Long-Term Effects of Biochar Application With Reduced Chemical Fertilizer on Paddy Soil Properties and japonica Rice Production System
- Biochar Engineering and Technology Research Center of Liaoning Province, Agronomy College, Shenyang Agricultural University, Shenyang, China
To clarify the effects of co-application of rice-straw biochar and different chemical fertilizer doses on paddy soil properties and japonica rice production in Northeast China, a located-field experiment was adopted for 5 years. The treatments included fertilization (F, no biochar, control) and rice-straw biochar (7.5 t ha−1) combined with different chemical fertilizer doses (100, 80, and 60% that of F) set as CF, CF1, and CF2, respectively. The results showed that the co-application of biochar and chemical fertilizers after 5 years reduced soil bulk density, increased soil total and capillary porosity, changed the soil solid–liquid–gas ratio (increased the liquid and air ratios and decreased the solid ratio), and increased soil macroaggregates (>0.25 mm) and aggregate stability, which enhanced the soil’s physical properties. Furthermore, the co-application improved soil pH and soil enzyme activity (urease, sucrase, and catalase) and had a significant effect on promoting SOM. The soil total N, P, and K of CF and CF1, were 23.48, %, 47.28%, and 26.59% and 9.47%, 43.98%, and 27.87% higher than those of the control, respectively. The soil available nutrients (N, P, and K) increased in the co-application treatment, shown as the nitrogen was promoted higher in the early growth of rice, while phosphorus and potassium were exhibited greater in the late growth. With the soil amendment, the co-application increased the rice plant height and dry matter accumulation, and the CF and CF1 increased yields by 5.78 and 2.41%, respectively, by increasing the rice effective panicles, grains per panicle, and seed-setting rate. The co-application of biochar and chemical fertilizers could significantly amend soil properties and reduce chemical fertilizer use to ensure rice–grain output, which has an important significance for enhancing sustainable soil and crop productivity.
Introduction
Rice is one of the major food crops in China and even the world. The rice cultivation area in northeast China (Liaoning, Jilin, and Heilongjiang Provinces) was 5.229 × 106 ha, accounting for 17.4% of the rice cultivation area in China, and the rice production center had significantly shifted to the north (Xu et al., 2021). For a long time, rice production in northeast China has made important contributions to ensuring Chinese food security. However, with increasing crop yield, the input of chemical fertilizers has become higher. According to statistics, the chemical fertilizer use in China has increased significantly since 1980, and the contribution rate of fertilizer-to-crop yield increased significantly from 1988 to 2017, but this yield-increasing trend has gradually weakened (Han et al., 2019), indicating that large chemical fertilizer inputs have reduced the sustainable productivity of soil and crops and has had a negative impact on the environment. Therefore, reducing fertilizer application and improving fertilizer utilization efficiency have become important challenges for sustainable agriculture development in China. In addition to a large amount of fertilizer input, the traditional high-intensity cultivation measures have made the once vast black soil layer thinner, soil acidification intensified, and organic matter decreased, resulting in a decreased fertilizer utilization rate and other issues that are increasingly prominent. Protecting the black soil “panda” in China has become an important strategy. Therefore, how to reduce chemical fertilizer input, improve soil fertility, and promote sustainable crop production has become a more important challenge in China.
In Northeast China, a large amount of agricultural waste such as straw was produced annually, most of which is burned or discarded. There is no doubt that straw returning is the most direct and effective way to use straw and maintain soil fertility. However, the climate is cold in Northeast China, and the straw was difficult to decompose, which often affected cultivation operations in the forthcoming year, such as transplanting, accompanied by increasing pest infestation and weeds (Zhang et al., 2019). Using biomass-carbonization technology to transform straw and other agricultural wastes into biochar could amend soil structure, improve soil physicochemical properties, and promote crop growth and yield increases, while providing a new way to solve soil degradation and crop cultivation problems (Macdonald et al., 2014; Lehmann and Joseph, 2015; Chen et al., 2019; Agbede and Adekiya, 2020). Many studies have shown that biochar could affect the nutrients (N, P, K, etc.) for crops by release (nutrients of biochar) and adsorption (nutrients of the soil and fertilizer), affecting pathways (Atkinson et al., 2010; Major et al., 2010; Alkharabsheh et al., 2021). Biochar could adsorb NH4+-N and NO3−-N in the soil, reduce the leaching loss of N, promote the transformation of soil organic nitrogen, and increase soil-available nitrogen (Lehmann et al., 2003; Bai et al., 2015). Biochar could also adsorb PO43- in the soil solution, reducing the leaching of soil-available phosphorus and the competition of Al and Fe oxide colloids in the soil for the closed-state fixation loss of available phosphorus (Laird et al., 2010; Hossain et al., 2020). The K availability in biochar is high, which could enhance the number of ions exchanged after application to the soil, thus reducing the leaching loss of potassium (Lehmann et al., 2003; Hossain et al., 2020). The co-application of biochar and chemical fertilizers could retain the nutrients in soil, thereby reducing nutrient loss and improving soil nutrients for crops (Morales et al., 2013; Cissé et al., 2021). The high ion adsorption-exchange capacity of biochar could balance the amount of anion and cation exchange in the soil, thereby improving soil fertility (Liang et al., 2006; Sun et al., 2017). In addition, the retention of soil nutrients by biochar was closely related to the improvement of the soil’s physical properties, such as increased soil porosity and water retention capacity and decreased soil bulk density, etc. (Lu et al., 2014). Co-application of biochar and chemical fertilizers could improve soil nutrients and fertilizer-use efficiency, which was considered a low-carbon cultivation technology in rice production systems (Dempster et al., 2012; Zhou et al., 2016; An et al., 2022).
For the positive effects of biochar on the soil and fertilizers, biochar is most likely to increase crop yield by improving the soil fertility that replaces some chemical fertilizer effects, leading to use of less chemical fertilizers and maintaining soil fertility in cultivation. However, for a long time, the biochar was found to play positive-going effects, and the effects combined with chemical fertilizers on crop productivity are still unclear. Therefore, we carried out a long-term (5 years) field experiment in Northeast China. The experiment aimed to study the effects of co-application of biochar and different chemical fertilizer doses on paddy soil properties and japonica rice production and explore new cultivation measures for paddy soil and rice sustainable production.
Materials and Methods
Site Description and Biochar Characterization
The experimental site was located in the suburb of Xiuyan County, Liaoning Province, China. The climate is temperate monsoon in a humid northern temperate zone, with a mean annual rainfall of 824.8 mm and a mean annual temperature of 8.5°C. The field location of the experiment has been cultivated for 5 years (2017–2021), and this study was carried out in 2021. The tested soil was paddy soil, which had been cultivated for several decades, and its basic physicochemical property is shown in Table 1. The rice variety is japonica rice (Beigeng 2).
The biochar used was produced from rice straw at a temperature of 450°C. The biochar was granular with particle size 0.2–0.4 cm, and the basic nutrient properties are shown in Table 2, provided by Liaoning Jinhefu Agricultural Technology Development Company.
