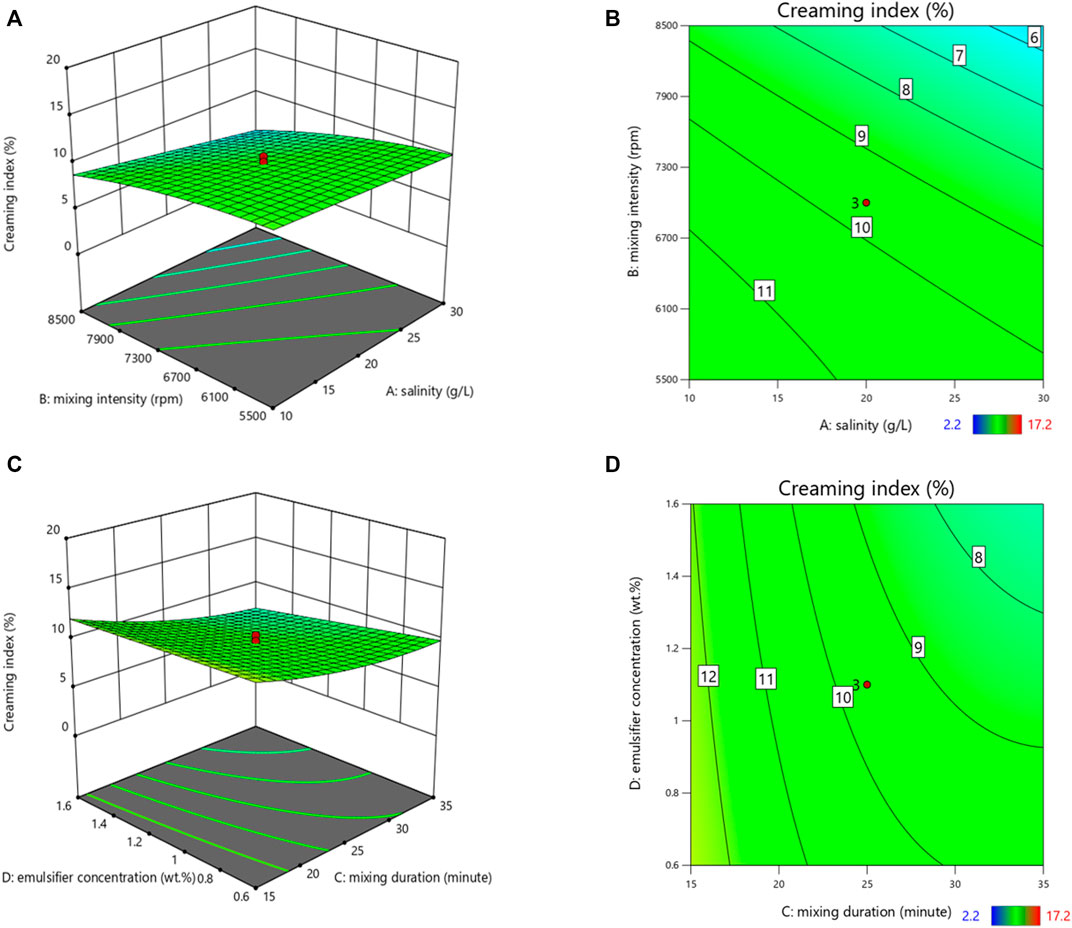
FIGURE 1. 3D surface plots (A,C) and their corresponding contour plots (B,D) showing the effects of variables on the creaming index.
3.2 Turbidity Change Rate
The turbidity in this study was best described by the regression equation provided (Table 6), after sequential omission of the non-significant factors. To predict a quadratic polynomial model, multiple regression coefficients were made by least squares technique, and concerning the coefficient significance, the following model was proposed:
where A is the salinity (g/L), B is the mixing intensity (rpm), C represents the mixing duration (minute), and D represents the Tween 20 concentration (wt%).
