Differential responses for stream algal assemblages exposed to factorial N and P enrichment along an in situ DOC gradient
- 1Department of Biology and Institute for Great Lakes Research, Central Michigan University, Mount Pleasant, MI, United States
- 2Department of Geography and Environmental Studies, Institute for Great Lakes Research, Central Michigan University, Mount Pleasant, MI, United States
Nitrogen (N) and phosphorus (P) enrichment from the surrounding landscape can enhance the growth and abundance of algal assemblages, both suspended in the water column and occurring as benthic biofilms in the receiving waters of resident stream ecosystems. However, it is unclear how enhanced levels of dissolved organic carbon (DOC) might mediate the response of algae to nutrients. In this study, we aim to understand the relationship between N and P enrichment in stream reaches with varying levels of DOC within a common watershed. Both suspended and benthic algal assemblages were exposed to increasing (low, moderate, high) levels of N and P in an in situ 3 × 3 factorial experimental design. Experiments were carried out simultaneously at three sites of varying DOC concentration, the results of which, showed that both assemblages responded very differently to enrichment. For suspended algae, biomass was enhanced following enrichment with both N and P singularly and in combination, particularly at the highest concentrations levels for both nutrients (3-way ANOVA interactions; p < 0.05). This pattern was consistent at all levels of DOC at the three sites (3-way ANOVA Nutrient-site interaction; F = 1.4, p = 0.228). In contrast, benthic algal biomass progressively increased with enhanced concentration of both N and P, but only at the lowest level of DOC (3-way ANOVA site term; F = 84.2, p = 0.0001). No appreciable biomass accumulation was observed under moderate or high DOC conditions and this pattern was likely attributed to low light penetration at these two sites (ANOVA). Our results indicate that eutrophication is likely to manifest itself in a complex manner throughout watersheds where loading of N and P interacts with DOC.
1 Introduction
Nitrogen (N) and phosphorus (P) play a key role in regulating the growth and accumulation of algae in freshwater lakes and streams (e.g., Smith et al., 1999; Tank and Dodds 2003), lending credence to the concept that nutrient synergisms are commonplace in regulating ecosystem production (Davidson and Howarth, 2007). N and P are often delivered at rates that create stoichiometric imbalances in the water column and in the tissues of resident stream organisms (Chaubey et al., 2007). Furthermore, external N and P loadings to streams through anthropogenic sources can further disrupt the natural balance between these potentially co-limiting nutrients (e.g., Asik et al., 2019). While suspended algae (phytoplankton) in lakes generally have a positive response to N and P enrichment, it is less well known how high concentrations of dissolved organic carbon (DOC) influence this response (e.g., Williamson et al., 1999).
DOC collectively refers to a suite of carbon-based chemicals components that is largely a natural complex of organic matter that has been leached from landscapes (Zhu et al., 2014) that is in some stage of decomposition (Cross et al., 2005). Like inorganic nutrients, DOC has allochthonous and autochthonous sources that can regulate concentrations and spatial-temporal availability (e.g., Williamson et al., 1999). High levels of colored DOC in lakes are associated with a shift from phytoplankton primary production towards suspended bacterial production (Jansson et al., 2000). Dominance of bacterioplankton production in river ecosystems tends to occur within dimly lit (dense forest cover) headwater regions that often contain low levels of dissolved inorganic nutrients (see Vannote et al., 1980). However, plankton dominate in the tail-waters of the river where there is reduced flow and higher amounts of light and nutrients (Wetzel 2001).
DOC can alter algal demands for additional N and P, by reducing light penetration in water and binding to nutrients, thereby further limiting their availability for use by phytoplankton (Jansson et al., 2000). Dissolved humic material (DHM) is a primary component of DOC that can influence the degree of nutrient limitation experienced by algal communities through chemical means. Specifically, DHM can bind with phosphate in the presence of trace materials such as iron or manganese, diminishing phosphorus availability to levels that can be limiting for resident algal communities (Jones et al., 1988). This raises the question about the role DOC might play in altering the response of algae to nutrient enrichment, through either diminished light availability or altering the supply of other micro-nutrient cofactors (e.g., Ylla et al., 2007).
Herein we studied the relationship between instantaneous enrichment with N and P and the response of natural stream algal assemblages (both suspended and benthic) along a natural DOC gradient manifested within a mixed land use watershed. We hypothesize that DOC mediates the growth response of algal assemblages by interacting with newly supplied N and P, either through reduced light penetration or by binding with cofactors that could further limit their growth (e.g., Klausmeiter et al., 2004). Specifically, we addressed three primary research questions: 1) can DOC regulate suspended alga biomass under varying levels of nutrient enrichment? 2) can DOC regulate benthic algal biomass under varying levels of nutrient enrichment? 3) is there a relationship between algal biomass and stream DOC for suspended and benthic algal assemblages in the Chippewa River watershed?
2 Methods
2.1 Field sampling and biogeochemical stream conditions
The Chippewa River watershed (total area: 2,952.8 km2) is a key contributor of material loading to Saginaw Bay (Lake Huron), where harmful algal blooms occur with some frequency (Brandon et al., 1991; Millie et al., 2014; respectively). The experimental component of the study focused on 3 sites (C2, C5, and C7) located in sub-watersheds of the Chippewa River basin whose land coverage is dominated by a mixture of forest and agriculture land covers (Figure 1). These sites were selected, because they had comparable physical conditions (water depth, stream width, canopy cover), yet had different DOC concentrations (see Marble, 2015). Site C2 (43° 36 41.46 N, 84° 57 12.42 W) had low DOC levels and occupied 254.8 km2 within a sub-watershed located in the western section of the Main Branch Chippewa. Site C5 (43° 43 34.98 N, 85° 0 13.20 W) had moderate DOC levels and was also located in the western section of the main branch, where it occupied 45.3 km2 of the sub-watershed. The substrate at both locations was composed of cobble and naturally round rocks approximately 64 mm –265 mm in size (diameter), with patches of sand and gravel. Site C7 (43° 43 38.10 N, 84° 51 11.16 W) had high DOC levels and occupied 76.4 km2 in the North Branch Chippewa River. The substrate in the reach was a mixture of sand and clay with some cobble.
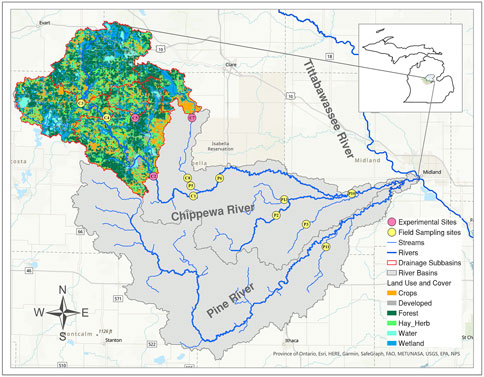
FIGURE 1. A map of the Chippewa River watershed that feeds the Tittabawassee River, and ultimately draining to coastal Lake Huron (Michigan, United States). The map depicts the 14 sampling points (colored circles), with the three experimental sites highlighted (pink-colored circles). Percent land use for the associated drainage subbasins was been delineated (forest, agriculture, and urban) where nutrient enrichment experiments were performed within the watershed.
Fourteen river sites were sampled in each of the 11 subbasins in the Chippewa River watershed. These samples were taken as part of a larger watershed monitoring program aimed at measuring biogeochemical conditions in overlying stream water (see Figure 1). Monthly water samples were collected at mid-depth in the center of each stream site from December 2012–13. Temperature, conductivity, pH was measured at in situ using a YSI sonde. Following this, a whole water samples were collected and stored in 500 mL (unfixed for DOC and chlorophyll analyses) and 125 mL (fixed with H2SO4 to pH < 2 for TP analysis) Nalgene bottles. Bottles were stored at 4°C for up to 28 days for total phosphorus (TP) analysis while chlorophyll samples were filtered (20 mL) onto membranes immediately upon returning to the lab (see below).
At all 14 monitoring sites, benthic biofilms were sampled from two rock substrata along one transect according to the USGS protocols used in the National Water-Quality Assessment Program (Moulton et al., 2002). Transects were divided in half (left and right); one rock was randomly sampled from each of these zones and intact stream biofilms were sampled from each rock substratum. The stream biofilm was removed from the rock by repeated scrubbing with a modified grout brush for 60 s, rinsing with reverse osmosis (RO) water, transferring the biofilm slurry to a plastic sample container, and recording total sample volume. The slurry collected from each rock was homogenized with a handheld food processer (30 s); aliquots were filtered for chlorophyll-a onto Whatman GFF filters (2 mL on 0.7 µm effective pore size) and for TP onto nitrocellulose filters (2 mL on 0.22 μm pore size). Once processed, all filter membranes were placed in cryotubes and stored in an ultra-low temperature freezer (−80°C) and held for subsequent analysis (see below).
