- 1Department of Geography, Faculty of Philosophy, University of East Sarajevo, East Sarajevo, Bosnia and Herzegovina
- 2Department of Geography, Faculty of Philosophy, University of Montenegro, Nikšić, Montenegro
- 3Department of Geospatial and Environmental Science, Faculty of Geography, University of Belgrade, Belgrade, Serbia
- 4Department of Plant Production, Faculty of Agriculture, University of East Sarajevo, East Sarajevo, Bosnia and Herzegovina
- 5Department of Forestry, Faculty of Agriculture, University of East Sarajevo, East Sarajevo, Bosnia and Herzegovina
- 6PhD Candidate of Faculty of Geography, University of Belgrade, Belgrade, Serbia
- 7Department of Geography, Faculty of Science, University of Sarajevo, Sarajevo, Bosnia and Herzegovina
- 8Department of Management, Faculty of Applied Sciences, Western Serbia Academy of Applied Studies, Užice, Serbia
Spatial assessment of soil erosion is an important indicator of ecological soil change and global environmental changes. This is especially true for countries with rich forest cover such as Bosnia and Herzegovina. In this study, the risk of soil erosion was assessed using the Revised Universal Soil Loss Equation (RUSLE) model and the impact of changes in the forest ecosystem, current conditions were compared with possible future forest management scenarios, and measures and solutions were proposed to reduce soil erodibility in vulnerable areas of the Pale Municipality in Bosnia and Herzegovina. The studied area is at increased risk of soil erosion due to natural conditions (mountain relief, climate change, and the frequency of extreme climatic events—drought and heavy rains, which occur more and more frequently in a short period of time) and due to anthropogenic factors, such as large-scale deforestation and conversion of mountain areas for tourism purposes, tracing and construction of ski slopes and ski resorts in general, and expansion of settlements. All this leads to threats to water conservation areas, landslides, floods, forest fires, and additional reduction of forest areas due to drying of forests and expansion of settlements. GIS as a tool provides us with a quick and accurate way to find possible solutions to problems resulting from the intensive use and inadequate monitoring. In this study, we have tried to offer possible solutions and show the benefits that can be obtained by varying the factors that affect soil erodibility and depend on vegetation cover, that is, land use (C-factor). This study presents the application of RUSLE methods in combination with GIS for the purpose of planning economic activities, such as winter tourism development in the community of Pale. An increase in soil loss due to inappropriate land use was found, with the average annual soil loss due to deforestation in the ski area increasing to 909.43 t ha−1 year−1.
1 Introduction
As a result of inadequate land-use planning on mountain slopes in temperate climates, due to large slope areas and extensive deforestation problems, as well as the rapid development of certain economic activities such as winter sport tourism, there is a significant process of soil degradation. The development of ski slopes and the construction of infrastructures for the development of ski centers make mountain areas very vulnerable facing significant soil erosion due to the following factors: constant deforestation, large longitudinal slopes, construction works, the so-called arrangement of ski slopes (disturbance of the now undisturbed plateaus on the slopes), and to the strong surface runoff expressed in the season of snow melting and rainstorm. Therefore, it is necessary to anticipate the negative effects caused by soil erosion in such areas and take measures to minimize them.
Soil erosion is a significant environmental problem that has serious consequences for human society and the economy. In European countries, eight main processes are responsible for soil degradation, of which soil erosion is considered the most important and widespread process (European Commision, 2004). According to Morgan (2005), the occurrence of erosion processes, their distribution, and the timing of their occurrence are closely linked to anthropogenic factors such as local social, economic, and political conditions.
At the global level, both agriculture and forestry, as also natural resources are affected by soil erosion (Pimentel et al., 1995; Bakker et al., 2005; Prasannakumar et al., 2012). Soil erosion as a process of land degradation can be affected by both natural and anthropogenic activities. Some authors state that nearly half of the Earth’s topsoil has been lost in the last 150 years (Chandra Pal and Chakrabortty, 2018). Hazards such as heavy rainfall and water runoff on bare soils cause serious erosion leading to soil degradation, especially in mountainous regions (Tamene and Vlek 2008; Ristić et al., 2012; Ashiagbor et al., 2013; Valjarević et al., 2015; Martín-Díaz et al., 2018) such as the area of the municipality of Pale. These regions are highly vulnerable to degradation due to landslides, soil loss on steep slopes, and deforestation. The main consequences of erosion in areas where erosion occurs are bare loss of soil fertility and degradation of soil resource quality, while in areas outside the zone of direct erosion, there is pollution of water bodies and deposition of sediments (Morgan et al., 1984; Blaikie and Brookfield 2015). It has a direct impact on the environment, economy, and agriculture in mountain areas (Vanacker et al., 2003; Thapa, 2020; Chandra Pal et al., 2021).
Numerous popular empirical models have been developed to evaluate erosion intensity: Erosion Potential Method—EPM, the Universal Soil Loss Equation—USLE, Modified Universal Soil Loss Equation—MUSLE, and Revised Universal Soil Loss Equation—RUSLE. The first systematic observations of erosion processes in Bosnia and Herzegovina were carried out by Lazarević R. in the first half of the eighties of the last century (from 1980 to 1984). The intensity of erosion processes was previously estimated using the Erosion Potential Method (Tošić et al., 2012), which is widely used in the countries of the former Yugoslavia (Lazarević, 1985; Gavrilović, 1988; Lazarević, 2010). These research studies have only partially dealt with some of the erosional processes registered in the wider surroundings of the Sarajevo mountains and in the Pale area, among others. Individual works were conducted using the USLE/RUSLE in Bosnia (Tošić et al., 2011; Tošić et al., 2013).
Similar studies have also been conducted in surrounding countries (Diodato, 2006; Mikoš et al., 2010; Simic Belnovic et al., 2013; Valjarević et al., 2014; Blinkov, 2015; Zdruli et al., 2016; Traykova-Pavlova et al., 2017; Bon Gregorič et al., 2018; Micić Ponjiger et al., 2021; Milentijević et al., 2021; Pandey et al., 2021). The RUSLE method in combination with GIS has been used in numerous modern works and research studies (Kouli et al., 2008; Prasannakumar et al., 2012; Tošić et al., 2013; Blackey et al., 2015; Efthimiou, 2016; Nasir and Selvakumar, 2018; Lanorte et al., 2019; Nolė et al., 2020; Polykretis et al., 2020; Milentijević et al., 2021; Sánchez Sánchez et al., 2021), which led to very satisfactory results.
Since soil erosion, in addition to soil degradation, is also responsible for water quality degradation and related environmental damage, it represents an important natural process for this area, which is the main water catchment area for the nearest largest city and the most visited winter tourism center in Bosnia and Herzegovina, Jahorina. Considering that preserved forest cover largely mitigates the negative effects of soil erosion, especially in mountainous areas, this study attempts to show how planned and unplanned deforestation affects the extent of soil erosion. Deforestation occurs primarily due to the expansion of the ski resort and its infrastructure facilities such as vertical transportation and power lines. Soil erosion as a process is not considered during planning, so preventive measures are not taken, and the consequences become visible after the winter—snow seasons or even during construction works under the occurrence of heavy rainfalls.
Soil erosion potential has been estimated using RUSLE in conjunction with GIS as one of the accepted and accurate methods of modern management. RUSLE and GIS provide sufficient information, even in areas where accurate measurements have not been made and based on relatively readily available data, to determine the necessary measures to reduce topsoil degradation. The study outlines how land-use management affects soil erosion, which in turn has direct implications for future economic activities.
