- 1Faculty of Life Sciences, Research and Transfer Centre Sustainability and Climate Change Management (FTZ-NK), Hamburg University of Applied Sciences, Hamburg, Germany
- 2Department of Civil, Polytechnic School, Chemical and Environmental Engineering (DICCA), University of Genoa, Genova, Italy
- 3Ticass S.c.r.l, Genova, Italy
- 4IVL Swedish Environmental Research Institute, Kristineberg Center for Marine Research and Innovation, Fiskebäckskil, Sweden
- 5Alfred Wegener Institute, Helmholtz Centre for Polar and Marine Research, Bremerhaven, Germany
- 6Department of Environmental Engineering, Faculty of Life Sciences, Hamburg University of Applied Sciences, Hamburg, Germany
- 7Institute of Environmental Engineering and Building Installations, Lodz University of Technology, Lodz, Poland
- 8CESAM—Centre for Environmental and Marine Studies and Department of Biology, University of Aveiro, Aveiro, Portugal
Among the highly diverse range of biobased polymers, polylactic acid (PLA) received vast attention in recent years due to its versatility for different applications and being the first commercially used polymer produced from renewable sources. Production and application of bio-based, biodegradable plastics will have one of the most crucial roles in tackling worldwide plastic pollution.
Methods: This study is based on integrative ecotoxicological assessment of an innovative PLA-based agricultural mulch film (BPE-AMF-PLA), developed under the H2020 EU project “BIO-PLASTICS EUROPE”, towards organisms from different environmental compartments (soil, fresh water and marine) and from different trophic levels. Such comprehensive evaluation has an overarching goal to promote environmentally safe and sustainable use of these PLA-based plastics for agricultural and other potential applications.
Results: Low-to-no phytotoxicity was obtained in both single-species standardized bioassays, and in a multi-species microcosms experiment. Earthworm reproduction was negatively affected at the lowest test concentration of 0.1% w/w of PLA-based plastic particles. For freshwater Daphnia, reproduction was found a sensitive endpoint, upon exposure to the leachates of the PLA-based plastic. However, the reported toxicity seemed to be caused by the presence of 2-methylnaphthalene, which can be avoided in the production process. As for the marine organisms, algae growth was inhibited with a LOEC = 25 g L−1, whereas test with brine shrimp only revealed stimulation of lipase upon digestion of micro-sized PLA-based plastics. Marine lugworm ingested pristine and UV pre-treated micro-sized plastics, yet without impact either on biological activity, or on the health of the test individuals.
Discussion: The approach used in the present work will contribute to product development, environmental safety and sustainable applications of the PLA-based mulch film BPE-AMF-PLA, in the scope of project BIO-PLASTICS EUROPE. Furthermore, the tools and results obtained in this work are a relevant contribution in the framework development for additional support in the certification of the bio-based polymers, being aligned with European zero waste and non-toxicity strategies, certification, and regulations.
1 Introduction
Bio-based polymers (or bio-based plastics) are one of the most suitable resources to tackle the large environmental challenge produced by plastic pollution (Narancic & O’Connor, 2019). Because of their origin from renewable sources, agricultural byproducts, or microbial sources, bio-based plastics can have the property of renewability, and in some cases biodegradability (Reddy et al., 2013; Madadi et al., 2021), which implies fewer greenhouse gas (GHG) emissions and possible reduced plastic debris generation (European Bioplastics, 2021a). Moreover, biodegradation rate of both fossil-based and bio-based plastics depend on their chemical formation and conditions such as the presence of additives, crystallinity and the presence of proper microorganisms, temperature, moisture, and pH of the environment (Mohee & Unmar, 2007). Currently, bio-based plastics comprise only about 1% of all plastic production, but is expected to grow from 2.2 Mt (million tonnes) in the year 2022 to approximately 6.3 Mt by 2027 (European Bioplastics, 2020). From the variety of biopolymers used currently, polylactic (PLA) is one of the most commercialized in a global context (Rezvani Ghomi et al., 2021).
1.1 Benefits of bio-based plastics
Nevertheless, the largest benefit gained from the use of bio-based plastics is the contention that they provide against climate change (Filiciotto & Rothenberg, 2021). The use of fast-growing microorganisms, such as bacteria or algae may result in a considerable annual reduction in CO2 emissions (Spierling et al., 2018). Additionally, the implementation of agricultural wastes in the production of bio-based plastics could reduce pressure on food supply and security, since the crops would not be used for that purpose (Koul et al., 2022). Thus, with the use of feedstocks such as lignocellulosic or agro-based, only 0.01% of the agricultural area of a total of 5 billion hectares is occupied (European Commission, 2018). Furthermore, provided that there is proper and efficient reuse and recycling, bio-based plastics can strongly contribute to the process of a circular economy with their use, and as a result, contribute to reduce the amount of plastics debris reaching the oceans (Di Bartolo et al., 2021).
1.2 Benefits and constraints behind biodegradation process of novel bio-based plastics
Since conventional plastics are persistent, they will not biodegrade in nature, but disintegrate into microplastics, therefore the removal of plastics once they have entered the ecosystem is often either prohibitively expensive or impossible (Gall and Thompson, 2015; Bråte et al., 2017). For these reasons, the production and use of biodegradable bio-based plastics is an important component to combat worldwide plastic pollution. Something all biodegradable polymers have in common is that the monomers are connected by enzymatically degradable linkages, which can be hydrolysed by various enzymes (Luyt and Malik, 2019). During biodegradation, the biopolymers disintegrate to smaller fragments until, ideally, the polymer is completely mineralized to CO2, H2O and new biomass. Further, during the degradation process, bio-based as well as conventional, fossil-based plastics may leach harmful accompanying and metabolite compounds, like plasticizers and other additives, lubricants, non-intentionally added substances, oligomers and monomers (Asiandu et al., 2021; Zimmermann et al., 2020). Several of these compounds are of high environmental concern because they may exhibit properties like persistence, bioaccumulation, endocrine disruption and toxicity (Stibany et al., 2017). Consequently, during degradation, bio-based and biodegradable plastics may release micro-fragments and harmful compounds into terrestrial and aquatic environments where they can accumulate in organisms via suspended particles, from the consumption of contaminated sediment and foods or directly from the water.
1.3 PLA-based plastics
Among bio-based polymer materials individuated as a potential and suitable replacement for traditional plastics, polylactic acid (PLA) has received much attention in recent years (Castro-Aguirre et al., 2016) due to its versatility for different applications and for being the first commercially used polymer produced from renewable sources (Henton et al., 2005). PLA is an aliphatic polyester that can be obtained from agricultural products and the synthesis takes place through a multistep process starting from the production of lactic acid, followed by the intermediate step of lactide formation, and ending with the polymerization reaction (Hartman, 1998). It shows good processability in standard equipment and a much lower environmental impact in comparison to fossil plastics. However, it has also some disadvantages as far as low toughness, slow degradation rates and hydrophobic characteristics (Farah et al., 2016). The biodegradation rate of PLA (measured as loss of weight at high soil moisture content and air temperature of 40°C) was higher when used as a composite in combination with other biopolymers (i.e., starch), then that of pure PLA (Yu et al., 2020). Improved biodegradability was also observed in combination with chitosan (Vasile et al., 2018). Baltrán-Sanahuja et al. (2021) emphasize that the environmental factors crucial for the process of biodegradation of bio-based plastics (including PLA-based) in soil can have higher variability than those in aquatic, therefore urging for inclusion of reference to the performance under specific environmental conditions within the biodegradability certification. Virgin PLA usually can be blended with different kinds of fillers (Haave et al., 2019; Moliner et al., 2020) to improve their mechanical properties and expand their range of applications (Bledzki and Jaszkiewicz, 2010). Biodegradability, recyclability, compostability, and possible toxicity of PLA-based compounds have been studied in detail by the partners of the BIO-PLASTICS EUROPE funded project, performing specific experiments within different working packages.
1.4 Application of mulch films
The use of PLA-based materials in agriculture for mulch applications has developed only in recent years (Serrano-Ruiz et al., 2021). Studies on the effects of individual bio-based mulches on the growth and development of plants and on the impact on soil microorganisms still need to be thoroughly investigated in order to produce sustainable mulches and assure environmental safety. This work intends to contribute to the study of PLA based compounds for plasticulture applications, helping to shed light on doubts about their toxicity, in order to replace fossil plastics with environment-friendly biobased compounds.