The stability of emulsions can be determined by measuring the change in turbidity with time; stable emulsion has a minor change in turbidity over time, whereas unstable emulsion has a greater change in turbidity over time. As shown in Figure 2, a decrease in mixing duration enhanced the rate of change in turbidity. Iqbal et al. (2020) investigated the effect of stirring duration on droplet size and emulsion stability. They found that the turbidity change rate after 24 h decreased when a longer mixing duration was applied, which is consistent with the present study. This could be attributed to a correlation between the shorter mixing time and larger, more mobile oil droplets. Hence, according to Stock’s law, the pronounced upward movement of larger droplets resulted in more oil rising to the top, leading to a greater decrease in turbidity after 24 h. In addition, oil droplet size decreased with increasing Tween 20 concentrations, as the surfactant reduces droplet coalescence by producing a film at the oil-water interface. Likewise, Kundu et al. (2013) prepared O/W emulsions using diesel oil and they found that with an increase in surfactant concentration, the oil droplet size decreased. They concluded that the increased surfactant concentration resulted in an increase in the interfacial area between oil phase and water phase, which helps to form oil droplets with small size and large number thus improves the emulsion stability.
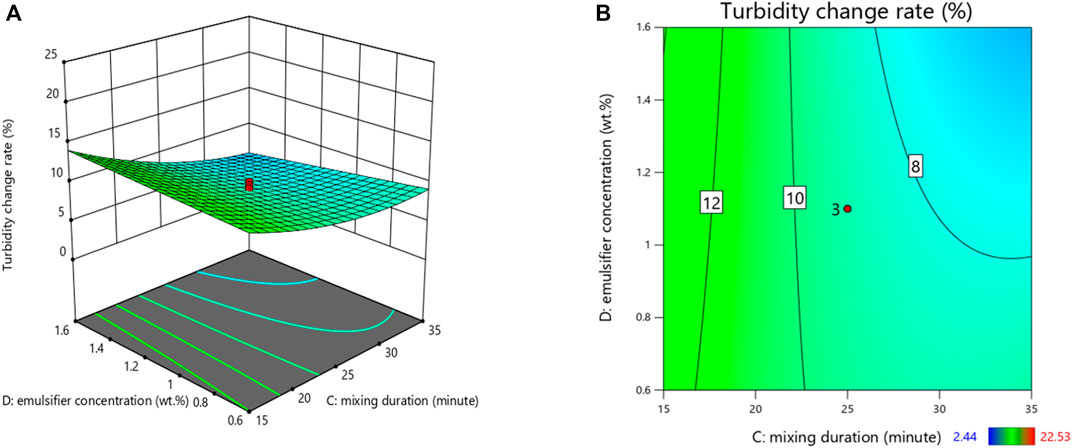
FIGURE 2. 3D surface plot (A) and its corresponding contour plots (B) showing the effects of variables on the turbidity change rate.
3.3 Droplet Size Index
Based on data collected (Figure 3), the largest average oil droplet size was 44.9 μm, which occurred at salinity of 20 g/L, mixing intensity of 7,000 rpm, mixing duration of 5 min, and emulsifier concentration of 1.1 wt%. In contrast, the smallest average oil droplet size was 16.12 μm, which occurred at salinity of 30 g/L, mixing intensity of 8,000 rpm, mixing duration of 35 min, and emulsifier concentration of 1.6 wt%.
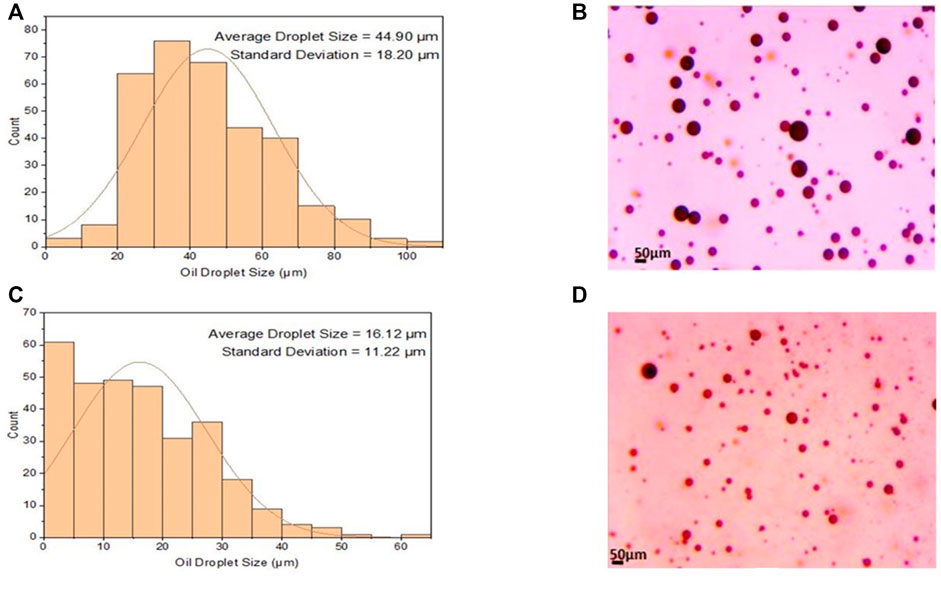
FIGURE 3. Oil droplet size distributions and microscope images of the most stable (C,D) and least stable emulsions (A,B).
