- 1Faculty of Agricultural Sciences and Technology–Campus Dracena, Department of Plant Production, São Paulo State University (UNESP), Dracena, Brazil
- 2Faculty of Engineering, Department of Phytotechnics, Food Technology, and Partner Economics–Ilha Solteira Campus, São Paulo State University (UNESP), Ilha Solteira, Brazil
- 3Faculty of Engineering, Department of Plant Health, Rural Engineering and Soils–Campus de Ilha Solteira, São Paulo State University (UNESP), Ilha Solteira, Brazil
- 4Institute of Higher Education of Rio Verde, Faculty Objective, Rio Verde, Brazil
- 5Chapadão Foundation, Chapadão do Sul, Brazil
- 6Federal Institute of Triangulo Mineiro (IFTM), Uberaba, Brazil
- 7Faculty of Social and Agrarian Sciences of Itapeva, Itapeva, Brazil
- 8Department of Agronomy, Federal University of Technology—Paraná (UTFPR), Pato Branco, Brazil
- 9Botany and Microbiology Department, College of Science, King Saud University, Riyadh, Saudi Arabia
- 10Integrated Molecular Plant Physiology Research, Department of Biology, University of Antwerp, Antwerp, Belgium
Mechanical soil scarification and cover crops cultivation are promising tools to minimize surface soil layer compaction in no-tillage systems. The objective of the current study was to evaluate the effects of mechanical soil scarification associated with predecessor and successive growth of cover crops on grain yield and industrial quality of upland rice in a no-tillage system. The research was carried out in randomized block design in a 5 × 2 factorial scheme with four replications in a Rhodic Haplustox soil with a clayey texture. The treatments consisted of five cover crops (Cajanus cajan, Crotalaria juncea, Urochloa ruziziensis, Pennisetum glaucum and fallow) andmechanical soil scarification (without and with). The fallow and C. cajan as a cover crop in mechanical soil scarification increased number of panicles plant−1 while cultivation of C. cajan, P. glaucum and C. juncea as covers crop in without scarification increased number of grains panicle−1, total number of grains panicle−1 and filled grains. Rice milling yield was increased in mechanical soil scarification regardless of the cover crop as well as scarification, and C. cajan as covers crop has increased grain yield of upland rice.
Introduction
Rice (Oryza sativa L.) has great economic, social, and food importance due to its daily consumption habit. The annual consumption per capita of rice in Brazil varies between 25 and 50 kg inhabitant−1 year−1 (FAOSTAT, 2011). Rice grain is an excellent source of carbohydrates, proteins, minerals (mainly phosphorus, iron and calcium) and B vitamins such as B1 (thiamine), B2 (riboflavin) and B3 (niacin). Rice was cultivated on a total area of 1.7 million hectares with production of 10.6 million tons and an average yield of 6.300 kg ha−1 in 2019–2020 in Brazil (Conab, 2020). Therefore, it is important to adopt sustainable practices and proper management to ensure high quality and production of rice in tropical savannah.
Soil compaction in no-tillage system (NTS) mostly occurs in superficial soil layers due to heavy traffic of machinery and implements in the soil with high moisture content or by mobilizing soil only in sowing furrow (Carof et al., 2007; Francaviglia et al., 2015). The heavy intervention of machinery in the absence of adequate agricultural planning for cultivation of cover or successive crops contributed to the intensification of compacted surface soil layers in NTS that could disrupt soil structure with affecting soil fertility and crop productivity (Franchini et al., 2012). Soil compaction increased density and root penetration resistance, reduced total porosity, macro-porosity, and altering water and nutrients dynamics (Tokura et al., 2017). Therefore, some sustainable and environmental friendly soil management practices like mechanical scarification with subsoiler and scarifiers while biological scarification with cover crops cultivation are needed to adopt to counter the harsh effects of soil compaction in long term NTS.
Mechanical intervention is carried out by means of scarifiers or subsoilers with cutting discs in front of stems that resist crop residues to incorporate into the soil. However, long-term effects of mechanical soil scarification is altering with time limit, ranging from few months (Seidel et al., 2018; Nazari et al., 2021) to few years (Boreta Junior et al., 2021), relaying on the redisposition of soil particles as a result of soil type, weather conditions, machines and implements intervention, and predominant management practices in particular production system (Silva et al., 2017). Soil compaction has a direct on affect physical and mechanical characterizations of soil which consequently impair plant growth and development. In general, soil compaction impairs water and nutrients uptake by limiting root length and penetration that all ultimately lead to poor plant growth and yield (D’Or and Destain, 2016). Scarification breakdown compacted soil layers and generating macro-pores to improve soil permeability and facilitate root penetration and corrective movement.
Thus, management of plant crops in the no-tillage system, such as cultivation of cover crops or in intercropping systems is a promising alternative to increase plant biomass productivity and nutrient accumulation in no-tillage system (Pereira et al., 2016; Chieza et al., 2017). The plants of Poaceae family such as Pennisetum glaucum, Urochloa ruziziensis, U. brizantha and Avena sativa are fast establishing species and capable of high biomass production and nutrient cycling (Teixeira et al., 2012; Mauro et al., 2015; Pereira et al., 2016). The introduction of Fabaceae family species in production systems, alone or intercropped with Poaceae family species have the ability to increase soil nitrogen (N) availability from biological nitrogen fixation (BNF) and mineralization (Stagnari et al., 2017). In addition, cover crops are important for regulating soil sustainability, nutrients recycling and providing several benefits to the crops of economic interest.
The inclusion of cover plant species (leguminous and/or grasses) have the capability to alleviate soil compaction by acting as barrier between machinery and soil as well as increasing mineralization and nutrient recycling. In addition, natural BNF capacity of leguminous plants allow the reduction of N supply by up to 23% in the first cropping season (Adeux et al., 2021), while increasing grain yield in the absence of N fertilization in coverage (Mahama et al., 2020). The use of Poaceae species as cover crops provide longer period to cover soil with their residues due to thier high C:N ratio and organic matter mineralization (Raza et al., 2019). The combination of mechanical scarification with cover crops reduce compaction of topsoil layer, increasing root development and penetration, yield and quality of successive crops (Melkonian et al., 2017; Sarto et al., 2018; Fin et al., 2018; Fin et al. al., 2018; Adeux et al., 2021).
Considering the above discussion of maintenance and alterations in management practices in NTS and a diverse role of cover crop for successive crops, the hypothesis adopted that would mechanical soil scarification and cover crops in NTS have positive influence on grain yield and quality of rice. The objective of the study aimed to evaluate the effects of mechanical soil scarification associated with previous and successive cultivation of cover crops on grain yield and industrial quality of upland rice in tropical Cerrado of Brazil, being no-tillage system for 12 years.
Material and Methods
Local of the Experimental Area
The research was conducted in 2012/2013 and 2013/2014 growing periods in Research and Extension Farm, Sao Paulo state University (UNESP) at Selvíria, Brazil (51°22’ W, 20°22’ S, and altitude of 335 m) in a Rhodic Haplustox soil with clayey texture (EMBRAPA, 2018).
