- College of Grassland Agriculture, Northwest A&F University, Yangling, China
Soil microorganisms play a key role in soil fertility. Exploring the microbial community composition and diversity in response to cover crops is important for improving soil fertility in orchards. This study investigated how cover cropping can improve soil fertility by altering microbial community composition and the interrelations among soil microorganisms. Soil physicochemical and biological indicators and microbial community composition were evaluated after a 6-years application of cover cropping in an apple (Malus pumila cv. Fuji) orchard located on the Loess Plateau, China. Three treatments were applied: Trifolium repens treatment (TR), Lolium perenne treatment (LP), clear tillage treatment (CT). The soil of the cover crop treatments had a more complex microbial co-occurrence network than that of the clear tillage treatment; in particular, the correlations among fungi were significantly increased. Cover cropping increased the levels of soil organic matter (SOM), microbial biomass carbon (MBC), and total nitrogen (TN) in orchard soil. Changes in the fungal community were more related to microbial biomass nitrogen (MBN), TN, and carbon-nitrogen ratio (C:N). This indicates that cover cropping not only increases the diversity of soil microorganisms but also increases the interrelations between microbial groups, thereby improving the soil fertility of apple orchards on the Loess Plateau.
Introduction
The semi-arid Loess Plateau in northwestern China is one of the most fragile agricultural ecosystems in the world. It is severely affected by soil erosion and a lack of soil organic carbon. It is also one of the world’s largest apple-producing areas; large-scale apple cultivation has started in the 1980s (Wang et al., 2019). In 2020, the apple production in Shaanxi Province reached 11.85 million tons, accounting for 1/3 of China’s total apple production (National Bureau of Statistics of China, 2020).
In recent years, cover cropping has been widely adopted as a modern soil management system. In orchards, cover cropping can improve the soil nutrient status, increase enzyme activities, and enhance the structure and functional diversity of soil microbial communities (Snapp and Surapur, 2018; Wang et al., 2020a), thus improving soil health (Wang et al., 2020b). Several studies have shown that planting legume and non-legume cover crops on cultivated soil can improve the physical and chemical properties of the soil, such as increasing soil organic matter levels and the potential for nutrient mineralization (Hwang et al., 2015; Khan et al., 2020). Different cover crops may change the availability of C and N, thereby affecting the functions of soil microbial communities and ecosystems, consequently affecting plant productivity (Chavarria et al., 2016).
White clover (Trifolium repens) and ryegrass (Lolium perenne) are common cover crops on the Loess Plateau and have been used in local orchard production systems for decades, indicating that they have adapted to the climatic and soil conditions of the Loess Plateau (Qian et al., 2015). Soil organic matter is an important component of the soil and can be used as an indicator of soil fertility. Maintaining a high SOM content is an important condition to obtain high fruit yields (Marquez-Garcia et al., 2013; Nieto et al., 2013; Vicente-Vicente et al., 2017; Wang et al., 2020c). Legumes, as nitrogen-fixing plants, play an important role in the contribution of N to the soil (Poeplau and Don, 2015). In addition, the C: N ratio in legume residues is low, accelerating decomposition and resulting in rapid increases in SOM (Wang et al., 2020b). In contrast, the C:N ratio in gramineous residues is high, along with high cellulose and hemicellulose contents in the biomass, resulting in impeded decomposition of gramineous plants and, consequently, in soil carbon accumulation. In addition, the dense fibrous root system is conducive to increasing soil stability (Zhu et al., 2016; Wang et al., 2020a).
Soil microorganisms play a key role in ecosystem functions by promoting the decomposition of SOM and the biogeochemical cycling of nutrients, thereby promoting plant growth, symbiosis, and pathogenic interaction with plants (Bardgett and van der Putten, 2014), thus playing an important role in the productivity and sustainability of agricultural ecosystems (Banerjee et al., 2019). Soil microbial biomass is also the precursor and builder of SOM (Miltner et al., 2012). Microbial parameters are used as indicators of soil fertility under different soil management practices (Balota and Auler, 2011), and microbial properties seem to be more sensitive compared to soil physicochemical properties, especially in the early evaluation of different cultivation management practices (Zagal et al., 2009). Although several studies based on high-throughput sequence analysis have found that cover cropping can change the structure of soil bacterial communities (Brennan and Acosta-Martinez, 2017; Zhang et al., 2017; Wang et al., 2020a), few studies have focused on the specific effects of different cover crops, such as those of legume and non-legume cover crops on soil microbial communities (Zheng et al., 2018b; Wang et al., 2020c). Co-occurrence network analysis can contribute to our understanding of the complexity of microbial communities and how this complexity changes with different environments (Banerjee et al., 2019) and provide insights into the interaction of microbial communities with the environment, promote our understanding of how the metabolism of bacteria and fungi is shaped by environmental properties (Zheng et al., 2018b; Lax et al., 2019). In addition, keystone species are highly connected taxa that play an important role in the microbial community, and their absence will lead to significant changes in the composition and function of the microbiome (Herren and McMahon, 2018). Regardless of their abundance in space and time, the keystone microbial taxa are highly correlated and have a significant impact on the structure and function of the microbiome (Banerjee et al., 2018). Zheng et al. (2018b) investigated the responses of the soil microbial networks in the whole soil and different soil aggregate fractions to organic material inputs in a cover cropped apple orchard based on network correlation analysis among microorganisms. However, it is still largely unclear whether long-term cover cropping has unintended consequences for microbial co-occurrence networks in semi-arid apple orchards on the Loess Plateau.
In this study, we investigated the responses of the soil microbial networks, microbial community, diversity structure to different cover cropping systems in a semi-arid apple orchard. This study analyzed the structure of the soil bacterial and fungal communities via 16S rDNA and ITS2 high-throughput sequencing, the objectives were: 1) to reveal the effect of long-term cover cropping on the physicochemical and biological properties of apple orchard soil, 2) to investigate whether cover cropping has changed the composition of soil bacterial and fungal communities, 3) to explore the responses of soil bacteria-fungi interaction networks to cover cropping.
Materials and Methods
Site Characteristics and Experimental Design
The experiment is located at the Apple Experimental Station of Northwest A&F University (109°56′E, 35°21′N; elevation of 850 m) in Luochuan County, Shaanxi Province, China. The annual frost-free period is 165 days, and the annual average rainfall is 577 mm. The soil in this apple orchard is silt loam (87% silt, 8% clay, and 4% sand) and is classified as Haplustalfs based on the United States Department of Agriculture textural classification system.
The experiment was established in a Fuji apple (Malus pumila cv. Fuji) orchard in 2015. Apple trees were planted in 2006 on M.26 (rootstock) with a density of 2,500 plants ha−1. The experiment was established using a randomized complete block design with three replications. In total, nine plots of two rows each were used, with 12 apple trees per row. The surface area of each replicate was approximately 48 m2. In total three treatments were applied: 1) Trifolium repens treatment (TR). For TR, Trifolium repens was sown in each inter-row of apple trees at a depth of 1.5 cm and a sowing rate of 6.0 kg per hectare of the orchard. 2) Lolium perenne treatment (LP). For the LP treatment, Lolium perenne seeds were sown in a 3 m strip between tree rows, at the rate of 7.5 kg per hectare. The TR and LP cover crops were mowed three times per year (July, August, and September), and plant residues were left on the soil surface as mulch. 3) For the clear tillage treatment (CT), no cover crop was planted and weeds were controlled manually.
