Plant diversity patterns along an elevation gradient: the relative impact of environmental and spatial variation on plant diversity and assembly in arid and semi-arid regions
- 1Key Laboratory of Eco-Environments in the Three Gorges Reservoir Region (Ministry of Education), State Cultivation Base of Eco-Agriculture for Southwest Mountainous Land, College of Life Sciences, Southwest University, Chongqing, China
- 2Biological Science Research Center, Academy for Advanced Interdisciplinary Studies, Southwest University, Chongqing, China
- 3Breeding Base for State Key Laboratory of Land Degradation and Ecological Restoration in Northwest China, School of Ecology and Environment, Ningxia University, Yinchuan, China
- 4State Key Laboratory of Seedling Bioengineering, Ningxia Forestry Institute, Yinchuan, China
- 5Ningxia Helan Mountain Forest Ecosystem Research Station, National Nature Reserve Administration Bureau of Ningxia Helan Mountain, Yinchuan, China
The spatial distribution pattern of species diversity and its driving variables are essential to understanding how biodiversity will respond to the threat of future climatic instability. This study examines how the alpha and beta diversity indices vary along the elevation gradient and which factors are more responsible for arid and semi-arid plant diversity and community assembly. There were 121 species from 90 genera and 42 families found at elevations ranging from 1,200 to 2,600 m within the Ningxia Helan Mountain National Nature Reserve in China. These were primarily shrubs and herbs with characteristics adapted to extreme temperatures. The diversity of both the shrub layer and the herb layer was highest at mid-elevation, underscoring the importance of protecting mid-elevation ecosystems. Climatic distance and topographic distance were more influential than spatial distance in driving the species composition change of shrubs and herbs. In the herb layer, climatic, topographic, and spatial factors contributed more to community assembly than shrub communities. In this case, improving the environment in the community or introducing herbs could help the restoration of these places. These findings provide insight into biogeographic patterns, biodiversity growth mechanisms, and community formation processes. Moreover, it can improve projections of climate change within and across ecosystems and provide the scientific basis for the use and management of plant resources in arid and semi-arid regions.
1 Introduction
Dryland ecosystems are susceptible to climate change due to their infertile soils and lack of vegetation (Bradford et al., 2020; Zhou et al., 2021). The hydroclimatic response to global warming is projected to be a dominant feature of the 21st century, resulting in fewer precipitation events and longer dry periods worldwide (Giorgi et al., 2014). As a result of these trends, drylands are likely to grow, and more people are likely to experience land degradation and water shortages. Consequently, living environments may become more fragile, and minor disturbances can have catastrophic consequences (Anjum et al., 2010; Bhargava, 2019). Therefore, the conservation of drylands requires understanding how drylands function (Moreno-Jiménez et al., 2019). The mountain ecosystem is a vital biodiversity center and an ecological function area in arid and semi-arid regions (Geng et al., 2022). Mountain ecosystems show apparent ecological vulnerability and are very difficult to recover from disturbances (Ahmad et al., 2020; Arif et al., 2023). Vegetation must be adequately managed and protected to reduce ecosystem vulnerability as species diversity loss may weaken ecosystem stability (Huang et al., 2016; Li et al., 2021; Arif et al., 2022).
The spatial distribution pattern of plant species diversity is a comprehensive reflection of various ecological gradient changes (Chi et al., 2014). α-diversity describes the species diversity within a community at a small scale or local scale, generally the size of one ecosystem, β-diversity describes the species diversity between two communities or ecosystems. But most of them have only focused on α-diversity, which might limit our understanding of the governing rules concerning biodiversity patterns along elevational gradients (Sarker et al., 2019). In fact, both α- and β-diversity play a vital role in understanding biogeographic patterns and underlying ecological processes (Mori et al., 2018), which should be integrated into the research.
It has long been recognized that the species diversity of different organisms changes in a predictable way with increasing elevation (Wiens and Donoghue, 2004; Muhammad and Changxiao, 2022). As with other environmental gradients, understanding the ecological processes associated with elevation gradients is imperative for developing more general patterns of spatial biodiversity (Rosindell et al., 2011). Abiotic factors such as temperature and precipitation change with elevation (Currie et al., 2004), but the corresponding changes in biodiversity are not consistent (Sun et al., 2020; Arif et al., 2022). In the context of different spatial and taxonomic scales, the changes in biodiversity along the altitudinal gradient need to be further explored (Pourmasoumi et al., 2019). A metacommunity is defined as a set of local communities that are linked by the diffusion of multiple potentially interacting species. The fundamental issues of metacommunity studies are the mechanism of multiple species coexistence in the same system and the causes and maintenance of biodiversity (Leibold et al., 2004). As a result, the metacommunity provides an effective idea and method for community assembly. It also explains the observed spatial and temporal distribution pattern of species, as well as the distribution and maintenance mechanism of biodiversity. Moreover, the community assembly mechanism and its process have always been an important and controversial topic in ecology. Generally, there are two basic community assembly processes, namely, the deterministic community assembly process based on niche theory and the stochastic community assembly process based on neutral theory (Laland et al., 2016; Rocha et al., 2018). According to niche theory, environmental factors such as climate and physics dominate plant species diversity patterns on various scales (Siefert et al., 2012; Qin et al., 2017; Ding et al., 2022), and community similarity decreases with the increase of environmental distance (Sun et al., 2020). However, some studies suggest that spatial autocorrelation of species diversity may also be caused by the stochastic community assembly process (Alonso and McKane, 2004; Volkov et al., 2007).
For plants, β-diversity is usually associated with the environment and spatial distance. Two components that are often interpreted as signals for different community assembly processes, such as environmental filtering and diffusion limitation (Keita et al., 2021), and that the relative contributions of the two are dependent on the study scale and ecosystem type (Legendre et al., 2009; Chase, 2010). Therefore, a decomposition of the relative contributions of the two processes to community assembly may facilitate a better understanding of community assembly processes (Götzenberger et al., 2012; Guo et al., 2018; Pinto et al., 2020). Environmental components indicate the importance of abiotic environmental controls on species distribution (environmental filtering), and climatic and topographic variables can serve as environmental variables (Keita et al., 2021). The spatial component can be calculated as a variable based on the original coordinates (longitude and latitude, and/or their polynomial values) (Borcard et al., 2004). Researchers have studied the impact of climate on the spatial replacement of different species (Davey et al., 2013; Jones et al., 2016). Topography not only increases the heterogeneity of environmental factors, including microclimate, but also increases the spatial isolation of species, thereby reducing the similarity between regions or communities, so some researchers consider it a separate factor (Qian and Ricklefs, 2012). In addition, the neutral theory holds that with an increase in spatial distance, the dispersal ability of species will show a trend of decline. This is because there is a spatial dispersal limitation for species. These three factors all affect the assembly of plant communities. Related studies have been conducted in various ecosystems, including tropical or subtropical forests (Chen et al., 2020; Li et al., 2021; Hu et al., 2022), temperate forests (Chudomelova et al., 2017), desert plant communities (Jiang et al., 2021), and plain areas (Marteinsdottir et al., 2018). Yet, their application to arid and semi-arid mountain forest ecosystems is limited, especially when considering the different plant layers. This gap has resulted in a lack of understanding of the processes shaping plant communities and an insufficient scientific basis for conserving biodiversity in arid and semi-arid mountain ecosystems (Andersen et al., 2015).