The site received an annual average rainfall, temperature and relative humidity of 1,370 mm, 23.5°C and 75% respectively. The climate of the region is classified as Aw-type according to Koppen climate classification, characterized as humid tropical with a rainy season in summer and a dry in winter. The climatic data recorded during experiments are shown in Figure 1. Irrigation was carried out by a central pivot sprinkler irrigation system at water depth of 14 mm after every 3 days or according to crop requirements.
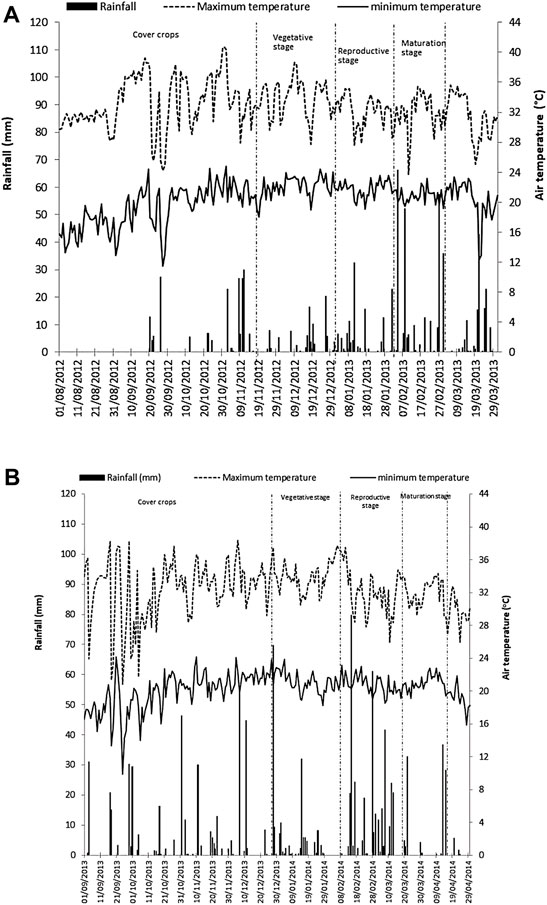
FIGURE 1. Rainfall (mm), maximum and minimum temperatures (oC) during the rice growing period of 2012/2013 (A) and 2013/2014 (B) in Selvíria, MS, Brazil.
Experimental Design and Treatments
The experiments were designed in randomized blocks in a 5 × 2 factorial scheme with four replications. The treatments were consisted of five cover crops (Cajanus cajan, Crotalaria juncea, Urochloa ruziziensis, Pennisetum glaucum and fallow) associated or not with mechanical soil scarification. The fallow treatments associated or not with mechanical soil scarification were allowed to the development of spontaneous vegetation of predominant weeds species of the region such as Ipomoea acuminata, Bidens pilosa, Leonotis nepetaefolia, Conyza spp., Commelina benghalensis and Zea mays (voluntary corn). Each experimental unit has a total area of 12.0 × 7.0 m and a useful area of 10.0 × 5.0 m.
The soil of research site consisted of 385, 120, and 495 g kg−1 of sand, silt and clay respectively with bulk density of 1.54 Mg m−3 in 0.00–0.20 m layer according to Embrapa (1997). The chemical attributes of the soil were determined before experiment installation by collecting 20 random samples in entire experimental area to form a composite sample in 0.00–0.20 m layer, presented the following results: 25 mg dm−3 of P (resin); 16 g dm−3 of OM; 4.7 pH (CaCl2); K+, Ca2+, Mg2+, H + Al, SB and CEC = 1.6, 13.5, 9.5, 35.5, 24.6, and 60.1 mmolc dm−3 respectively and 41% base saturation according to procedures of Raij et al., 1997. Based on soil analysis and recommendation of Raij et al., 1997, soil correction was carried out 35 and 123 days before cultivation of cover crops and upland rice respectively. The field site was applied with a dose of 1.6 Mg ha−1 dolomitic limestone (CaCO3) having effective neutralizing power of 85% of effective calcium carbonate equivalent (ECCE) to raise base saturation to 70%.
The mechanical soil scarification was carried out on 9th August 2012 before cultivation of cover crops. The seven-shank scarifier (three on the front bar and four on rear) and chisel tip with spacing between 300 mm, attack angle of 22° and crushing roller coupled to the tractor traction bar was used before cover crops cultivation. The average working depth was adjusted to 0.30 m and cutting swath width was adjusted to 2.10 m. The operations were performed at a soil moisture content close to friability point as well as disk harrow was carried out in chiseled plots.
All cover crops were sown on 14th August 2012 and 5th September 2013 by manual seeding machine with 0.45 m row spacing without application of mineral fertilizer. The sowing density of 60 kg ha−1 for C. cajan, 30 kg ha−1 for C. juncea, 12 kg ha−1 for U. ruziziensis and 12 kg ha−1 for P. glaucum was maintained during cultivation. These cover crops are either grasses or legumes. The cover crop C. cajan and C. juncea are a legume crop species, highly adapted to tropical Cerrado of Brazil and are capable to tolerate drought, increase soil N content by BNF and produced high straw yield with intermediate C/N ratio (Wutke et al., 2014; Silva Filho et al., 2018). In addition, U. ruziziensis and P. glaucum are among the most adaptable grasses in Brazilian Cerrado due to their high straw production, high C/N ratio as well as increasing water and nutrients recycling and protecting soil from degradation (Pacheco et al., 2013; Ferreira Junior et al., 2018; Silva Filho et al., 2018).
All cover crops and fallows were chemically desiccated at 68 days after sowing (DAS) in 2012 and at 63 DAS in 2013 with application of glyphosate + 2,4-D (1.440 + 670 g ha−1 of a.i.) by a tractor sprayer at 200 L ha−1 spray volume. The mechanical management of crop residues was carried out after 10 days of desiccation cover crops in all treatments with the help of a horizontal mechanical straw crusher at a cutting height of 0.10 m above ground level.
Rice cultivar (IAC 203) was sown on 13 November 2012 and 21 December 2013. The cultivar had modern architecture with upright and small size leaves, and resistant to lodging with a 50% flowering at 69 days and an average cycle of 112 days (Regitano Neto et al., 2013). The rice seeds were treated with pyraclostrobin + thiophanate-methyl + fipronil (5 g + 45 g + 50 g a. i. per 100 kg seeds respectively) a day before plantation. Seeds treatment is a common technique in Brazil to avoid pathogen and insect attack in tropical soils. Each plot was consisted of 20 rows of 12 m length and 0.35 m apart with 18 central rows (0.5 m discarded at both ends of each line) being considered as useful area. Fertilization in the sowing furrows and topdressing was calculated according to the chemical characteristics of the soil and recommendations of Cantarella and Furlani. (1997). The fertilization was performed in sowing furrow at the dose of 250 kg ha−1 of NPK (04-14-08) in both cultivations. Nitrogen fertilization (60 kg ha−1) in topdressing was applied 30 days after emergence (DAE) from the source of ammonium sulfate (20% N and 22% S).