At experimental sites C2, C5, and C7, light extinction coefficients were estimated by measuring photosynthetically active radiation (PAR) in the water column. PAR measurements were made using a 2 Π Licor underwater PAR sensor at sequential water depths of 0.0, 0.25. 0.50 m, 0.75 m, and 1.00 m in the water column. Light extinction coefficients were estimated by regression PAR versus depth for each site. Soil Water Assessment Tool (SWAT) was used to generate daily stream discharges (m3/s) at C2, C5, and C7 during the experimental period (month of October in 2013). By arranging the sites as sub-basins within the Chippewa River Watershed, the SWAT model was calibrated to the daily discharge flow of the Chippewa River obtained from the USGS 04154000-gauge station (Qiao et al., 2017). We previously described the SWAT model calibration and validation in (Tian et al., 2023).
2.2 Nutrient enrichment bioassays (NEB): Suspended algal assemblage
On 09 October 2013, additional collections of stream water were taken from the water column at sites C2, C5, and C7 to perform a series of nutrient enrichment bioassays (NEB). Overlying stream water was collected in carboys from each of these three sites along the streams in the watershed, that represented the range of DOC conditions throughout the watershed (see Table 1). Upon arrival back at the laboratory, water from the carboys was poured into duplicate treatment polycarbonate flasks (500 mL), and the nutrient concentration was altered for each treatment flask.
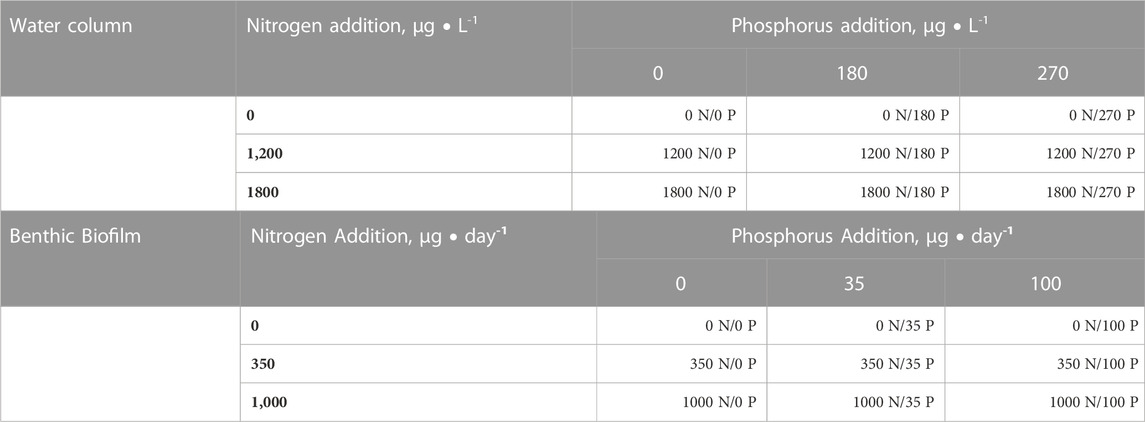
TABLE 1. Schematic outlining the 3 × 3 factorial design used for experiments performed in the Chippewa River watershed, Michigan (October 2013). For the water column experiments, nutrients were added at three levels for both N and P (as a single spike in μg L-1) to triplicate water samples. For benthic biofilms, nutrients were added at three levels of loading (over time as μg day-1) for both N and P to triplicate in situ nutrient releasing substrata.
Varying nutrient treatments were simulated using a 3 × 3 factorial design using three different concentrations of nitrogen and phosphorus (see Table 1). The nutrient additions were prepared using reagent grade sodium salt solutions of dissolved inorganic nitrate and phosphate (as NaNO3 and NaH2PO4, respectively). Experimental bottles were augmented with three levels of N enrichment (final N concentration of N1 = 0 μg L-1, N2 = 1,200 μg L-1, or N3 = 1,800 μg L-1) and P enrichment (final P concentration of either P1 = 0 μg L-1, P2 = 180 μg L-1, or P3 = 270 μg L-1), where the N1 and P1 were not augmented with added nutrients so that the nutrient concentrations in these bottle were considered no different from those present in ambient stream water. Experiments were designed in a 3 × 3 factorial, where a series of 9 treatments were administered in duplicate (9 treatments × 2 replicated = 18 bottles per site). Nutrient enrichment doses were set at concentrations that were approximately the same (P2 and N2) or double (P3 and N3) the typical TP and TN concentrations measured in the Chippewa River. TP concentrations were measured at 14 sites sampled throughout the watershed over a 9-month period (range 6.1–589.4 μg L-1; average ± one SD, 73.0 ± 90.9 μg L-1). TN concentrations were measured by the Saginaw Chippewa Indian Tribe (2013, data provided by J. Bailey) in 2 of 3 sites in the watershed that corresponded with our experiments (range, 641 to 2,640 μg L-1; average ± one SD; 1,641 ± 1,414 μg L-1). Treatment flasks were incubated in a Percival plant growth chamber until a growth asymptote was achieved (from 9–31 October 2013). The flasks were incubated at ambient light and temperature to match the in situ stream conditions in the field simulating the natural day-length period (0,800 h–2,100 h at 19.0°C). Flasks were randomly placed in the incubator and rotated daily. Subsamples were removed daily to measure in-vivo fluorescence using a 10-AU Turner Fluorometer until and asymptote in biomass was achieved, thereby indicating the growth promoted by our nutrient additions. After the end of the experimental period, the biomass algal accumulation (chlorophyll) was assessed by filtering subsamples from each flask onto filtered onto Whatman GFF filters, and these were analyzed for chlorophyll context as described below.
2.3 In Situ enrichment system (ISES): Benthic algal assemblage
The in situ enrichment system (ISES) was used to perform simultaneous field experiments at all three field sites (C2, C5, and C7) in tandem with the series of NEB experiments on 09–31 October 2013. NEB experiments have been used before to carry out environmentally relevant in situ experiments (Carrick and Price, 2011a; Taylor et al., 2022). The ISES experiments were run simultaneously with the NEB experiments to standardize the temporal period for all incubations and therefore the river algal assemblages that were naturally occurring at the outset of each experiment. The experimental design included enrichment with three levels of increasing P and three levels of increasing N (see Table 1); this was done to evaluate the effect both loading rates and nutrient combination had on the biomass accumulation of benthic biofilms.
Briefly, 50 mL polystyrene vials (approximately 8.0 cm × 2.7 cm) were filled with ultra-pure agar (2% w/v Difco ultra-Pure Agar Noble). Nutrient mixtures as the agar cooled to avoid potential toxicity (Tank et al., 2017) and inadvertent enrichment with unwanted trace elements in the agar (Carrick and Lowe, 1988). Agar was enriched with one of nine different combinations of N and P (added as salts of NaNO3 and NaH2PO4). Specifically, three molar nutrient stock solutions were made for nitrogen (final N concentration of N1 = 0.000M, N2 = 0.045M, N3 = 0.500 M) and phosphorus (final P concentration of P1 = 0.000M, P2 = 0.045M, and P3 = 0.500 M). Experiments were designed in a 3 × 3 factorial, where a series of 9 treatments were arranged that contained triplicate experimental substrata with all combinations of each nutrient (Table 1). Nutrient additions were chosen because the release of N and P by the ISES substrate mimicked average daily N and P loads that bracketed low and high loading conditions to natural stream ecosystems (see Corkum, 1996; Carrick and Price, 2011b; Taylor et al., 2022). For example, P treatments approximated P loading rates observed from a range of watersheds (see Ritter and Shirmohammadi 2001), but were modest compared with some large-scale PO4 −3 fertilization studies (range, 1–3.45 g P • day-1, see Miller et al., 1992).
Treatments were administered in triplicate (9 treatments × 3 replicates = 27 substrata per site). Porous, porcelain crucible lids (2.6-cm diameter) were fixed to the top of the agar-filled vials using inert aquarium sealant; the agar and sealant was allowed to harden overnight. These lids provided the substrate for biofilms to colonize on, as well as a porous surface, through which N and P were released during the course of the experiments (Gibeau and Miller 1989; Carrick and Price, 2011a). At each site, the 27 vials were randomly arranged in a 3 × 9 metal wire rack and transported to the stream sites where they were deployed at a uniform depth at each site (1.0 m in stream channel). All experiments were deployed in the central portion of each stream where stream depths were relatively uniform and comparable among the three sites.
