- MOE Key Laboratory of Resources and Environmental Systems Optimization, College of Environmental Science and Engineering, North China Electric Power University, Beijing, China
Phosphorus (P) sorption by red soil is strong due to its acidic pH and high content of metal oxides. Biochar addition may increase the availability of red soil P by altering P sorption characteristics. This study examined the influences of doping ratios of the biochar, phosphate concentration, solution pH, and biochar-derived dissolved black carbon (DBC) on P sorption in red soil. The specific sorption mechanisms were explored using 3D fluorescence spectroscopy, infrared spectroscopy, and zeta potential analysis. The results show that the bioavailable P content in the biochar-amended red soil increased to 1.92 mg kg−1, which is an increase of 255% compared with the original red soil. With the increase in the doping ratio of biochar, the maximum sorption amount of P initially decreased and then increased. Moreover, the pH value and the content of the dissolved organic carbon (DOC) of the red soil were effectively elevated by biochar addition. The increase in the DOC content is likely caused by the DBC released from biochar. As the soil pH value increased, the amount of P sorption was reduced due to electrostatic repulsion. P sorption by the red soil decreased with the increasing DBC concentration. The humic acid-like substances in DBC can compete with phosphate for soil sorption sites, leading to a decrease in P absorption. This study demonstrates that the addition of biochar affects P sorption of the red soil mainly by changing its physicochemical properties or by releasing DBC to occupy the sorption sites.
Introduction
Phosphorus (P) is one of the essential nutrient elements for the growth and metabolism of animals and plants. Simultaneously, P is also a non-renewable resource (Hartono et al., 2005). Research shows that due to the increase of population growth and food demand, human demand for P is also increasing. Therefore, it is particularly important to study how to make rational use of P resources and improve the bioavailability of P in soil (Jalali and Peikam, 2013). Red soil, one of the important components of the earth’s soil environment, widely occurs in humid tropical and subtropical regions. As a result of the strong leaching and weathering processes under the humid climate situation, the red soil tends to be acidic and accumulates a massive amount of aluminum (Al) and iron (Fe) oxides (Helyar and Porter, 1989). P can be tightly associated with these metal oxides mainly via the formation of M-O-P bonds (M = a metal atom) and hydrogen bonds (O-H) (Zhang et al., 2020), which may greatly influence the transportation, transformation, and bioavailability of P in soils (Zhang et al., 2019). It has been demonstrated that the P utilization efficiency of seasonal crops in the red soil is substantially lower than that in other soils (Hu et al., 2001; Jalali and Peikam, 2013). Therefore, how to reduce the adsorption of P by the red soil and improving P availability have always been the focus of P nutrition management in acidic soils (Daniels and Lee, 2016).
Biochar is a solid material generated from the pyrolysis of biomass under hypoxic or anoxic conditions (Das et al., 2016; Sun et al., 2016). Because of its large specific surface area, extensive source of raw materials, rich surface functional groups, and abundant pore structures, biochar has an excellent application prospect in the field of soil improvement and remediation (Wang et al., 2009; Butterly et al., 2011; Wang et al., 2016). At present, biochar has generated increasing interest to be used to improve the bioavailability of nutrition in the soil. The influence of biochar on soil P sorption processes is regulated by soil types and biochar properties. As a result, controversial findings of P sorption in biochar-amended soils have been obtained (Hartono et al., 2005). Some studies have reported that P was greatly absorbed by biochar, and the content of available P in soil was reduced with the increasing content of biochar in soil (Xu et al., 2014; Shepherd et al., 2017). In contrast, other studies found that biochar supplementation in soil can decrease the adsorption of P via the competition between phosphate and the biochar surface functional groups for the adsorption sites on soil minerals (Morales et al., 2013). In addition, biochar can also indirectly influence P release by changing soil properties (e.g., mineral sorption ability and soil pH) (Xu et al., 2013).
Currently, the study on the effect of biochar on soil P adsorption largely focuses on biochar particles. Actually, after adding biochar to the soil, the dissolved black carbon (DBC), the biochar water-soluble fraction, can be easily released from the parent materials. The following dispersion and diffusion of DBC in soil environments would inevitably affect the soil P mobility, which, however, remains largely unknown. Previous studies have shown that adding dissolved organic matter (DOM), such as citric acid and oxalic acid, to soil raised the free P content in soil solutions (Guppy et al., 2005; Jalali and Peikam, 2013). Some oxygen (O)-containing groups of DOM can act as organic anions to compete with phosphate for the adsorption sites in soil, thus facilitating P desorption (Ruban et al., 2001; Schneider et al., 2016). The complexation of DOM and Fe/Al oxides is highly dependent on the aromaticity and O-containing groups of DOM. DBC has a high degree of aromatization and contains massive carboxylic acid groups. Therefore, DBC may have a high affinity to Fe/Al (oxy)hydroxides and leads to enhanced P release from the mineral surface. In line with our expectation, DBC has reportedly inhibited the adsorption of P on goethite (Ruban et al., 2001). However, the effect of DBC on P release in acidic red soil is still unclear.