Weeds were controlled with herbicides applied by tractor sprayer at a flow rate of 200 L ha−1. The pre-emergence herbicide pendimethalin (1.400 g a.i. ha−1) was applied in both seasons to control weed infestation. In addition, post-emergence herbicides metsulfuron methyl (2.0 g a.i. ha−1) and bentazon (720 g a.i. ha−1) at 15 and 13 days after emergence in 2012/2013 and 2013/2014 rice cropping seasons. The rest of the weeds were eradicated manually with help of a hoe.
A Preventive fungicide containing trifloxystrobin (75 g a.i. ha−1) + tebuconazole (150 g a.i. ha−1) was applied at 58 and 79 DAE in 2012/2013 cropping season and at 60 and 82 DAE in 2013/2014 cropping season to control rice blast disease. The other crop agronomic managements were those generally recommended for the cultivation of upland rice in the region. The crop was harvested on 4 March 2013 and 10 April 2014.
Assessments
The following evaluations recorded were: a) plant height (m)—ten random plants in central rows of each plot were measured at physiological maturity from soil surface to the upper end of the highest panicle; b) number of panicles meter−2 was calculated by counting number of panicles in 1.0 m row in the useful area of each plot and then converted into per square meter; c) total number of grains panicle−1 were calculated by counting the number of grains in 20 random collected panicles in each plot at the time of harvest; d) number of filled and empty grains per panicle were calculated by counting number of filled and empty grains in 20 panicles after their separation by air flow; e) 100-grain weight was calculated by counting two samples of a hundred random grains in each plot weighed on13% wet basis; f) grain yield was calculated by harvesting three central rows, keeping in Sun for drying, threshed and weighed by electric balance, then converting into kg ha−1 at 13% humidity; g) hectoliter mass was calculated by measuring grain mass in a specific scale of 250 g at grain moisture content of 13%; h) milling yield was calculated by collecting a sample of 100 g rice grains from each plot and processed in a test rice milling machine for 1 minute, then polished grains were weighed and the value found was considered as benefit income. Subsequently, the polished grains were placed in the rice length grader (Number 1) for 30 s to separate whole and broken grains. The grains remained in the rice length grader were weighed to obtain yield of whole and broken grains, the results were expressed in percentage.
Statistical Analysis
The data were submitted to normality test and then to analysis of variance (F test). The means of cover crops (CC) were compared by Tukey test at 5% significance. The means of mechanical soil scarification (MC) were compared by F test at 5% significance. When there was a significant interaction between sources of variation (CC and MC) then means were also compared by Tukey test at 5% significance level (Gomes and Garcia, 2002). The statistical software Sisvar version 5.6 was used for all the analysis (Ferreira, 2019).
Results
Rice seedlings in both cropping seasons were emerged uniformly on the sixth day after sowing (DAS), reached to full flowering at 82 days after emergence (DAE) and harvested at 106 DAE. There was no problem of plant lodging in both cropping seasons. The cultivation of predecessor cover crops positively influenced plant height in 2013/2014 cropping season (Table 1). The cultivation of U. ruziziensis and C. cajan as cover crops increased plant height by 4.7 and 2.8% in relation to C. juncea, which was noted with dwarf plants.
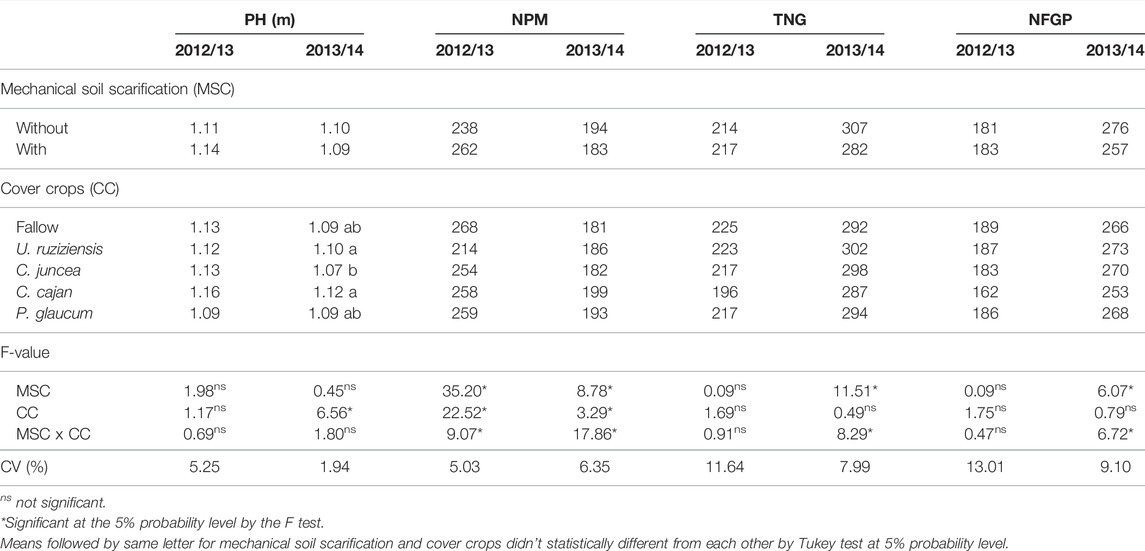
TABLE 1. Plant height (PH), number of panicles m−2 (NPM), total number of grains (TNG) and number of filled grains panicle−1 (NFGP) of upland rice after mechanical soil scarification and cover crops cultivation in 2012/2013 and 2013/2014.
The interactions of cover crops and mechanical soil scarification were significant for number of panicles m−2 in both cropping seasons while the interactions for total number grains panicle−1, number of filled grains panicle−1 and empty grains panicle−1 were significant only in 2013–2014 cropping season in no-tillage system (Table 1).
Number of panicles m−2 of rice were increased with cover crops cultivation associated or not with mechanical soil scarification in no-tillage system (NTS) in both cropping seasons (Table 1; Figures 2A,B). Highest number of panicles m−2 in 2012/2013 were observed in fallow treatments which was statistically similar within the treatments of C. cajan in association with mechanical soil scarification as compared to other treatments (Figure 2A). In addition, number of panicles m−2 in second cropping season were increased with mechanical soil scarification in the treatments incorporated with crop residues of C. cajan which were statistically at per with P. glaucum and fallow area. The lowest number of panicles m−2 were observed with residues of C. juncea in mechanical soil scarification in NTS (Figure 2B). However, treatments without scarification were observed with greater number of panicles m−2 of rice when previously cultivated with P. glaucum and C. juncea and lowest with U. ruziziensis in first cropping season (Figure 2A). In second cropping season, previous cultivation of P. glaucum produced higher number of panicles m−2 which was statistically similar with C. juncea and U. ruziziensis in non-scarifies treatments while the lowest was noted within fallow treatments (Figure 2B).
