- 1Department of Civil and Environmental Engineering, University of Nebraska—Lincoln, Lincoln, NE, United States
- 2Department of Agronomy and Horticulture, University of Nebraska—Lincoln, Lincoln, NE, United States
- 3Nebraska Department of Environment and Energy, Lincoln, NieE, United States
Conservation practices such as crop rotation, filter strips, and constructed wetlands are nature-based approaches intended to safeguard natural resources in agricultural landscapes. In this study, we reviewed the literature on how conservation practices, both at watershed and field scales, have been proven to subdue flood peaks, surface runoff, soil erosion, sediment transport, and nutrient loss. We classified different conservation practices based on the mode of their application (i.e., in-field, edge-of-field, and structural practices) and described what prior research efforts have concluded about the efficacy of different practices. At the field scale, practices such as reduced or no-till farming, grassed waterways, and creation of wetlands significantly reduced the peak flow. Similarly, water quality was improved with implementation of conservation practices such as using cover crops, filter strips, and managing residue and tillage. The assessment of conservation practices across the literature was found to be challenging as different conservation practices showed a similar response, thus making it complex to assess the individual effect. A wide range of challenges related to the data, modeling/analysis, and management aspects of conservation practices were identified, and recommendations were provided to overcome these challenges.
Highlights
• Conservation practices are found to effectively control peak flows and improve water quality at the field and watershed level in experiments across the globe.
• The effectiveness of conservation practices changes with spatial and temporal variations due to different factors such as weather, soil, and cropping systems.
• Conservation practices were effective in subduing the impacts of climate change. However, their efficacy was lowered with extreme precipitation and increased greenhouse gas concentration.
• The processes by which conservation practices affect flooding and water quality are often similar, which complicates evaluating their efficacy individually.
• Major challenges for assessment found in literature include limited availability of data, inclusion of different conservation practices in models, variability in assessment results, and limitations associated with long-term predictions.
1 Introduction
Around the world, about 12 million hectares of cultivable landmass are abandoned each year due to increased land deterioration, leading to reduced agricultural production (Rickson et al., 2015). This land deterioration is attributed to a wide range of factors, including the rise of surface runoff, soil erosion and degradation, sediment deposition, and nutrient loss, which occur despite increasing awareness about the ecosystem and the implementation of different preventive measures (Heathcote et al., 2013). In this period of competing demands on natural resources due to factors such as population increase, shifting diets, and climate change, understanding how the negative impacts of agricultural production can be reduced through the implementation of different conservation practices has gained considerable attention (Adusumilli and Wang, 2018). Expansion in urban infrastructures and human alteration to natural systems have intensified runoff, making it one of the major challenges in water resource management (Dakhlalla and Parajuli, 2016). Furthermore, the adverse impacts of increased soil erosion and fresh-water scarcity on agricultural production, ecological conservation, and human health are becoming increasingly apparent (O’Connell et al., 2007). Studies suggest a distressing future with the rising population and declining land productivity (Dakhlalla and Parajuli, 2006; Rickson et al., 2015; Adusumilli and Wang, 2018).
Conservation practices can be considered a nature-based solution to counter peak flows, soil erosion, and non-point source pollution. Different attributes that govern the effectiveness of these practices are technical achievability, ecological soundness, financial feasibility, and social acceptance (Herweg and Ludi, 1999). The implementation of conservation practices comes with different challenges for landowners and managers, including limited knowledge and skills, equipment access, and lack of financial assistance. Furthermore, soil characteristics change from region to region, making the implementation of conservation practices highly context-specific (Knowler and Bradshaw, 2007; Lahmar, 2010; Corbeels et al., 2014; Telles et al., 2019).
In this review paper, we focus on the role of different conservation practices in reducing two specific and significant environmental concerns related to agricultural operations—attenuating peak flow and enhancing water quality through reducing soil erosion, sediment transport, and nutrient loss—by reviewing prior research. We also reviewed assessment approaches of these practices followed by challenges and the future scope in the context of data, modeling/analysis, and management. The current review paper follows a snowballing approach (outlined in Figure 1) by first referring to different papers based on diverse themes in the context of the assessment of conservation practices and their influence on flooding and water quality. This paper then furthers the literature review by assessing the various papers cited within the previously mentioned literature. This approach allows the report to be impartial to ideas and approaches included across the agriculture conservation domain.
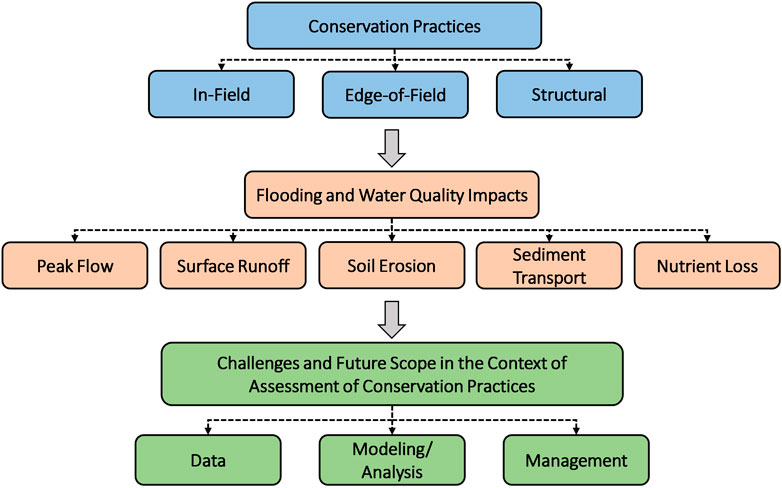
FIGURE 1. Structure of review paper—1) Review of different conservation practices based on mode of implementation, i.e., in-field, edge-of-field, and structural practices. 2) Review of the impact of conservation practices on flooding and water quality. 3) Review of assessment approaches of these practices followed by challenges and the future scope in the context of data, modeling/analysis, and management.
The paper reviews gaps in research and knowledge at a global scale across field and watershed levels to understand how conservation practices can counter flooding conditions and improve water quality. Conservation practices are classified as in-field, edge-of-field, and structural practices to categorize the process by which it influences flooding and water quality. We also study the effects of climate change with respect to conservation practices effectiveness and conclude by examining challenges associated with the assessment of conservation practices and the future scope of the research.
2 Impact of conservation practices in prior studies
The study of the impact of conservation practices at watershed and field scale provides an understanding of the interfaces of the environment, weather conditions, land, runoff, and contaminant transport processes (Sharpley et al., 2002; Renschler and Lee, 2005). Numerous prior studies have reported water quality and quantity problems through both field observation and modeling approaches and have indicated that the application of conservation practices has significantly improved the peak flow condition and water quality (Hu et al., 2007; Her et al., 2016; Muenich et al., 2016; Singh et al., 2018). In context to water quality, one study reported non-point source pollution concerns across the West Fork watershed of the Trinity River Basin in Texas (Santhi et al., 2006). The study found that implementing management practices such as filter strips, nutrient management, manure management, etc., significantly reduced up to 99% of non-point source pollution on farms. In another case, higher nitrate content was found in the Upper Embarras River watershed in East Central Illinois, due to excessive nitrogen application (Hu et al., 2007). The model predicted a reduction in nitrate up to 43% with the implementation of a nutrient management plan. The Upper Big Walnut Creek watershed in Ohio was a similar case. The Environmental Protection Agency had classified it as an impaired watershed with high nutrient and pathogen concentrations (King et al., 2008). Conservation practices such as precision nutrient and drainage water management were implemented to counter this.
Other instances of the impact of conservation practices on agricultural operations and ecosystem restorations include a study which reported on non-point source pollution that resulted in a 95% increase in total nitrate content in the Des Moines River (Schilling and Wolter, 2009). The study found that applying nutrient management plans resulted in a 38% reduction in nitrate load. Agricultural areas surrounding Lake Erie were identified as a source of nutrient loading (soluble P), which turned some parts of Lake Erie into a place of algal bloom (Her et al., 2016; Muenich et al., 2016). Results of this study suggested that even if the application of different fertilizers ceased, some regions might take many years to recover from excessive nutrient contamination. The study reported the benefits of conservation practices such as nutrient management, filter strips, cover crops, crop rotation, and biofuels in improving the scenario. Similarly, Singh et al. (2018) mentioned the increasing concentration of nitrogen and phosphorus leading to hypoxia in the Gulf of Mexico due to excessive fertilizer application and the tile drainage system. Their results highlighted the fact that the application of conservation practices, such as critical area planting, filter strips, irrigation land levelling, and nutrient management reduced sediment concentration up to 80%, and nutrient levels dropped significantly. These cases highlighted the need for research to provide different nature-based solutions to improve water quality.
In the context of flooding, the United States flood management recommendation emphasizes employing non-structural measures to reduce peak flows (De Laney, 1995). Flood risk management is shifting towards new concepts that put emphasis on providing more water space by restoring flood plains (Brémond et al., 2013). In such scenarios, the role of conservation practices becomes crucial. Different authors have reported the potential of conservation practices in attenuating flood peaks and frequency. A study found a reduction in peak flow due to construction of wetlands (De Laney, 1995). The study concluded that 5%–10% of the wetlands area in the watershed could attenuate around 50% of peak floods. Similarly, another research effort reported the effectiveness of conservation practices in reducing the peak flow in the Lower Pearl River watershed in the South Mississippi region (Dakhlalla and Parajuli, 2016). The study also reported this region experienced a destructive flood in 1979, which caused more than $250 million in damage. Likewise, frequent occurrences of flooding were reported in the Yellow River Basin. This basin was listed among the most flood-prone areas in the world (Bai et al., 2016). The study concluded conservation practices such as level terrace, afforestation, grass planting, and check-dam were effective in attenuating the peaks.
3 Conservation practices: A potential solution to the problem
Conservation practices have the potential to play a significant role in reducing peak flow and improving water quality. To capture their response, it is essential to compare pre-conservation practice scenarios and post-conservation practice scenarios (Her et al., 2016; Muenich et al., 2016; Singh et al., 2018). Different authors have reported the effectiveness of applying various conservation practices in their field of interest (Hu et al., 2007; Her et al., 2016; Muenich et al., 2016; Singh et al., 2018). The effectiveness of conservation practices changes based on spatial and temporal scales. The potential factors which control its effectiveness were climate, crop type, biophysical and socioeconomic conditions, and labor input (Herweg and Ludi, 1999; Hatfield and Prueger, 2011; Dakhlalla and Parajuli, 2016).
This review summarizes different results, which can help researchers, farmers, and local stakeholders by providing insight into conservation practices that might be applied in context to existing problems in their regions. We have classified conservation practices to reflect the National Resources Conservation Service (NRCS) definition. They classified them as in-field, edge-of-field, and structural practices as per their mode of implementation (Basche et al., 2020) (Table 1; Table 2,; Table 3).
4 Effectiveness of conservation practices in addressing flooding and water quality
4.1 In the context of flooding
In agricultural land-use management, flood hazard can be defined as the probability of exceeding critical peak discharge, resulting in economic damage and raising food security concerns (O’Connell et al., 2007). Usually, runoff generated from small streams is considered less severe. Still, there is a high chance that these insignificant changes will propagate, resulting in higher runoff at a larger catchment scale. The runoff changes on the local scale need to be monitored, as they can result in flooding. Modern flood management is typically designing a system that prefers allowing water to be stored by restoring flood plains. However, these restorations significantly affect the agricultural areas, ultimately burdening productivity (Brémond et al., 2013).
