- 1Department of Geology, Faculty of Science, Chulalongkorn University, Bangkok, Thailand
- 2Department of Geology, Center of Excellence in Morphology of Earth Surface and Advanced Geohazards in Southeast Asia (MESA CE), Faculty of Science, Chulalongkorn University, Bangkok, Thailand
- 3Center of Excellence on BCG Towards Sustainable Development, Chulalongkorn University, Bangkok, Thailand
- 4Department of Geology, Basin Analysis and Structural Evolution Research Unit (BASE RU), Faculty of Science, Chulalongkorn University, Bangkok, Thailand
Microplastics have polluted our environment, particularly mangrove ecosystems, the barriers between land and sea that trap sediments and pollutants. The abundance of microplastics has increased in microplastic deposition and is expected to rise in the future. In this study, mangrove sediment cores were collected from the Mae Klong River mouth and Queen Sirikit Park (shoreline), Samut Songkhram province. The microplastics were analyzed using a modified flotation method, then recasted and removed organic matter. Fiber microplastics is composed of 92% and 82% of the total microplastics that found in the Mae Klong River mouth and Queen Sirikit Park, respectively. There were significant changes in the abundance of microplastics in relation to the sediment particle size (p < 0.05). The difference in location between the Queen Sirikit Park and Mae Klong River mouth sites was significant (p < 0.01). The accumulation of microplastics in the sediments was influenced by grain size and location. The polymer types of microplastics were those normally used in textiles, indicating human activity. Moreover, micro-Fourier transform infrared (µ-FTIR) spectroscopy analysis identified compounds of other small particles, including tire rubber, pigment, paint, dyes, and flame retardant. These results imply that microplastics and microparticles have polluted the mangrove sediment in the Mae Klong River basin, which is an important fishery area in the upper Gulf of Thailand.
1 Introduction
Plastic is used globally for producing packages and components of consumer goods and other devices. It was invented in the 1900s and has been dramatically deployed since the 1950s (Gilbert, 2017) because of its features, such as durability, low production cost, flexibility, and chemical and water resistance. However, although plastic durability is an outstanding feature, plastic waste requires centuries to degrade. Thus, plastic is broken down into smaller pieces that become microplastics, whose particle size is smaller than 5 mm. Microplastics can be spread and accumulated in the environment for a long time (Claessens et al., 2011; Van Cauwenberghe et al., 2013).
Microplastics have extensively polluted the environment, as shown by historical microplastic records, including lake and marine sediments, ice sheets, and peat sequences. The evidence reveals that microplastics have accumulated in the environment from the late 1950s to now. Microplastics have been found in ice sheets (cores), for example, from the Arctic Ocean and Antarctic Sea ice (Kanhai et al., 2020; Kelly et al., 2020). They were also found in lake sediment areas, such as a dated-lake sediment core in the North London Lake (Turner et al., 2019), a dated sediment core in Japan, and the Donghu Lake in China (Dong et al., 2020). Meanwhile, microplastic sedimentation in China’s Donghu Lake has increased 10 times over the past 60 years. Lake sediment potentially hosts great amounts of microplastics produced by human activities (Turner et al., 2019). Moreover, microplastics are omnipresent in the marine environment. For example, the ocean is the destination of plastic waste generated on land and transported via rivers or coastlines (Jambeck Jenna et al., 2015). Furthermore, microplastics are also found in deep-sea sediment cores, such as the Rockall Trough, North Atlantic Ocean (Abel et al., 2022), and the Kuril Kamchatka Trench, Pacific Ocean (Abel et al., 2022).
Studies about microplastics in mangrove areas reveal that plastic has been buried across the Red Sea and the Arabian Gulf since the 1930s and has exponentially increased since the 1950s in line with global plastic production (Martin et al., 2020). Moreover, microplastics were found in core samples from Thailand, Malaysia, and South Africa (Matsuguma et al., 2017). Previous studies showed the occurrence of microplastics on land, especially in the boundary line between land and mangrove areas. Mangrove areas have a high sinking potential for sediment load and microplastics because of their plant root systems and low-energy environments (Mohamed Nor and Obbard, 2014). Moreover, the investigation of undated sediment cores from mangrove areas in southern Thailand showed that microplastics occurred in greater amounts in mangrove areas than that in outside areas because of the trapping potential of mangrove roots (Pradit et al., 2022). In addition, there was a significant amount of microplastics in the shelter of wetlands and the surrounding community area, whereas there was a lower amount in open sea-facing areas, the Gulf of Thailand, and the outer community area (Pradit et al., 2022).
Rivers are pathways that release plastic waste from land to the ocean (Meijer et al., 2021). Plastic waste ends up in the sea through various paths, including runoff, wastewater, rubbish dumping, and soil erosion (Zhang et al., 2018). In this regard, Thailand was ranked sixth in terms of mismanaged plastic waste among 192 coastal countries in 2010 (Jambeck Jenna et al., 2015). Nowadays, Thailand has also been listed as one of the top 20 countries in terms of annual plastic emissions into the ocean (Meijer et al., 2021). Because of improper waste management in Thailand, plastic debris is released into the sea. Most of the plastic debris in the ocean is caused by land activities, including wasteland, drain water, urban areas, and tourism, and a further 20% of the plastic waste is generated by marine activities, including commercial boats, fishing boats, shipping freight, and ocean tourism (Pollution Control Department, 2019). Plastic debris in the environment is eventually turned into microplastics by natural wind, tides, ocean currents, and UV radiation.
Microplastics can enter an organism’s body through ingestion (Wright et al., 2013). Previous studies showed that microplastics have been detected in bivalve tissues (Ding et al., 2021). Clams, oysters, mussels, and scallops can potentially receive suspended particles in the water, including microplastics. Microplastics can obstruct and damage an organism’s tissues (Deng et al., 2017; Ding et al., 2021). In addition, the microplastic surface can absorb heavy metals and toxic chemicals that may be accumulated in the body and cause diseases (Gallo et al., 2018).
Don Hoi Lot, listed as the Ramsar site under the Ramsar Convention, is Thailand’s most distinct wetland ecosystem in the Mae Klong River. This deep mangrove area is along the shoreline east of the Mae Klong River mouth (Pumijumnong, 2014). The Mae Klong River flows through Kanchanaburi, Ratchaburi, and Samut Songkhram and ends up in the upper Gulf of Thailand. This river is surrounded by urban and aquaculture areas and is thus an important place for fisheries and for rearing clams, mussels, and giant tiger prawns (Department of Marine and Coastal Resources, 2018).
Many studies have been conducted to study environmental changes, human activities related to the environment, and toxic metal elements in Samut Songkhram. As a result of a growing city, human activities cause environmental pollution (Qiao et al., 2015; Punwong et al., 2018; Klangnurak and Chunniyom, 2020). Meanwhile, the mangrove environment is a significant ecosystem providing young ocean animals with food and habitat. In this regard, one study collected and analyzed coastal sediments in Singapore and provided evidence of environmental change in the country (Mohamed Nor and Obbard, 2014). However, studies in this regard on Thailand’s core and mangrove sediments are limited. Thus, this study focused on a mangrove area that has potential microplastic sedimentation. This study estimated microplastic contamination in core sediments from the Mae Klong River’s mouth mangrove ecosystem.