The aim of the current study is to provide a comprehensive ecotoxicological assessment of an innovative PLA-based agricultural mulch film material, BPE-AMF-PLA, developed under the EU H2020 project “BIO-PLASTICS EUROPE,” towards organisms from different environmental compartments such as soil, fresh water and the marine environment, and from different trophic levels, including primary producers, and first and second level consumers (Figure 1). The integrative evaluation of and the discussion on the ecotoxicity of the PLA-based mulch film material will promote its safe and sustainable use as a polymer for agricultural and other potential applications.
Each project partner involved in toxicity testing contributed specific experimental and analytical data, which together form a comprehensive picture on the various aspects of ecotoxicity in a multi-environmental approach. No consistent test was possible due to variety of environments.
2 Materials and methods
2.1 Test material
The material examined in the present work is a PLA-based compound blended with polybutylene adipate terephthalate (PBAT) from a producer NaturePlast SAS (Ifs, France). The ratio of PLA:PBAT is 30:1, as confirmed with nuclear magnetic resonance (NMR) spectroscopy. More detailed characterization regarding 1H- and 13C-NMR spectra can be found in Miksch et al. (2022), the study also carried out in the scope of BIO-PLASTICS EUROPE. This material is intended to be fully degradable in situ, and non-toxic in soil, freshwater, and marine environments, yet its biodegradability in nature is not documented. The enzymatic degradability in seawater of the material was very low under environmentally relevant conditions, whereas hydrolysis rate is 30 nmol·min-1 when incubated with lipase at 30°C (Miksch et al., 2022).
2.2 Ecotoxicity towards soil organisms
2.2.1 Toxicity towards Sorghum saccharatum, Lepidium sativum and Sinapis alba
Phytotoxicity of the bio-based plastics was evaluated according to ISO Standards 18763 (ISO 18763, 2016) with the commercially available Phytotoxkit Solid Samples (order no. TK 61) provided by Microbiotests Inc. (Gent, Belgium). The test included three species of higher plants: one monocotyledon, Sorghum saccharatum (Sorghum, series no. SOS041019), and two dicotyledones, Lepidium sativum (Garden Cress, series no. LES260820) and Sinapis alba (Mustard, series no. SIA020719). The tests were run for 72 h according to ISO Standards 1873. For the control tests the reference OECD soil prepared in agreement with OECD method no. 207 (OECD, 1984) was used only, while for the other tests the plastic particles (3 mm × 2.5 mm) were added to the OECD soil. A 105 g of soil was used in each replication. The soil was saturated up to 100% with deionised water. The concentrations of plastics in the soil were 0.02, 0.095, 0.48, 2.38, and 11.9% w/w. The wide range of concentrations of plastic particles in the soil was selected based upon the literature data concerning the quantity of plastics in the terrestrial compartment (Fuller and Gautam, 2016; Piehl et al., 2018; Scheurer & Bigalke, 2018). Each concentration was tested in three replications for each plant species, while the control test was conducted in six replications per each species test. The lengths of roots and stems as well as Germination Index (GI) were determined. The detailed description of the procedure is presented elsewhere (Liwarska-Bizukojc, 2022a). One-way analysis of variance (ANOVA) was applied to determine statistical differences in the lengths of roots and stems, respectively, exposed to the bio-based plastics and the controls without bio-based plastics at a confidence level of 95% (p = 0.05).
2.2.2 Toxicity towards earthworms Eisenia andrei
The effects of bio-based plastic particles on earthworms were evaluated according to the method OECD 222 (OECD, 2004). In this test, specimens of the earthworm Eisenia andrei were used. They originated from the synchronized culture of Institute of Environmental Protection - National Research Institute (Warsaw, Poland). Ten earthworms were put into the container with 600 g of the reference OECD soil (three replicates) with the bio-based plastic particles (3 mm × 2.5 mm), or without (representing control).
The reproductive output of the earthworms exposed to the test material (in this case the bio-based plastic particles BPE-AMF-PLA) was compared with a control of pure soil. The final concentrations of plastics in the dry soil were 0.1, 0.5, 2.5 and 12.5% w/w that corresponded with the literature data (Fuller and Gautam, 2016; Piehl et al., 2018; Scheurer & Bigalke, 2018). The test comprised two stages. In the first stage, the mortality and the body mass of adult earthworms was determined after 28 days. In the second stage, which lasted for another 28 days, the number of cocoons was counted and the effect on the reproductive ability of earthworms was assessed. Relative changes in body mass of earthworms (RM) exposed to BPE-AMF-PLA were calculated for each concentration tested (Eq. 1).
where: M28 is the mean body mass of the individual earthworm exposed to BPE-AMF-PLA after 28 days of the test and M28,control is the mean body mass of the individual earthworm not exposed to BPE-AMF-PLA (control run) after 28 days of the test. The positive values of RM indicate the increase in earthworm body mass, while the negative values show its decrease. One-way ANOVA was used to determine statistical differences between the number of cocoons found in the soil containing BPE-AMF-PLA and the number of cocoons in the control tests at a confidence level of 95% (p = 0.05).
2.3 Toxicity towards freshwater organisms
The toxicity of bio-based plastic (BPE-AMF-PLA) towards freshwater invertebrates was investigated with the crustacean Daphnia magna applying acute and chronic tests according to the guidelines OECD 202 and OECD 211, respectively. The test medium, ISO water, was prepared according to OECD 202 (2004) and sterilized by autoclaving (Systec, DE-23). Toxicity of mulch film (thickness ≥ 150 µm towards D. magna was tested in two different approaches:
1) Contact test: mulch film pieces of 10 mm × 10 mm were introduced directly into the test,
2) Leaching test: mulch film pieces of 10 mm × 10 mm were incubated for 14 days in ISO water on a horizontal shaker at 20°C in the dark with a shaking frequency of 200 rpm. The leachates were decanted before application to avoid plastic pieces in the test, and the organisms were exposed only to the liquid fraction.
The mulch film concentrations in both experimental approaches ranged from 1.5625 to 50 g L−1. The concentration range was chosen in order to determine the dose-response curves under realistic conditions as close to natural conditions as possible. Lithner et al. (2009, 2011) observed toxic effects (immobility) for D. magna in 9 of 32 products for conventional plastics, with 24-h and 48-h EC50-values ranging from 5 to 80 g L−1. Incubation (KBF 240, Binder) of the D. magna took place under defined conditions at 20 °C ± 1°C and a light-dark rhythm of 8:16 h. The test duration was 48 h for acute and 21 days for chronic test, respectively. During chronic tests daphnids were fed with 16 × 106 cells per day of the algae Chlorella vulgaris. No feeding was performed in acute tests. In accordance with OECD 202 and OECD 211, potassium dichromate was used as the positive control. Data were tested for normality (Kolmogorov–Smirnov test with Lilliefors significance correction) by software GraphPad Prism (Version 9.3.1). The results of BPE-AMF-PLA were compared to determine the toxic effects of treatment using one-way analysis of variance (ANOVA) with a significance level of 0.05.
2.3.1 Acute toxicity towards freshwater invertebrate Daphnia magna
Acute contact and leaching tests were performed according to OECD 202 (2004) using 6-well flat bottom polystyrene plates (Macro plate PS 6 F with lid, Boettger GmbH, Bodenmais, Germany). For contact testing the same concentrations as for leaching tests were used. Four groups of 5 daphnids and 10 mL medium, each, for the respective mulch film concentration were placed on one well-plates. Mulch film leachates were diluted with ISO water in steps of two until the level 1:32 was reached (test concentrations: 50, 12.5, 6.25, 3.125, and 1.5625 g L−1). To prevent evaporation of the medium during acute testing, the well-plates were covered with a non-sterile polyester film (Adhesive Film for Microplates, VWR) and lids. Immobilization of daphnids was recorded after 24 and 48 h pH and oxygen content were measured at the beginning and end of each test (Al15, AQUALITIC).