2 Materials and methods
2.1 Study area
The study area is located in the central–eastern part of Bosnia and Herzegovina (Figure 1), covers an area of 492 km2 and has about 20,359 inhabitants (Lukić Tanović et al., 2019). The concentration of the population is due to the vertical structure of the relief, and usually the concentration of the population decreases with increasing altitude (Lukić Tanović et al., 2014). This area belongs to the macroregion of the Dinaric morphological system, the Inner Dinarides zone.
The lithological composition consists of rocks of the Paleozoic complex, mainly clasts, and Mesozoic formations, consisting of clasts of the Early Triassic and carbonates of the Middle Triassic, and partly of the volcanic–sedimentary complex. The Cenozoic formations are represented mainly by the accumulation of sediments along river valleys and vertical mountain slopes.
A mountain relief dominates with an average terrain elevation of 1,082 m, and elevations ranging from 600 to 1,910 m above the sea level. The municipality of Pale is bordered by mountains on all sides, except in the east, where the terrain is open along the valley of the river Prača. Between these mountains there are two smaller valleys (Paljanska and Mokranjska). In the highest mountainous areas, the karst process is present, as 30% of the area is covered with limestone and, to a lesser extent, dolomite. However, fluvial processes associated with slope processes dominate, while the periglacial process depends on the climate and hypsometric position and is therefore observed exclusively in the highest parts of the Jahorina Mountain. This process is strongly dependent on climate variations and anthropogenic influences such as the movement of the upper forest boundary (Golijanin, 2021).
A temperate continental and mountain climate prevails, with chilly summers and cold winters. A moderately warm and humid climate prevails in the lower parts of the study area, and a moderately cold and humid climate is pronounced with increasing elevation. The average annual temperature is 6.8°C and the precipitation of 1,080 l/m2 is present throughout the year. Due to its large extension as well as altitude, the Jahorina Mountain Range is a kind of protective shield against the influence of climatic factors from the south on the Paljanska and Mokranjska valleys in the north. Therefore, there are frequent temperature inversions in winter, making the mountainous parts warmer than the valleys. The study area is well drained, and there are many smaller streams and few rivers, such as Prača and Miljacka. Due to the karst hydrography, there are also a few underground rivers. There are no natural lakes, but there are three artificial lakes created for snowmaking on the ski trails.
Thirty-six soil types have been classified in the study area. The predominant soils in the area are cambic classes (67.34%), with various combinations of brown soils dominating with a percentage of 57.59% (Golijanin, 2021). At lower elevations, on gentle slopes and along valleys, more fertile soils (alluvial–deluvial soils) have been developed. These soils are used for agricultural purposes unless degraded or rendered unusable by anthropogenic factors (construction of settlements, roads, etc.). The humidity of the climate and the leaching of the soil increase with altitude, so that very dystric and washout soils can be found in areas with altitudes above 1,000 m. The vegetation that grows on these soils consists almost exclusively of forests and pastures. When afforested, these soils are quite well protected from erosion processes, but due to improper mechanization, construction of wide ski trails, etc., they suffer significant losses due to the occurrence of gully erosion. On the limestones, there are different soil series, which are mostly located on higher terrain. These carbonate soils are thin and mostly under pastures and forest vegetation (Golijanin et al., 2019).
Detailed land-use data based on European Environment Agency (2000), European Environment Agency (2018) are provided in Table 2. From these data, it is observed that the studied area is predominantly covered with forest vegetation (broad-leaved, coniferous, and mixed forest). In total, these zones cover over 344 km2 of the study area, which is about 70% of the territory. This percentage has not changed significantly over the 2000/2018 period, but there are differences in terms of forest type. Recent maps show a decrease in the area covered by broad-leaved forest and coniferous forest and a significant increase in mixed forest (Table 2). Land principally occupied by agriculture, with significant areas of natural vegetation occupying about 11% of the surveyed area and slightly decreased during the observation period. Areas under pastures were also decreased from 37.09 to 32.59 km2, as well as transitional woodland-shrub, while complex cultivation patterns and bare rocks were represented in the same proportion. On the other hand, areas of natural grasslands, discontinuous urban fabric, ski resorts, and power lines increased in the most recent measurements, especially areas designated as ski resorts (more than doubled) and discontinuous urban fabric.
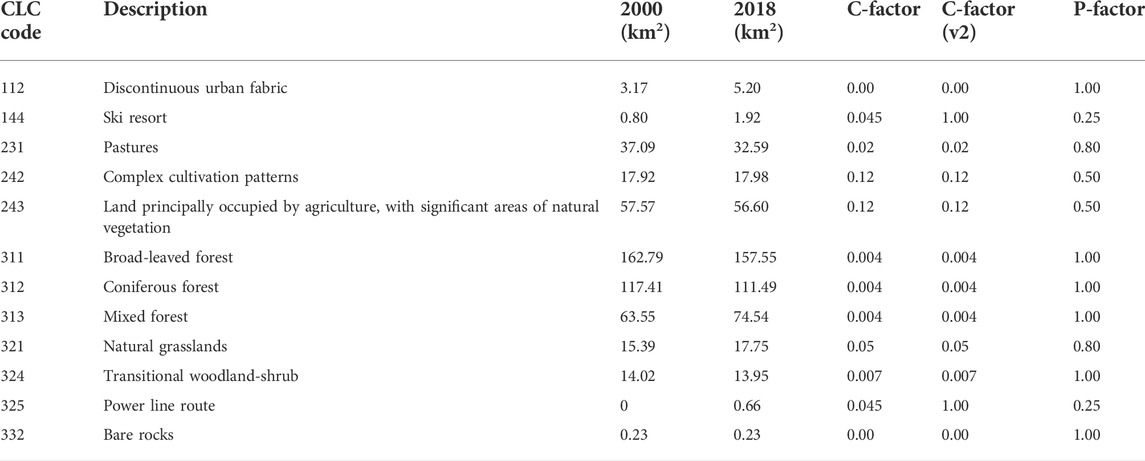
TABLE 2. CORINE land-use code and corresponding land-use factor (C) and conservation practice factor (P).
2.2 Methods
The method used in this study is the Revised Universal Soil Loss Equation (RUSLE). This widely used model for estimating soil erosion intensity, developed by the U.S. Department of Agriculture for land conservation and land-use planning, is the most common modification of the USLE method used to predict the average rate of loss of agricultural land (Renard et al., 1997).
The RUSLE method is based on the same formula as the USLE method with certain modifications to the critical factors. This method was developed primarily for use on agricultural land, that is, to determine the average annual loss for surface and furrowed washes. Numerous studies and publications have proven the usefulness and validity of the results obtained with this method. The method provides a direct link between the slope of the terrain, precipitation, and soil type and is based on relatively readily available data. The use of GIS allows us to obtain very accurate results at low cost and in a very short time. Conventional methods of measuring erosion levels are time-consuming and quite expensive for mass deployment.
The RUSLE method is represented by the following equation:
where A is the average annual soil loss per area unit (t ha−1 year−1), R is the rainfall erosivity factor (MJ mm ha−1 h−1 year−1), K is the soil erodibility factor (t ha h MJ−1 mm−1), L is the slope length factor, S is the slope steepness factor, C is the land-use factor, and P is the support and conservation practices factor.
2.2.1 Rainfall erosivity factor
The R factor is the factor of the erosion effect of rain and represents the energy interaction between precipitation and soil. Based on the available measurements for the study area and the surrounding area, the model of Renard and Freimund was selected to calculate the R factor (Renard and Freimund, 1994), which is calculated based on a modified Fournier index that takes into account the average monthly and annual precipitation.
