- 1State Key Laboratory for Managing Biotic and Chemical Threats to the Quality and Safety of Agro-products, Institute of Environment, Resource, Soil and Fertilizer, Zhejiang Academy of Agricultural Sciences, Hangzhou, China
- 2College of Environment, Zhejiang University of Technology, Hangzhou, China
- 3Engineering Research Center of Biochar of Zhejiang Province, Hangzhou, China
- 4School of Resources and Environmental Engineering, Wuhan University of Technology, Wuhan, China
The specific effect of long-term successive biochar application on soil fertility, α-diversity, and composition of bacterial community and their correlations remain unclear. A field experiment was conducted to investigate the effects of straw biochar application on soil physical and chemical properties and the diversity and composition of the bacterial communities in 12 consecutive crop seasons. Four treatments: BC1 (2.25 Mgha−1 biochar), BC5 (11.25 Mgha−1 biochar), RS (2.25 Mgha−1 rice straw), and blank control (CK, without biochar or rice straw) were set up. The results indicated that biochar and rice straw reduced the richness indexes of the soil bacterial community (Chao1 and ACE by 10.3%–27.4% and 12.2%–26.4%, respectively). High-throughput sequencing results showed that the relative abundance of Cyanobacteria, Bacteroidetes, and Actinobacteria increased as the amount of biochar increased, while those of norank_c__Acidobacteria and norank_f__Anaerolineaceae Proteobacteria, Acidobacteria and Nitrospirae decreased. Long-term successive biochar application significantly increased soil pH, available potassium, total organic carbon, total nitrogen, and cation exchange capacity by 5.7%–25.9%, 40.0%–680.0%, 48.0%–217.1%, 51.0%–109.5%, and 5.4%–24.0%, respectively. Soil dissolved organic carbon was slightly decreased by 1.4%–4.0%. Soil pH and C/N were the two major environmental factors affecting the composition of the soil bacterial community according to redundancy analysis. Furthermore, the phylogenetic investigation of communities by reconstruction of unobserved states showed that biochar significantly increased the relative abundance of information on the functions of the metabolism of other amino acids, metabolism of terpenoids and polyketides, and biosynthesis of other secondary metabolites (p < 0.05). Therefore, long-term successive biochar amendment in rice-wheat rotation systems improved soil fertility, altered the structure of the soil bacterial community, and increased the functions of soil bacteria, but decreased the α-diversity of the bacterial community. This study will provide technical and theoretical support for rice-straw carbonization and long-term soil remediation from the perspective of microorganisms.
Introduction
Rice straw, an important biomass resource in the agricultural crop system, is becoming a threat through excess abundance. The burning of rice straw by farmers has resulted in a massive waste of resources, and aggravated ecological pollution (Zeng et al., 2007). Returning straw to the field is a reasonable and efficient way of addressing this and would result in the utilization of agricultural waste resources, which contain nutrients such as silicon, potassium, and cellulose, as well as reduce the risk of air pollution and fire hazards (Marschner and Renge., 2007; Zang et al., 2008). Biochar is a carbon-rich material prepared from biomass such as agricultural straw and forestry waste through pyrolysis under oxygen-limited conditions. Owing to its developed pores, large specific surface area, and good adsorption capacity, the biochar derived from crop straw is widely used in farmland ecosystems for soil amendment to address resource utilization and environmental pollution (Huang et al., 2021).
Microbial communities play an indispensable role in farmland soil environments, particularly with respect to soil ecological balance and nutrient transfer and transformation (Zhu et al., 2015; Lin et al., 2017). The abundance and composition of the bacterial community in paddy soils are impacted by many factors such as organic matter, pH, and moisture. In particular, organic matter can provide essential nutrients for bacterial metabolism and growth (Kennedy and Smith, 1995; Falkowski et al., 2008). As an exogenous additive, biochar amendment changes the physicochemical properties of soil, and thus affects the microbial activities of soil and alters its community abundance and composition. For example, straw residues and wood chip biochar amendments significantly enhanced bacterial abundance by 161.0% after 135 days of incubation (Li et al., 2016). Soil bacterial diversity significantly increased within a short period after biochar application, and was positively correlated with the amount of biochar (Chen et al., 2013; Nan et al., 2016). Owing to its well-developed pores adsorbing sufficient nutrients, biochar increased the diversity and abundance of the soil bacterial community and provided a more optimized habitat for bacterial aggregation and survival. However, biochar reduced soil microbial biomass and microbial community abundance by adsorbing nitrification-inhibited compounds (α-pine olefin) and microbial toxic aromatic compounds (e.g., PHCs) among other substances, especially in ammonia-oxidizing bacteria (Clough et al., 2010; Cheng et al., 2012; Dempster et al., 2012). Therefore, the response of the soil bacterial community to biochar is complex and may be related to the type and amount of biochar, and its length of life.
Previous research (Yuan et al., 2019) has mostly focused on the short-term effects of biochar on soil bacteria (i.e., <2–3 years), and the related results have been inconsistent. Considering the excitability and limitation of short-term biochar, its application time has become particularly important. The structure and properties of biochar vary according to different preparation conditions, raw materials, and weathering times (Schmidt et al., 2001). Weathering and aging determine the physical and chemical properties of biochar stably, particularly in the available nutrients. Considering that soil microbial activity is affected by exogenous additives, our current understanding of the effects of biochar on soil may not be conclusive (Noyce et al., 2015). This necessitates the examination of the long-term effects of biochar on soil ecosystems (Nelissen et al., 2015a). Given the significant roles that microorganisms play in the soil ecology environment, we explored the long-term effects (i.e., >4 years) of biochar application on soil bacterial diversity, the abundance and composition of the soil bacterial community were crucial. Compared with the one-time application method (Fan et al., 2020), successive biochar amendments in each planting season of rice-wheat rotation provided the technical support required to determine the maximum carbon tolerance of the soil.
In this study, a field experiment was conducted to investigate the changes in the α-diversity, composition, and predictive function of the soil bacterial community following the successive addition of straw biochar and rice straw for six rice-wheat rotation cycles. The relationship between soil environmental factors and bacterial community parameters was also investigated. This study will elucidate the long-term effects of successive biochar application on soil microorganisms and provide a reference for the rational allocation of straw resources.
Materials and Methods
Study Site and Experimental Design
A 6-year field experiment on rice-wheat rotation was conducted in 2010 during the rice-growing season at the Yixing Base for Agri-Environment Research, Changshu, Jiangsu province (31°07′N–31°37′N, 119°31′E–120°03′E). The study site is located in a subtropical monsoon climate with an average temperature of 15.7°C and annual rainfall of 1207 mm. The paddy soil contains 8.3% sand (> 0.05 mm), 81.5% silt (0.02–0.05 mm), and 10.2% clay (< 0.02 mm). The basic soil characteristics with no biochar at the beginning of the field experiment were as follows: 15.4 gkg−1 total organic carbon (TOC), 1.8 gkg−1 total nitrogen (TN), 28.6 gkg−1 soil organic matter, 0.06 gkg−1 available phosphorus (AP), 0.05 gkg−1 available potassium (AK), and pH 6.1.
Six rice-wheat rotation cycles were completed from October 2010 to June 2016. Two application rates of biochar in a randomized complete block design in triplicate: BC1, BC5, (2.25, 11.25 Mgha−1 biochar, respectively), and RS (2.25 Mgha−1 rice straw) for each crop season (rice usually was planted in May and wheat in October), as well as a control (CK, without biochar or rice straw), were established. The experimental design offered a guide for evaluating the potential of straw carbonization from the perspective of resource utilization. In Taihu Basin, the yield of rice straw was approximately 7.5 Mgha−1 for each crop season (Zhao X et al., 2014). RS treatment was 2.25 Mgha−1, which was equal to 30% of the seasonal production of rice straw amounts. Based on the 30% rate of conversion of straw into biochar, BC1 and BC5 treatments at 2.25 and 11.25 Mgha−1 biochar amendment amounts were 1 and 5 times the production amount of rice straw converted to biochar and placed in soil per growing season.
Each plot was 20 m2 (4 m × 5 m), and the plots received urea as basal fertilizers at 200 kg Nha−1 for wheat and 300 kg Nha−1 for rice, and at the basic, tillering, and elongation differentiation growth stages at a ratio of 3:4:3. The 60 kgha−1 of P2O5 (superphosphate) and K2O (KCl) were also input as basal fertilizers. Biochar was applied to soil within a depth of 20 cm, which was prepared from rice straw at a pyrolysis temperature of 500°C for 8 h. The biochar contained 620 g Ckg−1, 13.3 g Nkg−1, 9.19 pH (1: 2.5 H2O), 18.9 cmolkg−1 cation exchange capacity (CEC), and 276 gkg−1 ash.