2.3.2 Chronic toxicity towards freshwater invertebrates Daphnia magna
Chronic tests of mulch film toxicity towards D. magna were carried out in 100-mL glass beakers with ten replicates of the respective concentration. Therefore, one daphnid in 50 mL medium was incubated according to OECD, 2012. The concentrations of mulch film contact and leachate tests were 50, 12.5, 6.25, 3.125, and 1.5625 g L−1. Observation of offspring was performed 5 times a week (Monday till Friday). Medium was renewed three times a week and the daphnids were fed with 16 × 106 cells per day of the microalgae Chlorella vulgaris. pH levels and oxygen content were monitored before and after every medium exchange.
2.4 Toxicity towards marine organisms
2.4.1 Toxicity towards marine microalgae Phaeodactylum tricornutum
Leachate of mulch film BPE-AMF-PLA (thickness ≥ 150 µm) was prepared by cutting the film without pre-washing into pieces of 10 mm × 10 mm according to Lithner et al. (2009). Five g of mulch film pieces were placed into a 250-mL glass bottle and 100 mL artificial ISO standard seawater (ASW) were added (DIN, 2015). The bottle was placed on a horizontal shaker (neoLab-Orbital-Shaker, Plattform 409 mm × 297 mm, 10 mm Amplitude) at 200 rpm for 14 days at 20°C ± 1°C in the dark. Afterwards, the leachate was diluted with ASW in a two-step procedure to the lowest concentration of 0.39 g l−1 (1:128). Concentration of leachates ranged from 0.39 g l−1 to 50 g l−1 since Luo et al. (2019) observed growth inhibition of marine algae at 1.6 g microplastic per l. To ensure the observation of effects the concentration range was increased compared to Luo et al. (2019). Algae toxicity tests with the marine algae Phaeodactylum tricornutum were performed according to Ratte et al. (2016) in a miniaturized form on 24-well plates with 3,5-Dichlorophenol (3,5-DCP) as positive control and ASW as negative control. During the test period the well-plates were placed on shakers (IKA MTS 2/4) at 120 rpm and incubated at 20°C ± 1°C with a constant light intensity of 80 μmol m−2·s−1 (Climate chamber ICH750L, Memmert). Growth inhibition of algae was observed after 24, 48 and 72 h by measuring the fluorescence (Tecan infinite F200Pro, Software i-control 1.8 SP1). Kolmogorov–Smirnov test with Lilliefors significance correction was used to test for normality (GraphPad Prism, Version 9.3.1). For the determination of toxic effects of BPE-AMF-PLA an ANOVA with a significance level of 0.05 was used. Organic contaminants in the test media were analyzed by gas chromatography-mass spectroscopy (GC: 7890A GC system, Agilent Technologies; quadrupole MS: 5975C Inert XL MSD with Triple-Axis Detector) from the leaching media and from the two highest concentrations (50 and 25 g L−1) at the end of each test.
2.4.2 Toxicity towards brine shrimp Artemia nauplii
Brine shrimp, Artemia spec., are established model organisms in ecophysiological and ecotoxicological research (Nunes et al., 2006). Brine shrimp may either bear live nauplii or produce stress-resistant dormant eggs (“cysts”) from which the larvae hatch under favorable conditions. For our experiments, nauplii were raised from cysts of Artemia persimilis (Art. no, 10745, REBIE-Zoologischer Versandgroßhandel, Bielefeld, Germany) as per the supplier’s instructions. The medium was natural seawater (32 PSU) filtered through 0.45 µm membrane filters, hereafter referred to as filtered seawater (FSW).
Maintenance and exposure experiments were carried out in non-pyrogenic and non-cytotoxic 24-well tissue culture plates (Sarstedt, NC 28658, USA). Before the start and between experiments, the tissue culture plates were stored submersed in FSW for 24 h to leach out any soluble chemicals. The 24-well plates were incubated in a KBS-E400 Incubator (RUMED, Rubarth Apparate GmbH, Germany) at 24°C. A LED panel (Tween Light, 16 W, 30 cm × 30 cm × 5 cm) provided continuous and homogeneous illumination.
2.4.2.1 Preparation of microparticles
BPE-AMF-PLA pellets (5 mm) were ground in liquid nitrogen with a cryogenic mill (6775 Freezer/Mill, SPEX SamplePrep, USA). The protocol involved 15 min of pre-cooling and 4 cycles of milling (2 min each) with 2 min of cooling in between. One gram of the plastic material was processed per run. The ground particles were separated with a stainless-steel sieve to obtain the fraction smaller than 200 µm.
2.4.2.2 Ingestion of microplastics
To test whether Artemia nauplii ingest microplastics, specimens were incubated with fluorescent polymer beads (Fluoro-Max™, Fremont, CA 94538 USA, 9.9 µm diameter) and BPE-AMF-PLA microparticles, respectively. Up to ten freshly hatched Artemia nauplii each were transferred into the wells of the cell-culture plates containing 3 mL FSW. Five µl of the microplastic suspensions (0.1% w/v) were added to each well and the Artemia nauplii were left to feed for 2 h. Thereafter, the nauplii were examined and photographed under a fluorescence microscope (Nikon SMZ 25). The high concentration of particles was chosen to clearly observe and document ingestion or avoidance by the nauplii and to test whether or not these particles may induce biochemical reactions in the digestive tract.
2.4.2.3 Exposure of Artemia nauplii
Artemia cysts were incubated in seawater for 24 h at 24°C to hatch. About 300 of the hatched Artemia nauplii were transferred to each well of a 24-well plate, which contained 300 µL FSW and 1.5 mL of plastic particle suspension (3 g l−1) per well. The control contained only FSW without micro-particles. The well-plate was incubated for 24 h under permanent illumination. After incubation, 75 randomly taken Artemia were transferred from each well to separate 1.5-mL reaction tubes with 300 µL of the incubation fluid. The Artemia were homogenised with a micro-pestle and centrifuged for 10 min at 20,000 g and 4°C. The supernatant was pipetted into new 1.5-mL reaction tubes and stored at −80°C until further use.
2.4.2.4 Enzyme assays of Artemia nauplii
MUF (4-methyl-umbelliferone) derivatives of butyrate (C4) and oleate (C18) were used as fluorogenic substrates for esterase and lipase enzymes. The substrates were dissolved in dimethyl-sulfoxide (DMSO) and then diluted with 0.1 M Tris/HCl-buffer (pH 7.5). The stock solution contained a substrate concentration of 0.1 mmol L−1 and 2% DMSO. The assay was run in triplicate in 96-well plates (3 wells per plate). The Artemia extract (20 µL) was given into the wells of the plate and 250 µL of the substrate stock solution subsequently were added. The fluorescence was measured every 30 s for 20 min at 25°C (Fluoroskan Ascent FL, Thermo Fisher Scientific). The MUF standard curve was prepared from 0 to 35 μmol l−1 and contained 2% DMSO. Statistical analyses were done with two-tailed t-tests on data sets of three replicates.
2.4.3 Toxicity towards the marine infaunal lugworm Arenicola marina
The effects of microparticles from BPE-AMF-PLA and LDPE, a conventional, fossil-based plastic used for agricultural mulch films, was investigated on the marine infaunal polychaetae lugworm Arenicola marina. A. marina is a non-selective deposit feeder, found in high densities in shallow, sandy to muddy bays around Europe, likely to ingest large amounts of microplastics accumulated in sediments in polluted areas. A. marina and sediment were collected from mudflats on the Swedish west coast (mean worm weight: 4.1 ± 1.0 g, n = 50; no significant differences between treatments; One-way ANOVA, F = 0.39, df = 4, p = 0.81). Sediment was dry sieved (2 mm) for removal of macro fauna and worms were acclimatized on sieved sediment before the start of the experiment. Flow-through of surface seawater was used at all times, with experimental conditions resembling the worms’ natural environment (temperature 15°C, oxygen level 9.7 ± 0.4 mg L−1, with ambient salinity fluctuation at 26–31 PSU and a 10:14 h light-dark regime). Microplastics prepared with a cryogenic mill as described in 2.4.3.1, were sieved through 100 and 300 µm nylon filters (Bopp Utildi, Sweden) to attain 100–300 µm particles used in these experiments, i.e., within the size range of natural food particles ingested by A. marina. Pristine BPE-AMF-PLA and LDPE microplastics were compared with those pre-treated with UV-A (350–400 nm, peak at 370 nm, intensity ca. 12 W/m2) and UV-B (290–315 nm, peak at 300 nm, intensity ca. 1.8 W/m2) light for 7 days, to be able to compare whether ecotoxicological effects are affected by this simulated weathering process (by use of Philips fluorescent lamps; Actinic BL TL-K 40W/10-R for UV-A light, and TL 20 W/12 RS SLV/25 with a pre-burnt cellulose acetate UV-C filter (Nordbergs Tekniska AB, Vallentuna, Sweden) for UV-B light, at 10 cm distance). All pristine and UV treated microplastics were stained with Nile Red (Sigma Aldrich) fluorescent dye (dissolved in methanol (Merck, for analysis EMSURE® ACS,ISO,Reag. Ph Eur) at 10 µg per mL−1) for 30 min at 37°C, to enhance subsequent microplastic identification and analysis.