A quadratic polynomial model was proposed in terms of coded factors for predicting the average oil droplet size in Eq. 6.
where A is the salinity (g/L), B is the mixing intensity (rpm), C represents the mixing duration (minute), and D represents the Tween 20 concentration (wt%).
ANOVA analysis (Table 7) showed that the proposed model is significant with a p-value lower than 0.05. The R2 is 0.942 2, which indicates that only 5.78% of the variance could not be explained by the independent variables in this regression model. The predicted R2 of 0.863 3 is in reasonable agreement with the adjusted R2 of 0.920 9.
As shown in Figure 4, the average oil droplet size in the prepared emulsion was adversely affected by the processing conditions in the mechanical homogenizer (i.e. mixing intensity), which indicated that higher mixing intensity led to more stable emulsions. Similarly, Kundu et al. (2019) also observed a decrease in oil droplet size when they increased the homogenization speed from 1,500 rpm to 4,700 rpm. They concluded that with higher mixing speed, more surfactants can be absorbed at the interface to stabilize the generated oil droplets in the emulsion. Furthermore, an increased salinity resulted in a slight decrease in the average oil droplet size which led to a more stable emulsion; a result is in line with previous studies (Esmaeili et al., 2019; Aman et al., 2015). As stated by Rocha et al. (2016), with the increase of water salinity, the IFT between the aqueous phase and oil phase decreased, reducing the driving force for droplet coalescence. In addition, increasing salinity may enhance the activity and effectiveness of natural surfactants (e.g., asphaltenes and resins) in the crude oil, which helps to stabilize the emulsion (Aman et al., 2015). Based on the study carried out by Esmaeili et al. (2019), another reason for the formation of a more stable emulsion with increased salinity might be because the addition of more salt would ionize the solution and form micelle to cover the oil droplets and prevent their coalescence.
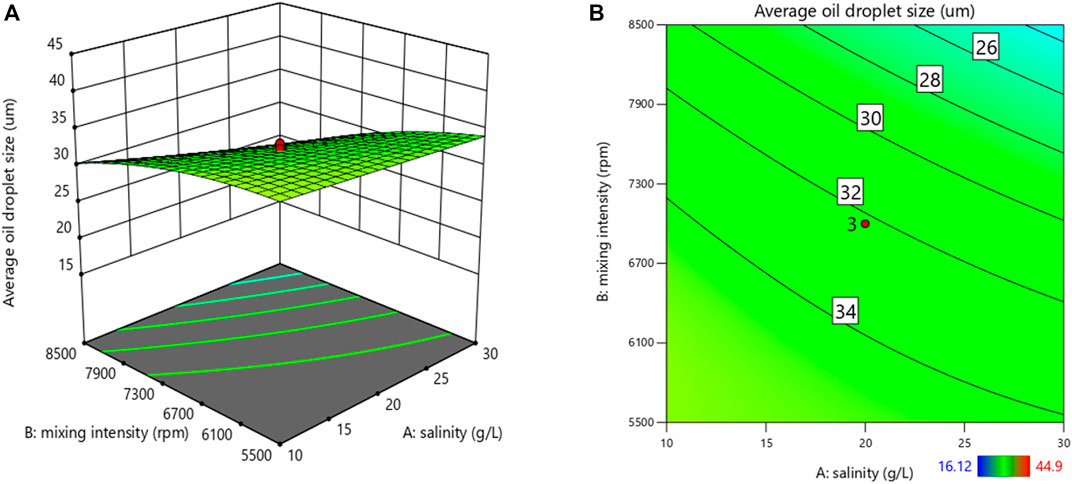
FIGURE 4. 3D surface plot (A) and its corresponding contour plots (B) showing the effects of variables on the average oil droplet size.