In summary, we assume that after adding biochar to the red soil, soil P mobility can be affected not only by the alteration in the soil pH value but also by the DBC released from the biochar. To test our hypothesis, the rice straw biochar was generated and utilized in a red soil at ratios of 5, 3, and 1% (w/w). Subsequently, the effects of the doping ratios of the biochar, exogenous P concentration, solution pH, and DBC on P sorption by the red soil were investigated in detail. Three-dimensional excitation-emission matrix (3D-EEM) fluorescence spectroscopy, infrared spectroscopy, and zeta potential analysis were also applied to explore the underlying mechanisms. This research will be of great significance to improve P availability in acidic soils and will contribute to the reasonable application of biochar in soil improvement.
Materials and Methods
Raw Materials and Chemicals
Rice straw was sampled from the suburbs of Jinan, Shandong Province, China. The straw samples were first cleaned, dried, and crushed before being pyrolyzed under O-limited conditions at 550°C in a muffle furnace for 120 min. The heating rate was kept at 10°C min−1. After rinsing the biochar samples with ultrapure water, they were then dried, ground, sieved (0.25 mm), and stored for further use. The red soil used in this study was sampled from Meizhou, Guangdong Province. The biochar–soil mixtures were prepared by mixing the biochar and red soil in proportions of 1, 3, or 5% (biochar/soil; w/w). All of the chemicals and solvents employed in the present study were analytical grade with the highest purity (≥98%).
The biochars produced at 550°C were extracted using ultrapure water at a solution to solid ratio of 10:1 to acquire DBC. The suspensions were stirred at a temperature of 25°C at 120 rpm in darkness for 3 days. The acquired suspensions were then filtered through the PTFE filters (0.45 μm, Jinteng, Tian Jin, China). The filtrates were stored in darkness at a temperature of −4°C for further use.
P Sorption Experiments
Batch P adsorption experiments were carried out using the pure and biochar-amended soil as adsorbents. In the adsorption test, 4 g of pure soil or the biochar–soil mixture was weighed and placed in 40 ml glass vials. Next, 40 ml of KH2PO4 solution at various concentrations (0–240 mg P L−1) was prepared with the background solution containing 0.01 mol L−1 KCl to keep a constant ionic strength. The KH2PO4 solution was then added to the vials at a liquid to soil ratio of 10:1. The vials were then shaken on a rotary at room temperature (25°C) for 2 days to achieve an apparent adsorption equilibrium. Next, the vials were centrifuged at 3,000 r min−1 for 10 min. After centrifugation, the supernatant was immediately filtered through a PES filter (0.45 μm, Jinteng, Tian Jin, China) and the filtrate was collected and stored at a temperature of −4°C for the subsequent analysis.
DBC at varying concentrations (0, 5, 15, and 45 mg L−1) was chosen to examine its influence on P sorption by the red soil. Briefly, KH2PO4 and DBC solutions were added to the vials containing soil samples. The soil to liquid ratio and the procedures of shaking, centrifugation, and filtration were identical to those used in the batch sorption test.
The DOC content (mg kg−1) in the filtrate was determined using a total organic carbon analyzer (N3-1176/AR, Jena, Germany). The content of phosphate in the equilibrium solution was measured using the molybdenum blue approach on a UV-vis spectrophotometer.
Sequential Extraction
Hedley’s sequential chemical extraction was carried out to explore the mobility and speciation of P in the pure and biochar-amended soils (Smebye et al., 2016). Briefly, NaHCO3 (0.5 mol/L), NaOH (0.1 mol/L), and HCl (1.0 mol L−1) were sequentially utilized for the extraction of bioavailable, Fe/Al-bound, and Ca-bound P, respectively. After shaking for 16 h, the suspension acquired from each step of extraction was centrifuged (10 min, 3,000 rpm) to isolate the aqueous and solid phases. Subsequently, a small portion of supernatant was vacuum filtered (0.45 μm) and analyzed for P concentration as mentioned above.
Sample Characterization
By using the surface area and porosity analyzer (Micromeritics ASAP 2460, Norcross, GA, United States), the specific surface area (SSA; m2 g−1) could be measured with the N2 desorption and adsorption approach. The pH values of the soil were determined using a Sartorius pH meter (PB-10/C, GER) after extracting the soil samples with 0.01 mol L−1 CaCl2. A Malvern Zetasizer Nano ZSE nanoparticle size potentiometer (Worcestershire, United Kingdom) was used for detecting the zeta potential of biochar–soil mixtures via electrophoresis. Briefly, 0.1 g of the soil powder was weighed in a conical flask. Subsequently, distilled water (200 ml) was added to prepare a 0.5 g L−1 suspension. In addition, hydrochloric acid and KOH were used to adjust the solution pH to 3–9. After being shaken at 150 r/min for 2 h, the solutions were sampled for zeta potential analysis.
