- 1National School of Arts and Humanities, Australian Catholic University, Brisbane, QLD, Australia
- 2Department of Geography and the Environment, University of Texas at Austin, Austin, TX, United States
- 3Department of Geography and GIS, University of Cincinnati, Cincinnati, OH, United States
- 4Department of Geography, University College London, London, United Kingdom
The Mexico and Central American region has a history of mercury use that began at least two millennia before European colonisation in the 16th century. Archaeologists have reported extensive deposits of cinnabar (HgS) and other mercury materials in ancient human settlements across the region. However, there has been no consideration to date of the environmental legacy of this long history of anthropogenic mercury use. This review begins by synthesising our knowledge of the history and nature of anthropogenic mercury in ancient Mesoamerica based on archaeological data, with a particular focus on the Maya culture of lowland Guatemala, Belize, the Yucatan of Mexico, El Salvador, and Honduras. The Classic Period Maya used mercury for decorative and ceremonial (including funerary) purposes: Cinnabar (HgS) predominantly, but the archaeological record also shows rare finds of elemental mercury (Hg0) in important burial and religious contexts. In this review, we have located and summarised all published data sets collected from (or near) ancient Maya settlements that include environmental mercury measurements. Comparing mercury determinations from pre-Columbian Maya settlements located across the region confirms that seven sites from ten have reported at least one location with mercury concentrations that equal or exceed modern benchmarks for environmental toxicity. The locations with elevated mercury are typically former Maya occupation areas used in the Late Classic Period, situated within large urban settlements abandoned by c. 10th century CE. It is most likely that the mercury detected in buried contexts at Maya archaeological sites is associated with pre-Columbian mercury use, especially of cinnabar. In more complex contexts, where modern biological or specifically anthropogenic inputs are more probable, legacy mercury in the environment will have a more complex, and time transgressive input history. This review identifies current research gaps in our understanding of the long history of Maya mercury use and in the collection of robust total mercury datasets from the Maya world. We identify important areas for future research on the environmental persistence and legacy of mercury, including the need to interpret environment mercury data in the context of mercury exposure and human health at Maya archaeological sites.
Introduction
Mercury1 (Hg) is a toxic pollutant that affects human and ecosystem health. Enhanced mercury concentrations in the surface systems of our planet are primarily connected with increasing industrialisation and urbanisation. Mining and fossil-fuel power generation activities are responsible for at least half of known global mercury emissions today (Outridge et al., 2018). The cycling of mercury through the environment is driven by modern emissions such as these, but also includes re-mobilised legacy mercury from past anthropogenic activities (Nriagu, 1993).
The history of global societies modifying the planetary mercury cycle begins with the actions of past cultures in disparate locations of the world. In some settings, the anthropogenic mercury in environmental systems cannot be directly mapped to modern industrial activities, but instead, part of the anthropogenic mercury budget is from human actions and “industries” undertaken millennia before the Industrial Revolution (Brooks, 2012). Like other toxic heavy metals such as lead, the history of anthropogenic mercury is intertwined with long and complex human histories of mining and mineral and ore use that began in the Holocene alongside other early environmental transformations (Stephens et al., 2019). Humans were extracting and using mercury ores by the ninth and eighth millenniums BCE in the eastern Mediterranean region of Western Asia (Mellaart, 1967; Molleson et al., 1992; Schotsmans et al., 2022). Early and ongoing mercury use in ancient societies led to mercury exposure in humans and, presumably, detrimental health impacts, as studies on ancient human remains indicate (Gliozzo, 2021 and references therein). Much of our understanding of the long history of mercury use and its impacts has come from Europe and Asia, while in other regions, the long-term accounts of humans modifying mercury processes and inputs to the environment are not well documented.
An important example of a multi-millennial record of mercury use is from present-day Mexico and Central America, where the Maya used mercury (mainly cinnabar) for many centuries before European Contact in the 16th century. The possible environmental consequence of this long, region-wide preindustrial mercury use is yet to be investigated. Mercury is scarce in the geology of the Maya world and the natural “background” level of mercury across the region is thought to be negligible. Mercury is a rare element in the natural environment, with average concentrations of around 0.05 μg g−1 in the Earth’s upper crust (Rudnick and Gao, 2003). The mercury content in limestone bedrock, such as that underlying much of the Maya region, is even lower again, contributing only negligible amounts of mercury and other trace elements into soils (Reimann and Caritat, 1998; Cook et al., 2006). Against this backdrop of negligible mercury, the onset of anthropogenic mercury in the region by pre-Columbian peoples may have been of sufficient magnitude as to disrupt the mercury cycle.
Some of the strongest evidence for anthropogenic alterations to the mercury cycle comes from records of mercury deposition preserved in environmental archives (Cooke et al., 2009; Cooke et al., 2013; Cooke et al., 2022). Environmental geochemical studies have the potential to provide the datasets now required by archaeologists to reconstruct the historic use of mercury by the Maya, and help answer questions regarding the source, trade, use and legacy of mercury use. Additionally, producing a detailed account of the long history of human mercury use and its environmental consequences across Central America is required to ensure we have accurate and complete accounts of anthropogenic mercury change in our regional and global models of the mercury cycle.
Geochemical studies of soils and sediments associated with ancient cultures and settlements in Mesoamerica have been site-specific, and often sharply focused on archaeological (rather than environmental) research objectives. In this review, the literature now available on anthropogenic mercury accumulation across the Maya region is evaluated and summarised with the aim of understanding past mercury use as part of constructing a multi-millennial environmental history of mercury in the Maya world. We aim to draw linkages, where possible, between archaeological and environmental datasets on mercury and in doing so, develop a region-wide model of preindustrial mercury transport and use, mercury accumulation in the environment, and the environmental legacy of up to two millennia of mercury use. A related aim is to consider the implications of antecedent environmental mercury from the pre-Columbian Maya era for ongoing research activities and human health by evaluating legacy mercury data against modern guidelines for toxicity. Finally, we identify current research gaps and opportunities, and propose research agendas that can advance our understanding of the history of anthropogenic mercury in the Maya world.
Mercury, Mesoamerica, and the pre-Columbian Maya
Cinnabar (HgS) is the most abundant form of Hg in the lithosphere. HgS is dimorphic in nature with two crystal forms; red-coloured cinnabar (hexagonal α -HgS) and black-coloured metacinnabar (cubic β -HgS). Ancient cinnabar mining marks the beginning of the global history of anthropogenic mercury cycling (Goldwater, 1972; Nriagu, 1979; Smith et al., 2015). Our current understanding, based on archaeological studies, is that cinnabar was first mined and used in Central America by early Mesoamerican societies (particularly the Olmec) in the lowland regions of southern Mexico from the second to first millennia BCE (Langenscheidt, 1986). Anthropogenic mercury inputs to the environment may, therefore, have commenced by this time, at least in some parts of Mexico. Intricate statues, figurines and jade carvings decorated with cinnabar were created by the Olmec starting in the Early Formative period (c. 1200 BCE) (Figure 1) while powdered cinnabar was used in Olmec-period burials and other special contexts (Drucker et al., 1959; Tate 2012).
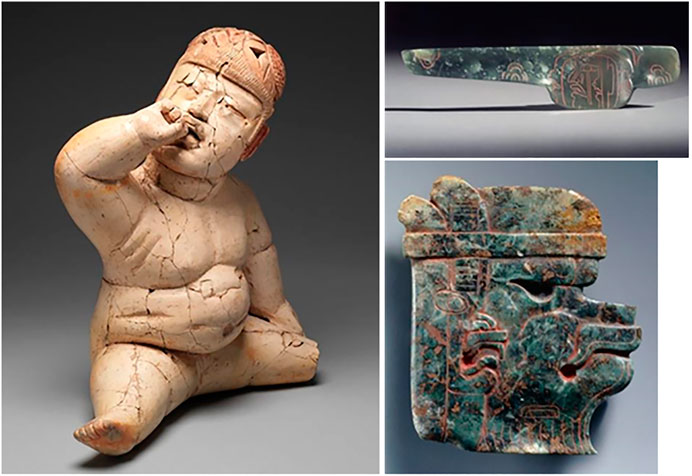
FIGURE 1. Archaeological evidence of early (before c. 500 BCE) cinnabar (HgS) use in Mesoamerica. Left: “Hollow-baby” an Early Formative (?) period Olmec seated figure decorated with cinnabar powder (Courtesy Metropolitan Museum of Art, Michael C. Rockefeller Memorial Collection, Bequest of Nelson A. Rockefeller, 1979, no. 1979.206.1134). Top Right: Olmec “spoon” in jade with cinnabar in incised lines (Courtesy of the Brooklyn Museum). Bottom Right: Middle Formative (c. 1,000–500 BCE) Olmec jade pendant with cinnabar with cinnabar in incised lines. Source: “Plaque, 1996-11,” Princeton University Art Museums collections online, 10 April 2022, https://artmuseum.princeton.edu/collections/objects/44888.
The Maya people have inhabited the lowland areas of Mexico and Central America for millennia, with major cultural, societal, and technological developments beginning around 2000 BCE (Table 1). The archaeological record of mercury use by the Maya consists of three broad types of artefacts: Objects painted with mercury-based paints; cinnabar powder, cinnabar ore fragments and cinnabar “pigment” materials (cinnabar powder mixed with clays and carbonate materials to create paints) used for decorative or ceremonial purposes; and deposits of elemental mercury (Hg0) (that is, liquid mercury) in Maya contexts. There are no known references to cinnabar or elemental mercury in Maya pictorial art or epigraphy: the archaeological record is the only source of information on how the Maya interacted with mercury.
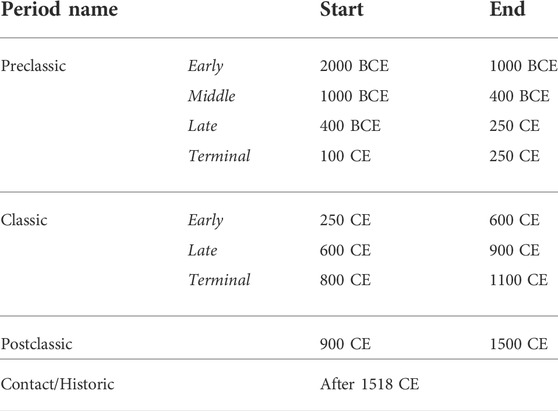
TABLE 1. Prehistoric and historic cultural periods of the lowland Maya region of Mesoamerica (cultural period information adapted from Demarest (2004) and Sharer and Traxler (2006).
Our modern knowledge of mercury use at ancient Maya settlements dates to the end of the 19th century when Maudslay (1889–1902), and later Gann (1925), described elemental mercury and cinnabar in early Classic Maya burials at Copan, Honduras. Archaeologists have since reported mercury artefacts (including small deposits of elemental mercury, and much more commonly, powdered cinnabar and cinnabar-painted objects) at pre-Columbian Maya settlements across a wide area of present-day Mexico, Guatemala, Belize, Honduras and El Salvador [see Gliozzo (2021) for a recent summary of Maya sites with evidence of cinnabar use in burial contexts]. Gazzola (2009) and other archaeologists correctly note that many reports of cinnabar in the archaeological literature do not mention how cinnabar was identified, and some reports of cinnabar may be other red-coloured minerals, such as haematite.
Cinnabar was being used by the Maya in burial contexts from the Middle Preclassic onward at sites including Tikal (Welsh, 1988), Chiapa de Corzo (Lowe and Agrinier 1960:46) and possibly Colha (Thompson, 2005). Maya burials in the Preclassic (and later periods) also achieved red decorations through the use of minerals such as ochre and haematite (Izzo et al., 2022). From the Early Classic onward, cinnabar use became much more widespread across the Maya world. Archaeological reports contain references to cinnabar-painted objects and cinnabar paint and pigment found in situ (Houston et al., 2009), burials (largely elite) dusted with powdered cinnabar, and much rarer instances of elemental mercury in vessels.
Powdered cinnabar (and rarely cinnabar ore) is ubiquitous across the Maya world but instances of elemental mercury at ancient settlements are very rare. Elemental mercury has been reported at just ten pre-Columbian sites (Table 2; Figures 2, 3), usually associated with ritual caches or elite burials alongside cinnabar and other precious materials and objects (Pendergast, 1982). The oldest deposit of elemental mercury found was in the Temple of the Feathered Serpent at the massive pre-Columbian city of Teotihuacan. Here, c. 200 ml of elemental mercury was deposited beneath the temple sometime between 100 and 300 CE (S. Gómez Chávez (2016, personal communication, 20 May). This places it some centuries earlier than other elemental mercury finds at Maya sites, which typically date to the Early to Late Classic in age (Table 2). The most recent Maya context found to contain elemental mercury was a ritual cache beneath a ballcourt in Lamanai, Belize, dating to the 10th century CE (Terminal Classic) (Figure 3) (Pendergast, 1980, 1982).