Soil Sampling
In mid-April 2021, three 0–15 cm deep soil cores were randomly collected from each plot using soil augers. The soil cores were well mixed and combined into a single sample, which yielded 18 soil samples in total (3 treatments × 6 replicates). After removing stones and plant residues, each soil sample was divided into two parts: one was placed in a 4°C refrigerator to determine the physicochemical and biological properties of the soil; the other was stored in a −80°C refrigerator and sent for DNA extraction.
Physicochemical and Biological Analysis
Microbial biomass carbon (MBC) and microbial biomass nitrogen (MBN) were determined by the CHCl3-K2SO4 extraction method (Jansson and Persson, 1982). Total carbon (TC) was measured by the NaOH-Na4P2O7 extraction method. Total nitrogen (TN) content was determined with the Kjeldahl method (Storvick, 1950). Ammonium nitrogen (AN), nitrate nitrogen (NN), available phosphorus (AP), and available potassium (AK) were quantified by the alkali-hydrolyzed reduction diffusing method. SOM was determined by K2CrO7–H2SO4 oxidation method (Xiong et al., 2008).
Microbial Community Analysis
The total DNA was extracted with E. Z.N.A. Soil DNA Kit (Omega, United States), the DNA was quantified by UV spectrophotometer. 341F (5′-CCTACGGGNGGCWGCAG-3′) and 805R (5′GACTACHVGGGTATCTAATCC-3′) primers were used to PCR amplify the 16S rDNA (V3+V4) variable region. ITS1 (5′-GTGARTCATCGAATCTTTG-3′) and ITS2 (5′-TCCTCCGCTTATTGATATGC-3′) (Karlsson et al., 2014) were used to PCR amplify variable regions of fungi (ITS2). The PCR amplification products were detected by 2% agarose gel electrophoresis, and the AxyPrep PCR Cleanup Kit was used for recovery. The PCR products were purifyied by AMPure XT beads (Beckman Coulter Genomics, United States) and quantified by Qubit (lnvitrogen, United States). The amplicon pools were prepared for sequencing and the size and quantity of the amplicon library were assessed on Agilent 2,100 Bioanalyzer (Agilent, United States) and with the Library Quantification Kit for Illumina (Kapa Biosciences, United States), respectively. Sequencing was performed using NovaSeq PE250 platform. Using QIIME2 (Caporaso et al., 2010) for sequence comparison and species annotation. SILVA (bacteria) and UNITE (fungi) databases were used to annotate taxonomic information.
Statistical and Network Analysis
ANOVA analysis was performed using IBM SPSS 26.0, where data were log-transformed if they did not satisfy the requirements. Principal Co-ordinates Analysis (PCoA) was conducted with the “vegan” package in R v.4.0.5. QIIME2 was used to analyze the beta diversity index of different samples. R 4.0.5 was used for graph drawing. Spearman correlation was used to analyze environmental factors and microbial communities, and the ranking and significance of environmental factors on microbial effects were obtained. Species with significant differences were analyzed by Linear discriminant analysis Effect Size (LEfSe) (Segata et al., 2011). The analysis of the interaction relationship between soil microorganisms based on the random matrix theory of molecular ecology network model (Molecular Ecological Network Analysis Pipeline, MENAP) is completed on the MENAP website (http://ieg4.rccc.ou.edu/mena) (Zhou et al., 2011). Cytoscape (3.7.2) software was used to visualize the network property parameters obtained by MENAP analysis.
Results
Changes in Soil Physicochemical and Biological Properties Under Cover Cropping
Soil physicochemical and biological properties between cover cropping and clear tillage treatment had changed siginificantly after long-term trial. (Table 1). Compared to CT treatment, TR and LP treatments significantly increased the levels of soil TC, SOM, and moisture (p < 0.05). In the LP treatment, compared to CT treatment, TC, SOM, and moisture levels increased by 10.96, 28.77, and 35.72%, respectively, whereas in TR, TC, SOM, MBC, and moisture increased by 26.45, 71.14, 57.99, and 65.21%, respectively. However, cover cropping reduced the AP levels. In addition, TR significantly increased the levels of AN, and AK (p < 0.05), and LP significantly improved the soil C: N ratio (p < 0.05).
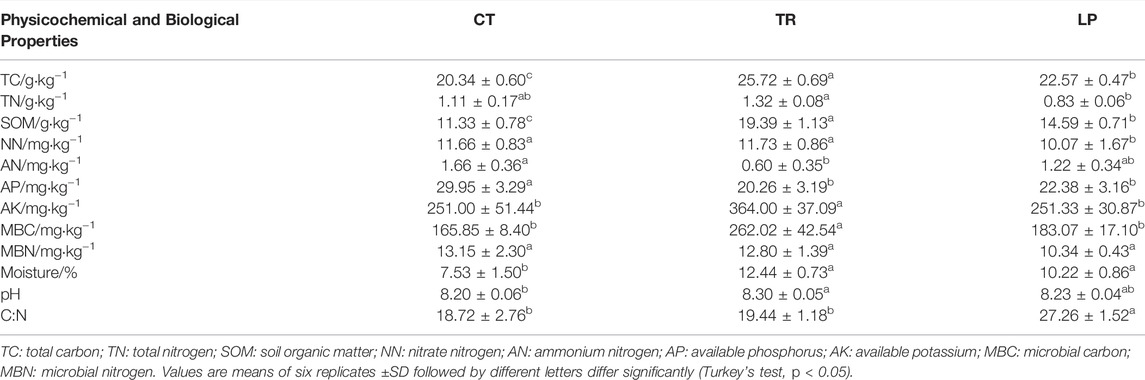
TABLE 1. Soil physicochemical and biological properties under Trifolium repens treatment (TR), Lolium perenne treatment (LP), and Clear tillage treatment (CT) in the apple orchard.
Effects of Cover Cropping on Microbial Community Composition
A total of 1,306,909 high-quality 16S rDNA sequences and 1,434,317 high-quality ITS2 sequences were obtained from 18 soil samples. The most dominant bacterial phylum was Proteobacteria, which accounted for 35.47% of the total sequences, followed by Acidobacteria, Actinobacteria, and Gemmatimonadetes, which accounted for 20.57, 11.44, and 7.70% of the total sequences, respectively. Regarding soil fungi, the main phylum was Ascomycota, which accounted for 45.48% of the total sequences, followed by Basidiomycota and Zygomycota, which accounted for 22.60 and 17.49% of the total sequences, respectively (Figure 1).