A. marina was exposed to either of five microplastic treatments; i.e., surface sediment spiked with pristine BPE-AMF-PLA and LDPE, or UV-treated BPE-AMF-PLA and LDPE, at a concentration of 0.1% per dry weight sediment, or to sediment without added microplastics for the controls. The experimental set up comprised 10 replicate aquaria per treatment with one worm per aquarium (in total 50 aquaria and worms) (Figure 2). Each aquarium (15 cm × 11 cm × 12 cm) was filled with a 6 cm bottom layer of clean sediment (i.e., no microplastics added) and a 2 cm-layer of surface sediment spiked with microplastics, or clean control sediment. A thin layer of 0.5 cm clean sediment covered the spiked or control surface sediment, to prevent microplastics from floating away, and sediment was let to settle for several hours before introducing a 3.5 cm layer of gentle flow-through of surface seawater. Sediment and microplastics were pre-incubated for 4 days before the introduction of lugworms, to enable formation of natural biofilm. At the start of the experiment, one A. marina (pre-purged of gut content for 24 h) was added to each aquarium. Lugworms were exposed to microplastics for 15 days, to assess effects on their health and biological activity.
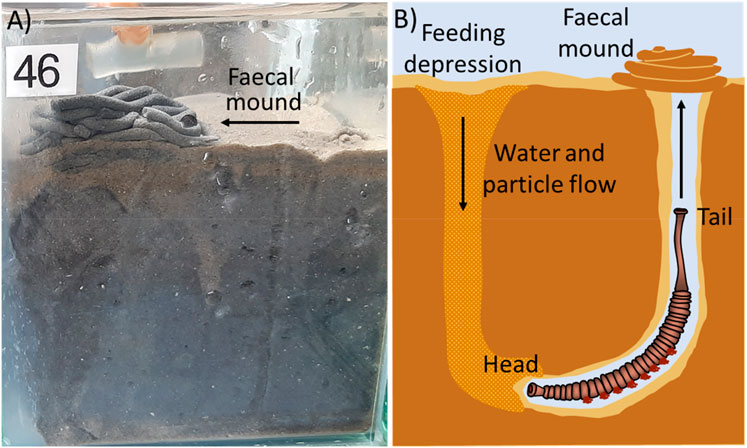
FIGURE 2. Experimental set up with the lugworm Arenicola marina in individual aquaria with sediment surface layer spiked with microplastics (A). The lugworm creates a U-shaped burrow in the sediment, feeding and defecating on the sediment surface (B).
The effect of microplastics was tested, using the following effect endpoints: a) time to initiate borrowing (the time it took for A. marina to start burrowing into the sediment), b) time to complete burrowing (time from start burrowing until completely buried in the sediment), c) overall feeding rate (averaged volume of faecal mound produced per ww lugworm and hour), d) change of weight {[(final ww-initial ww)/initial ww] ×100}, and e) induction of oxidative stress (lipid peroxidation, LPO). Induction of LPO was measured as an increase in malondialdehyde (MDA) and 4-hydroxyalkenals (4-HNE) concentrations (i.e., toxic by-products of LPO) in lugworm soft body tissue homogenate as an indication of oxidative stress (using G-bioscience LPO assay kit, combined with DetectX bicinchoninic acid (BCA) protein assay kit to quantify total protein content of the samples). To test for statistical differences between treatments, one-way analysis of variance (ANOVA) was applied, after log or arcsin transformation of data if required, followed by Tukey HSD post hoc test, using SPSS v. 26 (IBM) and statistical significance set to p < 0.05. Sediment and faecal mound samples were collected at the end of the 15-day exposure period and stored at 4°C for subsequent microplastic analyses for confirmation of microplastic ingestion. Lugworms were collected, rinsed and gut purged in filtered (0.45 µm) seawater for 24 h at 15°C, weighed and stored at either −80°C for oxidative stress assay, or at −20°C for microplastic analysis. Microplastics were extracted by gentle enzymatic treatment according to the modified method of von Friesen et al. (2016). Briefly, using 1 pancreatic enzyme capsule (Creon® 25000 pankreatin, BGP Products AB, Stockholm) per 15 mL 1 M Tris buffer, pH 8.0 (Biotechnology Grade, VWR Life Science), incubating samples on shaker at 37.5°C for 24 h. Sediment and faecal mound samples from all treatments were density separated by use of saturated sodium iodide (NaI) solution (1.8 kg L−1) in glass funnels, subsequently filtering the supernatant onto 20 µm nylon filters (Bopp Utildi, Sweden), and were analysed for microplastic >20 µm content by use of fluorescence stereo microscope (Leica MZ FLIII).
3 Results
3.1 Toxicity of bio-based agricultural mulch towards soil organisms
3.1.1 Effect of bio-based agricultural mulch on germination and early growth tests with Sorghum saccharatum, Lepidium sativum and Sinapsis alba
BPE-AMF-PLA did not affect seed germination of any of the three higher plants studied. The germination index (GI) ranged from 93% to 100% depending on the plant and the concentration of the bio-based plastic particles in the soil (Figure 3A). The values were at the same level as those determined for the control runs.
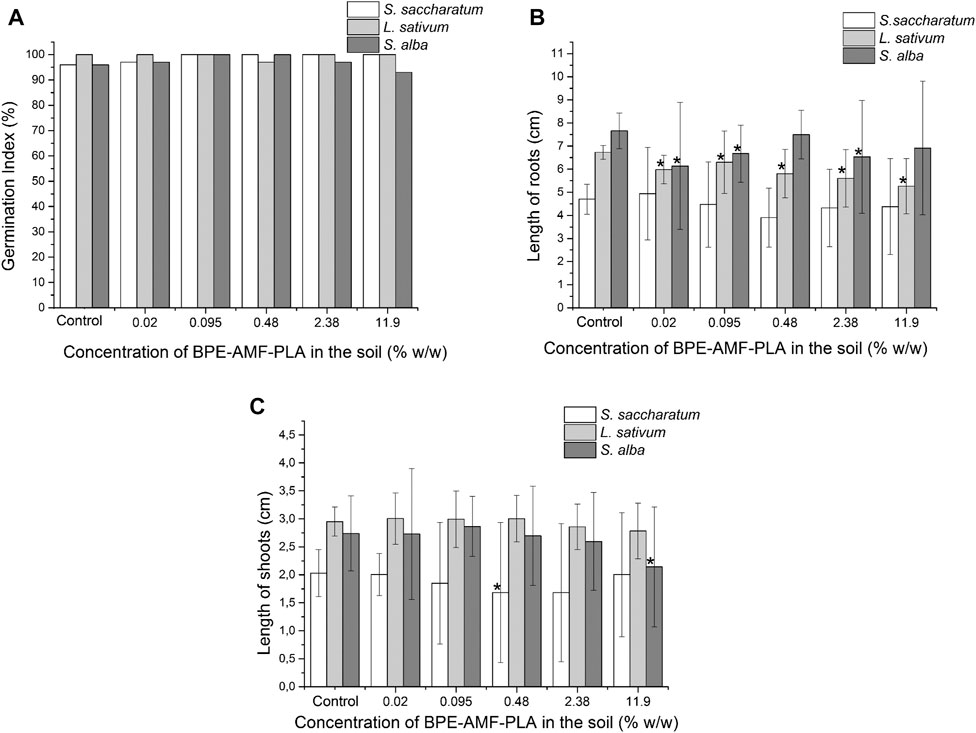
FIGURE 3. (A) Effect of BPE-AMF-PLA on seed germination of three higher plants (S. saccharatum L. sativum, S. alba). Standard deviation (SD) was below 2% in each case. (B) Effect of BPE-AMF-PLA on root growth of three higher plants (S. saccharatum, L. sativum, S. alba). The error bars reflect the values of SD. The asterisks refer to the statistically significant difference compared to control. (C) Effect of BPE-AMF-PLA on shoot growth of three higher plants (S. saccharatum, L. sativum, S. alba). The error bars reflect the values of SD. The asterisks refer to the statistically significant difference compared to control.