We investigated how the alpha and beta diversity indices vary with altitude in the Helan Mountain of China. In addition, the role of environmental filtering and dispersal limitations is also of importance in determining the diversity and composition variation of plants in arid and semi-arid regions (Hu et al., 2009). As the study area is in the temperate steppe climate zone of north-western China, it is assumed that the distribution area types of plant families and genera in the region are dominated by temperate components. Unimodal patterns have previously been observed in gradients in the Dhauladhar Mountains in the Lesser Himalayas and the Mount Namjagbarwa region (Ahmad et al., 2020; Sun et al., 2020), while a monotonic response to altitude has been observed in the Swedish mountains and a subarctic mountain tundra (Måsviken et al., 2019; Naud et al., 2019). Furthermore, we expect a unimodal or monotonically decreasing diversity with increasing altitude based on empirical observations. Based on ecological niche theory and neutrality theory, we hypothesize that environmental filtering and diffusion processes jointly explain the assembly of plant communities in the study area. However, their contribution needs to be further explored. The knowledge acquired from this study can assist protected area authorities in developing strategies for biodiversity conservation in arid and semi-arid areas.
2 Materials and methods
2.1 Study area
The Ningxia Helan Mountain National Nature Reserve (Figure 1), which traverses the junction of temperate grassland and desert, is a typical representative area of China’s wind-sand dry forest ecosystem (38°19′-39°22′N, 105°49′-106°41′E). Ningxia Helan Mountain can be divided into three parts: north, middle, and south. The vegetation in the northern section of the mountain is sparsely distributed. The middle section is rich in vegetation. The peaks above 3,000 m are centrally distributed here, but these areas are difficult to reach. The southern part of the mountain is low and sparsely vegetated. The Helan Mountain area has a typical temperate continental climate, and the annual average temperature can drop from 7.3°C at the front of the mountain to −0.9°C on the main peak; the annual precipitation span ranges from 200 mm at the base of the mountain to 500 mm at the main peak (Su et al., 2018). The vegetation of Helan Mountain has obvious vertical distribution characteristics, which may be affected by climate, soil, elevation and other factors (Geng et al., 2022). With the increase in elevation, vegetation types are desert steppe, terrace meadow, arboreal forest, and alpine meadow (Su et al., 2018). The corresponding soil types are grey desert soil, brown calcic soil, grey cinnamon, and subalpine meadow soil (Wu et al., 2021). “Near-natural recovery” is currently used as an important guideline for the restoration of degraded ecosystems in this region, aiming to restore biodiversity, structural and functional integrity, stability, and sustainability.
2.2 Field investigation and data acquisition
A total of 23 plots (20 m2 × 20 m2) were laid along the elevation transect ranging from 1,200 to 2,600 m ultimately. The sample plots were pre-established at an interval of 100 m. However, at some elevations, there were constraints pertaining to the availability of spatial area or due to the presence of steep slopes or large rocks between the sampling areas. If the environmental conditions were favorable for the setting of the sample plots, we also appropriately added some sample plots. Within each plot, five quadrats of 5 m2 × 5 m2 and five quadrats of 1 m2 × 1 m2 were marked for recording shrubs and herbs, respectively (Ahmad et al., 2020). Finally, 8 tree quadrats (only seven plots have trees), 115 shrub quadrats, and 115 herb quadrats were deployed, and all vegetation types in the study area were covered along the elevation gradient. We recorded the following in each plot: the diameter at breast height, height, and crown width of the trees, the base diameter, height, coverage, and number of clusters of shrubs, and the coverage, height, abundance of herbs (Fang et al., 2009). Elevation (EL), latitude (LAT), and longitude (LON) were collected with GPS (model: Geoxh6000, Trimble, United States). The slope (SLO) and the aspect (ASP) of the land were also recorded. Topographic variables include ASP, SLO and EL, LAT and LON served as spatial variables.
2.3 Climatic data collection
From the WorldClim database, temperature and precipitation data with a spatial resolution of 1 km at the corresponding coordinates of each plot were calculated in ArcGIS 10.5 to represent the climate variables (Liu et al., 2015; Fick and Hijmans, 2017). We selected the most commonly used metrics based on previous studies (Li et al., 2016; Liu et al., 2016; Wang et al., 2017; Wu et al., 2018; Sun et al., 2021): mean annual temperature (MAT), mean temperature of the coldest month (MTCM), mean temperature of the warmest month (MTWM), the annual variation of mean annual temperature (AVMAT), mean annual precipitation (MAP), precipitation of the driest quarter (PDQ), and precipitation of the wettest quarter (PWQ).
2.4 Statistics and analysis
The importance value demonstrates the relative significance of species in plant communities. The formula is as follows (Michelsen and Lindner, 2015):
Where IV is the important value, RC is the relative coverage, RD is the relative density, and RF is the relative frequency.
Biogeographical patterns of α-diversity were characterized using the following three indices (Zhang, 2018).
Shannon-Wiener index
Simpson index
Pielou index
Where Ni is the number of individuals in species i; N is the total number of individuals in all species, and S is the number of species.
As another dimension of diversity, β-diversity was used to quantify the variation in species composition across different communities (Socolar et al., 2016; Geng et al., 2022; Lu et al., 2018).
Jaccard index
Cody index
Where a and b are the number of unique species in the two communities, c is the number of species shared.
To reveal the elevation patterns of plant diversity, a generalized additive model (GAMs) was implemented by R software (version 3.2.3) using the “mgcv” library (Sarker et al., 2019). To construct the GAMs model, the distribution family and the corresponding link function need to be determined based on the data type and distribution characteristics of the response variable. As such, the distribution type of the response variable in this study was determined before the model was constructed. When continuous biodiversity data was considered as the response variable, previous studies have applied Gaussian distribution with an identity link function to GAMs (Donoghoe and Marschner, 2015). Therefore, we assumed the response variable approximated a normal distribution and applied Gaussian distribution with an identity link function to GAMs. Thin plate regression splines were chosen as smoothers for the separate variables (Yi et al., 2018). Redundancy analysis (RDA) was performed using the vegan package in R software (Oksanen et al., 2019) to investigate the diversity indices-variable relationships (Chen et al., 2020). For the explanatory variables, we screened before conducting RDA to exclude explanatory variables with high covariance, and then conducted model verification after RDA analysis. The Mantel test was used to assess the effect of environmental, topographic and spatial distances on the dissimilarity in the composition of vegetation assemblages (Ahmad et al., 2020). For this, a total of four matrices were formulated: (i) species composition (abundance), (ii) environmental composition, (iii) topographic composition, and (iv) geographical coordinates (spatial distance matrix for latitude and longitude). The dissimilarity (distance) matrices between the samples are calculated from the two sets of variables, i.e., the sample distances calculated from species abundance (Bray-Curtis distances) and from certain environmental parameters (Euclidean distances) respectively. Mantel tests based on Pearson’s correlation were performed to explore the correlations between community structure and environmental and spatial variables (Anderson and Walsh, 2010; Zheng et al., 2023). The analyses were performed using the ecodist package (Goslee and Urban, 2007). Each Mantel test produced an r-value similar to Pearson’s correlation index, which represents the correlation between the distance matrices. The significance of the correlation was assessed by randomizing the distance matrix 999 times. Finally, we used the vegan package to conduct variance partitioning analysis and analyzed the independent interpretations of the climatic distance, topographical distance, and spatial distance relative to the community assembly (Zheng et al., 2023). Environmental differences between plots were estimated using Euclidean distances. The spatial distance between each pair of plots was calculated using GPS coordinates taken from each plot and the R package fossil (Zheng et al., 2022).
3 Results
3.1 Composition and geographical flora in Helan Mountain
We identified a total of 121 plant species from 42 families and 90 genera between 1,200 m and 2,600 m in the study area. Families with the highest number of species were Asteraceae (16 species), Poaceae (13), Rosaceae (13), and Fabaceae (10). At relatively low elevation (1,200–1,500 m), the dominant species were Salsola laricifolia, Reaumuria trigyna, Ajania fruticulosa, Convolvulus tragacanthoides, Ulmus glaucescens, and Stipa Przewalski. At mid-elevation (1,560–1768 m), the dominant species were Amygdalus Mongolica, S. Przewalskyi, U. glaucescens, Agropyron mongolicum, and Stipa tianschanica. At high elevation (2013–2,600 m), the dominant species were composed of U. glaucescens, Picea crassifolia, Carex tristachya, Potentilla parvifolia, and Pinus tabuliformis (Table 1).