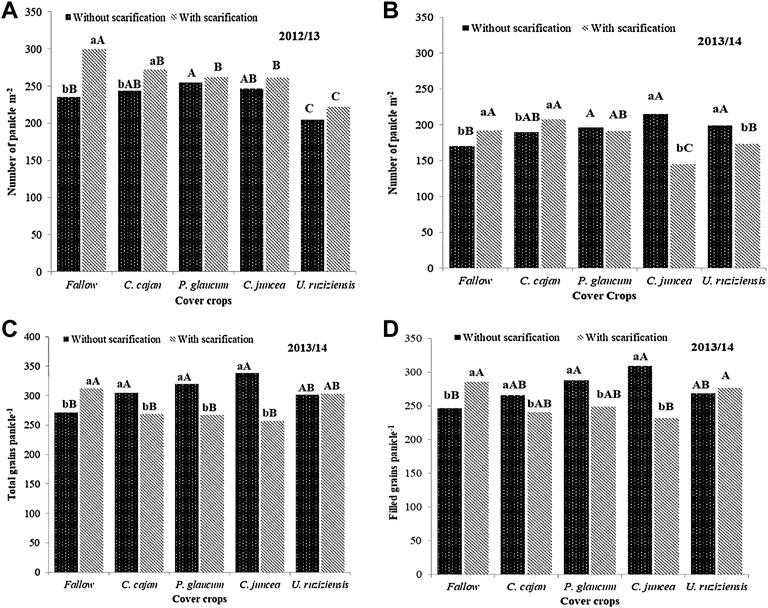
FIGURE 2. Effect of mechanical soil scarification (MS) and cover crops (CC) in no-tillage system on number of panicles m−2 of upland rice in 2012/2013 (A) and 2013/2014 (B), total number of grains panicle−1 in 2013/2014 (C) and number of filled grains panicle−1 in 2013/2014 (D). Means followed by same lowercase letter for CC within MS and uppercase for MS within CC were statistically not different by Tukey test at 5% probability.
The mechnical scarification in fallow crop treatments were oberved with greater total number of grains panicle−1 which was statistically similar with U. ruziziensis treatments while cultivation of P. glaucum, C. juncea and C. canjan as a cover crop without scarification produced greater total number of grains panicle−1 in relation to fallow in second cropping season (Figure 2C). The lowest total number of grains panicle−1 in rice were observed with cultivation of P. glaucum, C. juncea and C. canjan in scarified treatments while without scarification in fallow treatments (Figure 2C).
The number of filled grains panicle−1 in 2013/2014 were higher with residues of U. ruziziensis and fallow treatments in mechanical soil scarification. The cultivation of P. glaucum and C. juncea as cover crops in non-scarified treatments were observed with higher number of filled grains panicle−1 (Figure 2D). The lowest number of filled grains panicle−1 were observed with C. juncea in scarification and in fallow without scarification in relation to other treatments (Figure 2D).
The interaction of mechanical soil scarification and cover crops for empty grains panicle−1, 100-grain weight and hectoliter mass were significant only in 2013/2014 cropping season (Tables 2; Figure 3). The highest number of empty grains in mechanical soil scarification was observed with treatment of C. juncea as a cover crop which was statistically similar with fallow treatments. The lowest empty grains panicle−1 were observed with the effect of scarification in soils previously cultivated with P. glaucum as compared to other treatments (Figure 3A). In addition, treatments of C. canjan in non-scarified soils produced higher number of empty grains panicle−1 which was statistically not different from the treatments of P. glaucum and U. ruziziensis. The lowest empty grains panicle−1 were observed with fallow treatments in non-scarified soil (Figure 3A).
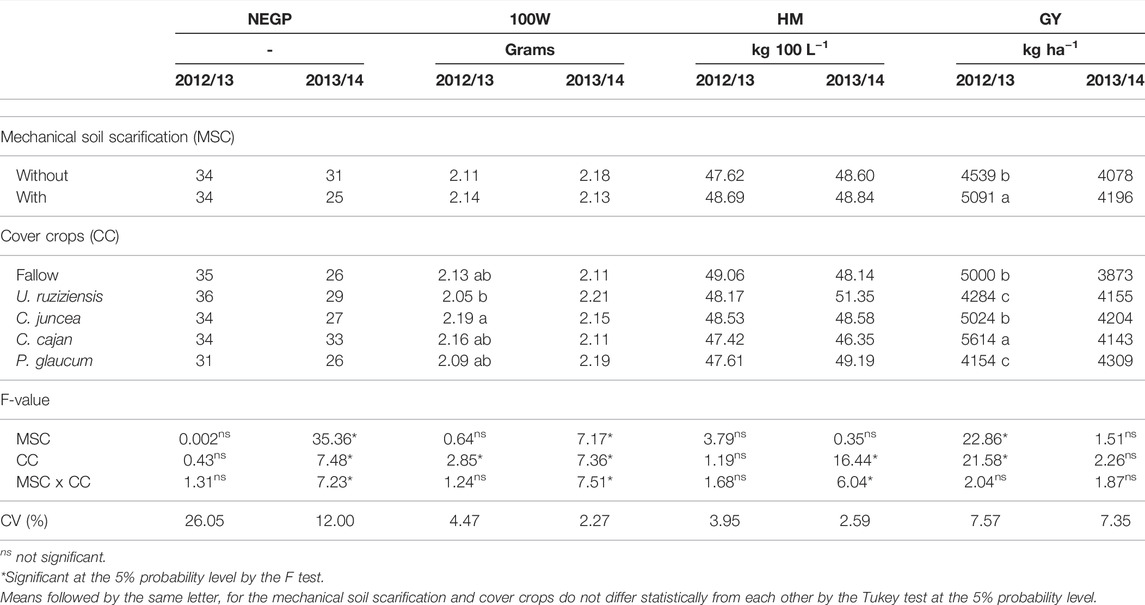
TABLE 2. Number of empty grains per panicle (NEGP), 100-grain weight (100W), hectoliter mass (HM) and grain yield (GY) of upland rice after mechanical soil scarification and cover crops cultivation in 2012/2013 and 2013/2014.