After 21 days of incubation, the racks were removed from stream sites, so that the biofilms that developed on each experimental treatment could be scraped from the porous caps (using the modified grout brush) and washed into 50 mL storage vials (polyethylene, 50 mL) using three RO water rinses. Storage vials were sealed and transported to the lab for chlorophyll-a analyses within 2 h of collection. Once in the laboratory, a series of 2 mL aliquots from each of the treatment samples were concentrated onto Whatman GFF filters and stored at −80°C for subsequent chlorophyll (see below). In addition, a core was taken from the agar remaining from each treatment replicate, and this was placed into glass vials, and stored at −80°C for subsequent P analysis described below. As expected, benthic P loading rates delivered by the ISES substrata varied predictably among the nine experimental treatments and loading rates differed significantly among the nine treatments (two-way ANOVA; F = 3.42; p < 0.0001). Mean (± one standard deviation) experimental release rates from the treatments were 0.0 ± 1.32, 11.8 ± 6.48, and 161.7 ± 71.1 μg • day-1 for treatments P1, P2, and P3, respectively. Moreover, P loading rates were consistent among the three sites (F = 1.49; p = 0.216). We did detect release of P from the control treatment (P1), because the small P concentration in these experimental tubes (before and after the experiment) was not different from zero. Because no appreciable P was leached from the agar in the P0 treatment, we concluded that this treatment served as a proxy for the ambient phosphorus stream nutrient conditions. These results were consistent with previous studies using the ISES experiments (see Taylor et al., 2022).
2.4 Analytical procedures
2.4.1 Temperature, conductivity, pH, and light
Instantaneous, in-situ water temperature (°C), specific conductivity (μS • cm-1), and pH (units) were collected using a YSI 6600 Sonde meter at mid-depth in center of the stream. Instruments were calibrated prior to use with analytical standards (DEP 2003). Light extinction coefficients were determined for the overlying stream water column at each site by measured PAR versus depth profiles using a Licor LI-192 underwater sensor. Extinction coefficients were calculated according to Wetzel (2001).
2.4.2 Total phosphorus
Total phosphorus (TP) was measured on both water and biofilm samples collected during the stream survey; this analyte represents the collective P pool that included all organic and inorganic P present in each sample. For water column samples, a known volume of river water was analyzed (30 mL). For benthic biofilm samples, aliquots of biofilm slurry (2 mL) were filtered, placed into tubes, and treated with potassium persulfate. This mixture was then autoclaved for 30-min (at 110°C) to oxidize the P into DIP. The resulting DIP was measured using the colorimetric procedure described above using a Beckman Coulter series 700 spectrophotometer (USEPA 1997; USEPA, 2002).
2.4.3 Dissolved organic carbon (DOC)
The DOC concentration of the water column samples was determined (December 2012–2013) for all the 14 sampling locations. Water samples were filtered through membranes (0.25 mm, GF/F) (two duplicates) into glass vials (30 mL). The water was then acidified with two drops of concentrated HCL (12 N). Samples were analyzed at Central Michigan University (Uzarski laboratory) with high temperature combustion using a Shimadzu TOC-VCPH total organic carbon analyzer (Qiao et al., 2017).
2.4.4 Chlorophyll analysis
Chlorophyll concentrations were measured as a proxy for algal biomass in stream and experimental samples (Carrick and Schelske, 1997). Subsamples were filtered (2 mL aliquots) onto glass-fiber membranes (Whatman GF/F), placed in cryo-tubes and stored at −80°C. Within one month, filters were removed from the freezer and placed in vessels with a 60:40 dimethylsulfoxide to 90% acetone mixture to extract chlorophyll-a present in the samples. This supernatant was removed and analyzed on a 10-AU Turner fluorometer corrected for phaeopigments and chlorophyll-b concentrations (see Welschmeyer 1994).
2.4.5 Statistical analyses
Descriptive statistics were calculated for stream water quality (e.g., temperature, conductivity, pH), benthic algal chlorophyll, as well suspended chlorophyll and TP concentrations at the three sites where experiments were performed. One-way multivariate analysis of variance (MANOVA) tests were used to compare water quality measurements made using the YSI meter (temp., pH, Conductivity) and water samples (TP, chlorophyll) during the annual cycle at all three sites, where stream site was considered a fixed factor. Percent land use (five categories) was compared among the sites using a Chi-square contingency test.
Variation in algal biomass for both the NEB and ISES treatments was assessed using a three-way ANOVA, where average chlorophyll concentrations were compared among three fixed factors [i.e., site (DOC level), N treatment, and P treatment] and all interactions terms. Blocked one- and two-way analysis of variance coupled with Tukey post hoc multiple means comparisons (alpha = 0.05) were used to isolate the pair-wise differences to identify significant differences among treatment thereby characterizing any pattern in the response to enrichment within a site. To evaluate the relationship between algal biomass and stream DOC (sampled during December 2012–13), the ambient chlorophyll values for both suspended and benthic algal biomass (as chlorophyll) were correlated (Spearman Rank Correlations) with DOC concentrations in the overlying water column at all 14 river sites.
All data were log transformed (natural log) to meet assumptions of normality and homogeneity of variances. Normality was achieved for all ambient stream data, although normality was not achieved for any experimental data according to the Wilkes-Shapiro test; however, the residual distribution approached a normal distribution with no outliers in the data set. Homogeneity of variances was evaluated using the Bartletts test. Thus, these data met the assumptions for normality and homogeneity of variations and allowed the use of inferential statistics (i.e., ANOVA, linear regressions). Statistical analyses were conducted using the statistical software R programming version 4.2.2 (packages: Statistics, Graphics, ggplot, ggsignif, Fonts and colours, Lattice) and SPSS version 28.
3 Results
3.1 Biogeochemical conditions at experimental stream sites
We chose to run these experiments during the late growing season, because the Chippewa River tends to run at base flow during this period and the ambient nutrients are low. These conditions were reasonably met and were reflected in the environmental conditions we measured. Water chemistry was similar among the three stream sites where we performed experiments in the Chippewa River (Table 2). Of the 9 biogeochemical parameters evaluated, only DOC concentrations varied significantly among the three sites, where concentrations were similar at C2 and C5, but both sites had lower DOC concentrations compared with site C7 (Table 2; F = 25.3, p = 0.0018). Site C2 had the lowest DOC concentration of the three sites, modest conductivity values, circumneutral pH, and the lowest TP concentrations nutrients. Site C5 had median levels of DOC, higher TP concentrations, and similar pH, while site C7 had the highest levels of DOC, high TP concentrations, the lowest pH. PAR changed predictably with water depth at all three sites (all regressions of PAR versus depth had r2 values > 0.93), such that light extinction coefficients (slope of regression) were similar at C2 and C5, and these were less than that measured at site C7. Average conductivity and total dissolved solids values were similar among the three sites, despite the fact they all exhibited a large, seasonal range (range 174–631 μS cm-1 and 164–531 mg L-1, respectively). Stream discharge was measured daily for the entire period of the experiment (21 days from 09–31 October 2013); values among the three sites were very similar and ranged from 0.098 to 0.105 m-3 s-1.
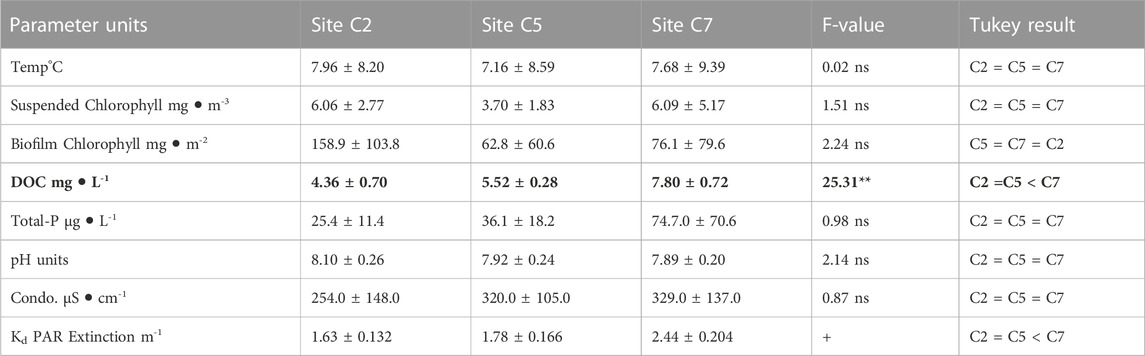
TABLE 2. Average (± one standard deviation) biogeochemical conditions compared among three stream sites in the Chippewa River watershed where experiments were carried out. Measurements were made at approximately monthly intervals; naturally occurring biofilms were sampled from two stream rocks, while all other water chemistry measurements were made from single grab samples collected from the overlying water column (December 2012–13). Comparisons were made using one-way MANOVA coupled with Tukey’s HSD pairwise mean tests (where ns = not significant, **p < 0.001). + For light extinction, Tukey’s comparisons were done on pairwise differences between the extinction coefficients. F values in bold were statistically significant.