Soil Sampling and Analysis
After the 2016 rice harvest, fresh soil samples were collected from each plot with 6 years of rice-wheat rotation where biochar was applied for 12 seasons. Five soil subsamples (2 cm in diameter, 10 cm in depth) were collected from each plot and then mixed to form an independent mixed sample to obtain a uniform soil sample for subsequent measure and analysis. All soil samples were placed in a sterilized bag, sealed for storage, and taken back to the laboratory immediately. Debris such as roots and stones were removed from all soil samples, which were then passed through a 2 mm sieve for division into two parts: one part was stored at −75°C for DNA extraction and the other part was air-dried to determine soil characteristics after 6 years of biochar consecutive amendments.
For chemical analysis, soil TN was measured using the Kjeldahl procedure, and TOC was determined using a Leco CN-2000 analyzer (Leco Corp., St. Joseph, MI, United States). The soil pH was determined using a pH meter in distilled water at a ratio of 1:2.5 (w/v). The CEC was determined (Hailegnaw et al., 2019) by a modified ammonium acetate exchange method, and was titrated with 1 molL−1 ammonium acetate after distillation at pH 7.0. Soil dissolved organic C/N (DOC/DON) was extracted from 10 g of fresh soil and added to 50 ml of distilled water, which was shaken on a shaker for 1 h, and then placed in a centrifuge at 3500 rpm for 20 min. The solution was then filtered through a 0.45-µm filter, which measured the C/N ratio by a multi-C/N analyzer.
DNA Extraction and High Throughput Sequencing of 16S Ribosomal RNA
DNA was extracted from 0.5 g of fresh soil using a FastDNA SPIN kit (MP Biomedicals, Santa Ana, CA, United States). The concentration, purity, and integrity of DNA were then examined by 1% agarose gel electrophoresis. Appropriate volumes of DNA samples were placed in centrifuge tubes and diluted with sterilized water to 1 ng μl−1.
The V4–V5 region of the bacterial 16S ribosomal RNA gene was amplified by primers F515 and R907, and the bacterial composition was evaluated using the Illumina MiSeq platform. The bar code sequence of 8 bases was added to each primer to facilitate sample identification. The PCR reaction was performed in a 50-μl reaction mixture containing 25 μl Premix Taq DNA polymerase (Takara Biotech, Dalian, China). Each primer was 0.5 μM, and each DNA template 1 μL. The conditions of PCR cycling were as follows: initial 2-min denaturation at 95°C, followed by 30 cycles at 95°C for 30 s, 55°C for 30 s, and 72°C for 30 s, and a final extension at 72°C for 5 min (Zheng et al., 2016). The PCR products were purified using a gel extraction kit (Takara Biotech, Dalian, China) conducted by the manufacturer’s instructions. The purified amplicons were polymerized in an equimolar manner and paired-end sequenced (2 × 250) on an Illumina MiSeq platform. The NCBI Nucleotide Sequence Database records the raw reads.
Sequencing Data and Statistical Analyses
The raw sequences, which perfectly corresponded to bar codes, were segmented into sample libraries and trimmed with QIIME (Version 1.17; Wang et al., 2007) to remove low-quality sequences (quality < 20 and length < 250 bp). All overlap reads were removed, all singletons were filtered, and available sequences were clustered into operational taxonomic units (OTUs) through Uparsae (Version 7.0) with 97% similarity. The ribosome database project (RDP) classifier was used to classify the representative sequences of each OTU with a confidence threshold of 0.70.
Data Analysis
The initial analysis of the raw data was performed to remove extreme data points (> 95%) from every treatment using WPS Excel 2020. Analyses were computed in SPSS (version 17.0; IBM Corp, Chicago, IL, United States). Differences among soil properties and the α-diversity of the soil bacterial community were determined using analysis of variance (ANOVA), and p < 0.05 represents a significant difference. The α-diversity indices, including the richness index (ACE and Chao1) and evenness index (Shannon and Simpson), were evaluated using Mothur (Version 1.30). Using R language (Version 3.3.1) for the Principal coordinate analysis (PCoA) and redundancy analysis (RDA), the figures were prepared.
Results
Soil Properties
The long-term addition of successive biochar and rice straw led to varying soil properties. Table 1 shows significant differences across treatments, with consistent increases in soil pH (0.22–1.35), TOC (5.8–26.2 gkg−1), TN (0.31–1.61 gkg−1), CEC (0.6–2.7 cmolkg−1), and AK (0.02–0.34 gkg−1). However, biochar and rice straw amendment decreased soil DOC to approximately 1.2 mgkg−1 and had no significant effects on soil AP. The C/N ratio in BC1, BC5, and RS were significantly higher than that of CK within the range of 2.64–4.00. After long-term successive biochar application, soil CEC increased considerably (5.4%–24.0%), and the differences among different treatments were significant (p < 0.05). The content of soil CEC was 12.2 cmolkg−1 was close to BC1 (12.0 cmolkg−1).
Soil Bacterial Community Diversity
The α-Diversity of Soil Bacterial Community
A total of 397,702 sequences with mean lengths of 423 bp from all samples, were obtained by quality filtering the raw reads. Through 16S rRNA amplicon sequencing, these high-quality reads were clustered into 1407 OTUs. Table 2 shows significant differences among CK, BC1, BC5, and RS (p < 0.05). Long-term successive straw biochar (BC1, BC5) and rice straw (RS) amendment decreased the number of OTUs in rice-wheat rotation by 10.7%, 32.9%, and 17.7%, respectively (Table 2).
Bacterial α-diversity was determined by the Chao1 and ACE richness indexes and Shannon and Simpson evenness indexes, both of which were calculated using 6645 sequences per sample. To evaluate the sampling integrity, we determined good coverage, which exceeded 0.9995 for all treatments, indicating that the vast majority of bacterial communities were captured at this sequencing depth. The bacterial richness index of all soil samples showed that the long-term successive biochar and rice straw amendments were different. BC1, BC5, and RS treatments reduced Chao1 by 10.3%, 27.4%, and 14.9%, respectively and ACE by 12.2%, 26.4%, and 14.5%, respectively (Table 2). Although ACE and Chao1 were different indexes of bacterial richness, they led to the same conclusion and verified each other. The Shannon index was lower in biochar and rice straw treatments than in CK, while the Simpson index of each treatment was higher than that of CK. The results confirmed that long-term successive biochar and rice straw amendment could significantly reduce the α-diversity of the soil bacterial community in rice-wheat rotation. Plus, there were significant differences in the α-diversity among different biochar treatments (Table 2).
The β-Diversity of Soil Bacterial Community
Sample hierarchical clustering tree, which was constructed to analyze sample distance matrix to evaluate the similarity and difference of different samples, accurately. From Figure 1, the soils applied with biochar could cluster together completely and were far away from the CK, indicating the alteration of the soil bacterial community in a certain direction. Furthermore, biochar amendment could significantly change the structure of the soil bacterial community in rice-wheat rotation.
The Bray–Curtis distance was used, and a matrix was constructed based on OTU abundance information. Multivariate statistical principal coordinate analysis (PCoA) was performed to evaluate the effects of long-term applications of straw biochar and rice straw on the bacterial community composition in paddy soil for consecutive years. As shown in Figure 2, principal components 1 (PC1) and 2 (PC2) were the most significant causes of differences across all samples, accounting for 32.11% and 16.60% of the total variance, respectively. Results showed a clear separation of soil treatments on soil bacterial community composition from BC5 and RS to CK and BC1 along PC1. The separation of BC5 and CK from BC1, BC5, and RS was clearly revealed when comparing the bacterial community for four treatments along PC2. Therefore, PCoA showed that long-term application of successive biochar and rice straw in the rice-wheat rotation could change the soil’s bacterial community structure significantly (Figure 2).
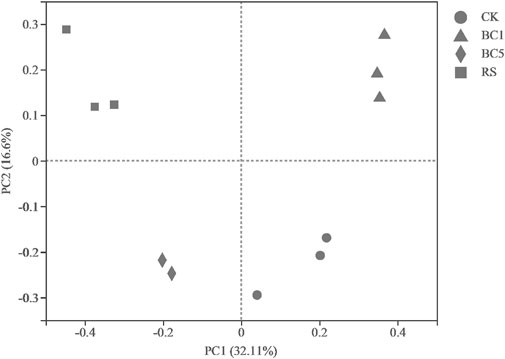
FIGURE 2. Principal coordinate analysis (PCoA) based on Bray–Curtis distances of bacterial communities for different treatments. CK: without biochar or rice straw; BC1: 2.25 Mgha−1 biochar; BC5: 11.25 Mgha−1 biochar; RS: 2.25 Mgha−1 rice straw.