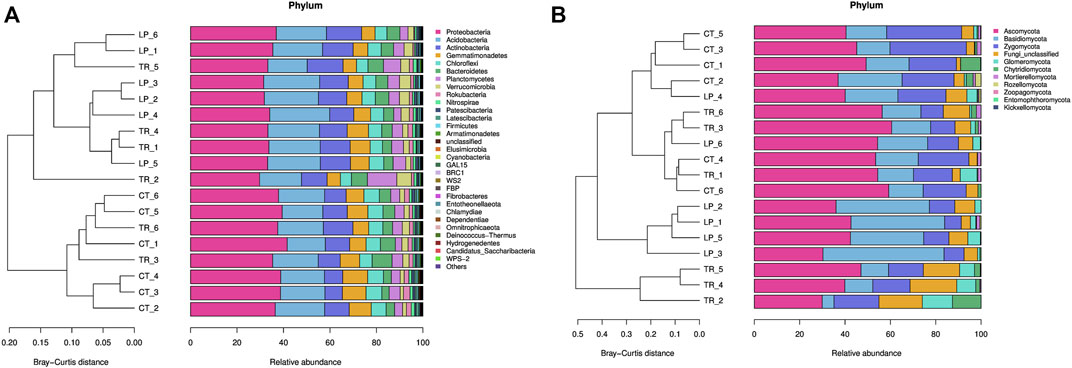
FIGURE 1. Cluster and taxonomic composition of soil bacterial (A) and fungal (B) communities at the levels of phylum.
Analysis of top 10 phyla in terms of relative abundance of the bacterial and fungi composition (Figure 2) showed that after 6 years of cover cropping, the relative abundance of Actinobacteria increased significantly in TR and LP treatments (p < 0.05). Moreover, the relative abundance of Acidobacteria in LP treatment increased significantly (p < 0.05). In contrast, compared with the CT treatment, LP and TR treatments reduced the relative abundances of Proteobacteria and Chloroflexi. In addition, TR treatment increased the relative abundance of Glomeromycota, and LP treatment significantly increased the relative abundance of Basidiomycota (p < 0.05). However, compared with the CT treatment, LP and TR treatments significantly reduced the relative abundance of Zygomycota.
Effects of Cover Cropping on Microbial Community Diversity
The linear discriminant analysis (LDA) effect size (LEfSe) method was used to detect groups or species with significant differences among the different treatments. As shown in Figure 3, after long-term cover cropping, numerous specific species were identified from different treatments. 30 bacteria clades and 35 fungi clades exhibited statistically significant differences among the different cover cropping treatments. For bacterial communities, LP treatment significantly increased the relative abundance of Acidobacteria (p < 0.05), whereas TR treatment significantly increased the relative abundance of Sphingomonadales. Regarding soil fungi, LP treatment increased the relative abundance of Basidiomycota, and TR treatment that of Glomeromycota.
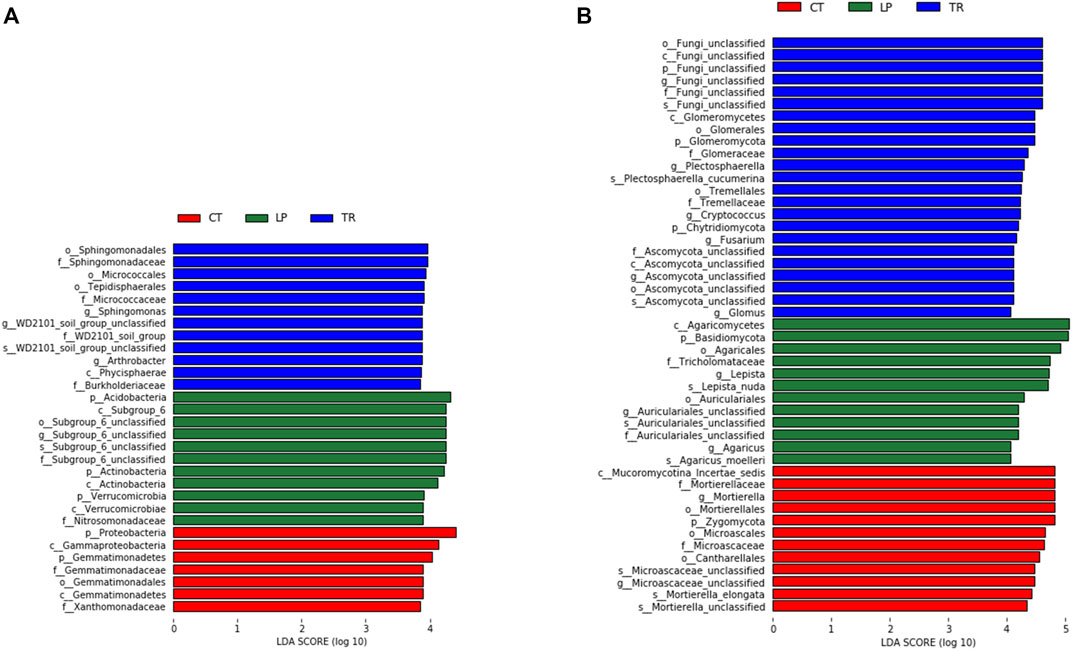
FIGURE 3. The Linear discriminant analysis (LDA) Effect Size (LEfSe) analysis was used to identify bacteria (A) and fungi (B) that respond significantly to cover crop treatment.
Principal coordinate analysis (PCoA) of the community structure of soil bacteria revealed that the LP and CT treatments formed distinct clusters in the plotted ordination space (Figure 4). Analysis of soil fungi showed that the points of TR treatment were separated from those of the other two treatments, whereas CT and LP partially over-lapped. The differences between the three treatments can be basically explained by the PCoA1 and PCoA2 axes. Furthermore, we evaluated the differences in soil microbial β-diversity under CT, LP, and TR treatments based on the weighted Unifrac distance. Compared with CT, TR increased the β-diversity of soil bacteria.
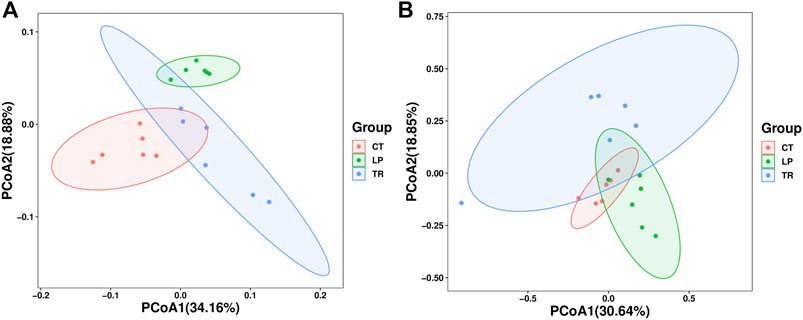
FIGURE 4. β-diversity of bacteria (A) and fungi (B). PCoA of Bray-Curtis distance with each sample colored according to the treatment group. PCoA1 and PCoA2 represent the top two principal coordinates that captured most of the diversity. The fraction of diversity captured by the coordinate is given as a percentage.
The Spearman correlation analysis of soil microorganisms and environmental factors (Figure 5) showed that SOM, TC, and AN mainly affected the composition of microorganisms at the phylum level. Bacterial community composition was more affected by SOM and TC, whereas the fungal community was mainly affected by MBN, TN, and C: N.