2 Materials and methods
2.1 Study area
Sediment core CP1 and S1 were collected from mangrove area. This study was conducted at a mangrove area along the Mae Klong River mouth, Samut Songkhram, Thailand (Figure 1A). This area is part of the upper Gulf of Thailand. The S1 site was located at the river mouth near Don Hoi Lot (Figure 1B). Meanwhile, the CP1 site was situated in Queen Sirikit Park (Figure 1C), known as a mangrove conservation area. The Mae Klong River Estuary area’s unique characteristic, known as Don Hoi Lot, covers 4 km wide at the Mae Klong River mouth. A mudflat area consisting of sand and mud was smoothly plain with less than 1% slope. According to the location of CP1 site surrounding the exuberant mangrove ecosystem, this site has been an important resource for inhabiting aquatic species. Moreover, bamboo walls along the shore of Samut Songkhram province protected the mangrove area from coastal erosion (Figure 1C).
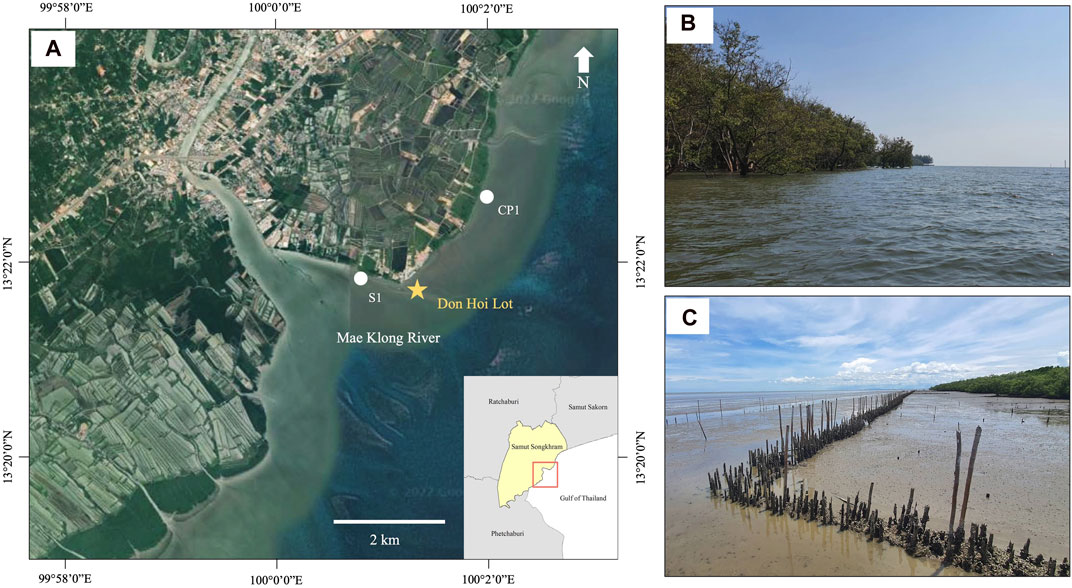
FIGURE 1. Study area. (A) Location of the Mae Klong River mouth on the upper gulf of Thailand. (B) Landscape of the S1 site located near Don Hoi Lot. (C) Landscape of the CP1 site located on the east side of the Mae Klong River Mouth.
2.2 Core sampling
Considering plastic contamination in the field and laboratory, the use of a plastic-made tool was avoided, and the spoon, blade, and glass bottle were rinsed with distilled water before use. The equipment should be sealed or covered away from airborne microplastic contamination. Two sediment cores were collected from two sites in front of the mangrove area at the Mae Klong River mouth. The samples were picked up at the border of the mangrove forest to prevent root disruption. The sediment cores were taken using a Russian corer (100 cm in length and 8 cm in diameter). The CP1 core (Queen Sirikit Park) was collected from the overlap sediment core, and the total length of the site was 142 cm in depth. A 52-cm core was collected at the S1 site (near Don Hoi Lot). After returning to the laboratory, the core samples were sliced into 1-cm-thick pieces using a stainless blade and individually stored in a container for further analysis.
2.3 Sediment analysis
Each consecutive 2-cm interval sample was used to estimate the qualitative changes in organic matter of sediments through loss on ignition (LOI). The samples were dried in an oven at 105°C for 12 h and then combusted at 550°C for 6 h. The organic content was calculated from the weight loss percentage (Heiri et al., 2001).
The sediment’s particle size was analyzed using a laser diffraction machine (Malvern 3,000). Each consecutive 5-cm interval core sediment was dried in an oven at 60°C for 48 h. Then, 1–2 g of dried sediment was sieved through a No. 4 mesh (4.75 mm) and sent to the Scientific and Technological Research Equipment Centre, Chulalongkorn University.
2.4 Microplastic analysis
Microplastic abundances were determined for each consecutive 1-cm slice of the core. Wet sediment was dried in an oven at 60°C for 48 h. First, the floating method was used to separate particles using a ZnCl2 solution (density 1.6–1.7 g mL−1). The ZnCl2 solution allowed sediments with higher density than that of the solution to sink to the bottom while plastics and some organics floated up in the supernatant. All solutions were filtered using 1.2 μm mesh size filter paper before use to avoid MPs contamination. Next, 5-g dry-weight sediment was mixed with ZnCl2 (50 mL) at 500 rpm for 5 min and allowed to settle for 3 h. The supernatant containing particles less dense than 1.6 g mL−1 was poured through a Millipore filter (glass microfiber filter GF/C, 1.2-µm pore, 70-mm diameter, Whatman) using vacuum filtration. The floating method was repeated three times [modified from Lo et al. (2018)]. Next, 30% hydrogen peroxide was used to remove the organic substance associated with microplastics. The filter containing microplastics and organic substances was rinsed using 50 mL of H2O2. The solution was heated at 70°C for 1 h and allowed to settle overnight [modified from Duan et al. (2020)]. Then, microplastics were counted and observed under a stereomicroscope. Their characteristics and plastic properties were primary checked by hot needle technique test and appearance inspection including non-cellular structure, equally thick especially in fiber, homogeneous texture (Hidalgo-Ruz et al., 2012; De Witte et al., 2014). The limitation of the identification process was that microplastics smaller than 0.1 mm (100 µm) were hard to observe under the microscope. Finally, the representative microplastic samples were identified polymer type by using micro-Fourier transform infrared (µ-FTIR) spectroscopy LUMOS II at the Professor Aroon Sorathesn Center of Excellence in Environmental Engineering, Faculty of Engineering, Chulalongkorn University.
2.5 Pollution load index
To understand the contamination level in core sediment at each site from Mae Klong River estuary, an integrated pollution load index (PLI) was calculated based on Tomlinson et al. (1980). The PLI at each site is related to microplastic concentration factor (CFi) as given below:
At each site, CFi is the quotient of the microplastic concentration at surface (top 5 cm) (Ci) and the background microplastic concentration (Coi). The microplastic concentration at the bottom of the sediment core (bottom 5 cm) was considered as a background value.
3 Results
3.1 Sediment analysis
Sediment from each consecutive 5-cm depth interval was measured using a laser diffraction machine (Malvern 3,000). The sediment sample from the CP1 site (Queen Sirikit Park) had high concentrations of silt (0.002–0.05 mm) and sand (0.06–2.0 mm), whereas the sample from the S1 site (the Mae Klong River mouth) was silt dominated. The average grain size from each 5-cm depth interval ranged from 38.9 to 166.0 and 21.4–65.7 µm in the CP1 and S1 sites, respectively. The lithostratigraphy and grain size proportion of the sediment cores are shown in Figure 2. LOI was analyzed by combusting the sediment sample from each consecutive 2-cm interval. LOI reflects the water, organic, and carbonate contents contained in the sediment sample. Lithostratigraphy and grain size proportion of CP1 core (Figures 3A,B) are performed with microplastic abundance graph and LOI percentage graph in Figure 3. The LOI value in the 142-cm core from the CP1 site was high at the top of the core and had a slightly decreasing trend from top to bottom of the core (Figure 3D). The result showed that the levels of organic substances contained at the top of the core sample were higher than that at the bottom of the core. Meanwhile, the LOI value had no difference in the 52-cm core from S1. Organic matter in each depth was equally accumulated at the S1 site.