Land cover was evaluated for the three sites as defined by five major land use categories; the sites were compared and no significant difference was observed (Chi-square χ2 = 6.882, df = 8, p = 0.5494). All three sites exhibited mixed land use within their respective sub-watershed. The sites were dominated by forest cover (>30%) and a mix of subdominant cover types. The sites supported similar levels of agriculture, urban, and wetland cover (5%–15%), while herbaceous cover was slightly higher (>20%) at sites C2 and C5 (low and moderate DOC, respectively).
3.2 Suspended algae and nutrient enrichment
Results from the NEB experiments showed that suspended algal biomass (as chlorophyll) was affected by interactions between both N and P enrichment and site location (Table 3). Suspended algal biomass was greatest at the highest DOC site (blocked one-way ANOVA, Figure 2) and varied significantly as a function of the singular effects of site (F = 212.97, p < 0.000), N enrichment (F = 662.52, p < 0.0001), and P enrichment (F = 266.01, p < 0.0001). Suspended biomass also varied significantly among the two-way interactive effects (F > 18.13, p < 0.0001 for all). The interactive response of suspended algae to the 3-way interaction of these factors was marginally significant (see Table 3).
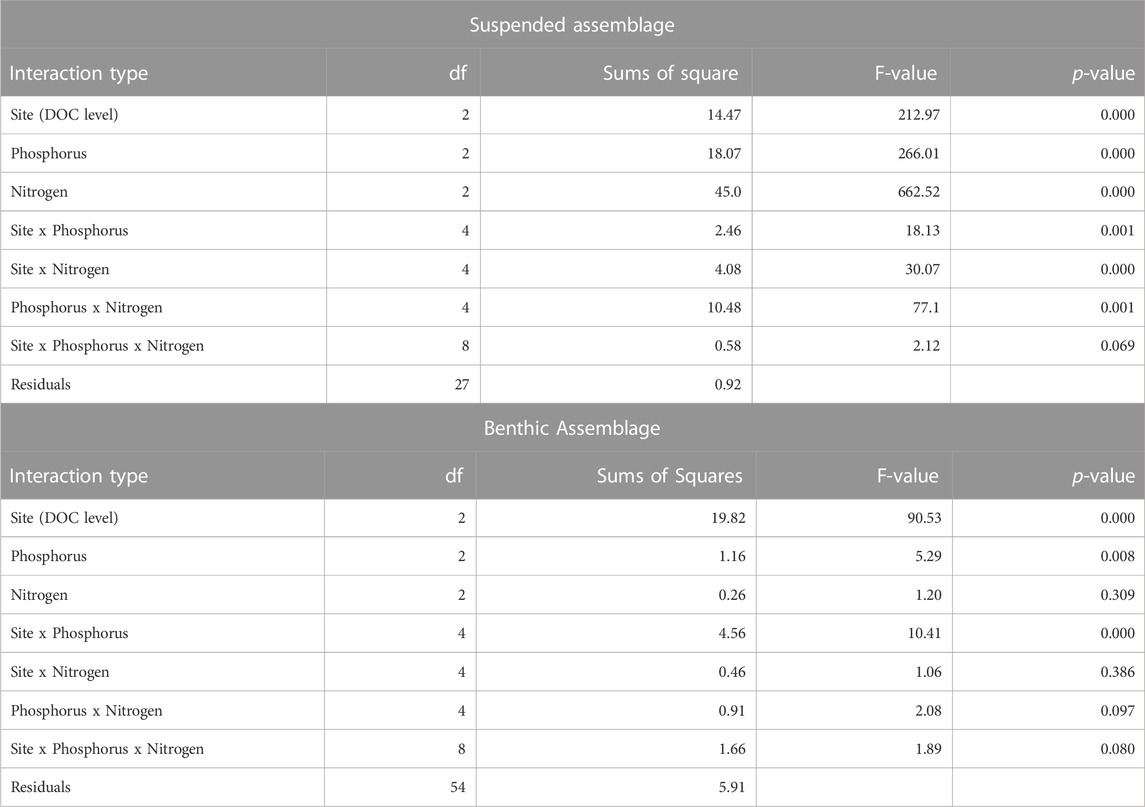
TABLE 3. Results from 3-way ANOVA evaluating the effects factorial nutrient enrichment had on both suspended and benthic algal biomass (as chlorophyll) among three stream sites of varying DOC concentration.
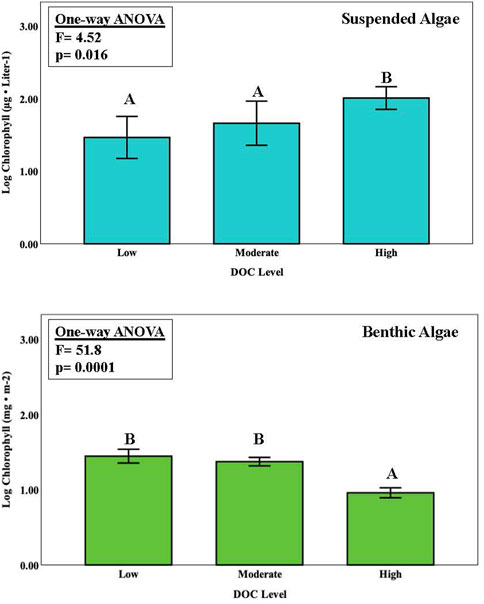
FIGURE 2. Chlorophyll concentrations (average ± one standard error) for suspended and benthic algal assemblages among three sites in the Chippewa River watershed where 3 × 3 factorial experiments were performed for varying levels of N and P enrichment (9 nutrient treatments). Common lettering joins treatments where no significant differences were observed (Tukey HSD pairwise comparisons, p < 0.05). One-way ANOVA was run separately (blocked) for the site treatment.
Overall, P enrichment led to an increase in suspended algal biomass (as chlorophyll concentrations) at all sites, where biomass was greater on the P2 and P3 treatments compared with the P1 controls (Figure 3; Table 3). Similarly, N also enhanced algal biomass at all sites; the biomass response between the N2 and N3 treatments was higher compared with the N1 treatment. Interestingly, there was no difference in biomass among the P1, P2, or P3 enrichment at the lowest N1 treatment at all three sites (no N enrichment). Chlorophyll concentrations in the N and P treatments were lower at the high DOC sites compared with the moderate and low DOC sites, although the there was no difference among control treatments among sites.
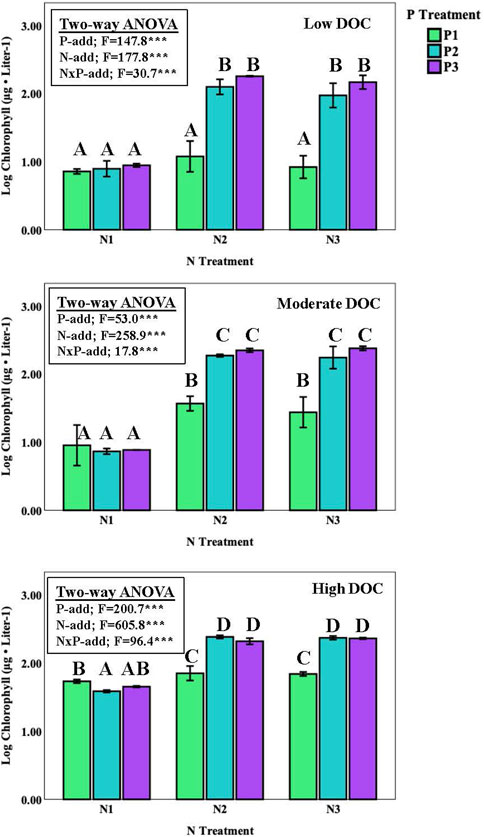
FIGURE 3. Chlorophyll concentrations (average ± one standard error) for suspended algal assemblages in response to varying levels of N and P enrichment (9 nutrient spike treatments). Common lettering joins treatments where no significant differences were observed (Tukey HSD pairwise comparisons, p < 0.05). One way ANOVA was run separately (blocked) for site and nitrogen treatment.