4 Conclusion
In this study, conventional heavy crude oil-in-water emulsions prepared by mechanical homogenization had oil droplet sizes less than 50 μm. Second-order models were proposed to simulate the optimal formation of conventional heavy crude O/W emulsions with the minimum creaming index, lowest turbidity change rate, and smallest average oil droplet size by RSM-CCD in design expert software. The investigated independent variables all had significant effects on emulsion stability. Over the range of experimental test conditions, increase in the mixing intensity and duration as well as salinity led to increased emulsion stability and a reduction in creaming index, turbidity change rate, and average oil droplet size. In addition, it was found that the application of Tween 20 as an emulsifier had great potential in stabilizing O/W emulsions. The results showed that the combination of 30 g/L water salinity, 8,500 rpm mixing intensity, 35 min mixing duration, and 1.6 wt% emulsifier concentration achieved the most stable O/W emulsion.
Data Availability Statement
The original contributions presented in the study are included in the article/Supplementary Material, further inquiries can be directed to the corresponding author.
Author Contributions
WS: Conceptualization, Methodology, Formal analysis, Investigation, Visualization, Writing—original draft preparation. NK: Conceptualization, Methodology, Formal analysis, Investigation, Visualization, Writing—original draft preparation. DM: Writing—review and editing. KL: Writing—review and editing. MZ: Writing—review and editing. JL: Supervision, Writing—review and editing.
Funding
This research was financially supported by the Natural Sciences and Engineering Research Council of Canada (NSERC) and Fisheries and Oceans Canada’s Multi-Partner Oil Spill Research Initiative (MPRI) (Project number MPRI 4.01 and 4.03).
Conflict of Interest
The authors declare that the research was conducted in the absence of any commercial or financial relationships that could be construed as a potential conflict of interest.
Publisher’s Note
All claims expressed in this article are solely those of the authors and do not necessarily represent those of their affiliated organizations, or those of the publisher, the editors, and the reviewers. Any product that may be evaluated in this article, or claim that may be made by its manufacturer, is not guaranteed or endorsed by the publisher.
Acknowledgments
The authors would like to thank the University of Manitoba for analyzing oil properties.
Abbreviations
O/W, oil/water; W/O, water/oil; W/O/W, Water/oil/water; O/W/O, Oil/water/oil; %, Percentage; IFT, interfacial tension; NaCl, sodium chloride; mPa·s, millipascal-second; RSM, response surface methodology; CCD, central composite design; μm, micrometer; g/L, gram per liter; g/cm3, gram per cubic centimeter; g, Gram; ppm, parts per million; wt%, Percentage by weight; CI, creaming index.