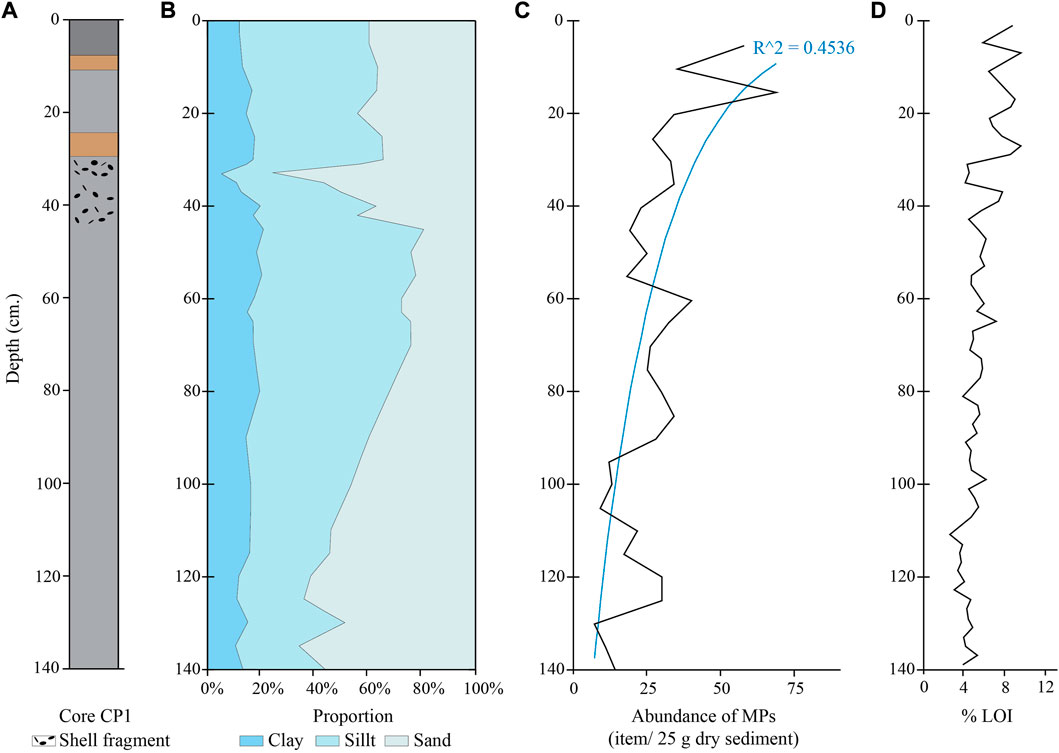
FIGURE 3. Sediment core CP1: (A) lithostratigraphy of core CP1; (B) percentage proportions of the size of sediment in the CP1 core; (C) microplastic abundance in the CP1 core; (D) LOI percentage of sediment in the CP1 core.
3.2 Microplastic analysis
3.2.1 Microplastic abundance
Microplastics were found in almost the entire depth length of the CP1 and S1 core samples. A total of 794 and 63 microplastics were identified in the CP1 and S1 cores, respectively. Microplastics in each 1-cm depth interval in the sediment cores ranged from 0 to 5200 items per kilogram of sediment, with an average of 1102.1 items per kilogram for the CP1 site and from 0 to 1400 items per kilogram in dry sediment, with an average of 426.9 items per kilogram for the S1 site. The microplastic abundance decreased with increasing sediment depth in the CP1 core; conversely, the abundance of microplastics in each depth had no trend in the S1 core. In addition, pollution load index (PLI) was evaluated by microplastic concentration at each site. The PLI values of CP1 and S1 sites were 2.04 and 1.22, respectively.
3.2.2 Characteristics of the microplastics
Four different shapes of microplastics (i.e., fiber, fragment, stick, and plate) were observed under a microscope. Examples of the microplastics found in this study are shown in Figure 4. At Queen Sirikit Park (CP1 site), most microplastics were in fiber shape (92%), followed by fragment (5%) and stick (3%), respectively. The plate shape was not found at the CP1 site. At the Mae Klong River mouth (S1 site), the common shape was also fiber (82%). The other shapes were fragment (11%), plate (4%), and stick (3%), respectively (Figure 5). Meanwhile, fiber was the only shape found at the deeper part of the CP1 core (118- to 142-cm depth).
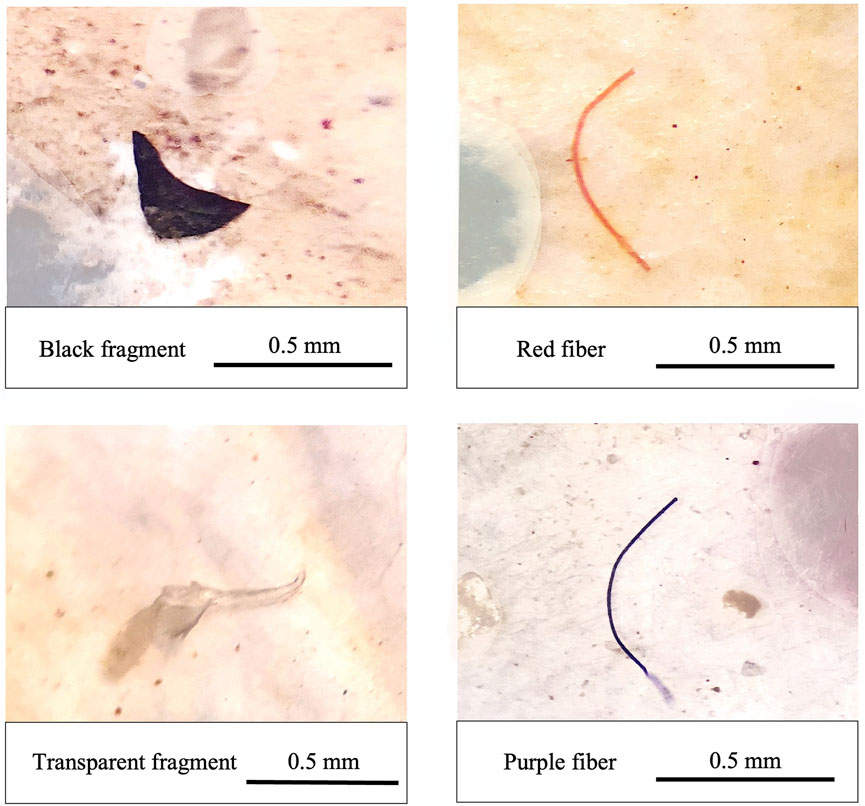
FIGURE 4. Examples of microplastics found in this study (from the top left in the clockwise direction): black fragment, red fiber, purple fiber, and transparent fiber.