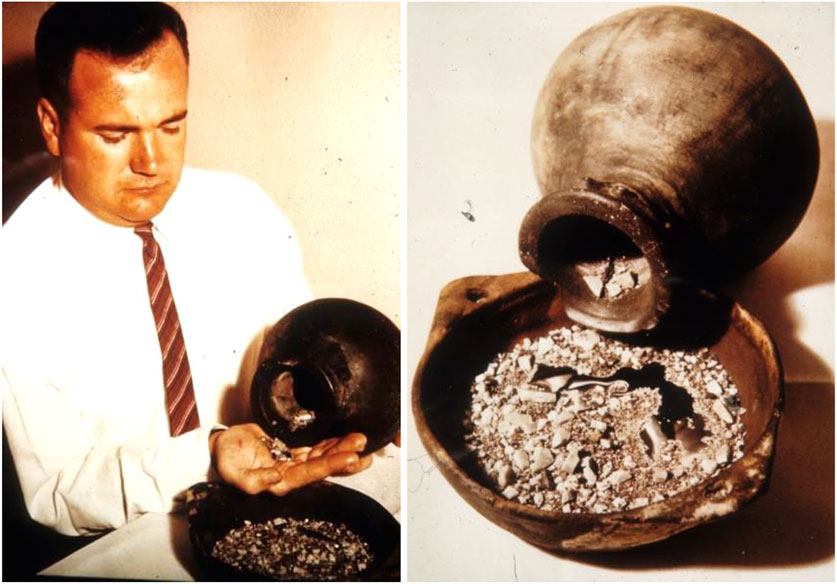
FIGURE 2. Geologist Jim Kitchen (left) holding the vessel he retrieved from the submerged site Lavaderos in Lake Amatitlán, Guatemala, and (right) a closer view of the contents of the vessel, showing stone debitage (?) suspended on elemental mercury. Estimates of the size of the bowl and depth of liquid suggest that there may have been 500 cm3 or more of elemental mercury inside, which accounts for the notable weight of the vessel reported by Kitchen (Deuel, 1974). Scanned version of original slides archived in the Milwaukee Public Museum. Used with permission.
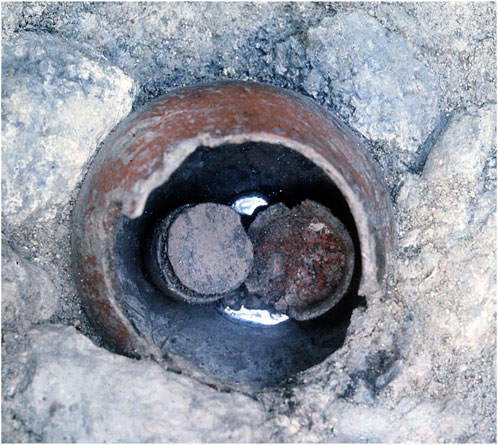
FIGURE 3. A brown thick-walled, deep bowl, photographed in situ in an excavated cache deposit from c 0.900 CE beneath the ballcourt at the Maya settlement of Lamanai in northern Belize. The contents of the bowl include two small vessels, one of which contained a small amount of cinnabar power, and beneath these, a small quantity of mercury in the base of the bowl. Scanned version of original slide taken by D.M. Pendergast, used with permission.
Despite rich archaeological documentation of Maya cinnabar and elemental mercury use, there remains much about its spatial and temporal history that is unknown. Focusing on the present, this includes very little research dedicated to understanding the environmental consequences of mercury use and deposition in antiquity. To expand on the archaeological data sets on ancient cinnabar and elemental mercury use, multidisciplinary teams working on Maya archaeological projects in recent decades have undertaken geochemical studies of excavated Maya occupation areas and environmental materials (mainly soils) (Cook et al., 2022). We may turn to the growing body of mercury data collected during these investigations as a means of quantifying the environmental legacy of ancient mercury use by the Maya. This review is the first to identify all known research on mercury in ancient Maya settlements across Mexico and Central America and to synthesis these findings to construct a region-wide environmental history of anthropogenic (and anthropogenically-related) mercury, beginning eighteen centuries before the onset of the industrial revolution.
Mercury in soils and sediments at Maya sites
Literature review
To understand the environmental context and consequences of the long history of mercury use by the ancient Maya, we conducted a literature review that spanned the period 1970 to 2022 using Web of Science and Google Scholar databases to identify all known published research (in any language) where the mercury content of soils and sediments from historic Maya contexts had been determined. We used search terms for all fields (title, abstract, keywords, full text), including “mercury” and “cinnabar” with “archaeology”, “history”, “ancient”, “land use”, and “impacts” with geographic-specific terms used to broadly match the extent of the Mesoamerica region (present day Mexico, Guatemala, Belize, El Salvador and Honduras) (Figure 4). We did not include unpublished and non-peer reviewed research, which meant some unpublished research and thesis studies were not considered further.
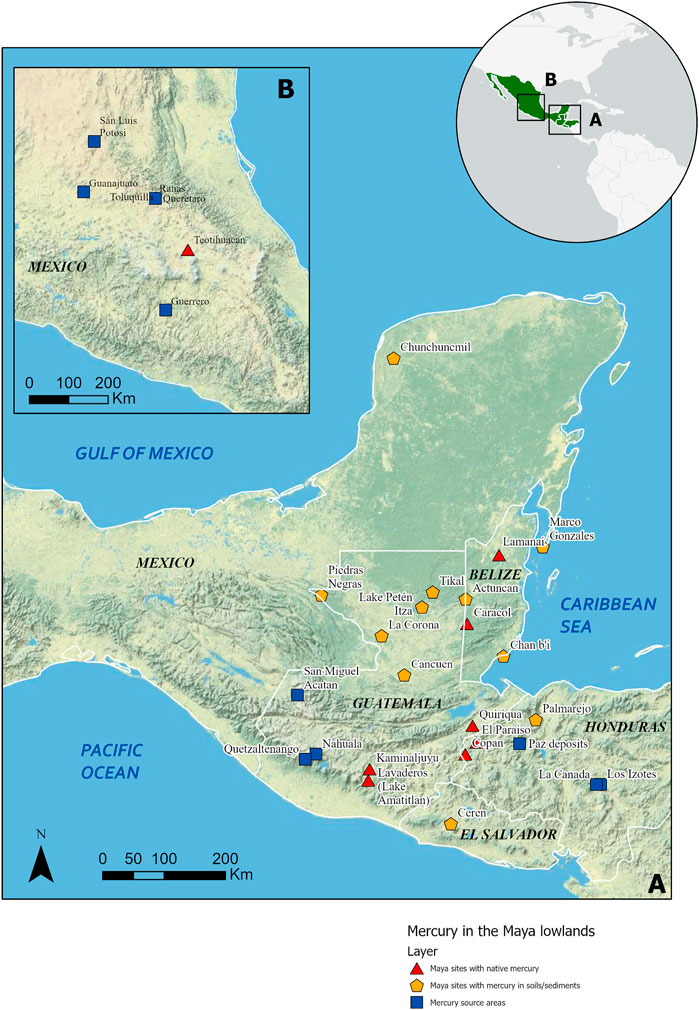
FIGURE 4. The Maya world and broader Mesoamerica region, encompassing Mexico, Guatemala, Belize and parts of Honduras and El Salvador, showing locations of sites discussed in this paper. Map prepared by Anaïs Zimmer using the National Geographic World Map (2011) in ArcGIS Pro. Date sources: National Geographic, Esri, DeLorme, HERE, UNEP–WCMC, USGS, NASA, ESA, METI, NRCAN, GEBCO, NOAA, iPC.
The literature identified in our search mostly featured mercury measurements on samples collected from well-defined, previously sealed, archaeological contexts, often with co-located chronological markers that provided broad temporal context (Section 4). Mercury data from these studies, therefore, can be viewed as contemporaneous with, and a consequence of, the human actions recorded in the archaeological and sedimentological strata investigated.
The literature review shows two broad categories of research papers with mercury datasets from pre-Columbian Maya settlements: 1) measurements of total mercury content and details of mercury determination parameters included (including evidence of standard geochemical approaches, use of mercury standards, evidence of measurement reproducibility and experiment detection limits). These studies may also have a research design optimised to assess the presence of anthropogenic mercury in Maya landscapes; 2) measurements of mercury that do not reproduce the absolute concentration of mercury due to the use of partial chemical extractions, typically non-concentrated acid digestion or other non-standard approaches, and research designs (Table 3). We stress that categorisation of previous research is not a comment on the quality of the research or datasets in any way, but simply a way of broadly assessing the applicability of prior research to the aims of this review.
Mercury analytical approaches
Mercury analysis of soils and sediments from Maya sites has been undertaken using a variety of analytical approaches meaning that direct comparisons between past datasets need to be undertaken with some caution (Turner et al., 2021). Most of the studies identified in our review detail the particle-size fraction of samples analysed for Hg content. This is an important consideration given the propensity for heavy metal concentrations to generally increase with decreasing particle size, with the highest particle-size effect often seen in the silt fraction (for example, in the <45 μm fraction in Wang et al., 2006). Most of the studies in this review reported Hg values determined on the <2 mm soil fraction (from Chunchucmil, Tikal, Lake Petén Itza, Piedras Negras, Cancuén, Cerén), but we note that most Maya lowland region soils are dominated by much finer clay-rich (<2 μm) material (Beach et al., 2015; Cook et al., 2022). The Hg data from La Corona, Chan b’i and Actuncan are from samples that were not sieved, but instead pulverized prior to analysis. At Palmarejo, Hg was measured on the <1 mm fraction after pulverisation, and at Marco Gonzalez the <125 μm fraction was selected for Hg analysis.
Mercury analytical methods in the reviewed literature fall into two broad categories: total sample decomposition and partial chemical extraction (Table 3). Studies that have used total sample decomposition approaches have either leached samples with concentrated acids or have directly decomposed samples prior to elemental measurements on soil extractants (by ICP-AES, ICP-MS, or CVAFS), or via direct combustion of powdered sample and AAS (e.g., Direct Mercury Analysis [DMA])2. These approaches provide the opportunity for the total concentration of Hg in environmental materials to be measured. Hg data obtained by these approaches should closely approximate the “real” concentration of Hg in a sample (high accuracy), and measurements of standards with known concentrations of Hg are used to determine accuracy. Partial extraction approaches are successful in liberating Hg from soil samples, with extractants subsequently measured by ICP methods. The partial leaching of soils using diluted HCl (e.g., Wells, 2004) or a mixture of diluted HNO3 and HCl acids (Aqua Regia) has been the most common method used in the Maya soil literature to date. LeCount et al. (2016) and Fulton (2019) reported that their results using partial digestion methods showed “… 5% variation on the NIST CRM (US. National Institute of Standards and Technology Certified Reference Material) for all standards and samples (including replicates), and ≤5% error on internal quality control (blanks)”. We note that certified elemental concentrations for NIST soil and sediment CRMs are determined by total digestion methods, and that partial digestion of CRMs may not result in full certified elemental concentrations being reproduced.
Another common methodology applied to soils from the Maya region is digestion by diluted DTPA (diethylenetriaminepentaacetic acid). This partial extraction procedure is thought to extract up to c. 20% of the total elemental fraction in a soil. Analysis of NIST SRMs by Parnell et al. (2002a) using the DTPA approach resulted in differences (presumably underestimates) from the certified Hg values of more than 20%, but less than 20% for other elements measured. The DTPA approach has its origins in the agricultural soil science literature and along with other partial extraction approaches (such as dilute Aqua Regia extraction) is thought to approximate the non-matrix bound component of a soil or sediment sample, allowing analysts to measure a fraction of the elemental concentrations in a sample rather than the soil bulk chemistry. Researchers using this approach argue that it results in concentration data that approximate the anthropogenic chemical “additions” to a soil, and so the use of partial extraction approaches in soil chemistry is thought to provide datasets applicable to archaeological studies.
Numerous studies from across Mesoamerica have shown the utility of these different analytic approaches in soil archaeological prospection studies. When using a partial extraction procedure, analysts can monitor analytical precision through replicate samples digested via the same approach that should give similar results. However, partial extraction experiments provide limited opportunities to measure the accuracy of measurements. Certified reference materials (CRMs) contain elemental standards of known concentrations that are determined by total decomposition approaches (Cook et al., 2006): using standards of known concentration then in partial extractions experiments means that stated certified values for all elements cannot be reproduced in full. Nonetheless, Maya soil research that has used partial extraction methods has been critically important in identifying the presence of elevated levels of Hg in ancient contexts. Indeed, by their very nature and intent, partial extraction experiments will generate measurements of mercury in soils and sediments that underestimate (by some margin) the absolute magnitude of Hg in archaeological contexts. Whether total or partial abundances of Hg are measured, at the site scale, it is the relative changes in measured Hg (and other elemental concentrations) and resulting spatial or temporal (downcore) patterns that have been useful for archaeological and paleoenvironmental interpretation (Wells et al., 2000). If research objectives require the determination of absolute values of Hg, as part of an assessment of mercury toxicity in soils and sediments, for example, then there should be a preference towards approaches that provided total mercury concentrations that are directly comparable with certified values.
Classic period Maya settlements with elevated environmental mercury
Chunchucmil
The most northerly study site in this review is the archaeological zone of Chunchucmil, a former Maya settlement in the northwest of the Yucatan Peninsula of Mexico (Figure 4). Chunchucmil became an urban centre during the Early Classic period, with a peak population greater than 30,000 (Hutson and Terry, 2006). The site is situated on a low relief, low elevation limestone plain. Surface materials range from skeletal soils through to caliche or petrocalcic horizons of up to 1 m thickness. Beneath are deeper weathering layers of carbonate saprolite (known locally as sascab [sahcab]) (Luzzadder-Beach et al., 2011).
Hutson and Terry (2006) investigated the inorganic chemistry of plaster floor areas on soils in three Maya domestic structures at Chunchucmil dating to the Early Classic to determine past activities. A total of 139 samples were partially digested using the 0.005 M DTPA approach of Lindsay and Norvell (1978). The extractant was analysed by ICP-AES for the concentrations of nine elements, including Hg. Most floor areas had concentrations of Hg less than 0.1 μg g−1 (and half of all samples had Hg values >0.05 μg g−1) but a small area adjacent to a human burial with rich offerings recorded four values of Hg above 10 μg g−1, reaching a maximum of 11.2 μg g−1 (Hutson and Terry, 2006; SI). Hutson and Terry (2006) explain the localised maximum Hg values as recording past cinnabar use as part of the mortuary treatment and installation of the burials beneath the floor.