According to the classification scheme of seed plant distribution areas in China and related research (Wu et al., 2006; Zhao et al., 2014), we found that, on the regional scale (Table 2), there were 7 geographical distribution types of plant families. The type of “cosmopolitan distribution” (F1) contained the largest number of families (up to 17), and it was not counted as usual (Zhao et al., 2014). The type of “pantropic distribution” (F2) accounted for 32.00% of the total families. The “temperate distribution” (F8-F14) accounted for 64.00%, with the “north temperate distribution” accounting for the most (84.62% of the “temperate distribution”). In comparison, most of the genus-level geographical types have the attributes of “temperate distribution” (F8-F14, 72 genera in total). The “northern temperate distribution” (F8, 28 genera, accounting for 38.88% of the “temperate distribution”) accounted for the most proportion.
3.2 Change of α- and β-diversity indices of plant community along an elevation gradient
There are several native species of trees found on Helan Mountain, including P. crassifolia, P. tabuliformis, and Populus davidiana, and the undergrowth species are relatively abundant. Therefore, we only considered changes in the species diversity of the shrub and herb layers along the elevation gradient. For shrubs and herbs, a hump-shaped relationship that initially increased and then decreased was found between the α-diversity and elevation (Figure 2). The Cody index reflects the rate at which species are replaced along the habitat gradient. The Jaccard index indicates the proportion of species shared between sites to the total number of species in a region. The β-diversity characterized by the Cody index of shrubs and herbs showed a hump-shaped pattern across the elevation gradient, indicating a distinct species composition change among communities at mid-elevation. For shrubs, the Jaccard index varies along an elevation gradient with an inverted hump pattern, unlike the Cody index. The Jaccard index for herb species, on the other hand, remains higher at mid-elevation which shows an opposite trend to that of shrubs (Figure 3).
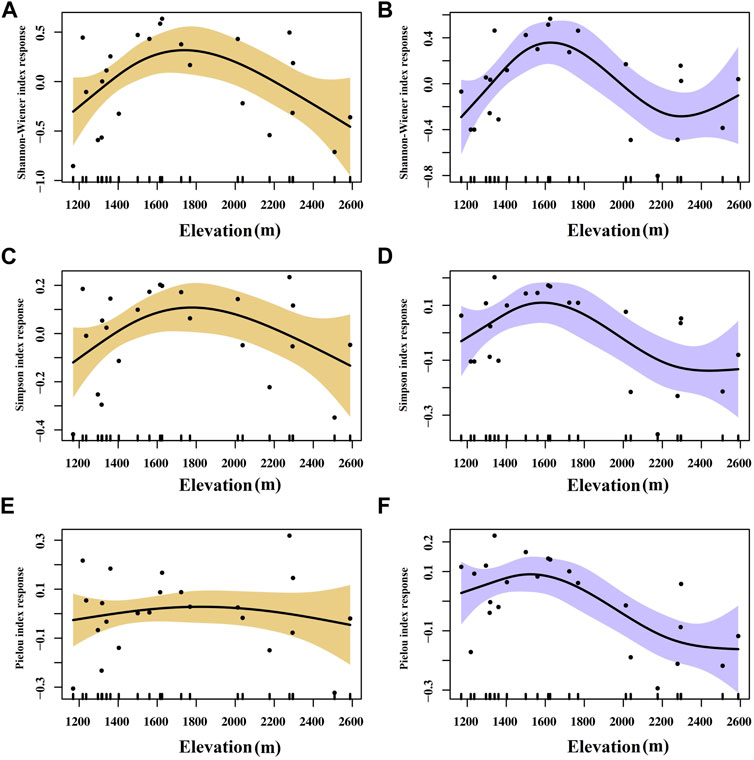
FIGURE 2. Response curves of Shannon-Wiener index, Simpson index, and Pielou index of shrub (A,C,E), and herb (B,D,F) plants to elevation in the GAM analysis. The vertical axes indicate the relative influence of each explanatory variable on the prediction. Shaded areas indicate 95% confidence limits.
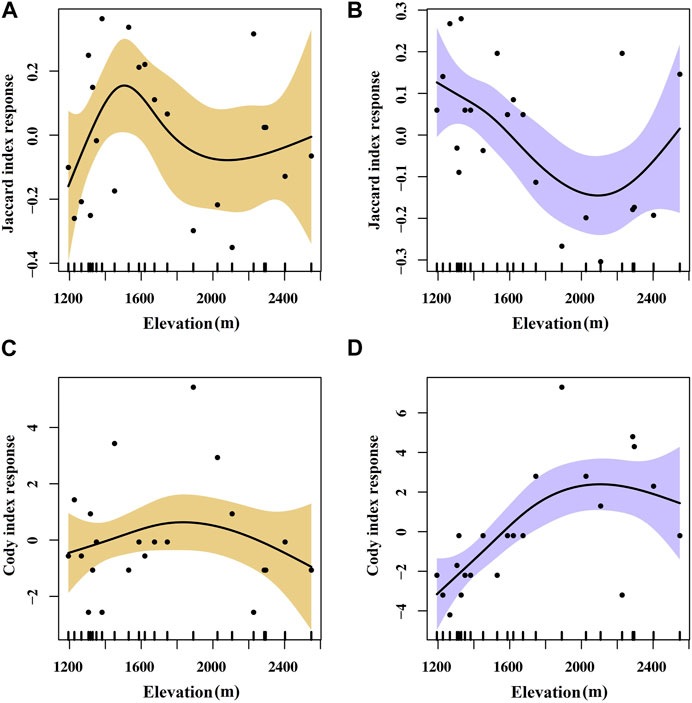
FIGURE 3. Response curves of Jaccard index, Cody index of shrub (A,C), and herb (B,D) plants to elevation in the GAM analysis. The vertical axes indicate the relative influence of each explanatory variable on the prediction. Shaded areas indicate 95% confidence limits.
3.3 Influence of environmental and spatial variables on plant diversity and community assembly
The results showed that (Figure 4), MAT and α-diversity of the herb layer showed a close positive correlation, while MAP showed scarcely negative correlation with α-diversity of the herb layer. For the shrub layer, MAP was positively correlated with the α-diversity index and weaker correlation with Shannon Wiener Index. For spatial factors, both LAT and LON were negatively correlated with the α-diversity index of the shrub layer. LON was positively correlated with the α-diversity of the herb layer, while LAT was negatively correlated with the α-diversity of the herb layer. The first axis was associated with MAT, ASP, SLO, LON, ELE, and LAT. The second axis was related to MAP and ELE. ELE and MAT have comparable interpretation rates on both axes. It was worth noting that the species diversity of the herb layer was positively correlated with LON and negatively correlated with MAP. On the contrary, the diversity indices of the shrub layer were positively correlated with MAP while negatively correlated with LON.
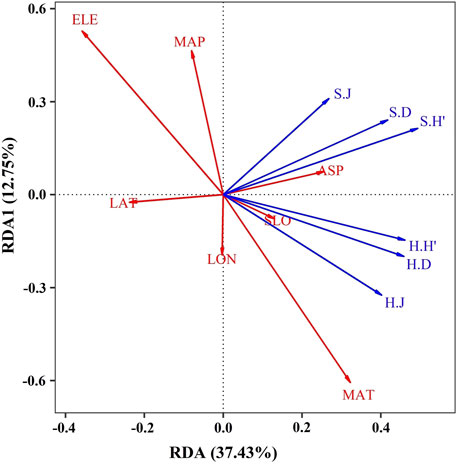
FIGURE 4. Biplots of RDA analysis between species diversity indices and topographical, spatial and environmental factors. Red lines represent environmental and spatial factors. The abbreviations used in the figure denote: EL, elevation; MAP, mean annual precipitation; ASP, aspect; SLO, slope; MAT, mean annual temperature; LON, longitude; LAT, latitude. Blue lines represent shrub and herb plants; shrub was abbreviated as “S” while herb was abbreviated as “H”.