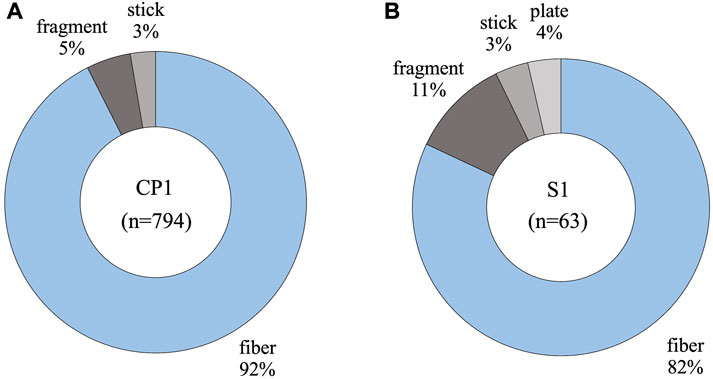
FIGURE 5. Shapes of microplastics in the sediment cores. Percentage proportions of shape categories of microplastics in (A) the CP1 sample and (B) the S1 sample.
For color identification, multiple microplastic colors were detected, including black, colorless, green, red, white, and brown. The CP1 site had all these colors, whereas the S1 site had all colors except brown. The most prevalent colors from the two sediment cores were equally black and colorless.
The total microplastics found in this study were generally 1.0–5.0 mm in size. Microplastics with sizes ranging from 0.1 to 1.0 mm (62%) and 1.0–5.0 mm (38%) were found at the CP1 site. As in the S1 site, microplastics 0.1–1 mm in length (55%) constituted a dominant proportion. The residual part comprised microplastics 0.1–1 mm in length (45%) (Figure 6). Microplastics in the CP1 site accumulated with silt–sand sediment size (0.002–2.0 mm), whereas the S1 site had more silt-size sediment and a smaller microplastic proportion (0.1–1.0 mm) than that in the CP1 site (Figure 6).
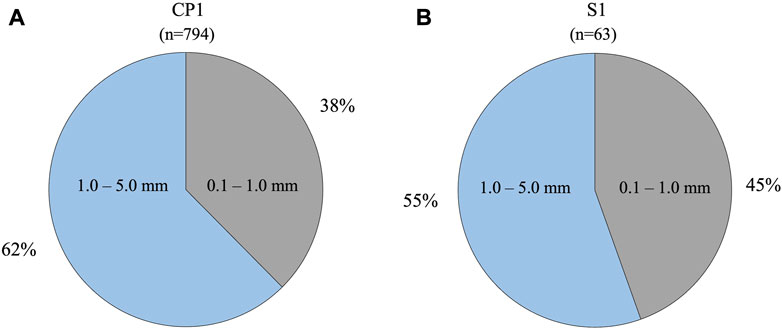
FIGURE 6. Sizes of microplastics in the sediment cores: Percentage proportions of microplastic size in (A) the CP1 sample and (B) in the S1 sample.
3.2.3 Polymer type of microplastics
The µ-FTIR analysis analyzed a total of 38 suspected particles. The result show that 30 samples were indicated as plastic and eight samples were non-plastic including natural dyes and cotton. Consequently, the particles with the same characteristic as non-plastic were deducted from the total number of microplastic. As the spectra of µ-FTIR can analyze the surface of the sample, coatings or contaminations on the surface can influence the spectra. Moreover, the fiber shape mostly found in this study was pretty hard to analyze because of the limited area for detection. The polymer types of the microplastics from the two mangrove sediment cores consisted of rayon, polyester (PES), polyamide (PA), polyethylene (PE), polybutylene terephthalate (PBT), polypropylene (PP), and polycarbonate (PC). An example of a polymer type spectrum of these microplastics is shown in Figure 7. The fiber was detected in various commercial names, including rayon, olefin, spandex, and nylon, which differed depending on the polymer type. In addition, this study detected other microparticles that contaminated sediments, such as pigment, tire rubber, paint, dyes, and flame retardant material. An example of a microparticle’s spectrum is shown in Figure 8.
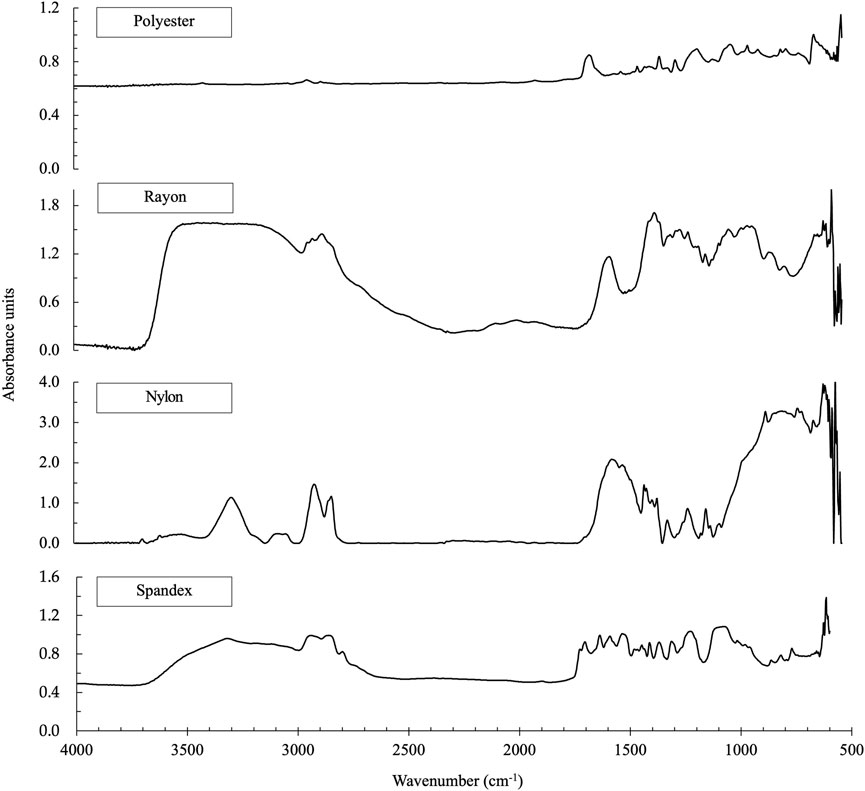
FIGURE 7. Polymer type spectrum from the FTIR analysis in this study. The FTIR spectra show microplastic types (e.g., polyester, rayon, nylon, and spandex).
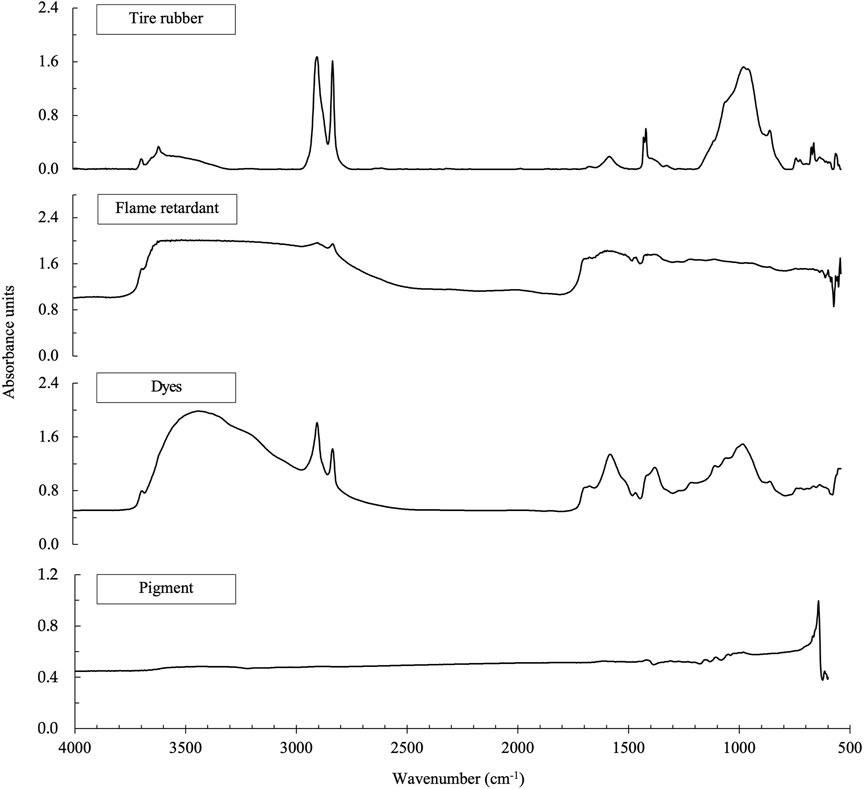
FIGURE 8. FTIR spectra showing types of micromaterials found contaminating the sediment sample from this study (e.g., tire rubber, flame retardant, dyes, and pigment).