In addition, the 3D-EEM spectra of the DOM component in the sorption solution supernatant were acquired using a fluorescence luminescence spectrometer (F-7000; Hitachi, Japan) equipped with 700-V Xe lamp and a 1 cm quartz cell, and ultrapure water was utilized to subtract the blank. Through scanning 280–550 nm of emission wavelengths and 220–450 nm of excitation wavelengths, the synchronous fluorescence spectra for DOM samples were collected. The emission/excitation slit widths were set as 5.0 nm. The photomultiplier tube voltage and scanning speed were 400 V and 2,400 nm min−1, respectively (Wagner et al., 2018).
Data Analysis
The sorption data of P by the raw red soil and the biochar–soil mixtures were fitted with Langmuir (Eq. 1) and Freundlich (Eq. 2) sorption isotherms:
in which Qmax (mg·g−1) stands for the theoretical largest adsorption capacity; Ce represents the P concentration in the solution phase when the reaction reaches equilibrium; KL (mg1−n·Ln·g−1) represents the adsorption constant in the Langmuir model; KF (L·mg−1) is the adsorption affinity parameter; and n represents the dimensionless nonlinear coefficient.
In the experiment, the removal efficiency of P (Removal, %) and the adsorption amount Qe at equilibrium are calculated, respectively, as follows:
in which C0 (mg∙L−1) represents the initial P concentration in solution, and V (ml) and m (mg), respectively, denote the volume of the reaction solution and the sorbent mass.
Results and Discussion
Effect of Biochar Addition on the Physicochemical Properties of the Red Soil
Biochar is well known for its beneficial effects on soil properties. As clearly illustrated in Figure 1, with the increase in biochar dosage, the DOC concentration and the pH value of red soil exhibited an upward trend. For instance, after applying the biochar at a rate of 5%, the DOC content in the sorption equilibrium solution was substantially increased in the range of 5.1–27.1 mg L−1, and the soil pH values were increased in the range of 4.30–6.04. The increase of soil pH value can be attributed to the presence of various K, Ca, Na, and Mg oxides, carbonate, hydroxide, and other alkaline substances in the biochar. When the biochar is applied to the soil, it can increase the soil base saturation and improve the pH value. The increase in the DOC content is mainly caused by the DBC released from the biochar (Weng et al., 2008).
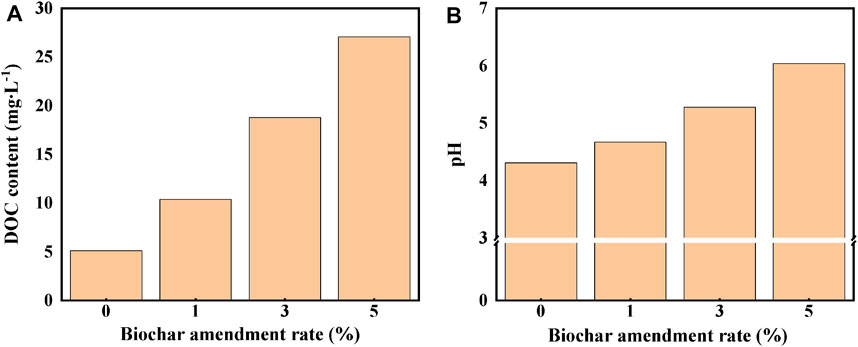
FIGURE 1. Effects of biochar addition on the DOC concentration (A) and pH value (B) of the red soil.
Moreover, N2 adsorption–desorption measurements were performed to obtain detailed textural information about the samples (Figure 2). It can be seen that the characteristic curves of both materials belong to type IV isotherms with H2 hysteresis loops, indicating that both samples have layered porous structures. BET analysis showed that after biochar amendment, the specific surface area of the red soil was slightly raised. For particle adsorption, the increase in the surface area will lead to an increase in adsorption sites, and then the corresponding adsorption amount will also increase. The elevation of pore volume may also exert a positive effect on P adsorption. In contrast, the addition of biochar to the red soil demonstrated a negligible influence on the pore size.
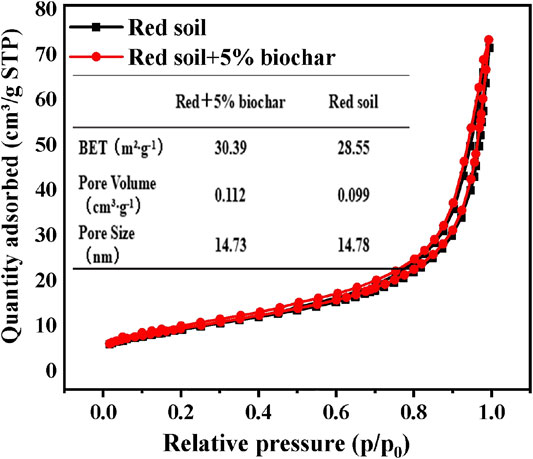
FIGURE 2. N2 adsorption/desorption isotherms, the surface area, pore size, and pore volume of the rice straw biochar-amended red soil.