Since no meteorological measurements are available for the studied area, data from three neighboring meteorological stations were used: Sarajevo, Sokolac and Bjelašnica. The recorded monthly precipitation values from these three stations were used to determine the mean annual value of the R factor. The analyzed data are from continuous series of the 20-year period (2000–2020) (Table 1). It should be noted that in the recent research, the analysis of annual and seasonal precipitation has been presented in a very heterogeneous manner spatially and temporally (seasonally) (Luković et al., 2014; Popov et al., 2017). The modified Fournier index, which takes into account annual monthly precipitation and annual precipitation, is calculated according to the formula (Arnoldus, 1977):
where F is the modified Fournier index, pi is the average monthly precipitation [mm], and P is the average annual precipitation [mm].
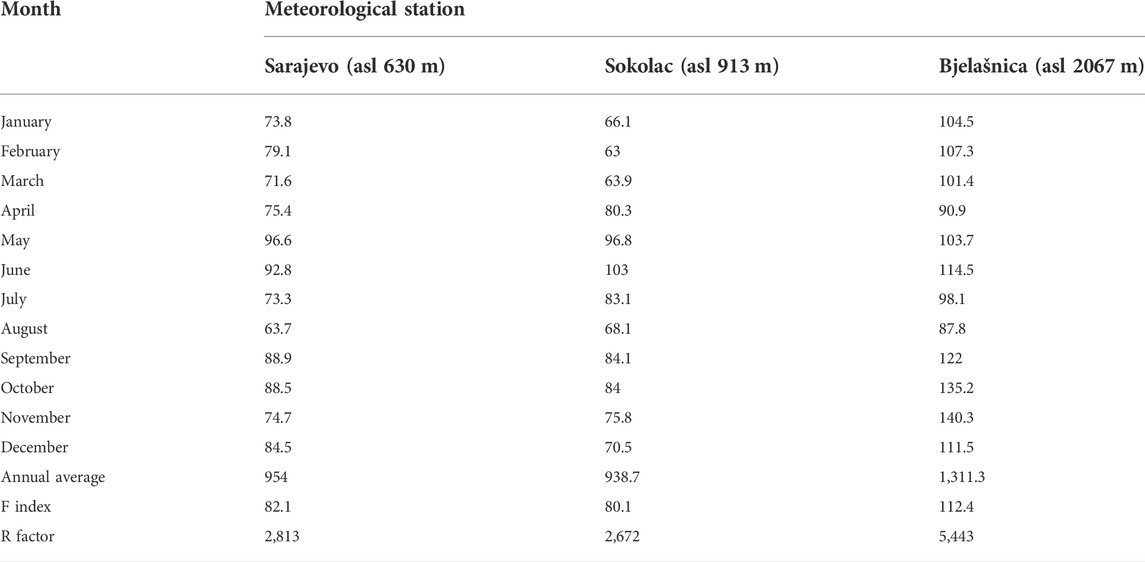
TABLE 1. Average monthly and annual values of precipitation for the period (2000–2020) with the resulting R factor.
According to Renard and Freimund (1994), the relationship between the modified Fournier index and the R factor (MJ mm ha−1 h−1 year−1) for F>55 mm (Table 1) is given by the equation:
R factors were determined for three neighboring meteorological stations for the period from 2000 to 2020. Then, by the deterministic interpolation method inverse distance weighting (IDW) and by spatial analysis in ArcGIS, a map of R factors for the studied area was created.
2.2.2 Soil erodibility factor
The K-factor is a measure of soil tendency to erodibility due to the action of running water and raindrops. According to the model of Wischmeier and Smith (1978) and Renard et al. (1997), five soil characteristics must be determined: coarse sand content, fine sand content, organic matter content, soil structure, and soil water permeability. The K-factor is then calculated using the following formula:
where OM is the percentage of organic matter, s is the structural class of the soil, p is the water permeability class of the soil, and M is the particle size coefficient and is calculated according to
As a database for the calculation of the K-factor map of the studied area, we made vectorization of a 1:50,000 scale pedological map (Zavod za agropedologiju, 1979-1983). Soil properties were determined based on the representative samples of pedological profiles for each pedological unit. Pedological profiles were sampled during the process of creation of a pedological map, for the period from 1979 to 1983. Also, each sample was analyzed in the laboratory. For the purpose of making the pedological map of Bosnia and Herzegovina, a total of 39,473 profiles were opened. There are 61 different pedological units registered in the study area, and the detailed analysis of the representative profiles was carried out in cooperation with experts from the Institute of Agropedology from Sarajevo.
2.2.3 Slope length and slope steepness factor
The LS factor is a topographic factor representing the influence of length (L) and inclination of slope (S). The map of factor LS was calculated from DEM based on a 1:25,000 scale vectorized topographic map (Vojnogeografski institut, 1968-1986), in accordance with the methodology and equation recommended by Moore and Burch (1986) and Moore and Wilson (1992):
where As is the specific catchment area [m], θ is the angle of slope inclination [rad], exponent dependent on slope length m = 0.4 (values 0.4–0.6), exponent dependent on slope inclination n = 1.3 (values 1.22–1.3).
2.2.4 Land-use factor
According to Chandra Pal and Chakrabortty (2019), the C-factor is the most important dimensionless factor indicating the extent of soil erosion, which is directly and indirectly related to vegetation cover. The C-factor defines the influence of vegetation cover and land use on the extent of soil erosion. In the studied area, there are 12 types of land use according to Lastoria et al. (2008) registered and defined. The vector forms of European Environment Agency (2000) and European Environment Agency (2018) were used as the database for the calculation of the C-factor and later the P-factor. CORINE land cover B&H (2000) represents the situation before the expansion of ski resorts and the construction works for the breakthrough of routes for vertical transport, arrangements of ski slopes, the construction of resorts, roads, and other infrastructures in the tourist part of the area which is very mountainous and with large slopes. In 2000, these particular areas were covered with forest. On the other hand, CORINE land cover B&H (2018) was also missing updated land-use details of interest for this study. In addition, the zones of newly deforested areas were updated, high-resolution Google Maps Imagery/CNES/Airbus, Maxar Technologies, Map data 2022 were used as a source for this process. These areas are mainly converted into ski resorts and areas with the function of tracing vertical transportation and support facilities. Here, two possible scenarios for the calculation of the C-factor based on CORINE land cover B&H (2018) are analyzed. The first scenario represents the situation when grassing of deforested zones has been performed, while in the second scenario (v2) the situation with the bare ground after deforestation and construction works is analyzed.
The following values of factor C were determined depending on the vegetation cover on newly formed/repurposed areas (Roose, 1977; Drzewiecki and Mularz, 2005; Bazzoffi, 2013):
For bare soil after construction work C = 1.0.
For sodded ground C = 0.045.
For afforested ground C = 0.001.
Maps for factors C and P were based on CORINE land cover B&H (2000/2018); detailed data are provided in Table 2.
2.2.5 Conservation practice factor
The dimensionless factor P defining the impact of soil erosion prevention measures is defined according to Rosse (1977), Drzewiecki and Mularz (2005), Bazzoffi (2013), Gołąb and Urban (2017):
where D is the direction of cultivation, in this particular case D = 1.0 is assumed, and Md is the cultivation method, considering the fact that the main zones of interest for the analysis are ski slopes, where there are no usual measures to prevent erosion (cross direction of plowing, field bunding, contour bunding etc.) are preferred, we have given the following values of the P-factor:
For bare soil and sodded ground p = 0.25.