4 Discussion
The 142-cm core from the CP1 site and the 52-cm core from the S1 site were analyzed in detail. The results demonstrated that microplastics polluted the mangrove sediment even at a 142-cm depth. A comparison of the findings from this study with those of previous studies in selected regions is shown in Table 1. The microplastic abundance in the mangrove sediment cores from the Samut Songkhram province was approximately equal to those in Tokyo Bay, Japan, and the Red River Delta, Vietnam. Meanwhile, the abundance of microplastics in this study was higher than that in other areas in Thailand. Microplastic accumulation and distribution are influenced by environmental parameters such as geography, location, hydrodynamics, environmental pressure, and time (Das, 2016). Because the mangrove area where the CP1 and S1 sites are located is dominated by Pneumatophores (respiratory roots), which enhance the deposition of plastic particles on sediment, the mangrove sediment is a suitable plastic sink (Martin et al., 2020).
The number of microplastics detected at every layer from the S1 site was lower than that detected in CP1. The difference in location between the CP1 and S1 sites is significant (p < 0.01) (Mann–Whitney U test). Geomorphologically, S1 is located at the river mouth and is thus tide-dominated, whereas CP1 is located near the shoreline and is under coastal protection behind bamboo walls (see Figure 1). Thus, CP1, located at a low-wave-energy area, is a more favorable sink for particles and microplastics (Lo et al., 2018).
The sedimentary grain size analysis showed that the particle size of the CP1 and S1 sites ranged from very coarse silt to fine sand and from coarse silt to very fine sand, respectively. Consequently, the sediment samples from S1 had a grain size finer than that of the sediments from CP1. The environment at the S1 site was suitable for silt domination, and fine-grained sediment has accumulated in the Mae Klong River mouth. Meanwhile, estuarine environments accumulate various ranges of sediment sizes, but finer grains dominate most estuaries (Das, 2016). In addition, there were significant changes in the abundance of microplastics and the particle sizes of the sediments from the two core samples, with a significance of 0.022 (p < 0.05) (Pearson correlation). Moreover, there was a linear relationship between the number of microplastics and the grain sizes of the sediment.
The abundance of microplastics negatively decayed exponentially with deeper depths of sediment core CP1 (r = 0.6734; p < 0.05). The trend is shown in Figure 3C. This relation was also found in the sediment profile in Kuwait Bay (Aba et al., 2014) and the United Kingdom’s continental shelf and slope (Kukkola et al., 2022). The results concluded that the number of microplastic accumulations decreased with increasing sediment depth. Accordingly, the trends of plastic consumption from the past and microplastic accumulation in the core were in the same direction. The pollution load index was normally evaluated the level of pollution in each area. According to this study, the PLI value is applied to evaluate the contamination level in microplastic at each depth in core sediment. The PLI value from CP1 site (2.04) had greater than S1 site (1.22) which these PLI values were less than 10. According to the study on risk assessment by Xu et al. (2018), Hazard level at Mae Klong River estuary indicated Hazard level I. This result may imply that the microplastic contamination from past to present has been polluted (Tomlinson et al., 1980).
The microplastics found in this study were mostly in fiber form, as in previous studies in mangrove core sediments in southern Thailand, Hangzhou Bay, China, and Northern Vietnam (Li et al., 2020a; Luu Viet et al., 2021; Pradit et al., 2022). Moreover, many previous studies also suggested that the fiber type is detected as a major shape of microplastics in mangrove environments (biota: (Hastuti et al., 2019; Klangnurak and Chunniyom, 2020), sediments: (Mohamed Nor and Obbard, 2014; Wang et al., 2020; Zuo et al., 2020)). Synthetic fiber is usually used for clothing, carpets, and various other products made from polymer-based materials such as nylon (PA), spandex (polyether–polyurea copolymer), and rayon (generated cellulose) (Loasby, 1951). Synthetic fiber can release microfibers into the environment during its manufacturing or washing process (Šaravanja et al., 2022). With microfibers dumped into wastewater, some microfibers slip through water filtration systems and finally enter the environment (Vassilenko et al., 2021).
Accordingly, the microplastics found in this study can be implied as sourced from households around the study area. Besides the microplastics found in mangrove sediment cores from Pattani and Songkhla, southern Thailand, rubber and paint have been reported (Pradit et al., 2022). Other synthetic particles were also discovered associated with plastic polymers in this study, and the µ-FTIR analysis identified compounds of small particles, tire rubber, pigment, paint, dyes, and flame retardant. Currently, tire rubber is made from a mixture of natural and synthetic rubbers. Wear and tear from car tires can generate tire wear particles recognized as microplastics resulting from the mechanical abrasion between tires and road surfaces (Kole et al., 2017; Wagner et al., 2018). Meanwhile, pigments, dyes, paints, and flame retardants are normally used in coating materials or as additives in polymers to improve the polymers’ properties (Ambrogi et al., 2017). According to a study in the United Kingdom, synthetic dyes were found contained in fiber (Turner et al., 2019). Moreover, paint partially made from plastic polymer contribute microplastics into the ocean and waterways. Plastic leakage from paint is heavier than other sources of microplastic leakage (textile fibers and tire dust) (Paruta et al., 2022). The size of the synthetic materials in this study (tire rubber, pigment, paint, dyes, and flame retardant) was less than 5 mm. Synthesis materials and chemicals also play a role in microparticle contamination in the environment.
The 1950s was the period of widespread plastic use (Chalmin, 2019). In this regard, many studies have revealed long-term evidence records of microplastic accumulation in sediments. For example, dated sediment cores from Kuwait represented about 60 years of record with a 59-cm sediment depth. Microplastics were detected at the bottom of the core since 1951 (Aba et al., 2014). Similarly, microplastic contamination started in the 1950s and increased toward the surface layer in a dated sediment core from Tokyo Bay, Japan (Matsuguma et al., 2017). In the Gulf of Thailand, microplastics were detected at 10- to 12-cm depths in an undated sediment core sampling near the Chao Phraya River, but the deeper part of the core had no microplastics (Mohamed Nor and Obbard, 2014). Moreover, the notable core sampling 8 km away from the Mae Klong River mouth only found microplastics at the top sediment layer (0–6 cm) (Matsuguma et al., 2017).
The core samples from this study were not dated, but the data from the sediment record in the Klong Khon subdistrict, Samut Songkhram province, provided a sedimentation rate of 0.6 cm per year using OxCal v4.10 (Punwong et al., 2018). According to evidence from the CP1 core, the 33- to 42-cm depths were filled with sand-size grains and shell fragments that may have been interrupted by a strong event. The strong event that brought coarse-grain deposition in this mangrove area was assumed to be typhoon Vae, which attacked the upper Gulf of Thailand in 1962 (Suphat, 2007). The depth from the top to the marked layer (33–42 cm; see Figure 3) and the period of occurrence of the Vae typhoon were calculated, and the rate of sedimentation was approximately 0.57 cm per year. This study’s result is similar to the sedimentation rate at the Klong Khon subdistrict (Punwong et al., 2018) located west of the Mae Klong River, Samut Songkhram province.