The Mantel test (Table 3) showed that with increasing environmental differences (except for SLO distance and ASP distance) and spatial distance, the β-diversity among communities increased; that is, the species turnover rate within the communities increased. Species turnover of shrubs and herbs had significant positive correlations with changing climatic distance (except for PDQ distance). More importantly, the correlations between temperature-related distances and species turnover have all reached a significant level (p < 0.01). It is particularly in the shrub layer that MAT has the greatest effect on species turnover. Concerning spatial distance, slope and aspect distances showed a non-significant statistical correlation with the Bray-Curtis dissimilarity of the shrub and herb layer. As an indicator of habitat heterogeneity, elevation distance significantly impacted the β-diversity (p < 0.05), implying that species composition diverges with increasing elevation distance, particularly in herb communities. The correlations between species dissimilarity and spatial distance (except for LON distance in the shrub layer) showed positive correlations (p < 0.05). Variation partitioning analysis showed (Figure 5) that in terms of the size of the overall effect, the proportion of climatic, topographic, and spatial factors in the herb communities assembly was greater than that of the shrub layer, with a value of 0.41, 0.30, and 0.29; while the value in the shrub layer was 0.20, 0.07, and 0.08 respectively, interpretation of two or three variables also occupies a certain proportion. The combined effect of climate and space contributed to the community assembly of the herb layer with a value of 0.05, and the combined effect of climate, space, and topography also contributed to a certain extent, with a value of 0.02. For the shrub layer, the combined effect among the factors all contributed to the community assembly. In terms of independent effects, both climatic and topographic factors explained more variance than spatial factors for shrub and herb assembly, suggesting that changes in species β-diversity are more sensitive to environmental differences. The spatial variables were measured based on latitude and longitude. Plant distribution patterns, seed dispersal, and community assembly processes all depend on spatial characteristics (Grigoropoulou et al., 2022). Furthermore, the part explained by the spatial variables alone may represent the role of geological history and dispersal limitations on species diversity. In this study, the lower proportion of spatial factors explained the lower impact of a neutral process on community assembly.
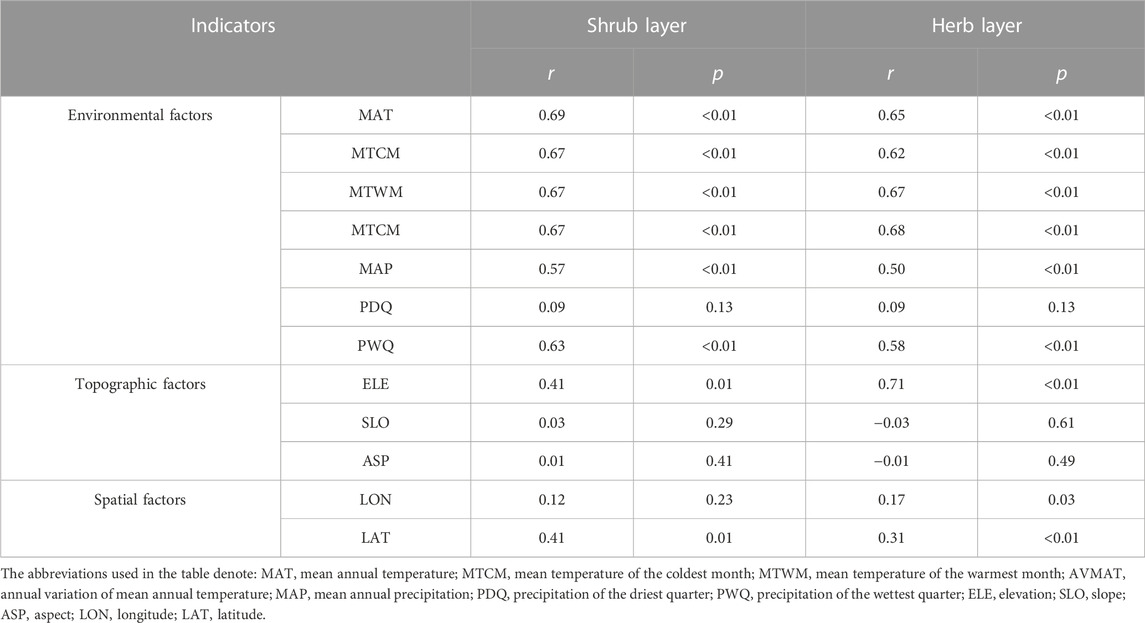
TABLE 3. Correlations between the Jaccard dissimilarity index, environmental, topographic and spatial factors for shrubs and herbs using the Mantel test.
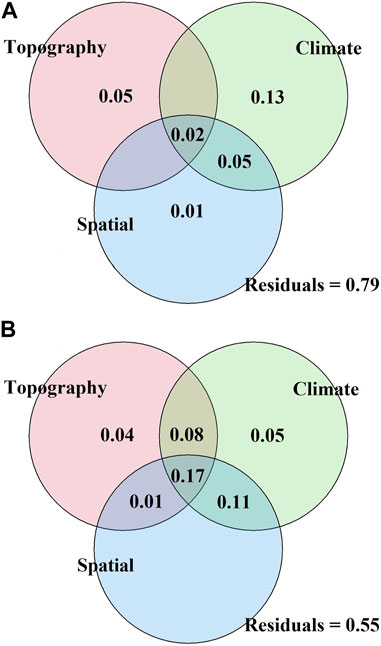
FIGURE 5. Variation partitioning analysis of the shrub (A) and herb (B) communities. The environmental and spatial variables explained the variation (adjusted R2). Climatic variables (MAT, MTCM, MTWM, AVMAT, MAP, PDQ, PWQ), topographic variables (ASP, SLO, EL) and spatial variation (x, y, x2, y2, xy, x2y, and xy2). Values less than zero were not displayed.
4 Discussion
4.1 Elevation patterns of α- and β-diversity indices
Altitude significantly influenced the α- and β-diversity. We found that the α-diversity initially increased and declined thereafter (Figure 2). This observed hump-shaped pattern of α-diversity is corroborated by similar findings in other regions. For example, in the Eastern Himalaya, India (Acharya et al., 2011), Alborz mountains, Iran (Mahdavi et al., 2013; Gómez-Díaz et al., 2017), Chilean andes (Lopez-Angulo et al., 2018), South-eastern pyrenees and nearby mountains of Catalonia (Grau et al., 2012). The most plausible explanation for the hump pattern was that the lower elevations of the Helan Mountain are dominated by shrub species of the genera Spinosa and Ajania. Herb species beneath these shrubs are scarce. In addition, the lower elevations are subject to frequent anthropogenic pressure regimes, as a result, the natural vegetation in this area was destroyed and the α-diversity was low (Zhang et al., 2016; Ahmad et al., 2020). The greater α-diversity at mid-elevation was largely associated with water availability and optimum temperature (Zhang et al., 2016; Vibhuti et al., 2018). This hump-shaped curve may also be closely related to habitat diversity at the median elevations (Chawla et al., 2008). Obviously, the higher elevation does not foster a rich diversity of plants, and only some shrub species and alpine meadows were found (Lee et al., 2013; Gómez-Díaz et al., 2017; Ahmad et al., 2020). More importantly, at higher elevations, plants usually face harsh environmental conditions such as extremely low temperatures, shorter growing seasons, and a high frequency of fog. These environmental conditions might have resulted in an overall reduction in species diversity at higher elevations.