Prior research has studied the effects of different conservation practices on flooding. Practices referring to reduced or no-till farming methods minimized soil disruption and allowed soil biota and structure to reorient. The presence of soil biota often led to the formation of micro and macro sized pores (Sun et al., 2020; Hovis et al., 2021). These pores at larger scales absorbed the excess water during rainfall, thus delaying the peak flows and improving soil infiltration capacity (De Laney, 1995; Hovis et al., 2021). Practices such as bioretention, vegetated grass strips, and filter strips reduced runoff through accelerating storage, evapotranspiration, and infiltration. Wild and Davis (2009) reported that these practices successfully reduced peak volume by 42%. Dakhlalla and Parajuli (2016) reported grassed waterways were effective in reducing peak flow (up to 8.4%) by increasing the roughness of the surface. Similarly, a study found grassed waterways were effective in attenuating muddy flooding (Evrard et al., 2008). There was a 69% reduction in peak discharge. The study also reported that the efficacy of grassed waterways could be increased with the combination of alternating farming practices, such as reduced tillage.
Studies have also referred to cover crops’ effectiveness in reducing the peak flow during high flood frequency scenarios (Muenich et al., 2016; Antolini et al., 2020). Since these crops were planted once primary harvesting is complete, they were most effective at controlling flood peaks during winters or early springs (Hovis et al., 2021). It was also found that in the post-flooding scenario, applying a cover crop immediately after the soil was dry promotes the growth of microorganisms, which are essential for nutrient cycling (Al-Kaisi, 2019). Wetlands could be one potential solution to counter peak flow problems as it delays flood water movement and reduces downstream water flow. A study identified two types of wetlands, wetland retention basins and forested wetlands, in countering stream velocity and sediment transport (Hovis et al., 2021). The wetland’s location in the watershed significantly impacts its effectiveness in lowering the flood peaks. A global study cited the efficacy of multiple small freshwater marshes at the watershed’s upper reach in reducing the peak flow (Mitsch and Gosselink, 2000). These small multiple units mitigated the chances of wetlands’ destruction from abnormal precipitation. It can also act as a detention basin which can delay and reduce peak flows. Similarly, Ogawa and Male (1983) reported the effectiveness of wetlands when located downstream.
Apart from the practices cited above, this review has identified other practices that have effectively reduced the peak flow (magnitude based on spatial scale at which studies were carried out). The practices included utilizing afforestation (up to 80%) (Huang et al., 2003), detention ponds (up to 6%) (Dakhlalla and Parajuli, 2016), grade stabilization structures (up to 25%) (Kaini et al., 2008), grasslands (40%–45%) (Gerla, 2007), parallel terraces (up to 3.1%) (Dakhlalla and Parajuli, 2016), soil bunds infused with grasses and short trenches (up to 55%) (Sultan et al., 2018), and urban forests (3%–8%) (Lormand, 1988).
Surface Runoff - Surface runoff refers to water movement on the land surface due to excessive precipitation, stormwater, snowmelt, or any other source that is no longer infiltrated in the soil. Excessive surface runoff often results in flooding and is one of the major sources of non-point source pollution (Environmental Protection Agency, 2018). These pollutants can be sediments, pesticides, and nutrients, which heavily affect off-site water bodies (Wallace et al., 2017). The variables that control surface runoff include temperature, precipitation, relative humidity, and wind speed (Kurothe et al., 2014; Adimassu et al., 2017; Luo et al., 2017).
Different conservation practices were found to be effective in reducing surface runoff. Straw mulch was effective in improving the infiltration rate and the cohesive strength against erosive forces (Olkeba et al., 2012). Antolini et al. (2020) found the effectiveness of cover crops in reducing runoff as they provided a protective layer to the surface, which reduced surface runoff and runoff velocity. Conservation practices referring to no-tillage, ridge-farming tillage, and stubble mulch farming tillage effectively reduced surface runoff (Kurothe et al., 2014). The study concluded that no-tillage resulted in the minimum disturbance in soil, which promoted filtration and reduced runoff (up to 16%). Similarly, ridge formation along a contour and tillage along the contour created a barrier to overland flow, which increased the surface detention time and, thus, the infiltration period. This reduced surface runoff by up to 69%.
Farahani et al. (2016) discussed the effectiveness of contour farming in reducing surface runoff. Contour cultivation reduced the runoff by 10% compared to when crops were planted perpendicular to the slope. The study also found that the effectiveness of contour farming was further increased with the combination of a no-tillage system. Bunds were also found to be effective in controlling runoff because of their ability to retain water across the slope. The studies found different soil bunds, such as grade, level, and stone, to reduce runoff by 25.2%, 60.1%, and 34.4%, respectively (Herweg and Ludi, 1999; Araya et al., 2011; Adimassu et al., 2014). Similarly, Blanco-Canqui et al. (2004) discussed the effectiveness of buffer strips in reducing runoff through changing hydrologic pathways, resulting in a higher infiltration period and reduced surface flow. However, studies have observed that buffer strips were only effective for reducing sediment and nutrient load (Chaubey et al., 2010; Wagena and Easton, 2018). Such cases indicated that conservation practice effectiveness changes with weather, soil, and cropping system.
4.2 In the context of water quality
The deterioration in water quality severely impacts living beings, as it adversely affects crop productivity and environmental sustainability (Hurni, 1996; Adimassu et al., 2017). The upcoming generation of farming and landscape requires multifunctional and ecological sustainability (Kassam et al., 2014). In achieving these requirements, conservation practices can play a crucial role (Santhi et al., 2006; Kassam et al., 2014; Adimassu et al., 2017; Singh et al., 2018). The impact of conservation practices on water quality in this review was studied by analyzing their influence on soil erosion, soil sediments, and soil nutrients.
Soil Erosion—Soil erosion refers to the removal of the topmost layer of soil on the earth’s surface. These fine layers have the highest amount of plant nutrients and have a significant concentration of agrochemicals, soil biota, soil carbon, and organic matter. Soil erosion reduces the effective mass and depth of in situ soil, thus reducing the storage capacity of moisture and nutrients (Rickson et al., 2015). Several factors affecting soil erosion are existing vegetative cover, deforestation rate, soil and water conservation measure, population growth, and climate change (Zhao et al., 2013). Soil erosion has caused severe damage to the food production system (Adimassu et al., 2014). It degrades the land quality as it deteriorates soil conditions. Additionally, soil biota, which is severely affected by soil erosion, reduces fertility and infiltration (Pimentel et al., 1995). Different literature has provided their input on how soil erosion affected crop productivity. For instance, Young (1989) found that eroded soil decreased crop productivity by 50%–75%. Similarly, Bakker et al. (2004) found a loss of 4.3%–26.6% productivity per 10 cm in the soil layer.
Studies have stated their findings regarding the effectiveness of different conservation practices at the watershed and field levels. It is important to note that the effectiveness of these practices varies with change in weather, soil, and cropping system. According to a study, the potential conservation practices that can counter the increasing soil erosion rate were crop rotation, strip cropping, grass strips, cover crops, mulches, living mulches, agroforestry, and windbreaks (Pimentel et al., 1995). Other research reported the effectiveness of terracing, afforestation, natural rehabilitation, and check-dams construction in reducing the impact (Zhao et al., 2013). Practice such as residue and tillage management addresses the amount, orientation, and distribution of crop and plant residue. Crops are grown in narrow slots or tilled strips to reduce soil disturbance, thus, reducing erosion (Hengsdijk et al., 2005). Merten et al. (2015) suggested that the presence of residue effectively controlled erosion as it dissipated rain-drop energy, improved infiltration capacity, and increased surface roughness, thus reducing the runoff velocity. Further, the mulching treatment was effective as it could minimize erosion up to 98% (Adimassu et al., 2014). Conservation practices such as graded and level soil bunds and grass strips were also found to be effective in retaining the water, thus minimizing erosion (Herweg and Ludi, 1999). The combination of different practices was found to be effective in countering erosion. Farahani et al. (2016) found the effectiveness of contour farming in reducing erosion when combined with conservation tillage. Likewise, tied ridge and field yard manure effectively reduced soil erosion (Araya et al., 2011). Implementation of these practices can be effective. However, demographic and socioeconomic characteristics are among the factors that influence conservation measures and, subsequently, erosion. Effective planning for applying soil conservation measures, new techniques, and modifications in educational/training programs can effectively increase awareness among the population regarding soil erosion (Ersedo, 2021).
Soil Sediments—The movement of soil sediments can be attributed to soil disturbance. Runoff carries these sediments from a non-point source are one of the major sources of surface water pollution (Muñoz-Carpena et al., 1999). Sediment transport is often interlinked with soil erosion and nutrient loss. Soil erosion on a larger scale result in sediment deposition in water bodies (Vanacker et al., 2003). These sediments are often accompanied by nutrients that degrade water quality and soil health (Zhao et al., 2013).
Although conservation practices have diversified benefits, some were highly effective in controlling sediment loading. Conservation practices such as filter strips, field borders, and grassed waterways effectively trapped sediment movement (Dillaha et al., 1986; Magette et al., 1989; Muenich et al., 2016). These practices reduced sediment transport from runoff through different processes such as filtration, deposition, adsorption, and decomposition (Muñoz-Carpena et al., 1999). These processes reduced flow resulting in reduced sediment transport capacity. Studies have found that these practices, on average, can reduce sediment transport by up to 40%–45% (Santhi et al., 2006; Her et al., 2016). Studies have reported the effectiveness of the water and sediment control basin, which reduced sediment loading (Her et al., 2016; Turnbow, 2021). Water and sediment control basins (WASCoBs) reduced the erosive potential of surface water by holding it with berms and allowing it to flow through stable outlets (Turnbow, 2021). Water exit from stable outlets helped in reducing sediment transport by allowing more deposits. Her et al. (2016) found that WASCoBs effectively reduced sediment transport by up to 47% at the field level. Similarly, slash-and-burn shifting cultivation with long rotation (1-year farming and 8 years uncultivated) effectively reduced sediment loss. Long rotation helped increase soil cover, thus reducing sediment movement (Valentin et al., 2008).
The literature also showed that the no-tillage condition in farming helped in reducing sediment deposition (Kurothe et al., 2014; Her et al., 2016). The study concluded that minimum disturbance under no-tillage conditions and mulch surface protection effectively reduced sediment concentration. Cover crops were effective in reducing sediment load. Her et al. (2016) found that sediment transport was reduced by 48%–72% with the implementation of a cover crop as they provided continuous cover to the soil. Contour farming also effectively controlled sediment transport (Santhi et al., 2006; Farahani et al., 2016). Growing crops perpendicular to the slope direction prevented sheet and rill erosion and, thus, sediment movement (Santhi et al., 2006). Further, check dams were also considered an effective way to reduce sediment movement. On-stream check-dams were often built upstream of a watershed, which ceases the infiltration of course- and medium-sized sediments (Mishra et al., 2007). The study concluded that the presence of check-dams could reduce sediment transport by up to 64% at a watershed level. These results indicated the effectiveness of practices in reducing sediment transport.
Soil Nutrients—Soil nutrients are a complex fusion of organic remnants in varying stages of decomposition (Hofman, 2004). Conventional practices such as continuous cropping and tillage farming lead to the exhaustion of organic matter in the soil. This deteriorates soil structure, moisture, and nutrient retention (Stockdale et al., 2002). The movement of nutrients poses a severe threat to the agricultural system. The studies have indicated that the movement of nutrients can also occur through an irrigation network resulting in non-point source pollution (Quemada et al., 2013). In the context of soil nutrients, conservation practices aim to reduce nutrient losses (organic matter, nitrogen content, and phosphorus content). Surface runoff and leaching are often considered primary sources of nutrient loss. In such scenarios, practices that effectively control runoff can reduce nutrient loss.