The presence of different functional groups in the pure and biochar-amended soil samples was analyzed by FTIR spectroscopy (Figure 3). In the pure red soil sample, characteristic peaks at 875, 1080, 1687, and 3,500 cm−1 correspond to C-H, C-O, C=C, and O-H functional groups, respectively. Due to the addition of the biochar, the peak signal at 1080 and 875 cm−1 was enhanced, and the intensity increased with the increase of biochar addition, indicating that there was greater aromaticity in the red soils amended with biochar. In addition, previous studies have also shown that aromatic functional groups can interact with the hydroxyl groups on the metal minerals surface in soil (Schneider et al., 2016; Yang et al., 2020), occupying the sites that are originally for phosphate ion adsorption. Therefore, it is expected that the enhanced aromaticity of the soil caused by biochar addition would lead to the release of P from the Fe/Al mineral surface.
Phosphate adsorption by soil samples can be greatly affected by the surface charge of soil particles. Therefore, the zeta potential of the red soil before and after biochar addition was also measured in this study. As shown in Figure 5A, the isoelectric point (IEP) of the red soil was approximately 6.5. With an increase in the dose of the biochar, the IEP of the soil sample was lowered to 4.3; meanwhile, the zeta potential became more negative. After adding biochar, the IEP of the soil was reduced likely due to the elevation of the soil pH value, which may hinder the static sorption of P by the soil (Antelo et al., 2007). Fe/Al oxides, rich in hydroxyl groups, are well known as major carriers of the soil variable charge. When the soil pH value increases with biochar addition, the hydroxyl groups on the Fe/Al oxides surface would be deprotonated and demonstrate higher negative charges, reducing the zeta potential. At a fixed pH value, the addition of biochar enabled the surface charge of the red soil to be more negative.
P Speciation in the Pure and Biochar-Amended Soils
The results of the sequential extraction for P from the pure and biochar-amended red soils are shown in Table 1. For the pure red soil, the bioavailable P content was only 0.51 mg kg−1. After biochar amendment, the bioavailable P content in the red soil increased significantly. Specifically, when biochar was added at concentrations of 1%, 3%, and 5%, the bioavailable P content in the red soil substantially increased to 1.32, 1.52, and 1.92 mg kg−1, respectively, which was an increase of 159–255%. Given that the bioavailable P concentration in the biochar was only 0.22 mg kg−1, we can conclude that a large proportion of the increased bioavailable P was converted from other forms of P in the soil. This implies that biochar can facilitate the transformation of bio-unavailable P to available forms. Consistently, the amount of Fe/Al-bound P in the original red soil was raised up to 41.43 mg kg−1, while Ca-bound P was relatively low at 1.73 mg L−1. After adding biochar, the amount of Fe/Al-P in the red soil was markedly attenuated to approximately 11 mg g−1, with a reduced rate of about 75%. The reduction rate for Ca-P was about 41%. These results clearly confirmed that after adding biochar, the soil P that can be utilized by plants in the medium (Fe/Al-P) to long term (Fe/Al-P) was transformed into a short-term available form.
Effect of Biochar Addition on the P Sorption Behavior by the Red Soil
The Langmuir isotherms for P sorption by the pure and biochar-amended soil samples revealed higher coefficients of determination (R2 = 0.95–0.98) and fitted the detected data better than Freundlich isotherms (R2 = 0.69–0.81) (Figure 4; Table. 2). It can be seen that P adsorption by the red soil was mainly monolayer adsorption at room temperature (25°C). The P sorption parameter values (Qmax) of different soil samples obtained from Langmuir isotherm fitting were further analyzed. Contrary to expectation, inconsistent trends were found in Qmax values as the biochar amendment rate increased. The Qmax of the red soil decreased from 864.9 to 716.8 mg kg−1 as the rate of biochar amendment raised from 0 to 1%, but it was rapidly elevated to 934.0 mg kg−1 with a further increase of the biochar concentration to 5%. The explanations for these phenomena are as follows: 1) similar to low molecular weight organic acids, DBC released by the biochar (Figure 1A) might compete with the phosphate for adsorption sites on the surface of soil minerals, resulting in decreased P adsorption; 2) With the elevation of the soil pH after biochar addition, the surface charges of soil particles became more negative as evidenced by the zeta potential analysis (see details in Figure 5). These negative charges of soil particles could lead to a reduction in P adsorption through electrostatic attraction. 3) Biochar has a strong adsorption capacity for P (Xu et al., 2014; Shepherd et al., 2017). As the amendment rate of biochar raised from 1 to 5%, the facilitation role of the biochar in the P adsorption process was also gradually enhanced.