Relative Abundance of Soil Bacterial Community
At the phylum level, long-term successive biochar application significantly altered the soil bacterial relative abundance (Figure 3). OTUs were classified as 501 genera belonging to 29 phyla, 76 classes, 167 orders, and 295 families across all soil samples. Overall, Proteobacteria (23.4%–36.3%), Acidobacteria (13.7%–35.8%), Cyanobacteria (1.3%–36.2%), Bacteroidetes (5.1%–14.8%), and Chloroflexi (6.0%–8.6%) were the five most dominant phyla, accounting for more than 75% of soil bacterial composition. Furthermore, the occupation rate of 8 phyla (Planctomycetes, Nitrospirae, Actinobacteria, Gemmatimonadetes, Ignavibacteriae, Latescibacteria, Firmicutes, and Chlorobi) was lower than 10% but exceeded 1% of the total composition (Figure 3). However, the composition of the bacterial community differed between the biochar and rice straw soils (p < 0.05). The most abundant phylum was Proteobacteria in CK (34.1%), BC1 (36.3%), and BC5 (31.4%), while Cyanobacteria was the most dominant phylum accounting for 23.4% in RS. The relative abundance of Cyanobacteria, Bacteroidetes, and Actinobacteria increased while that of Proteobacteria, Acidobacteria, and Nitrospirae decreased with increased biochar application. Other bacterial relative abundance changed slightly owing to long-term successive biochar amendment. Furthermore, compared to CK, rice straw returning decreased the relative abundance of Proteobacteria, Acidobacteria, Bacteroidetes, and Nitrospirae, but Cyanobacteria enhanced it (Figure 3).
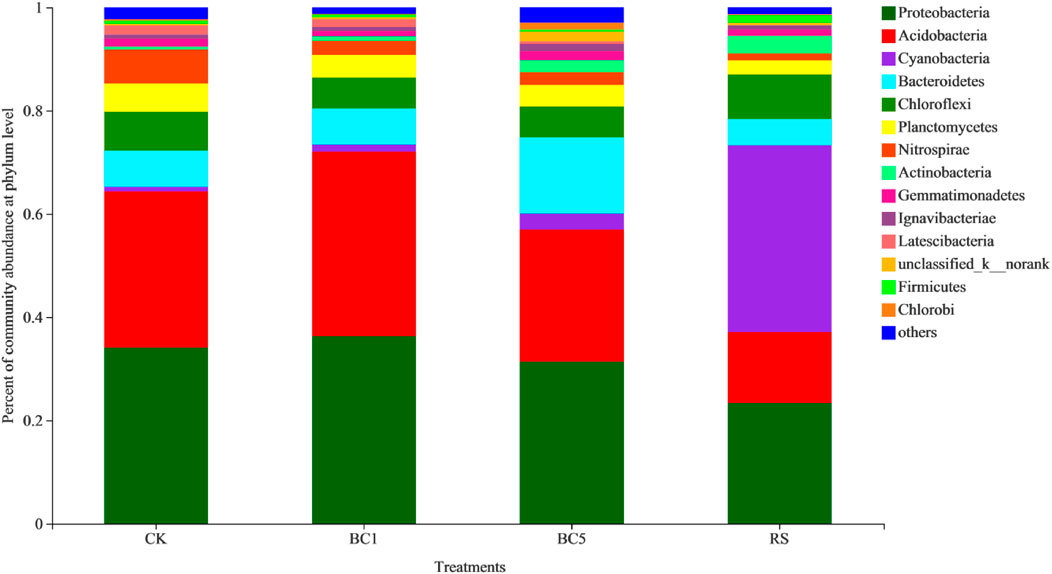
FIGURE 3. Relative abundance of dominant phyla as affected by the long-term successive biochar and rice straw amendment. CK: without biochar or rice straw; BC1: 2.25 Mgha−1 biochar; BC5: 11.25 Mgha−1 biochar; RS: 2.25 Mgha−1 rice straw.
The T-test was performed in different soils to determine the relative abundance of bacterial communities, which showed that at the phylum level, biochar had varying effects (Figures 4A–C). Compared with CK, the relative abundance of Nitrospirae, Gemmatimonadetes, and Tectomicrobia decreased significantly in BC1 (p < 0.05, Figure 4A); the relative abundance of Nitrospirae and Latescibacteria decreased significantly in BC5 (p < 0.05, Figure 4B); the relative abundance of Acidobacteria, Planctomycetes, Nitrospirae, and Latescibacteriae (p < 0.05; p < 0.01) decreased significantly, while that of Cyanobacteria and Actinobacteria increased significantly in RS (p < 0.05, Figure 4C).
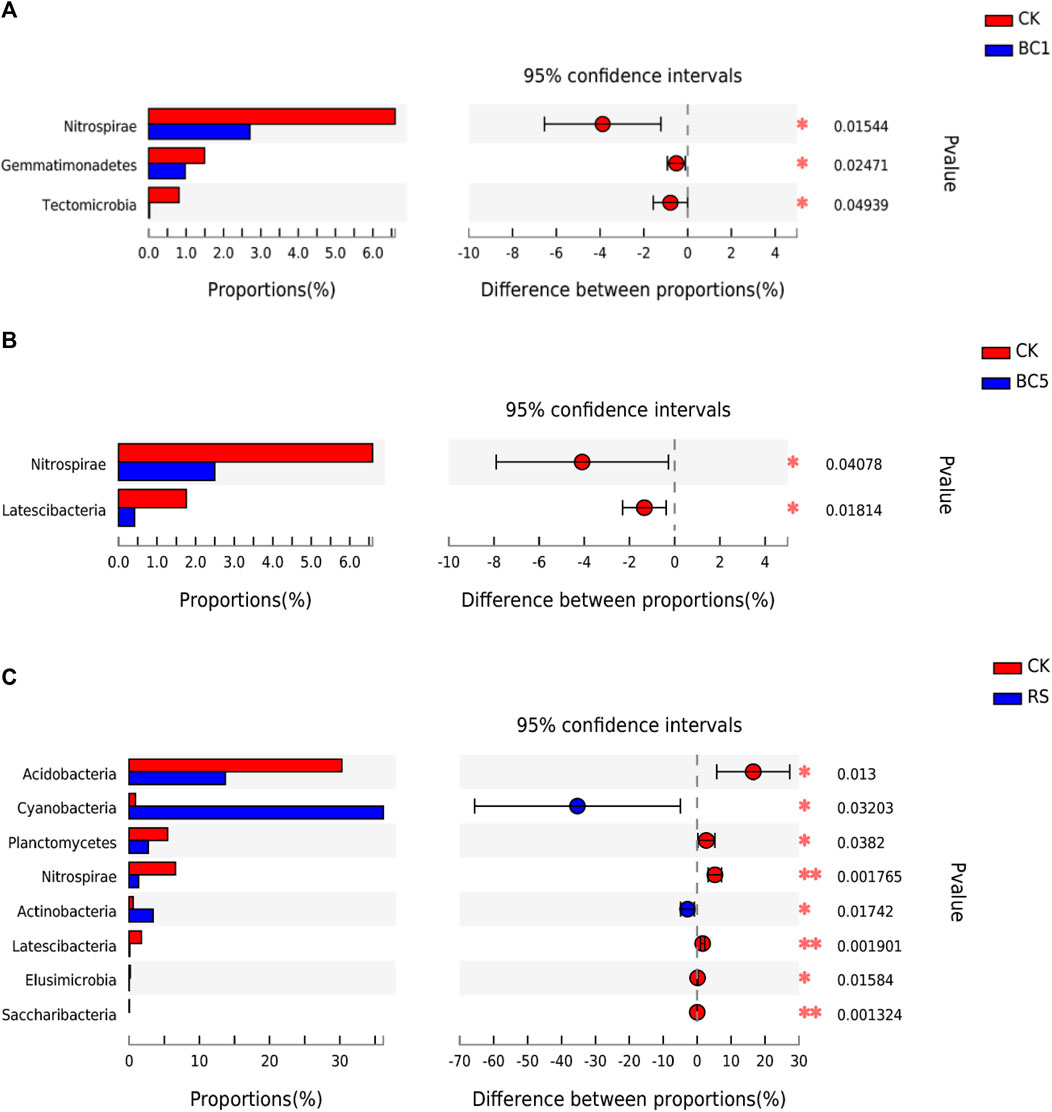
FIGURE 4. Soil bacterial phyla with significant differences among CK and BC1 (A), BC5 (B), and RS (C). CK: without biochar or rice straw; BC1: 2.25 Mgha−1 biochar; BC5: 11.25 Mgha−1 biochar; RS: 2.25 Mgha−1 rice straw. **p < 0.01 and *p < 0.05.