In the context of conservation practices, cover crops were effective in reducing nutrients’ leaching. A meta-analysis found that the winter cover crop has the potential to reduce leaching by up to 70% (Tonitto et al., 2006). Similarly, one study showed nitrate leaching reduction by up to 56% through nonleguminous cover crops (Thapa et al., 2018). They concluded that the effect varied with soil type, cover crops, and climate conditions. It is observed that a combination of practices often increased the efficiency in the performance of practices. Studies have reported the effectiveness of no-tillage with the cover crop, which significantly decreased movement of organic nutrients in the soil (Rickson et al., 2015). Similarly, Baker (2001) found the effectiveness of crop rotation, no tillage, and nutrient management practices in reducing leaching by up to 30%. Retaining the crop residue can also be one feasible way to restore the organic content in the soil, as the top layer has a higher concentration of soil nutrients, making it necessary to conserve the top layer of the soil (Rickson et al., 2015).
Conservation practice referring to filter strips has also shown the ability to slow the runoff rate, allowing the infiltration process to reduce nutrient losses (Magette et al., 1989). Studies have found a reduction of up to 80% in nutrient transport post-implementation of filter strips (Dickey and Vanderholm, 1989). Likewise, in a qualitative study, Gilley and Risse (2000) reported the effectiveness of manure in reducing nutrient loss, while Adimassu et al. (2014) reported the efficacy of graded soil bunds in reducing the organic matter loss, nitrogen, and phosphorus content. These cases indicated that nature-based solutions have the potential to reduce nutrient loss.
5 Influence of conservation practices under climate change
Climate plays a defining role in hydrologic processes and ultimately influences surface runoff, soil erosion, sediment transport, and nutrient loss that conservation practices can address. According to the Intergovernmental Panel on Climate Change (IPCC), in the last 1,400 years, 1983–2012 was recorded as the warmest 30-year period in the Northern Hemisphere (IPCC, 2014). The increase in temperature and intensified precipitation have resulted in accelerated erosion processes, which has led to poor soil quality and productivity, and loss in organic matter content (Delgado et al., 2013). In the context of agricultural production, literature has cited that the increase in maximum and minimum daily temperature across the globe will hamper farming operations. The water demand for crops will rise, increasing crop water stress (Hatfield and Prueger, 2011). Further, the phenological development of plants will accelerate, leading to a decline in productivity (Herrero and Johnson, 1980; Commuri and Jones, 2001; Salem et al., 2007; Prasad et al., 2008). The increase in temperature may also result in early snowmelt, which will affect the cropping system resulting in crop destruction and irregular freshwater availability (Hatfield et al., 2013), and a significant change in the nutrient load with a difference in the atmospheric conditions (dry, wet, average) will be experienced, which will result in non-point source pollution (Muenich et al., 2016).
Conservation practices can help in reducing climate change’s impact (Delgado et al., 2013; Wagena and Easton, 2018). However, it may be less effective in the case of extreme precipitation and higher CO2 concentration (Klik and Eitzinger, 2010; Dakhlalla and Parajuli, 2016; Qiu et al., 2020). A study reported that the implementation of cover crops and crop rotation could help in maintaining the quality of the soil, especially in rising temperature that accelerates soil organic matter mineralization (Delgado et al., 2013). Similarly, practices such as tile drainage and strip-cropping, when simulated under changing climate scenarios, have shown positive results in reducing surface runoff, sediment export, and phosphorus content (Wagena and Easton, 2018). Klik and Eitzinger (2010) reported on the effectiveness of no-till conditions and grassland scenarios. The results suggested that practices were still effective in reducing surface runoff. However, there was a reduction of 16%–53% in performance under climate change from baseline scenarios. Shi et al. (2012) reported an increase in total runoff due to extreme events, though soil erosion control practices effectively controlled up to 50%. Conservation practices such as drainage systems, wetlands, hilling, and shifting the planting season were potential ways to combat water management challenges (Delgado et al., 2013). The study of complex interaction among the rising concentration of CO2, increasing temperature, and water availability is required to analyze the efficacy of the application of conservation practices and to develop future adaptive strategies for their enhancement. The overall results suggested that conservation practices have a significant role in subduing the impacts of climate change.
6 Assessment of conservation practices’ effectiveness in improving peak flow and water quality conditions
The assessment of the effectiveness of conservation practices was carried out through different approaches like observing time series of hydrometeorological and water quality indicators (Kuhnle et al., 2008), runoff monitoring, including runoff samples, to study sediment movement and nutrient loss (Cullum et al., 2010), application of remote sensing (normalized difference vegetation index was used to estimate nutrient uptake efficiency of winter cover crops) (Hively et al., 2009), field monitoring (A study analyzed the effectiveness of vegetated filter strips in reducing nutrient loss and sediment transport at plot scale) (Magette et al., 1989), and modeling, for analyzing the impact on flow, sediment transport, and nutrient loss (Singh et al., 2018). Out of these, most of the studies followed a modeling approach (Santhi et al., 2006; Parajuli et al., 2009; Rao et al., 2009; Osmond et al., 2012a; Michalak et al., 2013). Some applications of models in the context of conservation practices included evaluating how much existing conditions would be improved if different sets of conservation practices were implemented or predicting their impacts to assess other watershed management options (Chaubey et al., 2010).
The availability of complex models and high-performance computational systems to carry out different simulations has encouraged its usage (Sharpley et al., 2002). Studies have applied different models for the assessment. For instance, Hengsdijk et al. (2005) followed a multimodal approach, including crop growth, nutrient monitoring, and a hydrological erosion model to quantify the effectiveness of bunds, mulching, and reforestation in improving water availability, nutrient availability, and reducing erosion. Francesconi et al. (2015) applied the Agricultural Policy/Environmental eXtender (APEX) model to quantify the effectiveness of nature-based solutions in reducing runoff, sediment transport, and nutrient loss. Zhang et al. (2012) used the Rangeland Hydrology and Erosion Model (RHEM) to see the effectiveness of conservation practices in reducing the impact of climate change on runoff and erosion. A widely used model to quantify the impacts of conservation practices is the Soil Water Assessment Tool (SWAT) (Hengsdijk et al., 2005; Bracmort et al., 2006; Arabi et al., 2008; Chaubey et al., 2010). In this study, we classified assessment approaches into two subsections: model-based and model-free analysis (Table 4).
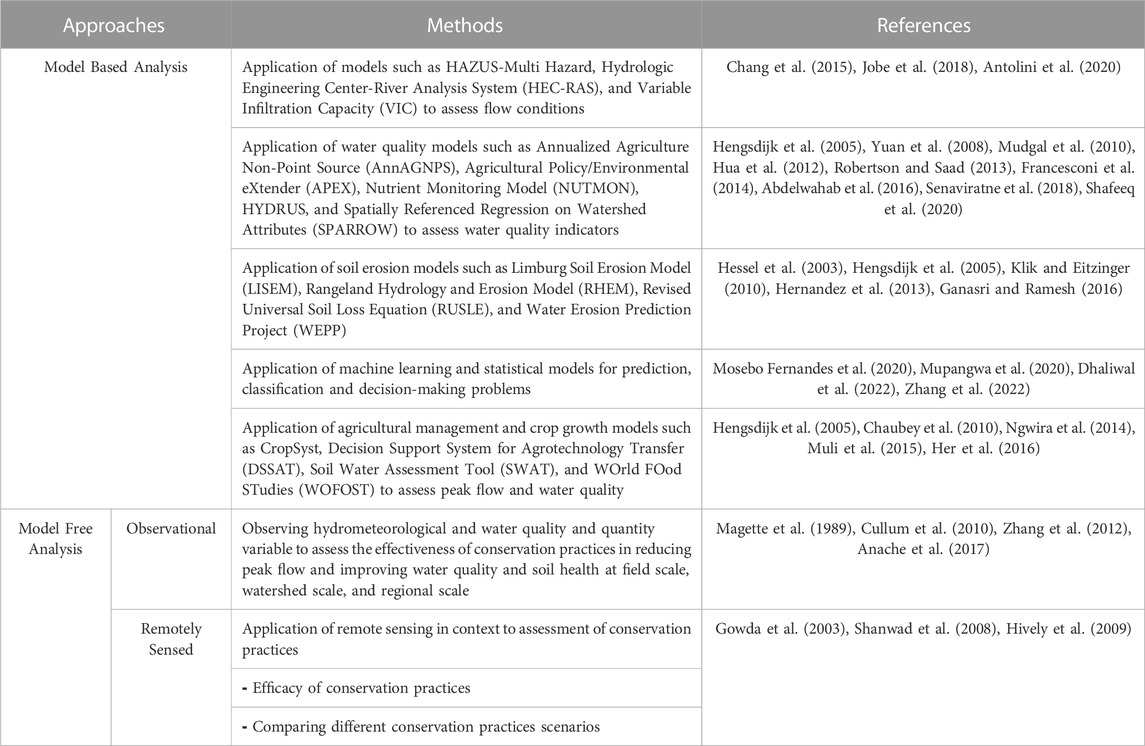
TABLE 4. Summary of Approaches followed for Assessing the Effectiveness of Conservation Practices in Improving Peak Flow and Water Quality Conditions.
The assessment of conservation practices across the literature included different types of datasets with information on conservation practices, hydrometeorological variables, water quantification and quality variables, watershed conditions, climate change scenarios, and stakeholder’s socio-economic status. We provided a summary of the information found during our review process (Table 5).
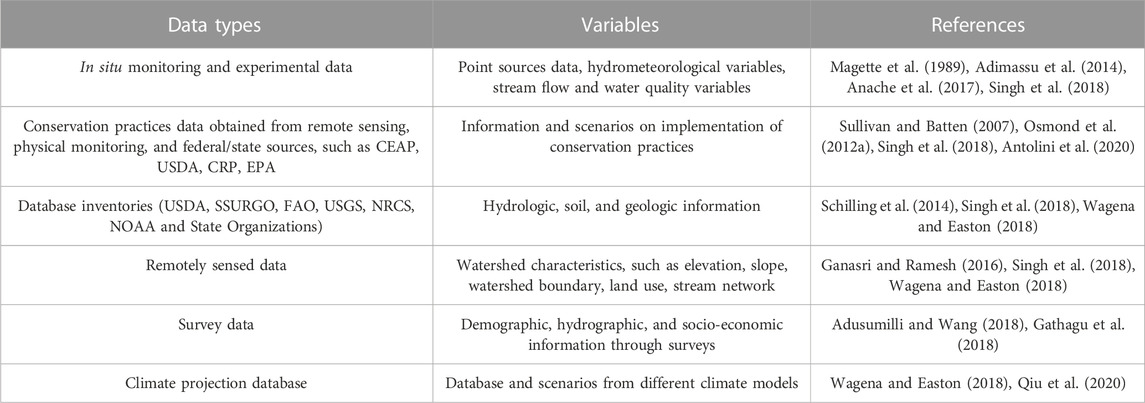
TABLE 5. Summary of Datasets used for Assessing the Effectiveness of Conservation Practices in Improving Peak Flow and Water Quality Conditions.