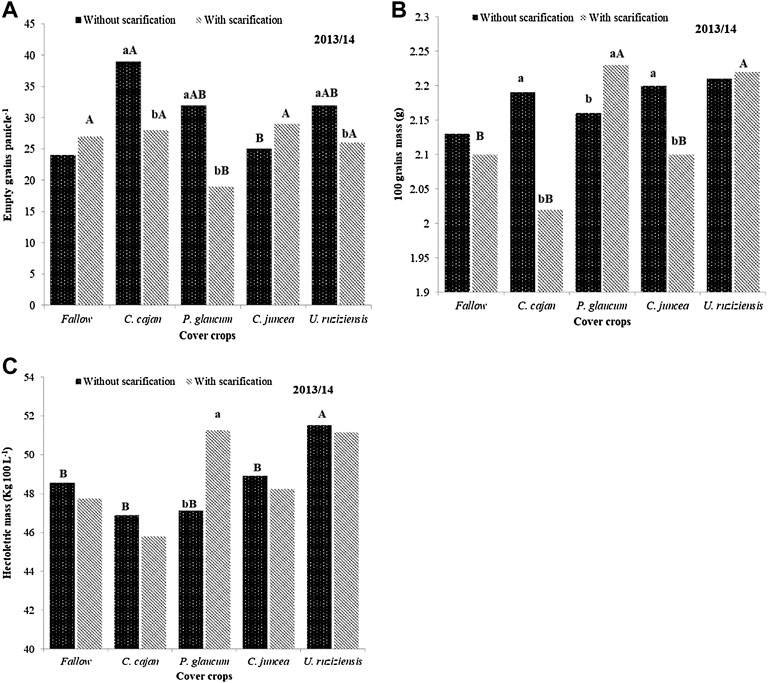
FIGURE 3. Effect of mechanical soil scarification and cover crops in the no-tillage system on tnumber of empty grains panicle−1 (A), 100-grain weight (B), and hectoliter mass (C) of upland rice in 2013/2014 cropping season. Means followed by same lowercase letter for CC within MS and uppercase for MS within CC were statistically not different by Tukey test at 5% probability.
The treatments with U. ruziziensis and P. glaucum in NTS with mechanical soil scarification had greater 100-grains weight. The lowest grains weight in mechanical soil scarification was observed with previous cultivation of C. canjan as cover crop in NTS (Figure 3B). In addition, 100-grains weight of rice was increased with C. cajan and C. juncea without mechanical soil scarification in NTS. However, without scarification, low grains weight was noted within fallow treatments (Figure 3B).
Hectoliter mass of rice grains was increased with mechanical soil scarification in previous cultivation of P. glaucum under NTS. (Figure 3C). The mechanical soil scarification within previous cultivated U. ruziziensis in NTS showed potential for increasing hectoliter mass of rice in 2013/2014 cropping season. The lowest hectoliter mass in mechanical soil scarification was observed with previous cultivation of C. canjan (Figure 3C). The non-scarification within treatments of P. glaucum as cover crop also increased hectoliter mass of rice while the lowest was noted with C. canjan as a cover crop in relation to other treatments (Figure 3C).
The management with mechanical scarification and cover crops significantly influenced grain yield of upland rice in 2012/13 cropping season while treatments effect in 2013/14 and their interactions in both seasons were not significant (Table 2). Mechanical soil scarification increased grain yield of upland rice by 12.2 and 2.9% as compared to without scarification in 2012/13 and 2013/14 respectively. In addition, previous cultivation of C. juncea and C. canjan have increased grain yield of upland rice by 12.3 and 8.5% as compared to fallow treatments in 2012/13 and 2013/14 cropping season.
Milling yield was significant only with mechanical soil scarification in 2012/13 cropping season (Table 3). The operation of mechanical soil scarification improved milling yield by 1.8% in relation to without mechanical soil scarification. The whole and broken grains yield of upland rice in both cropping seasons were not influenced by mechanical soil scarification and cover crops, and their interactions grains in the two harvests, there was a significant effect only for the milling yield in the 2012/2013 harvest (Table 3).
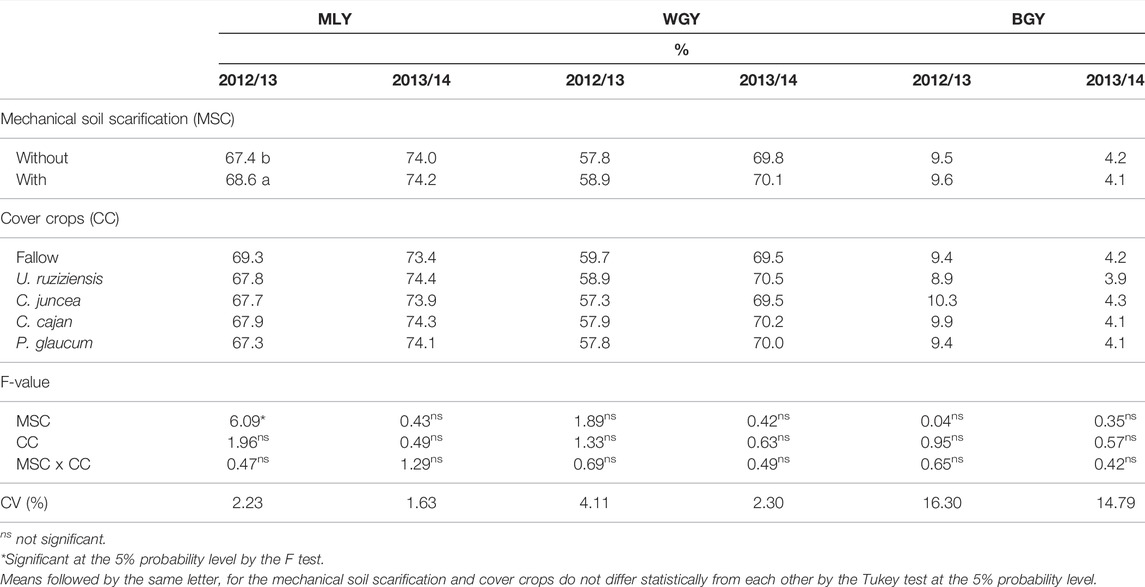
TABLE 3. Milling yield (MLY), whole grain yield (WGI) and broken grain yield (BGI) of upland rice after mechanical soil scarification and cultivation of cover crops in the harvest of 2012/2013 and 2013/2014.
Based on the results, soil management with mechanical scarification and cover crops under sprinkler irrigation are promising alternatives to develop sustainable agriculture system in NTS of low-altitude Cerrado of Brazil.
Discussion
The superficial mechanical scarification of soil provided greater environment for development of rice plants in all cover crops cultivation. This is due to the fact that mechanical scarification of soil allowed the plants to develop greater roots system (Chieza et al., 2017). Since, the current experiment was conducted in soils being under no-tillage system (NTS) for 12 years and accumulated soil particles of low porosity due to traffic heavy machines in crop cultivation, treatments application and harvesting. This high degree compaction of soil surface layer may harm soil profile by limiting penetration of tap and secondary roots in depth as well as reducing water and nutrients absorption, that all hinder growth and productivity of crops (Seki et al., 2015; Monteiro et al., 2017).
The highest number of panicles m−2, number of total grains and number of filled grains panicle−1 of upland rice in 2013/2014 cropping were observed with C. juncea and P. glaucum as cover crops without mechanical soil scarification and in fallow with mechanical soil scarification in NTS (Figure 2). Reis et al. (2017) also reported that inclusion of C. juncea as a cover crop in rice cultivation system had increased number of panicles and panicle mass in a no-tillage system without mechanical soil scarification. The incorporation of crop residues by rotary tiller in NTS has improved physical and chemical characterizations of soil, even though resulted in greater grain yield of rice due to greater organic matter retention (Tang et al., 2020). Similarly, Klein et al. (2008) indicated that number of grains spikelet−1, spikelets spike−1 and hectoliter mass were increased with mechanical soil scarification in a NTS than without mechanical soil scarification. Therefore, the present results also showed that mechanical soil scarification in the treatments with U. ruziziensis and P. glaucum as cover crops in NTS were observed with greater 100-grains mass and hectoliter mass of upland rice (Figures 3B,C). The might be due to the role of mechanical scarification in NTS that reduces soil compaction, sustaining organic matter, increasing infiltration and retention of water in deep soil layers and also increasing soil aeration (Monteiro et al., 2017).