References
Abbasi, H., Karimi, S., and Gharibzahedi, S. M. T. (2020). Rhamnolipid as a Unique Emulsifier to Stabilize Sesame Oil‐in‐water Beverage Emulsions Formed by Ultrasound‐induced Cavitation: Optimizing the Formulation and Physical Properties. J. Food Process. Preserv. 44, 14810. doi:10.1111/jfpp.14810
Abed, S. M., Abdurahman, N. H., Yunus, R. M., Abdulbari, H. A., and Akbari, S. (2019). Oil Emulsions and the Different Recent Demulsification Techniques in the Petroleum Industry - A Review. IOP Conf. Ser. Mater. Sci. Eng. 702 (No. 1), 012060. doi:10.1088/1757-899X/702/1/012060
Alade, O. S., Mahmoud, M., Al Shehri, D. A., and Sultan, A. S. (2021). Rapid Determination of Emulsion Stability Using Turbidity Measurement Incorporating Artificial Neural Network (ANN): Experimental Validation Using Video/Optical Microscopy and Kinetic Modeling. ACS Omega 6, 5910–5920. doi:10.1021/acsomega.1c00017
Alahmer, A., Yamin, J., Sakhrieh, A., and Hamdan, M. A. (2010). Engine Performance Using Emulsified Diesel Fuel. Energ. Convers. Manag. 51, 1708–1713. doi:10.1016/j.enconman.2009.11.044
Aman, Z. M., Haber, A., Ling, N. N. A., Thornton, A., Johns, M. L., and May, E. F. (2015). Effect of Brine Salinity on the Stability of Hydrate-In-Oil Dispersions and Water-In-Oil Emulsions. Energy Fuels 29, 7948–7955. doi:10.1021/acs.energyfuels.5b02277
Ashrafizadeh, S. N., and Kamran, M. (2010). Emulsification of Heavy Crude Oil in Water for Pipeline Transportation. J. Pet. Sci. Eng. 71, 205–211. doi:10.1016/j.petrol.2010.02.005
Campelo, P. H., Junqueira, L. A., Resende, J. V. d., Zacarias, R. D., Fernandes, R. V. d. B., Botrel, D. A., et al. (2017). Stability of Lime Essential Oil Emulsion Prepared Using Biopolymers and Ultrasound Treatment. Int. J. Food Properties 20, S564–S579. doi:10.1080/10942912.2017.1303707
Carpenter, A. (2019). Oil Pollution in the North Sea: the Impact of Governance Measures on Oil Pollution over Several Decades. Hydrobiologia 845, 109–127. doi:10.1007/s10750-018-3559-2
Costa, C., Medronho, B., Filipe, A., Mira, I., Lindman, B., Edlund, H., et al. (2019). Emulsion Formation and Stabilization by Biomolecules: The Leading Role of Cellulose. Polymers 11, 1570. doi:10.3390/polym11101570
Esmaeili, H., Esmaeilzadeh, F., and Mowla, D. (2019). Effect of Salinity, pH, and Temperature on Stability of Gas Condensate in Water Emulsions Using Different Surfactants. Iran. J. Chem. Chem. Eng. 38, 151–166. doi:10.30492/IJCCE.2019.31061
Ferreira, M. R. A., Santiago, R. R., de Souza, T. P., Egito, E. S. T., Oliveira, E. E., and Soares, L. A. L. (2010). Development and Evaluation of Emulsions from Carapa Guianensis (Andiroba) Oil. AAPS PharmSciTech 11, 1383–1390. doi:10.1208/s12249-010-9491-z
Gavahian, M., Chen, Y.-M., Mousavi Khaneghah, A., Barba, F. J., and Yang, B. B. (2018). In-pack Sonication Technique for Edible Emulsions: Understanding the Impact of acacia Gum and Lecithin Emulsifiers and Ultrasound Homogenization on Salad Dressing Emulsions Stability. Food Hydrocolloids 83, 79–87. doi:10.1016/j.foodhyd.2018.04.039
Goodarzi, F., and Zendehboudi, S. (2019). A Comprehensive Review on Emulsions and Emulsion Stability in Chemical and Energy Industries. Can. J. Chem. Eng. 97, 281–309. doi:10.1002/cjce.23336
Iqbal, N., Kumar, N., Saini, M. K., Dubey, S., Agrawal, A., and Kumar, J. (2020). Role of High Shear Mixing in Improving Stability and Bio-Efficacy of Botanical Oil in Water Formulation for Early Stage Mosquito Eradication. Heliyon 6, e03380. doi:10.1016/j.heliyon.2020.e03380
Jha, P. K., Mahto, V., and Saxena, V. K. (2014). Emulsion Based Drilling Fluids: an Overview. Int. J. Chemtech Res. 6, 2306–2315.