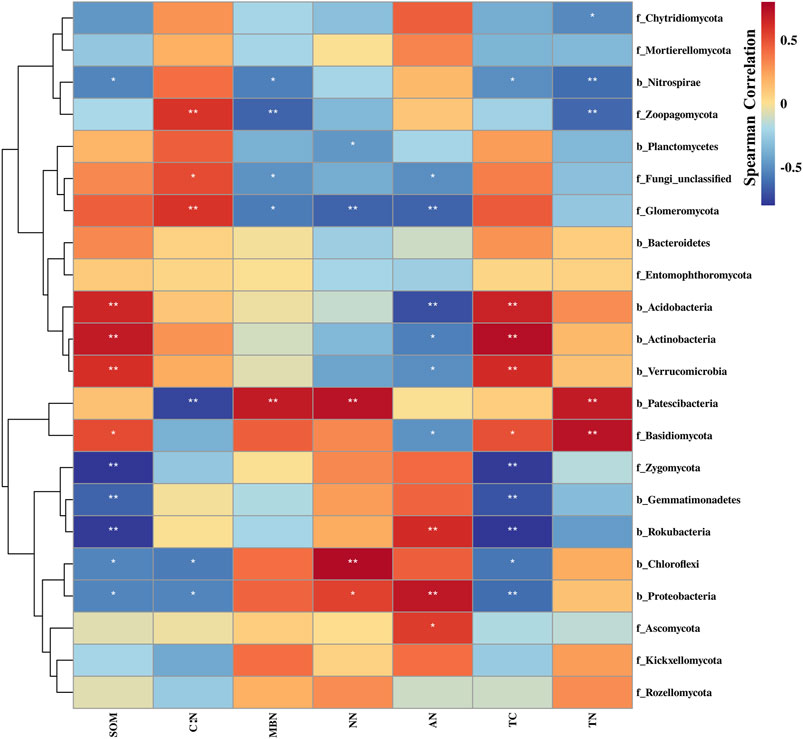
FIGURE 5. The spearman correlation heatmap between species and environmental factors. * and ** indicate p < 0.05 and p < 0.01.
Interactions Among Microbial Taxa in Networks
Network analysis was used to analyze the symbiosis modes of soil bacteria and fungi at the genus level under TR, LP, and CT treatments (Figure 6). The numbers of positive and negative connections between bacteria-bacteria, fungi-fungi, bacteria-fungi under different treatments, as well as their proportions in the total number of connections, were determined (Table 2). Compared with CT treatment, the total links of the microbial network of TR and LP treatments increased, although the increase of negative links was larger than that of positive links. In bacteria-bacteria, the increase in negative links of TR and LP treatments was higher than that of positive links. In fungi-fungi, the positive links of TR and LP treatments increased by 12.65 and 11.04%, respectively. In bacteria-fungi, the positive links of TR and LP treatments increased by 26.69 and 4.47%, respectively.
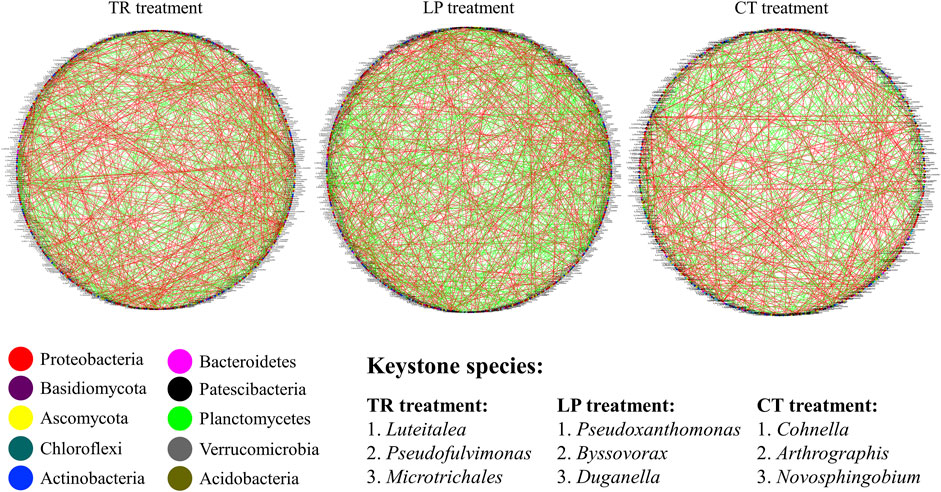
FIGURE 6. Co-occurrence networks between bacterial and fungal communities under Trifolium repens (TR), Lolium perenne (LP), and Clear tillage (CT) treatments. Red lines represent significant positive (Spearman’s correlation, p < 0.05) relationships, and blue lines denote negative (Spearman’s correlation, p < 0.05) relationships. For each treatment, 1–3 indicates the three keystone species (i.e., those having the highest betweenness centrality scores) in the network.
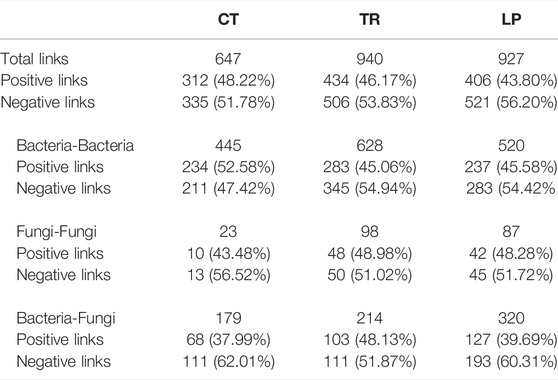
TABLE 2. Numbers of links in the networks of soil bacteria and fungi obtained for soil samples under Trifolium repens treatment (TR), Lolium perenne treatment (LP), and Clear tillage treatment (CT).
In addition, the top three genera were classified via multiple correlations with other microorganisms as keystone species based on their highest betweenness centrality scores. In the CT treatment, the soil network was mainly influenced by Cohnella, Arthrographis, Novosphingobium; keystone species in TR were Luteitalea, Pseudofulvimonas, and Microtrichales. The soil network was mainly influenced by Pseudoxanthomonas, Byssovorax, and Duganella in LP treatment.
Discussion
Soil microbial diversity is closely related to the versatility of soil ecosystems (Knight et al., 2018). Soil microorganisms are important in determining soil fertility, agriculture, and ecosystem sustainability (Essel et al., 2019). The β-diversity, a measure of composition among samples, links local to larger-scale diversity and is often used to examine patterns of diversity at different scales. The β-diversity of microorganisms represents the difference in the characteristics of the microbial community among samples (Wang et al., 2021). In the present study, different cover cropping treatments differently affected the bacterial and fungal community composition, indicating that the β-diversity of the microbial community in the cover cropping treatment changed significantly. Most likely, the plant residue in the cover cropping sites provided a stable habitat and more nutrients for the growth of microorganisms. (Verzeaux et al., 2016).