7 Challenges and future directions associated with the assessment of conservation practices
7.1 In the context of data
One of the challenges associated with the assessment of conservation practices is the limited availability of spatially and temporally extensive conservation practice data (Her et al., 2016; Singh et al., 2018). The literature review demonstrated that this is a global challenge for researchers. For instance, Sullivan and Batten (2007) created geographic databases of conservation practices based on the information listed in previous files and maps. Jha et al. (2010) and Tomer et al. (2008) extracted the information from field surveys. Gowda et al. (2003) carried out aerial imaging to detect different practices applied in their region, while Pennington et al. (2008) conducted interviews with farmers. Notably, all these studies could not find complete information on conservation practices associated with their region, which limits their ability to carry out an exhaustive assessment. One way to overcome the challenges referring to conservation practices information, land use, soil, and weather data, can be through the advanced application of remote sensing. Although, several studies have considered the application of remote sensing for identifying the type and location of conservation practices to assess their effectiveness (Gowda et al., 2003; Tomer et al., 2008; Osmond et al., 2012b). Future studies can work more towards high-resolution mapping of different conservation practices over time, enhanced land use–land cover (LULC) information, and improved remotely sensed meteorological datasets such as cloud cover, surface temperature, snow, ice cover, etc., across higher temporal and spatial scales. Further, survey data can be considered a reliable source of information as it directly comes from the individuals who have implemented conservation practices and have seen its impact on flooding and water quality (Carletto et al., 2021). They can be used as a validation instrument for other data sources. The information obtained from the opinion of farmers is also helpful in cost-benefit analyses and understanding individuals’ attitudes toward nature-based solutions (Traoré et al., 1998). This review finds that application of such datasets is not frequently seen across the literature, though it can potentially improve the accuracy of assessments. Survey/feedback data can be helpful for assessments at field and watershed scales. However, they may be less effective on a larger scale due to a lack of spatial and temporal resolution in information, leading to sizable errors.
Limited monitoring stations often result in the unavailability of precise spatial data, especially when it comes to modeling non-point source pollution (Sharpley et al., 2002). This inconsistency in information across different sub-watersheds within a watershed presents an enormous task to handle. The extrapolation of these datasets is challenging, as most models are developed for specific soil and climatic conditions (Hofman, 2004). Moreover, with spatial variability, the nature and properties of soil and water samples show dynamic inconsistency (Adimassu et al., 2017). In the absence of sufficient observations, studies usually rely on different calibration parameters from watersheds where similar modeling efforts are performed, which increases the uncertainty associated with assessment (Antolini et al., 2020). The lack of available datasets also limits the model’s ability to capture different conservation practices’ responses to water quality and soil health (Sharpley et al., 2002). In such conditions, there is a need to focus on improving the data quality, which can be achieved by increasing the number of observation networks and improving the efficiency of monitoring devices to detect changes occurring in the watershed (O’Donnell, 2012). Different studies in this context have come up with various methods like the Internet of Things (IoT), such as global positioning-based remote-controlled monitoring (Suma et al., 2017) and smart sensors (Mathurkar et al., 2014). Future studies can focus on improving the monitoring network capacity and performance to increase assessment capabilities.
7.2 In the context of modeling/analysis
The evaluation of conservation practices requires multiple sub-assessments of different management and environmental components related to hydrology, crop growth, pesticide, and more. These sub-assessments are carried out for multiple basins. In such situations, it is essential to include different hydrological, geomorphological, and landscape processes, which are necessary to understand the interactions among them and assess the effectiveness of those practices. One way to understand multiple processes is through the applications of coupled modeling. Studies in the past have applied it in different cases; for example, Cai et al. (2020) applied a coupled rainfall-runoff-erosion model (regional climate model, SCS-CN model, and MUSLE model) to assess the effectiveness of conservation practices in reducing runoff and erosion. Naseri et al. (2021) applied SWAT and a genetic algorithm to see the tradeoff between the implementation of conservation practices and the reduction in sediment yield and flow. Studies can apply such models to understand the effectiveness of conservation practices in sustaining environmental and economic objectives. Future research can also focus on implementing an integrated modeling approach to tackle this problem. This can be an integration of model-free and model-based approaches based on the availability of methods for simulating different hydrologic processes (precipitation, runoff, groundwater, surface water inflow, etc.), tracking water quality parameters, and analyzing the interactions of conservation practices with different ecosystem processes. The results of one approach can be input for another and provide assessments at different scales, i.e., field-to-watershed scale, watershed-to-regional scale, and regional-to-global scale. Furthermore, multi-scale assessments can provide significant value. All these approaches can increase the realism of the assessments and result in the development of more effective conservation plans.
In general, models apply conservation practices homogenously to their resolution capacity. However, actual management can have spatial and temporal heterogeneities at even smaller scales (Antolini et al., 2020). This challenge was observed in many studies; for instance, in Singh et al. (2018), the effectiveness of the filter strip varied spatially; however, it was not accommodated in the model. Further, pastures were simulated homogenously over barren lands. Though there were rocky formations in a few barren lands, the model does not provide the flexibility to account for that. This homogeneity restricts the accurate assessment of the effectiveness of conservation practices. In such cases, studies can look for integrating high-resolution conservation practice information (e.g., remote sensing sources) with existing lithological and hydrological information to improve the simulation ability of models. Likewise, application of machine learning (ML) can be helpful when it comes to the data-intensive assessment of conservation practices’ effectiveness. In the context of agricultural management, ML algorithms have been applied in several past studies (e.g., Liakos et al., 2018; Meshram et al., 2021). These algorithms can be convenient for predicting yield, disease detection, weed detection, crop quality, soil composition, soil moisture, and species recognition, providing rich recommendations to farmers and decision-makers (Liakos et al., 2018; Meshram et al., 2021). However, ML has not been implemented extensively in the assessment of conservation practices and their interactions with hydrology. These algorithms are well poised to address several crucial problems related to the impacts of conservation practices through exploratory data analysis, pattern recognition, feature selection, analysis of spatial-temporal changes and variabilities, prediction of conservation-relevant processes, and understanding of the effectiveness and efficiency of different conservation practices.
The results from the modeling approaches have a degree of uncertainty. These uncertainties can stem from various sources. For example, imperfect or limited representation of conservation practices in models due to the lack of available information related to those practices can contribute to uncertainty. Limitations of models due to the underlying assumptions, model structure, and the associated parameters, calibration schemes, etc., can restrict their ability to accurately simulate the coupled processes. Errors propagating from the input and target datasets can also affect the performance of a model and bias its calibration and validation (Sharpley et al., 2002; O’Connell et al., 2007). Models are often restricted to a fixed resolution (point-scale, hillslope-scale, hydrologic response unit-scale, basin-scale, etc.), which further restricts their ability to carry out multi-scale simulations. Tackling these problems requires the development of new modeling schemes and parameterizations that can effectively simulate the coupled nature of the conservation practice impacts on hydrology. Oftentimes, simple structural improvements in a model can lead to a drastic improvement in its performance (Roy et al., 2017). Once an improved structure/parameterization is established, the sensitivity of the associated parameters needs to be thoroughly assessed to get more insights into the underlying physical processes. It is also important to understand how uncertainty propagates through these coupled parameterizations and how improvements in these parameterizations can result in the reduction of uncertainty at different levels. Focus also needs to be drawn toward understanding how the dynamics of these coupled processes vary across scales. Proper characterization of these uncertainties can better inform potential management interventions.
Most of the studies reviewed in our literature have not considered the geological aspects such as lithological properties of strata and their relative position, faults and folds, the orientation of surface, elevation, and natural drainage network conditions in landscapes. This may be due to a lack of information or the inability of the models to simulate them. It is important to note that landscapes are shaped by geomorphological (aeolian, biological, fluvial, etc.) and pedological processes (soil formulation, soil evolution, etc.) and significantly influence soil properties, erosion, and sediment movement (Conacher, 2002). Future studies can include information from geomorphic assessments carried out in the past to perform studies on historical, existing, and future conditions of channels and their response to land-use changes that can help in understanding the impact of geology on soil conditions. This assessment needs to be considered more frequently as findings can be helpful in understanding the underlying causal relationships.
7.3 In the context of management
Studies have found the application of conservation practices sometimes decreases the overall crop productivity (Hatibu et al., 2003; Kinama et al., 2011). One reason is that the application of nature-based measures often utilizes the lands intended for production. In such a case, it is challenging for policymakers, researchers, and farmers to develop different policies and plans to carry out sustainable production. Future studies can also provide helpful insights into developing and implementing government policies and initiatives such as commodity support programs, incentives, and compensation. Further, research can guide the government in including private sectors to promote the application of conservation practices.
The economic perspective of implementing conservation practices is yet to be explored in most of the literature, though some studies have identified this (Schilling et al., 2014; Telles et al., 2019). This requires attention as it plays a vital role in building a strong perception of implementing conservation practices in the mind of farmers, policymakers, and local people. Further, studies have reported the importance of the role of socio-economic factors, policies, and people’s perceptions in adopting conservation practices. Studying these factors can help decision-making be more legitimate, salient, robust, and practical (Bennett et al., 2017). Thus, it is essential to consider all such information while assessing the effectiveness of conservation practice. The social-economic factor also plays a vital role in adopting conservation practices. In such cases, studying social vulnerability can be required before formulating policies or quantifying its risk.
Most studies have directed their efforts toward reducing surface runoff, soil erosion, soil nutrient loss, and sediment transport. But to attain sustainability, it is essential to consider other aspects of the ecosystem. These include the study of quantitative links between conservation practices and ecological responses, the influence of conservation practices on physical habitat quality, etc. (Lizotte et al., 2021). Future studies can focus on those attributes by developing a framework that links conservation practices and programs to the benefits of the ecosystem at national and regional scales.
8 Summary and conclusion
This paper provided an overview of the effectiveness of conservation practices in reducing flooding and improving water quality across watershed and field scales globally, which hindered agricultural productivity and posed severe threats to the ecosystem. The critical issues identified across the literature included peak flows (which often result in flooding), surface runoff, soil erosion, sediment transport, and nutrient loss. These issues were often linked to one another and affected the landscape and aquatic systems. We reviewed the mechanisms of frequently cited conservation practices that improved the existing conditions (peak flows, surface runoff, soil erosion, sediment transport, and nutrient loss), along with their limitations and effectiveness under climate change. The review found that the combinations of conservation practices were more effective than single-practice implementation. The effectiveness of conservation practices changed with spatial and temporal variations (the same methods were applied in two different areas, and the results were distinct). Further, in some cases, several conservation practices were found to have similar actions or effects on surface runoff, soil erosion, sediment transport, and nutrient loss, making them complex to quantify. The review also realized that assessment of the impact of future conservation practices in the region were affected by uncertainties in the projection of hydroclimatic variables, especially under climate change.
This review aims to help concerned authorities and researchers in improving future planning and management of their area of interest. The adoption of nature-based solutions for flood mitigation and improving water quality has a prominent level of interest on a local, regional, and global scale. The demand for sustainable production by utilizing natural resources effectively is key to meeting the population demand while minimizing environmental impact. In such a scenario, the role of conservation practices will be vital.
Author contributions
SS, AB, ET, and TR conceptualized this review paper. SS wrote this initial review paper. AB, ET, and TR contributed to reviewing and editing the manuscript. All authors have read and approved the submitted version of the manuscript.
Funding
This research is supported by the Nebraska Department of Environment and Energy with funds through EPA Section 319 Award 56-2083 (Title: Impacts of conservation practices on the water quality and quantity in the Shell Creek Watershed, Nebraska).
Conflict of interest
The authors declare that the research was conducted in the absence of any commercial or financial relationships that could be construed as a potential conflict of interest.
Publisher’s note
All claims expressed in this article are solely those of the authors and do not necessarily represent those of their affiliated organizations, or those of the publisher, the editors and the reviewers. Any product that may be evaluated in this article, or claim that may be made by its manufacturer, is not guaranteed or endorsed by the publisher.