The operation of mechanical soil scarification and cover crops influenced grain yield of upland rice in the first cropping season (Table 1). Soil management with mechanical scarification has increased grain yield of rice by 12.2% in relation to treatments without mechanical scarification. Milling yield was 1.8% higher with mechanical scarification in no-till soil as compared to non-scarified soil (Table 2). These results can be explained by the fact that mechanical soil scarification increases total porosity and macro-porosity, reduces soil density by disrupting compaction of soil surface layers (Monteiro et al., 2017). This might be due to the reduction of penetration resistance of roots to mechanical scarification of soil which improved root system (Valentine et al., 2012) with greater absorption of water and nutrients (Stagnari et al., 2017; Sarto et al., 2018). The greater efficiency of soil exploration by roots has a direct effect on water and nutrient transport from the roots to aerial part of the plants that has ultimately increasing photosynthesis and transport of photo-assimilates to grains Fin et al., 2018), leading to better grains filling. However, this effect decreases with time as traffic of heavy agricultural machinery in NTS lead to the compaction of soil due to natural reorganization of soil particles (Troccoli et al., 2015). Nunes et al. (2014) described that positive effects of mechanical scarification on physical attributes of soil and root development of corn didn’t persisted for more than 18 months. Therefore, the effect of frequently used machinery on soil can be mitigated by the intorduction of cover crops, a large amount of straw residues provided by grass species. In addition to the ability of some cover crop species to reduce surface soil compaction, they also allow to increase crop productivity (Sarto et al., 2018; Ogilvie et al., 2021).
The effects of cover crops in 2012/13 cropping season indicated that cultivation of C. cajan provided greater grain yield of upland rice than P. glaucum, U. ruziziensis and fallow treatments (Table 2). This might be due to greater decomposition potential of C. cajan that consequently release nutrients by mineralization for the crop in succession, in this case rice is successive crop. It was reported that P. glaucum straw can reduce nitrogen supply by biological N fixation and mineralization of P. glaucum as crop residue thus contribute to higher grain yield of rice (Reis et al., 2017). In other case it was indicated that U. ruziziensis was the best option of cover crop before rice cultivation however, low grain yield was due to the deficiency of water during pre-flowering stage (Pacheco et al., 2013), different from the experiment in question as it was irrigated with sprinkler irrigation system.
The integrated use of grasses as cover crops and mechanical scarification provided greater soil exploration by soybean roots which has consequently increased grain yield (Fin et al., 2018). The crop residues of C. juncea are very similar to poaceae with relatively high persistence due to high lignin content in stems that reduce soil temperature and water loss therefore, promoting root-shoot growth of crops in succession (Valentine et al., 2012; Nascimento et al., 2019). The use of U. ruziziensis as cover crop improved fertilizers use efficiency such as macronutrients and managing to provide high amounts of these nutrients for subsequent crops (Janegitz et al., 2016). In addition, Pacheco et al. (2011) reported that highest rice grain yield in no-tillage system was obtained after cultivation of P. glaucum and U. ruziziensis. The introduction of legumes as cover crops can reduce N fertilization by 23% as well as increasing transportation and accumulation into the shoot and grains of sorghum (Mahama et al., 2020; Adeux et al., 2021). Pedrinho et al. (2018) indicated that using grasses as cover crops increased grain yield and reduced N fertilization by 50 kg ha−1 while legumes as cover crop has reduced N fertilization by 100 kg ha−1. Thus, use of cover crops in agriculture system are able to reduce nitrogen fertilizers as well as increasing profitability and economy along sustainable production of rice.
Conclusion
Mechanical soil scarification and cover crops in no-tillage system has positive impact on growth, yielding components, yield and quality of upland rice in succession. The cultivation of C. cajan as a cover crop in mechanical soil scarification provided greater number of panicles m−2 of upland rice.
The mechanical soil scarification in fallow provided greater number of total grains and filled grains of upland rice. The cultivation of C. cajan as covers crop increased number of empty grains in without scarification treatments. Mechanical scarification within previous cultivation of U. ruziziensis and P. glaucum provided higher 100-grains mass whereas greater hectoliter mass was noted withot scarification in cultivation of U. ruziziensis as a cover crop.
Mechanical soil scarification and Cajanus cajan as cover crop increase grain yield and milling yield of upland rice in tropical conditions of Brazil. The grains quality of upland rice was less effected by treatments as compared to productive attributes.
Data Availability Statement
The raw data supporting the conclusions of this article will be made available by the authors, without undue reservation.
Author Contributions
VN and OA conceptualizing and supervising; VN and AJ original writing with contributions of CO, AA, and MO. MA, ES, PS, FK, MS, and MT; edited and corrected by SF, AJ, RS, and FK; sampling and data collection by OA, AA, YA, MO; formal analysis and validation by MT, CO, AA, MO, YA, and HA.
Funding
The current manuscript is the part of doctoral thesis of the first author with the FAPESP process No: 2012/05945-0. The authors extend their appreciation to the Researchers Supporting Project number (RSP-2021/219), King Saud University, Riyadh, Saudi Arabia.
Conflict of Interest
The authors declare that the research was conducted in the absence of any commercial or financial relationships that could be construed as a potential conflict of interest.
Publisher’s Note
All claims expressed in this article are solely those of the authors and do not necessarily represent those of their affiliated organizations, or those of the publisher, the editors and the reviewers. Any product that may be evaluated in this article, or claim that may be made by its manufacturer, is not guaranteed or endorsed by the publisher.
Acknowledgments
The authors extend their appreciation to the Researchers Supporting Project number (RSP-2021/219), King Saud University, Riyadh, Saudi Arabia. The authors are thankful to FAPESP and CNPq for financial aid. The authors are also acknowledged to UNESP, Faculty of Engineering, Campus of Ilha Solteira, Sao Paulo for the availability of infrastructure and human resources.
References
Adeux, G., Cordeau, S., Antichi, D., Carlesi, S., Mazzoncini, M., Munier-Jolain, N., et al. (2021). Cover Crops Promote Crop Productivity but Do Not Enhance Weed Management in Tillage-Based Cropping Systems. Eur. J. Agron. 123, 126221. doi:10.1016/j.eja.2020.126221
Barbosa Teixeira, M., Loss, A., Gervasio Pereira, M., and Pimentel, C. (2012). Decomposição e ciclagem de nutrientes dos resíduos de quatro plantas de cobertura Do solo. Idesia 30, 55–64. doi:10.4067/S0718-34292012000100007
Boreta Junior, E., Silva, A. A. P., Sens, T. M. Z. G., Colecha, K., Rampim, L., and Pott, C. A. (2021). Soil Physical Properties in Variable Levels of Soil Compaction. Res. Soc. Dev. 10 (2), e2341028686.