Neither root growth nor shoot growth of the monocotyledonous plant S. saccharatum were affected in the presence of BPE-AMF-PLA in the soil (one-way ANOVA, p > 0.05, n = 3, Figure 3B). The same was found for the shoot growth of both dicotyledonous plants L. sativum and S. alba (Figure 3C). However, the root growth of both dicotylodonous species was inhibited (Figure 3B). The length of roots exposed to BPE-AMF-PLA was statistically lower than that of the control without BPE-AMF-PLA material (p < 0.05). The reduction in the length of roots varied from 6.4% to 21.8% in the case of cress (L. sativum) and from 2.1% to 19.9% in the case of mustard (S. alba).
3.1.2 Toxicity towards earthworms Eisenia andrei
No mortality of earthworms appeared after 28 days and after 56 days, irrespective of the concentration of BPE-AMF-PLA particles in the soil. BPE-AMF-PLA did not contribute to the decrease of the body mass of earthworms tested (Table 1).

TABLE 1. Relative change in earthworm body mass, presented as mean ± standard deviation of the mean.
However, presence of BPE-AMF-PLA in the soil significantly affected the reproduction ability of E. andrei. Compared to the controls, the number of cocoons decreased by 63.8%–71.4% depending on concentration of the bio-based plastic in the soil (Figure 4). The differences in the number of cocoons between the tests with BPE-AMF-PLA and the control tests were statistically relevant (one-way ANOVA, p < 0.05). The ‘lowest observed effect concentration’ LOEC is equal to 0.1% w/w of BPE-AMF-PLA particles.
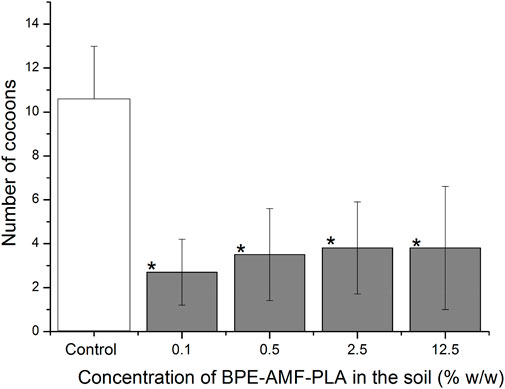
FIGURE 4. Number of cocoons from the earthworm species E. andreiexposed and not exposed to BPE-AMF-PLA. Error bars represent the values of SD (n = 3). The asterisks refer to the statistically significant difference compared to control.
3.2 Toxicity of bio-based agricultural mulch towards freshwater invertebrates
3.2.1 Acute in vitro toxicity to Daphnia magna
Effective concentrations 50 (EC50) values of D. magna exposed to potassium dichromate for 48 h (positive control) ranged from 0.8 to 0.9 mg L−1. The pH values were between 7.6 and 8.5. These values are in accordance with OECD 202 (2004), confirming the validity of the assay. The immobilization in standard reference water (negative control) was 0%. The acute contact and leaching tests showed no immobilization of D. magna after 24 and 48 h, respectively.
3.2.2 Chronic toxicity to Daphnia magna
The mortality of daphnids in the negative control of the first tested BPE-AMF-PLA charge (n = 10) of the chronic contact tests was 0% with an offspring of 7.4 ± 0.45 neonates per daphnid during 21 days. The pH ranged between 7.8 and 8.6 during the test period, which is in accordance with validity requirements (OECD, 2012). The chronic contact tests with first charge of the bio-based mulch film (BPE-AMP-PLA) showed no significant deviation from the negative control (Table 2). The mulch film leaching toxicity tests showed a decreasing number of offspring by increasing concentrations of BPE-AMF-PLA and the observed LOEC was 1.5625 g L−1. Therefore, leachates of the first charge of BPE-AMF-PLA provoked adverse effects towards D. manga and influence the reproduction of the limnic invertebrate. The second charge of BPE-AMF-PLA leachates did not provoke toxic effects towards D. magna.
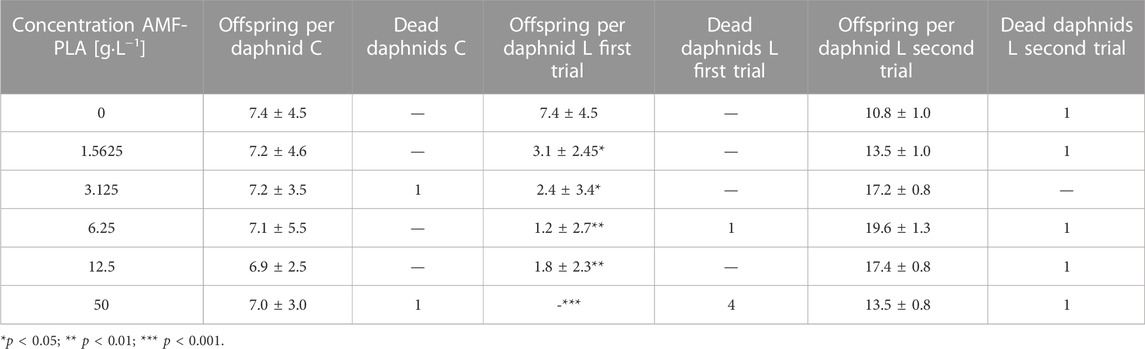
TABLE 2. Offspring and survival of D. magna after exposition to AMF-PLA contact (C) and leachates (L) for 21 days.
3.3 Toxicity of bio-based agricultural mulch towards marine algae and invertebrates
3.3.1 Toxicity towards marine algae Phaeodactylum tricornutum
The tests with P. tricornutum were performed after the results of the first test with D. magna were available. For the second batch, the production process was changed, thus avoiding contamination of the material. Only the second batch was used for the tests. The initial pH of the BPE-AMF-PLA leachates was between 7.9 and 8.0 for all dilutions, which is within the optimal pH range according to DIN, 2015 (optimal 8.0 ± 0.2). During the tests, the pH value increased up to 8.4, which also complies with the guideline (the pH value must not have increased by more than 1.0). The cell density in the negative control increased exponentially by a factor of 16 ± 2 (DIN, 2015). EC50-values ranged from 1.27 to 1.33 mg L−1 for 3,5-DCP as positive control. Growth inhibition was observed for BPE-AMF-PLA mulch film material (F (7, 64) = 11.65, p < 0.001). The daily growth rate of P. tricornutum was 0.9–1.0. NOEC was 12.5, and LOEC was 25 g of BPE-AMF-PLA per liter (Figure 5). No harmful substances were detected by GC-MS in the leaching medium.
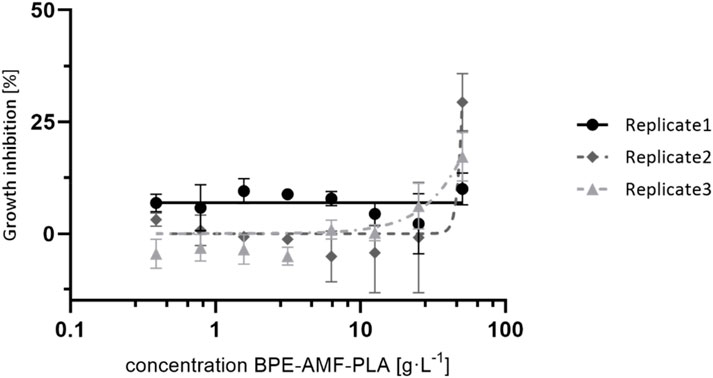
FIGURE 5. Concentration-response curves for bio-based mulch film in chronic marine algae test with P. tricornutum after 72 h of exposure. Plotted are the mean growth inhibition and standard deviations of three independent replicates.