In our study, the main bacterial phyla were Proteobacteria, Acidobacteria, and Actinobacteria, as found in previous studies on cover cropping (Zheng et al., 2018b; Wang et al., 2020d). The abundance of Actinobacteria increased under TR and LP treatments; this taxon contains important decomposers of plant residues that secrete β-glucosidase and xylanase (Tiwari et al., 2016). Similar findings have been reported by Wang et al. (2020c) in an apple orchard. In this study, LP treatment also increased the abundance of Acidobacteria in the soil.
In the fungal community, LP treatment increased the abundance of Glomeromycota. Fungi in the phylum Glomeromycota include arbuscular mycorrhizal fungi (AMF), which are associated with vascular plants and thalloid bryophytes (Brundrett and Tedersoo, 2018), Lehman et al. (2012) also find that cover cropping can increase arbuscular mycorrhizal fungi levels in the soil. The LP treatment also increased the relative abundance of Basidiomycota, most likely because of the increase in plant litter after cover cropping, which increases the lignin content in the soil (Blackwood et al., 2007). Lehman et al. (2015) also demonstrate that cover cropping can increase the fungal dominance of the microbial community, which is conducive to SOM stabilization and soil fertility.
Compared with CT, cover cropping increased the levels of TC, SOM in orchard soil, indicating that this approach has a significant effect on the soil carbon content, which is similar to the results of other studies (Vicente-Vicente et al., 2017; Zheng et al., 2018a; Chenu et al., 2019). The SOM level is generally used as an important indicator of soil fertility (Poeplau and Don, 2015). In turn, the quality and quantity of plant residues have a great impact on SOM (Ali et al., 2019). Changes in cover crops induce variations in the quality of plant residue inputs and in the soil microbial community structure and activity, which may enhance the storage and protection of soil organic carbon (Almagro et al., 2021). In addition, orchard soil under cover cropping reduces the oxidation of organic matter (Mbuthia et al., 2015).
Correlation analysis showed that SOM, TC, and AN are the most important factors determining the composition of soil bacterial communities. Previous studies suggest that AN is the main factor impacting soil bacterial community composition (Nie et al., 2018), as it is the preferred nutrient for most bacteria (Merrick and Edwards, 1995). In our study, C: N, MBN, and TN affected the composition of soil fungal communities. This is in agreement with the findings of previous studies (Fontaine et al., 2011; Qi et al., 2021), where fungal activity was closely related to the total quality and conditions of the soil. Yang et al. (2013) also point out that changes in the soil microbial community structure are mainly controlled by the soil C: N ratio and the AN level. Decomposition of material with a higher C: N ratio is favored by fungi, whereas bacteria are better at decomposing material with a lower C: N ratio. Thus, the input of fresh organic matter with a high C: N ratio may preferentially stimulate the growth and proliferation of fungi (Zhong et al., 2018; Hu et al., 2019).
The microbial network structure changes along with changes in the microbial community composition. In studies on plant and animal ecology, network structure analysis based on biological communities is widely used (Bastolla et al., 2009). Our microbial networks show that compared with CT, the total links in cover cropping treatments were increased. In microbial networks, positive connections often indicate a cooperative relationship between species, whereas negative connections indicate an antagonistic relationship (Zheng et al., 2018c). In this study, the links of bacteria-bacteria accounted for the largest number of total links, which may be related to root exudates under cover crops, because bacteria usually react quickly to rhizosphere exudates (Berg and Smalla, 2009). In general, the numbers of positive and negative links of bacteria-bacteria links increased in TR and LP treatments, but the increase in positive links was lower than that in negative links, and the proportion of positive links in bacteria-bacteria decreased, indicating that cover cropping increased soil bacterial connections and aggravated the competition among bacteria. The positive links of fungi-fungi and bacteria-fungi increased more than significantly than the negative links, and we therefore speculate that the increase in the abundance and diversity of microorganisms in the soil may promote the frequency and extent of the interaction between fungi-fungi and bacteria-fungi. In addition, network analysis showed that compared with CT treatment, the fungi-fungi links among TR and LP increased significantly. The proportion of positive links increased, indicating that fungi are more capable of using SOM than bacteria. Previous studies have also suggested that fungal communities are more sensitive to vegetation changes than bacterial communities (de Vries et al., 2018). Fungal hyphae can enable fungi to use different kinds of nutrient resources, facilitating the use of some difficult-to-decompose organic matter compared to bacteria (de Boer et al., 2005). Moreover, due to the competition between fungi and bacteria, fungi-fungi contact is more frequent, which is conducive to the flow of energy, material, and information between fungi (Mille-Lindblom et al., 2006). Interestingly, the positive links between bacteria-fungi also increased. Bacteria and fungi usually also participate in the decomposition of plant residues together (Delgado-Baquerizo et al., 2016; Wang et al., 2020c). Abundant plant residues may enhance the synergistic and antagonistic interactions between bacterial and fungal communities (Yu et al., 2018), and complex networks with greater connectivity are more resistant to environmental disturbances than simple networks with lower connectivity (Santolini and Barabasi, 2018).
Conclusion
The composition and network structure of the soil microbial community plays an important role in the orchard ecosystem. In this study, we observed that different cover cropping treatments in an apple orchard differently influenced the composition of soil bacterial and fungal communities; in general, cover cropping increased the links among microorganisms. In particular, the interaction between fungi improved significantly. Fungi were more sensitive to cover cropping than bacteria. Cover cropping increased the levels of SOM and TN in orchards. The composition of the fungal community is mainly affected by MBN, TN, and C: N. In general, mulching not only increased the diversity of soil microbial communities but also increased the interconnection between microbial groups and improved the soil quality of apple orchards on the Loess Plateau. Further research on the effects of cover cropping on soil microorganisms is necessary to reveal the specific changes in soil microorganisms after cover cropping, evaluate the sustainability of the apple orchard ecosystem, and gain a more comprehensive understanding of the ecology of semi-arid apple orchards on the Loess Plateau in Northwest China.
Data Availability Statement
The datasets presented in this study can be found in online repositories. The names of the repository/repositories and accession number(s) can be found below: NCBI [accession: PRJNA745043, PRJNA745048].
Author Contributions
Conceptualization: M-XL. Data curation: C-YL. Formal analysis: C-YL, Y-YW. Funding acquisition: M-XL. Investigation: C-YL, X-MJ. Methodology: M-XL, C-YL. Project administration: M-XL. Resources: C-YL, X-YC, and QL. Software: C-YL, HT. Supervision: M-XL. Validation: M-XL. Writing-original draft: M-XL, C-YL. Writing-review and editing: M-XL, S-BH.
Funding
The work was supported by Major scientific and technological projects of Shaanxi Agricultural collaborative innovation and extension alliance in 2021 (grant no. LMZD202103), Sci-tech innovation and achievement transformation of experimental demonstration station of NWAFU (grant no. Z2220220018), Study on carbon and nitrogen transport mechanism of public mycorrhizal network in conservation sowing of alfalfa and millets (grant no. 32071878).
Conflict of Interest
The authors declare that the research was conducted in the absence of any commercial or financial relationships that could be construed as a potential conflict of interest.