Cantarella, H., and Furlani, P. R. (19971997). “Arroz de sequeiro,” in Furlani AMC. Recomendações de adubação e calagem para o Estado de São Paulo. 2. Editors B. V. RAIJ, H. Cantarella, and J. A. Quaggio (Campinas: Instituto Agronômico e Fundação IAC), 285p. (Portuguese).
Carof, M., De Tourdonnet, S., Coquet, Y., Hallaire, V., and Roger-Estrade, J. (2007). Hydraulic Conductivity and Porosity under Conventional and No-Tillage and the Effect of Three Species of Cover Crop in Northern France. Soil Use Manag. 23, 230–237. doi:10.1111/j.1475-2743.2007.00085.x
Chieza, E. D., GuerraAraújo, J. G. M. E. D. S., Araújo, E. d. S., Espíndola, J. A., and Fernandes, R. C. (2017). Produção e aspectos econômicos de milho consorciado com Crotalária juncea L. em diferentes intervalos de semeadura, sob manejo orgânico. Rev. Ceres 64, 189–196. doi:10.1590/0034-737x201764020012
Conab. Companhia Nacional de Abastecimento (2020). Follow-Up of Brazilian Grain crop. Fourth Survey, Brasília, DF: Conab. Available at: http://www.conab.gov.br (Accessed Jan 3, 2020).
D’Or, D., and Destain, M. F. (2016). Risk Assessment of Soil Compaction in the Walloon Region in Belgium. Math. Geosci. 48 (1), 89–103.
EMBRAPA (2018). National Soil Research Center. Brazilian Soil Classification System. 5th ver. ed. DF. Embrapa, Brasília. Available at: https://www.embrapa.br/en/busca-de-publicacoes/-/publicacao/1094001/brazilian-soil-classification-system (Accessed Jan 25th, 2020).
FAOSTAT (2011). Food and Agriculture Data. Available at: http://www.fao.org/docrep/016/al993e/al993e00.pdf (Accessed Jan 22th, 2020).
Ferreira, D. F. (2019). Sisvar: a Computer Analysis System to Fixed Effects Split Plot Type Designs. Rev. Bras. Biom. 37 (4), 529–535. doi:10.28951/rbb.v37i4.450
Francaviglia, R., Carroni, A. M., Ruda, P., Salis, M., Bazzoffi, P., Carnevale, S., et al. (2015). Environmental Effectiveness of GAEC Cross-Compliance Standard 3.1 'Ploughing in Good Soil Moisture Conditions' and Economic Evaluation of the Competitiveness Gap for Farmers. Ital. J. Agron. 10, 695. doi:10.4081/ija.2015.695
Franchini, J. C., Debiasi, H., Balbinot Junior, A. A., Tonon, B. C., Farias, J. R. B., Oliveira, M. C. N. d., et al. (2012). Evolution of Crop Yields in Different Tillage and Cropping Systems over Two Decades in Southern Brazil. Field Crops Res. 137, 178–185. doi:10.1016/j.fcr.2012.09.003
Gomes, F. P., and Garcia, C. H. (2002). Statistics Applied to Agronomic and Forestry Experiments: Exposition with Examples and Guidelines for Using Applications. Piracicaba Fealq. 309p.
Janegitz, M. C., Souza, E. A. d., and Rosolem, C. A. (2016). Brachiaria as a Cover Crop to Improve Phosphorus Use Efficiency in a No-Till Oxisol. Rev. Bras. Ciênc. Solo 40, e0150128. doi:10.1590/18069657rbcs20150128
Klein, V. A., Vieira, M. L., Durigon, F. F., Massing, J. P., and Fávero, F. (2008). Porosidade de aeração de um Latossolo Vermelho e rendimento de trigo em plantio direto escarificado. Cienc. Rural. 38, 365–371. doi:10.1590/S0103-84782008000200011
Mahama, G. Y., Prasad, P. V. V., Roozeboom, K. L., Nippert, J. B., and Rice, C. W. (2020). Reduction of Nitrogen Fertilizer Requirements and Nitrous Oxide Emissions Using Legume Cover Crops in a No-Tillage Sorghum Production System. Sustainability 12, 4403. doi:10.3390/su12114403
Mauro, R. P., Anastasi, U., Lombardo, S., Pandino, G., Pesce, R., Alessia, R., et al. (2015). Cover Crops for Managing Weeds, Soil Chemical Fertility and Nutritional Status of Organically Grown Orange Orchard in Sicily. Ital. J. Agron. 10, 101. doi:10.4081/ija.2015.641
Melkonian, J., Poffenbarger, H. J., Mirsky, S. B., Ryan, M. R., and Moebius‐Clune, B. N. (2017). Estimating Nitrogen Mineralization from Cover Crop Mixtures Using the Precision Nitrogen Management Model. Agron. J. 109 (5), 1944–1959. doi:10.2134/agronj2016.06.0330
Monteiro, M. A. C., Zoz, A., Limede, A. C., Oliveira, C. E. d. S., and Zoz, T. (2017). Efeito Do Preparo Do Solo Com Diferentes Implementos Sobre a Resistência Do Solo À Penetração. Rev. Agric. Neotrop. 4, 63–68. doi:10.32404/rean.v4i2.1468
Nascimento, V. d., Arf, O., Alves, M. C., Souza, E. J. d., Silva, P. R. T. d., Hiroshi Kaneko, F., et al. (2019). Soil Mechanical Scarification Increases the Dry Matter Yield of Cover Crops under No-Tillage. Idesia 37 (4), 29–39. doi:10.4067/s0718-34292019000400029
Nazari, M., Eteghadipour, M., Zarebanadkouki, M., Ghorbani, M., Dippold, M. A., Bilyera, N., et al. (2021). Impacts of Logging-Associated Compaction on Forest Soils: A Meta-Analysis. Front. For. Glob. Change 4, 780074. doi:10.3389/ffgc.2021.780074
Nunes, M. R., Pauletto, E. A., Denardin, J. E., Faganello, A., Spinelli Pinto, L. F., and Scheunemann, T. (2014). Persistência dos efeitos da escarificação sobre a compactação de Nitossolo sob plantio direto em região subtropical úmida. Pesq. Agropec. Bras. 49, 531–539. doi:10.1590/S0100-204X2014000700005
Ogilvie, C. M., Ashiq, W., Vasava, H. B., and Biswas, A. (2021). Quantifying Root-Soil Interactions in Cover Crop Systems: A Review. Agriculture 11, 218. doi:10.3390/agriculture11030218
Pacheco, L. P., Barbosa, J. M., Leandro, W. M., Machado, P. L. O. D. A., Assis, R. L. D., Madari, B. E., et al. (2013). Ciclagem de nutrientes por plantas de cobertura e produtividade de soja e arroz em plantio direto. Pesq. Agropec. Bras. 48, 1228–1236. doi:10.1590/S0100-204X2013000900006
Pacheco, L. P., Barbosa, J. M., Leandro, W. M., Machado, P. L. O. D. A., Assis, R. L. D., Madari, B. E., et al. (2011). Produção e ciclagem de nutrientes por plantas de cobertura nas culturas de arroz de terras altas e de soja. Rev. Bras. Ciênc. Solo 35, 1787–1800. doi:10.1590/S0100-06832011000500033
Pedrinho, A., Mariano, E., Merloti, L. F., Danielson, R. E., and Sá, M. E. (2018). Common Bean Response to Cover Crop Straw and Topdressing Nitrogen Fertilization. Aust. J. Crop Sci. 12 (7), 1129–1138. doi:10.21475/ajcs.18.12.07.pne1062
Pereira, F. C. B. L., Mello, L. M. M. D., Pariz, C. M., Mendonça, V. Z. D., Yano, É. H., Miranda, E. E. V. D., et al. (2016). Autumn Maize Intercropped with Tropical Forages: Crop Residues, Nutrient Cycling, Subsequent Soybean and Soil Quality. Rev. Bras. Ciênc. Solo 40, 1–20. doi:10.1590/18069657rbcs20150003
Raij, B. V., Cantarella, H., Quaggio, J. A., and Furlani, A. M. C. (1997). Fertilization and Liming Recommendations for the State of São Paulo, 100. Campinas-SP: Campinas: Instituto Agronômico/Fundação IAC. (Boletim Técnico, 285.