3.3 Benthic algae and nutrient enrichment
Results from the ISES experiments showed that benthic algal biomass (as chlorophyll concentration) exhibited a more straightforward response to nutrient enrichment compare with that for suspended algae in the Chippewa River (Figures 2, 4; Table 3). Overall, benthic algal biomass varied significantly among singular effects of site (3-way ANOVA, F = 90.53, p < 0.0001), P enrichment (F = 5.29, p < 0.008), and the interaction of between P enrichment with site (F = 40.41, p < 0.0001). Chlorophyll levels on the ISES substrata varied significantly along the DOC gradient, with values being 2 to 3-fold higher at the low DOC site (C2) compare with those present at the moderate and high DOC sites (blocked one-way ANOVA, Figure 2). N-enrichment had no measurable effect on benthic chlorophyll values at any of the sites, although depressed levels of chlorophyll were observed with the highest loads at low DOC. Benthic algal biomass was only enhanced with P enrichment at the lowest DOC level (site C2); significant biomass differences were not evident at the other two sites. At the low DOC site, P enrichment produced an incremental increase in algal biomass with P enrichment (Figure 4). No nutrient effect was observed at sites with moderate DOC and high DOC concentrations (Figure 4).
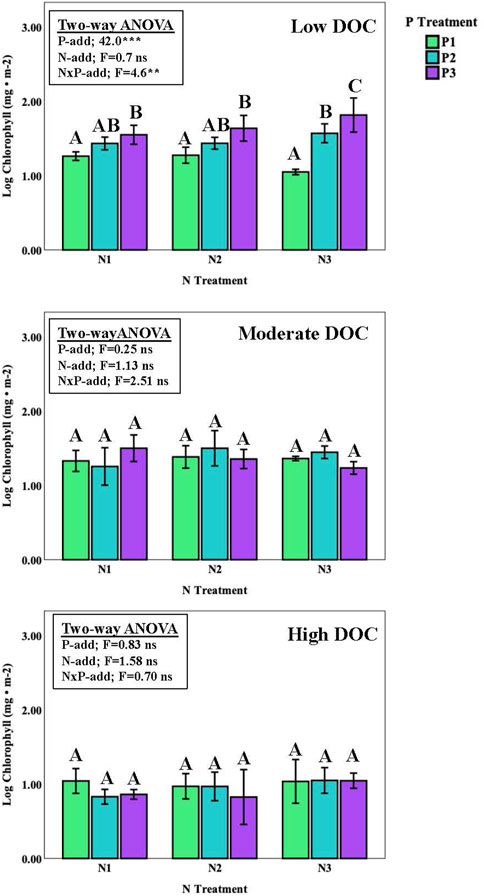
FIGURE 4. Chlorophyll concentrations (average ± one standard error) for benthic algal assemblages in response to varying levels of N and P enrichment (9 nutrient loading treatments). Common lettering joins treatments where no significant differences were observed (Tukey HSD pairwise comparisons, p < 0.05). One way ANOVA was run separately (blocked) for site and nitrogen treatments.
3.4 Algal biomass versus DOC
We used survey data to address the question of whether there was a relationship between algal chlorophyll concentrations and stream DOC for both suspended and benthic algal assemblages situated within the Chippewa River watershed (Figure 5). While there was no relationship between suspended algae chlorophyll and DOC among the 14 stream sites sampled (spearman rank correlation; rho = 0.041, p = 0.614, n = 151). Benthic algal chlorophyll was correlated with DOC (Figure 5).
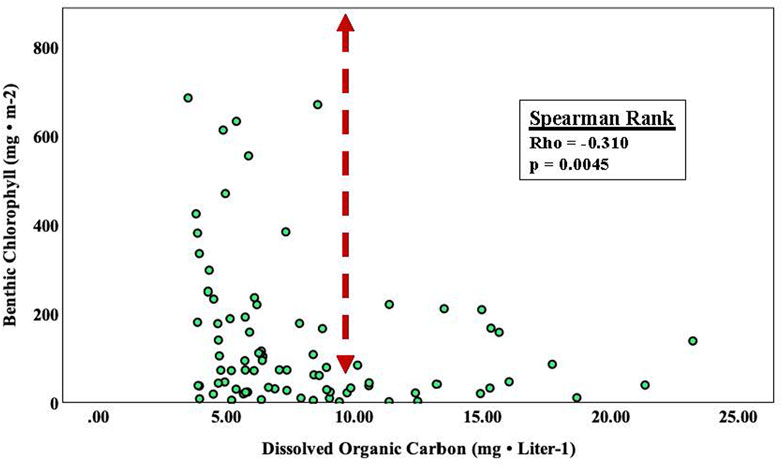
FIGURE 5. Seasonal survey data assessing the relationship between benthic algal chlorophyll concentrations and stream DOC at 14 samples locations within the Chippewa River watershed.
4 Discussion
N and P are known to be key factors regulating the biomass of algae in freshwater lakes and streams; however, these nutrients are delivered to streams along with a complex of potentially interacting substances (e.g., O’Brien and Dodds, 2010; Tian et al., 2023). DOC is primarily loaded to streams through allochthonous inputs, and therefore has been shown to interact with dissolved inorganic nutrients in various ways (Jansson et al., 2000; Ylla et al., 2007). The experiments we performed here were designed to evaluate the role DOC might play in altering the response of algae to nutrient enrichment, through direct (light availability) and more indirect (altering other micro-nutrient cofactors) mechanisms at results reflect a typical nutrient synergism, where algal biomass was limited by more than one nutrient. Specifically, algal yield was greater when both nutrients were added together compared with biomass yields when either nutrient was added singularly (see Davidson and Howarth 2007; Conley et al., 2009). Finally, DOC augmented the response of suspended algae, while DOC limited the response of benthic algae through apparent light limitation.
4.1 Algal response to nitrogen and phosphorus additions
Suspended algal chlorophyll responded to nutrient additions similarly among the sites, although the magnitude was greater at sites with higher DOC (see Figures 2, 3). This pattern of nutrient response is consistent with the idea that DOC augments the effect of nutrient limitation, perhaps providing additional sources of trace elements that further augment growth (e.g., micronutrients and metal cofactors, Evans et al., 2005). The greatest biomass was achieved when both N and P were added to the treatment flasks. Absolute concentrations in these flasks supported chlorophyll levels of ∼200 mg m-3 that were commensurate with levels of suspended algae typically measured in eutrophic lakes and streams (Wetzel 2001; Dodds 2007, respectively).
The N and P colimitation observed in our experiment agrees with experiments across a range of ecosystems (Smith et al., 1999; Elser et al., 2007) and is reasonable given the ratio of nutrients present in the water column (average ratio of TN 1641 to TP 74.7 μg L-1 = 22.0, see Tilman 1982). Given the low levels of suspended algal biomass in our control treatment (∼2 mg m-3) and the ratio of nutrients in the water column, it stands to reason that the algae were limited by both nutrients, so that considerably growth was achieved when nutrients were augmented above ambient concentrations. Nutrient colimitation (synergism) occurs when natural nutrient supplies are low and accompanying plant needs exist for more than one nutrient, so that both resources exist in close balance between need and supply; in such a case, there can be a rotation between the need for N and P (e.g., Allgeier et al., 2011; Asik et al., 2019). The significant interaction of nutrients with DOC levels in our experiments suggests that micronutrients, in addition to N and P, could have promoted the further production of suspended algal biomass in our experiments. Multi-species complexes often produce resultant colimitation of total algal biomass, which is result of differences in the individual resource needs of the numerous algal species present in the algal assemblage (see Tilman 1982). Although we did not quantify species composition in our experiments, there were multiple taxa of diatoms (e.g., Cyclotella spp., Melosira spp.) and Cyanobacteria (e.g., Chroococcus spp., Phormidium spp.) present in our experimental flasks. These taxa have varying requirements for nutrients (Tilman 1982; Tank and Dodds 2003), which can also explain why there was a difference in the algal biomass among the sites.
The benthic algal assemblage in our experiments only exhibited a response to N and P enrichment at the site with the lowest DOC concentration, indicating that other variables such as alterations in nutrient supply or light (see below) overrode the effect of nutrients as the primary limiting factor (see Carrick and Lowe 2007; Gergel et al., 1999, respectively). Our findings dovetail with other research on benthic algal biofilms, where nutrient limitation is not widespread and is not readily predictable based upon ambient water nutrient concentrations and ratios (see Francoeur et al., 1999). A meta-analysis done by Francoeur (2001) showed that a large fraction of experiments (using similar methods to those used here) yielded no nutrient response by benthic algae. Moreover, a comparison of nutrient enrichment studies among a series of ten streams, reported only 22% experiments that showed P limitation for benthic algal chlorophyll (Tank and Dodds 2003). The large fraction of experiments that showed no limitation is consistent with previous work showing that benthic algae can obtain resources from additional nutrient sources (e.g., lake sediments), thereby releasing them from primary or secondary limitation (see Carrick and Lowe 2007). Previous work also shows that intact, benthic biofilms removed nutrients from the overlying water column (for both PO4−3 and NO3−) thereby building internal stores of benthic nutrients over time in a cumulative sense (Mazumder et al., 1989; Blumenshine et al., 1997). There was low stream flow (∼3 m-3 s-1) at all three sites throughout the course of the experiment, and these conditions can increase the residence time of local nutrient sources. This in turn can enhance the period of sediment-water contact for the microbial assemblage present, conditions that have been found to promote the retention of N through denitrification and sedimentation within stream beds (Saunders and Kalff, 2001). Interestingly, our results appear to indicate that the biogeochemical conditions on these ISES substrata created a different nutrient environment than was available to the suspended algal assemblage; the later relied only on nutrient supplies in the overlying water column.