Norank_c__Acidobacteria (3.9%–12.5%), norank_f__Anaerolineaceae (4.1%–6.7%), norank_f__Nitrosomonadaceae (1.9%–6.9%), RB41 (1.0%–7.4%) and Nitrospira (1.3%–6.6%) were the five dominant genera across all soils (Figure 5). However, certain differences were observed between the biochar and rice straw soils (p < 0.05); for example, the most abundant genus was norank_c__Acidobacteria in CK (12.5%), BC1 (11.5%), and BC5 (6.9%), whereas Microcoleus was the most dominant genus account for 16.6% in RS. The relative abundance of norank_c__Acidobacteria, norank_f__Anaerolineaceae and Nitrospira decreased by 8.0%–45.2%, 29.9%–34.0%, and 58.9%–62.1%, respectively. Other genes changed diversely by long-term successive biochar amendment. Furthermore, compared to CK, RS decreased the relative abundance of norank_c__Acidobacteria, norank_f__Nitrosomonadaceae, and Nitrospirae, but enhanced norank_f__Anaerolineaceae and Microcoleus drastically (Figure 5).
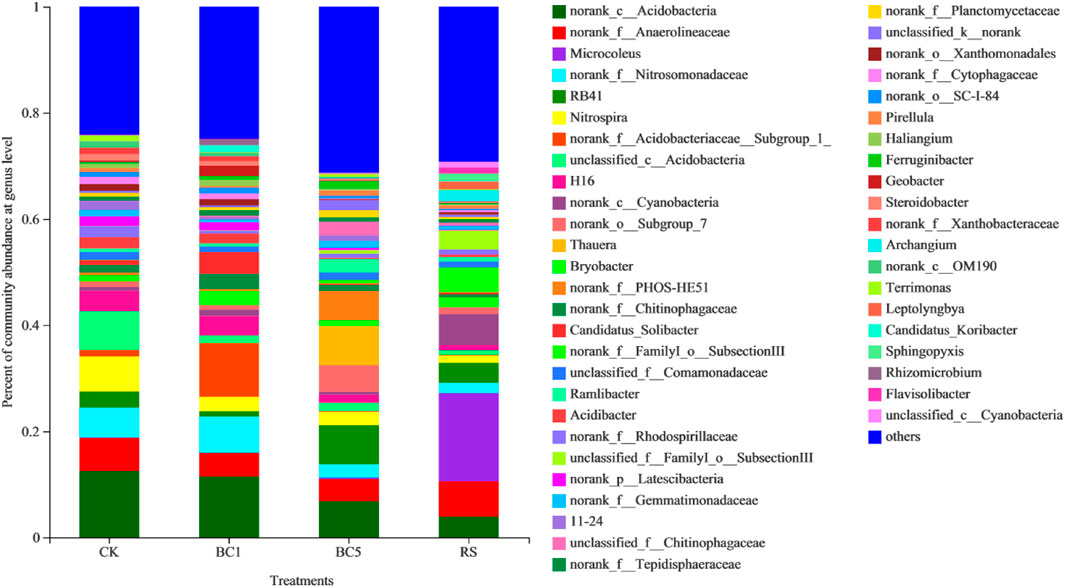
FIGURE 5. Relative abundance of dominant genera as affected by the long-term successive biochar and rice straw amendment. CK: without biochar or rice straw; BC1: 2.25 Mgha−1 biochar; BC5: 11.25 Mgha−1 biochar; RS: 2.25 Mgha−1 rice straw.
The bacteria in the top 50 of rice-wheat rotation soil were used to construct a community heat map at the genus level (Figure 6). Microcoleus, Archangium, and SubsectionIII in BC5 and RS increased significantly, compared with CK and BC1. Chitinophagaceae was clearly enhanced after long-term biochar and rice straw amendment.
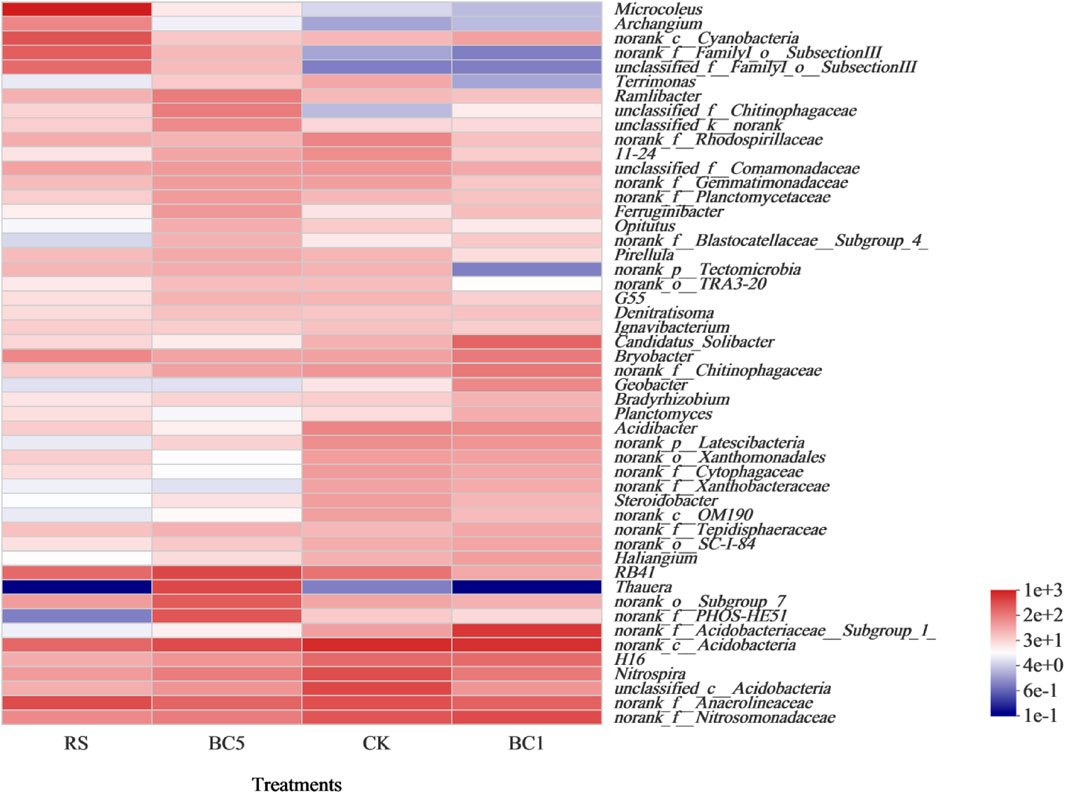
FIGURE 6. The heatmap of soil bacterial community at the genus level in soils. CK: without biochar or rice straw; BC1: 2.25 Mgha−1 biochar; BC5: 11.25 Mgha−1 biochar; RS: 2.25 Mgha−1 rice straw.
Soil Bacterial Community Structure and Correlation with Environmental Factors
Redundancy Analysis
To reveal the relationships between soil bacterial community composition and environmental parameters, the relative abundance of soil bacterial phyla and genera level were used as response variables, and soil pH, AP, AK, CEC, C/N, TN, and TOC were used as explanatory variables for RDA. As shown in Figure 7A, the RDA1 and RDA2 components illuminated 79.81% of the total variation (RDA1 illuminated 75.84% and RDA2 illuminated 3.97%). The pH, AP, AK, TN, TOC, CEC, and C/N significantly altered the soil bacterial community structure along RDA1and RDA2. The soil C/N and pH were two main environmental factors, which affected the soil bacterial community structure at the phylum level (Figure 7A). Figure 7B shows that the RDA1 and RDA2 components illuminated 67.49% of the total variation (RDA1 illuminated 51.28% and the RDA2 illuminated 16.21%). The soil pH and C/N were also two major environmental factors, which affected the soil bacterial community structure at the genus level (Figure 7B). Long-term successive biochar and rice straw application altered the soil bacterial community structure indirectly owing to the effect of soil properties in the rice-wheat rotation observed in RDA.