The β-diversity calculated by the Cody index and Jaccard index indicated that community diversity was markedly affected by the elevation gradient (Figure 3). At mid-elevation gradients, the Cody index showed that species composition changed significantly, which could be explained by unique habitat and environmental conditions. Consequently, mid-elevation regions support unique species such as P. crassifolia, P. tabuliformis, and P. davidiana, with the structure and composition of the plant communities differing considerably from those at other elevation gradients. The maximum diversity at mid-altitudes could be explained by the mid-domain effect which aims to explain spatial patterns in species richness by invoking only stochasticity and geometrical constraints (Rangel and Diniz, 2005; Ahmad et al., 2020). Therefore, efforts should be made to prioritize the conservation of species in mid-elevational forests (Muhammad et al., 2022). Furthermore, the herb Jaccard index remained higher at mid-elevation, showing an opposite trend to that of shrubs, which was related to the life-history responses of different life-type plants and their response to the environment (Qin et al., 2019). It may also be related to competition between different species and disturbance factors (Whittaker et al., 2001). Our results showed that the α- and β-diversity show different distribution patterns along the altitudinal gradient, implying that the conservation of plant diversity in the Helan Mountains requires consideration of both scales of diversity indices (Chen et al., 2020).
4.2 Relative importance of environmental filtering and dispersal limitation in shaping plant diversity and assembly
In dryland and mountain ecosystems, temperature and precipitation strongly influence plant communities (Hawkins and Porter, 2003; Ulrich et al., 2014). They both function to regulate plant diversity across elevations. Since altitude correlates with significant changes in species diversity and vegetation composition in mountainous plant communities, there is a need to build a framework to understand and predict such patterns. Bray-Curtis dissimilarity reflects temporal and spatial changes in species turnover. It quantifies the effects of environmental and spatial factors on species turnover and can reveal the mechanisms of plant community assembly (Chen et al., 2020). In this study, the results of the Mantel test indicated that climatic, topographic, and spatial variables all affect species turnover (Table 3). The relatively weak influence of spatial factors on species turnover compared to topographic (elevation) and climatic factors suggests that environmental filtering was more important than dispersal limitations in shaping plant community assembly in Helan Mountain. The variational partitioning analysis further supported the results of the Mantel test (Figure 5). Climatic factors and topographic factors are more important than spatial factors in driving community turnover in shrub and herb communities. Compared to herb species, the more limited long-distance dispersal of shrub species may be due to the larger size of individuals or seeds (Farjalla et al., 2012), which was reflected in the association between shrub community assembly and spatial distance. In the present study, we found that climate has an important influence on community assembly, i.e., environmental filtering has greater importance than diffusion limitation in plant community assembly, which is consistent with previous research in desert regions of Northwest China (Wang et al., 2021). As a result, for plant protection of Helan Mountain, it is necessary to select species for artificial planting according to plant adaptability. Moreover, environmental filtering contributes more to herb community assembly than to shrub community assembly. In conjunction with the Ningxia Helan Mountain Forest Ecosystem Orientation Observation Research Station, the project team is working with the Ningxia Helan Mountain National Nature Reserve Administration to carry out some anthropogenic interventions to promote further restoration, as the study found little or no herbaceous presence in the undergrowth of the oil pine forest. We suggest that conducting environmental protection and enhancing species dispersal between communities [such as overcoming barriers to the spread of plant propagules (seeds) by means of artificial seed dispersal] will be necessary measures to promote community restoration and reconstruction.
The relative contributions of deterministic and stochastic processes to community assembly patterns at spatial scales remain controversial. For example, ecological niche differentiation plays a major role in communities that are relatively simple in structure, whereas neutral processes dominate the maintenance of species diversity in species-rich areas (Gravel et al., 2006). According to the variation partitioning analysis of our study, environmental differences have a stronger effect on changes in species composition than spatial distance (Figure 5). Given the lack of competition and other historical biogeographical factors, it seems that environmental filtering plays a more critical role than dispersal limitations in driving the assembly of plant communities in mountainous arid and semi-arid regions. In addition, the unexplained variations in plant species composition may be partly related to stochastic processes such as evolutionary and historical factors (Varzinczak et al., 2018). Moreover, other environmental factors not measured in our study, might contribute to the unexplained variations (Zheng et al., 2022), which should be taken into account in future work. Nevertheless, our results confirmed that environmental heterogeneity and dispersal limitations shaped the plant community assembly in this arid and semi-arid region of China.
5 Conclusion
At Helan Mountain, 121 plant species belonging to 42 families and 90 genera with obvious temperate characteristics have been identified. A unimodal hump-shaped pattern for α-diversity indexes was observed along the elevation gradient. At mid-elevation, obvious species composition changes were observed among the communities. Climatic factors and topographic factors were more influential than spatial factors in the assembly of shrub and herb communities, which indicates that changes in species β-diversity were more sensitive to environmental differences. Both environmental filtering and dispersal limitations had an impact on community assembly. It was found that environmental distance in shrub and herb communities explained more variation than spatial distance.
Data availability statement
The raw data supporting the conclusion of this article will be made available by the authors, without undue reservation.
Author contributions
XH, MA, JZ, and CL conceptualized and designed the study. XH, XN, ZY, QZ, JW, JZ, DD, and CL performed the fieldwork. XH, MA, and CL wrote and revised the manuscript. All authors contributed to the article and approved the submitted version.
Funding
This work was supported by Ningxia Key Research and Development Project (No. 2020BFG03006, 2021BEG02005), Ningxia Natural Science Foundation Project (No. 2020AAC03107), Foreign Young Talent Program (No. QN2022168001L); Chongqing Housing and Urban-Rural Construction Committee (No. Chengkezi-2022-6-3); Chongqing Municipality Key Forestry Research Project (2021-9); Forestry Extension Project of China Central Finance (No. Yulinketui 2023-8), China Postdoctoral Science Foundation (2021M703137), and Chongqing Postdoctoral Science Foundation (cstc2021jcyj-bsh0080). The Chongqing Municipal Education Commission for postgraduates innovation program, China (CYB22116).
Conflict of interest
The authors declare that the research was conducted in the absence of any commercial or financial relationships that could be construed as a potential conflict of interest.
Publisher’s note
All claims expressed in this article are solely those of the authors and do not necessarily represent those of their affiliated organizations, or those of the publisher, the editors and the reviewers. Any product that may be evaluated in this article, or claim that may be made by its manufacturer, is not guaranteed or endorsed by the publisher.