Luzzadder-Beach et al. (2011) determined the inorganic chemistry of soils and sediment from a range of contexts across the Chunchucmil site. In this study, they compared partial digestion methods (0.005 M DTPA) with total digestion (Nitric/Perchloric acids (NP)) of their samples. Many locations recorded negligible Hg, but their survey detected several highly localised values of Hg in the 1–10 μg g−1 range. Sediments co-located with Early Classic Maya vessels had 9.5 and 9.7 μg g−1 (NP) of total Hg measured. Elsewhere, a buried soil with co-located Maya vessels recorded total Hg of 7.2 μg g−1 (NP). Sediments that have infilled Maya reservoirs at Chunchucmil were sampled by Luzzadder-Beach et al. (2011) for their geochemical properties. One reservoir had sediments with maximum Hg values of 11.3 and 13.9 μg g−1 (DTPA); a second reservoir sampled had sedimentary infill with a maximum value of 5.1 μg g−1 of Hg (NP).
Marco Gonzalez
The Maya site of Marco Gonzalez provides an important contrast to other Maya settlements in this review because Hg measured here was not clearly associated with ancient mercury use. The site is located at the southern end of Ambergris Caye, 17 km off the mainland coast of Belize, and was active in circum-peninsular trade and commerce between c. 300 BCE and 1500 CE (Graham and Pendergast, 1989; Guderjan, 1995; Aimers et al., 2017; Graham et al., 2017; Graham and Howie, 2021). The first evidence of environmental Hg was revealed as part of broad investigations into the impact of ancient human activities on soil formation (Graham et al., 2017). Employing a total sample decomposition approach (concentrated Aqua Regia leaching and measurement by CV-AFS), Macphail et al. (2017) identified elevated total Hg (up to 0.5 μg g−1) in organic-rich surface soils from Maya structures (Op 13/2-3 (Str.19 and 8) and Structure 25) that related to underlying Hg-enriched anthropogenic deposits (fish bones).
Turner et al. (2021) conducted research across several geomorphic and archaeological contexts at Marco Gonzalez to test for the presence of mercury as a record of past Maya activity. The study mapped complex patterns of Hg enrichment across the site in surface soils and temporally via sediment cores. Turner et al. (2021) found the greatest concentrations of total Hg were in organic matter-rich anthrosols (a peak value of 1.3 μg g−1 detected near the centre of the site, adjacent to Maya structures) and in a low organic anthrosol containing ceramic, charcoal and obsidian fragments (0.78 μg g−1). Significantly, a comparable peak concentration (0.8 μg g−1) corresponding with 20th century fossil-fuel combustion atmospheric indicators in a210Pb-dated core from a site-adjacent pool. Low Hg contexts and sediments were almost uniformly those consisting of sediments from naturally low Hg reef-limestone bedrock and reef sediments as would be expected in biogenic, non-mineral crustal materials. Some contexts sampled for mercury at Marco Gonzalez may have experienced blurring of modern and past mercury accumulation, from human and biogeochemical processes, meaning that apportioning a strictly pre-Columbian anthropogenic source to mercury anomalies here is not entirely straightforward, i.e., there are very clear inter-relationships with other trace metals and organic matter in the sediments.
La Corona
The Maya site of La Corona is in the north-western Petén karst region of Guatemala. Traces of human settlement at La Corona date from the Early Classic (350–600 CE), with a peak population in the Late Classic, and site abandonment by c. 900 CE (Canuto and Barrientos, 2011). Geoarchaeological research on three Maya patios associated with the site’s palace in the Northwest Group was undertaken by Lamoureux-St-Hilaire et al. (2019) to better understand Classic Maya palaces and the activities that once occurred within them. Excavated plaster floors, that date to c. 790–850 CE (the later history of La Corona) were sampled following a metre-grid and 215 plaster-soil samples were analysed for the inorganic chemistry by ICP-MS following a partial acid extraction (dilute Aqua Regia: 0.6 M HCl and 0.16 MHNO3). Samples were not heated as part of the preparatory or analytical procedures. Most of the areas sampled for their study had little or no measurable Hg. Seven samples had Hg values ≥0.01 μg g−1 while the maximum Hg concentration in this study was 0.058 μg g−1 (sample F-9 #11). The authors proposed that the elevated Hg values, located on two areas on Maya patio areas, may relate to past use of cinnabar, or from the contribution of cinnabar-based based paints on structures that have decayed through time and enriched the floor area adjacent to previously painted walls and doorways.
Tikal
Tikal, located in present day northern Guatemala, was one of the dominant centres of Maya world during the Late Classic period, c. 600–850 CE (Martin and Grube, 2008). Beginning as a village centred on a rare natural spring around 700 BCE, Tikal grew into a regionally prominent centre during the Late Preclassic period, c. 400 BCE –100 CE, and grew to even greater prominence in the Early Classic period, c. 250–600 CE, including establishing an alliance of uncertain nature with the Central Mexican city-state of Teotihuacan (Dunning et al., 2015a). Situated at the southern extreme of the karst Elevated Interior Region on the Yucatan Peninsula, urbanisation at Tikal was dependent on the development of a system of reservoirs to capture and store rainwater during the annual wet season to survive the 5-months-long winter-spring dry season (Dunning et al., 2012, 2022; Scarborough et al., 2012).
Initial laboratory testing of the sediments in many of the reservoirs at Tikal included palaeoecological, mineralogical and geochemical assays (Lentz et al., 2014; Lentz et al., 2015; Dunning et al., 2015b; Tankersley et al., 2015), but not Hg. However, subsequent geochemical examinations included Hg, targeted because cinnabar was used in burial and other ritual contexts at Tikal by the Maya (Harrison, 1999; Haviland, 2015). Cinnabar was found in multiple architectural contexts in the North Acropolis and Central Acropolis, areas of the city that shed runoff into the Temple and Palace reservoirs, a circumstance that created a potential health hazard for anyone who consumed their waters (Lentz et al., 2020).
Total Hg content of sediment samples from the infilled Tikal reservoirs was determined by Todd P. Luxton of the U.S. Environmental Protection Agency (Cincinnati) using atomic absorption spectrophotometry following catalytic decomposition and gold amalgamation using a Milestone Direct Mercury Analyzer after USEPA method 7473 (Lentz et al., 2020). A total of 32 samples were analysed from the Temple, Palace, Corriental, and Perdido reservoirs (Lentz et al., 2020). Twenty-five samples contained total Hg content that exceeded the toxic effect threshold (TET), the level above which the sediments are deemed to be heavily polluted (MacDonald et al. 2003). Ten samples had levels below the TET (1.0 μg g−1). Four samples from the Palace Reservoir and one sample from the Temple Reservoir had Hg levels exceeding ten times the TET concentration, with a peak total Hg concentration of 17 μg g−1 measured, in sediments dating to the Late-Terminal Classic Period. Four of the five samples with extremely high Hg readings were from strata associated with the Late or Terminal Classic periods, the time just before the abandonment of the site. Concentrations during these late times were likely exacerbated by severe droughts that would have lowered water levels in the reservoirs (Lentz et al., 2020).
Lake Petén Itzá
Lake Petén Itzá is a large (c. 99 km2) and deep (up to 160 m) water body in the northern Petén of Guatemala, encircled by numerous pre-Columbian settlements, including the former capital of the Itza people, Tayasal (Rice and Rice, 2018). Palaeolimnological research from Lago Petén Itza has produced a recorded of changing mercury input into the lake basin over millennia (Battistel et al., 2018). Sediment core PI 5 VI 02 11B was collected in c. 50 m water depth in the northern basin of Lake Petén Itzá (Hillesheim et al., 2005) and the inorganic chemistry (including mercury) of consecutive sediment samples analysed by ICP-MS following total digestion in concentrated Aqua Regia (in sealed microwave digestion vessels). Radiocarbon dating of the core suggests that it represents sediment accumulation during the last 5,500 years, including the Maya Preclassic, Classic and Postclassic periods. Core PI 5 VI 02 11B is the longest record of mercury accumulation published for the Maya region and provides an opportunity to examine long-term variations through Maya history. The lowest Hg value in this core was 0.032 μg g−1, which dates to around 2600 BP. The 5500-years record features a distinct peak in Hg concentrations that appears to have begun after c. 2500 BP, with Hg values increasing to the highest total Hg content recorded in the core, 0.11 μg g−1 at c. 2250 BP. Battistel et al. suggest that the peak (approximately) correlates with a known eruption of volcano Acatenango in southern Guatemala, and that the mercury in their sediment core is “probably natural”, the consequence of atmospheric deposition of volcanic ejecta. No direct evidence of volcanic input into the lake sediments (e.g., tephra from Acatenango) in the late Holocene was reported in this study, but other cores published from the Lake Petén Itzá do report tephra layers, but only before c. 10 ka, and none from any later time in the Holocene (Kutterolf et al., 2016). Other possible sources for the peak in Hg could include an erosive episode transporting older catchment materials with elevated Hg into the basin, or anthropogenic Hg from nearby Maya settlements.
Piedras Negras
Piedras Negras was a large Maya city in NW Guatemala, located on the Usumacinta River bordering present-day Mexico (Figure 4). Human settlement at Piedras Negras began in the 7th century BCE and the site was abandoned by the early 10th century CE. During this period, the site experienced two population peaks: one in the Late Preclassic, and then a second in the Late Classic Period around 500 years later. The site lies on a Cretaceous carbonate rock plateau in a region of tropical semi-evergreen rainforest (Beach and Dunning, 1995). Soils at Piedras Negras are primarily rendolls derived from the underlying Hg-poor limestone parent material, with some quaternary alluvium input from the nearby Usumacinta (Aliphat Fernández, 1996). Wells et al. (2000) collected soil samples from two excavated parts of the city (Operation 10 and 33) that dated from the Late Classic (650–800 CE) Maya occupation, based on ceramics identified (Parnell et al., 2002a). Thirty soil samples were collected from an excavated level that spanned Maya platforms, patios, and areas between house-mounds (Operation 10). An additional thirty samples were collected from floor areas of an excavated elite Maya residential complex in the South Group Plaza region (Operation 33). Soil samples were also collected from “off-site” areas to calculate geochemical background datasets against which the anthropogenic samples could be compared. Samples were air-dried and sieved to 2 mm and leached using 0·005 M DTPA buffered at pH 7·3 to partially leach the samples. The leachate was analysed for multi-elemental content using ICP-AES.
Hg concentrations in the soils varied substantially across the floor areas studied. Soils collected from Operation 10 had the greatest variability, with the highest Hg values being localised, and the greatest value (2.7 μg g−1) detected in the centre of a patio area. Most locations recorded comparatively lower Hg values (0.4–0.8 μg g−1). By contrast, all materials analysed from Operation 33 had elevated Hg values ≥2.0 μg g−1 with a maximum value of 5.6 μg g−1 measured on soil collected next to a Maya wall. Wells et al. proposed that this wall may have been painted with cinnabar-based pigment, a practice observed at some Maya settlements, with the decay of the painted wall contributing Hg to the floor surface.
Chan b’i
Chan b’i is a coastal pre-Columbian Maya site in southern Belize (Figure 4). Chan b’i is one part of a pre-Columbian salt production area, identified by preserved wooden structures and the remains (briquetage) from salt production by heating brines in ceramic vessels from the Classic Maya period (Sills et al., 2016). Forty surface samples of seafloor mangrove peat sediment were analysed for their geochemical properties (including Hg content) with the aim of providing additional information on Maya structures and past industrial activities (Sills et al., 2016). Samples were collected along two transects along an excavated trench c. 0.4 m below the water; there is no information in Sills et al. (2016) about the possible chronology or stratigraphic context of samples. The geochemical data in Sills et al. (2016) shows Hg concentration in the sediments were extremely low and (presumably) below detection limits (40 values reported as 0.000; no units given). A single Hg determination of 0.001 μg g−1 was reported in a later publication (Sills in Turner et al., 2021). The site’s history as a coastal salt works, with no domestic or ceremonial architecture, makes it highly unlikely that the Maya used cinnabar here. The negligible mercury detected at this site precludes it from further consideration in this review.
Actuncan
Actuncan is a former Maya urban centre located in the Mopan River valley of western Belize. The site was established in the Middle Preclassic period c. 1000 BC. Actuncan is described as having two distinct parts. Actuncan North was the main residential area, while Actuncan South comprised public spaces dedicated to religious matters, including a temple complex and related structures. Two publications on Actuncan report Hg measurements made on excavated soil and plaster floor areas. First, LeCount et al. (2016) sampled 12 excavated plaster floors and patio areas in Group 8 in Actuncan North. Most of these contexts were Late Classic age, though a smaller number of floor areas sampled were from the earliest history of the site, from the Terminal Preclassic and Early Classic periods. Second, Fulton (2019) reported the results of inorganic chemical analysis of 703 on-site soils samples collected across Group 1 (Late to Terminal Classic occupation areas), Group 5 (Operation 47), Group 6 (Operation 46), and from excavated spaces between architecture (Operation 48), and also eight off-site control samples.
Both studies at Actuncan used a “weak acid” digestion procedure followed by measurement of multiple elements (including Hg) with the aim of measuring a fraction of elemental concentrations in soils, rather than total (or near-total) concentrations. Individual measurements are not reported in LeCount et al. (2016); instead, average elemental concentrations across each floor area are tabled. The highest average Hg value reported (0.0016 μg g−1) came from a Late Classic plaster and soil floor in Structure 21B “Squash”, while the second highest average Hg value came from another Late Classic floor, in Structure 19–1st “Blue” (0.0013 μg g−1). Elevated Hg, along with Fe, on these floors was explained as a possible relict of past ochre and cinnabar use. Fulton (2019) reported a maximum Hg concentration of 0.74 μg g−1 at Actuncan from the Group 1 occupation areas. This was the only highly elevated Hg measurement recorded across Group 1; three other samples contained Hg at 0.01 μg g−1, while the remaining 192 samples analysed from this area had reported Hg values of 0.00 μg g−1 (Fulton, 2019; SI).