For afforested ground p = 0.01.
3 Results and discussion
An individual map was created for each of the factors in the RUSLE equation (Figure 2). For the studied area, the values of the rainfall erosivity factor (R) for the 20-year period ranged from 2,778.82 to 3,656.34, with a mean value of 3,118.36 MJ mm ha−1 h−1 year−1. The R value is the lowest in the northern and eastern parts of the study area, while the mountainous southwestern area has a higher value. High values of the R factor were also found in the southern part of the study area, which had the highest monthly precipitation (area of Jahorina Mountain). In general, it should be noted that the erosion potential of this factor and its spatial distribution vary due to the mostly pluviometric regime.
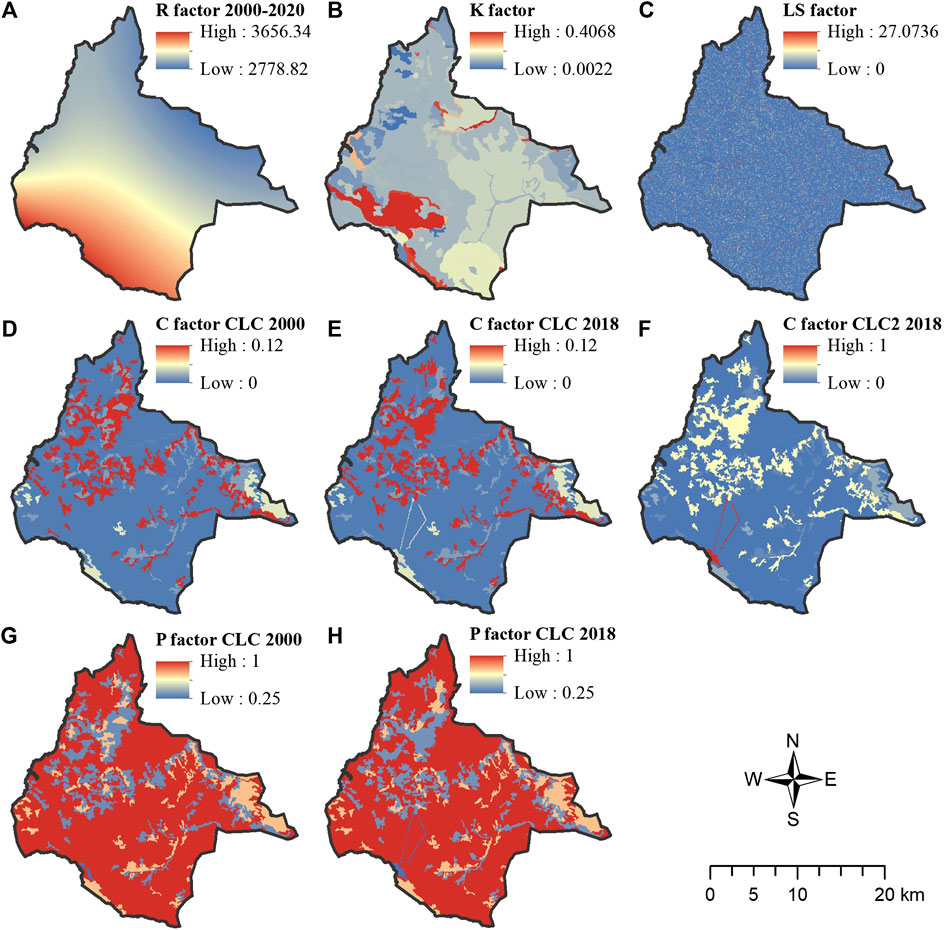
FIGURE 2. Spatial distribution of (A) rainfall erosivity factor, (B) soil erodibility factor, (C) slope length and slope steepness factor, (D) land-use factor CLC 2000, (E) land-use factor CLC 2018, (F) land-use factor CLC 2018 v2, (G) conservation practice factor CLC 2000, and (H) conservation practice factor CLC 2018 in the study area.
The determined values of the soil erodibility factor (K) ranged from 0.0022 to 0.4068, with a mean value of 0.0577 t ha hha−1 MJ−1 mm−1. The map of the K-factor shows that higher values of the K-factor are found in the parts of the Jahorina Mountain, and its flatter part called Ravna Planina (south and southwest), and slightly in the east, at the foot of the Romanija Mountain. The lower values are common in a much larger part of the area, especially in the eastern and northern parts of the study area (Figure 2), which could be due to the soil texture or the sand content of the soils. Soils with a higher sand and silt content formed on siliceous parent material such as chert, and sandstone are more susceptible to erosion than soils with a heavier (clay) texture formed on the rocks with a lower quartz content (clay, limestone, marl, etc.). It should be kept in mind that most of the study area is located in the mountains, where these areas are predominantly covered with limestone, mainly at higher elevations and under forest vegetation. These soils are better structured, have a higher humus content, and a better water-air balance, which could lead to a lower value of the soil erodibility factor.
The slope length and slope inclination factor (LS factor) ranged from 0 to 27.0736 in the study area with an average value of 0.2381. The spatial analysis shows that low values are characteristic of flatter parts of the terrain—valleys and plateaus, while mountainous parts (south, southwest, and northeast) of the area have higher average values for this factor (Figure 2). The greatest variability in terms of elevation, as well as the longest and steepest slopes, is found in the southeastern and northwestern parts of the study area. Prača and Miljacka rivers and their tributaries have formed deep valleys with gorges and canyons in this part of the study area and have highly dissected the terrain. Therefore, there is a high LS factor. In the peripheral eastern and central part of the area, the slopes are lower, as well as on the flat terrain of the karst plateau Gosina (east), Romanija (northeast), and Ravna Planina (south), which contributed to low average values of the LS factor.
During the erodibility analysis, a variation of factor C was made, based on land use and vegetation cover. Three maps were created showing different observation periods as well as changes in vegetation cover. Since the field observations showed the greatest increase in slope erodibility in the tourist area of Jahorina and Ravna Planina (Figure 3), a more detailed analysis of factor C was undertaken, which can shed light on whether there are changes in soil erosion and how they manifest themselves when examined from different perspectives. The spatial distribution of factor C is quite heterogeneous. The study area consists of 12 land use/land cover classes, of which only 10 have significance for soil erosion.
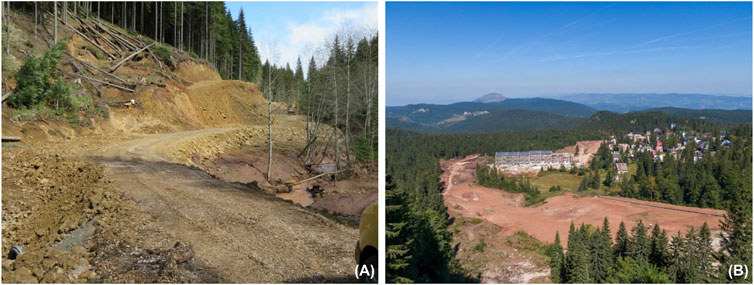
FIGURE 3. Forest devastation caused by construction on Jahorina [sources: (A) Ekapija, 2021; (B) Filter, 2021].
The first map of the C-factor was created based on the situation in 2000, when there were no new trails and routes for ski resorts and routes for vertical transport, as well as many roads and facilities, and these areas were mostly covered with forest. Based on this, an erodibility map was created showing that the factor C varies between 0 and 0.12, with an average value of 0.024.
The second map of factor C, based on the 2018 land-use map (CLC 2018), in which the trails of new paths and routes of vertical transport and infrastructures were subsequently drawn, assuming that grassing of these areas was completed, shows the same values of factor C as the previous map.