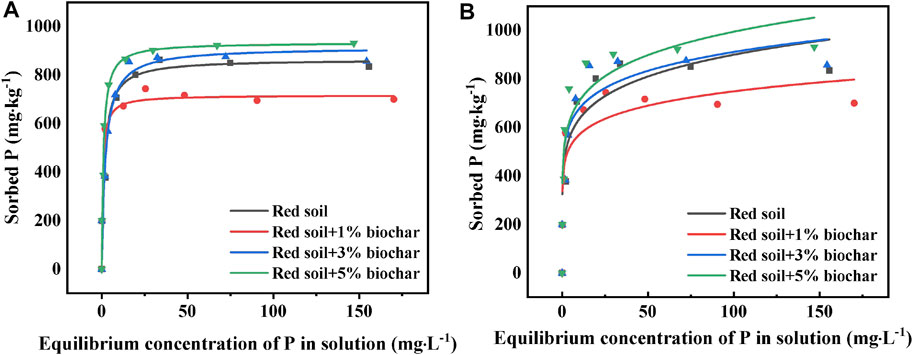
FIGURE 4. Langmuir (A) and Freundlich (B) adsorption isotherms of P by the red soils amended with the rice straw biochar at doses of 0, 1, 3, and 5% (w/w).

TABLE 2. The parameters of Freundlich and Langmuir isotherms for P absorption by the pure and biochar-amended red soils.
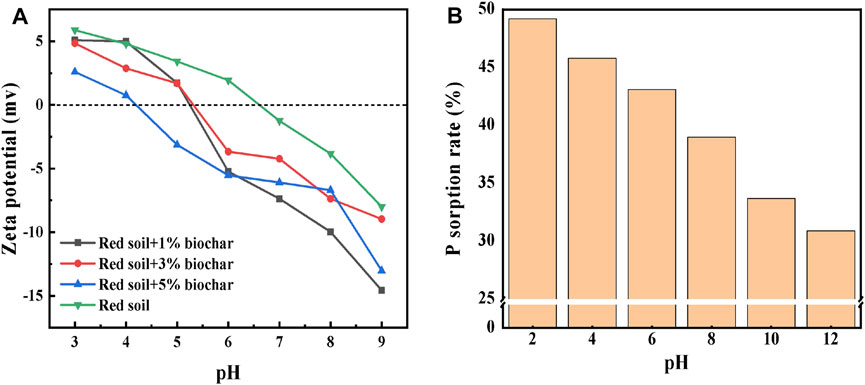
FIGURE 5. (A) Zeta potential of the rice straw biochar-amended red soil and (B) adsorption rate of P by the red soil with the increasing pH value.
On the basis of the aforementioned results, we concluded that biochar can affect the P sorption behavior by the red soil by elevating the soil pH value and releasing DBC. To further explore the specific effects of pH and DBC on P sorption, the P adsorption by the red soil at different pH values and different DBC concentrations was examined separately. The results (Figure 5) show that as the pH value of the soil increased from 2 to 12, the zeta potential of the soil samples became more negative. At an exogenous P concentration of 20 mg L−1, the P adsorption capacity of the red soil showed an obvious decreasing trend with an increasing pH value. With an increase in the aqueous solution pH, the deprotonation of the oxygenated functional groups (such as -OH and -COOH) was enhanced. Consequently, the electrostatic repulsion between the negatively charged surfaces of the soil particles and the negatively charged phosphate ions greatly hindered P adsorption, which would in turn increase the available P concentration in the soil solution.
To probe the DBC effect on P absorption in red soils, DBC was extracted from the rice straw biochar by simple water extraction. The obtained DBC solution was then used in the sorption systems to determine the phosphate competitive adsorption. As illustrated in Figure 6D, as the concentration of DBC increased from 0 to 45 mg L−1, the P adsorption capacity by the red soil decreased significantly from 513 to 411 mg kg−1 and from 812 to 698 mg kg−1 at initial P concentrations of 10 and 20 mg L−1, respectively. The results indicated that the DBC with a high number of functional groups is an effective competitor, which will result in intense oxyanion competition and hence high P concentrations in soil solution. Under the condition of large-scale use of the biochar, substantial amounts of DBC will inevitably be released into the soil environment. The resulting oxyanion competition and subsequent increase in dissolved phosphate will enhance P availability in soil. Similarly, it was clearly shown in previous research studies that the adsorption of natural DOM on mineral surfaces in soils was weakened by the presence of P, while the adsorption of P on mineral surfaces was also decreased by the presence of natural DOM (Wagner and Jaffé, 2015; Luo et al., 2019; Kong et al., 2021), implying a competitive relationship between natural DOM and P. It is noted that compared with DOM, DBC contains more O-containing functional groups (Chen et al., 2003; Luo et al., 2019). It is expected that DBC may bring about more P release from soil mineral surfaces, which remain to be explored.