4.2 DOC effects on nutrient limitation of suspended algae
The impact of nutrient enrichment on lotic ecosystems typically does not necessarily manifest itself similarly for suspended versus benthic biofilms (e.g., Alexander et al., 2007). For this reason, we conducted simultaneous experiments to evaluate the response of both the benthic and pelagic algae to a combination of N and P enrichment delivered along a DOC gradient. Our findings show a varied response, where suspended algae were closely regulated by nutrients (type and concentration), while benthic algae only exhibited a response to nutrients at the low DOC site. This lack of response at the other sites could be attributed to increasing DOC that appeared to override any nutrient effect. However, we recognize that the experimental incubation conditions differed for each assemblage. The suspended algae were held in the laboratory at ambient light (100 μEinsteins m2 • s-1 of PAR, comparable to upper 0.25 m of water column), day length, and temperature matched to conditions in the stream. The relative constancy of the laboratory conditions may have allowed algae to better adapt to the environmental conditions present, and better utilize nutrient resources. At the same time, benthic algae were enriched under in situ conditions in the parent stream, where ambient conditions (light and temperature) were not controlled, and as a result, the field conditions may have limited the development of algal biomass on the ISES substrata.
DOC appeared to have augmented the effect of nutrients on suspended algae in the Chippewa River watershed. DOC can alter water chemistry as it complexes with a vast array of different metals and nutrients (e.g., Dillon and Molot, 1997; Sobczak et al., 2003). For example, iron has been reported in high concentrations in the presence of organic matter within aquatic ecosystems (Theis and Singer 1974), which if present, could have complexed with P in our experiments and muted the response at the moderate and high DOC sites. Iron is an essential element for aquatic organisms that can be taken up for use as active centers for enzyme activity, nutrient assimilation, and photosynthesis (Morel et al., 1991). Primary production of suspended particles is linked to the abundance and availability of iron and other trace metals (Morel and Price 2003). DOC can also reduce the over-abundance of potentially harmful heavy metals present within a solution, such as copper or mercury that are toxic to many aquatic species (Jackson et al., 1980; Williamson et al., 1999). The increase in suspended algal biomass with increasing DOC, might reflect a simultaneous decline in the concentration of toxic heavy metals if they were present.
Increasing concentrations of DOC in lake water have been shown to effectively decrease the penetration of ultraviolet radiation (UV wavelengths of 320 nm–400 nm) into lake and stream ecosystems (Gergel et al., 1999; Williamson et al., 1999). Ultraviolet radiation can inhibit photosynthesis due to damage of the photosystem and bleaching of pigments (Bothwell 1985; Xenopoulos et al., 2002). Again, DOC provides a barrier between the radiation and suspended particles because it acts as an attenuation agent (Morris et al., 1995). A UV barrier as described above could explain the enhanced response of suspended algae to nutrient enrichment in our experiments with moderate and high DOC water. The importance of a UV barrier has been studied using long-term mesocosm experiments, where phytoplankton protected from UV at higher levels of DOC and enriched with N and P exhibited an enhanced response to nutrients (see Zhang et al., 2021).
4.3 Role of DOC in regulating benthic algal yields in streams
Our findings reflect patterns in stream benthic algal biomass throughout the Chippewa River and perhaps to other streams that reside within temperate, mixed land-use watersheds. The benthic algal response to nutrients in our experiments was overridden at sites with moderate to high levels of DOC. Our data showed that these sites differ from one another in terms of their DOC levels and associated diminished PAR penetration in the water, at the same time other biogeochemical conditions were similar among the three sites. Light availability is a primary factor that regulates benthic algal biomass (Hansson 1992; Hauer and Hill 2006) and PAR penetration is strongly diminished by the presence of DOC in lake and ocean water (see Williamson et al., 1999), such that the rate of light diminution (extinction coefficient, Kd) increases predictably with increasing DOC (see Ask et al., 2009). Therefore, diminished light transmission associated with the DOC concentrations in stream water most likely mediated the benthic algal response to nutrients in the Chippewa River watershed (e.g., Mosisch et al., 2001).
Along these lines, the percent PAR reaching our experiments were 20.0%, 16.6%, and 10.0% for the low, medium, and high DOC sites, respectively. Fork et al. (2020) identified a unimodal relationship between chlorophyll accumulation and the proportion of PAR transmitted to the stream bottom, based upon a similar set of experiments to those performed here. They show peak chlorophyll levels at 50% PAR transmission, followed by a sharp decline at percentages from 40% to 0% transmission. According to their model, a 10%–50% reduction in biomass would be expected given the PAR transmission values delivered to the substrata in our experiments, an estimate that agrees well with the reductions between our control treatments under low/moderate versus high DOC sites (see Figure 2; chlorophyll 25.5 versus 9.6 mg m-2, respectively). An environmental stressor such as light limitation can induce algae to appropriate energy towards carbon versus nutrient metabolism, perhaps accounting for lower growth despite enhanced nutrient supply (see Lean and Pick, 1981). Moreover, community dynamics such as competition between the algae and other actors could have accounted for muted algal growth on the N and P treatments, particularly given the low light environment present in these streams (Bernhardt and Likens 2004).
To further explore the importance of DOC, we used survey data to address the question of whether there was a relationship between algal chlorophyll concentrations and stream DOC for both suspended and benthic algal assemblages situated within the Chippewa River watershed. While there was no relationship between suspended algae chlorophyll and DOC among the 14 stream sites sampled (spearman rank correlation; rho = 0.041, p = 0.614, n = 151), benthic algal chlorophyll was correlated with DOC (see Figure 5). Ask et al. (2009) estimated a decline in the contribution of benthic algal production with increasing Kd values and based on their report and those presented by Fork et al. (2020), a reduction in benthic production would be expected at DOC level of 8 mg L-1 (a corresponding Kd value of 2.0). Our results showed a similar pattern, as the range in chlorophyll concentrations narrowed considerably at DOC concentrations > 10 mg L-1. The results have important ecosystem implications, because in streams and lakes with DOC concentration of 8–15 mg L-1, the photic zone only extends 1–2 m below the surface (Jansson et al., 2000). With this in mind, DOC can set limits for production at higher trophic levels over those predicted based strictly upon nutrient concentrations (see Karlsson et al., 2009).
Data availability statement
The datasets presented in this study can be found in online repositories. The names of the repository/repositories and accession number(s) can be found in the article/supplementary material.
Author contributions
HC and CM designed the study. CM and HC collected and analyzed the data. HC, CM, and YT interpreted the data. CM and HC drafted the manuscript. HC and YT provided critical revision.
Funding
The research was supported by a grant to HC from the United States Environmental Protection Agency through the Pennsylvania Department of Environmental Protection, grant number 4300402290.
Acknowledgments
The paper was completed in partial fulfillment of the Master of Biology degree at Central Michigan University by CM; S. McNaught and Y. Tian served on her academic committee. The paper benefitted from comments provided by two reviewers. The following people provided technical assistance in the laboratory and field: E. Butts, K. Carrick, M. Hurley, N. Jammer, and A. Stimetz, and S. Taylor. We thank the Saginaw Chippewa Tribe for providing us with nitrogen data for the river (J. Baily). Dissolved organic carbon measurements were made by D. Uzarski. This paper is contribution number 183, Institute for Great Lakes Research at Central Michigan University.
Conflict of interest
The authors declare that the research was conducted in the absence of any commercial or financial relationships that could be construed as a potential conflict of interest.
Publisher’s note
All claims expressed in this article are solely those of the authors and do not necessarily represent those of their affiliated organizations, or those of the publisher, the editors and the reviewers. Any product that may be evaluated in this article, or claim that may be made by its manufacturer, is not guaranteed or endorsed by the publisher.