Cancuén
Cancuén is a Maya archaeological site located in the upper Pasión River valley in the southern Petén lowlands of Guatemala (Figure 4). The epicentre of Cancuén occupies a c. 3 km2 peninsula on the inside meander bend of the Pasión River. Cancuén’s position on a main river thoroughfare through the Maya world allowed its inhabitants to benefit from the trade of essential materials and high-value goods (including geological resources) from the volcanic highlands in the south to Maya cities located north throughout Mesoamerica (Cook et al., 2006; Andrieu et al., 2014; Demarest, 2014). Products such as jade and obsidian arriving overland from the upland regions experienced additional production at Cancuén before transportation northwards to Maya cities such as Ceibal, Tikal, Piedras Negras, and Palenque. Evidence for the trade connections between Cancuén and the major Maya cities to the north includes geochemical studies on jade and other geological and archaeological materials (Kovacevich et al., 2004; Bishop, 2014).
Geoarchaeological investigations at Cancuen have focused primarily on providing new insights into how different parts of the city were used, but also some palaeoenvironmental data sets (Kovacevich et al., 2004; Beach et al., 2006; Cook et al., 2006; Cook et al., 2017). Cook et al. (2006) collected soil samples using a grid sampling pattern across two excavated Maya floor areas associated with domestic living and workshop spaces (Kovacevich et al., 2004). All soil samples were collected from recently excavated (sealed) contexts ensuring that the soil floor geochemistry was associated with (and a consequence of) Maya occupation with no modern inputs or modifications. The total Hg content of soil samples was determined by ICP-MS following strong acid (concentrated HNO3) digestion of the soils in sealed microwave digestion vessels following NIST method 3051. Limit of detection for Hg analyses was 0.008 μg g−1. Background Hg levels at Cancuen are thought to be low due to the mercury-poor limestone bedrock: Cook et al. (2006) determined a maximum background (pre-Maya) Hg level (mean plus two standard deviations) of 0.042 μg g−1. All 82 Maya floor samples analysed recorded total Hg content that was enriched with respect to the site’s pre-Maya (natural) background, at levels of up to 24 times (total Hg: 1.0 μg g−1) greater than the mean background Hg concentration. The highest total Hg concentrations were associated with special Maya deposits, such as human burials and ritual caches, where cinnabar is very likely to have been used by the Maya, while broader-scale enrichment across the floors suggests that mercury enrichment from cinnabar, as a powder or as vermillion pigment, occurred as part of craft production activities by the Cancuen Maya (Cook et al., 2006: 636–637).
Palmarejo
Palmarejo is a Classic Period Maya settlement in present-day northwest Honduras. Research to date has identified the remains of 93 pre-Columbian buildings, most of which date to the Classic period, c. 400–1000 CE. Fulton et al. (2017) investigated the North Plaza area of Palmarejo, a 40 × 40 m space encircled by six Maya platforms, using a 2 m square sampling grid. Soil samples (n = 297) were collected from a Late Classic period anthropogenic horizon (identified as a distinct lime-plaster floor layer preserved beneath post-abandonment soil A-horizons). Additional soil samples (n = 25) were collected from locations distant from any Maya archaeology with the aim of determining the pre-Anthropogenic, or background, environmental chemistry. Anthrosol and background soil samples were leached using the diluted Aqua Regia extraction approach (0.60 M HCl +0.16 M HNO3 extraction at room temperature for 0.5 h) used previously in the soil geochemical studies (after Wells et al., 2007) followed by the measurement of the concentration of 22 elements (including Hg) using ICP-MS. Fulton et al. (2017) (Table 1, pg. 1064) published summary statistics of the geochemistry of the Maya soils, showing Hg concentrations varied from 0 (presumably, below detection limits) to 3.4 μg g−1, with a mean Hg value of 0.3 μg g−1; the mean “background” Hg concentration was 0.1 μg g−1. Despite at least one sample recording high Hg content, Fulton et al. (2017) concluded that Hg was (in general) too low across the study site, with little spatial variation, to be useful for detecting former Maya activity areas (pg. 1068).
Cerén
The Cerén archaeological site was a small Maya village in present-day El Salvador at the southernmost extent of the Classic Maya world (Sheets, 1992; 2010). Cerén is a unique archaeological site in that it was not gradually abandoned at the end of the Classic Period like other Maya settlements in this review. Instead, it was rapidly buried by c. 4 m of tephra from an eruption of the nearby Laguna Caldera Volcano in c. 6th century CE (Sheets, 1979). Archived soil samples, collected during the excavation of the site in the early 1990s, were provided to Parnell et al. (2002b) for geochemical testing in 1998. The original samples (n = 27) were collected from a soil floor area of a small domestic structure and included materials from inside the structure and immediately outside in occupation areas. The soils were digested using the weak DTPA method detailed previously. The peak Hg value at Cerén (10.14 μg g−1) was record in the northern end of the East room in Structure 10. However, all samples from inside and outside the Maya structure recorded elevated Hg concentrations, from 3.58 to 10.14 μg g−1. Other Hg measurements from soils nearby (from a midden, a path and an old milpa area), also recorded elevated Hg, but lower than what was measured in and around the Maya house structure. The archaeology of these excavated spaces included numerous materials related to ceremonial activities. Artefacts included elaborately painted vessels, ritual costume accessories, and pigment containers found in the centre room (Parnell et al., 2002b). While cinnabar-based paint or pigments were not located in the room, it is feasible, given the focus on crafts and decorations, that cinnabar-pigment had previously been used in this space.
Mercury enrichment at Maya settlements: Data summary
Researchers have determined the mercury content of soils and sediments contemporaneous with the Classic Period Maya at ten sites across present-day Mexico, Guatemala, Belize and El Salvador. Collectively, these datasets confirm that at (or near) ancient Maya settlements, elevated mercury (often many times greater than the TET) has been preserved in environmental materials. Only one of the Maya sites investigated, Chan b’i, did not have measurable Hg reported, best explained by the site not being permanently occupied by the Maya and because it has no archaeological evidence of previous cinnabar or elemental mercury use. Seven of the studies in the review determined Hg concentrations using partial digestion procedures. It is feasible, therefore, that Hg data from these seven sites (Cerén, Piedras Negras, Palmarejo, La Corona, Actuncan) may be an underestimate of the total Hg content from the Classic Maya period.
Discussion
Spatial and chronological patterns of preindustrial mercury
Elevated Hg has been detected across many former occupation spaces and in environmental archives (sediment cores) at (or near) ten Classic Period Maya settlements across a region of more than 250,000 km2. At the site level, Hg enrichment appears highly localised in “hotspots” on internal Maya floor areas or on external patio spaces, often at very small (<102 m) spatial scales. An extreme example of this pattern comes from the work of Fulton (2019) at Actuncan, where just four out of 196 samples collected across ancient Maya occupation spaces had measurable Hg. Less commonly, some former Maya sites have elevated Hg across entire occupation areas, such as at Piedras Negras. In some studies, elevated Hg concentrations were co-located with archaeological evidence of Maya mercury (cinnabar) use. It is probable, therefore, that outside of areas of substantial Maya use of cinnabar or elemental mercury, the amount of Hg in the environment may decrease quickly to background levels. There are few data available that confirm this (Cook et al., 2006; Hutson et al., 2007), but the Hg record in sediments samples from the central Petén provide some support for this position. Core PI 5 VI 02 11B, collected in the deep-water Lake Petén Itza, recorded peak total Hg content of 0.11 μg g−1 in sediments dated to the second/third century BCE (the Late Preclassic period) which is at least an order of magnitude lower than Hg levels detected in soils within some Maya settlements (Table 2; Figure 5). In contrast, mercury incorporated into soils and other catchment materials was concentrated in the reservoir sediments of the Maya at Tikal, in the central sector of the city. There are some broad inter-site differences in Hg values reported in the literature that are archaeologically significant: soils samples from Palace areas (La Corona and Actuncan) had lower concentrations of Hg, while the floors of domestic and workshop areas (e.g., Cancuen, Marco Gonzalez) recorded higher levels of Hg (Figure 5).
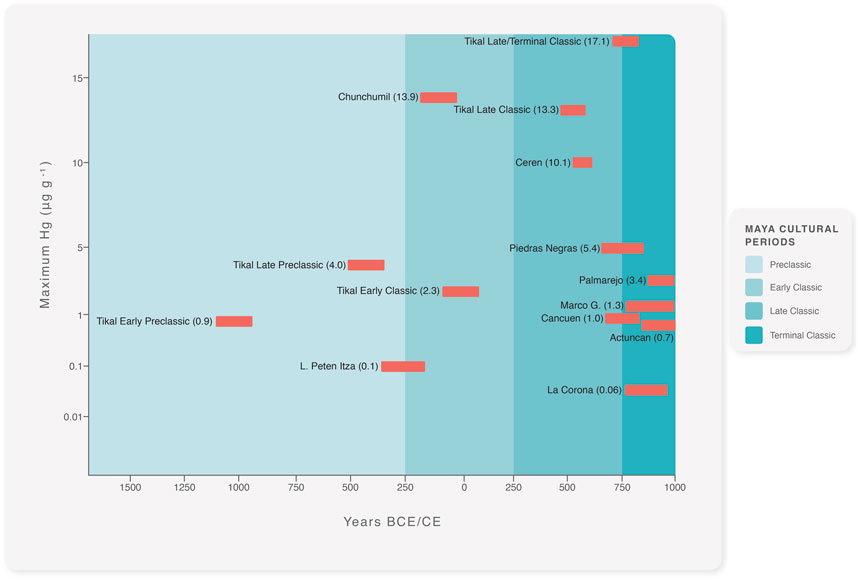
FIGURE 5. The chronology and magnitude of mercury pollution in soils and sediments at (or near) ten pre-Columbian Maya sites. Mercury data and chronological information collated from the sources cited in Section 4.
The quality of chronological information associated with the elevated soil and sediment Hg is varied. Some soils investigated have co-located artefacts of known age, providing a terminus post quem for Hg additions. The two sedimentary records of Hg accumulation (from Tikal and Lake Petén Itza) are dated by radiocarbon and archaeological information. These two records are, at present, the only detailed studies of changing Hg inputs through the Maya period. Datasets from Maya sites that predate the Late-Terminal Classic Period are nearly absent (Figure 5), and we must be aware that a bias towards mercury accumulation near the end of Classic Maya society is likely. Certainly, the temporal record of Hg accumulation at Tikal suggests that people here were using mercury resources (certainly cinnabar) extensively by the Late Preclassic Period, increasing through the Early Classic and reaching its highest levels in the Late-Terminal Classic (Figure 5) (Lentz et al., 2020). The Lake Petén Itza record is, in contrast, a less definitive account of Hg accumulation, as the core was collected at distance from any particular Maya site, and so the record here of a Late Preclassic period peak in Hg of 0.1 μg g−1, while chronologically precise, is difficult to connect to any specific source, and it may be the cumulative account of mercury inputs from around the immediate catchment in the Preclassic or even distant volcanism. There is one instance of in situ elevated Hg from an earlier Maya occupation level; LeCount et al. (2016) reported Hg in Maya floors dating to the Terminal Preclassic (100–250 CE) at Actuncan.
Ancient Maya mercury use, origins, environmental accumulation and persistence
The low natural mercury content of Maya lowland soils and sediments is arguably due to the near absence of mercury in the limestone environment. Aeolian inputs to Maya lowland soils include local and regional ejecta from Central American volcanoes (which may contain trace level Hg) and aeolian dust from the Sahara-Sahel region (Cabadas-Báez et al., 2010; Bautista et al., 2011; Tankersley et al., 2011; Tankersley et al., 2016). Not all Maya sites are located on carbonate substrates, however; one of the Maya sites in this review, Cerén, is built upon soils derived from multiple late Holocene tephra deposits (Sheets, 1992) which could contribute some Hg. However, the spatial patterns of elevated mercury across ancient Maya surfaces at Cerén cannot be explained by a diffuse mercury input such as tephra, and Maya locations at Cerén recorded mercury levels that are higher than those found at offsite locations.
Other non-anthropogenic sources of Hg enrichment in soils can include groundwater and plant remains, the bioaccumulation of Hg in fish and other species, and volcanic ejecta (Cook et al., 2006). Battistel et al. (2018) proposed that volcanic tephra might explain localised peaks in Hg sediments from the Late Preclassic Maya period in cores from Lake Petén Itza, based on 1) similar timing in Hg peaks and past volcanic eruptions, 2) previous studies that show that tephra may have elevated Hg. This explanation does not readily account for the trends in elevated Hg at Maya sites described in this review. First, if tephra from volcanic eruptions was responsible for region-wide elevated Hg then we might expect similar concentration of Hg in many soils across the region, and also much lower levels of Hg than what has been reported at Maya sites. Secondly, research to date shows that Hg at Maya sites exhibits very high spatial variability: high levels of Hg are often spatially constrained in “hot spots” while other nearby areas have little or no Hg above background levels (of detection limits). Modern industrial pollutants, such as those from coal-fired power plants, can be a significant source of mercury (Schneider et al., 2021), though the remote locations of most Maya settlements in this review, far from industrial sources, and often deep sedimentary strata samples, and their sealed contexts since the Classic Period, exclude a modern industrial pollution source of the elevated Hg.
Where no other feasible explanations are available, Hg enrichment detected at Maya sites that dates the first millennia CE and earlier is likely a consequence of preindustrial anthropogenic mercury use by the Maya. Research that investigates the exact forms and nature of mercury in Maya soils and sediments is yet to be undertaken. However, the archaeological record suggests cinnabar or perhaps related HgS minerals, sourced from geological sources to the west and south of the Classic Maya region, were extensively used and are a likely source of the elevated Hg levels. Elemental mercury use by the pre-Columbian Maya is another possible source of environmental Hg accumulation. However, elemental mercury has not been found at the ten Maya sites with elevated soil Hg levels.