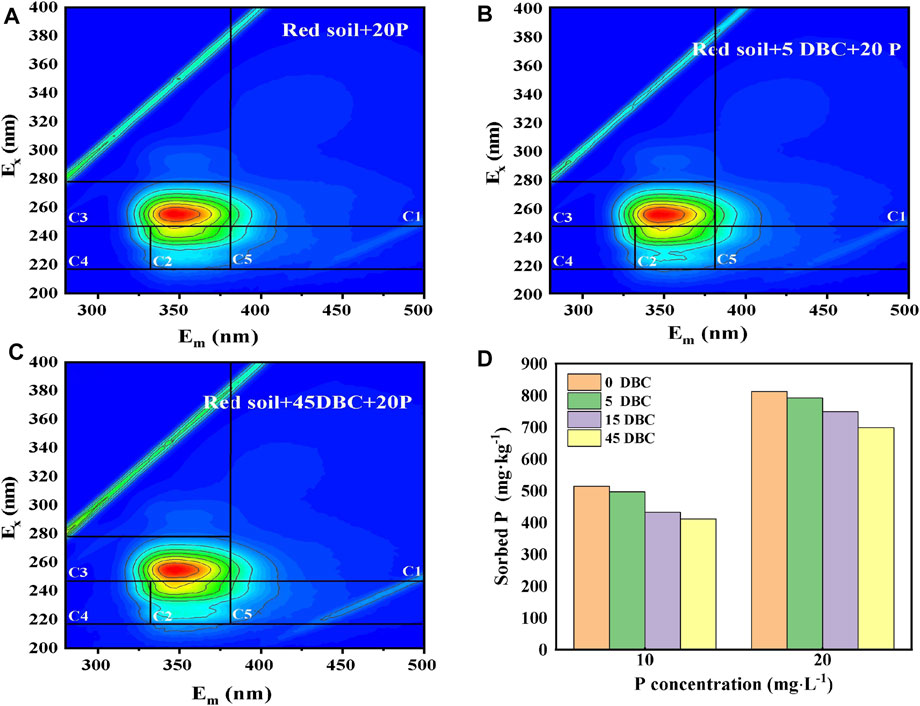
FIGURE 6. 3D-EEM of the dissolved organic matter in the phosphorus adsorption equilibrium solution by the DBC (0, 5, or 45 mg L−1)-amended red soils (A–C); P absorption capacity of the DBC-amended red soils (D).
The composition of DOM in the solution after sorption equilibrium was analyzed by 3D-EEM fluorescence spectroscopy (Fellman et al., 2010; He et al., 2014). As illustrated in Figure 6, the following five EEM components were recognized: C1 and C3 represent the low-molecular-weight humic acid-like substances in the UV region; C2 denotes the high-molecular-weight humic acid-like substance in the UV region; C4 and C5 are protein-bound and free tyrosine and tryptophan-like substances, respectively, which are collectively referred to as protein-like substances. It has been observed that the fluorescence intensities obviously changed with the increasing biochar amendment rate. The intensified fluorescence diffraction peaks in the C2 and C3 regions suggest that DBC addition can increase the content of the humic acid-like substance in the soil solution. The humic acid-like substances in DBC were provably the major components responsible for the competition with phosphate.
Conclusion
In this study, by measuring the speciation distribution of P, it is concluded that P bioavailability in the red soil was greatly related to Al and Fe minerals. After adding the rice straw biochar, the bioavailable P in the red soil was increased, while the contents of Ca-P and Fe/Al-P were reduced. The isotherm data showed that with the increase in the rate of biochar from 0 to 5%, the maximum P adsorption capacity of the red soil was first reduced and subsequently increased. The adsorption of P by the red soil was negatively related to the pH of the soil solution due to electrostatic repulsion. The electrochemical test results show that the addition of biochar will increase the pH of the red soil, shifting the IEP of the red soil in the negative direction. In addition, biochar can also weaken P adsorption in the soil by releasing DBC molecules. The P adsorption ability of the red soil was weakened with the increase of the DBC concentration. DBC is rich in humus-like substances, which will compete with phosphate for the adsorption sites on the surface of the soil mineral particle. However, since the hummus-like component is a complex mixture, the functional groups that play the decisive role in the competitive adsorption in the hummus-like component still need further research and analysis (He et al., 2014).
Data Availability Statement
The original contributions presented in the study are included in the article/Supplementary Material; further inquiries can be directed to the corresponding author.
Author Contributions
YW developed the concept and design of the work. ZZ and CH collected data. YW drafted the thesis. JJ made significant changes to the thesis.
Funding
This study was supported by the National Natural Science Foundation of China (42177204).
Conflict of Interest
The authors declare that the research was conducted in the absence of any commercial or financial relationships that could be construed as a potential conflict of interest.
Publisher’s Note
All claims expressed in this article are solely those of the authors and do not necessarily represent those of their affiliated organizations, or those of the publisher, the editors, and the reviewers. Any product that may be evaluated in this article, or claim that may be made by its manufacturer, is not guaranteed or endorsed by the publisher.