References
Alexander, R. B., Boyer, E. W., Smith, R. A., Schwarz, G. E., and Moore, R. B. (2007). The role of headwater streams in downstream water quality. Water Resour. 43, 41–59. doi:10.1111/j.1752-1688.2007.00005.x
Allgeier, J. E., Rosemond, A. D., and Layman, C. A. (2011). The frequency and magnitude of non-additive responses to multiple nutrient enrichment. J. Appl. Ecol. 48, 96–101. doi:10.1111/j.1365-2664.2010.01894.x
Asik, L., Kulik, J., Long, K. R., and Peace, A. (2019). Seasonal variation of nutrient loading in a stoichiometric producer-consumer system. Bull. Math. Biol. 81, 2768–2782. doi:10.1007/s11538-019-00629-6
Ask, J., Karlsson, J., Persson, L., Ask, P., Bystron, P., and Jansson, M. (2009). Terrestrial organic matter and light penetration: Effects on bacterial and primary production in lakes. Limnol. Oceanogr. 54, 2034–2040. doi:10.4319/lo.2009.54.6.2034
Bernhardt, E. S., and Likens, G. E. (2004). Controls on periphyton biomass in heterotrophic streams. Freshw. Biol. 49, 14–27. doi:10.1046/j.1365-2426.2003.01161.x
Blumenshine, S. C., Vadeboncoeur, Y., Lodge, D. M., Cottingham, K. L., and Knight, S. E. (1997). Benthic-pelagic links: Responses of benthos to water-column nutrient enrichment. J. North Am. Benthol. Soc. 16, 466–479. doi:10.2307/1468138
Bothwell, M. L. (1985). Phosphorus limitation of lotic periphyton growth rates: An intersite comparison using continuous flow troughs (thompson river system, British columbia). Limnol. Oceanogr. 30, 527–542. doi:10.4319/lo.1985.30.3.0527
Brandon, D. L., Lee, C. R., Simmers, J. W., Tatem, H. E., and Skogerboe, J. G. (1991). Information summary, area of concern: Saginaw river and Saginaw Bay. PDF.
Carrick, H. J., Aldridge, F. J., and Schelske, C. L. (1993). Wind influences phytoplankton biomass and composition in a shallow, productive lake. Limnol. Oceanogr. 38, 1179–1192. doi:10.4319/lo.1993.38.6.1179
Carrick, H. J., and Lowe, R. L. (2007). Nutrient limitation of benthic algae in lake Michigan: The role of silica. J. Phycol. 43, 228–234. doi:10.1111/j.1529-8817.2007.00326.x
Carrick, H. J., and Lowe, R. L. (1988). Response of Lake Michigan benthic algae to in situ enrichment with Si, N, and P. Can. J. Fish. Aquat. Sci. 45, 271–279. doi:10.1139/f88-032
Carrick, H. J., and Price, K. J. (2011b). Meta-analytical approach to explain variation in microbial phosphorus uptake rates in aquatic ecosystems. Aquat. Microb. Ecol. 65, 89–102. doi:10.3354/ame01537
Carrick, H. J., and Price, K. J. (2011a). Determining variation in TMDL reduction criteria. Final report. Pennsylvania: Pennsylvania Department of Environmental Protection, 84.
Carrick, H. J., and Schelske, C. L. (1997). Have we underestimated the importance of small phytoplankton in productive waters?. Limnol. Oceanogr. 42, 1613–1621. doi:10.4319/lo.1997.42.7.1613
Chaubey, , Sahoo, D., Haggard, H. E., Matlock, M. D., and Costello, T. A. (2007). Nutrient retention, nutrient limitation, and sediment-nutrient interactions in a pasture-dominated stream. Agric. Biol. Eng. 50, 35–44. doi:10.13031/2013.22409
Conley, D. J., Paerl, H. R., Boesch, D. F., Seitzinger, S. P., Havens, K. E., Lancelot, C., et al. (2009). Controlling eutrophication: Nitrogen and phosphorus. Nitrogen phosphorus 323, 1014–1015. doi:10.1126/science.1167755
Corkum, L. D. (1996). Patterns of nutrient release from nutrient diffusing substrates in flowing water. Hydrobiologia 333, 37–43. doi:10.1007/bf00020962
Cross, W. F., Benstead, J. P., Frost, P. C., and Thomas, S. A. (2005). Ecological stoichiometry in freshwater benthic systems: Recent progress and perspectives. Freshw. Biol. 50, 1895–1912. doi:10.1111/j.1365-2427.2005.01458.x
Davidson, E. A., and Howarth, R. W. (2007). Nutrients in synergy. Nature 449, 1000–1001. doi:10.1038/4491000a
Dillon, P. J., and Molot, L. A. (1997). Effect of landscape form on export of dissolved organic carbon, iron, and phosphorus from forested stream catchments. Water Resour. Res. 33, 2591–2600. doi:10.1029/97wr01921
Dodds, W. K. (2007). Trophic state, eutrophication, and nutrient criteria in streams. Trends Ecol. Evol. 22, 669–676. doi:10.1016/j.tree.2007.07.010
Elser, J. J., Bracken, M. E. S., Cleland, E. E., Gruner, D. S., Harpole, W. S., Hillebrand, H., et al. (2007). Global analysis of nitrogen and phosphorus limitation of primary producers in freshwater, marine, and terrestrial ecosystems. Ecol. Lett. 10, 1135–1142. doi:10.1111/j.1461-0248.2007.01113.x
Evans, C. D., Monteith, D. T., and Cooper, D. M. (2005). Long-term increases in surface water dissolved organic carbon: Observations, possible causes and environmental impacts. Environ. Pollut. 137, 55–71. doi:10.1016/j.envpol.2004.12.031
Fork, M. L., Karlsson, J., and Sponseller, R. A. (2020). Dissolved organic matter regulates nutrient limitation and growth of benthic algae in northern lakes through interacting effects on nutrient and light availability. Limnol. Oceanogr. Lett. 5, 417–424. doi:10.1002/lol2.10166
Francoeur, S. N. (2001). Meta-analysis of lotic nutrient amendment experiments: detecting and quantifying subtle responses. J. North Am. Benthol. Soc. 20 (3), 358–368. doi:10.2307/1468034
Francoeur, S. N., Biggs, B. J. F., Smith, R. A., and Lowe, R. L. (1999). Nutrient limitation of algal biomass accrual in streams: Seasonal patterns and a comparison of methods. J. North Am. Benthol. Soc. 18, 242–260. doi:10.2307/1468463
Gergel, S. E., Turner, M. G., and Kratz, T. K. (1999). Dissolved organic carbon as an indicator of the scale of watershed influence on lakes and rivers. Ecol. Appl. 4, 1377–1390. doi:10.1890/1051-0761(1999)009[1377:docaai]2.0.co;2
Gibeau, G. G., and Miller, M. C. (1989). A micro-bioassay for epilithon using nutrient-diffusing artificial substrata. J. Freshw. Ecol. 5, 171–176. doi:10.1080/02705060.1989.9665226
Hansson, L. A. (1992). Factors regulating periphytic algal biomass. Limnol. Oceanogr. 37, 322–328. doi:10.4319/lo.1992.37.2.0322
Hauer, F. R., and Hill, W. R. (2006). “Temperature, light, and oxygen,” in Methods in stream ecology. Editors F. R. Hauer, and G. A. Lamberti 2nd Edition (Academic Press), 103–117.
Jackson, T. A., Kipphut, G., Hesslein, R. H., and Schindler, D. W. (1980). Experimental study of trace metal chemistry in soft-water lakes at different pH levels. Can. J. Fish. Aquatic Sci. 37, 387–402. doi:10.1139/f80-053
Jansson, M., Bergstrom, A. K., Blomqvist, P., and Drakare, S. (2000). Allochthonous organic carbon and phytoplankton/bacterioplankton production relationships in lakes. Ecology 81, 3250–3255. doi:10.1890/0012-9658(2000)081[3250:aocapb]2.0.co;2
Jones, R. I., Salonen, K., and DeHaan, H. (1988). Phosphorus transformations in the epilimnion of humic lakes: Abiotic interactions between dissolved humic materials and phosphate. Freshw. Biol. 19, 357–369. doi:10.1111/j.1365-2427.1988.tb00357.x
Karlsson, J., Bystrom, P., Ask, J., Ask, P., Persson, L., and Jansson, M. (2009). Light limitation of nutrient poor lake ecosystems. Nature 460, 506–509. doi:10.1038/nature08179
Lean, D. R. S., and Pick, F. R. (1981). Photosynthetic response of lake plankton to nutrient enrichment: A test for nutrient limitation. Limnol. Oceanogr. 26, 1001–1019. doi:10.4319/lo.1981.26.6.1001
Klausmeiter, C. A., Litchman, E., Daufresne, T., and Levin, S. A. (2004). Optimal nitrogen-to-phosphorus stoichiometry of phytoplankton. Nature 429, 171–174. doi:10.1038/nature02454
Marble, C. S. (2014). Nutritional status of benthic biofilms in the Chippewa River watershed. M.S. in Biology. Central Michigan University, 47.