There are two main arguments to support an ancient anthropogenic source for the elevated Hg values measured at Maya sites. First, there is evidence from numerous Maya settlements of the use of mercury (mainly cinnabar, but also rarely, elemental mercury) before c. 1000 CE. Secondly, some artefacts of mercury use have been located adjacent to soils with elevated Hg levels (Cancuen, Cerén, Piedras Negras). It is entirely plausible, therefore, that the use of mercury by the Maya directly contributed cinnabar into soil floors, and that cinnabar powder and pigments could be transported from patio and floor areas in suspension or solution away from activity areas and into reservoirs, resulting in elevated concentrations of anthropogenic Hg in the environment of the Maya. A second, but related, process that may have contributed to historic Hg enrichment is the long-term decomposition, disintegration and perhaps leaching of Hg from cinnabar painted objects (plasters, ceramic shards) and these fragments and leachates being preserved in soils and sediments from the Maya period. The implication is that the mercury content of buried soils and sediment sequences may continue to increase after the abandonment of settlements by the Maya as cinnabar-decorated materials disintegrate. Buried Maya soils, excavated and studied today, frequently contain ceramic remains (including cinnabar-painted ceramic remains or perhaps ground cinnabar powder), so a model of post-abandonment Hg enrichment may be applicable across many parts of the Maya region where cinnabar or cinnabar-decorated objects where used.
Once cinnabar is reworked into soils and sediments it is likely to be relatively immobile, based on research on soils with ancient cinnabar inputs from Europe (Higueras et al., 2003), but it is plausible that fine-grained cinnabar in Maya anthrosols may be susceptible to the same physical translocation processes as other soil components in tropical environments (bioturbation, illuviation, eluviation). However, multiple studies have shown that subsoil (pre-Maya) soil horizons and “off site” soil locations have much lower Hg content, similar to that detected in the limestone bedrock geochemistry. In the absence of any evidence of substantial physical movements through the soil profile, research to date suggest that anthropogenic HgS, once incorporated into soil, remains part of the soil layer in which it was first accumulated.
Cinnabar or cinnabar-painted objects have been reported at (or near) half of the study sites where elevated soil Hg has been detected (Table 2). The sites which do not have an archaeology of mercury use by the Maya, nonetheless, may have a site history of Maya mercury use based on other lines of evidence (e.g., Chunchucmil (Hutson and Terry, 2006; Hutson et al., 2007)). In these cases, the elevated environmental Hg values reported may be considered a geochemical artefact or marker (Beach et al., 2015) of a specialised set of Maya actions, and while this evidence is invisible, it is observable, and is not a case of “archaeology of absence”. In these locations, and undoubtedly elsewhere, legacy mercury in pre-Columbian contexts may be the most unambiguous proof of past Maya mercury use, and the various ritual, industrial and domestic activities that used mercury minerals, that have left behind a permanent mark in the buried and preserved in the soilscape. Indeed, this association between elevated Hg and past Maya activities has been put forward as prima facie evidence that cinnabar must have been used at some Maya sites in the past (e.g., Lamoureux-St-Hilaire et al., 2019; pg. 778).
The geological sources for the cinnabar and elemental mercury found at Maya archaeological sites are not known. Earlier discussions of the origin of elemental mercury located at Maya settlements favoured a geologic source (cf. Fuson, 1969; Pendergast, 1982) and the literature refers to known and potential cinnabar sources in Mexico, southern Guatemala and Honduras (Figure 4). Cinnabar produced in the Central Mexico region (Figure 4) could have reached the rest of the Maya world via trade routes associated with Teotihuacan (Sharer, 2003; Sharer and Traxler, 2006). Central and West Mexico, while more distant from major Maya settlements, has arguably the best documented and abundant mercury resources in the region; mainly cinnabar, but also elemental mercury deposits usually co-located with other mercury minerals, but also found with non-mercuric lithologies and minerals (e.g., Gallagher and Siliceo, 1948; Panczner, 1987; Anon, 1996; Rodríguez-Galeotti, 2006). Archaeologists have proposed that pre-Columbian sites in Central Mexico near major cinnabar deposits, such as Ranas and Toluquilla (Figure 4), may have played a key role in cinnabar production and supply across Mesoamerica (e.g., Velasco Mireles, 2006; Nalda, 2010). Some of the mercury deposits in Central Mexico (in Guanajuato, Guerrero, and San Luis Potosí States), have been known since the earliest accounts of mining in the Spanish colony (Panczner, 1987, pg. 51, 269). Records from the Contact period (and archaeological data from before Contact) show that indigenous people of Mexico were mining minerals and metals since the 10th century BCE, perhaps earlier (Langenscheidt, 1986; Cárdenas Vargas, 1992). In the early Colonial period, vast amounts of mercury were needed for silver and gold mining in Mexico, which was initially imported from Spain, but later, from cinnabar sources in Peru (Nriagu, 1994; Lacerda, 1997; Cooke et al., 2009).
In the southern periphery of the Maya world, in present-day Honduras, the Paz cinnabar mining region is a short distance from Maya sites such as Copan and Cerén (this study), and the Los Izotes, and La Canada cinnabar deposits are a further 100 km south, all of which are part of the Metapan geological formation (Roberts and Irving, 1957). Gorokhovich et al. (2020) examined ore sources near Copan in northern Honduras and confirmed (using XRF) the presence of mercury-bearing materials nearby.
Some cinnabar and elemental mercury finds at Maya settlements have been associated with evidence of burning, and some archaeologists have hypothesised that these remains may reflect elemental mercury being derived (presumably by high-temperature heating and capture) from cinnabar. Subsequent claims that the elemental mercury located at Maya settlements came from the Maya smelting cinnabar (e.g., Looper, 2003) are yet to be substantiated and there is (to date) no archaeological record of elemental mercury production from cinnabar burning in Classic Maya society. Heating cinnabar ore in a sealed vessel, as proposed, to produce elemental mercury using conventional Maya vessels would be difficult due to the very high temperatures required, sufficient air flow and the specific vessel design required to capture gaseous Hg0 in such a way that elemental Hg could be collected with any degree of success. The earliest records we have of cinnabar smelting with retorts in the region show that it was underway by the mid-16th century in Mexico, using approaches imported to “New Spain” from Europe, possibly Germany. Barba and Herrera (1988) describe a small object recovered from San Jose Ixtapa, Mexico which may have been used in liberating elemental mercury from cinnabar, but this technology probably dates from after the Maya Classic period.
Instead of smelting, element mercury found at Maya sites may have come from some of the known geological sources of elemental mercury that are co-located with other mercury mineral deposits on the periphery of the Maya world, in present-day Mexico and Honduras, and possibly in the highlands of southern Guatemala (though sources here have not been confirmed) (Pendergast, 1982). It is difficult to envisage a means of elemental mercury collection, transport, and trade across long distances, though it would not have been an impossible achievement by the Maya. We agree with Pendergast’s (1982) observation that this may have been a painstaking and difficult process ensuring that elemental mercury was rare and highly prized. Additionally, we note it is may have been possible that interstitial Hg0 associated with mercury minerals found in mines, could be liberated by moderate heating of samples to release elemental mercury for collection from the mineral surface. Further, there are records globally of pre-Modern era elemental mercury production by simply burning cinnabar ore on brushwood fires, and then collecting pools of elemental mercury from the ashes (Farrar and Williams, 1977; Greenwood and Earnshaw, 2012):
This simple approach is one that could have been employed by the Maya to obtain elemental mercury, and if so, there would be little or no physical trace of this in the archaeological record over a millennium later. With no contemporary accounts or archaeological data to draw on, the exact means by which the Maya obtained elemental mercury may only be speculated on at this time. In any case, the rarity of elemental mercury and difficulty in obtaining and transporting it across the Maya lowland region may explain why so few instances of elemental mercury have been found at Maya sites. It is also plausible that other instances of elemental mercury use at Maya settlements did occur, but that these deposits have not survived until now, or instead, have not yet been located. Williamson (1996), for example, describes elemental mercury observed in a buried soil context at Copan that was likely held in a Maya container that has since disintegrated. There are very few instances globally where ancient elemental mercury has been successfully preserved until today. In the Mesoamerican region, only the Late Classic specimen from the Maya site of Lamanai and the recent finds from Teotihuacan still exist in archives, according to our records.
Pre-Columbian anthropogenic mercury: Pollution and human health
Anthropogenic Hg preserved at Maya sites greatly exceeds natural “background” levels based on the regional limestone geological substrate and upper range crustal concentrations. Each of the ten Maya settlements in this review have one or more contexts with Hg concentrations above “background” levels. Another means of assessing the magnitude of ancient Maya Hg enrichment is to compare the Classic Period Maya Hg levels with modern guidelines for safe exposure to total Hg in soils and sediments, as Lentz et al. (2020) at Tikal and Turner et al. (2021) at Marco Gonzalez have recently. If we use the sediment TET value of 1 μg g−1, then six of the ten ancient Maya sites in this review have at least one location with Hg values that exceed the TET. Some locations, such as Tikal and Cerén, recorded Hg above the TET in all samples analysed. The Late Classic Maya site of Cancuen recorded a maximum total Hg in soil of 1.0 μg g−1 on a soil floor area. While just at the TET, this concentration of Hg is 24 times greater than the maximum upper limit for background samples at the site (Cook et al., 2006; p. 638). Along with the anthropogenic geochemical enrichment processes previous detected across the Maya world, elevated Hg in soils and sediment from pre-Columbian cinnabar mining and use may be considered one of the “golden spikes” of an early Anthropocene (or “Mayacene”); an enduring and distinct region-wide geochemical marker of pre-Columbian landscape modification (Beach et al., 2015; Wagreich and Draganits, 2018; Penny and Beach, 2021; Cook et al., 2022).
The historical and environmental evidence now available, and synthesised in this review, supports the notion that Maya were exposed to Hg, due to its presence in cinnabar-based paints on ancient ceramic ware and crafts (Gazzola, 2000), from using cinnabar and elemental mercury in ritual and ceremonial activities (including burials) and, probably, its migration into food and water, and via direct human absorbance, as a result (Ávila et al., 2014; Lentz et al., 2020). A small but growing body of osteoarchaeological studies indicates that exposure to mercury may have resulted in substantial Hg accumulation in ancient Maya people, and (presumably) toxicity and negative health impacts. The Maya site of Palenque offers a key case study in ancient Maya mercury use and exposure, with elite burials of past kings and queens in tombs covered with cinnabar powder (Ruz Lhuiller, 1973; González Cruz, 2011). A molar of a female from Palenque, dating from the Terminal Classic, contained elevated total Hg in the tooth enamel, thought to be accumulated while living at Palenque, rather than from post mortem absorbance (Ochoa-Lugo et al., 2018). Analysis of bones from two different human burials at Palenque showed Hg enrichment in the bone material. One bone sample in this study (from the burial dated to the early seventh century CE) shows that the Hg was likely accumulated while alive, indicative of substantial mercury exposure at the site (Cervini-Silva et al., 2018). Ávila et al. (2014) investigated human bones from the pre-Columbian cinnabar region of Ranas (Mexico, Figure 4) and showed that the hydroxyapatite in bone contained Hg ions in the lattice. They interpreted this as evidence of mercuric poisoning due to high-level exposure to cinnabar in local mines or by poisoning through polluted dishes or toxic food. In their report on extremely high Hg levels in Pre-Contact Maya reservoir sediments at Tikal, Lentz et al. (2020) describe how one of the last rulers of Tikal, “Dark Sun”, may have suffered from metabolic syndrome related to chronic mercury poisoning from extensive use of cinnabar at Tikal.
Research gaps and recommendations for Maya mercury investigations
The work of geoscientists and archaeologists at Maya settlements in the last two decades has revealed the environmental legacy of region-wide anthropogenic Hg use that began before the first millennium CE. This challenges the idea that the pre-Columbian Maya existed in an environment free of heavy metals, and highlights the long record of mercury use in Central America (and its environmental legacy) with implications for regional and global histories of anthropogenic mercury. This review identifies five interconnected research gaps. First, we have no evidence yet that can explain where the Maya sourced mercury-based materials from. Most of the Maya world is located far distances from known geological sources of mercury, though it is feasible that cinnabar (and, perhaps, elemental mercury) was transported and traded to Maya sites along well-known trade routes used for other natural resources, such as jade and obsidian.
Second, we still know very little regarding how, why or when the Maya first acquired and used elemental mercury. There are no archaeological data yet recovered that suggest that the Classic Maya produced elemental mercury from smelting cinnabar, though this knowledge and technology arrived in Mexico following European contact in the 16th century.
Third, we have a growing body of pre-Columbian mercury pollution data from Maya sites, but very few research projects (only those from Tikal and Marco Gonzalez) have featured research designs optimised to develop well-dated and accurate records of past mercury use. Similarly, more than half of the Maya sites that have reported anthropogenic mercury data have used non-standard and partial extraction processes that may have underestimated the absolute magnitude of Hg inputs. We submit that future research on the environmental legacy of Maya mercury aim to use standardised and replicable methodologies and ensure that samples are obtained from sealed and well-dated ancient contexts and from regions of the Maya world where we do not yet have data from (such as the Maya regions of Mexico, much of Belize, and southern Guatemala). While exploring the legacy of mercury use at Maya settlements with clear evidence of past use of mercury, examining the mercury content of environmental materials at settlements with no archaeological record of mercury can help archaeologists map the extent and timing of mercury use as part of a broader understanding of the trade and cultural use of mercury during the last three millennia.