References
Antelo, J., Arce, F., Avena, M., Fiol, S., López, R., and Macías, F. (2007). Adsorption of a Soil Humic Acid at the Surface of Goethite and its Competitive Interaction with Phosphate. Geoderma 138, 12–19. doi:10.1016/j.geoderma.2006.10.011
Butterly, C. R., Bhatta Kaudal, B., Baldock, J. A., and Tang, C. (2011). Contribution of Soluble and Insoluble Fractions of Agricultural Residues to Short-Term pH Changes. Eur. J. Soil Sci. 62, 718–727. doi:10.1111/j.1365-2389.2011.01387.x
Chen, W., Westerhoff, P., Leenheer, J. A., and Booksh, K. (2003). Fluorescence Excitation−Emission Matrix Regional Integration to Quantify Spectra for Dissolved Organic Matter. Environ. Sci. Technol. 37, 5701–5710. doi:10.1021/es034354c
Daniels, W. L., and Lee, W. (2016). The Nature and Properties of Soils, 15th Edition Ray R. Weil and Nyle C. Brady. Pearson Press, Upper Saddle River NJ, 2017. 1086 P. $164.80. ISBN-10: 0-13-325448-8; ISBN-13: 978-0-13-325448-8. Also Available as eText for $67.99. Soil Sci. Soc. America J. 80, 1428. 15th edition. doi:10.2136/sssaj2016.0005br
Das, O., Bhattacharyya, D., and Sarmah, A. K. (2016). Sustainable Eco-Composites Obtained from Waste Derived Biochar: a Consideration in Performance Properties, Production Costs, and Environmental Impact. J. Clean. Prod. 129, 159–168. doi:10.1016/j.jclepro.2016.04.088
Fellman, J. B., Hood, E., and Spencer, R. G. M. (2010). Fluorescence Spectroscopy Opens New Windows into Dissolved Organic Matter Dynamics in Freshwater Ecosystems: A Review. Limnol. Oceanogr. 55, 2452–2462. doi:10.4319/lo.2010.55.6.2452
Guppy, C. N., Menzies, N. W., Moody, P. W., and Blamey, F. P. C. (2005). Competitive Sorption Reactions between Phosphorus and Organic Matter in Soil: a Review. Soil Res. 43, 189–202. doi:10.1071/sr04049
Hartono, A., Funakawa, S., and Kosaki, T. (2005). Phosphorus Sorption-Desorption Characteristics of Selected Acid Upland Soils in Indonesia. Soil Sci. Plant Nutr. 51, 787–799. doi:10.1111/j.1747-0765.2005.tb00113.x
He, X.-S., Xi, B.-D., Cui, D.-Y., Liu, Y., Tan, W.-B., Pan, H.-W., et al. (2014). Influence of Chemical and Structural Evolution of Dissolved Organic Matter on Electron Transfer Capacity during Composting. J. Hazard. Mater. 268, 256–263. doi:10.1016/j.jhazmat.2014.01.030
Helyar, K. R., and Porter, W. M. (1989). “Soil Acidification, its Measurement and the Processes Involved,” in Soil Acidity and Plant Growth, 61–101. doi:10.1016/b978-0-12-590655-5.50007-4
Hu, H. Q., Tan, C. Y., Cai, C. F., He, J. Z., and Li, X. Y. (2001). Availability and Residual Effects of Phosphate Rocks and Inorganic P Fractions in a Red Soil of central China. Nutrient Cycling in Agroecosystems 59, 251–258. doi:10.1023/A:1014454705792
Jalali, M., and Peikam, E. N. (2013). Phosphorus Sorption-Desorption Behaviour of River Bed Sediments in the Abshineh River, Hamedan, Iran, Related to Their Composition. Environ. Monit. Assess. 185, 537–552. doi:10.1007/s10661-012-2573-5
Kong, M., Han, T., Chen, M., Zhao, D., Chao, J., and Zhang, Y. (2021). High Mobilization of Phosphorus in Black-Odor River Sediments with the Increase of Temperature. Sci. Total Environ. 775, 145595. doi:10.1016/j.scitotenv.2021.145595
Luo, L., Chen, Z., Lv, J., Cheng, Y., Wu, T., and Huang, R. (2019). Molecular Understanding of Dissolved Black Carbon Sorption in Soil-Water Environment. Water Res. 154, 210–216. doi:10.1016/j.watres.2019.01.060
Morales, M. M., Comerford, N., Guerrini, I. A., Falcão, N. P. S., and Reeves, J. B. (2013). Sorption and Desorption of Phosphate on Biochar and Biochar-Soil Mixtures. Soil Use Manage 29, 306–314. doi:10.1111/sum.12047
Ruban, V., López-Sánchez, J. F., Pardo, P., Rauret, G., Muntau, H., and Quevauviller, P. (2001). Harmonized Protocol and Certified Reference Material for the Determination of Extractable Contents of Phosphorus in Freshwater Sediments - A Synthesis of Recent Works. Fresenius' J. Anal. Chem. 370, 224–228. doi:10.1007/s002160100753