Experimental Design and Performance
The experiment adopted a randomized block arrangement design, which set up four treatments: 1) chemical fertilization: the chemical fertilization application was adopted from the plant cultivator recommended by the local government, with no biochar, set as F (control); 2) co-application of biochar and chemical fertilizers: the biochar application dose was 7.5 t ha−1, and mixed chemical fertilizer doses were 100, 80, and 60% of the F treatment, set as CF, CF1, and CF2, respectively. The specific application dose is shown in Table 3. Each treatment was replicated three times. The biochar application dose was calculated according to the average biochar production ratio of the straw (straw: biochar = 3: 1, the local average output of rice straw was 22.5 t ha−1·year−1), which was equivalent to the rice straw of one season all carbonized to biochar returned in the field. Rice was planted once a year, and the co-application of biochar and chemical fertilizer was applied each year.
Each experimental plot covered an area of 30 m2. Before the experiment began, the biochar and chemical fertilizers were weighed according to the co-application dose (Table 3). Fifteen days before rice transplantation, the biochar and fertilizers were mixed evenly by a rotary tiller machine in the topsoil layer (20 cm). Rice seedlings were planted in a greenhouse and transplanted (May 21), with three seedlings per hole. During the rice growth stages, the irrigation, pest control, and other cultivation measures were the same as those of the local plant cultivator.
Soil samples were collected at key growth stages of rice (tillering stage, June 22; filling stage, September 22; and maturing stage, October 21). For each treatment plot, three random soil cores were taken at a depth of 20 cm and shipped to the laboratory. A portion of the sample was air-dried and screened by 20 mesh and 100 mesh sieves,, filled into sealed storage bottles to test the soil properties, and the other part was stored at 4°C for determination of soil enzyme.
Rice plant samples were collected at the tillering stage, filling stage, and maturity stage, with five representative plant samples selected in each plot. Then, the samples were shipped to the laboratory for the determination of growth indicators.
Experimental Method
Soil bulk density and porosity were determined by using the ring-knife method (Bao, 2000; Quesada et al., 2010), and the soil solid-, liquid-, and air-phase ratios were measured by a soil phase analyzer (DIK-1150, Japan) at the maturity stage.
The following indicators were determined using rice mature soil samples:
Determination of total soil nutrients: Soil total nitrogen content was determined by an element analyzer (Vario MACRO Cube, Elementar, Germany). The contents of total phosphorus and total potassium in the soil were determined by using a sodium hydroxide melting-molybdenum antimony colorimetric method and sodium hydroxide melting-flame photometric method, respectively (Bao, 2000). Soil organic matter (SOM) was determined by using the hydrothermal potassium dichromate oxidation-colorimetric method (Bao, 2000). Determination of soil enzyme activity: the potassium permanganate oxidation method was used to determine soil catalase activity. Soil sucrase activity was determined by 3,5-dinitrosalicylic acid colorimetry. Determination of soil urease activity was carried out by using the indophenol blue method (Lin, 2010).
The following indicators were determined using soil samples at the key growth stages of rice (tillering stage, filling stage, and maturity stage):
Using precision pH meter (HI2210, Italy), soil: distilled water (CO2 removal by boiling) = 1: 2.5, determination of soil pH (Quesada et al., 2010). Soil-available nitrogen was determined by using the alkali diffusion method, soil-available phosphorus was determined by using the sodium bicarbonate method, and soil-available potassium was determined by the ammonium acetate extraction method (Bao, 2000).
Plant height and dry matter accumulation were measured at the rice tillering stage, filling stage, and maturity stage. Dry matter accumulation after killing green at 105°C, dried at 80°C until constant weight, cooled to room temperature, and weighted.
At the mature stage of rice, the plants with uniform growth vigor were selected from each plot (five plants) and air-dried to study the yield traits, such as effective panicle number, panicle length, grain number per panicle, 1000-grain weight, biomass yield, and seed setting rate. The rice yield was actually harvested.
Soil aggregates were determined by the wet sieve method (Sun et al., 2020). The specific method was as follows: the 3-point sampling method was used to collect soil samples from each plot at the mature stage of rice, small shovels were used to remove impurities and debris on the surface of the straw, and special in situ soil samples were used to shove undisturbed soil. In the process of collection and transportation, soil disturbance should be avoided as much as possible to keep it undisturbed. After the soil was collected, 40 g air-dried soil was taken and placed in the uppermost part of the aggregate sieve (XY-100, China) and immersed in distilled water for 5 min. The instrument duration was set to be 15 min, and the power was set to be 100 r·min−1. After completion, the sieve was taken out in turn, and the aggregates on each sieve were washed into the aluminum box to obtain four aggregates with different particle sizes: large macroaggregates (>2 mm), small macroaggregates (2–0.25 mm), microaggregates (0.25–0.053 mm), and silt and clay parts (<0.053 mm), which were dried at 60°C for 48 h. Weighed and stored in bags, we calculated the proportion of different particle size aggregates in the soil; the formula is as follows.
Mean weight diameter (MWD), geometric mean diameter (GMD) (Mazurak, 1950; Van Bavel, 1950), and >0.25 mm aggregate content (R0.25) were used. The calculation formula is as follows.
where Xi is the average diameter of a certain level of aggregates. The average diameter of the aggregates at all levels in this study is large macroaggregate (2 mm), small macroaggregate (1.125 mm), microaggregate (0.1515 mm), and silt and clay components (0.053 mm), and Wi is the mass of the i-level aggregates.
where MX<0.25 is the mass of aggregates with particle size <0.25 mm and MT is the total mass of aggregates.
Data Processing and Statistical Analysis
Excel 2019 was used for preliminary data processing, SPSS 18.0 analysis software was used for variance and multiple comparison analyses and GraphPad Prism 5 software was used for drawing.
Results
Effects of Co-application of Biochar and Chemical Fertilizer on Paddy Soil Physical Properties
Soil Bulk Density
The soil bulk density of different treatments was CF<CF1<CF2<F (Figure 1). CF, CF2, and CF1 decreased by 5.61, 4.81, and 4.55%, respectively, compared with that of the control. In general, the co-application of biochar and chemical fertilizers reduced soil bulk density, and CF had the greatest effect.
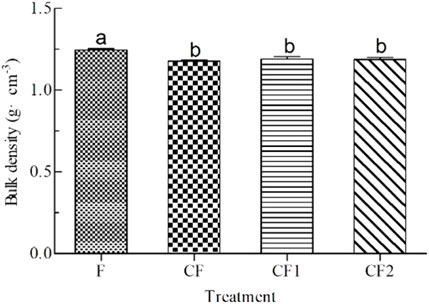
FIGURE 1. Effect of co-application of biochar and chemical fertilizer on soil bulk density. Note: the histogram in the figure represents the average value and standard deviation of each treatment; Lowercase letters represent multiple comparisons of different treatments (p < 0.05), the same below.
Soil Porosity
As shown in Figure 2, the soil total porosity showed CF2>CF1>CF>F. CF2, CF1, and CF increased by 7.49, 7.31,, and 7.18%, respectively, compared with that of the control, and the difference was significant. Among the treatments of biochar and chemical fertilizers, the soil porosity of CF2 was the highest.
Soil capillary porosity showed CF>CF1>CF2>F. CF, CF1, and CF2 significantly increased by 10.01, 9.54,, and 5.36%, respectively, with an average increase in 8.30%. Among the combined biochar and chemical fertilizer treatments, CF and CF1 had higher values than CF2, and the differences were significant.
The soil non-capillary porosity showed CF2>CF1>CF>F. CF2, CF1 and CF increased by 12.45, 2.13, and 0.61%, respectively, compared with that of the control. The difference between CF2 and the control was significant.
In conclusion, co-application of biochar and chemical fertilizers could improve soil total porosity and capillary porosity to a certain extent, improve soil aeration and permeability, and improve soil water holding capacity.