Fourth, Late Classic Maya mercury pollution data are overrepresented in our datasets, and we have very little information on mercury accumulation in the cities and villages of the Maya before the eighth century CE. This is a significant research gap given that the archaeological record shows that the Maya were using cinnabar and elemental mercury since the Middle Preclassic period. We recommend that future mercury studies in Maya archaeological contexts target Early Classic and Preclassic sites, or contexts within Maya sites with long site histories that extend back to the first millennia BCE. The final research direction we propose requires attention is on the implications for safe practice in archaeology given the evidence of widespread Hg enrichment at Maya sites and the possibility of exposure to mercury (in many forms) by workers. Maya archaeologists Freiwald (2019) and Sheets (1992) have shared similar concerns regarding the safety of archaeologists at Maya sites from possible exposure to mercury. While research on the degree of mercury toxicity should be prioritised for Maya contexts with obvious mercury contamination from cinnabar (such as burials and tombs, which also suffer from limited fresh air supply), we also recommend that screening of ancient Maya soils and occupation spaces be considered to determine mercury concentrations so that researchers may use appropriate risk mitigation strategies and protective equipment. Some forms of mercury are much more toxic to humans than others: organomercuric compounds (especially dimethylmercury) are extremely toxic to human health (Liu et al., 2008). We do not yet have information on the exact forms that mercury takes in soils, sediments, floors and other contexts at excavated ancient Maya sites. Even in cases where cinnabar appears to be the dominant form of mercury [in bulk quantities up to 10 kg, in one Maya tomb at Tikal, for example (Haviland, 2015)], we should not assume that transformations to other mercury forms have not occurred in the many centuries since Maya settlements were abandoned. Nriagu (1993, 1994), for example, cautioned that the tropical climate of Mexico provides conducive conditions for mercury, bound in historic abandoned mine waste and sediments, to be methylated and released into the atmosphere.
Conclusion
This review is the first to synthesise the growing body of geochemical investigations undertaken at ancient Maya sites in Mexico and Central America, with the aim of identify the timing, magnitude, and geography of Maya mercury contamination of the environment. The history of anthropogenic mercury in the Maya world is especially long, but not recognised in great detail. We know that cinnabar mining and use likely began sometime in the first millennia BCE and persisted for at least 15 centuries, quickly spreading to many major Maya settlements across a wide swathe of Middle America, in present-day Mexico, Guatemala, Belize, El Salvador and Honduras. The literature contains numerous accounts of cinnabar found during archaeological excavations at Maya settlements, often in burial contexts, ceremonial structures, but also in Maya households and domestic working areas, such as small-scale craft and jewellery production zones. Far less common have been finds of elemental mercury, preserved in ceramic vessels, at only nine Maya settlements during excavations. Elemental mercury was evidently rare in the Maya world, probably very difficult to come by, and was likely considered an extremely precious material with exceptional properties, used only in burials of important individuals or in other elite ritual contexts.
Geochemical investigations of buried soils (anthrosols), infilled ancient reservoirs and other environmental archives at (and near) abandoned Maya settlements confirms that the use of mercury by the Classic Period Maya resulted in substantial and persistent mercury pollution. While direct and absolute comparisons between individual Maya sites requires some caution (due to different analytical procedures and measurement outcomes), we can say that mercury concentrations in the Maya landscape were often very high. Seven of the Maya settlements investigated had buried soils with Hg concentrations that exceed modern guidelines for toxicity (TET; 1 μg g−1). We have evaluated the region-wide record of pre-Columbian Maya Hg pollution in the context of the archaeological record of cinnabar and elemental mercury use, including the possible sources of cinnabar and how mercury may have been obtained by the ancient Maya. The archaeological record of extensive cinnabar use at sites such as Tikal, for example, may be directly compared with Classic Maya period Hg concentrations measured at the same site of up to 17 μg g−1. Some Maya sites with elevated Hg have a clear archaeological record of mercury use by the Maya. In cases where the archaeology is silent on past mercury use, such as at Chunchucmil in the Yucatan region, we propose that the legacy mercury data from Maya soils and sediments be considered artefacts of past Maya mercury use. Finally, we consider the evidence of multi-millennial anthropogenic mercury accumulation in the environment of the Maya from the perspective of human health (past and present) and mercury’s potential for toxicity. We propose that Maya archaeological projects with evidence of extensive past mercury use evaluate what additional protective practices could be employed for researchers working in close contact with cinnabar, or other artefacts or environmental materials that may be rich in legacy mercury (Figure 6).
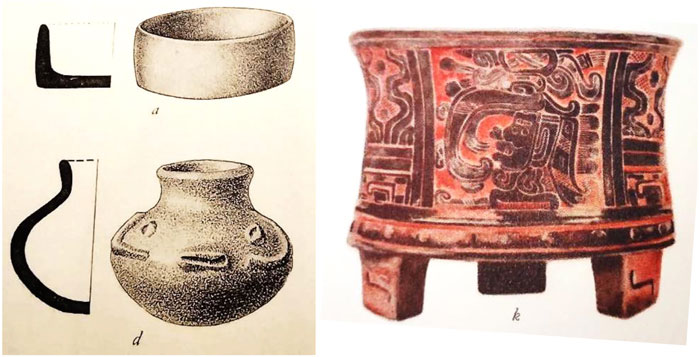
FIGURE 6. Early Classic mercury artefacts from the Maya site of Kaminaljuyu, southern Guatemala from Kidder et al. (1946). Two examples of small Maya paint pots found to have contained cinnabar (left), and a cinnabar-painted vessel (right). Elemental mercury was also reported at Kaminaljuyu (Table 2), but there is no imagery of this available. Credit: Carnegie Institution for Science: imagery reproduced with permission.
Author contributions
DC designed research, performed research, analyzed data, and wrote the paper. TB, SL-B, ND, and ST analyzed data and wrote the paper. All authors contributed to revising the manuscript and have read, and approved, the final submitted version.
Funding
DC would like to acknowledge funding support from the Australian Research Council (grant DP180101986) and from Australian Catholic University (RSP 21 Program). ND would like to acknowledge funding from the U.S. National Science Foundation (grant BCS-0810118: Lentz PI; Dunning and Scarborough co-PIs) that enabled the reservoir sampling for Hg at Tikal. ST acknowledges funding support from The Leverhulme Trust for the project “The role of past human activity in structuring modern landscapes and soils“ (2013-2016) (Elizabeth Graham, PI). TB and SL-B acknowledge funding from the U.S. National Science Foundation (9307435, 9910545, 0320256 (B. Dahlin PI); 0919330 (A. Magnoni PI, T. Beach co-PI); 0716015 (B. Dahlin, PI., T. Beach, Co-PI)), a National Geographic Society CRE grant (PI, B.L. Dahlin, Co-I’s T. Beach and S. Luzzadder-Beach), the American Association of Geographers Anne U. White Fund (S. Luzzadder- Beach, PI., Co-PI, T. Beach), the Cinco Hermanos Chair, Georgetown University (PI, T. Beach), The Planet Texas 2050 Initiative, the CB Smith Centennial Chair in US-Mexico Relations, and the RC Dickson Centennial Professorship in Liberal Arts (PIs T. Beach and S. Luzzadder-Beach, UT Austin).
Acknowledgments
We acknowledge the research of Elizabeth Graham and David Pendergast on mercury in the Maya world that has been greatly influential on our own. We also thank them for their discussions on Maya mercury over many years, and their feedback on an earlier version of this article. We thank Anaïs Zimmer and Kat Davies for their assistance in preparing figures in this paper. ND would like to thank T.P. Luxton of the U.S. Environmental Protection Agency for providing laboratory measurements of Hg. TB and SL-B acknowledge the work of R.L. Terry measuring Hg in sediment samples at the Brigham Young University Environmental Analytical Lab. ST thanks Handong Yang of UCL Geography for processing Hg samples. Finally, we wish to thank the five reviewers for their comments on an earlier draft of this paper.
Conflict of interest
The authors declare that the research was conducted in the absence of any commercial or financial relationships that could be construed as a potential conflict of interest.
Publisher’s note
All claims expressed in this article are solely those of the authors and do not necessarily represent those of their affiliated organizations, or those of the publisher, the editors and the reviewers. Any product that may be evaluated in this article, or claim that may be made by its manufacturer, is not guaranteed or endorsed by the publisher.
Footnotes
1In this review “mercury” is used to refer to mercury present in the environment, regardless of its chemical form. We use the symbol for mercury, Hg, when referring to measurements of the concentration of mercury in soils and sediments. Where specific forms of mercury are discussed, these are referred to explicitly by their common names. For example, the liquid form of mercury (Hg0) is referred to as elemental mercury, or simply by its symbol, Hg0. Cinnabar and its chemical formula, HgS, are used interchangeably. We use “cinnabar” when referring to cinnabar ore and powdered cinnabar.
2ICP-AES: Inductively coupled plasma atomic emission spectroscopy; ICP-MS: Inductively coupled plasma mass spectrometry; CVAFS: Cold vapour atomic fluorescence spectroscopy; AAS: Atomic absorption spectroscopy.
References
Aimers, J. J., McCarron, K., Simmons, S., and Graham, E. (2017). The pottery of Marco gonzalez, Belize. Res. Rep. Belizean Archaeol. 14, 363–372.
Aliphat Fernández, M. M. (1996). Arqueología y paisajes del Alto Usumacinta. Arqueol. Mex. 4 (22), 24–29.
Andrieu, C., Rodas, E., and Luin, L. (2014). The values of classic Maya jade: A reanalysis of Cancuén's jade workshop. Anc. Mesoam. 25, 141–164. doi:10.1017/s0956536114000108
Anon (1996). Mercurio en México. Coordinación general de Minas, secretaría de Comercio y fomento industrial (SECOFI). México.
Argote, D. L., Torres, G., Hernández-Padrón, G., Ortega, V., López-García, P. A., and Castaño, V. M. (2020). Cinnabar, hematite and gypsum presence in mural paintings in Teotihuacan, Mexico. J. Archaeol. Sci. Rep. 32, 102375. doi:10.1016/j.jasrep.2020.102375
Ashmore, W. E., Schortman, M., and Sharer, R. J. (1983). “The quirigua project: The 1979 season,” in Quirigua reports ll. Editors E. M. Schortman, and P. A. Urban (Philadelphia: University Museum, University of Pennsylvania), 55–78.
Ashmore, W. (1984). Quirigua archaeology and history revisited. J. Field Archaeol. 11 (4), 365–386. doi:10.2307/529316
Audet, C. M. (2006). Political Organization in the Belize River valley: Excavations at Baking Pot, Cahal Pech, and Xunantunich. Doctoral dissertation. Nashville, TN: Vanderbilt University.
Ávila, A., Mansilla, J., Bosch, P., and Pijoan, C. (2014). Cinnabar in Mesoamerica: Poisoning or mortuary ritual? J. Archaeol. Sci. 49, 48–56. doi:10.1016/j.jas.2014.04.024
Barba, L., and Herrera, H. (1988). San Jose Ixtapa: Un sitio dedicado a la produccion de mercurio. An. Antropol. 23, 87–104.
Battistel, D., Roman, M., Marchetti, A., Kehrwald, N. M., Radaelli, M., Balliana, E., et al. (2018). Anthropogenic impact in the Maya lowlands of Petén, Guatemala, during the last 5500 years. J. Quat. Sci. 33 (2), 166–176. doi:10.1002/jqs.3013
Bautista, F., Palacio Aponte, G., Quintana, P., and Zinck, J. A. (2011). Spatial distribution and development of soils in tropical karst areas from the peninsula of Yucatán, Mexico. Geomorphology 135, 308–321. doi:10.1016/j.geomorph.2011.02.014
Beach, T., and Dunning, N. P. (1995). Ancient Maya terracing and modern conservation in the Petén rain forest of Guatemala. J. Soil Water Conservation 50 (2), 138–145.
Beach, T., Dunning, N., Luzzadder-Beach, S., Cook, D. E., and Lohse, J. (2006). Impacts of the ancient Maya on soils and soil erosion in the central Maya Lowlands. Catena 65 (2), 166–178. doi:10.1016/j.catena.2005.11.007
Beach, T., Luzzadder-Beach, S., Cook, D., Dunning, N., Kennett, D., Krause, S., et al. (2015). Ancient Maya impacts on the earth’s surface: An early anthropocene analog? Quat. Sci. Rev. 124, 1–30. doi:10.1016/j.quascirev.2015.05.028
Bell, E. E. (2007). Early classic ritual deposits within the copan Acropolis: The material foundations of political power at a classic period Maya center. Doctoral thesis. University of Pennsylvania.
Bishop, R. L. (2014). Instrumental approaches to understanding mesoamerican economy: Elusive promises. Anc. Mesoam. 25 (01), 251–269. doi:10.1017/s0956536114000157
Borhegyi, S. F. (1959). “Underwater archaeology in guatemala,” in Actas del XXXIII Congreso Internacional de Americanistas, San José, Costa Rica, July 20–27, 1958 2, 229–240.
Brooks, W. E. (2012). “Industrial use of mercury in the ancient world,” in Mercury in the environment: Pattern and process. Editor M. A. Bank (Los Angeles, CA: University of California Press).
Cabadas-Báez, H., Solleiro-Rebolledo, E., Sedov, S., Pi-Puig, T., and Gama-Castro, J. (2010). Pedosediments of karstic sinkholes in the eolianites of ne yucatán: A record of late quaternary soil development, geomorphic processes and landscape stability. Geomorphology 122 (3-4), 323–337. doi:10.1016/j.geomorph.2010.03.002
Canuto, M. A., and Barrientos, T. (2011). La Corona: Un acercamiento a las políticas del reino kaan desde un centro secundario del noroeste del Petén. Estud. Cult. Maya 37, 11–43. doi:10.19130/iifl.ecm.2011.37.12
Cárdenas Vargas, J. (1992). Monografía Geológico-Minera del Estado de Guanajuato. Mexico City, Mexico: Consejo de Recursos Minerales.
Cervini-Silva, J., de Lourdes Muñoz, M., Palacios, E., Jimenez-Lopez, J. C., and Romano-Pacheco, A. (2018). Ageing and preservation of HgS-enriched ancient human remains deposited in confinement. J. Archaeol. Sci. Rep. 18, 562–567. doi:10.1016/j.jasrep.2018.02.010
Chase, A. F., and Chase, D. Z. (1995). “External impetus, internal synthesis, and standardization: E Group assemblages and the crystallization of classic Maya society in the southern lowlands,” in The emergence of lowland Maya civilization. Editor N. Grube (Möckmühl, Germany: A. Saurwein), 87–101.