The third factor C map was based on the 2018 land-use map (CLC 2018), assuming that only bare land remained after construction works, which was mostly confirmed during field observations. The difference can be seen in the significant increase in the variation of the C-factor, the range of which is the largest and varies from 0 to1, with an average value of 0.029. Ski resort and power line routes, although occupying a smaller portion of the terrain in the study area, and had the largest jump from 0.05 to 1 (Table 2).
It should be noted, however, that most of the terrain is covered with forest vegetation and poorly cultivated. The high values of the C-factor are found in the central and northwestern part of the study area, especially in the tourist zones on karst surfaces and flattened areas with agricultural activities. Low values are measured under forest, natural grasslands, pasture, and transitional woodland-shrub.
Since factor P has no significant effect because no measures were taken to prevent erosion, the previously mentioned values were accepted and included in the maps to complete the RUSLE equation. It should be noted that a value of 1 was given for land-use classes for which this factor has no effect, and therefore, the P-factor map analysis may give a false impression of the influence of this factor. For example, a value of 1 was assigned for all forest land-use types because this factor has no influence on these areas. The two maps obtained show the situation of 2000 and the current situation (of 2018). For the 2000 data, the conservation practice factor (P) varies between 0.25 and 1, with an average value of 0.9004. The same value of the P-factor applies to the 2018 situation, with a slightly lower average value of 0.8994 (Figure 2).
The average annual soil loss (A) in the studied area was calculated by overlaying the five maps using RUSLE with the help of ArcGIS. The erosion categories are defined by the work of Tošić et al. (2013). The value of max A refers to the maximum value of soil erosion at a single point. Comparing the data from CLC 2000, CLC 2018, and CLC 2018 v2, it is noticeable that the maximum value has not changed in the period from 2000 to 2018, considering the planned and preferred land management, but it has increased dramatically (max A), considering the data of the real situation and indicating the actual situation in the field, that is, a significant amount of construction work on the site of Jahorina Mountain.
The average annual soil loss (A) in the municipality of Pale is shown in the three resulting maps (for CLC 2000, CLC 2018, and CLC 2018 v2), Table 3; Figure 4. It can be seen on all three maps that the highest erosivity was registered along the main river valleys (Prača and Miljacka) and their tributaries, as well as on the bare and forested areas of the mountain slopes in the east (Gosina) and south (Jahorina). These areas are covered by various soil types, with degraded shallow soils or some more complex types of brown soils with a higher proportion of sand and silt on siliceous lithological material such as sandstone and chert. Prevailing, which are more sensitive to erosion. On the other hand, the category “very low erosion” is the most widespread in the studied area. This is mainly due to the high percentage of forested areas, which makes the areas covered with forest and pasture vegetation less susceptible to erosion, resulting in lower soil erosion values. In addition, there are very few agricultural lands in this mountainous area, which basically cover the highest percentage of erodibility.
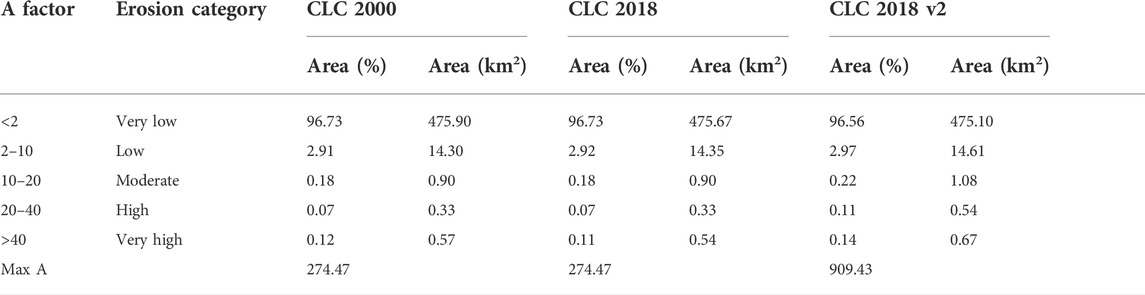
TABLE 3. Categories of soil erosion rate for different categories and different CLC databases for Pale.
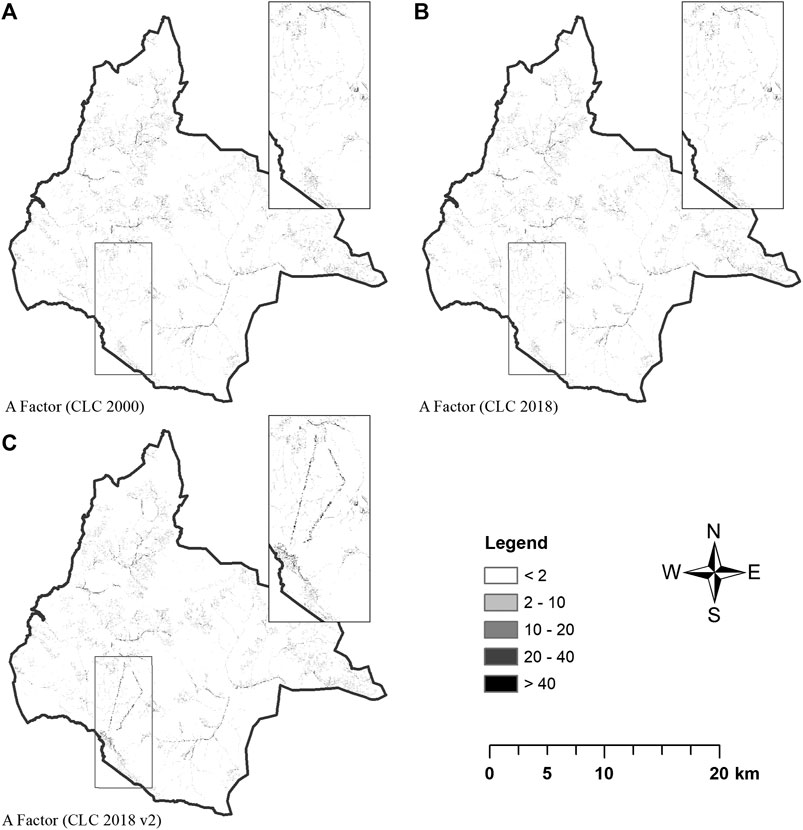
FIGURE 4. Distribution maps of average annual soil loss rate in Pale Municipality (A) according to CLC 2000; (B) according to CLC 2018; (C) according to CLC 2018 v2.
The first and second maps show the average annual soil loss rates according to the situation between 2000 and 2018, and in terms of percentage values, they are very similar. Thus, the range of average annual soil loss for both maps is from 0 to 274.47 t ha−1 year−1, with an average value of 0.37 t ha−1 year−1. The results show that more than 96.73% of the study area suffers from very low erosion and 2.91% (2.92% for CLC 2018) of the area suffers from low erosion. Moderate erosion, from 10 to 20 t ha−1 year−1, occurs on 0.18% of the area, and high and very high erosion was estimated at almost 0.19% (0.18% for CLC 2018) of the study area.
The analysis of the map made on the basis of the modified data CLC 2018 v2 (Figure 4C) shows some differences. First, the difference is large in terms of the range of average annual soil loss, which ranges from 0 to 909.43 t ha−1 year−1, while the average value of 0.42 t ha−1 year−1 is similar. As for the percentage ratios, the difference is most evident in the increase of areas with moderate (0.22%), high (0.11%), and very high (0.14%) erosion. The percentage of the category “low erosion” has also increased (2.97%), so that the area with very low erosion has partially decreased, amounting to (96.56%). Looking at the study area, it is noticeable that soil erosion is most pronounced in the mountainous areas where intensive deforestation has taken place without taking measures to prevent soil erosion, and in the areas with stripping surfaces areas caused by intensive construction of infrastructure and facilities in the tourist part of Jahorina. These areas are shown separately and analyzed cartographically in Figure 4.