Soil Solid–Liquid–Air Phase Ratio
The soil solid ratios of CF, CF1, and CF2 were 6.98, 7.12, and 7.29% lower than those of the control, respectively, indicating that the soil solid ratio increased with the decrease of chemical fertilizer application (Figure 3). The soil liquid ratios of CF2, CF, and CF1 increased by 7.74, 7.64, and 6.57%, respectively. The soil air-phase ratio was CF1>CF2>CF>F, and the air-phase ratio of CF1 was the highest, which was 8.17% higher than that of the control.
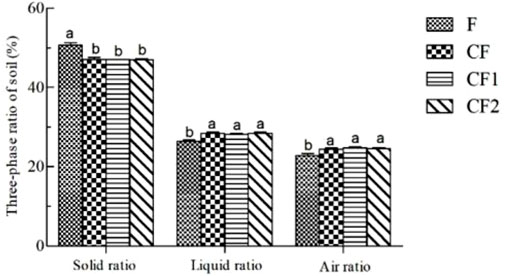
FIGURE 3. Effects of co-application of biochar and chemical fertilizer on the soil solid-liquid-air ratio.
In general, co-application of biochar and decreased chemical fertilizer changed the soil solid-, liquid- and air-phase ratios, reduced the soil solid-phase ratio, and increased the soil liquid- and air-phase ratios, indicating that the co-application of biochar and chemical fertilizer reduction could improve soil ventilation and water retention.
Soil Aggregate Distribution and Stability
Soil Aggregate Particle Size Distribution
From the soil aggregate size distribution results (Figure 4), combined biochar and chemical fertilizers increased the soil with >2 mm aggregate content; the CF and CF1 increased by 16.77 and 10.57%, respectively, compared with those of the control, and the difference was significant. For particle sizes of 2–0.25 mm, the CF, CF1, and CF2 increased by 17.45, 15.45, and 12.23%, respectively, compared with those of the control, with an average increase of 15.08%, among which CF and CF1 were significantly different from those of the control. In the 0.25–0.053 mm range, the order was CF1>CF2>CF. For <0.053 mm, the values of CF1, CF, and CF2 decreased by 20.99, 10.4, and 8.51%, respectively, compared with those of the control.
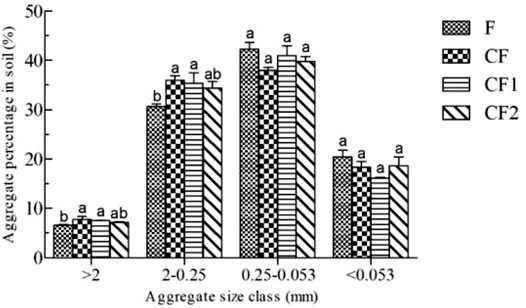
FIGURE 4. Effect of co-application of biochar and chemical fertilizer on particle size distribution of soil aggregates.
In general, biochar combined with chemical fertilizers increased the content of soil macroaggregates (>0.25 mm), and the effects of CF and CF1 were significant.
Soil Aggregate Stability
The MWD and GMD of CF, CF1, and CF2 were 14.55, %, 12.73%, and 9.09% and 14.81%, 18.52%, and 11.11% higher than those of the control, respectively (Table 4). CF and CF1 were significantly higher than those of control. The content of soil water–stable macroaggregates (R0.25) followed the order CF>CF1>CF2>F, where the CF and CF1 increased by 17.30 and 15.01%, respectively, and the difference was significant.
The aforementioned results showed that the combined biochar and chemical fertilizers could improve the stability of soil aggregates, and the effects of CF and CF1 were obvious.
Effects of Co-application of Biochar and Chemical Fertilizer on Soil Fertility and Enzyme Activities
pH
As shown in Figure 5, the soil pH of CF, CF1, and CF2 was significantly higher than that of the control at the tillering stage, filling stage, and maturity stage of rice. At the tillering stage, CF, CF1, and CF2 increased by 5.24, 4.58, and 3.93%, respectively. At the filling stage, soil pH showed CF>CF2>CF1>F, and the pH of CF, CF2, and CF1 increased by 8.23, 8.12, and 7.30%, respectively. At the maturity stage, the pH of CF, CF1, and CF2 increased by 6.93% on average compared with that of the control.
Overall, the co-application of biochar and chemical fertilizers could effectively improve the pH of paddy soil, and the difference was greater in the late growth stage than in the early growth stage of rice.
Soil Available Nutrient
As shown in Figure 6, at the tillering stage, the soil-available nitrogen content showed the order CF1>CF>CF2>F. The contents of CF1 and CF significantly increased by 18.42 and 16.77%, respectively, with an average of 17.60%. At the filling stage, the values of CF and CF1 were 13.26 and 12.79% higher than those of the control, respectively, with an average increase in 13.03%. At the maturity stage, the content of CF significantly increased by 10.14% compared with that of the control. In the key growth stages of rice, the co-application of biochar and chemical fertilizers can improve the soil-available nitrogen content, especially in the early growth stage.
At different rice growth stages, the soil-available phosphorus of the combined biochar and chemical fertilizer treatments was higher than that of the control. At the tillering stage, the order was CF>CF1>CF2>F, and the values of CF, CF1, and CF2 increased by 36.37, 31.98, and 8.79%, respectively. At the filling stage, the CF1, CF, and CF2 were 53.70, 49.50, and 5.35% higher than those of the control, respectively, with an average increase of 36.18%. At the maturity stage, the CF, CF1, and CF2 increased by 62.40, 50.90, and 16.77%, respectively, compared with those of the control, with an average increase of 43.36%. The aforementioned results indicated that combined biochar and chemical fertilizers could effectively improve the soil-available phosphorus content, especially in the late rice growth stage, and the effects of CF and CF1 were notable.
In the key growth period of rice, the soil-available potassium contents of the biochar and chemical fertilizer treatments were higher than those of the control. At the tillering stage, the order was CF>CF1>CF2>F. The CF, CF1, and CF2 increased by 28.27, 20.67, and 2.95%, respectively, compared with F, with an average increase of 17.30%. At the filling stage, the CF, CF1, and CF2 increased by 52.94, 48.87, and 41.62%, respectively, compared with those of the control, and the difference was significant. At the maturity stage, the CF, CF1, and CF2 increased by 30.07, 24.81, and 18.04%, respectively, with an average increase of 24.31%. The results showed that co-application of biochar and chemical fertilizers could increase the soil-available potassium at the key growth stages of rice, especially at the later stages.
In general, the co-application of biochar and chemical fertilizers had a significant effect on improving soil-available nitrogen, phosphorus, and potassium nutrients. The promotion of nitrogen was greater in the early stages of rice growth, and the effect of phosphorus and potassium was more obvious in the later stage.
Soil Total Nutrient
Co-application of biochar and chemical fertilizers significantly increased the soil total nitrogen content (Figure 7). The CF, CF1, and CF2 treatments increased the content by 23.48, 9.47, and 8.71%, respectively, compared with those of the control. CF was significantly different from CF1 and CF2. Combined biochar and chemical fertilizers played an important role in improving the soil total nitrogen content. The total phosphorus content of the soil followed the order CF>CF1>CF2>F. The CF, CF1, and CF2 increased by 47.28, 43.98, and 6.59%, respectively, compared with those of the control. CF and CF1 were significantly different from those of the control. Thus, biochar and chemical fertilizer treatments can significantly improve the soil total phosphorus content, and the CF and CF1 treatment effects were greater; the total potassium of the combined biochar and chemical fertilizer treatments was higher than that of the control. The CF, CF1, and CF2 increased by 26.59, 27.87, and 20.76%, respectively.