Chase, D. Z., and Chase, A. F. (2017). Caracol, Belize, and changing perceptions of ancient Maya society. J. Archaeol. Res. 25 (3), 185–249. doi:10.1007/s10814-016-9101-z
Cheek, C. D. (1983). “Excavaciones en la Plaza Principal,” in Introduccion a la Arqueologia de Copan, Honduras, tomo II. Tegucigalpa, Honduras. Editor C. F. Baudez (Tegucigalpa: Secretaria de Estado en el Despacho de Cultura y Turismo), 191–289.
Cheek, C. D., and Milla Villeda, D. E. (1983). “Estructura 10l–4,” in Introduccion a la Arqueologia de Copan, Honduras, tomo II. Editor C. F. Baudez (Tegucigalpa, Honduras: Secretaria de Estado en el Despacho de Cultura y Turismo), 37–91.
Cook, D. E., Kovacevich, B., Beach, T., and Bishop, R. (2006). Deciphering the inorganic chemical record of ancient human activity using ICP‐MS: A reconnaissance study of late classic soil floors at Cancuén, Guatemala. J. Archaeol. Sci. 33 (5), 628–640. doi:10.1016/j.jas.2005.09.019
Cook, D. E., Beach, T., and Demarest, A. A. (2017). Soil and slaughter: A geoarchaeological record of the ancient Maya from Cancuén, Guatemala. J. Archaeol. Sci. Rep. 15, 330–343. doi:10.1016/j.jasrep.2017.08.005
Cook, D. E., Beach, T. P., Luzzadder-Beach, S., Dunning, N. P., and Smith, B. A. (2022). “Long-term geomorphic change in the Maya lowlands of central America,” in Treatise on geomorphology. Editor J. F. Shroder. Second Edition (Academic Press), 504–546. doi:10.1016/B978-0-12-818234-5.00178-4
Cooke, C. A., Balcom, P. H., Biester, H., and Wolfe, A. P. (2009). Over three millennia of mercury pollution in the Peruvian Andes. Proc. Natl. Acad. Sci. U. S. A. 106, 8830–8834. doi:10.1073/pnas.0900517106
Cooke, C. A., Hintelmann, H., Ague, J. J., Burger, R., Biester, H., Sachs, J. P., et al. (2013). Use and legacy of mercury in the Andes. Environ. Sci. Technol. 47 (9), 4181–4188. doi:10.1021/es3048027
Cooke, C. A., Curtis, J. H., Kenney, W. F., Drevnick, P., and Siegel, P. E. (2022). Caribbean lead and mercury pollution archived in a crater lake. Environ. Sci. Technol. 56 (3), 1736–1742. doi:10.1021/acs.est.1c06791
Demarest, A. (2004). Ancient Maya: The rise and fall of a rainforest civilization. Cambridge, NY: Cambridge University Press.
Deuel, L. (1974). Conquistadors without swords: Archaeologists in the Americas; an account with original narratives. New York, NY: Schocken Books.
Drucker, P., Heizer, R. F., and Squier, R. J. (1959). “Excavations at La venta, tabasco, 1955,”. Bureau of American ethnology bulletin (Washington DC: Smithsonian Institute), 170, 1–312.
Dunning, N., Beach, T., and Luzzadder-Beach, S. (2012). Kax and kol: Collapse and resilience in lowland Maya civilization. Proc. Natl. Acad. Sci. U. S. A. 106, 3652–3657. doi:10.1073/pnas.1114838109
Dunning, N., Lentz, D., and Scarborough, V. (2015a). “Tikal – land, forest, and water: An introduction,” in Tikal: Paleoecology of an ancient Maya city. Editors D. Lentz, N. Dunning, and V. Scarborough (Cambridge, NY: Cambridge University Press), 1–15.
Dunning, N., Griffin, R., Jones, J., Terry, R., Larsen, Z., and Carr, C. (2015b). “Life on the edge: Tikal in a bajo landscape,” in Tikal: Paleoecology of an ancient Maya city. Editors D. Lentz, N. Dunning, and V. Scarborough (Cambridge, NY: Cambridge University Press), 95–123.
Dunning, N. P., Brewer, J., Carr, C., Anaya Hernández, A., Beach, T., Chmilar, J., et al. (2022). “Harvesting ha: Ancient water collection and storage in the elevated interior region of the Maya lowlands,” in Sustainability and water management in the Maya world and beyond. Editors J. Larmon, L. Lucero, and F. Valdez (Boulder, CO: University Press of Colorado), 13–52.
Farrar, W. V., and Williams, A. R. (1977). “A history of mercury,” in The chemistry of mercury. Editor C. A. McAuliffe (London, UK: Palgrave Macmillan), 1–45.
Foshag, W. F. (1957). Mineralogical studies on Guatemalan jade. Smithson. Misc. Collect. 135 (5), 1–60.
Freiwald, C. (2019). Excavation and curation strategies for complex burials in tropical environments. Adv. Archaeol. Pract. 7 (1), 10–22. doi:10.1017/aap.2018.43
Fulton, K. A. (2019). Community identity and shared practice at Actuncan, Belize. Lat. Am. Antiq. 30 (2), 266–286. doi:10.1017/laq.2019.18
Fulton, K., Wells, E. C., and Storer, D. (2017). Ritual or residential? An integrated approach to geochemical prospection for understanding the use of plaza spaces at Palmarejo, Honduras. Archaeol. Anthropol. Sci. 9, 1059–1076. doi:10.1007/s12520-013-0170-3
Fuson, R. H. (1969). The orientation of Mayan ceremonial centers. Ann. Assoc. Am. Geogr. 59 (3), 494–512. doi:10.1111/j.1467-8306.1969.tb00687.x
Gallagher, D., and Siliceo, R. P. (1948). Geology of the huahuaxtla mercury district, State of Guerrero, Mexico (report No. 960). Washington, DC: US Government Printing Office.
Gann, T. (1925). Maya Jades. XXIe Congrès International des Américanistes, 1924. Sweden: Göteburg Museum, 274–282.
Gazzola, J. (2000). Les utilisations du cinabre à Teotihuacan. Doctoral thesis. Paris, France: University of Paris 1 Panthéon-Sorbonne.
Gliozzo, E. (2021). Pigments—mercury-based red (cinnabar-vermilion) and white (calomel) and their degradation products. Archaeol. Anthropol. Sci. 13 (11), 210–253. doi:10.1007/s12520-021-01402-4
Gorokhovich, Y., Block, K. A., McNeil, C. L., Barrios, E., and Marionkova, M. (2020). Mercury source in Copan (Honduras): Local mining or trade? J. Archaeol. Sci. Rep. 33, 102471. doi:10.1016/j.jasrep.2020.102471
Graham, E., and Howie, L. (2021). “Mobility as resilience: A perspective on coastal to inland migration in the eastern Maya lowlands,” in Mobility and migration in ancient mesoamerican cities. Editors M. C. Arnauld, C. Beekman, and G. Pereira (Louisville, CO: University Press of Colorado), 109–130.
Graham, E., Macphail, R., Turner, S., Crowther, J., Stegemann, J., Arroyo-Kalin, M., et al. (2017). The Marco Gonzalez Maya site, Ambergris Caye, Belize: Assessing the impact of human activities by examining diachronic processes at the local scale. Quat. Int. 437, 115–142. doi:10.1016/j.quaint.2015.08.079
Graham, E., and Pendergast, D. M. (1989). Excavations at the Marco gonzalez site, Ambergris cay, Belize, 1986. J. Field Archaeol. 16 (1), 1–16. doi:10.2307/529877
Greenwood, N. N., and Earnshaw, A. (2012). Chemistry of the elements. 2nd Edition. Oxford, UK: Elsevier.
Guderjan, T. H. (1995). Maya settlement and trade on Ambergris Caye, Belize. Anc. Mesoam. 6 (1), 147–159. doi:10.1017/S0956536100002157
Haviland, W. A. (2015). Excavations in residential areas of Tikal-group 7F-1: Tikal report 22. Philadelphia, PA: University of Pennsylvania Press.
Higueras, P., Oyarzun, R., Biester, H., Lillo, J., and Lorenzo, S. (2003). A first insight into mercury distribution and speciation in soils from the Almadén mining district, Spain. J. Geochem. Explor. 80 (1), 95–104. doi:10.1016/s0375-6742(03)00185-7
Hillesheim, M. B., Hodell, D. A., Leyden, B. W., Brenner, M., Curtis, J. H., Anselmetti, F. S., et al. (2005). Climate change in lowland Central America during the late deglacial and early Holocene. J. Quat. Sci. 20 (4), 363–376. doi:10.1002/jqs.924
Houston, S., Brittenham, C., Mesick, C., Tokovinine, A., and Warinner, C. G. (2009). Veiled brightness: A history of ancient Maya color. Austin, TX: University of Texas Press.
Hutson, S. R., Stanton, T. W., Magnoni, A., Terry, R., and Craner, J. (2007). Beyond the buildings: Formation processes of ancient Maya houselots and methods for the study of non-architectural space. J. Anthropol. Archaeol. 26 (3), 442–473. doi:10.1016/j.jaa.2006.12.001
Hutson, S. R., and Terry, R. E. (2006). Recovering social and cultural dynamics from plaster floors: Chemical analyses at ancient Chunchucmil, Yucatan, Mexico. J. Archaeol. Sci. 33 (3), 391–404. doi:10.1016/j.jas.2005.08.004
Izzo, F. C., Rigon, C., Vázquez De Ágredos Pascual, M. L., Campíns-Falcó, P., and van Keulen, H. (2022). Life after death: A physicochemical study of materials used by the ancient Maya in human bone ointments. Archaeol. Anthropol. Sci. 14 (1), 7–17. doi:10.1007/s12520-021-01473-3
Jones, C., and Sharer, R. J. (1986). Archaeological investigations in the site core of Quirigua. Guatemala. Austin, TX: University of Texas Press.
Kidder, A. V. (1945). Excavations at Kaminaljuyu, Guatemala. Am. Antiq. 11 (2), 65–75. doi:10.2307/275651
Kidder, A. V., Jennings, J. D., and Shook, E. M. (1946). Excavations at Kaminaljuyu, Guatemala, 561. University Park, PA: Pennsylvania State University Press.
Kovacevich, B., Cook, D., and Beach, T. (2004). “Áreas de actividad doméstica en Cancuen: Perspectivas basadas en datos líticos y geoquímicos,” in XVII Simposio de Investigaciones Arqueológicas en Guatemala (Guatemala City: Museo Nacional de Arqueología y Etnología), 897–912.
Kutterolf, S., Schindlbeck, J. C., Anselmetti, F. S., Ariztegui, D., Brenner, M., Curtis, J., et al. (2016). A 400-ka tephrochronological framework for central America from Lake Petén Itzá (Guatemala) sediments. Quat. Sci. Rev. 150, 200–220. doi:10.1016/j.quascirev.2016.08.023
Lacerda, L. (1997). Global mercury emissions from gold and silver mining. Water Air Soil Pollut. 97 (3), 209–221. doi:10.1007/bf02407459
Lamoureux‐St‐Hilaire, M., Canuto, M. A., Wells, E. C., Cagnato, C., and Barrientos, T. (2019). Ancillary economic activities in a classic Maya regal palace: A multiproxy approach. Geoarchaeology 34 (6), 768–782. doi:10.1002/gea.21750
Langenscheidt, A. (1986). El cinabrio y el azogue en el Mexico antiguo [Cinnabar and mercury in ancient Mexico]. Rev. Minero-Americana 17, 24–29.
LeCount, L. J., Christian Wells, E., Jamison, T., and Mixter, D. (2016). Geochemical characterization of inorganic residues on plaster floors from a Maya palace complex at Actuncan, Belize. J. Archaeol. Sci. Rep. 5, 453–464. doi:10.1016/j.jasrep.2015.12.022
Lentz, D. L., Dunning, N. P., Scarborough, V. L., Magee, K. S., Thompson, K. M., Weaver, E., et al. (2014). Forests, fields, and the edge of sustainability at the ancient Maya city of Tikal. Proc. Natl. Acad. Sci. U. S. A. 111 (52), 18513–18518. doi:10.1073/pnas.1408631111
D. L. Lentz, N. P. Dunning, and V. L. Scarborough (Editors) (2015). Tikal: Paleoecology of an ancient Maya city (Cambridge University Press).
Lentz, D. L., Graham, E., Vinaja, X., Slotten, V., and Jain, R. (2016). Agroforestry and ritual at the ancient Maya center of Lamanai. J. Archaeol. Sci. Rep. 8, 284–294. doi:10.1016/j.jasrep.2016.06.030
Lentz, D. L., Hamilton, T. L., Dunning, N. P., Scarborough, V. L., Luxton, T. P., Vonderheide, A., et al. (2020). Molecular genetic and geochemical assays reveal severe contamination of drinking water reservoirs at the ancient Maya city of Tikal. Sci. Rep. 10 (1), 10316–10319. doi:10.1038/s41598-020-67044-z
Lindsay, W. L., and Norvel, W. A. (1978). Development of a DTPA test for zinc, iron, manganese and copper Soil Sci. Soc. Am. J. 42, 421–428. doi:10.3181/0712-mr-336
Liu, J., Shi, J. Z., Yu, L. M., Goyer, R. A., and Waalkes, M. P. (2008). Mercury in traditional medicines: Is cinnabar toxicologically similar to common mercurials? Exp. Biol. Med. (Maywood). 233 (7), 810–817. doi:10.3181/0712-mr-336
Looper, M. G. (2003). Lightening warrior: Maya art and kingship at Quirigua. Austin, TX: University of Texas Press.