References
Abdelwahab, O. M. M., Bingner, R. L., Milillo, F., and Gentile, F. (2016). Evaluation of alternative management practices with the AnnAGNPS model in the Carapelle watershed. Soil Sci. 181 (7), 293–305. doi:10.1097/ss.0000000000000162
Adimassu, Z., Langan, S., Johnston, R., Mekuria, W., and Amede, T. (2017). Impacts of soil and water conservation practices on crop yield, run-off, soil loss and nutrient loss in Ethiopia: Review and synthesis. Environ. Manag. 59 (1), 87–101. doi:10.1007/s00267-016-0776-1
Adimassu, Z., Mekonnen, K., Yirga, C., and Kessler, A. (2014). Effect of soil bunds on runoff, soil and nutrient losses, and crop yield in the central highlands of Ethiopia. Land Degrad. Dev. 25 (6), 554–564. doi:10.1002/ldr.2182
Adusumilli, N., and Wang, H. (2018). Analysis of soil management and water conservation practices adoption among crop and pasture farmers in humid-south of the United States. Int. Soil Water Conservation Res. 6 (2), 79–86. doi:10.1016/j.iswcr.2017.12.005
Anache, J. A., Wendland, E. C., Oliveira, P. T., Flanagan, D. C., and Nearing, M. A. (2017). Runoff and soil erosion plot-scale studies under natural rainfall: A meta-analysis of the Brazilian experience. Catena 152, 29–39. doi:10.1016/j.catena.2017.01.003
Antolini, F., Tate, E., Dalzell, B., Young, N., Johnson, K., and Hawthorne, P. L. (2020). Flood risk reduction from agricultural best management practices. J. Am. Water Resour. Assoc. 56 (1), 161–179. doi:10.1111/1752-1688.12812
Arabi, M., Frankenberger, J. R., Engel, B. A., and Arnold, J. G. (2008). Representation of agricultural conservation practices with SWAT. Hydrol. Process. 22 (16), 3042–3055. doi:10.1002/hyp.6890
Araya, T., Cornelis, W. M., Nyssen, J., Govaerts, B., Bauer, H., Gebreegziabher, T., et al. (2011). Effects of conservation agriculture on runoff, soil loss and crop yield under rainfed conditions in Tigray, Northern Ethiopia. Soil Use Manag. 27 (3), 404–414. doi:10.1111/j.1475-2743.2011.00347.x
Bai, P., Liu, X., Liang, K., and Liu, C. (2016). Investigation of changes in the annual maximum flood in the Yellow River basin, China. Quat. Int. 392, 168–177. doi:10.1016/j.quaint.2015.04.053
Baker, J. L. (2001). Limitations of improved nitrogen management to reduce nitrate leaching and increase use efficiency. TheScientificWorldJOURNAL 1, 10–16. doi:10.1100/tsw.2001.457
Bakker, M. M., Govers, G., and Rounsevell, M. D. A. (2004). The crop productivity–erosion relationship: An analysis based on experimental work. CATENA 57 (1), 55–76. doi:10.1016/j.catena.2003.07.002
Barker, D. J., MacAdam, J. W., Butler, T. J., and Sulc, R. M. (2012). Forage and biomass planting. Conservation outcomes from pastureland and hayland practices: Assessment, recommendations, and knowledge gaps. Available at: https://www.afgc.org/wp-content/uploads/ch_2.pdf.
Basche, A., Tully, K., Álvarez-Berríos, N. L., Reyes, J., Lengnick, L., Brown, T., et al. (2020). Evaluating the untapped potential of U.S. Conservation investments to improve soil and environmental health. Front. Sustain. Food Syst. 4. doi:10.3389/fsufs.2020.547876
Bennett, N. J., Roth, R., Klain, S. C., Chan, K., Christie, P., Clark, D. A., et al. (2017). Conservation social science: Understanding and integrating human dimensions to improve conservation. Biol. Conserv. 205, 93–108. doi:10.1016/j.biocon.2016.10.006
Blanco-Canqui, H., Gantzer, C. J., Anderson, S. H., Alberts, E. E., and Thompson, A. L. (2004). Grass barrier and vegetative filter strip effectiveness in reducing runoff, sediment, nitrogen, and phosphorus loss. Soil Sci. Soc. Am. J. 68 (5), 1670–1678. doi:10.2136/sssaj2004.1670
Bracmort, K. S., Arabi, M., Frankenberger, J. R., Engel, B. A., and Arnold, J. G. (2006). Modeling long-term water quality impact of structural BMPs. Am. Soc. Agric. Biol. Eng. 49 (2), 367–374. doi:10.13031/2013.20411
Brémond, P., Grelot, F., and Agenais, A.-L. (2013). Review article: Economic evaluation of flood damage to agriculture – review and analysis of existing methods. Nat. Hazards Earth Syst. Sci. 13 (10), 2493–2512. doi:10.5194/nhess-13-2493-2013
Cai, M., An, C., Guy, C., and Lu, C. (2020). Assessment of soil and water conservation practices in the loess hilly region using a coupled rainfall-runoff-erosion model. Sustainability 12 (3), 934. doi:10.3390/su12030934
Carletto, C., Dillon, A., and Zezza, A. (2021). Agricultural data collection to minimize measurement error and maximize coverage. Handb. Agric. Econ. 5, 4407–4480. doi:10.1016/bs.hesagr.2021.10.008
Chang, J., Wang, Y., Istanbulluoglu, E., Bai, T., Huang, Q., Yang, D., et al. (2015). Impact of climate change and human activities on runoff in the Weihe River Basin, China. Quat. Int. 380, 169–179. doi:10.1016/j.quaint.2014.03.048
Chaubey, I., Chiang, L., Gitau, M. W., and Mohamed, S. (2010). Effectiveness of best management practices in improving water quality in a pasture-dominated watershed. J. Soil Water Conservation 65 (6), 424–437. doi:10.2489/jswc.65.6.424
Commuri, P. D., and Jones, R. J. (2001). High temperatures during endosperm cell division in maize: A genotypic comparison under in vitro and field conditions. Crop Sci. 41 (4), 1122–1130. doi:10.2135/cropsci2001.4141122x
Conacher, A. (2002). A role for geomorphology in integrated catchment management. Aust. Geogr. Stud. 40 (2), 179–195. doi:10.1111/1467-8470.00173
Corbeels, M., de Graaff, J., Ndah, T. H., Penot, E., Baudron, F., Naudin, K., et al. (2014). Understanding the impact and adoption of conservation agriculture in africa: A multi-scale analysis. Agric. Ecosyst. Environ. 187, 155–170. doi:10.1016/j.agee.2013.10.011
Cullum, R. F., Locke, M. A., and Knight, S. S. (2010). Effects of conservation reserve program on runoff and lake water quality in an oxbow lake watershed. United States: United States Department of Agriculture.
Dakhlalla, A. O., and Parajuli, P. B. (2016). Evaluation of the best management practices at the watershed scale to attenuate peak streamflow under climate change scenarios. Water Resour. Manag. 30 (3), 963–982. doi:10.1007/s11269-015-1202-9
De Laney, T. A. (1995). Benefits to downstream flood attenuation and water quality as a result of constructed wetlands in agricultural landscapes. J. Soil Water Conservation 50 (6), 620–626.
Delgado, J. A., Nearing, M. A., and Rice, C. W. (2013). Conservation practices for climate change adaptation. Adv. Agron. 121, 47–115. doi:10.1016/B978-0-12-407685-3.00002-5
Dermisis, D., Abaci, O., Thanos Papanicolaou, A. N., and Wilson, C. G. (2010). Evaluating grassed waterway efficiency in southeastern Iowa using WEPP. Soil Use Manag. 26 (2), 183–192. doi:10.1111/j.1475-2743.2010.00257.x
Dhaliwal, J. K., Panday, D., Saha, D., Lee, J., Jagadamma, S., Schaeffer, S., et al. (2022). Predicting and interpreting cotton yield and its determinants under long-term conservation management practices using machine learning. Comput. Electron. Agric. 199, 107107. doi:10.1016/j.compag.2022.107107
Dickey, E. C., and Vanderholm, D. H. (1989). Performance and design of vegetative filters for feedlot runoff treatment. Lincoln Nebraska: University Of Nebraska - Lincoln.
Dillaha, T. A., Sherrard, J. H., Lee, D., Shanholtz, V. O., Mostaghimi, S., and Magette, W. L. (1986). Use of vegetative filter strips to minimize sediment and phophorus losses from feedlots: Phase I. Experimental plot studies. Available at: https://vtechworks.lib.vt.edu/bitstream/handle/10919/46591/WRRC_Bull_151.pdf?sequence=1&isAllowed=y.
Environmental Protection Agency (2018). Surface runoff from agricultural land. Available at: https://enviroatlas.epa.gov/enviroatlas/DataFactSheets/pdf/ESN/Surfacerunoffagriculturallands.pdf.
Erkossa, T., Erkossa, T., Stahr, K., and Gaiser, T. (2005). Effect of different methods of land preparation on runoff, soil and nutrient losses from a Vertisol in the Ethiopian highlands. Soil Use Manag. 21 (2), 253–259. doi:10.1079/SUM2005319
Ersedo, A. E. (2021). Investigation of farmers’ perception on the problem of soil erosion and conservation knowledge in Anlemo Woreda, Hadiya zone, Snnpr. Int. J. Leg. Stud. ( IJOLS ) 9 (1), 121–138. doi:10.5604/01.3001.0015.2289
Evrard, O., Vandaele, K., van Wesemael, B., and Bielders, C. L. (2008). A grassed waterway and earthen dams to control muddy floods from a cultivated catchment of the Belgian loess belt. Geomorphology 100 (3–4), 419–428. doi:10.1016/j.geomorph.2008.01.010
Farahani, S., Soheilifard, F., and Amin Asoodar, M. (2016). Effects of contour farming on runoff and soil erosion reduction: A review study. Elixir Agric. 101 (1), 44089–44093.
Faulkner, S., Barrow, W., Keeland, B., Walls, S., and Telesco, D. (2011). Effects of conservation practices on wetland ecosystem services in the Mississippi Alluvial Valley. Ecol. Appl. 21 (3), S31–S48. doi:10.1890/10-0592.1
Francesconi, W., Smith, D. R., Flanagan, D. C., Huang, C. H., and Wang, X. (2015). Modeling conservation practices in APEX: From the field to the watershed. J. Gt. Lakes. Res. 41 (3), 760–769. doi:10.1016/j.jglr.2015.05.001
Francesconi, W., Smith, D. R., Heathman, G. C., Wang, X., and Williams, C. O. (2014). Monitoring and APEX modeling of no-till and reduced-till in tile-drained agricultural landscapes for water quality. Trans. ASABE 57 (3), 777–789. doi:10.13031/trans.57.10332
Ganasri, B. P., and Ramesh, H. (2016). Assessment of soil erosion by RUSLE model using remote sensing and GIS-A case study of Nethravathi Basin. Geosci. Front. 7 (6), 953–961. doi:10.1016/j.gsf.2015.10.007
Garrity, D. P. (1999). Contour farming based on natural vegetative strips: Expanding the scope for increased food crop production on sloping lands in Asia. Environ. Dev. Sustain. 1 (3–4), 323–336. doi:10.1023/a:1010091904395
Gathagu, J., Mourad, K., and Sang, J. (2018). Effectiveness of contour farming and filter strips on ecosystem services. Water 10 (10), 1312. doi:10.3390/w10101312
Gerla, P. J. (2007). Estimating the effect of cropland to prairie conversion on peak storm run-off. Restor. Ecol. 15 (4), 720–730. doi:10.1111/j.1526-100x.2007.00284.x
Gillespie, A. R., Jose, S., Benjamin, T. J., Hoover, W. L., and Seifert, J. R., (2000). Defining competition vectors in a temperate alley cropping system in the midwestern USA: 1. Production physiology. Agrofor. Syst. 48 (1), 25–40. doi:10.1023/A:1006285205553
Gilley, J. E., and Risse, L. M. (2000). Runoff and soil loss as affected by the application of manure. Trans. ASAE 43 (6), 1583–1588. doi:10.13031/2013.3058
Gowda, P. H., Mulla, D. J., and Dalzell, B. J. (2003). Examining the targeting of conservation tillage practices to steep vs. flat landscapes in the Minnesota River Basin. J. Soil Water Conservation 58 (1), 53–57.