Raza, M. A., Bin Khalid, M. H., Zhang, X., Feng, L. Y., Khan, I., Hassan, M. J., et al. (2019). Effect of Planting Patterns on Yield, Nutrient Accumulation and Distribution in Maize and Soybean under Relay Intercropping Systems. Sci. Rep. 9, 4947. doi:10.1038/s41598-019-41364-1
Regitano Neto, A., Gallo, P. B., Ramos Junior, E. U., Kiihl, T. A. M., and Azzini, L. E. (2013). IAC 203 and IAC 204: New Upland Rice Varieties for the State of São Paulo. Crop Breed. Appl. Biotechnol. 13, 367–370. doi:10.1590/S1984-70332013000400008
Reis, A. F. D. B., Almeida, R. E. M. D., Chagas Júnior, A. F., and Nascente, A. S. (2017). Effect of Cover Crops on Soil Attributes, Plant Nutrition, and Irrigated Tropical Rice Yield. Rev. Caatinga 30, 837–846. doi:10.1590/1983-21252017v30n403rc
Sarto, M. V. M., Bassegio, D., Rosolem, C. A., and Sarto, J. R. W. (2018). Safflower Root and Shoot Growth Affected by Soil Compaction. Bragantia 77 (2), 348–355. doi:10.1590/1678-4499.2017191
Seidel, E. P., Schneider, A. P. H., Sustakowski, M. C., Matté, L. M., Mottin, M. C., and Silva, J. H. (2018). Soybean Yield, Soil Porosity and Soil Penetration Resistance under Mechanical Scarification in No-Tillage System. Jas 10 (4), 268–277. doi:10.5539/jas.v10n4p268
Seki, A. S., Seki, F. G., Jasper, S. P., Silva, P. R. A., and Benez, S. H. (2015). Effects of Soil Decompaction Practices in the Area under No-Tillage System. Rev. Ciência Agronômica 46 (3), 460–468. doi:10.5935/1806-6690.20150027
Silva, M. C. C. D., Andreotti, M., Andreotti, M., Costa, N. R., Lima, C. G. D. R., and Pariz, C. M. (2017). Soil Physical Attributes and Yield of Winter Common Bean Crop under a No-Till System in the Brazilian Cerrado. Rev. Caatinga 30 (1), 155–163. doi:10.1590/1983-21252017v30n117rc
Stagnari, F., Maggio, A., Galieni, A., and Pisante, M. (2017). Multiple Benefits of Legumes for Agriculture Sustainability: an Overview. Chem. Biol. Technol. Agric. 4, 2. doi:10.1186/s40538-016-0085-1
Tang, H., Li, C., Xiao, X., Tang, W., Cheng, K., Pan, X., et al. (2020). Soil Physical and Chemical Quality as Influenced by Soil Tillage Managements under Double Cropping Rice System of Southern China. Acta Agric. Scand. Sect. B - Soil & Plant Sci. 70, 14–23. doi:10.1080/09064710.2019.1662082
Tokura, L. K., Secco, D., Júnior, L. A. Z., Siqueira, J. A. C., Santos, R. F., Martins, M. F. L., et al. (2017). Structuring Potential of Some Cover Crops and Crambe in Haplortox under No-Tillage System. Afr. J. Agric. Res. 12 (24), 2078–2086.
Troccoli, A., Maddaluno, C., Mucci, M., Russo, M., and Rinaldi, M. (2015). Is it Appropriate to Support the Farmers for Adopting Conservation Agriculture? Economic and Environmental Impact Assessment. Ital. J. Agron. 10, 169–177. doi:10.4081/ija.2015.661
Keywords: green manure, Oryza sativa L., scarifier plow, soil compaction, soil conservation management
Citation: Nascimento Vd, Arf O, Alves MC, Souza EJd, Silva PRTd, Kaneko FH, Teixeira Filho MCM, Jalal A, Oliveira CEdS, Sabundjian MT, Ferrari S, Prado EP, Lustosa Sobrinho R, Al-Hashimi A, Okla MK, Alwasel YA and AbdElgawad H (2022) Cover Crops and Mechanical Scarification in the Yield and Industrial Quality of Upland Rice. Front. Environ. Sci. 10:895993. doi: 10.3389/fenvs.2022.895993
Received: 15 March 2022; Accepted: 23 May 2022;
Published: 30 June 2022.
Edited by:
David Lopez-Carr, University of California, Santa Barbara, United StatesReviewed by:
Ilias Travlos, Agricultural University of Athens, GreeceYounes Rezaee Danesh, Urmia University, Iran
Copyright © 2022 Nascimento, Arf, Alves, Souza, Silva, Kaneko, Teixeira Filho, Jalal, Oliveira, Sabundjian, Ferrari, Prado, Lustosa Sobrinho, Al-Hashimi, Okla, Alwasel and AbdElgawad. This is an open-access article distributed under the terms of the Creative Commons Attribution License (CC BY). The use, distribution or reproduction in other forums is permitted, provided the original author(s) and the copyright owner(s) are credited and that the original publication in this journal is cited, in accordance with accepted academic practice. No use, distribution or reproduction is permitted which does not comply with these terms.
*Correspondence: Marcelo Carvalho Minhoto Teixeira Filho, bWNtLnRlaXhlaXJhLWZpbGhvQHVuZXNwLmJy
†ORCID: Renato Lustosa Sobrinho, orcid.org/0000-0003-2176-6745