3.3.2 Effects on digestive enzyme activities of Artemia persimilis nauplii
Artemia nauplii ingested both types of micro-particles. Compared to the empty gut (Figure 6A), ingested fluorescent microbeads appeared bright green (Figure 6B) and ingested BPE-AMF-PLA particles appeared densely dark packed in the gut (Figure 6C).
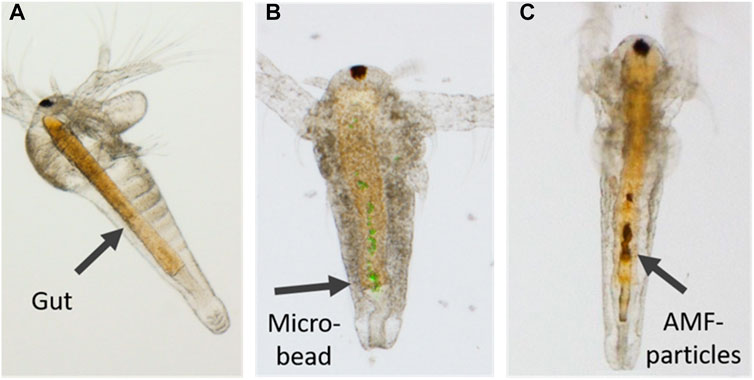
FIGURE 6. Freshly hatched Artemia persimilis nauplii with (A) empty gut, (B) ingested fluorescent microbeads (9.9 µm), and (C) ingested BPE-AMF-PLA microplarticles.
The average esterase activity of the control group (FSW without micro-particles) was 30.3 ± 5.8 mU ind−1 (Figure 7A). Artemia nauplii exposed to BPE-AMF-PLA showed similar activities of 30.8 ± 1.1 mU ind−1 (df = 4, t = 0.164, p = 0.878). Lipase activity increased significantly from 0.48 ± 0.13 mU ind−1 in the control group to 0.94 ± 0.18 mU ind−1 after ingestion of BPE-AMF-PLA (Figure 7B, df = 4, t = 3.604, p = 0.022).
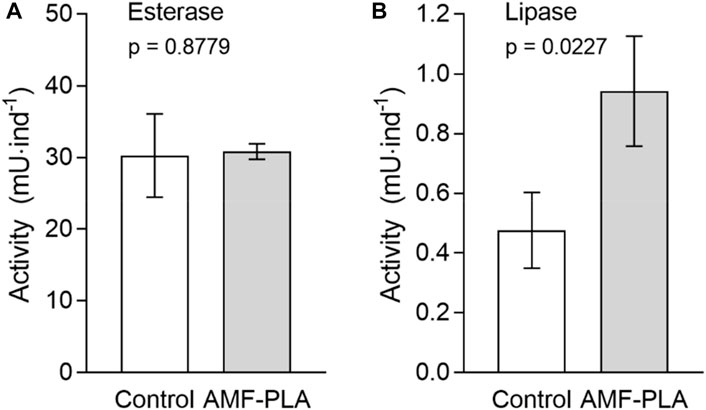
FIGURE 7. Activities of (A) esterase (C4) and (B) lipase (C18) in Artemia persimilis nauplii of the control and nauplii exposed to BPE-AMF-PLA (mean ± SD, n = 3).
3.3.3 Effects on biological activity and health of Arenicola marina
No adverse effects on Arenicola marina biological activity or general health were detected after 15 days exposure to BPE-AMF-PLA microparticles (100–300 μm, at 0.1% per sediment dry mass).
A. marina ingested all types of microplastics, shown by the presence of microplastics in the faeces of lugworms from all experimental treatments but the controls. Lugworms from the different treatments showed no significant difference in the time it took to initiate borrowing (One-way ANOVA, F = 1.88, df = 4, p = 0.13; Figure 8A), however, the time it took to complete burrowing varied significantly between treatments (One-way ANOVA, F = 3.95, df = 4, p = 0.008; IVL Figure 8B).
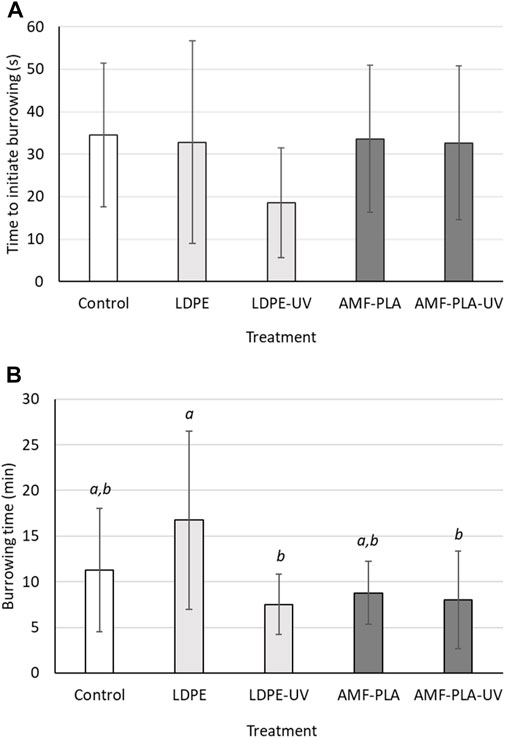
FIGURE 8. Time to initiate burrowing (s) (A) and time to complete burrowing (min) (B) for lugworms added to sediment spiked with different microplastic treatments, i.e., pristine or UV-treated LDPE and AMF-PLA, or to untreated control sediment. Significantly separated groups (p < 0.05) indicated with different letters above bars (One-way ANOVA and HSD Tukey post doc test; bars show means ± SD, n = 10, except AMF-PLA-UV in A where n = 9).
Lugworms on sediment with pristine LDPE microplastics took significantly longer time to bury, compared to those on sediment with UV-treated microplastics of both kinds (Tukey HSD, p < 0.05). There was no significant difference in feeding rate between treatments, investigated by measuring the volume of faecal mounds produced per wet weight lugworm and hour, average over the 15 days of exposure (One-way ANOVA, F = 0.22, df = 4, p = 0.92; Figure 9).
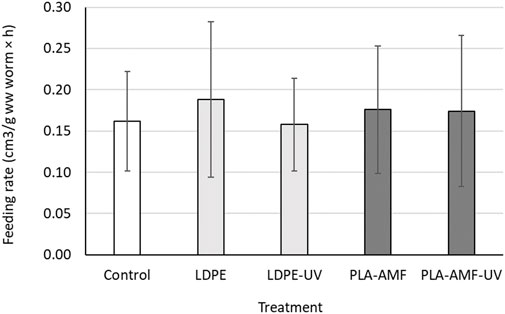
FIGURE 9. Feeding activity of Arenicola marina averaged over 15-days of exposure to sediment spiked with LDPE and AMF-PLA microplastics (0.1% based on dw sediment), either pristine or pre-treated with UV-light, or to untreated control sediment. Feeding activity is defined as average volume faeces produced per hour, divided by weight of each lugworm (bars show means ± SD, n = 9, except AMF-PLA-UV, which had n = 10).
The mortality was low throughout the experiment (0–1 individuals per treatment) and there were only minor changes in weight of the lugworms after 15 days of microplastic exposure (mean weight change: 0.039 ± 0.32 g, corresponding to a 0.36% weight change), with no statistical differences between treatments (One-way ANOVA, F = 1.32, df = 4, p = 0.28).
A minor increase of lipid peroxidation (LPO) could be detected in lugworm soft body tissue, but there was no indication of oxidative stress at microplastic exposure, instead MDA and 4-HNE concentrations were similar or even lower compared to the controls (One-way ANOVA, F = 1.70, df = 4, p = 0.21; Figure 10).
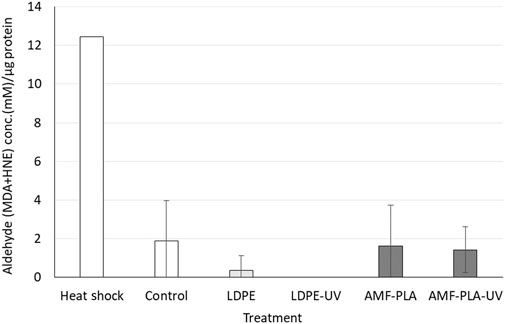
FIGURE 10. Oxidative stress in Arenicola marina soft body tissue after exposure to microplastics, measured as concentration (mM) of malondialdehyde (MDA) and 4-hydroxyalkenals (4-HNE) (i.e., toxic by-products of lipid peroxidation) per µg protein. Heat shock represents tissue homogenate from a lugworm not included in the experiment but pre-heated at 50°C for 1.5 h, used as a positive control (bars indicate means ± SD, n = 4 for LDPE, LDPE-UV, AMF-PLA-UV, n = 3 for Control, AMF-PLA).