Publisher’s Note
All claims expressed in this article are solely those of the authors and do not necessarily represent those of their affiliated organizations, or those of the publisher, the editors and the reviewers. Any product that may be evaluated in this article, or claim that may be made by its manufacturer, is not guaranteed or endorsed by the publisher.
References
Ali, A., Imran Ghani, M., Li, Y., Ding, H., Meng, H., and Cheng, Z. (2019). Hiseq Base Molecular Characterization of Soil Microbial Community, Diversity Structure, and Predictive Functional Profiling in Continuous Cucumber Planted Soil Affected by Diverse Cropping Systems in an Intensive Greenhouse Region of Northern China. Int. J. Mol. Sci. 20 (11), 2619. doi:10.3390/ijms20112619
Almagro, M., Ruiz-Navarro, A., Diaz-Pereira, E., Albaladejo, J., and Martinez-Mena, M. (2021). Plant Residue Chemical Quality Modulates the Soil Microbial Response Related to Decomposition and Soil Organic Carbon and Nitrogen Stabilization in a Rainfed Mediterranean Agroecosystem. Soil Biol. Biochem. 156, 108198. doi:10.1016/j.soilbio.2021.108198
Balota, E. L., and Auler, P. A. M. (2011). Soil Microbial Biomass under Different Management and Tillage Systems of Permanent Intercropped Cover Species in an Orange Orchard. Rev. Bras. Ciênc. Solo 35 (6), 1873–1883. doi:10.1590/S0100-06832011000600004
Banerjee, S., Schlaeppi, K., and van der Heijden, M. G. A. (2018). Keystone Taxa as Drivers of Microbiome Structure and Functioning. Nat. Rev. Microbiol. 16 (9), 567–576. doi:10.1038/s41579-018-0024-1
Banerjee, S., Walder, F., Büchi, L., Meyer, M., Held, A. Y., Gattinger, A., et al. (2019). Agricultural Intensification Reduces Microbial Network Complexity and the Abundance of Keystone Taxa in Roots. ISME J. 13 (7), 1722–1736. doi:10.1038/s41396-019-0383-2
Bardgett, R. D., and van der Putten, W. H. (2014). Belowground Biodiversity and Ecosystem Functioning. Nature 515 (7528), 505–511. doi:10.1038/nature13855
Bastolla, U., Fortuna, M. A., Pascual-García, A., Ferrera, A., Luque, B., and Bascompte, J. (2009). The Architecture of Mutualistic Networks Minimizes Competition and Increases Biodiversity. Nature 458 (7241), 1018–1020. doi:10.1038/nature07950
Berg, G., and Smalla, K. (2009). Plant Species and Soil Type Cooperatively Shape the Structure and Function of Microbial Communities in the Rhizosphere. FEMS Microbiol. Ecol. 68 (1), 1–13. doi:10.1111/j.1574-6941.2009.00654.x
Blackwood, C. B., Waldrop, M. P., Zak, D. R., and Sinsabaugh, R. L. (2007). Molecular Analysis of Fungal Communities and Laccase Genes in Decomposing Litter Reveals Differences Among Forest Types but No Impact of Nitrogen Deposition. Environ. Microbiol. 9 (5), 1306–1316. doi:10.1111/j.1462-2920.2007.01250.x
Brennan, E. B., and Acosta-Martinez, V. (2017). Cover Cropping Frequency Is the Main Driver of Soil Microbial Changes during Six Years of Organic Vegetable Production. Soil Biol. Biochem. 109, 188–204. doi:10.1016/j.soilbio.2017.01.014
Brundrett, M. C., and Tedersoo, L. (2018). Evolutionary History of Mycorrhizal Symbioses and Global Host Plant Diversity. New Phytol. 220 (4), 1108–1115. doi:10.1111/nph.14976
Caporaso, J. G., Kuczynski, J., Stombaugh, J., Bittinger, K., Bushman, F. D., Costello, E. K., et al. (2010). QIIME Allows Analysis of High-Throughput Community Sequencing Data. Nat. Methods 7 (5), 335–336. doi:10.1038/nmeth.f.303
Chavarría, D. N., Verdenelli, R. A., Serri, D. L., Restovich, S. B., Andriulo, A. E., Meriles, J. M., et al. (2016). Effect of Cover Crops on Microbial Community Structure and Related Enzyme Activities and Macronutrient Availability. Eur. J. Soil Biol. 76, 74–82. doi:10.1016/j.ejsobi.2016.07.002
Chenu, C., Angers, D. A., Barré, P., Derrien, D., Arrouays, D., and Balesdent, J. (2019). Increasing Organic Stocks in Agricultural Soils: Knowledge Gaps and Potential Innovations. Soil Tillage Res. 188, 41–52. doi:10.1016/j.still.2018.04.011
de Boer, W., Folman, L. B., Summerbell, R. C., and Boddy, L. (2005). Living in a Fungal World: Impact of Fungi on Soil Bacterial Niche Development. FEMS Microbiol. Rev. 29 (4), 795–811. doi:10.1016/j.femsre.2004.11.005
de Vries, F. T., Griffiths, R. I., Bailey, M., Craig, H., Girlanda, M., Gweon, H. S., et al. (2018). Soil Bacterial Networks Are Less Stable under Drought Than Fungal Networks. Nat. Commun. 9, 3033. doi:10.1038/s41467-018-05516-7
Delgado-Baquerizo, M., Maestre, F. T., Reich, P. B., Jeffries, T. C., Gaitan, J. J., Encinar, D., et al. (2016). Microbial Diversity Drives Multifunctionality in Terrestrial Ecosystems. Nat. Commun. 7, 10541. doi:10.1038/ncomms10541
Essel, E., Xie, J., Deng, C., Peng, Z., Wang, J., Shen, J., et al. (2019). Bacterial and Fungal Diversity in Rhizosphere and Bulk Soil under Different Long-Term Tillage and Cereal/legume Rotation. Soil Tillage Res. 194, 104302. doi:10.1016/j.still.2019.104302
Fontaine, S., Henault, C., Aamor, A., Bdioui, N., Bloor, J. M. G., Maire, V., et al. (2011). Fungi Mediate Long Term Sequestration of Carbon and Nitrogen in Soil through Their Priming Effect. Soil Biol. Biochem. 43 (1), 86–96. doi:10.1016/j.soilbio.2010.09.017
Herren, C. M., and McMahon, K. D. (2018). Keystone Taxa Predict Compositional Change in Microbial Communities. Environ. Microbiol. 20 (6), 2207–2217. doi:10.1111/1462-2920.14257
Hu, Y., Veresoglou, S. D., Tedersoo, L., Xu, T., Ge, T., Liu, L., et al. (2019). Contrasting Latitudinal Diversity and Co-Occurrence Patterns of Soil Fungi and Plants in Forest Ecosystems. Soil Biol. Biochem. 131, 100–110. doi:10.1016/j.soilbio.2019.01.001