Through the analysis of satellite imagery and the addition of spatial data, the results of this study provided accurate data on soil loss in the study area. Studies of this type have not been conducted for the B&H area, so this is evident that the addition of data to calculate the C-factor significantly influenced the research results. We attempted to obtain more accurate results based on the modest database we had available and to determine the impact of deforestation.
There are some similar studies that address the problem of soil loss using the RUSLE method and GIS, but not in the same way. The results obtained in this study are partially compared with those studies related to terrain characteristics and their purpose (mountainous terrains suitable for ski resorts) and climate, where it was confirmed that unplanned and irrational deforestation significantly increases the level of soil loss (Gołąb and Urban, 2017). Moreover, the values of soil erosion in the study area are consistent with the erosion map of the Republic of Srpska (B&H) (Tošić and Lazarević, 2012) for the case of higher mountain zones, where the degree of erosion is lower, as the highest percentage of soil loss occurred in the zone between 200 and 1,000 m, which depends on topographic features and land use.
The main limitations and shortcomings of the study are due to the lack of more accurate measurements and sources of information. First of all, there are no direct meteorological measurements in the studied area. The network of meteorological stations in Bosnia and Herzegovina is generally poor. Since the area of Pale Municipality is mainly characterized by mountainous terrain, accurate data on the amount of precipitation in the study area would be an important input parameter and would certainly provide more accurate values for soil erosion loss. On the other hand, there is a lack of soil maps with better resolution. We did not have access to maps with a higher resolution than the 1:50,000 scale. Regarding the accuracy of the data, the lack of CORINE maps on land cover is striking, since the smallest mapped unit is 0.25 km2 and the minimum width of 100 m for linear phenomena. In most developing countries, including Bosnia and Herzegovina, the availability of high-quality mapped data is low, limiting the application of this model. For this reason, we performed a correction of the maps with the parts of the studied area most at risk of soil erosion (tourist parts of the municipality), as changes detected in the field were not registered in the CORINE land cover B&H for 2018.
For further studies, it is recommended to narrow down the studied area to the zone where soil reuse works are planned. The results show that RUSLE is very useful not only for agricultural purposes, but especially for tourism development. The method proved to be sufficiently accurate given the size of the area where the changes were recorded. In a relatively short time and with easily accessible data, the problem can be detected and the right method for managing endangered areas can be identified and tested, and guidelines for further action can be established.
4 Conclusion
Previous studies on the territory of Bosnia and Herzegovina and the application of the RUSLE method in terms of soil erosion analysis by different land cover management models are very rare. Moreover, deforestation is a frequent and pronounced problem in Bosnia and Herzegovina, which brings various consequences. One of these consequences is frequent erosion of land in areas that become vulnerable to soil loss due to irrational human activities. Therefore, in this research, we tried to highlight the problems that are most pronounced in the study area in order to estimate the potential reduction of soil erosion under different land cover management models.
Future research on soil erosion is needed not only to provide new scientific and technical knowledge on this topic, but also because of the broad potential applications of this knowledge in practice. Special attention should be paid to the use of modern technologies and methods in the research process that allows easier and better data collection and processing, as well as modeling and monitoring of erosive processes. In addition to clearly defining areas that are already endangered by erosion, suitability is also demonstrated by the ability to define areas where erosion can potentially be a major problem due to irrational land use. This knowledge is of great importance to B&H because it provides the opportunity to manage space and natural resources more appropriately and to take preventive measures that are much cheaper and more effective than corrective ones.
Data availability statement
The raw data supporting the conclusion of this article will be made available by the authors, without undue reservation.
Author contributions
JG, GN, and AV contributed to the concept and design of the study. RI, MG, and MT contributed to the design and production of the manuscript. VT and SB have organized a database, especially on land types, and helped to analyze K factors. JG and EH gave general characteristics of the study area, and MP and SS analyzed similar research in Southeast Europe. JG made a rough draft of the manuscript and cartographic drawings. Parts of the manuscript were written by AV, MP, EH, and GN. JG systematized and interpreted the results of GIS analysis with the aid of AV and GN. JG, MT, RI, and MG collected information from the field on the problem of deforestation at the Pale ski resorts. All authors participated in the editing of the manuscript and reviewed the final version.
Conflict of interest
The authors declare that the research was conducted in the absence of any commercial or financial relationships that could be construed as a potential conflict of interest.
Publisher’s note
All claims expressed in this article are solely those of the authors and do not necessarily represent those of their affiliated organizations, or those of the publisher, the editors, and the reviewers. Any product that may be evaluated in this article, or claim that may be made by its manufacturer, is not guaranteed or endorsed by the publisher.
References
Arnoldus, H. M. J. (1977). Methodology used to determine the maximum potential average annual soil loss due to sheet and rill erosion in Morocco. FAO Soils Bull. 34, 39–51.
Ashiagbor, G., Forkuo, E. K., Laari, P., and Aabeyir, R. (2013). Modeling soil erosion using RUSLE and GIS tools. Int. J. Remote Sens. Geoscience (IJRSG) 2 (4), 1–17.
Bakker, M. M., Govers, G., Kosmas, C., Vanacker, V., Van Oost, K., Rounsevell, M., et al. (2005). Soil erosion as a driver of land-use change. Agric. Ecosyst. Environ. 105 (3), 467–481. doi:10.1016/j.agee.2004.07.009
Blackley, R., Sbrocchi, C., Steinfeld, C., Grundy, M., Biggs, A., and Silbum, M. (2015). Geographical systems approach to the assessment of soil erosion using RUSLE model. Aust. Reg. Environ. Accounts Work. Pap. Ser. 5, 63–74.
Blaikie, P., and Brookfield, H. (2015). Land degradation and society. London: Routledge, 222. doi:10.4324/9781315685366
Blinkov, I. (2015). The Balkans ˗ the most erosive part of Europe? Glas. Suma. Fakul. 111, 9–20. doi:10.2298/GSF1511009B
Bon Gregorič, N., Josipovič, D., and Kanjr, U. (2018). Linking geomorphological and demographic movements: The case of Southern Albania. Appl. Geogr. 100, 55–67. doi:10.1016/j.apgeog.2018.09.002
Chandra Pal, S., and Chakrabortty, R. (2018). Modeling of water induced surface soil erosion and the potential risk zone prediction in a sub-tropical watershed of Eastern India. Model. Earth Syst. Environ. 5, 369–393. doi:10.1007/s40808-018-0540-z
Chandra Pal, S., Chakrabortty, R., Roya, P., Chowdhuri, I., Dasa, B., Saha, A., et al. (2021). Changing climate and land use of 21st century influences soil erosion in India. Gondwana Res. 94, 164–185. doi:10.1016/j.gr.2021.02.021
Chandra Pal, S., and Chakrabortty, R. (2019). Simulating the impact of climate change on soil erosion in sub-tropical monsoon dominated watershed based on RUSLE, SCS runoff and MIROC5 climatic model. Adv. Space Res. 64, 352–377. doi:10.1016/j.asr.2019.04.033
Diodato, N. (2006). Predicting RUSLE (revised universal soil loss equation) monthly erosivity index from readily available rainfall data in mediterranean area. Environmentalist 26, 63–70. doi:10.1007/s10669-006-5359-x
Drzewiecki, W., and Mularz, S. (2005). The USPED model is a tool for predicting the effects of erosion and deposition of soil material. Rocz. Geomatyki III (2), 45–55.