Khan, J. A., Al-Kayiem, H. H., and Aris, M. S. (2015). “Stabilization of Produced Crude Oil Emulsion in the Presence of ASP,” in Proceeding of the SPE Asia Pacific Enhanced Oil Recovery Conference, Kuala Lumpur, Malaysia, August 2015 (OnePetro). doi:10.2118/174671-MS
Kumar, S., and Mahto, V. (2017). Use of a Novel Surfactant to Prepare Oil-In-Water Emulsion of an Indian Heavy Crude Oil for Pipeline Transportation. Energy Fuels 31, 12010–12020. doi:10.1021/acs.energyfuels.7b02437
Kundu, P., Agrawal, A., Mateen, H., and Mishra, I. M. (2013). Stability of Oil-In-Water Macro-Emulsion with Anionic Surfactant: Effect of Electrolytes and Temperature. Chem. Eng. Sci. 102, 176–185. doi:10.1016/j.ces.2013.07.050
Kundu, P., Paul, V., Kumar, V., and Mishra, I. M. (2016). An Adaptive Modeling of Petroleum Emulsion Formation and Stability by a Heuristic Multiobjective Artificial Neural Network-Genetic Algorithm. Pet. Sci. Tech. 34, 350–358. doi:10.1080/10916466.2015.1135169
Kundu, P., Arora, K., Gu, Y., Kumar, V., and Mishra, I. M. (2019). Formation and Stability of Water‐in‐oil Nano‐emulsions with Mixed Surfactant Using In‐situ Combined Condensation‐dispersion Method. Can. J. Chem. Eng. 97, 2039–2049. doi:10.1002/cjce.23481
Li, P., Cai, Q., Lin, W., Chen, B., and Zhang, B. (2016). Offshore Oil Spill Response Practices and Emerging Challenges. Mar. Pollut. Bull. 110, 6–27. doi:10.1016/j.marpolbul.2016.06.020
Ling, N. N. A., Haber, A., Graham, B. F., Aman, Z. M., May, E. F., Fridjonsson, E. O., et al. (2018). Quantifying the Effect of Salinity on Oilfield Water-In-Oil Emulsion Stability. Energy Fuels 32, 10042–10049. doi:10.1021/acs.energyfuels.8b02143
Liu, C., Li, M., Han, R., Li, J., and Liu, C. (2016). Rheology of Water-In-Oil Emulsions with Different Drop Sizes. J. Dispersion Sci. Tech. 37, 333–344. doi:10.1080/01932691.2015.1010729
Maaref, S., and Ayatollahi, S. (2018). The Effect of Brine Salinity on Water-In-Oil Emulsion Stability through Droplet Size Distribution Analysis: a Case Study. J. Dispersion Sci. Tech. 39, 721–733. doi:10.1080/01932691.2017.1386569
Mal, A., Ghosh, S., and Moulik, S. P. (2021). Time Dependent Physicochemical Changes of SDS-CTAB Interacted Self Assembled Vesicles: Ostwald Ripening Effect. Colloids Surf. A: Physicochemical Eng. Aspects 617, 126328. doi:10.1016/j.colsurfa.2021.126328
Mayer, S., Weiss, J., and McClements, D. J. (2013). Vitamin E-Enriched Nanoemulsions Formed by Emulsion Phase Inversion: Factors Influencing Droplet Size and Stability. J. Colloid Interf. Sci. 402, 122–130. doi:10.1016/j.jcis.2013.04.016
Mureșan, E. I., and Dănilă, A. (2020). Stabilization of W/O Emulsions Using Ecofriendly Emulsifiers. Bul. Inst. Politeh. Iaşi. 66, 23–30.
Nour, A. H. (2018). Emulsion Types, Stability Mechanisms and Rheology: A Review. Int. J. Innov. Res. Sci. Stud. 1, 11–17. doi:10.53894/ijirss.v1i1.4
Patil, U., and Benjakul, S. (2017). Characteristics of Albumin and Globulin from Coconut Meat and Their Role in Emulsion Stability without and with Proteolysis. Food Hydrocolloids 69, 220–228. doi:10.1016/j.foodhyd.2017.02.006
Payne, J. R., and Phillips, C. R. (2018). Petroleum Spills in the Marine Environment: The Chemistry and Formation of Water-In-Oil Emulsions and Tar Balls. Boca Raton, FL: CRC Press. doi:10.1201/9781351075480
Rocha, J. A., Baydak, E. N., Yarranton, H. W., Sztukowski, D. M., Ali-Marcano, V., Gong, L., et al. (2016). Role of Aqueous Phase Chemistry, Interfacial Film Properties, and Surface Coverage in Stabilizing Water-In-Bitumen Emulsions. Energy Fuels 30, 5240–5252. doi:10.1021/acs.energyfuels.6b00114
Saad, M. A., Abdurahman, N. H., Yunus, R. M., and Ali, H. S. (2020). Surfactant for Petroleum Demulsification, Structure, Classification, and Properties. A Review. IOP Conf. Ser. Mater. Sci. Eng. 991 (No. 1), 012115. doi:10.1088/1757-899X/991/1/012115
Sartomo, A., Santoso, B., Ubaidillah, O., and Muraza, O. (2020). Recent Progress on Mixing Technology for Water-Emulsion Fuel: A Review. Energ. Convers. Manag. 213, 112817. doi:10.1016/j.enconman.2020.112817
Sezer, P. (2019). Improving Gelation Properties of Fish Gelatin by Nonthermal Processes (Master's Thesis).