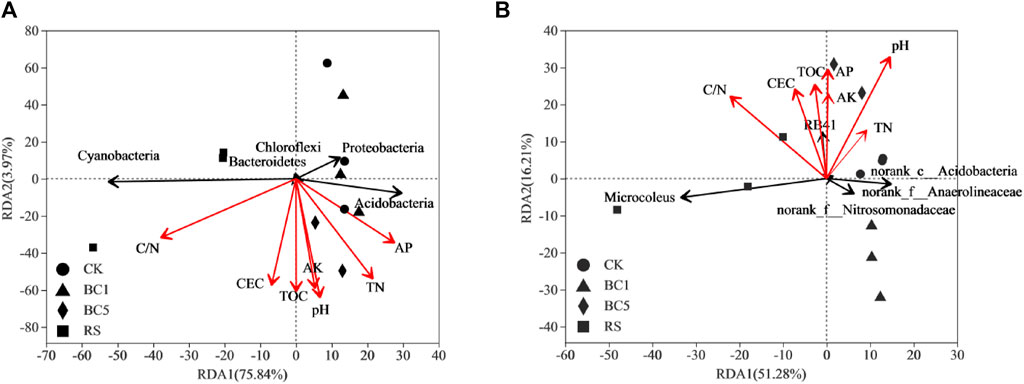
FIGURE 7. Redundancy analysis (RDA) of the relationship between the relative abundance of soil bacterial phyla (A) and genera (B) and environmental factors. CK: without biochar or rice straw; BC1: 2.25 Mgha−1 biochar; BC5: 11.25 Mgha−1 biochar; RS: 2.25 Mgha−1 rice straw. TN, total nitrogen; CEC, cation exchange capacity; TOC, total organic carbon; AK, available potassium; AP, available phosphorus.
Correlation Analysis
Spearman bivariate correlation analysis was performed on the top 15 bacterial phyla and environmental factors, which showed that soil’s physical and chemical properties affected the composition of the bacterial community (Figure 8A). Nitrospirae and Latescibacteria were negatively correlated with C/N, AK, TOC, and CEC (p < 0.05). Soil AK and C/N and the relative abundance of Latescibacteria (p < 0.01), were significantly negatively correlated (Figure 8A).
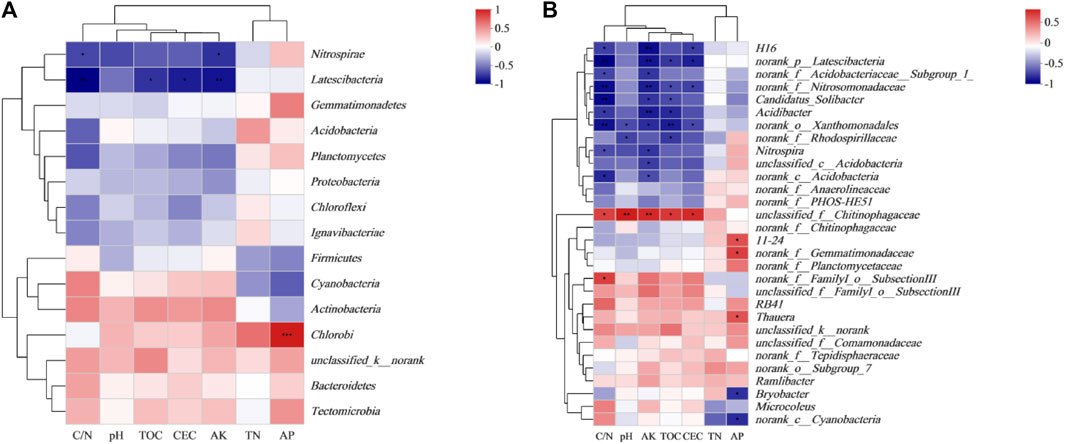
FIGURE 8. Correlations between dominant bacterial phyla (A) and genera (B) and soil chemical properties. CK: without biochar or rice straw; BC1: 2.25 Mgha−1 biochar; BC5: 11.25 Mgha−1 biochar; RS: 2.25 Mgha−1 rice straw. TN, total nitrogen; AK, available potassium; AP, available phosphorus; CEC, cation exchange capacity; TOC, total organic carbon. **p < 0.01 and *p < 0.05.
Spearman bivariate correlation analysis was performed on the top 30 bacterial genera and environmental factors (Figure 8B). The results showed that unclassified_f__Chitinophagaceae was positively correlated with CEC and TOC (p < 0.05), and was significantly positively correlated with pH and AK (p < 0.01). Meanwhile, many bacterial genera were negatively correlated with soil C/N, unclassified_c__Acidobacteria, norank_f__Acidobacteriaceae__Subgroup_1_, H16, Acidibacter, Nitrospira (p < 0.05, Figure 8B), norank_o__Xanthomonadales, norank_p__Latescibacteria, norank_f__Nitrosomonadaceae and Candidatus_Solibacter (p < 0.01) included. However, unclassified_f__Chitinophagaceae, and norank_f__FamilyI_o__SubsectionIII were positively correlated with C/N (p < 0.05, Figure 8B).
Prediction Results of Soil Bacterial Function
Phylogenetic investigation of communities by reconstruction of unobserved states (PICRUSt2) was performed to predict the function of the soil bacterial community. Six types of metabolic functions (level 1) were analyzed by comparison of Kyoto Encyclopedia of Genes and Genomes (KEGG) databases, including metabolism (77.26%–77.78%), genetic information processing (6.80%–7.30%), environmental information processing (5.20%–5.70%), cellular processes (4.40%–4.75%), human diseases (3.48%–3.77%), and organismal systems (1.75%–2.00%, Table 3).
At the same time, the second-order function prediction results showed that there were 46 pathways (level 2) in soil samples, including carbohydrate metabolism, transcription, and glycan biosynthesis and metabolism (Table 4). Major functional pathways with relative abundance exceeding 1% were selected for comparison, and there were 18 categories (one cellular process, two environmental information processing, three genetic information processing, and twelve metabolism).
The relative abundance of the secondary function of the soil bacterial community changed after biochar modification (Table 4). Biochar significantly increased the relative abundance information on the functions of the metabolism of terpenoids and polyketides, metabolism of other amino acids, and biosynthesis of other secondary metabolites (p < 0.05). Furthermore, biochar treatments had no significant differences (p < 0.05). The functions of the metabolism of terpenoids and polyketides, metabolism of cofactors and vitamins, energy metabolism, and membrane transport increased remarkably in soil bacterial community by rice-straw amendment, whereas the function of the amino acid metabolism, glycan biosynthesis, and metabolism, translation, and replication and repair decreased obviously (p < 0.05, Table 4).
Discussion
Long-term successive biochar and rice straw amendment in the rice-wheat rotation is an efficient solution for problems associated with wasteful agricultural and forestry resource management (Singh and Cowie, 2014). Furthermore, biochar application could improve soil fertility, thereby increasing carbon sinks and reducing emissions (Jones et al., 2011). Our study revealed the characteristics of soil fertility and bacterial α-diversity, the structure and predictive function of soil bacterial community following long-term successive straw biochar application for twelve crop seasons. In contrast to the short-term cultivation experiment, biochar applied to paddy soil changed constantly over time, along with its effects. Given China’s national straw conditions, long-term and continuous biochar and rice straw amendments in agricultural soil have more practical value.
After twelve crop seasons, the biochar and rice straw improved soil properties including pH, AK, TOC, TN, CEC, and C/N, except for AP and DOC (Table 1). Biochar is generally alkaline, but the pH of biochar derived from different raw materials varies from 6.2 to 10.0. The surface of biochar is rich in ash mineral elements (such as K, Na, Ca, and Mg), alkaline functional groups, carbonates, and surface negative charges, which increase soil acidity (Tan and Lin, 2019). On the one hand, previous reports have found that wood biochar could increase the pH of agricultural soil by 0.5–1.6 in field experiments (Gul et al., 2015). On the other hand, biochar was found to either have no effect or to decrease soil pH by being oxidized when applied to soil for a long time (Pfister and Saha, 2016). Our results were similar to those of the former study, with soil pH increasing by 0.22–1.35. This phenomenon may be caused by the straw biochar and rice straw being applied over the long term and successively for each crop season. Similarly, soil pH increased as biochar application increased, which was reported by Peng et al. (2011). Therefore, biochar at a high application rate (BC5) yielded a more optimized improvement of soil pH (Table 1). In this study, long-term successive biochar increased soil AK, which owing to biochar carries a large amount of potassium. With the increase in application time, the minerals contained in biochar were released and utilized continuously, and the porosity and structure of the soil were improved, then soil AK increased (Wang et al., 2015). The TOC content of biochar was higher than that of rice straw amendment, and the biochar properties were more stable and remained in the soil for a long time. Therefore, biochar on increasing soil TOC was more optimized than those of rice straws being returned directly. A previous study found that biochar drastically increased the soil DOC contentions within a short time, which showed a linear relationship with TOC (Ma et al., 2021). However, after six rice-wheat cycles, soil DOC content did not change significantly, and even decreased, which may have been caused by the decrease in soil DOC extraction efficiency owing to the adsorption of long-term successive biochar. Biochar (pyrolysis of spruce at 480°C) could significantly improve soil C/N, which was consistent with the results of the present study (Nelissen et al., 2015b). Moreover, biochar applied at high rates (BC5) showed a greater improvement in soil C/N than that prepared at low rates (BC1). Long-term similar agricultural operation measures showed that soil quality has been improved, such as reduced soil acidity and increased nutrients for crop growth. In terms of crop yields, long-term biochar plays a beneficial role in crop health (Moorman and Dowler, 1991). But considering the different preparation materials and methods of biochar, their specific results need to be further studied.