Microplastics found in the deep layers may have accumulated before the industrial age in the 1950s. According to a previous study in Turkey, microplastics contaminated estuary sediments before the 1950s (Belivermiş et al., 2021). In this study, microplastics found in the deep layers are supposed to be from microplastic infiltration (Figure 9). Microplastic infiltration, which transports microplastics into deeper areas, is affected by various factors, such as microplastic properties, soil texture, current flow, and biota disruption (Dong et al., 2022; Guo et al., 2022). Accordingly, tidal flat areas are influenced by tides and consist of unconsolidated sediments that typically have high porosity (Semeniuk, 2005). Thus, in this context, microplastics move inside soil cracks or are transported from surfaces to deep layers through leaching or bioturbation (Li et al., 2020b). Moreover, as mangrove environments have a great diversity of biota, including aerial root plants and benthic organisms, microplastics are transported into deeper sediment layers by benthic invertebrates (Näkki et al., 2017). Moreover, microplastics that contaminate mangrove sediments may be ingested by organisms in this area. Many studies revealed that microplastics were found in living organisms in mangrove ecosystems (Hamid et al., 2020; Zhang et al., 2021).
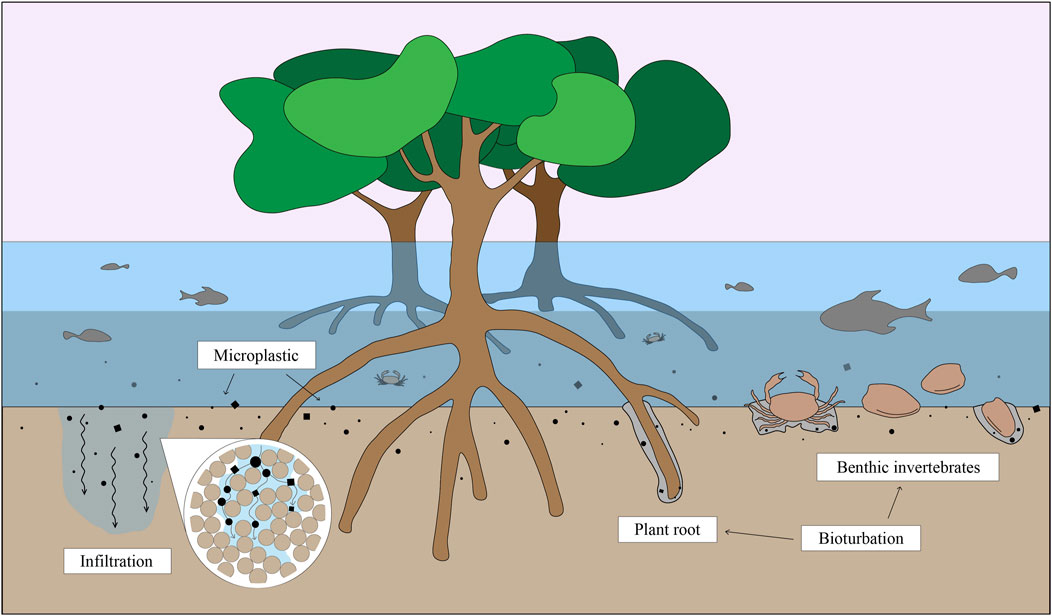
FIGURE 9. Graphic showing how bioturbation transports microplastics deep into the mangrove environment.
5 Conclusion
Microplastics were more abundant in the CP1 core possibly because of the geomorphology of the shoreline area, given that the S1 core is located in the Mae Klong River mouth area. The microplastic abundance corresponded to the sediment size distribution and location related to transportation energy. Microplastics were found at a 142-cm depth and have accumulated in deep sediment layers in association with microplastic infiltration and biota distribution. The abundance of microplastics increased from the deep toward the surface. This trend is expected to rise in the future. Microplastic types in this area were implied as due to human activity—waste from households, daily products, and fishing. As mangrove ecosystems are the habitat of diverse species, microplastics in these areas may affect living organisms. Thus, microplastic pollution in mangrove ecosystems should be a great concern.
Data availability statement
The original contributions presented in the study are included in the article/Supplementary Material, further inquiries can be directed to the corresponding author.
Author contributions
PC contributed to data analysis and writing—original draft preparation. TA and SP contributed to visualization and conceptualization. TA, SP, and AJ also contributed to writing—review and editing.
Funding
This Research is funded by Thailand Science research and Innovation Fund Chulalongkorn University CU_FRB65_dis (15)_103_23_33.
Acknowledgments
We would like to thank the Department of Geology, Faculty of Science, Chulalongkorn University for support in field and materials used for experiments. We would like to appreciate Assoc. Sakonvan Chawchai for guideline about microplastic research.
Conflict of interest
The authors declare that the research was conducted in the absence of any commercial or financial relationships that could be construed as a potential conflict of interest.
Publisher’s note
All claims expressed in this article are solely those of the authors and do not necessarily represent those of their affiliated organizations, or those of the publisher, the editors and the reviewers. Any product that may be evaluated in this article, or claim that may be made by its manufacturer, is not guaranteed or endorsed by the publisher.
References
Aba, A., Uddin, S., Bahbahani, M., and Al-Ghadban, A. (2014). Radiometric dating of sediment records in Kuwait’s marine area. J. Radioanalytical Nucl. Chem. 301 (1), 247–255. doi:10.1007/s10967-014-3140-z
Abel, S. M., Primpke, S., Wu, F., Brandt, A., and Gerdts, G. (2022). Human footprints at hadal depths: Interlayer and intralayer comparison of sediment cores from the Kuril Kamchatka Trench. Sci. Total Environ. 838, 156035. doi:10.1016/j.scitotenv.2022.156035
Ambrogi, V., Carfagna, C., Cerruti, P., and Marturano, V. (2017). “4—additives in polymers,” in Modification of polymer properties. Editors C. F. Jasso-Gastinel, and J. M. Kenny (William Andrew Publishing), 87–108.
Asadi, M., Ritonga, Y., Yona, D., and Hertika, A. (2019). Vertical Distribution of Microplastics in Coastal Sediments of Bama Resort, Baluran National Park, Indonesia. Nat. Environ. Pollut. Technol. 18 (4), 1169–1176.
Belivermiş, M., Kılıç, Ö., Sezer, N., Sıkdokur, E., Güngör, N. D., and Altuğ, G. (2021). Microplastic inventory in sediment profile: A case study of golden horn estuary, sea of marmara. Mar. Pollut. Bull. 173, 113117. doi:10.1016/j.marpolbul.2021.113117
Chalmin, P. (2019). The history of plastics: From the capitol to the tarpeian rock. Field Actions Sci. Rep. J. field actions 2019, 6–11.
Claessens, M., Meester, S. D., Landuyt, L. V., Clerck, K. D., and Janssen, C. R. (2011). Occurrence and distribution of microplastics in marine sediments along the Belgian coast. Mar. Pollut. Bull. 62 (10), 2199–2204. doi:10.1016/j.marpolbul.2011.06.030
Das, G. K. (2016). “Sediment grain size,” in Encyclopedia of estuaries. Editor M. J. Kennish (Dordrecht: Springer Netherlands), 555–558.