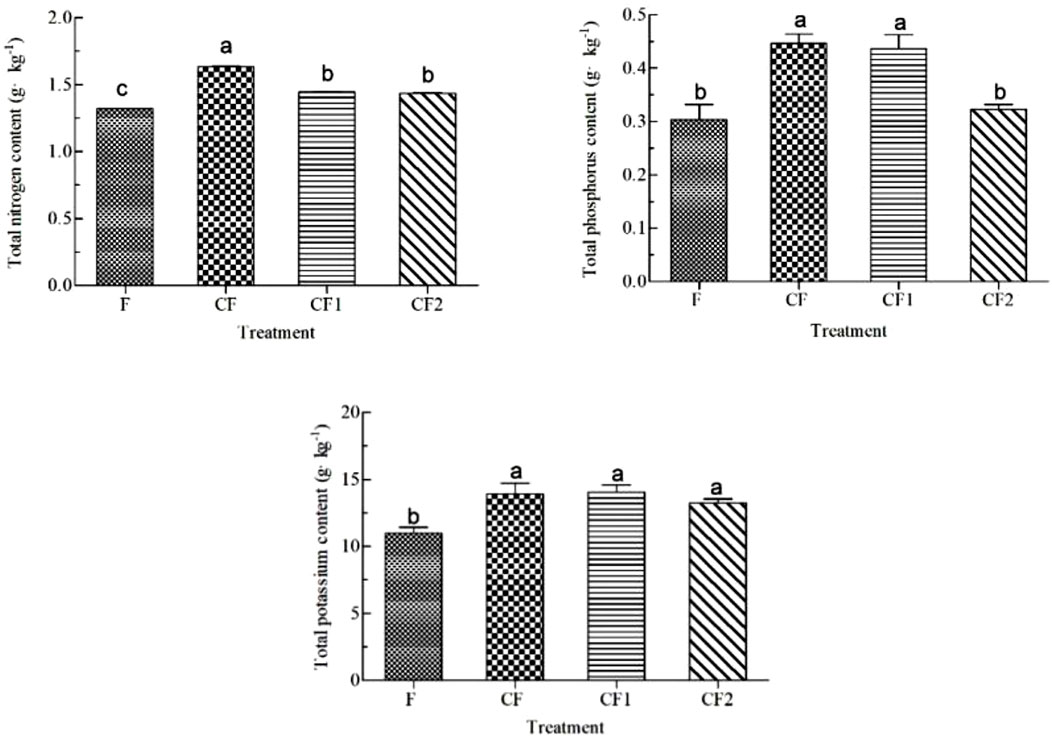
FIGURE 7. Effect of co-application of biochar and chemical fertilizer on soil total nutrient in the paddy soil.
In conclusion, the CF and CF1 significantly promoted soil total N, P, and K nutrients. This indicates that over time, biochar could slowly release its fixed nutrient elements and played a role in replacing the part of chemical fertilizers.
Soil Organic Matter
The SOM of CF, CF1, and CF2 was significantly increased by 36.55, 44.29, and 30.57%, respectively, compared with that of the control (Figure 8). There were significant differences between the biochar and chemical fertilizer treatments, with the order CF1>CF>CF2. Co-application of biochar and chemical fertilizer applications could improve the SOM.
Soil Enzyme Activity
As shown in Figure 9, soil urease showed CF>CF1>CF2>F. CF, CF1 and CF2 increased by 10.33, 9.72, and 6.64%, respectively, compared with those of the control. The soil sucrase content decreased with decreasing fertilizer application. The values of CF, CF1, and CF2 significantly increased by 3.43, 3.19, and 1.79%, respectively, compared with those of the control. The soil catalase contents of CF1, CF, and CF2 significantly increased by 27.39, 24.09, and 22.35%, respectively, compared with those of the control.
The aforementioned results indicated that the co-application of biochar and chemical fertilizers could increase soil enzyme activities (urease, sucrase, and catalase) and promote soil nutrient transformation and organic matter formation.
Effects of Co-application of Biochar and Chemical Fertilizer on Japonica Rice Growth
Plant Height
As shown in Figure 10, the plant height of rice showed the order CF>CF1>CF2>F at the tillering stage, and the CF and CF1 increased by 17.12 and 9.49%, respectively, compared with those of the control. Among the treatments, CF and CF1 were significantly different from CF2 and F, indicating that in the early stages of rice growth, biochar and chemical fertilizers (CF, CF1) had a promoting effect on rice plant height. At the filling stage, the CF, CF1, and CF2 increased by 5.56, 2.94, and 0.65%, respectively, compared with those of the control, and CF and CF1 were significantly different from those of the control. At the maturity stage, the same trend was maintained. The CF, CF1, and CF2 increased by 6.17, 3.24, and 0.97%, respectively, compared with those of the control. CF and CF1 were significantly different from CF2 and F.
In general, at the critical growth stage of rice, co-application of biochar and chemical fertilizers (CF and CF1) had a significant promotional effect on the plant height of rice.
Dry Matter Accumulation
As shown in Figure 11, at the tillering stage, the dry matter accumulation of rice followed the order CF>CF1>CF2>F, and the CF, CF1, and CF2 increased by 19.19, 5.49, and 0.79%, respectively, compared with those of the control. The difference between CF and the other treatments was significant. In the filling stage, the CF, CF1, and CF2 increased by 19.17, 5.50, and 0.78%, respectively, compared with those of the control, and the difference between CF and the other treatments was significant. At the maturity stage, the dry matter accumulation showed the order CF>CF1>F>CF2, and the CF and CF1 significantly increased by 5.04 and 3.21%, respectively, compared with those of the control. In general, co-application of biochar and chemical fertilizers promoted dry matter accumulation.
Yield and Its Components
As shown in Table 5, the effective panicle number of rice showed the order CF>CF1>F>CF2. The CF and CF1 increased by 6.95 and 5.47%, respectively, compared with those of the control, and the difference was significant. Grains per panicle of CF and CF1 significantly increased by 4.49 and 4.20%, respectively, compared with those of the control. The biomass was higher in the co-application of biochar and chemical fertilizers than in the control, and the CF and CF1 were significantly higher than those in F and CF2. The seed setting rate of CF was 1.00 and 0.35% higher than that of F and CF2, respectively, and that of CF1 was 0.79 and 0.15% higher than that of F and CF2, respectively. The yield of rice followed the order CF>CF1>F>CF2. The yields of CF and CF1 were 5.78 and 2.41% higher than those of the control, respectively, and the yield of CF2 was 1.35% lower than that of the control, but there was no significant difference. In summary, compared with the conventional fertilization treatment, the effective panicle number, grain number per panicle, 1,000-grain weight, biomass yield, and seed setting rate of rice treated with CF and CF1 increased, while there was no significant difference between CF2 and F. The seed setting rate of rice was higher in the co-application of biochar and chemical fertilizers than in the control, and the differences in CF and CF1 were significant. The yield of rice followed the order CF>CF1>F>CF2, among which the values of CF and CF1 increased by 5.78 and 2.41% compared with those of the control, respectively, and CF2 had no significant difference from the control.

TABLE 5. Effect of co-application of biochar and chemical fertilizers on rice yield and its components.