Silva, T. M., Cerize, N. N. P., and Oliveira, A. M. (2016). The Effect of High Shear Homogenization on Physical Stability of Emulsions. Int. J. Chem. 8, 52–61. doi:10.5539/ijc.v8n4p52
Taha, A., Ahmed, E., Ismaiel, A., Ashokkumar, M., Xu, X., Pan, S., et al. (2020). Ultrasonic Emulsification: An Overview on the Preparation of Different Emulsifiers-Stabilized Emulsions. Trends Food Sci. Tech. 105, 363–377. doi:10.1016/j.tifs.2020.09.024
Tansel, B., and Pascual, B. (2011). Removal of Emulsified Fuel Oils from Brackish and Pond Water by Dissolved Air Flotation with and without Polyelectrolyte Use: Pilot-Scale Investigation for Estuarine and Near Shore Applications. Chemosphere 85, 1182–1186. doi:10.1016/j.chemosphere.2011.07.006
Wong, S. F., Lim, J. S., and Dol, S. S. (2015). Crude Oil Emulsion: A Review on Formation, Classification and Stability of Water-In-Oil Emulsions. J. Pet. Sci. Eng. 135, 498–504. doi:10.1016/j.petrol.2015.10.006
Yang, Y., Marshall-Breton, C., Leser, M. E., Sher, A. A., and McClements, D. J. (2012). Fabrication of Ultrafine Edible Emulsions: Comparison of High-Energy and Low-Energy Homogenization Methods. Food Hydrocolloids 29, 398–406. doi:10.1016/j.foodhyd.2012.04.009
Yukuyama, M. N., Ghisleni, D. D. M., Pinto, T. D. J. A., and Bou‐Chacra, N. A. (2016). Nanoemulsion: Process Selection and Application in Cosmetics–A Review. Int. J. Cosmet. Sci. 38, 13–24. doi:10.1111/ics.12260
Keywords: emulsion stability, heavy crude oil, homogenizer, O/W emulsion, surfactant, turbidity
Citation: Shen W, Koirala N, Mukherjee D, Lee K, Zhao M and Li J (2022) Tween 20 Stabilized Conventional Heavy Crude Oil-In-Water Emulsions Formed by Mechanical Homogenization. Front. Environ. Sci. 10:873730. doi: 10.3389/fenvs.2022.873730
Received: 11 February 2022; Accepted: 07 April 2022;
Published: 29 April 2022.
Edited by:
Nsikak U. Benson, Covenant University, NigeriaReviewed by:
John Kapolis, University of the Peloponnese, GreecePankaj Tiwari, Indian Institute of Technology Guwahati, India
Partha Kundu, National Institute for Interdisciplinary Science and Technology (CSIR), India
Copyright © 2022 Shen, Koirala, Mukherjee, Lee, Zhao and Li. This is an open-access article distributed under the terms of the Creative Commons Attribution License (CC BY). The use, distribution or reproduction in other forums is permitted, provided the original author(s) and the copyright owner(s) are credited and that the original publication in this journal is cited, in accordance with accepted academic practice. No use, distribution or reproduction is permitted which does not comply with these terms.
*Correspondence: Jianbing Li, SmlhbmJpbmcuTGlAdW5iYy5jYQ==
†These authors have contributed equally to this work and share first authorship