De Witte, B., Devriese, L., Bekaert, K., Hoffman, S., Vandermeersch, G., Cooreman, K., et al. (2014). Quality assessment of the blue mussel (Mytilus edulis): Comparison between commercial and wild types. Mar. Pollut. Bull. 85 (1), 146–155. doi:10.1016/j.marpolbul.2014.06.006
Deng, Y., Zhang, Y., Lemos, B., and Ren, H. (2017). Tissue accumulation of microplastics in mice and biomarker responses suggest widespread health risks of exposure. Sci. Rep. 7 (1), 46687. doi:10.1038/srep46687
Department of Marine and Coastal Resources (2018). Information on marine and coastal resources in Samut Songkhram province information and surveying Technology for marine and coastal resources division. Available: www.dmcr.go.th/detailLib/3763 (Accessed March 3, 2022).
Ding, J., Sun, C., He, C., Li, J., Ju, P., and Li, F. (2021). Microplastics in four bivalve species and basis for using bivalves as bioindicators of microplastic pollution. Sci. Total Environ. 782, 146830. doi:10.1016/j.scitotenv.2021.146830
Dong, M., Luo, Z., Jiang, Q., Xing, X., Zhang, Q., and Sun, Y. (2020). The rapid increases in microplastics in urban lake sediments. Sci. Rep. 10 (1), 848. doi:10.1038/s41598-020-57933-8
Dong, S., Yu, Z., Huang, J., and Gao, B. (2022). “Chapter 9 - fate and transport of microplastics in soils and groundwater,” in Emerging contaminants in soil and groundwater systems. Editor B. Gao (Amsterdam, Netherlands: Elsevier), 301–329.
Duan, J., Han, J., Zhou, H., Lau, Y. L., An, W., Wei, P., et al. (2020). Development of a digestion method for determining microplastic pollution in vegetal-rich clayey mangrove sediments. Sci. Total Environ. 707, 136030. doi:10.1016/j.scitotenv.2019.136030
Fan, Y., Zheng, K., Zhu, Z., Chen, G., and Peng, X. (2019). Distribution, sedimentary record, and persistence of microplastics in the Pearl River catchment, China. Environ. Pollut. 251, 862–870. doi:10.1016/j.envpol.2019.05.056
Gallo, F., Fossi, C., Weber, R., Santillo, D., Sousa, J., Ingram, I., et al. (2018). Marine litter plastics and microplastics and their toxic chemicals components: The need for urgent preventive measures. Environ. Sci. Eur. 30 (1), 13. doi:10.1186/s12302-018-0139-z
Gilbert, M. (2017). “Chapter 1 - plastics materials: Introduction and historical development,” in Brydson's plastics materials. Editor M. Gilbert (Oxford, United Kingdom: Butterworth-Heinemann), 1–18.
Guo, Z., Li, P., Yang, X., Wang, Z., Lu, B., Chen, W., et al. (2022). Soil texture is an important factor determining how microplastics affect soil hydraulic characteristics. Environ. Int. 165, 107293. doi:10.1016/j.envint.2022.107293
Hamid, B. S. F., Jia, W., and Zakaria, R. B. M. (2020). Microplastics abundance and uptake by meretrix lyrata (hard clam) in mangrove forest. J. Eng. Technol. Sci. 52, 436–448. doi:10.5614/j.eng.technol.sci.2020.52.3.10
Hastuti, A. Y. U., Lumbanbatu, D. T. F., and Wardiatno, Y. (2019). The presence of microplastics in the digestive tract of commercial fishes off Pantai Indah Kapuk coast, Jakarta, Indonesia. Biodiversitas 20, 1233–1242. doi:10.13057/biodiv/d200513
Heiri, O., Lotter, A. F., and Lemcke, G. (2001). Loss on ignition as a method for estimating organic and carbonate content in sediments: Reproducibility and comparability of results. J. Paleolimnol. 25 (1), 101–110. doi:10.1023/A:1008119611481
Hidalgo-Ruz, V., Gutow, L., Thompson, R. C., and Thiel, M. (2012). Microplastics in the marine environment: A review of the methods used for identification and quantification. Environ. Sci. Technol. 46 (6), 3060–3075. doi:10.1021/es2031505
Jambeck Jenna, R., Geyer, R., Wilcox, C., Siegler Theodore, R., Perryman, M., Andrady, A., et al. (2015). Plastic waste inputs from land into the ocean. Science 347 (6223), 768–771. doi:10.1126/science.1260352
Kanhai, L. D. K., Gardfeldt, K., Krumpen, T., Thompson, R. C., and O’Connor, I. (2020). Microplastics in sea ice and seawater beneath ice floes from the Arctic Ocean. Sci. Rep. 10 (1), 5004. doi:10.1038/s41598-020-61948-6
Kelly, A., Lannuzel, D., Rodemann, T., Meiners, K. M., and Auman, H. (2020). Microplastic contamination in east Antarctic sea ice. Mar. Pollut. Bull. 154, 111130. doi:10.1016/j.marpolbul.2020.111130
Klangnurak, W., and Chunniyom, S. (2020). Screening for microplastics in marine fish of Thailand: The accumulation of microplastics in the gastrointestinal tract of different foraging preferences. Environ. Sci. Pollut. Res. 27 (21), 27161–27168. doi:10.1007/s11356-020-09147-8
Kole, P. J., Löhr, A. J., Van Belleghem, F., and Ragas, A. M. J. (2017). Wear and tear of tyres: A stealthy source of microplastics in the environment. Int. J. Environ. Res. Public Health 14 (10), 1265. doi:10.3390/ijerph14101265
Kukkola, A. T., Senior, G., Maes, T., Silburn, B., Bakir, A., Kröger, S., et al. (2022). A large-scale study of microplastic abundance in sediment cores from the UK continental shelf and slope. Mar. Pollut. Bull. 178, 113554. doi:10.1016/j.marpolbul.2022.113554
Li, J., Huang, W., Xu, Y., Jin, A., Zhang, D., and Zhang, C. (2020a). Microplastics in sediment cores as indicators of temporal trends in microplastic pollution in Andong salt marsh, Hangzhou Bay, China. Regional Stud. Mar. Sci. 35, 101149. doi:10.1016/j.rsma.2020.101149
Li, J., Song, Y., and Cai, Y. (2020b). Focus topics on microplastics in soil: Analytical methods, occurrence, transport, and ecological risks. Environ. Pollut. 257, 113570. doi:10.1016/j.envpol.2019.113570
Lo, H. S., Xu, X., Wong, C. Y., and Cheung, S. G. (2018). Comparisons of microplastic pollution between mudflats and sandy beaches in Hong Kong. Environ. Pollut. (Barking, Essex 1987) 236, 208–217. doi:10.1016/j.envpol.2018.01.031
Loasby, G. (1951). The development of the synthetic fibres. J. Text. Inst. Proc. 42 (8), P411–P441. doi:10.1080/19447015108663852
Luu Viet, D., Huu Duc, T., Linh, L., Ly, T., Duong, H., and Hao, N. (2021). Depth profiles of microplastics in sediment cores from two mangrove forests in northern Vietnam. J. Mar. Sci. Eng. 9, 1381. doi:10.3390/jmse9121381
Martin, C., Baalkhuyur, V., Saderne, V., Cusack, M., and Almahasheer, H. (2020). Exponential increase of plastic burial in mangrove sediments as a major plastic sink. Sci. Adv. 6, 5593. doi:10.1126/sciadv.aaz5593
Matsuguma, Y., Takada, H., Kumata, H., Kanke, H., Sakurai, S., Suzuki, T., et al. (2017). Microplastics in sediment cores from asia and Africa as indicators of temporal trends in plastic pollution. Archives Environ. Contam. Toxicol. 73, 230–239. doi:10.1007/s00244-017-0414-9
Meijer, L. J. J., van Emmerik, T., van der Ent, R., Schmidt, C., and Lebreton, L. (2021). More than 1000 rivers account for 80% of global riverine plastic emissions into the ocean. Sci. Adv. 7, eaaz5803. doi:10.1126/sciadv.aaz5803
Mohamed Nor, N. H., and Obbard, J. P. (2014). Microplastics in Singapore’s coastal mangrove ecosystems. Mar. Pollut. Bull. 79 (1), 278–283. doi:10.1016/j.marpolbul.2013.11.025
Näkki, P., Setälä, O., and Lehtiniemi, M. (2017). Bioturbation transports secondary microplastics to deeper layers in soft marine sediments of the northern Baltic Sea. Mar. Pollut. Bull. 119 (1), 255–261. doi:10.1016/j.marpolbul.2017.03.065
Paruta, P., Pucino, M., and Boucher, J. (2022). Plastic paints the environment. Lausanne, Switzerland: EA-Environmental Action).