Our results indicated that the long-term application of successive biochar and rice straw was not conducive to improving the α-diversity of soil bacteria in the rice-wheat rotation. The α-diversity index of bacteria reveals the richness and distribution of the soil microorganism community. Furthermore, the index is higher, the richness more optimized, and the microbial ecosystem function is more complex and stable. As one of evaluating the total number of species, Chao1 and ACE indexes are positively correlated with bacterial community richness. Shannon and Simpson indexes reflect the evenness of the bacterial community. Our research showed that OTU numbers and α-diversity indexes, such as Chao1, ACE, and Shannon, following treatment with different amounts of biochar (BC1 and BC5) and rice straw were lower than those of the control (Table 2). Likewise, Marris (2006) found that biochar could reduce the diversity and abundance of soil microorganisms. These results might have been caused by biochar’s limited soil nutrient content, which could be directly utilized by microorganisms. Large amounts of biochar stimulated the soil microbial community, possibly because it reduced soil acidity drastically (Pietikainen et al., 2000; Smebye et al., 2016), and the more nutrients contained in biochar, the more suitable the living environment for microorganisms (Zhu et al., 2017). However, in this study, BC5 had a greater decreased rate in soil bacterial diversity than BC1. This was attributed to the easy absorption of additional heavy metals and other substances that were detrimental to the survival of bacteria (Liu et al., 2018). The results of bacterial community β-diversity indicated that long-term biochar and rice straw application could promote the development of soil bacterial structure in a specific direction, which was similar to the results of Zhang et al. (2020). PCoA results of soils amended with biochar and rice straw return and the control soil were different, which indicated that long-term successive biochar changed the composition of the soil bacterial community, and different application rates of biochar had different effects. The result is similar to that of many previous studies, which reported that soil bacterial community structure altered after biochar addition (Kookana et al., 2011; Doan et al., 2014; Liu et al., 2016). Meanwhile, bacterial community separation was observed in different treatments (Figure 2). This may be because long-term biochar and rice straw affected the soil ecology environment continuously, which differs from the short-term effects of biochar.
Long-term successive biochar application altered the soil environmental factors and then affected bacterial community composition indirectly. In this study, Proteobacteria, Acidobacteria, Cyanobacteria, Bacteroidetes, and Chloroflexi were the five dominant phyla in the bacterial community across all soils. Our result was similar to that of Guo et al. (2017), who reported that Proteobacteria, Acidobacteria, and Chloroflexi were the three dominant bacterial communities, whose relative abundance was 37.3%, 19.5%, and 12.7%, respectively. Proteobacteria was the most dominant bacterial phylum, with a relative abundance ranging from 23.7% to 36.9% (Figure 3), which was consistent with the results by Fan et al. (2020). However, Chen et al. (2016) reported that the dominant phylum was Chloroflexi, with a relative abundance of 35% in arable soil in China. Most of the members of Proteobacteria and Chloroflexi are facultative anaerobic bacteria, which prefer anaerobic environments and are dominant during the rice season (Zhao J et al., 2014). However, our study inhibited the relative abundance of Chloroflexi in the rice-wheat rotation because long-term successive biochar increased soil permeability and was not conducive to the existence of an anaerobic environment. Acidobacteria contains oligotrophic bacteria that prefer soils with low organic carbon content (Fierer et al., 2007; Wang et al., 2017). In this study, biochar added to soil increased the soil pH and TOC content, which might inhibit the growth of Acidobacteria. Moreover, norank_c__Acidobacteria, norank_f__Anaerolineaceae, norank_f__Nitrosomonadaceae, RB41, and Nitrospira were the five dominant genera across all soils. RB41 plays a crucial role in keeping soil metabolism and ecological balance under low nutrient or stress conditions, and their increase in BC5 and RS could indicate that biochar improved soil nutritional conditions (Foesel et al., 2012). Nitrospira is closely related to the soil nitrogen cycle and is the main group involved in nitrite oxidation and the C-N cycle (You et al., 2009). In this study, long-term application of biochar and rice straw reduced the relative abundance of Nitrospira. The reason might be that high soil C/N inhibited the activity of Nitrospira, which was similar to the findings of Cheng et al. (2012). The effects of long-term successive straw and biochar returning on beneficial and pathogenic bacteria community of the soil in the rice-wheat rotation system are not clear yet, and it needs to be studied further.
Many studies have revealed that pH significantly affected the composition of the soil bacterial community (Xiong et al., 2012; An et al., 2019). Our study is consistent with these results as evidenced by RDA. Furthermore, bacterial community composition was connected with other environmental factors, including TOC, AK, AP, TN, CEC, and C/N. C/N and pH were the two main environmental factors influencing the soil bacterial community structure in this study. C/N, pH, AK, AP, CEC, and TOC had different correlations with different bacteria in the spearman bivariate correlation analysis, which was speculated to mean different bacteria had different living environments. Meanwhile, TN affected the relative abundance of bacteria. Generally, different bacteria have different physiological characteristics and living habits and show different preferences for soil environmental factors (Zhao et al., 2018).
Moreover, the soil bacteria community mainly involved 6 primary functional levels and 46 secondary functional levels in rice-wheat rotation by PICRUSt2 analysis. Biochar significantly increased the relative abundance of information on the functions of the metabolism of terpenoids and polyketides, metabolism of other amino acids, and biosynthesis of other secondary metabolites (p < 0.05). This study showed that the function of the soil bacterial community was altered by the effects of long-term biochar application on soil bacterial community structure (Landesman et al., 2014). However, PICRUSt2 analysis is limited to the KEGG database, which can be combined with metagenomic technology to examine the effect of biochar application on bacterial community function. At the same time, we should strengthen the dynamic observation of long-term biochar and straw returning on the soil microbial community in the rice-wheat rotation system.
Conclusion
In this study, we evaluated the response of the composition and α-diversity of bacterial community to successive biochar application to paddy soils for 12 consecutive crop seasons in a typical rice-wheat rotation system in China. The results found that the dominant bacterial phyla were Proteobacteria, Acidobacteria, Cyanobacteria, Bacteroidetes, and Chloroflexi, and the dominant bacterial genera were norank_c__Acidobacteria, norank_f__Anaerolineaceae, norank_f__Nitrosomonadaceae, RB41, and Nitrospira in all treatments. While the relative abundances of Cyanobacteria, Bacteroidetes, and Actinobacteria increased while those of Proteobacteria, Acidobacteria, Nitrospirae, norank_c__Acidobacteria, norank_f__Anaerolineaceae, and Nitrospira decreased as biochar application increased. RDA results revealed that pH and C/N were two major environmental parameters that altered the soil bacterial community composition in rice-wheat rotation. PICRUSt2 showed that biochar significantly increased the relative abundance of information on the functions of the metabolism of other amino acids, metabolism of terpenoids and polyketides, and biosynthesis of other secondary metabolites (p < 0.05), which may be related to the improvement of soil pH, AK, TOC, TN, and CEC. The response of the diversity and composition of the soil bacterial community to biochar is complex and is related to the type, amount, age, and soil type of biochar. Meanwhile, to identify the environmental risk to soil ecology, long-term continuous application of biochar should be studied further.
Data Availability Statement
The datasets presented in this study can be found in online repositories. The names of the repository/repositories and accession number(s) can be found below: NCBI, accession number PRJNA831553.
Author Contributions
YX contributed to conducting experiments, analyzing data, and writing and editing the original draft. LH contributed to designing the experiments, and reviewing and editing the original draft. JC contributed to the investigation, methodology, and formal analysis. HL contributed to software and formal analysis. YW contributed to software and formal analysis. LY contributed to software and formal analysis. SY contributed to conceptualization, supervision, and funding acquisition. YL contributed to reviewing and editing the original draft and funding acquisition. All authors contributed to manuscript revision, read, and approved the submitted version.