Efthimiou, N. (2016). Performance of the RUSLE in mediterranean mountainous catchments. Environ. Process. 3, 1001–1019. doi:10.1007/s40710-016-0174-y
Ekapija (2021). Construction of the apartment complex “Snježna dolina” on Jahorina. Available at: https://ba.ekapija.com/real-estate/3412402/KZIN-PR/krajem-2021-predvidjen-zavrsetak-apartmanskog-dijela-kompleksa-snjezna-dolina-na-jahorini-otvaranje (Accessed March 15, 2022).
European Commision (2004). Proposal for directive of the European parliament and of the council establishing a framework for the protection of soil and amending directive 2004/35/EC. COM(2006) 232 final. Brussels: EUR-Lex.
European Environment Agency (EEA) (2000). Copernicus land service, pan-European component, corine land cover factsheet.
European Environment Agency (EEA) (2018). Copernicus land service, pan-European component, corine land cover factsheet.
Filter (2021). On Jahorina construction of a new road and walkway. Available at: https://www.citajfilter.com/2021/05на-јахорини-изградња-новог-пута-и-паса/?script=lat (Accessed March 15, 2022).
Gavrilović, Z. (1988). “The use of an empirical method (Erosion Potential Method) for calculating sediment production and transportation in unstudied or torrential streams,” in Proceedings of International Conference of River Regime, Wallingford, England, May 18–20, 1988 (Wallingford: Hydraulics Research Limited), 411–422.
Gołąb, J., and Urban, K. (2017). Potential erosion of the areas deforested for ski slopes – an example of Mount Jaworzyna Krynicka. Infrastructure and ecology of rural areas. Polish Academy of Sciences. Crac. Branch 4 (1), 1415–1426. doi:10.14597/infraeco.2017.4.1.108
Golijanin, J. (2021). Geomorphological characteristics of the Pale municipality. Pale: Dis Company, 214.
Golijanin, J., Tunguz, V., Valjarević, A., and Kovačević, D. (2019). “Geoecological evaluation of soils and the other natural potentials for forestry usage – case study Ravna Mountain and Pale Valley (B&H),” in Proceedings at the International Scientific Conference „Eastern European Chernozems – 140 Years after Dokuchaev, Chisinau, Republic of Moldova, October 2–3, 2019, 92–99. https://agris.fao.org/agris-search/search.do?recordID=MD2021100021.
Kouli, M., Soupios, P., and Vallianatos, F. (2008). Soil erosion prediction using the revised universal soil loss equation (RUSLE) in a GIS framework, Chania, northwestern Crete, Greece. Environ. Geol. 57, 483–497. doi:10.1007/s00254-008-1318-9
Lanorte, A., Cillis, G., Calamita, G., Pilogallo, A., Tucci, B., Nole, G., et al. (2019). Integrated approach of RUSLE, GIS and ESA Sentinel-2 satellite data for post-fire soil erosion assessment in Basilicata region (Southern Italy). Geomat. Nat. Hazards Risk 10 (1), 1563–1595. doi:10.1080/19475705.2019.1578271
Lastoria, B., Miserocchi, F., Lanciani, A., and Monacelli, G. (2008). An estimated erosion map for the aterno-pescara river basin. Eur. Water 21/22, 29–39.
Lazarević, R. (1985). The erosion map of Bosnia and Herzegovina in scale 1:25,000. Belgrade: Institute of Forestry and Wood Industry.
Lukić Tanović, M., Golijanin, J., and Grmuša, M. (2014). The impact of relief on the distribution of the population in the area of East Sarajevo. Mediterr. J. Soc. Sci. 5 (22), 176–183. doi:10.5901/mjss.2014.v5n22p176
Lukić Tanović, M., Golijanin, J., and Šušnjar, S. (2019). Impact of population on the karst of east Sarajevo (Bosnia and Herzegovina). J. Geogr. Inst. “Jovan Cvijić” SASA 69 (2), 95–107. doi:10.2298/IJGI1902095L
Luković, J., Bajat, B., Blagojević, D., and Kilibarda, M. (2014). Spatial pattern of recent rainfall trends in Serbia (1961–2009). Reg. Environ. Change 14 (5), 1789–1799. doi:10.1007/s10113-013-0459-x
Martín-Díaz, J., Palma, P., Golijanin, J., Nofre, J., Oliva, M., and Čengić, N. (2018). The urbanisation on the slopes of SARAJEVO and the rise of geomorphological hazards during the post-war period. Cities 72, 60–69. doi:10.1016/j.cities.2017.07.004
Micić Ponjiger, T., Lukić, T., Basarin, B., Jokić, M., Wilby, R. L., Pavić, D., et al. (2021). Detailed analysis of spatial–temporal variability of rainfall erosivity and erosivity density in the central and southern pannonian basin. Sustainability 13, 13355. doi:10.3390/su132313355
Mikoš, M., Jošt, D., and Petkovšek, G. (2010). Rainfall and runoff erosivity in the alpine climate of north Slovenia: A comparison of different estimation methods. Hydrological Sci. J. 51 (1), 115–126. doi:10.1623/hysj.51.1.115
Milentijević, N., Ostojić, M., Fekete, R., Kalkan, K., Ristić, D., Bačević, R. N., et al. (2021). Assessment of soil erosion rates using RevisedUniversal soil loss equation (RUSLE)and GIS in Bačka (Serbia). Pol. J. Environ. Stud. 30 (6), 5175–5184. doi:10.15244/pjoes/135617
Moore, I. D., and Burch, G. J. (1986). Physical basis of the length-slope factor in the universal soil loss equation. Soil Sci. Soc. Am. J. 50, 1294–1298. doi:10.2136/sssaj1986.03615995005000050042x
Moore, I. D., and Wilson, J. P. (1992). Length-slope factors for the revised universal soil loss equation: Simplified method of estimation. J. Soil Water Conservation 47, 423–428.
Morgan, R. P. C., Morgan, D. D. V., and Finney, H. J. (1984). A predictive model for the assessment of soil erosion risk. J. Agric. Eng. Res. 30, 245–253. doi:10.1016/S0021-8634(84)80025-6
Morgan, R. P. C. (2005). Soil erosion & conservation. Oxford: Blackwell Publishing, 320. doi:10.1111/j.1365-2389.2005.0756f.x
Nasir, N., and Selvakumar, R. (2018). Influence of land use changes on spatial erosion pattern, a time series analysis using RUSLE and GIS: The cases of ambuliyar sub-basin, India. Acta Geophys. 66, 11211121–11211130. doi:10.1007/s11600-018-0186-2
Nolė, G., Santarsiero, V., Lanorte, A., Tucci, B., Carpuso, A. V., Ronco, V. F., et al. (2020). Model of post fire erosion assessment using RUSLE method, GIS tools and ESA sentinel DATA. International Conference on Computational Science and its Applications ICCSA 2020: Computational Science and its Applications ˗ ICCSA 2020, July 1–4, 2020, 505–516.