Overall, CF and CF1 promoted the effective panicle number, grain number per panicle, biomass yield, and seed setting rate, thus increasing the rice yield, while CF2 had no significant difference in the control.
Discussion
Effects of Co-application of Biochar and Chemical Fertilizer on Paddy Soil Properties
Soil physical property is an important basis that affects soil fertility and determines soil environmental conditions for crop growth. This study showed that co-application of biochar and chemical fertilizers amended the soil’s physical properties, mainly by reducing soil bulk density, improving soil porosity (total porosity and capillary porosity), reducing the ratio of the soil solid phase, and increasing the ratio of soil liquid phase and air phase. The main reason for these results was that biochar has abundant pore structure, light weight, and low density (Li et al., 2014; Wu et al., 2014). Therefore, the 5-year application of biochar reduced the soil bulk density. Moreover, the porous structure of biochar increased soil porosity by creating additional pore spaces after application (Yu and Lu., 2020). Soil porosity as gaseous and liquid materials in the soil transmission channel determined the transport form and rate of the material in soil. The effect of biochar on soil bulk density and porosity will inevitably lead to the change of the soil three-phase ratio, that is, the soil solid-phase ratio will decrease, while the ratio of air-phase and liquid-phase will increase. Due to the large specific surface area and porous structure of biochar, it has a strong adsorption capacity, improves the water holding capacity of soil (Jones et al., 2010), and increases the ratio of the liquid phase to the air phase, thereby changing the three phases of soil, improving soil aeration and water permeability, and promoting crop growth and development. The co-application of biochar and chemical fertilizers could improve the physical properties of soil water, gas, and heat, and provide good soil conditions for improving soil fertility and promoting crop growth.
Soil aggregate is an important basis for soil fertility formation, which plays an important role in the soil’s physicochemical properties and biological processes and maintaining soil property. Its stability is an important indicator of soil structure and stability (Bronick and Lal, 2004). In this study, biochar combined with different doses of chemical fertilizers could increase the content of soil macroaggregates (>0.25 mm) and improve the stability of soil aggregates. The reason was since the biochar had strong adsorption capacity, it adsorbed the surrounding soil particles or other organic matter and may gradually form soil macroaggregates with “biochar” as the core. Moreover, biochar had a rich microporous structure that not only provided habitats and breeding sites for microorganisms (Seleiman et al., 2019; Karimi et al., 2020) but also provided “energy” substances for soil microorganisms, enhancing their activities to secrete soil bio-cements and promoting the formation of soil macroaggregates. In addition, the aromatic structure of biochar made it difficult to decompose under soil physical, chemical, and microbial ways. Therefore, biochar existed stably in the soil for a long time, forming stable “biochar–soil” complexes and increasing the stability of soil aggregates (Fungo et al., 2017; Pituello et al., 2018). This study found that CF1 also significantly increased the content of soil macroaggregates because the biochar input for 5 years improved the distribution of the soil aggregates. Therefore, even if the fertilizer application rate of CF2 was lower than that of F, the content of soil macroaggregates was still significantly increased, and the improvement in soil structures promoted the performance of soil, water, and fertilizer conservation, thereby improving soil fertility.
The co-application of biochar and chemical fertilizers could effectively improve the pH of rice soil because biochar is mostly alkaline, with negatively charged phenols, carboxyl groups, and hydroxyl groups on the surface that combined with H+ in the soil solution (Chintala et al., 2014), thus reducing the concentration of hydrogen ions in the soil solution and ultimately increasing soil pH. This study showed that the difference between biochar treatment and control at the rice filling and maturity stages is greater than that at the tillering stage. This result may be primarily due to fresh biochar playing a principal role at the tillering stage. With the passage of time, biochar aged, and its surface oxygen-containing acid functional groups increased. The negative charge carried by acid functional groups neutralized H+ in the soil, further improving soil pH (Nguyen et al., 2017). At the same time, biochar after continuous input for 5 years was stable in the soil, which jointly played a role in regulating soil pH.
Soil nutrient is an important factor to measure soil fertility and determine the nutrient supply of crop growth (Reverchon et al., 2014). Co-application of biochar and chemical fertilizers not only improved the soil’s physical properties but also ensured the supply of soil nutrients to the crops. In this study, co-application of biochar and chemical fertilizers significantly promoted the soil-available N, P, and K contents. The result may be because biochar, as a new carbon source, stimulates soil microbes to mineralize biochar-labile organic compounds, which could reduce the loss of fertilizers (Singh and Cowie, 2014). The co-application of biochar and fertilizers is conducive to the retention of nutrients in the soil. The large specific surface area of biochar gives it a strong adsorption capacity, and biochar and fertilizers exist in a combined state, which increases the retention of nutrients in the soil, thus reducing the loss of nutrients in the soil (Steiner et al., 2007; Zhang et al., 2015). This study showed a great promoting effect on available N in the early stages of rice growth, while the effects on available P and K were more obvious in the later stage. This may be due to the gradual decrease of nitrogen released by biochar in the later stage. With increasing time, organic matter is adsorbed on the surface of biochar or in soil pores, making it more difficult for microorganisms to absorb as nutrients, thus decreasing the mineralization rate (Kuzyakov et al., 2009; Nguyen et al., 2017). The cation exchange capacity of biochar increased with time, increased adsorption capacity of potassium in soil, and reduced loss of potassium in the soil (Liu et al., 2021). In addition, studies have shown that biochar provides a favorable growth environment for phosphate-solubilizing microorganisms and potassium bacteria (Liu, 2016; Hossain et al., 2020; Yan et al., 2021), The pH in the later stage of rice may be more suitable for the survival of phosphate-solubilizing microorganisms and potassium bacteria, thus increasing the content of available P and K (Atkinson et al., 2010; Anderson en al., 2011). This study found that the CF2 was lower than CF and CF1 because the CF2 may increase the soil C/N ratio, thus accelerating the fixation of nitrogen by microorganisms and making the content of available nitrogen in the soil lower than that of CF and CF1.
Co-application of biochar and chemical fertilizers could improve soil enzyme activity (urease, sucrase, and catalase activity) and promote soil nutrient transformation and organic matter formation. This may be because, on the one hand, the biochar adsorption reaction substrate helps the enzymatic reaction, thus improving soil enzyme activity. On the other hand, biochar adsorbs enzyme molecules to protect the binding sites of enzymatic reactions and prevent enzymatic reactions (Lehmann et al., 2011). Studies have shown that the co-application of biochar and fertilizer could effectively improve the activities of soil urease and catalase, and it is considered that the available carbon sources contained in biochar and the nitrogen in the fertilizer jointly accelerate the reproduction of microorganisms, thereby improving enzyme activity (Meng et al., 2018). In this study, CF and CF1 significantly increased soil enzyme activity, which may be due to CF and CF1 improving soil fertility and promoting soil microbial activities, thereby increasing the soil enzyme activity due to their interaction. However, because many factors affect the soil enzyme activity and the annual changes are complex, the effects of biochar and chemical fertilizers on the soil enzyme activity need to be further explored.