Graves, A. R., Burgess, P. J., Palma, J. H. N., Herzog, F., Moreno, G., Bertomeu, M., et al. (2007). Development and application of bio-economic modelling to compare silvoarable, arable, and forestry systems in three European countries. Ecol. Eng. 29 (4), 434–449. doi:10.1016/j.ecoleng.2006.09.018
Gruenewald, H., Brandt, B. K. V., Schneider, B. U., Bens, O., Kendzia, G., and Hüttl, R. F. (2007). Agroforestry systems for the production of woody biomass for energy transformation purposes. Ecol. Eng. 29 (4), 319–328. doi:10.1016/j.ecoleng.2006.09.012
Gupta, A. K., Rudra, R. P., Gharabaghi, B., Daggupati, P., Goel, P. K., and Shukla, R. (2019). CoBAGNPS: A toolbox for simulating water and sediment control basin, WASCoB through agnps model. CATENA 179, 49–65. doi:10.1016/j.catena.2019.02.003
Ha, M., Wu, M., Tomer, M. D., Gassman, P. W., Isenhart, T. M., Arnold, J. G., et al. (2020). Biomass production with conservation practices for two Iowa watersheds. JAWRA J. Am. Water Resour. Assoc. 56 (6), 1030–1044. doi:10.1111/1752-1688.12880
Hatfield, J. L., Cruse, R. M., and Tomer, M. D. (2013). Convergence of agricultural intensification and climate change in the Midwestern United States: Implications for soil and water conservation. Mar. Freshw. Res. 64 (5), 423. doi:10.1071/MF12164
Hatfield, J. L., and Prueger, J. H. (2011). “Agroecology: Implications for plant response to climate change,” in Crop adaptation to climate change (United States: Wiley), 27–43. doi:10.1002/9780470960929.ch3
Hatibu, N., Young, M. D. B., Gowing, J. W., Mahoo, H. F., and Mzirai, O. B. (2003). Developing improved dryland cropping systems for maize in semi-arid Tanzania. Part 1: Experimental evidence for the benefits of rainwater harvesting. Exp. Agric. 39 (3), 279–292. doi:10.1017/S0014479703001285
Heathcote, A. J., Filstrup, C. T., and Downing, J. A. (2013). Watershed sediment losses to lakes accelerating despite agricultural soil conservation efforts. PLoS ONE 8 (1), e53554. doi:10.1371/journal.pone.0053554
Hengsdijk, H., Meijerink, G. W., and Mosugu, M. E. (2005). Modeling the effect of three soil and water conservation practices in Tigray, Ethiopia. Agric. Ecosyst. Environ. 105 (1–2), 29–40. doi:10.1016/j.agee.2004.06.002
Her, Y., Chaubey, I., Frankenberger, J., and Smith, D. (2016). Effect of conservation practices implemented by USDA programs at field and watershed scales. J. Soil Water Conservation 71 (3), 249–266. doi:10.2489/jswc.71.3.249
Hernandez, M., Nearing, M. A., Stone, J. J., Pierson, F. B., Wei, H., Spaeth, K. E., et al. (2013). Application of a rangeland soil erosion model using National Resources Inventory data in southeastern Arizona. J. Soil Water Conserv. 68 (6), 512–525. doi:10.2489/jswc.68.6.512
Herrero, M. P., and Johnson, R. R. (1980). High temperature stress and pollen viability of maize 1. Crop Sci. 20 (6), 796–800. doi:10.2135/cropsci1980.0011183X002000060030x
Herron, C. L., Ruark, M. D., and Minks, K. R. (2016). Conservation benefits of a grade stabilization structure. Wisconsin: University of Wisconsin--Extension.
Herweg, K., and Ludi, E. (1999). The performance of selected soil and water conservation measures—Case studies from Ethiopia and Eritrea. CATENA 36 (1–2), 99–114. doi:10.1016/S0341-8162(99)00004-1
Hessel, R., Messing, I., Liding, C., Ritsema, C., and Stolte, J. (2003). Soil erosion simulations of land use scenarios for a small Loess Plateau catchment. Catena 54 (1-2), 289–302. doi:10.1016/s0341-8162(03)00070-5
Hively, W. D., Lang, M., McCarty, G. W., KepplerSadeghi, A., and McConnell, L. L. (2009). Using satellite remote sensing to estimate winter cover crop nutrient uptake efficiency. J. soil water conservation 64 (5), 303–313. doi:10.2489/jswc.64.5.303
Hobbs, P. R., Sayre, K., and Gupta, R. (2008). The role of conservation agriculture in sustainable agriculture. Philosophical Trans. R. Soc. B Biol. Sci. 363 (1491), 543–555. doi:10.1098/rstb.2007.2169
Hofman, G. (2004). Soil and plant nitrogen soil and plant nitrogen. Available at: https://www.researchgate.net/profile/Oswald-Cleemput/publication/265247400_Soil_and_Plant_Nitrogen/links/540e14890cf2f2b29a3a770c/Soil-and-Plant-Nitrogen.pdf.
Hovis, M., Hollinger, J. C., Cubbage, F., Shear, T., Doll, B., Kurki-Fox, J. J., et al. (2021). Natural infrastructure practices as potential flood storage and reduction for farms and rural communities in the North Carolina Coastal Plain. Sustainability 13 (16), 9309. doi:10.3390/su13169309
Hu, X., McIsaac, G. F., David, M. B., and Louwers, C. A. L. (2007). Modeling riverine nitrate export from an East-Central Illinois watershed using SWAT. J. Environ. Qual. 36 (4), 996–1005. doi:10.2134/jeq2006.0228
Hua, L., He, X., Yuan, Y., and Nan, H. (2012). Assessment of runoff and sediment yields using the AnnAGNPS model in a three-gorge watershed of China. Int. J. Environ. Res. public health 9 (5), 1887–1907. doi:10.3390/ijerph9051887
Huang, M., Zhang, L., and Gallichand, J. (2003). Runoff responses to afforestation in a watershed of the Loess Plateau, China. Hydrol. Process. 17 (13), 2599–2609. doi:10.1002/hyp.1281
Huggins, D. R., and Reganold, J. P. (2008). No-till: The quiet revolution. Sci. Am. 299 (1), 70–77. doi:10.1038/scientificamerican0708-70
Hurni, H. (1996). Precious earth: From soil and water conservation to sustainable land management. Switzerland: Geographica Bernensia.
Jagger, P., and Pender, J. (2003). The role of trees for sustainable management of less-favored lands: The case of eucalyptus in Ethiopia. For. Policy Econ. 5 (1), 83–95. doi:10.1016/S1389-9341(01)00078-8
Jha, M. K., Schilling, K. E., Gassman, P. W., and Wolter, C. F. (2010). Targeting land-use change for nitrate-nitrogen load reductions in an agricultural watershed. J. Soil Water Conservation 65 (6), 342–352. doi:10.2489/jswc.65.6.342
Jobe, A., Kalra, A., and Ibendahl, E. (2018). Conservation Reserve Program effects on floodplain land cover management. J. Environ. Manag. 214, 305–314. doi:10.1016/j.jenvman.2018.03.016
Kaini, P., Artita, K., and Nicklow, J. W. (2008). Designing BMPs at a watershed-scale using SWAT and a genetic algorithm. In World Environmental and Water Resources Congress 2008: Ahupua’A, Honolulu, Hawaii, United, May 12–16, 2008, 1–10. doi:10.1061/40976(316)125
Kaini, P., Artita, K., and Nicklow, J. W. (2012). Optimizing structural best management practices using SWAT and genetic algorithm to improve water quality goals. Water Resour. Manag. 26 (7), 1827–1845. doi:10.1007/s11269-012-9989-0
Kalantari, Z., Lyon, S. W., Folkeson, L., French, H. K., Stolte, J., Jansson, P.-E., et al. (2014). Quantifying the hydrological impact of simulated changes in land use on peak discharge in a small catchment. Sci. Total Environ. 466–467, 741–754. doi:10.1016/j.scitotenv.2013.07.047
Kannan, N., Jeong, J., and Srinivasan, R. (2011). Hydrologic modeling of a canal-irrigated agricultural watershed with irrigation best management practices: Case study. J. Hydrologic Eng. 16 (9), 746–757. doi:10.1061/(ASCE)HE.1943-5584.0000364
Kassam, A., Derpsch, R., and Friedrich, T. (2014). Global achievements in soil and water conservation: The case of Conservation Agriculture. Int. Soil Water Conservation Res. 2 (1), 5–13. doi:10.1016/S2095-6339(15)30009-5
Kim, Y. J., Geohring, L. D., Jeon, J. H., Collick, A. S., Giri, S. K., and Steenhuis, T. S. (2006). Evaluation of the effectiveness of vegetative filter strips for phosphorus removal with the use of a tracer. J. Soil Water Conservation 61 (5), 293–302.
Kinama, J. M., Ong, C. K., Stigter, C. J., and Ng, J. K. (2011). Hedgerow intercropping maize or cowpea/senna for drymatter production in Semi-Arid Eastern Kenya. J. Agric. Sci. Technol. 1, 372–384.
King, K. W., Smiley, P. C., Baker, B. J., and Fausey, N. R. (2008). Validation of paired watersheds for assessing conservation practices in the Upper Big Walnut Creek watershed, Ohio. J. Soil Water Conservation 63 (6), 380–395. doi:10.2489/jswc.63.6.380
King, S. L., and Keeland, B. D. (1999). Evaluation of reforestation in the lower Mississippi river alluvial valley. Restor. Ecol. 7 (4), 348–359. doi:10.1046/j.1526-100X.1999.72029.x
Klik, A., and Eitzinger, J. (2010). Impact of climate change on soil erosion and the efficiency of soil conservation practices in Austria. J. Agric. Sci. 148 (5), 529–541. doi:10.1017/S0021859610000158
Knowler, D., and Bradshaw, B. (2007). Farmers’ adoption of conservation agriculture: A review and synthesis of recent research. Food Policy 32 (1), 25–48. doi:10.1016/j.foodpol.2006.01.003
Kuhnle, R. A., Bingner, R. L., Alonso, C. V., Wilson, C. G., and Simon, A. (2008). Conservation practice effects on sediment load in the goodwin Creek experimental watershed. J. Soil Water Conservation 63 (6), 496–503. doi:10.2489/jswc.63.6.496
Kurothe, R. S., Kumar, G., Singh, R., Singh, H. B., Tiwari, S. P., Vishwakarma, A. K., et al. (2014). Effect of tillage and cropping systems on runoff, soil loss and crop yields under semiarid rainfed agriculture in India. Soil Tillage Res. 140, 126–134. doi:10.1016/j.still.2014.03.005
Lahmar, R. (2010). Adoption of conservation agriculture in Europe. Land Use Policy 27 (1), 4–10. doi:10.1016/j.landusepol.2008.02.001
Lal, R. (2015). Restoring soil quality to mitigate soil degradation. Sustainability 7 (5), 5875–5895. doi:10.3390/su7055875
Liakos, K., Busato, P., Moshou, D., Pearson, S., and Bochtis, D. (2018). Machine learning in agriculture: A review. Sensors 18 (8), 2674. doi:10.3390/s18082674
Litton, L. L., and Lohnes, R. A. (1967). Soil-cement for use in stream channel grade-stabilization structures. Transp. Res. Rec. 839, 33–38.