References
Acharya, B. K., Chettri, B., and Vijayan, L. (2011). Distribution pattern of trees along an elevation gradient of Eastern Himalaya, India. Acta Oecol 37, 329–336. doi:10.1016/j.actao.2011.03.005
Ahmad, M., Uniyal, S. K., Batish, D. R., Singh, H. P., Jaryan, V., Rathee, S., et al. (2020). Patterns of plant communities along vertical gradient in dhauladhar mountains in lesser Himalayas in north-Western India. Sci. Total Environ. 716, 136919. doi:10.1016/j.scitotenv.2020.136919
Alonso, D., and McKane, A. J. (2004). Sampling Hubbell's neutral theory of biodiversity. Ecol. Lett. 7, 901–910. doi:10.1111/j.1461-0248.2004.00640.x
Andersen, K., Naylor, B., Endress, B., and Parks, C. (2015). Contrasting distribution patterns of invasive and naturalized non-native species along environmental gradients in a semi-arid montane ecosystem. Appl. Veg. Sci. 18, 683–693. doi:10.1111/avsc.12185
Anderson, M. J., and Walsh, D. C. I. (2010). PERMANOVA, ANOSIM, and the mantel test in the face of heterogeneous dispersions: What null hypothesis are you testing? Ecol. Monogr. 83, 557–574. doi:10.1890/12-2010.1
Anjum, S. A., Wang, L., Salhab, J., Khan, I., and Saleem, M. F. (2010). An assessment of drought extent and impacts in agriculture sector in Pakistan. J. Food. Agric. Environ. 8, 1359–1363. doi:10.1007/s12602-012-9123-x
Arif, M., Jiajia, L., Tahir, M., Jie, Z., and Li, C. (2023). Environmental literacy scenarios lead to land degradation and changes in riparian zones: Implications for policy in China. Land Degrad. Dev. 34 (1), 156–172. doi:10.1002/ldr.4450
Arif, M., Li, J., Ding, D., He, X., Geng, Q., Yin, F., et al. (2022). Effect of topographical features on hydrologically connected riparian landscapes across different land-use patterns in colossal dams and reservoirs. Sci. Total Environ. 851, 158131. doi:10.1016/j.scitotenv.2022.158131
Bhargava, A. (2019). Climate change, demographic pressures and global sustainability. Econ. Hum. Biol. 33, 149–154. doi:10.1016/j.ehb.2019.02.007
Borcard, D., Legendre, P., Avois-Jacquet, C., and Tuomisto, H. (2004). Dissecting the spatial structure of ecological data at multiple scales. Ecology 85, 1826–1832. doi:10.1890/03-3111
Bradford, J. B., Schlaepfer, D. R., Lauenroth, W. K., and Palmquist, K. A. (2020). Robust ecological drought projections for drylands in the 21st century. Glob. Chang. Biol. 26, 3906–3919. doi:10.1111/gcb.15075
Chase, J. M. (2010). Towards a really unified theory for metacommunities. Funct. Ecol. 19, 182–186. doi:10.1111/j.0269-8463.2005.00937.x
Chawla, A., Rajkumar, S., Singh, K. N., Lal, B., Singh, R. D., and Thukral, A. K. (2008). Plant species diversity along an altitudinal gradient of Bhabha Valley in Western Himalaya. J. Mt. Sci. 5, 157–177. doi:10.1007/s11629-008-0079-y
Chen, G., Wang, W., Liu, Y., Zhang, Y., Ma, W., Xin, K., et al. (2020). Uncovering the relative influences of space and environment in shaping the biogeographic patterns of mangrove mollusk diversity. ICES J. Mar. Sci. 77, 30–39. doi:10.1093/icesjms/fsz204
Chi, X., Tang, Z., and Fang, J. (2014). Patterns of phylogenetic beta diversity in China's grasslands in relation to geographical and environmental distance. Basic. Appl. Ecol. 15, 416–425. doi:10.1016/j.baae.2014.07.003
Chudomelova, M., Zeleny, D., and Li, C. (2017). Contrasting patterns of fine-scale herb layer species composition in temperate forests. Acta Oecol 80, 24–31. doi:10.1016/j.actao.2017.02.003
Currie, D. J., Mittelbach, G. G., Cornell, H. V., Field, R., Guegan, J. F., Hawkins, B. A., et al. (2004). Predictions and tests of climate-based hypotheses of broad-scale variation in taxonomic richness. Ecol. Lett. 7, 1121–1134. doi:10.1111/j.1461-0248.2004.00671.x
Davey, C. M., Devictor, V., Jonzen, N., Lindstrom, A., and Smith, H. G. (2013). Impact of climate change on communities: Revealing species' contribution. J. Anim. Ecol. 82, 551–561. doi:10.1111/1365-2656.12035
Ding, D., Arif, M., Liu, M., Li, J., Hu, X., Geng, Q., et al. (2022). Plant-soil interactions and C:N:P stoichiometric homeostasis of plant organs in riparian plantation. Front. Plant Sci. 13, 979023–979117. doi:10.3389/fpls.2022.979023
Donoghoe, M. W., and Marschner, I. C. (2015). Flexible regression models for rate differences, risk differences and relative risks. Int. J. Biostat. 11, 91–108. doi:10.1515/ijb-2014-0044
Fang, J., Wang, X., Shen, Z., Tang, Z., He, J., Yu, D., et al. (2009). Methods and protocols for plant community inventory. Biodivers. Sci. 17, 533–548. doi:10.3724/SP.J.1003.2009.09253
Farjalla, V. F., Srivastava, D. S., Marino, N. A. C., Azevedo, F. D., Dib, V., Lopes, P. M., et al. (2012). Ecological determinism increases with organism size. Ecology 93, 1752–1759. doi:10.1890/11-1144.1
Fick, S. E., and Hijmans, R. J. (2017). WorldClim 2: New 1km spatial resolution climate surfaces for global land areas. J. Climatol. 37, 4302–4315. doi:10.1002/joc.5086
Geng, Q., Arif, M., Yuan, Z., Zheng, J., He, X., Ding, D., et al. (2022). Plant species composition and diversity along successional gradients in arid and semi-arid regions of China. For. Ecol. Manag. 524, 120542. doi:10.1016/j.foreco.2022.120542
Giorgi, F., Coppola, E., and Raffaele, F. (2014). A consistent picture of the hydroclimatic response to global warming from multiple indices: Models and observations. J. Geophys. Res. Atmos. 11, 11695–11708. doi:10.1002/2014JD022238
Gómez-Díaz, J. A., Krömer, T., Kreft, H., Gerold, G., Carvajal-Hernández, C. I., and Heitkamp, F. (2017). Diversity and composition of herbaceous angiosperms along gradients of elevation and forest-use intensity. Plos One 12, e0182893. doi:10.1371/journal.pone.0182893
Goslee, S. C., and Urban, D. L. (2007). The ecodist package for dissimilarity-based analysis of ecological data. J. Stat. Softw. 22 (7), 1–19. doi:10.18637/jss.v022.i07
Götzenberger, L., de Bello, F., Bråthen, K. A., Davison, J., Dubuis, A., Guisan, A., et al. (2012). Ecological assembly rules in plant communities-approaches, patterns and prospects. Biol. Rev. 87, 111–127. doi:10.1111/j.1469185X.2011.00187.x
Grau, O., Ninot, J. M., Ferre, A., Font, X., and Grytnes, J. A. (2012). Altitudinal species richness patterns of vascular plants in the south-eastern Pyrenees and nearby mountains of Catalonia. Plant. Ecol. divers. 5, 115–126. doi:10.1080/17550874.2012.666027
Gravel, D., Canham, C. D., Beaudet, M., and Messier, C. (2006). Reconciling niche and neutrality: The continuum hypothesis. Ecol. Lett. 9, 399–409. doi:10.1111/j.1461-0248.2006.00884.x
Grigoropoulou, A., Schmidt-Kloiber, A., Murria, C., and Qiao, H. (2022). Incongruent latitudinal patterns of taxonomic, phylogenetic and functional diversity reveal different drivers of caddisfly community assembly across spatial scales. Glob. Ecol. Biogeogr. 31, 1006–1020. doi:10.1111/geb.13479
Guo, Q., Fei, S., Shen, Z., Iannone, B. V., Knott, J., and Chown, S. L. (2018). A global analysis of elevational distribution of non-native versus native plants. J. Biogeogr. 45, 793–803. doi:10.1111/jbi.13145
Hawkins, B. A., and Porter, E. E. (2003). Water-energy balance and the geographic pattern of species richness of Western Palearctic butterflies: Water-energy balance and butterfly species richness. Ecol. Entomol. 28, 678–686. doi:10.1111/j.1365-2311.2003.00551.x
Hu, G., Jin, X., Wan, L., and Cai, X. (2009). The relationship between vegetation growth and the combination of water and heat in the Qilian Mountain. J. Arid. Land Resour. Environ. 23, 17–20.