Funding
This work was supported by the Natural Science Foundation of Zhejiang (LY21D010003; LY20D010005) and State Key Laboratory for Managing Biotic and Chemical Threats to the Quality and Safety of Agro-products.
Acknowledgments
Thanks to the medical staff who worked day and night under the COVID-19 epidemic to ensure that I could finish the paper well-securely.
Conflict of Interest
The authors declare that the research was conducted in the absence of any commercial or financial relationships that could be construed as a potential conflict of interest.
Publisher’s Note
All claims expressed in this article are solely those of the authors and do not necessarily represent those of their affiliated organizations, or those of the publisher, the editors and the reviewers. Any product that may be evaluated in this article, or claim that may be made by its manufacturer, is not guaranteed or endorsed by the publisher.
References
An, J., Liu, C., Wang, Q., Yao, M., Rui, J., Zhang, S., et al. (2019). Soil bacterial community structure in Chinese wetlands. Geoderma 337, 290–299. doi:10.1016/j.geoderma.2018.09.035
Chen, C., Zhang, J. N., Lu, M., Qin, C., Chen, Y. H., Yang, L., et al. (2016). Microbial communities of an arable soil treated for 8 years with organic and inorganic fertilizers. Biol. Fertil. Soils. 52, 455–467. doi:10.1007/s00374-016-1089-5
Chen, J. H., Liu, X. Y., Zheng, J. W., Zhang, B., Lu, H. F., Chi, Z. Z., et al. (2013). Biochar soil amendment increased bacterial but decreased fungal gene abundance with shifts in community structure in a slightly acid rice paddy from Southwest China. Agric. Ecosyst. Environ. Appl. Soil Ecol. 71, 33–44.
Cheng, Y., Cai, Z. C., Chang, S. X., Wang, J., and Zhang, J. B. (2012). Wheat straw and its biochar have contrasting effects on inorganic N retention and N2O production in a cultivated Black Chernozem. Biol. Fertil. Soils. 48, 941–946. doi:10.1007/s00374-012-0687-0
Clough, T. J., Bertram, J. E., Ray, J. L., Condron, L. M., O'Callaghan, M., Sherlock, R. R., et al. (2010). Unweathered wood biochar impact on nitrous oxide emissions from a bovine-urine-amended pasture soil. Soil Sci. Soc. Am. J. 74, 852–860. doi:10.2136/sssaj2009.0185
Dempster, D. N., Gleeson, D. B., Solaiman, Z. M., Jones, D. L., and Murphy, D. V. (2012). Decreased soil microbial biomass and nitrogen mineralisation with Eucalyptus biochar addition to a coarse textured soil. Plant Soil 354, 311–324. doi:10.1007/s11104-011-1067-5
Doan, T. T., Bouvier, C., Bettarel, Y., Bouvier, T., Henry-des-Tureaux, T., Janeau, J. L., et al. (2014). Influence of buffalo manure, compost, vermicompost and biochar amendments on bacterial and viral communities in soil and adjacent aquatic systems. Appl. Soil Ecol. 73, 78–86. doi:10.1016/j.apsoil.2013.08.016
Falkowski, P. G., Fenchel, T., and Delong, E. F. (2008). The microbial engines that drive earth's biogeochemical cycles. Science 320, 1034–1039. doi:10.1126/science.1153213
Fan, S. X., Zuo, J. C., and Dong, H. Y. (2020). Changes in soil properties and bacterial community composition with biochar amendment after six years. Agronomy 10, 746. doi:10.3390/agronomy10050746
Fierer, N., Bradford, M. A., and Jackson, R. B. (2007). Toward an ecological classification of soil bacteria. Ecology 88, 1354–1364. doi:10.1890/05-1839
Foesel, B. U., Rohde, M., and Overmann, J. (2012). Blastocatella fastidiosa gen. nov., sp. nov., isolated from semiarid savanna soil-The first described species of Acidobacteria subdivision 4. Syst. Appl. Microbiol. 36, 82–89.
Gul, S., Whalen, J. K., Thomas, B. W., Sachdeva, V., and Deng, H. Y. (2015). Physico-chemical properties and microbial responses in biochar-amended soils: Mechanisms and future directions. Agric. Ecosyst. Environ. 206, 46–59. doi:10.1016/j.agee.2015.03.015
Guo, J. J., Liu, W. B., Zhu, C., Luo, G. W., Kong, Y. L., Ling, N., et al. (2017). Bacterial rather than fungal community composition is associated with microbial activities and nutrient-use efficiencies in a paddy soil with short-term organic amendments. Plant Soil 424, 335–349. doi:10.1007/s11104-017-3547-8
Hailegnaw, N. S., Mercl, F., Pračke, K., Száková, J., and Tlustoš, P. (2019). Mutual relationships of biochar and soil pH, CEC, and exchangeable base cations in a model laboratory experiment. J. Soils Sediments. 19, 2405–2416. doi:10.1007/s11368-019-02264-z
Huang, W., Wu, J. F., Pan, X. H., Tan, X., M., and Zeng, Y. J. (2021). Effects of long-term straw return on soil organic carbon fractions and enzyme activities in a double-cropped rice paddy in south China. J. Integr. Agr. 20, 236–247. doi:10.1016/s2095-3119(20)63347-0
Jones, D. L., Murphy, D. V., Khalid, M., Ahmad, M., Edwards-Jones, G., and Deluca, T. L. (2011). Short-term biochar-induced increase in soil CO2 release is both biotically and abiotically mediated. Soil Biol. biochem. 43, 1723–1731. doi:10.1016/j.soilbio.2011.04.018
Kennedy, A. C., and Smith, K. L. (1995). Soil microbial diversity and the sustainability of agricultural soils. Plant Soil 170, 75–86. doi:10.1007/bf02183056
Kookana, R. S., Sarmah, A. K., VanZwieten, L., Krull, E., and Singh, B. (2011). Biochar application to soil: Agronomic and environmental benefits and unintended consequences. Adv. Agron. 112, 103–143. doi:10.1016/b978-0-12-385538-1.00003-2
Landesman, W. J., Nelson, D. M., and Fitzpatrick, M. C. (2014). Soil properties and tree species drive β diversity of soil bacterial communities. Soil Biol. biochem. 76, 20–209. doi:10.1016/j.soilbio.2014.05.025
Li, M., Liu, M., Li, Z. P., Jiang, C. Y., and Wu, M. (2016). Soil N transformation and microbial community structure as affected by adding biochar to a paddy soil of subtropical China. J. Integr. Agr. 15, 209–219. doi:10.1016/s2095-3119(15)61136-4
Lin, Y. T., Whitman, W. B., Cpleman, D., Jien, S. H., and Chiu, C. Y. (2017). Cedar and bam- boo plantations alter structure and diversity of the soil bacterial community from a hardwood forest in subtropical mountain. Agric. Ecosyst. Environ. Appl. Soil Ecol. 112, 28–33. doi:10.1016/j.apsoil.2017.01.001
Liu, X. R., Li, J., Yu, L., Pan, H., Liu, H. Y., Liu, Y. M., et al. (2018). Simultaneous measurement of bacterial abundance and composition in response to biochar in soybean field soil using 16S rRNA gene sequencing. Land Degrad. Dev. 29, 2172–2182. doi:10.1002/ldr.2838
Liu, Y. X., Lu, H. H., Yang, S. G., and Wang, Y. F. (2016). Impacts of biochar addition on rice yield and soil properties in a cold waterlogged paddy for two crop seasons. Field Crops Res. 191, 161–167. doi:10.1016/j.fcr.2016.03.003
Ma, L. J., Min, W., Guo, H. J., Li, Q., Xu, W. X., and Hou, Z. N. (2021). Response of soil organic c fractions and enzyme activity to integrating n fertilization with cotton stalk or its biochar in a drip-irrigated cotton field. Acta. Agr. Scand. B-S p. 71, 1–14. doi:10.1080/09064710.2020.1853214
Marris, E. (2006). Putting the carbon back: Black is the new green. Nature 442, 624–626. doi:10.1038/442624a
Marschner, P., and Rengel, Z. (2007). The role of crop residues in improving soil fertility. Springer 10, 183–214.
Moorman, T. B., and Dowler, C. C. (1991). Herbicide and rotation effects on soil and rhizosphere microorganisms and crop yields. Agric. Ecosyst. Environ. 35, 311–325. doi:10.1016/0167-8809(91)90081-8
Nan, X., Tan, G. C., Wang, H. Y., and Gai, X. P. (2016). Effect of biochar additions to soil on nitrogen leaching, microbial biomass and bacterial community structure. Eur. J. Soil Biol. 74, 1–8.