Organic matter is the main indicator reflecting soil fertility. This study found that the co-application of biochar and chemical fertilizers could significantly increase the SOM. Because biochar is rich in carbon, including the organic carbon formed by biomass after carbonization, it could increase soil organic carbon after being added into the soil and effectively adsorb active organic substances in the soil to play a protective role, thereby reducing the mineralization of SOM and improving the SOM and soil carbon storage (Hua et al., 2010). Biochar input to the soil would change the C/N ratio of soil and provide rich carbon and nitrogen sources for soil microorganisms, thus providing nutrients and energy sources for microbial growth, promoting the survival and reproduction of soil microorganisms, and changing the structure of the soil microbial community. The vigorous growth and metabolism of microorganisms would promote the formation of SOM and increase the SOM (Atkinson et al., 2010; Anderson en al., 2011). In contrast to straw and other biomass amendments, biochar has a stable structure and properties and strong decomposition resistance to the physical, chemical, and microbial pathways and can exist in soil for a long time. Therefore, biochar could stabilize and improve SOM levels, thereby improving soil fertility. The treatment order is CF1>CF>CF2, and this is because the continuous co-application of biochar and chemical fertilizers may change the community structure of soil microorganisms, and the co-application of chemical fertilizers and biochar (CF1) may be more suitable for the growth of microorganisms related to decomposition and mineralization, thus accelerating the formation of SOM.
Effects of Co-application of Biochar and Chemical Fertilizer on Rice Growth
The growth and yield of rice are important indicators that reflect changes in soil properties. This study showed that the combination of biochar and chemical fertilizers could significantly increase the plant height and dry matter accumulation of rice, which was closely related to the improvement in soil physical and chemical properties. On the one hand, the improvement in soil physical properties by biochar provided a good soil environment for the growth and development of rice roots. Biochar reduced soil bulk density, improved the soil’s three-phase ratio, and increased soil porosity to improve the extension of rice roots. The alkaline effect of biochar creates a more favorable root-zone environment by reducing the effects of soil acidity and associated toxicity (Delhaize and Ryan, 1995; Van Zwieten et al., 2010), thereby expanding the range of nutrient absorption and utilization and providing the required nutrients for rice growth as much as possible. On the other hand, biochar application improves the chemical properties of paddy soil. Biochar itself contains abundant nutrient elements that can be absorbed and utilized by rice and that can be released slowly with the growth process of rice to supplement the nutrients needed for rice growth of the different growth stages. The porous structure, large specific surface area, and rich surface functional groups of biochar give it a strong nutrient absorption capacity and reduce nutrient leaching. Biochar improves soil moisture and pH, stimulates soil mineralization, and improves the availability of nutrient elements in soil (Saarnio et al., 2013), thereby improving soil nutrient use efficiency. In this study, CF and CF1 had obvious effects on improving the plant height of rice because the two treatments substantially improved soil fertility, thereby promoting the growth of rice.
The rice yield and yield components showed that co-application of biochar and chemical fertilizers (CF and CF1) could increase the number of effective panicles and grains per panicle and increase rice yield by increasing the 1,000-grain weight, biomass yield, and seed setting rate. The fundamental reason is that the application of biochar improves the growth environment of paddy soil in terms of water, fertilizer, gas, and heat, resulting in conditions more conducive to the growth of rice roots and the absorption and transportation of nutrients, thereby increasing the biomass accumulation of rice and increasing the yield. There was no significant difference between CF2 and F, indicating that under continuous cropping for 5 years, the effect of 40% reduced chemical fertilizer on soil fertility was not significantly different from that of the control, indicating that biochar could replace some of the chemical fertilizers used in rice production under long-term cumulative effects to use less chemical fertilizers and maintain the soil fertilizers in cultivation.
Co-application of biochar and chemical fertilizers could improve soil physical properties (water, gas, and heat) and soil fertility (nitrogen, phosphorus, potassium, and soil organic matter), which provides a new method for soil fertility preservation and fertilization. We also noticed that the effects of CF and CF1 with biochar and chemical fertilizers on rice growth and yield were relatively obvious, which further indicated that combined biochar and chemical fertilizers had a good application value and potential in promoting rice growth and increasing the yield and proved a new cultivation measure by using less chemical fertilizers, meanwhile amending the paddy soil properties a rice-sustainable production in Northeast China.
Conclusion
Over the long term, rice straw recycling use for biochar and co-application with different chemical fertilizer doses on paddy soil did not reduce the japonica rice yield, meanwhile amending soil properties (reduced bulk density, increased porosity, soil macroaggregates and aggregate stability, soil enzyme activity, soil nutrients (N, P, and K), and SOM). It contributed a priming sight for new cultivation by using less chemical fertilizer to guarantee yield output and soil sustainable productivity, which has important significance for soil fertility increasing and rice green-production.
Data Availability Statement
The original contributions presented in the study are included in the article/Supplementary Material; further inquiries can be directed to the corresponding author.
Author Contributions
WG: Data curation, Writing—original draft. YW: Validation, Investigation. ZF, DW, HZ, HY, YS, and LX: Investigation. WC: Obtain research funding, guiding research and resources WZ: Writing—review and editing, Funding acquisition. All authors contributed to manuscript revision, read and approved the submitted version.
Funding
This research was funded by the Strategic Priority Research Program of the Chinese Academy of Sciences (XDA28090300), the State Key Special Program of Biochar-based Fertilizer Development and Application Technology for Soil Fertility Improvement in Rice (2016YFD0300904-4), and the State Key Special Program of Biochar-Fertilizer Technology Research and Industrialization Demonstration (2017YFD0200802-02).
Conflict of Interest
The authors declare that the research was conducted in the absence of any commercial or financial relationships that could be construed as a potential conflict of interest.
Publisher’s Note
All claims expressed in this article are solely those of the authors and do not necessarily represent those of their affiliated organizations, or those of the publisher, the editors, and the reviewers. Any product that may be evaluated in this article, or claim that may be made by its manufacturer, is not guaranteed or endorsed by the publisher.