Schneider, F., Haderlein, S. B., and Stefan, B. (2016). Potential Effects of Biochar on the Availability of Phosphorus - Mechanistic Insights. Geoderma 277, 83–90. doi:10.1016/j.geoderma.2016.05.007
Shepherd, J. G., Joseph, S., Sohi, S. P., and Heal, K. V. (2017). Biochar and Enhanced Phosphate Capture: Mapping Mechanisms to Functional Properties. Chemosphere 179, 57–74. doi:10.1016/j.chemosphere.2017.02.123
Smebye, A., Alling, V., Vogt, R. D., Gadmar, T. C., Mulder, J., Cornelissen, G., et al. (2016). Biochar Amendment to Soil Changes Dissolved Organic Matter Content and Composition. Chemosphere 142, 100–105. doi:10.1016/j.chemosphere.2015.04.087
Sun, J., He, F., Pan, Y., and Zhang, Z. (2016). Effects of Pyrolysis Temperature and Residence Time on Physicochemical Properties of Different Biochar Types. Acta Agriculturae Scand. Section B - Soil Plant Sci. 67, 12–22. doi:10.1080/09064710.2016.1214745
Wagner, S., and Jaffé, R. (2015). Effect of Photodegradation on Molecular Size Distribution and Quality of Dissolved Black Carbon. Org. Geochem. 86, 1–4. doi:10.1016/j.orggeochem.2015.05.005
Wagner, S., Jaffé, R., and Stubbins, A. (2018). Dissolved Black Carbon in Aquatic Ecosystems. Limnol Oceanogr Lett. 3, 168–185. doi:10.1002/lol2.10076
Weng, L., Van Riemsdijk, W. H., and Hiemstra, T. (2008). Humic Nanoparticles at the Oxide−Water Interface: Interactions with Phosphate Ion Adsorption. Environ. Sci. Technol. 42, 8747–8752. doi:10.1021/es801631d
Wang, N., Li, J.-Y., and Xu, R.-K. (2009). Use of Agricultural By-Products to Study the pH Effects in an Acid tea Garden Soil. Soil Use Manag. 25, 128–132. doi:10.1111/j.1475-2743.2009.00203.x
Wang, L., Butterly, C. R., Tian, W., Herath, H. M. S. K., Xi, Y., Zhang, J., et al. (2016). Effects of Fertilization Practices on Aluminum Fractions and Species in a Wheat Soil. J. Soils Sediments 16, 1933–1943. doi:10.1007/s11368-016-1380-y
Xu, G., Wei, L. L., Sun, J. N., Shao, H. B., and Chang, S. X. (2013). What Is More Important for Enhancing Nutrient Bioavailability with Biochar Application into a sandy Soil: Direct or Indirect Mechanism? Ecol. Eng. 52, 119–124. doi:10.1016/j.ecoleng.2012.12.091
Xu, G., Sun, J., Shao, H., and Chang, S. X. (2014). Biochar Had Effects on Phosphorus Sorption and Desorption in Three Soils with Differing Acidity. Ecol. Eng. 62, 54–60. doi:10.1016/j.ecoleng.2013.10.027
Yang, L., Wu, Y., Wang, Y., An, W., Jin, J., Sun, K., et al. (2020). Effects of Biochar Addition on the Abundance, Speciation, Availability, and Leaching Loss of Soil Phosphorus. Sci. Total Environ. 758, 143657. doi:10.1016/j.scitotenv.2020.143657
Zhang, H., Elskens, M., Chen, G., and Chou, L. (2019). Phosphate Adsorption on Hydrous Ferric Oxide (HFO) at Different Salinities and pHs. Chemosphere 225, 352–359. doi:10.1016/j.chemosphere.2019.03.068
Keywords: red soil, biochar, phosphorus, adsorption, dissolved black carbon (DBC)
Citation: Wu Y, Zou Z, Huang C and Jin J (2022) Effect of Biochar Addition on Phosphorus Adsorption Characteristics of Red Soil. Front. Environ. Sci. 10:893212. doi: 10.3389/fenvs.2022.893212
Received: 10 March 2022; Accepted: 24 March 2022;
Published: 09 May 2022.
Edited by:
Jun Zhou, University of Massachusetts Lowell, United StatesReviewed by:
Piotr Kraska, University of Life Sciences of Lublin, PolandHao Zheng, Ocean University of China, China
Copyright © 2022 Wu, Zou, Huang and Jin. This is an open-access article distributed under the terms of the Creative Commons Attribution License (CC BY). The use, distribution or reproduction in other forums is permitted, provided the original author(s) and the copyright owner(s) are credited and that the original publication in this journal is cited, in accordance with accepted academic practice. No use, distribution or reproduction is permitted which does not comply with these terms.
*Correspondence: Jie Jin, amluamllMTlAMTI2LmNvbQ==