Hu, X., Arif, M., Ding, D., Li, J., He, X., and Li, C. (2022). Invasive plants and species richness impact litter decomposition in riparian zones. Front. Plant Sci. 13, 955656–955714. doi:10.3389/fpls.2022.955656
Huang, J., Yu, H., Guan, X., Wang, G., and Guo, R. (2016). Accelerated dryland expansion under climate change. Nat. Clim. Change. 6, 166–171. doi:10.1038/nclimate2837
Jiang, L., Lv, G., Gong, Y., Li, Y., Wang, H., and Wu, D. (2021). Characteristics and driving mechanisms of species beta diversity in desert plant communities. Plos One 16, e0245249. doi:10.1371/journal.pone.0245249
Jones, M. M., Gibson, N., Yates, C., Ferrier, S., Mokany, K., Williams, K. J., et al. (2016). Underestimated effects of climate on plant species turnover in the Southwest Australian Floristic Region. J. Biogeogr. 43, 289–300. doi:10.1111/jbi.12628
Keita, K., Naoto, S., Marc, W. C., and Akira, S. M. (2021). The latitudinal gradient in plant community assembly processes: A meta-analysis. Ecol. Lett. 25, 1711–1724. doi:10.1111/ele.14019
Laland, K., Matthews, B., and Feldman, M. W. (2016). An introduction to niche construction theory. Evol. Ecol. 30, 191–202. doi:10.1007/s10682-016-9821-z
Lee, C., Chun, J., Song, H., and Cho, H. (2013). Altitudinal patterns of plant species richness on the baekdudaegan mountains, South Korea: Mid-domain effect, area, climate, and rapoport’s rule. Ecol. Res. 28, 67–79. doi:10.1007/s11284-012-1001-1
Legendre, P., Mi, X., Ren, H., Ma, K., Yu, M., Sun, I. F., et al. (2009). Partitioning beta diversity in a subtropical broad-leaved forest of China. Ecology 90, 663–674. doi:10.1890/07-1880.1
Leibold, M. A., Holyoak, M., Mouquet, N., Amarasekare, P., Chase, J. M., Hoopes, M. F., et al. (2004). The metacommunity concept: A framework for multi-scale community ecology. Ecol. Lett. 7, 601–613. doi:10.1111/j.1461-0248.2004.00608.x
Li, G., Xu, G., Guo, K., and Du, S. (2016). Geographical boundary and climatic analysis of Pinus tabulaeformis in China: Insights on its afforestation. Ecol. Eng. 86, 75–84. doi:10.1016/j.ecoleng.2015.10.032
Li, Y., Ye, X., and Wang, S. (2021). Ecosystem stability and its relationship with biodiversity. Chin. J. Plant Ecol. 45, 1127–1139. doi:10.17521/cjpe.2020.0116
Liu, Q., Liu, Y., Sun, X., Zhang, X., Sarula, K., Ding, Y., et al. (2015). The explanation of climatic hypotheses to community species diversity patterns in Inner Mongolia grasslands. Biodivers. Sci. 23, 463–470. doi:10.17520/biods.2015011
Liu, Y., Xu, Y., Shi, S., Peng, P., and Shen, Z. (2016). Quantitative classification and environmental interpretations for the structural differentiation of the plant communities in the dry valley of Jinshajiang River. Biodivers. Sci. 24, 407–420. doi:10.17520/biods.2015353
Lopez-Angulo, J., Pescador, D. S., Sanchez, A. M., Mihoc, M. A. K., Cavieres, L. A., and Escudero, A. (2018). Determinants of high mountain plant diversity in the Chilean Andes: From regional to local spatial scales. Plos One 13, e0200216. doi:10.1371/journal.pone.0200216
Lu, W., Yu, J., Ren, H., Mi, X., Chen, J., and Ma, K. (2018). Spatial variations in species diversity of mid-subtropical evergreen broad-leaved forest community in Gutianshan National Nature Reserve. Biodivers. Sci. 26, 1023–1028. doi:10.17520/biods.2018138
Mahdavi, P., Akhani, H., and Van der Maarel, E. (2013). Species diversity and life-form patterns in steppe vegetation along a 3000 m altitudinal gradient in the Alborz Mountains, Iran. Folia Geobot. 48, 7–22. doi:10.1007/s12224-012-9133-1
Marteinsdottir, B., Svavarsdottir, K., and Thorhallsdottir, T. E. (2018). Multiple mechanisms of early plant community assembly with stochasticity driving the process. Ecology 99, 91–102. doi:10.1002/ecy.2079
Måsviken, J., Dalerum, F., and Cousins, S. A. O. (2019). Contrasting altitudinal variation of alpine plant communities along the Swedish mountains. Ecol. Evol. 10, 4838–4853. doi:10.1002/ece3.6237
Michelsen, O., and Lindner, J. P. (2015). Why include impacts on biodiversity from land use in LCIA and how to select useful indicators? Sustainability 7, 6278–6302. doi:10.3390/su7056278
Moreno-Jiménez, E., Plaza, C., Saiz, H., Manzano, R., Flagmeier, M., and Maestre, F. T. (2019). Aridity and reduced soil micronutrient availability in global drylands. Nat. Sustain. 2, 371–377. doi:10.1038/s41893-019-0262-x
Mori, A. S., Isbell, F., and Seidl, R. (2018). β-Diversity, community assembly, and ecosystem functioning. Trends Ecol. Evol. 33, 549–564. doi:10.1016/j.tree.2018.04.012
Muhammad, A., Behzad, H. M., Tahir, M., and Li, C. (2022). The impact of ecotourism on ecosystem functioning along main rivers and tributaries: Implications for management and policy changes. J. Environ. Manage. 320, 1–11. doi:10.1016/j.jenvman.2022.115849
Muhammad, A., and Changxiao, L. (2022). Impacts of environmental literacy on ecological networks in the Three Gorges Reservoir, China. Ecol. Indic. 145, 109571. doi:10.1016/j.ecolind.2022.109571
Naud, L., Måsviken, J., Freire, S., Angerbjörn, A., Dalén, L., and Dalerum, F. (2019). Altitude effects on spatial components of vascular plant diversity in a subarctic mountain tundra. Ecol. Evol. 9, 4783–4795. doi:10.1002/ece3.5081
Oksanen, J., Blanchet, F. G., Friendly, M., Kindt, R., Legendre, P., McGlinn, D., et al. (2019). vegan: community ecology package. Avaliable At: http://CRAN.R-project.org/package=veganN.