Hwang, H. Y., Kim, G. W., Lee, Y. B., Kim, P. J., and Kim, S. Y. (2015). Improvement of the Value of Green Manure via Mixed Hairy Vetch and Barley Cultivation in Temperate Paddy Soil. Field Crops Res. 183, 138–146. doi:10.1016/j.fcr.2015.08.001
Jansson, S. L., and Persson, J. (1982). “Mineralization and Immobilization of Soil Nitrogen,” in Nitrogen in Agricultural Soils, Madison, WI. Editor F. J. Stevenson, 22, 229–252. doi:10.2134/agronmonogr22.C6
Karlsson, I., Friberg, H., Steinberg, C., and Persson, P. (2014). Fungicide Effects on Fungal Community Composition in the Wheat Phyllosphere. PLoS One 9 (11), e111786. doi:10.1371/journal.pone.0111786
Khan, M. I., Gwon, H. S., Alam, M. A., Song, H. J., Das, S., and Kim, P. J. (2020). Short Term Effects of Different Green Manure Amendments on the Composition of Main Microbial Groups and Microbial Activity of a Submerged Rice Cropping System. Appl. Soil Ecol. 147, 103400. doi:10.1016/j.apsoil.2019.103400
Knight, R., Vrbanac, A., Taylor, B. C., Aksenov, A., Callewaert, C., Debelius, J., et al. (2018). Best Practices for Analysing Microbiomes. Nat. Rev. Microbiol. 16 (7), 410–422. doi:10.1038/s41579-018-0029-9
Lax, S., Cardona, C., Zhao, D., Winton, V. J., Goodney, G., Gao, P., et al. (2019). Microbial and Metabolic Succession on Common Building Materials under High Humidity Conditions. Nat. Commun. 10 (1), 1767. doi:10.1038/s41467-019-09764-z
Lehman, R. M., Taheri, W. I., Osborne, S. L., Buyer, J. S., and Douds, D. D. (2012). Fall Cover Cropping Can Increase Arbuscular Mycorrhizae in Soils Supporting Intensive Agricultural Production. Appl. Soil Ecol. 61, 300–304. doi:10.1016/j.apsoil.2011.11.008
Lehman, R., Cambardella, C., Stott, D., Acosta-Martinez, V., Manter, D., Buyer, J., et al. (2015). Understanding and Enhancing Soil Biological Health: The Solution for Reversing Soil Degradation. Sustainability 7 (1), 988–1027. doi:10.3390/su7010988
Márquez-García, F., González-Sánchez, E. J., Castro-García, S., and Ordóñez-Fernández, R. (2013). Improvement of Soil Carbon Sink by Cover Crops in Olive Orchards under Semiarid Conditions. Influence of the Type of Soil and Weed. Span. J. Agric. Res. 11 (2), 335–346. doi:10.5424/sjar/2013112-3558
Mbuthia, L. W., Acosta-Martínez, V., DeBruyn, J., Schaeffer, S., Tyler, D., Odoi, E., et al. (2015). Long Term Tillage, Cover Crop, and Fertilization Effects on Microbial Community Structure, Activity: Implications for Soil Quality. Soil Biol. Biochem. 89, 24–34. doi:10.1016/j.soilbio.2015.06.016
Merrick, M. J., and Edwards, R. A. (1995). Nitrogen Control in Bacteria. Microbiol. Rev. 59 (4), 604–622. doi:10.1128/mr.59.4.604-622.1995
Mille-Lindblom, C., Fischer, H., and J. Tranvik, L. (2006). Antagonism between Bacteria and Fungi: Substrate Competition and a Possible Tradeoff between Fungal Growth and Tolerance towards Bacteria. Oikos 113 (2), 233–242. doi:10.1111/j.2006.0030-1299.14337.x
Miltner, A., Bombach, P., Schmidt-Brücken, B., and Kästner, M. (2012). SOM Genesis: Microbial Biomass as a Significant Source. Biogeochemistry 111 (1-3), 41–55. doi:10.1007/s10533-011-9658-z
National Bureau of Statistics of China (2020). China Rural Statistical Year Book. Beijing: China Statistics Press.
Nie, Y., Wang, M., Zhang, W., Ni, Z., Hashidoko, Y., and Shen, W. (2018). Ammonium Nitrogen Content Is a Dominant Predictor of Bacterial Community Composition in an Acidic Forest Soil with Exogenous Nitrogen Enrichment. Sci. Total Environ. 624, 407–415. doi:10.1016/j.scitotenv.2017.12.142
Nieto, O. M., Castro, J., and Fernández-Ondoño, E. (2013). Conventional Tillage Versus Cover Crops in Relation to Carbon Fixation in Mediterranean Olive Cultivation. Plant Soil 365 (1-2), 321–335. doi:10.1007/s11104-012-1395-0
Poeplau, C., and Don, A. (2015). Carbon Sequestration in Agricultural Soils via Cultivation of Cover Crops - A Meta-Analysis. Agric. Ecosyst. Environ. 200, 33–41. doi:10.1016/j.agee.2014.10.024
Qi, D., Wieneke, X., Xue, P., He, L., and DeSilva, U. (2021). Total Nitrogen Is the Main Soil Property Associated with Soil Fungal Community in Karst Rocky Desertification Regions in Southwest China. Sci. Rep. 11 (1), 10809. doi:10.1038/s41598-021-89448-1
Qian, X., Gu, J., Pan, H.-J., Zhang, K.-Y., Sun, W., Wang, X.-J., et al. (2015). Effects of Living Mulches on the Soil Nutrient Contents, Enzyme Activities, and Bacterial Community Diversities of Apple Orchard Soils. Eur. J. Soil Biol. 70, 23–30. doi:10.1016/j.ejsobi.2015.06.005
Santolini, M., and Barabási, A.-L. (2018). Predicting Perturbation Patterns from the Topology of Biological Networks. Proc. Natl. Acad. Sci. U.S.A. 115 (27), E6375–E6383. doi:10.1073/pnas.1720589115
Segata, N., Izard, J., Waldron, L., Gevers, D., Miropolsky, L., Garrett, W. S., et al. (2011). Metagenomic Biomarker Discovery and Explanation. Genome Biol. 12 (6), R60. doi:10.1186/gb-2011-12-6-r60
Snapp, S., and Surapur, S. (2018). Rye Cover Crop Retains Nitrogen and Doesn't Reduce Corn Yields. Soil Tillage Res. 180, 107–115. doi:10.1016/j.still.2018.02.018
Storvick, C. A. (1950). Johan G. C. T. Kjeldahl. His Method for Determining Total Nitrogen. J. Am. Dietetic Assoc. 26 (10), 799–801. doi:10.1016/s0002-8223(21)30438-2
Tiwari, R., Kumar, K., Singh, S., Nain, L., and Shukla, P. (2016). Molecular Detection and Environment-Specific Diversity of Glycosyl Hydrolase Family 1 β-Glucosidase in Different Habitats. Front. Microbiol. 7, 1597. doi:10.3389/fmicb.2016.01597
Verzeaux, J., Alahmad, A., Habbib, H., Nivelle, E., Roger, D., Lacoux, J., et al. (2016). Cover Crops Prevent the Deleterious Effect of Nitrogen Fertilisation on Bacterial Diversity by Maintaining the Carbon Content of Ploughed Soil. Geoderma 281, 49–57. doi:10.1016/j.geoderma.2016.06.035