3.3.4 Summary of the results
As a summary of the results of this comprehensive ecotoxicological study, Table 3 provides the list of the experiments carried out in the three environmental compartments (soil, freshwater, marine), stating the organisms and/or test species, names of the tests/bioassays (standardized or well-established), indication if works are previously published or not in the scope of BIO-PLASTICS EUROPE project, test endpoints and concentration ranges.
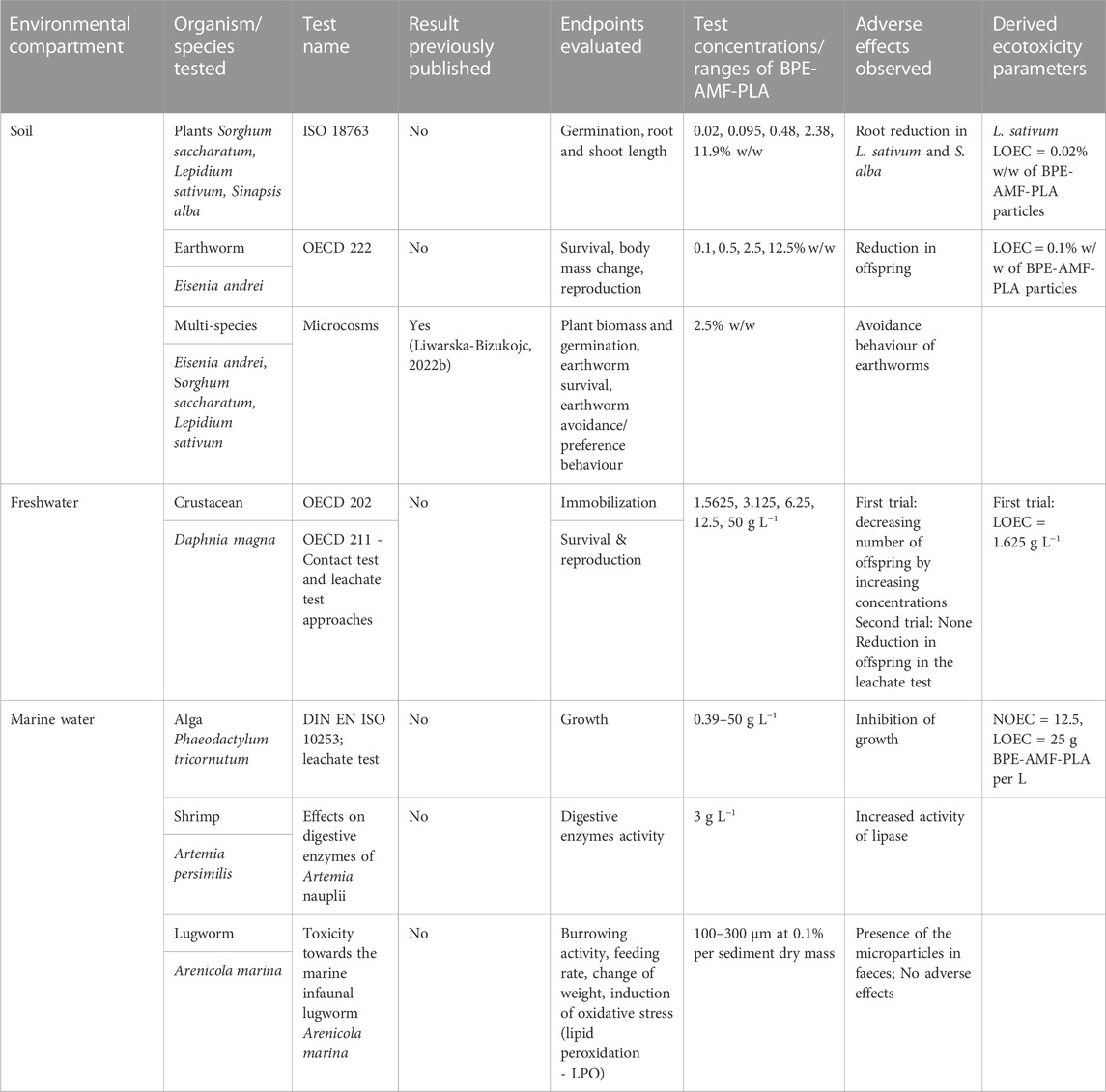
TABLE 3. Summary and the main outcomes of the ecotoxicity experiments conducted with BPE-AMF-PLA bio-based plastics in the scope of BIO-PLASTICS EUROPE project. LOEC—lowest observed effect concentration, NOEC—no observed effect concentration.
The table also reports the main (adverse) effects obtained in each bioassay, including the derived ecotoxicity parameters, where applicable (LOECs and NOECs; Table 3). The plant responses in a single-species bioassays were not validated with the microcosms approach, the latter showing no adverse impact of BPE-AMF-PLA on the tested species. However, the earthworm responses suggest a negative impact on the population level, including the risk to the habitat function of soil in the presence of the tested bio-based plastics. Reduction in daphnid reproduction when subjected to the leachates in comparison to the no-effect when exposed in the contact assay, emphasize the need to further address various environmental scenarios and develop methodologies that could be proxies for weathering or ageing of bio-based plastic films. Adverse effects on algae as primary producers raises concern on the effects on marine ecosystem, as an indirect sink of bio-based plastic and/or bio-microplastics.
4 Discussion
4.1 Toxicity towards soil organisms
The current results are in accordance with the published study from the higher tier approach with the terrestrial microcosms, being the integrative part of this ecotoxicological assessment of BPE-AMF-PLA within BIO-PLASTICS EUROPE. BPE-AMF-PLA did not deteriorate seed germination processes of any of the two plants (sorgho and cress) used in the microcosm tests (Liwarska-Bizukojć, 2022b). Also, the shoot fresh mass and shoot length of these plants were not affected. The differences between the fresh mass or the length of shoots exposed to BPE-AMF-PLA and the fresh mass or the length of shoots not exposed to this material were not statistically significant (p > 0.05) (Liwarska-Bizukojć, 2022b). BPE-AMF-PLA did not contribute to the mortality of E. andrei, resulting in survival of all test individuals. Also, the body mass of earthworms exposed to BPE-AMF-PLA was not affected. The significant differences in the depth distribution of earthworms between the small-scale terrestrial model eco-systems (STMEs) containing the particles of BPE-AMF-PLA and the control STME were observed. The presence of the bio-based plastics favoured the downward movement of earthworms Liwarska-Bizukojć, 2022b). This is an indication on the need for the integrative approaches that allow for assessment of organisms’ interactions under bio-based plastics application, and additional endpoints, including the impact on soil habitat function (i.e., using avoidance behaviour as indicator).
4.2 Toxicity towards freshwater invertebrates
The bio-based mulch film did not provoke acute toxic effects towards D. magna. These results are in accordance to Lithner et al. (2009, 2011) as most of the conventional plastics tested in the studies had EC50 values higher than 250 g L−1. There was a lack of the adverse effects in the acute test, but the reduction in offspring is aligned with the study of Schrank et al. (2019).
A strong effect was provoked by mulch film leaching tests since the leaching of accompanying and metabolite compounds like plasticizers might be harmful for daphnids. Therefore, the chemical analysis of the leaching medium detected an unusually high concentration of 186 µg 2-methylnaphthalene leaching from 1 g mulch film. Since 2-methylnaphthelene provoked immobilization of D. magna in acute tests (Bobra et al., 1983), mortality during chronic leaching tests was probably caused by the contamination of the mulch film with 2-methylnaphthlene. However, 2-methylnaphthalene was not an additive of the mulch film material but could be traced back to a contamination of the material with lubricating oil in the manufacturing process of the film. By repeating the chronic contact and leaching tests of bio-based mulch film with a new charge, no toxic effects were observed and no 2-methylnaphthalene could be detected by GC-MS.