Pollution Control Department (2019). Booklet on Thailand state of pollution 2018. Bangkok: S.Mongkon Press Limited Partnership. Available: https://www.pcd.go.th/publication/3657 (Accessed March 3, 2022).
Pradit, S., Noppradit, P., Loh, P. S., Nitiratsuwan, T., Le, T. P., Oeurng, C., et al. (2022). The occurrence of microplastics in sediment cores from two mangrove areas in southern Thailand. J. Mar. Sci. Eng. 10 (3), 418. doi:10.3390/jmse10030418
Pumijumnong, N. (2014). “Mangrove forests in Thailand,” in Mangrove ecosystems of asia: Status, challenges and management strategies. Editors I. Faridah-Hanum, A. Latiff, K. R. Hakeem, and M. Ozturk (New York, NY: Springer New York), 61–79.
Punwong, P., Sritrairat, S., Selby, K., Marchant, R., Pumijumnong, N., and Traiperm, P. (2018). An 800 year record of mangrove dynamics and human activities in the upper Gulf of Thailand. Veg. Hist. Archaeobotany 27 (4), 535–549. doi:10.1007/s00334-017-0651-x
Qiao, S., Shi, X., Fang, X., Liu, S., Kornkanitnan, N., Gao, J., et al. (2015). Heavy metal and clay mineral analyses in the sediments of Upper Gulf of Thailand and their implications on sedimentary provenance and dispersion pattern. J. Asian Earth Sci. 114, 488–496. doi:10.1016/j.jseaes.2015.04.043
Šaravanja, A., Pušić, T., and Dekanić, T. (2022). Microplastics in wastewater by washing polyester fabrics. Mater. (Basel) 15 (7), 2683. doi:10.3390/ma15072683
Semeniuk, V. (2005). “Tidal flats,” in Encyclopedia of coastal science. Editor M. L. Schwartz (Dordrecht: Springer Netherlands), 965–975.
Suphat, V. (2007). Impacts of typhoon Vae and linda on wind waves in the upper gulf of Thailand and east coast. Songklanakarin J. Sci. Technol. 29, 1199–1216.
Tomlinson, D., Wilson, J., Harris, C. R., and Jeffrey, D. W. (1980). Problems in the assessment of heavy-metal levels in estuaries and the formation of a pollution index. Helgoländer Meeresunters. 33, 566–575. doi:10.1007/BF02414780
Turner, S., Horton, A. A., Rose, N. L., and Hall, C. (2019). A temporal sediment record of microplastics in an urban lake, London, UK. J. Paleolimnol. 61 (4), 449–462. doi:10.1007/s10933-019-00071-7
Van Cauwenberghe, L., Vanreusel, A., Mees, J., and Janssen, C. R. (2013). Microplastic pollution in deep-sea sediments. Environ. Pollut. 182, 495–499. doi:10.1016/j.envpol.2013.08.013
Vassilenko, E., Watkins, M. J., Chastain, S., Mertens, J., Posacka, A., Patankar, S., et al. (2021). Domestic laundry and microfiber pollution: Exploring fiber shedding from consumer apparel textiles. PloS one 16, e0250346. doi:10.1371/journal.pone.0250346
Wagner, S., Hüffer, T., Klöckner, P., Wehrhahn, M., Hofmann, T., and Reemtsma, T. (2018). Tire wear particles in the aquatic environment - a review on generation, analysis, occurrence, fate and effects. Water Res. 139, 83–100. doi:10.1016/j.watres.2018.03.051
Wang, Y., Zou, X., Peng, C., Qiao, S., Wang, T., Yu, W., et al. (2020). Occurrence and distribution of microplastics in surface sediments from the Gulf of Thailand. Mar. Pollut. Bull. 152, 110916. doi:10.1016/j.marpolbul.2020.110916
Wright, S. L., Thompson, R. C., and Galloway, T. S. (2013). The physical impacts of microplastics on marine organisms: A review. Environ. Pollut. 178, 483–492. doi:10.1016/j.envpol.2013.02.031
Xu, P., Peng, G., Su, L., Gao, Y., Gao, L., and Li, D. (2018). Microplastic risk assessment in surface waters: A case study in the changjiang estuary, China. Mar. Pollut. Bull. 133, 647–654. doi:10.1016/j.marpolbul.2018.06.020
Zhang, K., Shi, H., Peng, J., Wang, Y., Xiong, X., Wu, C., et al. (2018). Microplastic pollution in China's inland water systems: A review of findings, methods, characteristics, effects, and management. Sci. Total Environ. 630, 1641–1653. doi:10.1016/j.scitotenv.2018.02.300
Zhang, S., Sun, Y., Liu, B., and Li, R. (2021). Full size microplastics in crab and fish collected from the mangrove wetland of Beibu Gulf: Evidences from Raman Tweezers (1–20 μm) and spectroscopy (20–5000 μm). Sci. Total Environ. 759, 143504. doi:10.1016/j.scitotenv.2020.143504
Zheng, Y., Li, J., Cao, W., Jiang, F., Zhao, C., Ding, H., et al. (2020). Vertical distribution of microplastics in bay sediment reflecting effects of sedimentation dynamics and anthropogenic activities. Mar. Pollut. Bull. 152, 110885. doi:10.1016/j.marpolbul.2020.110885
Keywords: microplastic, mangrove, core sample, organic sediments, μ-FTIR
Citation: Chaisanguansuk P, Phantuwongraj S, Jirapinyakul A and Assawincharoenkij T (2023) Preliminary study on microplastic abundance in mangrove sediment cores at Mae Klong River, upper Gulf of Thailand. Front. Environ. Sci. 11:1134988. doi: 10.3389/fenvs.2023.1134988
Received: 31 December 2022; Accepted: 13 March 2023;
Published: 23 March 2023.
Edited by:
Xiaofeng Wen, Changsha University of Science and Technology, ChinaCopyright © 2023 Chaisanguansuk, Phantuwongraj, Jirapinyakul and Assawincharoenkij. This is an open-access article distributed under the terms of the Creative Commons Attribution License (CC BY). The use, distribution or reproduction in other forums is permitted, provided the original author(s) and the copyright owner(s) are credited and that the original publication in this journal is cited, in accordance with accepted academic practice. No use, distribution or reproduction is permitted which does not comply with these terms.
*Correspondence: Thitiphan Assawincharoenkij, dGhpdGlwaGFuLmFAY2h1bGEuYWMudGg=