References
Agbede, T. M., and Adekiya, A. O. (2020). Influence of Biochar on Soil Physicochemical Properties, Erosion Potential, and Maize (Zea mays L.) Grain Yield under Sandy Soil Condition. Commun. Soil Sci. Plant Analysis 51 (20), 2559–2568. doi:10.1080/00103624.2020.1845348
Alkharabsheh, H. M., Seleiman, M. F., Battaglia, M. L., Shami, A., Jalal, R. S., Alhammad, B. A., et al. (2021). Biochar and its Broad Impacts in Soil Quality and Fertility, Nutrient Leaching and Crop Productivity: A Review. Agronomy 11 (5), 993. doi:10.3390/agronomy11050993
An, N., Zhang, L., Liu, Y., Shen, S., Li, N., Wu, Z., et al. (2022). Biochar Application with Reduced Chemical Fertilizers Improves Soil Pore Structure and Rice Productivity. Chemosphere 298, 134304. doi:10.1016/j.chemosphere.2022.134304
Anderson, C. R., Condron, L. M., Clough, T. J., Fiers, M., Stewart, A., Hill, R. A., et al. (2011). Biochar Induced Soil Microbial Community Change: Implications for Biogeochemical Cycling of Carbon, Nitrogen and Phosphorus. Pedobiologia 54, 309–320. doi:10.1016/j.pedobi.2011.07.005
Atkinson, C. J., Fitzgerald, J. D., and Hipps, N. A. (2010). Potential Mechanisms for Achieving Agricultural Benefits from Biochar Application to Temperate Soils: a Review. Plant Soil 337, 1–18. doi:10.1007/s11104-010-0464-5
Bai, S. H., Reverchon, F., Xu, C.-Y., Xu, Z., Blumfield, T. J., Zhao, H., et al. (2015). Wood Biochar Increases Nitrogen Retention in Field Settings Mainly through Abiotic Processes. Soil Biol. Biochem. 90, 232–240. doi:10.1016/j.soilbio.2015.08.007
Bronick, C. J., and Lal, R. (2004). Soil Structure and Management: A Review. Geoderma 124 (1), 3–22. doi:10.1016/j.geoderma.2004.03.005
Chen, W., Meng, J., Han, X., Lan, Y., and Zhang, W. (2019). Past, Present, and Future of Biochar. Biochar 1 (1), 75–87. doi:10.1007/s42773-019-00008-3
Chintala, R., Schumacher, T. E., Kumar, S., Malo, D. D., Rice, J. A., Bleakley, B., et al. (2014). Molecular Characterization of Biochars and Their Influence on Microbiological Properties of Soil. J. Hazard. Mater. 279, 244–256. doi:10.1016/j.jhazmat.2014.06.074
Cissé, D., Cornelis, J. T., Traoré, M., Saba, F., Coulibaly, K., Lefebvre, D., et al. (2021). Co‐Composted Biochar to Decrease Fertilization Rates in Cotton-Maize Rotation in Burkina Faso. Agron. J. 113, 5516–5526. doi:10.1002/agj2.20867
Delhaize, E., and Ryan, P. R. (1995). Aluminum Toxicity and Tolerance in Plants. Plant Physiol. 107 (2), 315–321. doi:10.1104/pp.107.2.315
Dempster, D. N., Jones, D. L., and Murphy, D. V. (2012). Organic Nitrogen Mineralisation in Two Contrasting Agro-Ecosystems Is Unchanged by Biochar Addition. Soil Biol. Biochem. 48, 47–50. doi:10.1016/j.soilbio.2012.01.013
Fungo, B., Lehmann, J., Kalbitz, K., Thionģo, M., Okeyo, I., Tenywa, M., et al. (2017). Aggregate Size Distribution in a Biochar-Amended Tropical Ultisol under Conventional Hand-Hoe Tillage. Soil Tillage Res. 165, 190–197. doi:10.1016/j.still.2016.08.012
Han, T. F., Ma, C. B., Huang, J., Liu, K. L., Xue, Y. D., Li, D. C., et al. (2019). Variation in Rice Yield Response to Fertilization in China: Meta-Analysis. J. Sci. Agric. Sin. 52 (11), 1918–1929. doi:10.3864/j.issn.0578-1752.2019.11.007
Hossain, M. Z., Bahar, M. M., Sarkar, B., Donne, S. W., Ok, Y. S., Palansooriya, K. N., et al. (2020). Biochar and its Importance on Nutrient Dynamics in Soil and Plant. Biochar 2, 379–420. doi:10.1007/s42773-020-00065-z
Hua, L., Zhang, C., Ma, H. R., and Yu, W. (2010). Environmental Benefits of Biochar Made by Agricultural Straw when Applied to Soil. Ecol. Environ. Sci. 19 (10), 2489–2492. doi:10.3969/j.issn.1674-5906.2010.10.040
Jones, B. E. H., Haynes, R. J., and Phillips, I. R. (2010). Effect of Amendment of Bauxite Processing Sand with Organic Materials on its Chemical, Physical and Microbial Properties. J. Environ. Manag. 91 (11), 2281–2288. doi:10.1016/j.jenvman.2010.06.013
Karimi, A., Moezzi, A., Chorom, M., and Enayatizamir, N. (2020). Application of Biochar Changed the Status of Nutrients and Biological Activity in a Calcareous Soil. J. Soil Sci. Plant Nutr. 20, 450–459. doi:10.1007/s42729-019-00129-5
Kuzyakov, Y., Subbotina, I., Chen, H., Bogomolova, I., and Xu, X. (2009). Black Carbon Decomposition and Incorporation into Soil Microbial Biomass Estimated by 14C Labeling. Soil Biol. Biochem. 41 (2), 210–219. doi:10.1016/j.soilbio.2008.10.016
Laird, D., Fleming, P., Wang, B., Horton, R., and Karlen, D. (2010). Biochar Impact on Nutrient Leaching from a Midwestern Agricultural Soil. Geoderma 158 (3/4), 436–442. doi:10.1016/j.geoderma.2010.05.012
Lehmann, J., da Silva, J. P., Steiner, C., Nehls, T., Zech, W., and Glaser, B. (2003). Nutrient Availability and Leaching in an Archaeological Anthrosol and a Ferralsol of the Central Amazon Basin: Fertilizer, Manure and Charcoal Amendments. J. Plant Soil 249, 343–357. Available at: https://www.jstor.org/stable/24123751. doi:10.1023/a:1022833116184
Lehmann, J., and Joseph, S. (2015). Biochar for Environmental Management: Science, Technology and Implementation. London and New York: Taylor & Francis. doi:10.4324/9780203762264
Lehmann, J., Rillig, M. C., Thies, J., Masiello, C. A., Hockaday, W. C., and Crowley, D. (2011). Biochar Effects on Soil Biota - A Review. J. Soil Biol. Biochem. 43, 1812–1836. doi:10.1016/j.soilbio.2011.04.022
Li, C. Y., Qu, Z. Y., Gou, M. M., Su, Y. L., and Huo, X. (2014). Effects of Biochar Amendment on Soil Water and Nutrient Utilization Efficiencies and Tomato Growth. J. J. Agro-Environment Sci. 33 (11), 2187–2193. doi:10.11654/jaes.2014.11.017
Liang, B., Lehmann, J., Solomon, D., Kinyangi, J., Grossman, J., O'Neill, B., et al. (2006). Black Carbon Increases Cation Exchange Capacity in Soils. Soil Sci. Soc. Am. J. 70 (5), 1719–1730. doi:10.2136/sssaj2005.0383
Lin, X. G. (2010). Principles and Methods of Soil Microbiology Research. Beijing: M. High Education Press.
Liu, J., Jiang, B., Shen, J., Zhu, X., Yi, W., Li, Y., et al. (2021). Contrasting Effects of Straw and Straw-Derived Biochar Applications on Soil Carbon Accumulation and Nitrogen Use Efficiency in Double-Rice Cropping Systems. Agric. Ecosyst. Environ. 311, 107286. doi:10.1016/j.agee.2020.107286
Liu, S. N. (2016). Effect of Biochar on Soil Microflora Associated with Phosphorus and Potassium. Shenyang, China: D. Shenyang Agricultural University.