Pinto, H. V., Villa, P. M., de Menezes, L. F. T., and Pereira, M. C. A. (2020). Effect of climate and altitude on plant community composition and richness in Brazilian inselbergs. J. Mt. Sci. 17, 1931–1941. doi:10.1007/s11629-019-5801-4
Pourmasoumi, M., Hadi, A., Mohammadi, H., and Rouhani, M. H. (2019). Effect of pycnogenol supplementation on blood pressure: A systematic review and meta-analysis of clinical trials. Phytother. Res. 34, 67–76. doi:10.1002/ptr.6515
Qian, H., and Ricklefs, R. E. (2012). Disentangling the effects of geographic distance and environmental dissimilarity on global patterns of species turnover. Glob. Ecol. Biogeogr. 21, 341–351. doi:10.1111/j.1466-8238.2011.00672.x
Qin, H., Dong, G., Zhang, Y., Zhang, F., and Wang, M. (2017). Patterns of species and phylogenetic diversity of Pinus tabuliformis forests in the eastern Loess Plateau, China. For. Ecol. Manag. 394, 42–51. doi:10.1016/j.foreco.2017.03.030
Qin, H., Zhang, Y., Dong, G., and Zhang, F. (2019). Altitudinal patterns of taxonomic, phylogenetic and functional diversity of forest communitie in Mount Guandi, Shanxi, China. Chin. J. Plant Ecol. 43, 762–773. doi:10.17521/cjpe.2018.0088
Rangel, T. F. L. V. B., and Diniz, J. A. F. (2005). Neutral community dynamics, the mid-domain effect and spatial patterns in species richness. Ecol. Lett. 8, 783–790. doi:10.1111/j.1461-0248.2005.00786.x
Rocha, M. P., Bini, L. M., Domisch, S., Tolonen, K. T., Jyrkankallio-Mikkola, J., Soininen, J., et al. (2018). Local environment and space drive multiple facets of stream macroinvertebrate beta diversity. J. Biogeogr. 45, 2744–2754. doi:10.1111/jbi.13457
Rosindell, J., Hubbell, S. P., and Etienne, R. S. (2011). The unified neutral theory of biodiversity and biogeography at age ten. Trends Ecol. Evol. 26, 340–348. doi:10.1016/j.tree.2011.03.024
Sarker, S. K., Reeve, R., Paul, N. K., and Matthiopoulos, J. (2019). Modelling spatial biodiversity in the world’s largest mangrove ecosystem-the Bangladesh sundarbans: A baseline for conservation. Divers. Distrib. 25, 729–742. doi:10.1111/ddi.12887
Siefert, A., Ravenscroft, C., Althoff, D., Alvarez-Yepiz, J. C., Carter, B. E., Glennon, K. L., et al. (2012). Scale dependence of vegetation-environment relationships: A meta-analysis of multivariate data. J. Veg. Sci. 23, 942–951. doi:10.1111/j.1654-1103.2012.01401.x
Socolar, J. B., Gilroy, J. J., Kunin, W. E., and Edwards, D. P. (2016). How should beta-diversity inform biodiversity conservation? Trends Ecol. Evol. 31, 67–80. doi:10.1016/j.tree.2015.11.005
Su, C., Ma, W., Zhang, X., Su, Y., Min, Y., Zhang, J., et al. (2018). Species composition and structure characteristics of the major montane shrub communities in the west slope of Helan Mountains, Nei Mongol, China. Chin. J. Plant. Ecol. 42, 1050–1054. doi:10.17521/cjpe.2018.0111
Sun, C., Zhou, Y., Fang, S., and Shang, X. (2021). Ecological gradient analysis and environmental interpretation of cyclocarya paliurus communities. Forests 12, 146. doi:10.3390/f12020146
Sun, L., Luo, J., Qian, L., Deng, T., and Sun, H. (2020a). The relationship between elevation and seed-plant species richness in the Mt. Namjagbarwa region (Eastern Himalayas) and its underlying determinants. Glob. Chang. Biol. 26, e01053–e03919. doi:10.1016/j.gecco.2020.e01053
Sun, Y., Hu, W., Yao, S., Sun, Y., and Deng, J. (2020b). Geographic patterns and environmental determinants of angiosperm and terrestrial vertebrate species richness in the Yellow River basin. Biodivers. Sci. 28, 1523–1532. doi:10.17520/biods.2020352
Ulrich, W., Soliveres, S., Maestre, F. T., Gotelli, N. J., Quero, J. L., Delgado-Baquerizo, M., et al. (2014). Climate and soil attributes determine plant species turnover in global drylands. J. Biogeogr. 41, 2307–2319. doi:10.1111/jbi.12377
Varzinczak, L. H., Lima, C. S., Moura, M. O., and Passos, F. C. (2018). Relative influence of spatial over environmental and historical processes on the taxonomic and phylogenetic beta diversity of Neotropical phyllostomid bat assemblages. J. Biogeogr. 45, 617–627. doi:10.1111/jbi.13150
Vibhuti, R., Bargali, K., and Bargali, S. S. (2018). Effects of homegarden size on floristic composition and diversity along an altitudinal gradient in Central Himalaya, India. Curr. Sci. 114, 2494–2503. doi:10.18520/cs/v114/i12/2494-2503
Volkov, I., Banavar, J. R., Hubbell, S. P., and Maritan, A. (2007). Patterns of relative species abundance in rainforests and coral reefs. Nature 450, 45–49. doi:10.1038/nature06197
Wang, J., Wang, W., Li, J., Feng, Y., Wu, B., and Lu, Q. (2017). Biogeographic patterns and environmental interpretation of plant species richness in desert regions of Northwest China. Biodivers. Sci. 25, 1192–1201. doi:10.17520/biods.2017149
Wang, J., Wang, Y., Li, M., He, N., and Li, J. (2021). Divergent roles of environmental and spatial factors in shaping plant beta-diversity of different growth forms in drylands. Glob. Ecol. Conserv. 26, e01487. doi:10.1016/j.gecco.2021.e01487
Whittaker, R. J., Willis, K. J., and Field, R. (2001). Scale and species richness: Towards a general hierarchical theory of species diversity. J. Biogeogr. 28, 453–470. doi:10.1046/j.1365-2699.2001.00563.x
Wiens, J. J., and Donoghue, M. J. (2004). Historical biogeography, ecology and species richness. Trends Ecol. Evol. 19, 639–644. doi:10.1016/j.tree.2004.09.011
Wu, A., Deng, X., Ren, X., Xiang, W., Zhang, L., Ge, R., et al. (2018). Biogeographic patterns and influencing factors of the species diversity of tree layer community in typical forest ecosystems in China. Acta Ecol. Sin. 38, 7727–7738. doi:10.5846/stxb201710191882
Wu, M., Chen, L., Pang, Z., Liu, B., Liu, L., Qiu, K., et al. (2021). Changes of the concentrations and stoichiometry of carbon, nitrogen and phosphorus in soil aggregates along different altitudes of Helan Mountain, Northwest China. Chin. J. Appl. Ecol. 32, 1241–1249. doi:10.13287/j.1001-9332.202104.029
Wu, Z., Zhou, Z., Sun, H., Li, D., and Peng, H. (2006). The areal-types of seed plants and their origin and differentiation. Jiangsu, China: Smithsonian Libraries and Archives.
Yi, Y., Sun, J., Yang, Y., Zhou, Y., Tang, C., Wang, X., et al. (2018). Habitat suitability evaluation of a benthic macroinvertebrate community in a shallow lake. Ecol. Indic. 90, 451–459. doi:10.1016/j.ecolind.2018.03.039
Zhang, J. T. (2018). Quantitative ecology. 3rd Edition. Wales, UK: University of Minnesota Libraries Publishing.
Zhang, W., Huang, D., Wang, R., Liu, J., and Du, N. (2016). Altitudinal patterns of species diversity and phylogenetic diversity across temperate mountain forests of northern China. Plos One 11, e0159995. doi:10.1371/journal.pone.0159995
Zhao, M., Wang, Y., Xing, K., Kang, M., Liu, Q., Li, Q., et al. (2014). Floristic traits and geographic patterns of natural montane forests at community level on the Loess Plateau, North China. Acta Geogr. Sin. 69, 916–925. doi:10.11821/dlxb201407004
Zheng, J., Arif, M., He, X., Ding, D., Zhang, S., Ni, X., et al. (2022). Plant community assembly is jointly shaped by environmental and dispersal filtering along elevation gradients in a semiarid area, China. Front. Plant Sci. 13, 1041742. doi:10.3389/fpls.2022.1041742
Zheng, J., Arif, M., He, X., Liu, X., and Li, C. (2023). Distinguishing the mechanisms driving multifaceted plant diversity in subtropical reservoir riparian zones. Front. Plant Sci. 14, 1138368. doi:10.3389/fpls.2023.1138368
Keywords: dryland, community ecology, α-diversity, β-diversity, environmental filtering, dispersal limitation
Citation: He X, Arif M, Zheng J, Ni X, Yuan Z, Zhu Q, Wang J, Ding D and Li C (2023) Plant diversity patterns along an elevation gradient: the relative impact of environmental and spatial variation on plant diversity and assembly in arid and semi-arid regions. Front. Environ. Sci. 11:1021157. doi: 10.3389/fenvs.2023.1021157
Received: 17 August 2022; Accepted: 02 May 2023;
Published: 11 May 2023.
Edited by:
Robert Guralnick, University of Florida, United StatesReviewed by:
Kulasekaran Ramesh, Indian Institute of Oilseeds Research (ICAR), IndiaCaitlin McDonough, University of Maine, United States
Copyright © 2023 He, Arif, Zheng, Ni, Yuan, Zhu, Wang, Ding and Li. This is an open-access article distributed under the terms of the Creative Commons Attribution License (CC BY). The use, distribution or reproduction in other forums is permitted, provided the original author(s) and the copyright owner(s) are credited and that the original publication in this journal is cited, in accordance with accepted academic practice. No use, distribution or reproduction is permitted which does not comply with these terms.
*Correspondence: Changxiao Li, lichangx@swu.edu.cn