Pandey, S., Kumar, P., Zlatic, M., Nautiyal, R., and Vijender, Panwar P. (2021). Recent advances in assessment of soil erosion vulnerability in a watershed. Int. Soil Water Conservation Res. 9 (3), 305–318. doi:10.1016/j.iswcr.2021.03.001
Pimentel, D., Harvey, C., Resosudarmo, P., Sinclair, K., Kurz, D., McNair, M., et al. (1995). Environmental and economic costs of soil erosion and conservation benefits. Science 267 (5201), 1117–1123. doi:10.1126/science.267.5201.1117
Polykretis, C., Alexakis, D. D., Grillakis, M., and Manoudakis, S. (2020). Assessment of intra-annual and inter-annual variabilities of soil erosion in Crete island (Greece) by incorporating the dynamic “nature” of R and C-factors in RUSLE modeling. Remote Sens. 12 (15), 2439. doi:10.3390/rs12152439
Popov, T., Gnjato, S., Trbić, G., and Ivanišević, M. (2017). Trends in extreme daily precipitation indices in Bosnia and Herzegovina. Collect. Pap. - Fac. Geogr. at Univ. Belgrade 65, 5–24. doi:10.5937/zrgfub1765005P
Prasannakumar, V., Vijith, H., Abinod, S., and Geetha, N. (2012). Estimation of soil erosion risk within a small mountainous sub-watershed in Kerala, India, using Revised Universal Soil Loss Equation (RUSLE) and geo-information technology. Geosci. Front. 3 (2), 209–215. doi:10.1016/j.gsf.2011.11.003
Renard, K. G., Foster, G. R., Weessies, G. A., McCool, D. K., and Yoder, D. C. (1997). Predicting soil erosion by water: A guide to conservation planning with the revised universal soil loss equation (RUSLE). Washington, DC: U.S. Department of Agriculture.
Renard, R., and Freimund, G. (1994). Using monthly precipitation data to estimate the R factor in the Revised USLE. J. Hydrology 157, 287–306. doi:10.1016/0022-1694(94)90110-4
Ristić, R., Kostadinov, S., Radić, B., Trivan, G., and Nikić, Z. (2012). “Torrential floods in Serbia-man made and natural hazards,” in 12th Congress INTERPRAEVENT 2012 – Grenoble/France. Conference Proceedings, Grenoble, France, April 23–26, 2012, 771–779.
Roose, E. J. (1977). “Application of the universal soil loss equation of wischmeier and Smith in West Africa,” in Conservation and soil management in the humid tropics. Editors D. J. Greenland, and R. Lal (Chichester, England: Wiley), 177–187.
Sánchez Sánchez, Y., Graña Martinez, A., and Francės˗Santos, F. (2021). Remote sensing calculation of the influence of wildfire on erosion in high mountain areas. Agronomy 11 (8), 1459. doi:10.3390/agronomy11081459
Simic Belnovic, S., Petrović, V., Kostadinov, S., Kadović, R., Vidojević, D., Knezevic, M., et al. (2013). Assessment of soil erosion intensity in Kolubara District, Serbia. Fresenius Environ. Bull. 22 (5), 1556–1563.
Tamene, L., and Vlek, P. L. (2008). “Soil erosion studies in northern Ethiopia,” in Land use and soil resources (Dordrecht, Netherlands: Springer), 73–100.
Thapa, P. (2020). Spatial estimation of soil erosion using RUSLE modeling: A case study of Dolakha district, Nepal. Environ. Syst. Res. (Heidelb). 9, 15. doi:10.1186/s40068-020-00177-2
Tošić, R., Dragićević, S., Kostadinov, S., and Dragović, N. (2011). Assessment of soil erosion potential by the USLE method: Case study: Republic of Srpska-BiH. Fresenius Environ. Bull. 20 (8), 1910–1917.
Tošić, R., Dragićević, S., and Lovrić, N. (2012). Assessment of soil erosion and sediment yield changes using erosion potential method - case study: Republic of Srpska-BiH. Carpathian J. Earth Environ. Sci. 7 (4), 147–154.
Tošić, R., Kapović, M., Lovrić, N., and Dragićević, S. (2013). Assessment of soil erosion potential using RUSLE and GIS: A case study of Bosnia and Herzegovina. Fresenius Environ. Bull. 22 (11), 3415–3423.
Tošić, R., and Lazarević, R. (2012). The erosion map of republic of Srpska in scale 1:25, 000. Bijeljina: Institute for water management Bijeljina.
Traykova˗Pavlova, E., Martinov, T. I., and Dimov, P. (2017). Evaluation of water erosion risk of Badinska river catchment, Southwest Bulgaria. Glas. Suma. Fakul. 115, 89–98. doi:10.2298/GSF1715089P
Valjarević, A., Srećković-Batoćanin, D., Živković, D., and Perić, M. (2015). GIS analysis of dissipation time of landscape in the Devil's city (Serbia). Acta Montan. Slovaca 20 (2), 148–155. doi:10.3390/ams20020148
Valjarević, A., Živković, D., Valjarević, D., Stevanović, V., and Golijanin, J. (2014). GIS analysis of land cover changes on the territory of the prokuplje municipality. Sci. World J. 2014, 805072. doi:10.1155/2014/805072
Vanacker, V., Govers, G., Barros, S., Poesen, J., and Deckers, J. (2003). The effect of short-term socio-economic and demographic change on landuse dynamics and its corresponding geomorphic response with relation to water erosion in a tropical mountainous catchment, Ecuador. Landsc. Ecol. 18 (1), 1–15. doi:10.1023/a:1022902914221
Vojnogeografski institut (Military Geographic Institute) (1968-1986). Topographic maps 1:25 000, sheets: Sarajevo, Ilovice, Pustopolje, Ravna Romanija, Pale, Podgrab, Prača, Kovanj, Jahorina, Gornja Prača, Ilovača. Belgrade: Military Geographic Institute.
Wischmeier, W. H., and Smith, D. D. (1978). Predicting rainfall erosion losses: A guide to conservation planning. Washington, DC: U.S. Department of Agriculture Science and Education Administration. Agriculture Handbook No. 537.
Zavod za agropedologiju (Institute of Agropedology) (1979-1983). Pedological maps of former Yugoslavia 1:50 000, sheets: Sarajevo 1, 2, 3 and 4. Sarajevo: Faculty of Forestry.
Keywords: erosion potential, Revised Universal Soil Loss Equation (RUSLE), C-factor, GIS, Pale Municipality
Citation: Golijanin J, Nikolić G, Valjarević A, Ivanović R, Tunguz V, Bojić S, Grmuša M, Lukić Tanović M, Perić M, Hrelja E and Stankov S (2022) Estimation of potential soil erosion reduction using GIS-based RUSLE under different land cover management models: A case study of Pale Municipality, B&H. Front. Environ. Sci. 10:945789. doi: 10.3389/fenvs.2022.945789
Received: 16 May 2022; Accepted: 11 July 2022;
Published: 08 August 2022.
Edited by:
Yiping Wu, Xi’an Jiaotong University, ChinaReviewed by:
Pengcheng Sun, Yellow River Institute of Hydraulic Research, ChinaSubodh Chandra Pal, University of Burdwan, India
Copyright © 2022 Golijanin, Nikolić, Valjarević, Ivanović, Tunguz, Bojić, Grmuša, Lukić Tanović, Perić, Hrelja and Stankov. This is an open-access article distributed under the terms of the Creative Commons Attribution License (CC BY). The use, distribution or reproduction in other forums is permitted, provided the original author(s) and the copyright owner(s) are credited and that the original publication in this journal is cited, in accordance with accepted academic practice. No use, distribution or reproduction is permitted which does not comply with these terms.
*Correspondence: Jelena Golijanin, amVsZW5hZ29saWphbmluQGdtYWlsLmNvbQ==