Nelissen, V., Rütting, T., Huygens, D., Ruysschaert, G., and Boeckx, P. (2015a). Temporal evolution of biochar’s impact on soil nitrogen processes: A 15N tracing study. Glob. Change Biol. Bioenergy 7, 635–645. doi:10.1111/gcbb.12156
Nelissen, V., Ruysschaert, G., Manka’Abusi, D., D’Hose, T., Beuf, K. D., Al-Barri, B., et al. (2015b). Impact of a woody biochar on properties of a sandy loam soil and spring barley during a two-year field experiment. Eur. J. Agron. 62, 65–78. doi:10.1016/j.eja.2014.09.006
Noyce, G. L., Basiliko, N., Fulthorpe, R., Sackett, T. E., and Thomas, S. C. (2015). Soil microbial responses over 2 years following biochar addition to a north temperate forest. Biol. Fertil. Soils. 51, 649–659. doi:10.1007/s00374-015-1010-7
Peng, X., Ye, L. L., Wang, C. H., Zhou, H., and Sun, B. (2011). Temperature and duration dependent rice straw-derived biochar: Characteristics and its effects on soil properties of an Ultisol in southern China. Soil Tillage Res. 112, 159–166. doi:10.1016/j.still.2011.01.002
Pfister, M., and Saha, S. (2016). Effects of biochar and fertilizer management on sunflower (helianthus annuus l.) feedstock and soil properties. Arch. Agron. Soil Sci. 63, 651–662. doi:10.1080/03650340.2016.1228894
Pietikainen, J., Kiikkila, O., and Fritze, H. (2000). Charcoal as a habitat for microbes and its effect on the microbial community of the underlying humus. Oikos 89, 231–242. doi:10.1034/j.1600-0706.2000.890203.x
Schmidt, M. W. I., Skjemstad, J. O., Czimczik, C. I., Glaser, B., Prentice, K. M., Gelinas, Y., et al. (2001). Comparative analysis of black carbon in soils. Glob. Biogeochem. Cycles. 15, 163–167. doi:10.1029/2000gb001284
Singh, B. P., and Cowie, A. L. (2014). Long-term influence of biochar on native organic carbon mineralisation in a low-carbon clayey soil. Sci. Rep. 4, 3687. doi:10.1038/srep03687
Smebye, A., Alling, V., Vogt, R. D., Gadmar, T. C., Mulder, J., Cornelissen, G., et al. (2016). Biochar amendment to soil changes dissolved organic matter content and composition. Chemosphere 142, 100–105. doi:10.1016/j.chemosphere.2015.04.087
Tan, W. F., and Lin, Q. M. (2019). Ecological functions of biochar. J. Integr. Agr. 18, 59. doi:10.1016/b978-0-12-815071-9.00003-8
Wang, J. C., Song, Y., Ma, T. F., Raza, W., Li, J., Howland, J. G., et al. (2017). Impacts of inorganic and organic fertilization treatments on bacterial and fungal communities in a paddy soil. Agric. Ecosyst. Environ. Appl. Soil Ecol. 112, 42–50. doi:10.1016/j.apsoil.2017.01.005
Wang, Q., Garrity, G. M., Tiedje, J. M., and Cole, J. R. (2007). Nave Bayesian classifier for rapid assignment of rRNA sequences into the new bacterial taxonomy. Appl. Environ. Microbiol. 73, 5261–5267. doi:10.1128/aem.00062-07
Wang, X. B., Zhou, W., Liang, G. Q., Song, D. L., and Zhang, X. Y. (2015). Characteristics of maize biochar with different pyrolysis temperatures and its effects on organic carbon, nitrogen and enzymatic activities after addition to fluvo-aquic soil. Sci. Total Environ. 538, 137–144. doi:10.1016/j.scitotenv.2015.08.026
Xiong, J. B., Liu, Y. Q., Lin, X. G., Zhang, H. Y., Zeng, J., Hou, J. Z., et al. (2012). Geographic distance and pH drive bacterial distribution in alkaline lake sediments across Tibetan Plateau. Environ. Microbiol. 14, 2457–2466. doi:10.1111/j.1462-2920.2012.02799.x
You, J., Das, A., Dolan, E. M., and Hu, Z. Q. (2009). Ammonia–oxidizing archaea involved in nitrogen removal. Water Res. 43, 1801–1809. doi:10.1016/j.watres.2009.01.016
Yuan, H. Z., Zhu, Z. K., Wei, X. M., Liu, S. L., Peng, P. Q., Gunina, A., et al. (2019). Straw and biochar strongly affect functional diversity of microbial metabolism in paddy soils. J. Integr. Agr. 18, 60–71. doi:10.1016/s2095-3119(18)62102-1
Zang, H. M., Wang, B. R., and Xu, M. G. (2008). Effects of inorganic fertilizer inputs on grain yields and soil properties in a long-term wheat-corn cropping system in south China. Commun. Soil Sci. Plant Anal. 39, 1583–1599.
Zeng, X. Y., Ma, Y. T., and Ma, L. R. (2007). Utilization of straw in biomass energy in China. Renew. Sust. Energy Rev. 11, 976–987. doi:10.1016/j.rser.2005.10.003
Zhang, Q., Zhang, Y., Zhang, X., and Shi, J. (2020). Effects of biochar on bacterial genetic diversity in soil contaminated with cadmium. Soil. Use manage. 37, 289–298.
Zhao, J., Ni, T., Li, Y., Xiong, W., Ran, W., Shen, B., et al. (2014). Responses of bacterial communities in arable soils in a rice-wheat cropping system to different fertilizer regimes and sampling times. PLoS ONE 9, e85301. doi:10.1371/journal.pone.0085301
Zhao, S., Liu, J. J., Banerjee, S., Zhou, N., Zhao, Z. Y., Zhang, K., et al. (2018). Soil pH is equally important as salinity in shaping bacterial communities in saline soils under halophytic vegetation. Rep 8, 4550. doi:10.1038/s41598-018-22788-7
Zhao, X., Wang, J. W., Wang, S. Q., and Xing, G. X. (2014). Successive straw biochar application as a strategy to sequester carbon and improve fertility: A pot experiment with two rice/wheat rotations in paddy soil. Plant Soil 378, 279–294. doi:10.1007/s11104-014-2025-9
Zheng, J. F., Chen, J. H., Pan, G. X., Liu, X. Y., Zhang, X. H., Li, L. Q., et al. (2016). Biochar decreased microbial metabolic quotient and shifted community composition four years after a single incorporation in a slightly acid rice paddy from southwest China. Sci. Total Environ. 571, 206–217. doi:10.1016/j.scitotenv.2016.07.135
Zhu, L. Q., Li, J., Tao, B. R., and Hu, N. J. (2015). Effect of different fertilization modes on soil organic carbon sequestration in paddy fields in south China: A meta-analysis. Ecol. Indic. 53, 144–153. doi:10.1016/j.ecolind.2015.01.038
Keywords: rice-wheat rotation, biochar, long-term, high-throughput sequencing, bacterial community
Citation: Xu Y, He L, Chen J, Lyu H, Wang Y, Yang L, Yang S and Liu Y (2022) Long-Term Successive Biochar Amendments Alter the Composition and α-Diversity of Bacterial Community of Paddy Soil in Rice-Wheat Rotation. Front. Environ. Sci. 10:921766. doi: 10.3389/fenvs.2022.921766
Received: 16 April 2022; Accepted: 09 June 2022;
Published: 16 August 2022.
Edited by:
Lu-Jun Li, Northeast Institute of Geography and Agroecology (CAS), ChinaReviewed by:
Xianqing Zheng, Shanghai Academy of Agricultural Sciences, ChinaJun Zhao, Nanjing Normal University, China
Copyright © 2022 Xu, He, Chen, Lyu, Wang, Yang, Yang and Liu. This is an open-access article distributed under the terms of the Creative Commons Attribution License (CC BY). The use, distribution or reproduction in other forums is permitted, provided the original author(s) and the copyright owner(s) are credited and that the original publication in this journal is cited, in accordance with accepted academic practice. No use, distribution or reproduction is permitted which does not comply with these terms.
*Correspondence: Shengmao Yang, eWFuZ3NoZW5nbWFvQDI2My5uZXQ=; Yuxue Liu, bGl1eXV4dWU1MDVAMTYzLmNvbQ==
†These authors have contributed equally to this work