Lizotte, R. E., Smiley, P. C., Gillespie, R. B., and Knight, S. S. (2021). Agricultural conservation practices and aquatic ecological responses. Water 13 (12), 1687. doi:10.3390/w13121687
Lormand, J. R. (1988). The effects of urban vegetation on stormwater runoff in an arid environment. Tucson, AZ: University Of Arizona.
Luo, K., Tao, F., Deng, X., and Moiwo, J. P. (2017). Changes in potential evapotranspiration and surface runoff in 1981-2010 and the driving factors in Upper Heihe River Basin in Northwest China. Hydrol. Process. 31 (1), 90–103. doi:10.1002/hyp.10974
Magette, W. L., Brinsfield, R. B., Palmer, R. E., and Wood, J. D. (1989). Nutrient and sediment removal by vegetated filter strips. Trans. ASAE 32 (2), 0663–0667. doi:10.13031/2013.31054
Mandal, A., and Bhardwaj, N. (2020). “Blocking stones perceived by farmers in utilizing the soil health management practices – a case study of West Bengal,” in Proceedings of the International Conference On Agriculture and Allied Sciences: The Productivity, Food Security and Ecology, Viswavidyalaya, India, 13th to 14th August 2018.
Mathurkar, S. S., Patel, N. R., Lanjewar, R. B., and Somkuwar, R. S. (2014). Smart sensors based monitoring system for agriculture using field programmable gate array. In Proceedings of the 2014 International Conference on Circuits, Power and Computing Technologies [ICCPCT-2014], Nagercoil, India, 20-21 March 2014, (pp. 339–344).
Mead, R., and Willey, R. W. (1980). The concept of a ‘Land Equivalent Ratio’ and advantages in yields from intercropping. Exp. Agric. 16 (3), 217–228. doi:10.1017/S0014479700010978
Merten, G. H., Araújo, A. G., Biscaia, R. C. M., Barbosa, G. M. C., and Conte, O. (2015). No-till surface runoff and soil losses in southern Brazil. Soil Tillage Res. 152, 85–93. doi:10.1016/j.still.2015.03.014
Meshram, V., Patil, K., Meshram, V., Hanchate, D., and Ramkteke, S. D. (2021). Machine learning in agriculture domain: A state-of-art survey. Artif. Intell. Life Sci. 1, 100010. doi:10.1016/j.ailsci.2021.100010
Michalak, A. M., Anderson, E. J., Beletsky, D., Boland, S., Bosch, N. S., Bridgeman, T. B., et al. (2013). Record-setting algal bloom in Lake Erie caused by agricultural and meteorological trends consistent with expected future conditions. Proc. Natl. Acad. Sci. 110 (16), 6448–6452. doi:10.1073/pnas.1216006110
Mielke, L. N. (1985). Performance of water and sediment control basins in northeastern Nebraska. J. Soil and Water Conservation 40 (6), 524–528.
Mishra, A., Froebrich, J., and Gassman, P. W. (2007). Evaluation of the SWAT model for assessing sediment control structures in a small watershed in India. Trans. ASABE 50 (2), 469–477. doi:10.13031/2013.22637
Mitsch, W. J., and Gosselink, J. G. (2000). The value of wetlands: Importance of scale and landscape setting. Ecol. Econ. 35 (1), 25–33. doi:10.1016/S0921-8009(00)00165-8
Moriasi, D. N., Gowda, P. H., Arnold, J. G., Mulla, D. J., Ale, S., and Steiner, J. L. (2013). Modeling the impact of nitrogen fertilizer application and tile drain configuration on nitrate leaching using SWAT. Agric. Water Manag. 130, 36–43. doi:10.1016/j.agwat.2013.08.003
Mosebo Fernandes, A. C., Quintero Gonzalez, R., Lenihan-Clarke, M. A., Leslie Trotter, E. F., and Jokar Arsanjani, J. (2020). Machine learning for conservation planning in a changing climate. Sustainability 12 (18), 7657. doi:10.3390/su12187657
Mudgal, A., Baffaut, C., Anderson, S. H., Sadler, E. J., and Thompson, A. L. (2010). APEX model assessment of variable landscapes on runoff and dissolved herbicides. Trans. ASABE 53 (4), 1047–1058. doi:10.13031/2013.32595
Muenich, R. L., Kalcic, M., and Scavia, D. (2016). Evaluating the impact of legacy P and agricultural conservation practices on nutrient loads from the Maumee River Watershed. Environ. Sci. Technol. 50 (15), 8146–8154. doi:10.1021/acs.est.6b01421
Muli, M. N., Onwonga, R. N., Karuku, G. N., Kathumo, V. M., and Nandukule, M. O. (2015). Simulating soil moisture under different tillage practices, cropping systems and organic fertilizers using cropsyst model. Kenya: Matuu division.
Munoz-Carpena, R., Parsons, J. E., and Gilliam, J. W. (1999). Modeling hydrology and sediment transport in vegetative filter strips. J. hydrology 214 (1-4), 111–129. doi:10.1016/s0022-1694(98)00272-8
Mupangwa, W., Chipindu, L., Nyagumbo, I., Mkuhlani, S., and Sisito, G. (2020). Evaluating machine learning algorithms for predicting maize yield under conservation agriculture in Eastern and Southern Africa. SN Appl. Sci. 2 (952). doi:10.1007/s42452-020-2711-6
Murphy, S. D., Clements, D. R., Belaoussoff, S., Kevan, P. G., and Swanton, C. J. (2006). Promotion of weed species diversity and reduction of weed seedbanks with conservation tillage and crop rotation. Weed Sci. 54 (1), 69–77. doi:10.1614/ws-04-125r1.1
Naseri, F., Azari, M., and Dastorani, M. T. (2021). Spatial optimization of soil and water conservation practices using coupled SWAT model and evolutionary algorithm. Int. Soil Water Conservation Res. 9 (4), 566–577. doi:10.1016/j.iswcr.2021.04.002
Ngwira, A. R., Aune, J. B., and Thierfelder, C. (2014). DSSAT modelling of conservation agriculture maize response to climate change in Malawi. Soil Tillage Res. 143, 85–94. doi:10.1016/j.still.2014.05.003
O’Connell, E., Ewen, J., O’Donnell, G., and Quinn, P. (2007). Is there a link between agricultural land-use management and flooding? Hydrology Earth Syst. Sci. 11 (1), 96–107. doi:10.5194/hess-11-96-2007
O’Donnell, T. K. (2012). Assessing watershed transport of atrazine and nitrate to evaluate conservation practice effects and advise future monitoring strategies. Environ. Manag. 49 (1), 267–284. doi:10.1007/s00267-011-9780-7
Ogawa, H., and Male, J. W. (1983). The flood mitigation potential of inland wetlands. United States: Water Resources Research Center Publication.
Olkeba, B., Shoeb, Q., and Bobe, B. (2012). Effects of straw mulch and farmyard manure on runoff, erosion, in-situ water conservation, and yield and yield components of wheat at the highlands of Bale, south eastern Ethiopia. Afr. J. Agric. Res., 7(44), 5879–5886. doi:10.5897/AJAR12.1467
Osmond, D. L., Meals, D., Hoag, D., Arabi, M., Luloff, A., Jennings, G., et al. (2012b). Improving conservation practices programming to protect water quality in agricultural watersheds: Lessons learned from the National Institute of Food and Agriculture-Conservation Effects Assessment Project. J. Soil Water Conservation 67 (5), 122A–127A. doi:10.2489/jswc.67.5.122A
Osmond, D. L., Tedesco, L., Turco, R., Prokopy, L., Frankenberger, J., Shively, G., et al. (2012a). Eagle Creek watershed, Indiana: National institute of food and agriculture–conservation effects assessment project. Ankeny, Iowa: Soil and Water Conservation Society.
IPCC (2014). “Climate change 2014: Synthesis report,” in Contribution of working groups I, II and III to the fifth assessment report of the intergovernmental Panel on climate change [core writing team. Editors R. K. Pachauri, and L. A. Meyer (Geneva, Switzerland: IPCC), 151.
Parajuli, P. B., Nelson, N. O., Frees, L. D., and Mankin, K. R. (2009). Comparison of AnnAGNPS and SWAT model simulation results in USDA-CEAP agricultural watersheds in south-central Kansas. Hydrol. Process. 23 (5), 748–763. doi:10.1002/hyp.7174
Pennington, J. H., Steele, M. A., Teague, K. A., Kurz, B., Gbur, E., Popp, J., et al. (2008). Breaking ground: A cooperative approach to collecting information on conservation practices from an initially uncooperative population. J. Soil Water Conservation 63 (6), 208A–211A. doi:10.2489/jswc.63.6.208A
Pimentel, D., Harvey, C., Resosudarmo, P., Sinclair, K., Kurz, D., McNair, M., et al. (1995). Environmental and economic costs of soil erosion and conservation benefits. Science 267 (5201), 1117–1123. doi:10.1126/science.267.5201.1117
Prasad, P. V. V., Pisipati, S. R., Ristic, Z., Bukovnik, U., and Fritz, A. K. (2008). Impact of nighttime temperature on physiology and growth of spring wheat. Crop Sci. 48 (6), 2372–2380. doi:10.2135/cropsci2007.12.0717
Qiu, J., Shen, Z., Hou, X., Xie, H., and Leng, G. (2020). Evaluating the performance of conservation practices under climate change scenarios in the Miyun Reservoir Watershed, China. Ecol. Eng. 143, 105700. doi:10.1016/j.ecoleng.2019.105700
Quemada, M., Baranski, M., Nobel-de Lange, M. N. J., Vallejo, A., and Cooper, J. M. (2013). Meta-analysis of strategies to control nitrate leaching in irrigated agricultural systems and their effects on crop yield. Agric. Ecosyst. Environ. 174, 1–10. doi:10.1016/j.agee.2013.04.018
Quinkenstein, A., Wöllecke, J., Böhm, C., Grünewald, H., Freese, D., Schneider, B. U., et al. (2009). Ecological benefits of the alley cropping agroforestry system in sensitive regions of Europe. Environ. Sci. Policy 12 (8), 1112–1121. doi:10.1016/j.envsci.2009.08.008
Rao, N. S., Easton, Z. M., Schneiderman, E. M., Zion, M. S., Lee, D. R., and Steenhuis, T. S. (2009). Modeling watershed-scale effectiveness of agricultural best management practices to reduce phosphorus loading. J. Environ. Manag. 90 (3), 1385–1395. doi:10.1016/j.jenvman.2008.08.011
Renschler, C. S., and Lee, T. (2005). Spatially distributed assessment of short- and long-term impacts of multiple best management practices in agricultural watersheds. J. Soil Water Conservation 60 (6), 446–456.