Lu, S.-G., Sun, F.-F., and Zong, Y.-T. (2014). Effect of Rice Husk Biochar and Coal Fly Ash on Some Physical Properties of Expansive Clayey Soil (Vertisol). Catena 114, 37–44. doi:10.1016/j.catena.2013.10.014
Macdonald, L. M., Farrell, M., Zwieten, L. V., and Krull, E. S. (2014). Plant Growth Responses to Biochar Addition: an Australian Soils Perspective. Biol. Fert. Soils 50 (7), 1035–1045. doi:10.1007/s00374-014-0921-z
Major, J., Rondon, M., Molina, D., Riha, S. J., and Lehmann, J. (2010). Maize Yield and Nutrition during 4 Years after Biochar Application to a Colombian Savanna Oxisol. Plant Soil 333, 117–128. doi:10.1007/s11104-010-0327-0
Mazurak, A. P. (1950). Effect of Gaseous Phase on Water-Stable Synthetic Aggregates. Soil Sci. 69 (2), 135–148. doi:10.1097/00010694-195002000-00005
Meng, F. H., Gao, J. L., Yu, X. F., Wang, Z. G., Hu, S. P., Qing, G. E., et al. (2018). Inprovent of Biochemical Property of Surface Soil by Combined Application of Biochar with Nitrogen Fertilizer. J. Plant Nutr. Fertilizers 24 (5), 1214–1226. doi:10.11674/zwyf.18154
Morales, M. M., Comerford, N., Guerrini, I. A., Falcão, N. P. S., and Reeves, J. B. (2013). Sorption and Desorption of Phosphate on Biochar and Biochar-Soil Mixtures. Soil Use Manage 29 (3), 306–314. doi:10.1111/sum.12047
Nguyen, T. T. N., Xu, C.-Y., Tahmasbian, I., Che, R., Xu, Z., Zhou, X., et al. (2017). Effects of Biochar on Soil Available Inorganic Nitrogen: a Review and Meta-Analysis. Geoderma 288, 79–96. doi:10.1016/j.geoderma.2016.11.004
Pituello, C., Dal Ferro, N., Francioso, O., Simonetti, G., Berti, A., Piccoli, I., et al. (2018). Effects of Biochar on the Dynamics of Aggregate Stability in Clay and Sandy Loam Soils. Eur. J. Soil Sci. 69 (5), 827–842. doi:10.1111/ejss.12676
Quesada, C. A., Lloyd, J., Schwarz, M., Patiño, S., Baker, T. R., Czimczik, C., et al. (2010). Variations in Chemical and Physical Properties of Amazon Forest Soils in Relation to Their Genesis. Biogeosciences 7, 1515–1541. doi:10.5194/bg-7-1515-2010
Reverchon, F., Flicker, R. C., Yang, H., Yan, G., Xu, Z., Chen, C., et al. (2014). Changes in δ15N in a Soil-Plant System under Different Biochar Feedstocks and Application Rates. Biol. Fertil. Soils 50 (2), 275–283. doi:10.1007/s00374-013-0850-2
Saarnio, S., Heimonen, K., and Kettunen, R. (2013). Biochar Addition Indirectly Affects N2O Emissions via Soil Moisture and Plant N Uptake. Soil Biol. Biochem. 58, 99–106. doi:10.1016/j.soilbio.2012.10.035
Seleiman, M. F., Refay, Y., Al-Suhaibani, N., Al-Ashkar, I., El-Hendawy, S., and Hafez, E. M. (2019). Integrative Effects of Rice-Straw Biochar and Silicon on Oil and Seed Quality, Yield and Physiological Traits of Helianthus Annuus L. Grown under Water Deficit Stress. Agronomy 9 (10), 637. doi:10.3390/agronomy9100637
Singh, B. P., and Cowie, A. L. (2014). Long-Term Influence of Biochar on Native Organic Carbon Mineralisation in a Low-Carbon Clayey Soil. Sci. Rep. 4, 3687. doi:10.1038/srep03687
Steiner, C., Teixeira, W. G., Lehmann, J., Nehls, T., de Macêdo, J. L. V., Blum, W. E. H., et al. (2007). Long Term Effects of Manure, Charcoal and Mineral Fertilization on Crop Production and Fertility on a Highly Weathered Central Amazonian Upland Soil. Plant Soil 291, 275–290. Available at: https://www.jstor.org/stable/24125543. doi:10.1007/s11104-007-9193-9
Sun, H., Lu, H., Chu, L., Shao, H., and Shi, W. (2017). Biochar Applied with Appropriate Rates Can Reduce N Leaching, Keep N Retention and Not Increase NH3 Volatilization in a Coastal Saline Soil. Sci. Total Environ. 575, 820–825. doi:10.1016/j.scitotenv.2016.09.137
Sun, Q., Meng, J., Sarkar, B., Lan, Y., Lin, L., Li, H., et al. (2020). Long-Term Influence of Maize Stover and its Derived Biochar on Soil Structure and Organo-Mineral Complexes in Northeast China. Environ. Sci. Pollut. Res. 27 (22), 28374–28383. doi:10.1007/s11356-020-08171-y
Van Bavel, C. H. M. (1950). Mean Weight-Diameter of Soil Aggregates as a Statistical Index of Aggregation. Soil Sci. Soc. Am. J. 14 (C), 20–23. doi:10.2136/SSSAJ1950.036159950014000C0005X
Van Zwieten, L., Kimber, S., Morris, S., Chan, K. Y., Downie, A., Rust, J., et al. (2010). Effects of Biochar from Slow Pyrolysis of Papermill Waste on Agronomic Performance and Soil Fertility. Plant Soil 327, 235–246. doi:10.1007/s11104-009-0050-x
Wu, Y., Xu, G., and Lv, Y. C. (2014). Effects of Biochar Amendment on Soil Physical and Chemical Properties: Current Status and Knowledge Gaps. J. Adv. Earth Sci. 29 (1), 68–79. doi:10.11867/j.issn.1001-8166.2014.01-0068
Xu, C. C., Ji, L., Chen, Z. D., and Fang, F. P. (2021). Historical Review and Prospect of China’s Rice Production, Market and Import and Export Trade. J. China Rice. 27 (4), 17–21. doi:10.3969/j.issn.1006-8082.2021.04.005
Yan, T., Xue, J., Zhou, Z., and Wu, Y. (2021). Effects of Biochar-Based Fertilizer on Soil Bacterial Network Structure in a Karst Mountainous Area. Catena 206, 105535. doi:10.1016/j.catena.2021.105535
Yu, X., and Lu, S. (2020). Double Effects of Biochar in Affecting the Macropore System of Paddy Soils Identified by High-Resolution X-Ray Tomography. Sci. Total Environ. 720, 137690. doi:10.1016/j.scitotenv.2020.137690
Zhang, W. M., Chen, W. F., Meng, J., Jin, L., Guo, W., and Zhao, H. L. (2019). Study of Straw-Biochar on Utilization Potential, Industry Model and Developing Strategy in Northeast China. J. Scientia Agricultura Sinica 52 (14), 2406–2424. doi:10.3864/j.issn.0578-1752.2019.14.003
Keywords: biochar, paddy soil, chemical fertilizer, rice, productivity
Citation: Gu W, Wang Y, Feng Z, Wu D, Zhang H, Yuan H, Sun Y, Xiu L, Chen W and Zhang W (2022) Long-Term Effects of Biochar Application With Reduced Chemical Fertilizer on Paddy Soil Properties and japonica Rice Production System. Front. Environ. Sci. 10:902752. doi: 10.3389/fenvs.2022.902752
Received: 23 March 2022; Accepted: 11 May 2022;
Published: 27 June 2022.
Edited by:
Yuxue Liu, Zhejiang Academy of Agricultural Sciences, ChinaReviewed by:
Rongjun Bian, Nanjing Agricultural University, ChinaFei Lian, Hebei University of Technology, China
Copyright © 2022 Gu, Wang, Feng, Wu, Zhang, Yuan, Sun, Xiu, Chen and Zhang. This is an open-access article distributed under the terms of the Creative Commons Attribution License (CC BY). The use, distribution or reproduction in other forums is permitted, provided the original author(s) and the copyright owner(s) are credited and that the original publication in this journal is cited, in accordance with accepted academic practice. No use, distribution or reproduction is permitted which does not comply with these terms.
*Correspondence: Weiming Zhang, biochar_zwm@syau.edu.cn