Vicente-Vicente, J. L., Gómez-Muñoz, B., Hinojosa-Centeno, M. B., Smith, P., and Garcia-Ruiz, R. (2017). Carbon Saturation and Assessment of Soil Organic Carbon Fractions in Mediterranean Rainfed Olive Orchards under Plant Cover Management. Agric. Ecosyst. Environ. 245, 135–146. doi:10.1016/j.agee.2017.05.020
Wang, Y., Liu, L., Wang, Y., Tao, H. X., Fan, J. L., Zhao, Z. Y., et al. (2019). Effects of Soil Water Stress on Fruit Yield, Quality and Their Relationship with Sugar Metabolism in 'Gala' Apple. Sci. Hortic-Amsterdam 258, 108753. doi:10.1016/j.scienta.2019.108753
Wang, Y., Liu, L., Yang, J., Duan, Y., Luo, Y., Taherzadeh, M. J., et al. (2020a). The Diversity of Microbial Community and Function Varied in Response to Different Agricultural Residues Composting. Sci. Total Environ. 715, 136983. doi:10.1016/j.scitotenv.2020.136983
Wang, Y., Liu, L., Tian, Y. L., Wu, X. P., Yang, J. F., Luo, Y., et al. (2020b). Temporal and Spatial Variation of Soil Microorganisms and Nutrient under White Clover Cover. Soil Till Res. 202, 104666. doi:10.1016/j.still.2020.104666
Wang, Y., Liu, L., Luo, Y., Awasthi, M. K., Yang, J., Duan, Y., et al. (2020c). Mulching Practices Alter the Bacterial-Fungal Community and Network in Favor of Soil Quality in a Semiarid Orchard System. Sci. Total Environ. 725, 138527. doi:10.1016/j.scitotenv.2020.138527
Wang, Y., Huang, Q., Liu, C., Ding, Y., Liu, L., Tian, Y., et al. (2020d). Mulching Practices Alter Soil Microbial Functional Diversity and Benefit to Soil Quality in Orchards on the Loess Plateau. J. Environ. Manage. 271, 110985. doi:10.1016/j.jenvman.2020.110985
Wang, Y., Li, C., Tu, B., Kou, Y., and Li, X. (2021). Species Pool and Local Ecological Assembly Processes Shape the β-Diversity of Diazotrophs in Grassland Soils. Soil Biol. Biochem. 160, 108338. doi:10.1016/j.soilbio.2021.108338
Xiong, Y., Xia, H., Li, Z. A., Cai, X. A., and Fu, S. (2008). Impacts of Litter and Understory Removal on Soil Properties in a Subtropical Acacia Mangium Plantation in China. Plant Soil 304 (1-2), 179–188. doi:10.1007/s11104-007-9536-6
Yang, Y., Wu, L., Lin, Q., Yuan, M., Xu, D., Yu, H., et al. (2013). Responses of the Functional Structure of Soil Microbial Community to Livestock Grazing in the Tibetan Alpine Grassland. Glob. Change Biol. 19 (2), 637–648. doi:10.1111/gcb.12065
Yu, J., Deem, L. M., Crow, S. E., Deenik, J. L., and Penton, C. R. (2018). Biochar Application Influences Microbial Assemblage Complexity and Composition Due to Soil and Bioenergy Crop Type Interactions. Soil Biol. Biochem. 117, 97–107. doi:10.1016/j.soilbio.2017.11.017
Zagal, E., Muñoz, C., Quiroz, M., and Córdova, C. (2009). Sensitivity of Early Indicators for Evaluating Quality Changes in Soil Organic Matter. Geoderma 151 (3-4), 191–198. doi:10.1016/j.geoderma.2009.04.004
Zhang, X., Zhang, R., Gao, J., Wang, X., Fan, F., Ma, X., et al. (2017). Thirty-one Years of Rice-Rice-Green Manure Rotations Shape the Rhizosphere Microbial Community and Enrich Beneficial Bacteria. Soil Biol. Biochem. 104, 208–217. doi:10.1016/j.soilbio.2016.10.023
Zheng, W., Gong, Q., Zhao, Z., Liu, J., Zhai, B., Wang, Z., et al. (2018a). Changes in the Soil Bacterial Community Structure and Enzyme Activities after Intercrop Mulch with Cover Crop for Eight Years in an Orchard. Eur. J. Soil Biol. 86, 34–41. doi:10.1016/j.ejsobi.2018.01.009
Zheng, W., Zhao, Z., Gong, Q., Zhai, B., and Li, Z. (2018b). Responses of Fungal-Bacterial Community and Network to Organic Inputs Vary Among Different Spatial Habitats in Soil. Soil Biol. biochem. 125, 54–63. doi:10.1016/j.soilbio.2018.06.029
Zheng, W., Zhao, Z., Gong, Q., Zhai, B., and Li, Z. (2018c). Effects of Cover Crop in an Apple Orchard on Microbial Community Composition, Networks, and Potential Genes Involved with Degradation of Crop Residues in Soil. Biol. Fertil. Soils 54 (6), 743–759. doi:10.1007/s00374-018-1298-1
Zhong, Y., Yan, W., Wang, R., Wang, W., and Shangguan, Z. (2018). Decreased Occurrence of Carbon Cycle Functions in Microbial Communities along with Long-Term Secondary Succession. Soil Biol. Biochem. 123, 207–217. doi:10.1016/j.soilbio.2018.05.017
Zhou, J., Deng, Y., Luo, F., He, Z., and Yang, Y. (2011). Phylogenetic Molecular Ecological Network of Soil Microbial Communities in Response to Elevated CO2. mBio 2 (4). doi:10.1128/mBio.00122-11
Keywords: bacterial community, fungal community, cover croppings, microbial network, soil fertility
Citation: Liu C-Y, Wang Y-y, Chen X-y, Tan H, Jin X-m, Lu Q, He S-b and Long M-X (2022) Cover Cropping Increases Soil Fungal-Bacterial Community Diversity and Network Complexity in Apple Orchards on the Loess Plateau, China. Front. Environ. Sci. 10:916288. doi: 10.3389/fenvs.2022.916288
Received: 09 April 2022; Accepted: 05 May 2022;
Published: 26 May 2022.
Edited by:
Mahesh Kumar Gathala, International Maize and Wheat Improvement Center (CIMMYT)-Bangladesh, BangladeshCopyright © 2022 Liu, Wang, Chen, Tan, Jin, Lu, He and Long. This is an open-access article distributed under the terms of the Creative Commons Attribution License (CC BY). The use, distribution or reproduction in other forums is permitted, provided the original author(s) and the copyright owner(s) are credited and that the original publication in this journal is cited, in accordance with accepted academic practice. No use, distribution or reproduction is permitted which does not comply with these terms.
*Correspondence: Shu-bin He, aGVzaHViaW5AbndzdWFmLmVkdS5jbg==; Ming-Xiu Long, bG9uZ21pbmd4aXVAbndzdWFmLmVkdS5jbg==