Richardson, C. W., Bucks, D. A., and Sadler, E. J. (2008). The conservation effects assessment project benchmark watersheds: Synthesis of preliminary findings. J. Soil Water Conservation 63 (6), 590–604. doi:10.2489/jswc.63.6.590
Rickson, R. J., Deeks, L. K., Graves, A., Harris, J. A. H., Kibblewhite, M. G., and Sakrabani, R. (2015). Input constraints to food production: The impact of soil degradation. Food Secur. 7 (2), 351–364. doi:10.1007/s12571-015-0437-x
Rivest, D., Cogliastro, A., Bradley, R. L., and Olivier, A. (2010). Intercropping hybrid poplar with soybean increases soil microbial biomass, mineral N supply and tree growth. Agrofor. Syst. 80 (1), 33–40. doi:10.1007/s10457-010-9342-7
Robertson, D. M., and Saad, D. A. (2013). SPARROW models used to understand nutrient sources in the Mississippi/Atchafalaya River Basin. J. Environ. Qual. 42 (5), 1422–1440. doi:10.2134/jeq2013.02.0066
Roy, T., Gupta, H. V., Serrat-Capdevila, A., and Valdes, J. B. (2017). Using satellite-based evapotranspiration estimates to improve the structure of a simple conceptual rainfall–runoff model. Hydrology Earth Syst. Sci. 21 (2), 879–896. doi:10.5194/hess-21-879-2017
Salem, M. A., Kakani, V. G., Koti, S., and Reddy, K. R. (2007). Pollen-based screening of soybean genotypes for high temperatures. Crop Sci. 47 (1), 219–231. doi:10.2135/cropsci2006.07.0443
Santhi, C., Kannan, N., White, M., Di Luzio, M., Arnold, J. G., Wang, X., et al. (2014). An integrated modeling approach for estimating the water quality benefits of conservation practices at the river basin scale. J. Environ. Qual. 43 (1), 177–198. doi:10.2134/jeq2011.0460
Santhi, C., Srinivasan, R., Arnold, J. G., and Williams, J. R. (2006). A modeling approach to evaluate the impacts of water quality management plans implemented in a watershed in Texas. Environ. Model. Softw. 21 (8), 1141–1157. doi:10.1016/j.envsoft.2005.05.013
Schilling, K. E., Gassman, P. W., Kling, C. L., Campbell, T., Jha, M. K., Wolter, C. F., et al. (2014). The potential for agricultural land use change to reduce flood risk in a large watershed. Hydrol. Process. 28 (8), 3314–3325. doi:10.1002/hyp.9865
Schilling, K. E., and Wolter, C. F. (2009). Modeling nitrate-nitrogen load reduction strategies for the Des Moines River, Iowa using SWAT. Environ. Manag. 44 (4), 671–682. doi:10.1007/s00267-009-9364-y
Senaviratne, G. A., Baffaut, C., Lory, J. A., Udawatta, R. P., Nelson, N. O., Williams, J. R., et al. (2018). Improved APEX model simulation of buffer water quality benefits at field scale. Trans. ASABE 61 (2), 603–616. doi:10.13031/trans.12655
Shafeeq, P. M., Aggarwal, P., Krishnan, P., Rai, V., Pramanik, P., and Das, T. K. (2020). Modeling the temporal distribution of water, ammonium-N, and nitrate-N in the root zone of wheat using HYDRUS-2D under conservation agriculture. Environ. Sci. Pollut. Res. 27, 2197–2216. doi:10.1007/s11356-019-06642-5
Shanwad, U. K., Patil, V. C., Gowda, H. H., and Dasog, G. S. (2008). Application of remote sensing technology for impact assessment of watershed development programme. J. Indian Soc. Remote Sens. 36, 375–386. doi:10.1007/s12524-008-0037-8
Sharpley, A. N., Kleinman, P. J. A., McDowell, R. W., Gitau, M., and Bryant, R. B. (2002). Modeling phosphorus transport in agricultural watersheds: Processes and possibilities. J. Soil Water Conservation 57 (6), 425–439.
Shi, C., Zhou, Y., Fan, X., and Shao, W. (2012). A study on the annual runoff change and its relationship with water and soil conservation practices and climate change in the middle Yellow River basin. CATENA 100, 31–41. doi:10.1016/j.catena.2012.08.007
Singh, G., Saraswat, D., and Sharpley, A. (2018). A sensitivity analysis of impacts of conservation practices on water quality in L’anguille River watershed, Arkansas. Ark. Water 10 (4), 443. doi:10.3390/w10040443
Soule, M. J., Tegene, A., and Wiebe, K. D. (2000). Land tenure and the adoption of conservation practices. Am. J. Agric. Econ. 82 (4), 993–1005. doi:10.1111/0002-9092.00097
Stockdale, E. A., Shepherd, M. A., Fortune, S., and Cuttle, S. P. (2002). Soil fertility in organic farming systems – fundamentally different? Soil Use Manag. 18 (3), 301–308. doi:10.1079/SUM2002143
Sullivan, D. G., and Batten, H. L. (2007). Little River experimental watershed, tifton, Georgia, United States: A historical geographic database of conservation practice implementation. Water Resour. Res. 43 (9), 1–5. doi:10.1029/2007WR006143
Sultan, D., Tsunekawa, A., Haregeweyn, N., Adgo, E., Tsubo, M., Meshesha, D. T., et al. (2018). Efficiency of soil and water conservation practices in different agro-ecological environments in the Upper Blue Nile Basin of Ethiopia. J. Arid. Land 10, 249–263. doi:10.1007/s40333-018-0097-8
Suma, N., Samson, S. R., Saranya, S., Shanmugapriya, G., and Subhashri, R. (2017). IOT based smart agriculture monitoring system. Int. J. Recent Innovation Trends Comput. Commun. 5 (2), 177–181.
Sun, B., Chen, X., Zhang, X., Liang, A., Whalen, J. K., and McLaughlin, N. B. (2020). Greater fungal and bacterial biomass in soil large macropores under no-tillage than mouldboard ploughing. Eur. J. Soil Biol. 97, 103155. doi:10.1016/j.ejsobi.2020.103155
Swanton, C. J., Clements, D. R., and Derksen, D. A. (1993). Weed succession under conservation tillage: A hierarchical framework for research and management. A Hierarchical Framew. Res. Manag. 7 (2), 286–297. doi:10.1017/s0890037x00027615
Sweeney, B. W., and Newbold, J. D. (2014). Streamside forest buffer width needed to protect stream water quality, habitat, and organisms: A literature review. J. Am. Water Resour. Assoc. 50 (3), 560–584. doi:10.1111/jawr.12203
Telles, T. S., Lourenço, M. A. P., Oliveira, J. F., Costa, G. V., and Barbosa, G. M. C. (2019). Soil conservation practices in a watershed in Southern Brazil. An. Acad. Bras. Cienc. 91 (3), e20180578. doi:10.1590/0001-3765201920180578
Thapa, R., Mirsky, S. B., and Tully, K. L. (2018). Cover crops reduce nitrate leaching in agroecosystems:a global meta-analysis. J. Environ. Qual. 47 (6), 1400–1411. doi:10.2134/jeq2018.03.0107
Tomer, M. D., Moorman, T. B., James, D. E., Hadish, G., and Rossi, C. G. (2008). Assessment of the Iowa river’s south Fork watershed: Part 2. Conservation practices. J. Soil Water Conservation 63 (6), 371–379. doi:10.2489/jswc.63.6.371
Tonitto, C., David, M. B., and Drinkwater, L. E. (2006). Replacing bare fallows with cover crops in fertilizer-intensive cropping systems: A meta-analysis of crop yield and N dynamics. Agric. Ecosyst. Environ. 112 (1), 58–72. doi:10.1016/j.agee.2005.07.003
Traoré, N., Landry, R., and Amara, N. (1998). On-farm adoption of conservation practices: The role of farm and farmer characteristics, perceptions, and health hazards. Land Econ. 74, 114–127. doi:10.2307/3147217
Tsonkova, P., Böhm, C., Quinkenstein, A., and Freese, D. (2012). Ecological benefits provided by alley cropping systems for production of woody biomass in the temperate region: A review. Agrofor. Syst. 85 (1), 133–152. doi:10.1007/s10457-012-9494-8
Turnbow, K. (2021). The impacts of water and sediment control basins (WASCoBs) on water quality near atterberry, Illinois. Carbondale, IL: Southern Illinois University Carbondale.
Valentin, C., Agus, F., Alamban, R., Boosaner, A., Bricquet, J. P., et al. (2008). Runoff and sediment losses from 27 upland catchments in Southeast Asia: Impact of rapid land use changes and conservation practices. Agric. Ecosyst. Environ. 128 (4), 225–238. doi:10.1016/j.agee.2008.06.004
Vanacker, V., Govers, G., Barros, S., Poesen, J., and Deckers, J. (2003). The effect of short-term socio-economic and demographic change on landuse dynamics and its corresponding geomorphic response with relation to water erosion in a tropical mountainous catchment, Ecuador. Landsc. Ecol. 18 (1), 1–15. doi:10.1023/A:1022902914221
Voorhees, W. B., and Lindstrom, M. J. (1983). Soil compaction constraints on conservation tillage in the northern Corn Belt. J. Soil and Water Conservation 28 (3), 307–311.
Wagena, M. B., and Easton, Z. M. (2018). Agricultural conservation practices can help mitigate the impact of climate change. Sci. Total Environ. 635, 132–143. doi:10.1016/j.scitotenv.2018.04.110
Wallace, C. W., Flanagan, D. C., and Engel, B. A. (2017). Quantifying the effects of conservation practice implementation on predicted runoff and chemical losses under climate change. Agric. Water Manag. 186, 51–65. doi:10.1016/j.agwat.2017.02.014
Wild, T. B., and Davis, A. P. (2009). Simulation of the performance of a storm-water bmp. J. Environ. Eng. 135 (12), 1257–1267. doi:10.1061/(ASCE)EE.1943-7870.0000106
Yuan, Y., Locke, M. A., and Bingner, R. L. (2008). Annualized agricultural non-point source model application for Mississippi Delta Beasley Lake watershed conservation practices assessment. J. soil water conservation 63 (6), 542–551. doi:10.2489/jswc.63.6.542
Zhang, L., Hu, Q., and Tang, Z. (2022). Using sentinel-2 imagery and machine learning algorithms to assess the inundation status of Nebraska conservation easements during 2018–2021. Remote Sens. 14 (17), 4382. doi:10.3390/rs14174382
Zhang, Y., Hernandez, M., Anson, E., Nearing, M. A., Wei, H., Stone, J. J., et al. (2012). Modeling climate change effects on runoff and soil erosion in southeastern Arizona rangelands and implications for mitigation with conservation practices. J. soil water conservation 67 (5), 390–405. doi:10.2489/jswc.67.5.390
Keywords: conservation practices, flooding, water quality, climate change, agricultural management
Citation: Srivastava S, Basche A, Traylor E and Roy T (2023) The efficacy of conservation practices in reducing floods and improving water quality. Front. Environ. Sci. 11:1136989. doi: 10.3389/fenvs.2023.1136989
Received: 03 January 2023; Accepted: 19 April 2023;
Published: 15 May 2023.
Edited by:
Dipankar Dwivedi, Berkeley Lab (DOE), United StatesReviewed by:
Matthew Helmers, Iowa State University, United StatesGeorge P. Karatzas, Technical University of Crete, Greece
Copyright © 2023 Srivastava, Basche, Traylor and Roy. This is an open-access article distributed under the terms of the Creative Commons Attribution License (CC BY). The use, distribution or reproduction in other forums is permitted, provided the original author(s) and the copyright owner(s) are credited and that the original publication in this journal is cited, in accordance with accepted academic practice. No use, distribution or reproduction is permitted which does not comply with these terms.
*Correspondence: Tirthankar Roy, cm95QHVubC5lZHU=