Mazumder, A., Taylor, W. D., McQueen, D. J., and Lean, D. R. S. (1989). Effects of fertilization and planktivorous fish on epilimnetic phosphorus and phosphorus sedimentation in large enclosures. Can. J. Fish. Aquat. Sci. 46, 1735–1742. doi:10.1139/f89-220
Miller, M. C., DeOliveria, P., and Gibeau, G. (1992). Epilithic diatom community response to years of P04-3 fertilization: Kuparuk River, Alaska (68 N Lat.). Hydrobiologia 240, 103–119. doi:10.1007/bf00013456
Millie, D. F., Weckman, G. R., Fahnenstiel, G. L., Carrick, H., Ardjmand, E., Young, W. A., et al. (2014). Using artificial intelligence for CyanoHAB niche modeling: Discovery and visualization of Microcystis-environmental associations within western Lake Erie. Can. J. Fish. and Aquat. Sci. 71, 1642–1654. doi:10.1139/cjfas-2013-0654
Morel, F. M. M., Hudson, R. J. M., and Price, N. M. (1991). Limitation of productivity by trace metals in the sea. Limnol. Oceanogr. 36, 1742–1755. doi:10.4319/lo.1991.36.8.1742
Morel, F. M. M., and Price, N. M. (2003). The biogeochemical cycles of trace metals in the oceans. Science 300, 944–947. doi:10.1126/science.1083545
Morris, D. P., Zagarese, H., Williamson, C. E., Balseiro, E. G., Hargreaves, B. R., Modenutti, B., et al. (1995). The attenuation of solar UV radiation in lakes and the role of dissolved organic carbon. Limnol. Oceanogr. 40, 1381–1391. doi:10.4319/lo.1995.40.8.1381
Mosisch, T. D., Bunn, S. E., and Davies, P. M. (2001). The relative importance of shading and nutrients on algal production in subtropical streams. Freshw. Biol. 46, 1269–1278. doi:10.1046/j.1365-2427.2001.00747.x
Moulton, S. R., Kennen, J. G., Goldstein, R. M., and Hambrook, J. A. (2002). Revised protocols for sampling algal, invertebrate, and fish communities as part of the national water-quality assessment program. Open file report 02-150. Australia: National Library of Australia.
O’Brien, J. M., and Dodds, W. K. (2010). Saturation of NO3− uptake in prairie streams as a function of acute and chronic N exposure. J. N. Am. Benthol. Soc. 29, 627–635. doi:10.1899/09-021.1
Qiao, H. J., Tian, Y. Q., Yu, Q., Carrick, H. J., Francek, M., and Li, J. (2017). Snowpack enhanced dissolved organic carbon export during a variety of hydrologic of events in an agricultural landscape, Midwestern USA. Agric. For. Meteorology 246, 31–41. doi:10.1016/j.agrformet.2017.06.002
Ritter, W. F., and Shirmohammadi, A. (2001). “Agricultural drainage and water quality,” in Agricultural nonpoint source pollution: Watershed management and hydrology. Editors W. F. Ritter, and A. Shirmohammadi (Boca Raton, FL: Lewis Publishers), 207–231.
Saginaw Chippewa Indian Tribe (2013). Nonpoint source assessment report for the Saginaw Chippewa Indian tribe of Michigan. Cleveland, OH: Tetra Tech Inc.
Saunders, D. L., and Kalff, J. (2001). Nitrogen retention in wetlands, lakes, and rivers. Hydrobiologia 443, 205–212. doi:10.1023/a:1017506914063
Smith, V. H., Tilman, G. D., and Nekola, J. C. (1999). Eutrophication: Impacts of excess nutrient inputs on freshwater, marine, and terrestrial ecosystems. Environ. Pollut. 100, 179–196. doi:10.1016/s0269-7491(99)00091-3
Sobczak, W. V., Findlay, S., and Dye, S. (2003). Relationships between DOC bioavailability and nitrate removal in an upland stream: An experimental approach. Biogeochemistry 62, 309–327. doi:10.1023/a:1021192631423
Tank, J. L., and Dodds, W. K. (2003). Nutrient limitation of epilithic and epixylic biofilms in ten North American streams. Freshw. Biol. 48, 1031–1049. doi:10.1046/j.1365-2427.2003.01067.x
Tank, J. L., Reisinger, A. J., and Rosi, E. J. (2017). Chapter 31-Nutrient limitation and uptake. Methods stream Ecol. 2, 147–171. doi:10.1016/B978-0-12-813047-6.00009-7
Taylor, S., Saia, S., Buda, A., Regan, J., Walters, T., and Carrick, H. J. (2022). Polyphosphate accumulation tracks incremental P-Enrichment in a temperate watershed (Pennsylvania, United States) as an indicator of stream ecosystem legacy P. Front. Environ. Sci. 10, 920733. doi:10.3389/fenvs.2022.920733
Theis, T. L., and Singer, P. C. (1974). Complexation of iron (II) by organic matter and its effect on iron (II) oxygenation. Environ. Sci. Technol. 8, 569–573. doi:10.1021/es60091a008
Tian, Y. Q., Yu, Q., Carrick, H. J., Becker, B., Francek, M., Anderson, O., et al. (2023). Analysis of spatiotemporal variation in dissolved organic carbon concentrations for streams with cropland-dominated watersheds. Sci. Total Environ. 861, 160744. doi:10.1016/j.scitotenv.2022.160744
Tilman, D. (1982). Resource competition and community structure. Princeton, NJ: Princeton University Press, 296.
U.S. Environmental Protection Agency (2002). National water quality inventory: 2000. Report. Washington, D.C: U.S. Environmental Protection Agency. Report EPA-841-R-02-001.
U.S. Environmental Protection Agency (1997). Volunteer stream monitoring: A methods manual. Washington, D.C: U.S. Environmental Protection Agency. Report EPA-841-R-02-001. EPA-841-B-97-003.
Vannote, R. R., Minshall, G. W., Cummins, K. W., Sedell, J. R., and Cushing, C. E. (1980). The river continuum concept. Can. J. Fish. Aquatic Sci. 37, 130–137. doi:10.1139/f80-017
Welschmeyer, N. A. (1994). Fluorometric analysis of chlorophyll a in the presence of chlorophyll B and pheopigments. Limnol. Oceanogr. 39, 1985–1992. –1992. doi:10.4319/lo.1994.39.8.1985
Wetzel, R. G. (2001). Limnology: Lake and river ecosystems. third edition. London, UK: Academic Press.
Williamson, C. E., Morris, D. P., Pace, M. L., and Olson, O. G. (1999). Dissolved organic carbon and nutrients as regulators of lake ecosystems: Resurrection of a more integrated paradigm. Limnol. Oceanogr. 44, 795–803. doi:10.4319/lo.1999.44.3_part_2.0795
Xenopoulos, M. A., Frost, P. C., and Elser, J. J. (2002). Joint effects of UV radiation and phosphorus supply on algal growth rate and elemental composition. Ecology 83, 423–435. doi:10.1890/0012-9658(2002)083[0423:jeoura]2.0.co;2
Ylla, I., Romani, A. M., and Sabater, S. (2007). Differential effects of nutrients and light on the primary production of stream algae and mosses. Fundam. Appl. Limnol. 170/1, 1–10. doi:10.1127/1863-9135/2007/0170-001
Zhang, Y., Li, K., Zhou, Q., Chen, L., Yang, X., and Zhang, H. (2021). Phytoplankton responses to solar UVR and its combination with nutrient enrichment in a plateau oligotrophic Lake Fuxian: A mesocosm experiment. Environ. Sci. Pollut. Res. Int. 28, 29931–29944. doi:10.1007/s11356-021-12705-3
Keywords: phosphorus, nitrogen, nutrient limitation, DOC, algae
Citation: Carrick HJ, Marble C and Tian YQ (2023) Differential responses for stream algal assemblages exposed to factorial N and P enrichment along an in situ DOC gradient. Front. Environ. Sci. 11:1066586. doi: 10.3389/fenvs.2023.1066586
Received: 11 October 2022; Accepted: 20 March 2023;
Published: 30 March 2023.
Edited by:
Thomas Hein, University of Natural Resources and Life Sciences Vienna, AustriaReviewed by:
Walter Dodds, Kansas State University, United StatesAdam Gordon Yates, University of Waterloo, Canada
Copyright © 2023 Carrick, Marble and Tian. This is an open-access article distributed under the terms of the Creative Commons Attribution License (CC BY). The use, distribution or reproduction in other forums is permitted, provided the original author(s) and the copyright owner(s) are credited and that the original publication in this journal is cited, in accordance with accepted academic practice. No use, distribution or reproduction is permitted which does not comply with these terms.
*Correspondence: Hunter J. Carrick, hunter.carrick@cmich.edu