Lowe, G. W., and Agrinier, P. (1960). “Mound 1, Chiapa de Corzo, Chiapas, Mexico,” in Papers of the new world archaeological foundation (Provo, Utah. Publication 7, No. 8.
Luzzadder‐Beach, S., Beach, T., Terry, R. E., and Doctor, K. Z. (2011). Elemental prospecting and geoarchaeology in Turkey and Mexico. Catena 85 (2), 119–129. doi:10.1016/j.catena.2010.09.001
MacDonald, D. D., Ingersoll, C. G., and Berger, T. A. (2003). Development and evaluation of consensus-based sediment quality guidelines for freshwater ecosystems. Arch. Env. Cont. & Tox. 39, 20–31.
Macphail, R. I., Graham, E., Crowther, J., and Turner, S. (2017). Marco gonzalez, Ambergris Caye, Belize: A geoarchaeological record of ground raising associated with surface soil formation and the presence of a dark earth. J. Archaeol. Sci. 77, 35–51. doi:10.1016/j.jas.2016.06.003
Manzanilla, L. R. (2012). “Teopancazco, a multi-ethnic neighborhood center of Teotihuacán,” in Archaeometric studies of the neighborhood center of Teopancazco in Teotihuacán (Mexico: UNAM), 17–66.
Martin, S., and Grube, N. (2008). Chronicles of the Maya kings and queens. London and New York: Thames and Hudson.
Mata Amado, G. (2002). “Exploraciónes subacuaticas en los Lagos de Guatemala,” in XV Simposio de Investigaciónes Arqueológicas en Guatemala. Editor J. La Porte (Teguchigalpa: Museo Nacional de Arqueologia y Ethologia), 45.
Mata Amado, G. (2011). in Arqueología subacuática: Amatitlán, Atitlán. Editors G. Mata Amado, and S. Medrano (Guatemala City, Guatemala: Museo Popol Vuh).
Maudslay, A. P. (1889-1902). Archaeology: Biologia Centrali Americana, or, Contributions to the Knowledge of the Fauna and Flora of Mexico and Central America, Vol. 5. London, UK: R. H. Porter and Dulau Co.
Molleson, T., Comerford, G., and Moore, A. (1992). A neolithic painted skull from Tell Abu Hureyra, Northern Syria. Cam. Arch. Jnl. 2 (2), 231–236. doi:10.1017/S0959774300000603
Nalda, E. (2010). “El clásico en el México antiguo,” in Historia general de México ilustrada: Volumen I (1st, edición conmemorativa ed. Editor E. V. Garcíaet al. (Mexico City, Mexico: El Colegio de Mexico), 72–131. doi:10.2307/j.ctv47w8kt
Nriagu, J. O. (1979). “Production and uses of mercury,” in Biogeochemistry of mercury in the environment. Editor J. O. Nriagu (Amsterdam: Elsevier), 23–39.
Nriagu, J. O. (1994). Mercury pollution from the past mining of gold and silver in the Americas. Sci. Total Environ. 149 (3), 167–181. doi:10.1016/0048-9697(94)90177-5
Ochoa‐Lugo, M., Cervini‐Silva, J., Muñoz, M. D. L., Palacios, E., Pérez‐Ramirez, G., Ramos‐Cruz, E., et al. (2018). Excellent preservation of archaeological, mercury‐enriched dental remains. Archaeometry 60 (6), 1346–1360. doi:10.1111/arcm.12378
Outridge, P. M., Mason, R. P., Wang, F., Guerrero, S., and Heimburger-Boavida, L. E. (2018). Updated global and oceanic mercury budgets for the united nations global mercury assessment 2018. Environ. Sci. Technol. 52 (20), 11466–11477. doi:10.1021/acs.est.8b01246
Parnell, J. J., Terry, R. E., and Nelson, Z. (2002a). Soil chemical analysis applied as an interpretive tool for ancient human activities in Piedras Negras, Guatemala. J. Archaeol. Sci. 29 (4), 379–404. doi:10.1006/jasc.2002.0735
Parnell, J. J., Terry, R. E., and Sheets, P. (2002b). Soil chemical analysis of ancient activities in Cerén, El Salvador: A case study of a rapidly abandoned site. Lat. Am. Antiq. 13 (3), 331–342. doi:10.2307/972114
Pendergast, D. M. (1980). As easy as picking up quicksilver with a fork. R. Ont. Mus. Archaeol. Newsl. Ser. 1, 180.
Pendergast, D. M. (1982). Ancient Maya mercury. Science 217 (6), 533–535. doi:10.1126/science.217.4559.533
Penny, D., and Beach, T. P. (2021). Historical socioecological transformations in the global tropics as an Anthropocene analogue. Proc. Natl. Acad. Sci. U. S. A. 118 (40), e2022211118. doi:10.1073/pnas.2022211118
Pérez Campos, E. M., and Herrera Muñoz, A. J. (2013). Minas y mineros: Presencia de metales en sedimentos y restos humanos al sur de la Sierra gorda de Querétaro en méxico. Chungará (Arica) 45 (1), 161–176. doi:10.4067/s0717-73562013000100008
Reimann, C., and Caritat, P. (1998). Chemical elements in the environment: FactSheets for the geochemist and the environmental scientist. Berlin: Springer-Verlag.
Rice, P. M. (2019). Early pottery and construction at nixtun-ch'ich’, Petén, Guatemala: Preliminary observations. Lat. Am. Antiq. 30 (3), 471–489. doi:10.1017/laq.2019.26
P. M. Rice, and P. M. Rice (Editors) (2018). Historical and archaeological perspectives on the itzas of Petén, Guatemala (Boulder, CO: University Press of Colorado).
Roberts, R. J., and Irving, E. M. (1957). Mineral deposits of Central America, 1034. Washington, DC: US Government Printing Office.
Rudnick, R. L., and Gao, S. (2003). “Composition of the continental crust,” in Treatise on geochemistry, vol. 3, the crust. Editors H. D. Holland, and K. K. Turekian (Oxford, UK: Elsevier-Pergamon), 1–64.
Scarborough, V., Dunning, N., Tankersley, K., Carr, C., Weaver, E., Grazioso, L., et al. (2012). Water and sustainable land use at the ancient tropical city of tikal, Guatemala. Proc. Natl. Acad. Sci. U. S. A. 109, 12408–12413. doi:10.1073/pnas.1202881109
Schneider, L., Rose, N. L., Myllyvirta, L., Haberle, S., Lintern, A., Yuan, J., et al. (2021). Mercury atmospheric emission, deposition and isotopic fingerprinting from major coal-fired power plants in Australia: Insights from palaeo-environmental analysis from sediment cores. Environ. Pollut. 287, 117596. doi:10.1016/j.envpol.2021.117596
Schotsmans, E. M. J., Busacca, G., Lin, S. C., Vasic, M., Lingle, A. M., Veropoulidou, R., et al. (2022). New insights on commemoration of the dead through mortuary and architectural use of pigments at Neolithic Çatalhöyük, Turkey. Sci. Rep. 12, 4055. doi:10.1038/s41598-022-07284-3
Sharer, R. J. (2003). “Founding events and teotihuacan connections at copán, Honduras,” in The Maya and teotihuacan. Editor G. E. Braswell (Austin, TX: University of Texas Press), 143–165.
Sharer, R. J., and Traxler, L. P. (2006). The ancient Maya. 6th ed. Stanford, CA: Stanford University Press.
Sheets, P. D. (1979). Maya recovery from volcanic disaster Ilopango and Cerén. Archeology 32 (3), 32–42.
Sheets, P. D. (1992). The Cerén site: A prehistoric village buried by volcanic ash in Central America. Orlando, FL: Harcourt College Publications.
Sheets, P. D. (2010). Before the volcano erupted: The ancient Cerén village in Central America. Austin, TX: University of Texas Press.
Sills, E. C., McKillop, H., and Wells, E. C. (2016). Chemical signatures of ancient activities at Chan b'i-A submerged Maya salt works, Belize. J. Archaeol. Sci. Rep. 9, 654–662. doi:10.1016/j.jasrep.2016.08.024
Smith, R. S., Wiederhold, J. G., and Kretzschmar, R. (2015). Mercury isotope fractionation during precipitation of metacinnabar (β-HgS) and montroydite (HgO). Environ. Sci. Technol. 49 (7), 4325–4334. doi:10.1021/acs.est.5b00409
Stephens, L., Fuller, D., Boivin, N., Rick, T., Gauthier, N., Kay, A., et al. (2019). Archaeological assessment reveals Earth’s early transformation through land use. Science 365 (6456), 897–902. doi:10.1126/science.aax1192
Tankersley, K. B., Scarborough, V. L., Dunning, N., Huff, W., Maynard, B., and Gerke, T. L. (2011). Evidence for volcanic ash fall in the Maya lowlands from a reservoir at Tikal, Guatemala. J. Archaeol. Sci. 38 (11), 2925–2938. doi:10.1016/j.jas.2011.05.025
Tankersley, K., Dunning, N., Scarborough, V., Carr, C., and Lentz, D. (2015). “Fire and water: The significance of tikal’s quaternary sediments,” in Tikal: Paleoecology of an ancient Maya city. Editors D. N. Lentz, N. Dunning, and V. Scarborough (Cambridge: Cambridge University Press), 186–211.
Tankersley, K. B., Dunning, N. P., Scarborough, V., Huff, W. D., Lentz, D. L., and Carr, C. (2016). Catastrophic volcanism and its implication for agriculture in the Maya lowlands. J. Archaeol. Sci. Rep. 5, 465–470. doi:10.1016/j.jasrep.2015.12.019
Tate, C. E. (2012). Reconsidering Olmec visual culture: The unborn, women, and creation. Austin, TX: University of Texas Press.
Thompson, E. H. (1897). The chultunes of labná, yucatan: Report of explorations by the Museum, 1888-89 and 1890-91. in Memoirs of the Peabody Museum of Archaeology and Ethnology, 1. Cambridge, MA: Harvard University.
Thompson, L. M. (2005). A comparative analysis of burial patterning: The preclassic Maya sites of Chiapa de Corzo, Kaminaljuyu, tikal, and Colha. Austin, TX: University of Texas Press.
Turner, S., Graham, E., Macphail, R., Duncan, L., Rose, N. L., Yang, H., et al. (2021). Mercury enrichment in anthrosols and adjacent coastal sediments at a Classic Maya site, Marco Gonzalez, Belize. Geoarchaeology 36 (6), 875–896. doi:10.1002/gea.21868
Wagreich, M., and Draganits, E. (2018). Early mining and smelting lead anomalies in geological archives as potential stratigraphic markers for the base of an early Anthropocene. Anthropocene Rev. 5 (2), 177–201. doi:10.1177/2053019618756682
Wang, X. S., Qin, Y., and Chen, Y. K. (2006). Heavy meals in urban roadside soils, part 1: Effect of particle size fractions on heavy metals partitioning. Environ. Geol. 50 (7), 1061–1066. doi:10.1007/s00254-006-0278-1
Wells, E. C. (2004). Investigating activity patterns in prehispanic plazas: Weak acid-extraction ICP-AES analysis of anthrosols at classic period el coyote, northwestern Honduras. Archaeometry 46, 67–84. doi:10.1111/j.1475-4754.2004.00144.x
Wells, E. C., Terry, R. E., Parnell, J. J., Hardin, P. J., Jackson, M. W., and Houston, S. D. (2000). Chemical analyses of ancient anthrosols in residential areas at Piedras Negras, Guatemala. J. Archaeol. Sci. 27 (5), 449–462. doi:10.1006/jasc.1999.0490
Wells, E. C., Novotny, C., and Hawken, J. R. (2007). “Quantitative modeling of soil chemical data from inductively coupled plasma–optical emission spectroscopy reveals evidence for cooking and eating in ancient Mesoamerican plazas,” in Archaeological chemistry: Analytical techniques and archaeological interpretation. Editors M. D. Glascock, R. J. Speakman, and R. S. Popelka-Filcoff (Washington, DC: American Chemical Society), 210–230.
Welsh, W. B. M. (1988). An analysis of classic lowland Maya burials. International series 409. Oxford, UK: British Archaeological Reports.
Williamson, R. (1996). “Excavations, interpretations, and implications of the earliest structures beneath structure 10l-26 at Copan, Honduras. Excavations, interpretations, and implications of the earliest structures beneath structure 10L26 at Copan, Honduras,” in Eighth Palenque round table, 1993. Editors M. J. Macri, and J. McHargue (San Francisco: The Pre-Columbian Art Research Institute), 169–175.
Keywords: mercury, pre-Columbian Maya, cinnabar, soil pollution, Mesoamerica, anthropogenic heavy metals
Citation: Cook DE, Beach TP, Luzzadder-Beach S, Dunning NP and Turner SD (2022) Environmental legacy of pre-Columbian Maya mercury. Front. Environ. Sci. 10:986119. doi: 10.3389/fenvs.2022.986119
Received: 04 July 2022; Accepted: 23 August 2022;
Published: 23 September 2022.
Edited by:
Larissa Schneider, Australian National University, AustraliaReviewed by:
Jean Remy Davee Guimaraes, Federal University of Rio de Janeiro, BrazilAnne Helene Fostier, State University of Campinas, Brazil
Saul Guerrero, Australian National University, Australia
Luiz Drude Lacerda, Federal University of Ceara, Brazil
Peter Davies, La Trobe University, Australia
Copyright © 2022 Cook, Beach, Luzzadder-Beach, Dunning and Turner. This is an open-access article distributed under the terms of the Creative Commons Attribution License (CC BY). The use, distribution or reproduction in other forums is permitted, provided the original author(s) and the copyright owner(s) are credited and that the original publication in this journal is cited, in accordance with accepted academic practice. No use, distribution or reproduction is permitted which does not comply with these terms.
*Correspondence: Duncan E. Cook, ZHVuY2FuLmNvb2tAYWN1LmVkdS5hdQ==