4.3 Toxicity towards marine invertebrates
Artemia nauplii are suspension feeders, which ingest a wide range of digestible and indigestible particles (Bour et al., 2020). Similar to our study, the closely related Artemia franciscana ingested particles in the size range of 6.8–27.5 µm (Kokalj et al., 2018). Most of the particles were egested after 24 h. Only a small amount remained in the intestine after 72 h (Eom et al., 2020).
Digestive enzymes play a crucial role in the utilization of food and, thus, energy metabolism. Changes in diet or starvation can affect digestive enzyme activity in various invertebrate species, including crustaceans and molluscs (Jones et al., 1997; Johnston & Freeman, 2005; Kreibich et al., 2008; Koussoroplis et al., 2017, Trestrail et al., 2021). Likewise, ingestion of microplastic particles has been shown to alter digestive activities in, e.g., crustaceans and fish (Gambardella et al., 2017; Romano et al., 2018; Korez et al., 2019; Han et al., 2021).
Esterases are a diverse group of enzymes, capable of hydrolysing ester bonds with wide substrate specificity. Herbivorous, omnivorous, and detritivorous organisms use esterases to degrade tannins and phenolic compounds (Hübner et al., 2015). An increase in esterase activity has been reported in the marine isopod Idotea emarginata after ingestion of food enriched with PMMA particles (Korez et al., 2019). Binding sites of esterases are present in the PMMA polymer. However, the biochemical background of the hydrolysis reaction is unknown. Exposure of Artemia nauplii to BPE-AMF-PLA caused no change in esterase activity as compared to the control animals although the polymer is linked by ester bonds.
Lipases hydrolyze longer-chained substrates than esterases. They usually split triacylglycerides into glycerol and fatty acids by hydrolyzing the ester bonds (Rivera-Pérez et al., 2011). Lipase activity in Daphnia magna increased when food of poor quality was given (Koussoroplis et al., 2017). The authors hypothesized that digestive enzyme secretion might be homeostatically controlled to ensure a sufficient uptake of the most limiting nutrients.
BPE-AMF-PLA is a blend of PLA and polybutylene adipate terephthalate (PBAT). Both PLA and PBAT contain ester groups. However, exposure to pure PLA does not enhance lipase activity in Artemia nauplii (data not shown). Therefore, degradation of PBAT within the BPE-AMF-PLA bio-based plastics may be more likely. Even though these results were unexpected, lipases were previously reported to degrade plastics. Several studies showed that some aliphatic polymers are degraded by bacterial lipases (Tan et al., 2021).
The results of the current study complement in-vitro observations by Miksch et al. (2022) who found that BPE-AMF-PLA is readily hydrolyzed by isolated lipase but not by esterase from micro-organisms. Apparently, ingestion of BPE-AMF-PLA microparticles by Artemia nauplii selectively activates the digestive system. Probably, the liberation of bio-based plastic oligomers stimulates the expression of lipase to enzymatically degrade the biopolymer. Whether the resulting oligo- or monomers can be metabolized as valuable energy source or the elevated enzyme activity is a false and metabolic costly reaction remains to be investigated.
Mulch films accidently or deliberately released to the environment will eventually generate microplastic particles, induced by weathering and fragmentation processes. Microplastics that end up in marine waters will likely sink to the bottom and accumulate in sediments that act as a sink, with possible impacts on benthic fauna. Indeed, high concentrations of conventional microplastics have been measured in sediments along the Spanish coast in areas with intense agricultural industry (Dahl et al., 2021). The marine lugworm A. marina is a non-selective deposit feeder, occurring at high densities in shallow, sandy to muddy bays around Europe, likely to ingest large amounts of microplastics in such polluted areas. Lugworms are important bioturbating bioengineers, as well as important food item for fish and seabirds and thus functions as a vector for the transfer of plastics and chemicals from sediments to higher trophic levels (Cadée, 1976). Thus, investigating effects of microplastics of BPE-AMF-PLA and conventional mulch film plastics on A. marina is of high ecological relevance. Here, we found no adverse effects on A. marina biological activity or general health at exposure to BPE-AMF-PLA or fossil-based LDPE microplastics mixed with surface sediment to 0.1% dw. A. marina ingested all microplastics, as shown by their presence in lugworm faeces, but there were no effects on burrowing or feeding behaviour of the pristine microplastics, and none of the microplastics affected the body mass of lugworms or induced oxidative stress or mortality, contradicting any negative impacts related to chemical exposure or dilution of edible organic material of the sediment at this concentration. We used induction of lipid peroxidation (LPO) as a measure of oxidative stress at microplastic exposure. LPO is a well-established example of oxidative damage in cell membranes, lipoproteins and other lipid-containing structures, often used as a biomarker related to pollutants in marine invertebrates (Lesser, 2006; Hannam et al., 2010). The great induction of LPO by heat shock treatment, used here as a positive control, validates the bioassay for this species, but other biomarkers may be more sensitive for stress responses induced by microplastic pollution not detected here.
Furthermore, microplastics that are affected by different abiotic and biotic processes in the environment undergo alterations in their physical and chemical characteristics that might affect their toxicity. Both increases and decreases in toxicity have been observed after UV-irradiation of different microplastics (Bejgarn et al., 2015; Simon et al., 2021). We found no effect of UV-weathering on BPE-AMF-PLA. However, it took significantly longer time for lugworms to bury in sediment mixed with pristine LDPE microplastics, compared to sediment mixed with UV-aged microplastics (Figure 8B). A possible explanation could be that A. marina senses and therefore avoids sediment contaminated with LDPE to minimize exposure, but that this effect is rescinded by an increased biofouling of microorganisms on plastic surfaces affected by weathering.
The exposure concentration used for the A. marina experiment represents high but still environmentally relevant levels of microplastics in sediments of highly polluted areas (Carson et al., 2011; Haave et al., 2019). Microplastics have previously been shown to affect A. marina by decreases in weight (Besseling et al., 2013), depletion of energy reserves (Wright et al., 2013), increased oxygen consumption (Green et al., 2016) and reduced feeding activity (Besseling et al., 2013; Wright et al., 2013; Green et al., 2016), although at higher concentrations (5%–10%) and longer expsoure time (ca. 4 weeks) compared to the current study. Although no adverse effects of BPE-AMF-PLA on A. marina were detected here, toxic effects may still exist at higher concentrations or after longer exposure times, but this remains to be investigated.
4.4 Development of the cross section and framework representing necessity of this kind of studies
As BPE-AMF-PLA is not yet commercially available, the ecotoxicity tools used in the current study are directly serving to the safe-by-design product development. The key iterative elements of preliminary framework for such product development are 1) design of bio-based plastic, 2) characterisation of the material (biopolymer itself, and the product—bio-based plastic film) and 3) environmental safety evaluation (Figure 11). Beyond this, the present case study of BPE-AMF-PLA can be used as a testing scheme for hazard assessment, or impact of bio-based plastics’ disposal to soil in support of EU regulations and strategies on environmental quality and biodiversity, such as EU Green Deal, EU Action Plan on zero pollution, EU Soil Strategy 2030 (EU Commission, 2019; EU Commission, 2021a; EU Commission, 2021a).
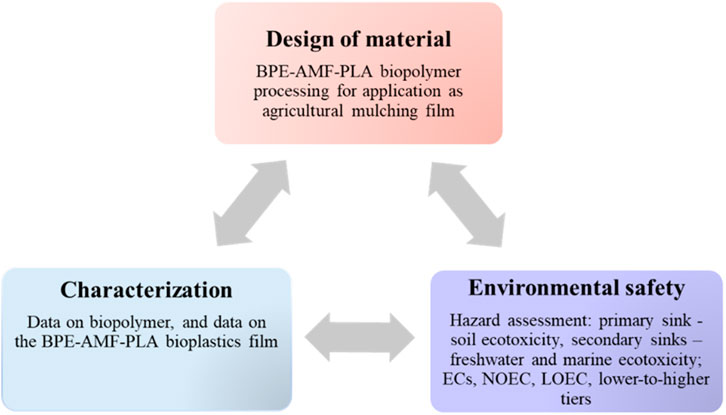
FIGURE 11. The scheme presenting a preliminary framework towards safe-by-design bio-based plastic mulch film development, using the BPE-AMF-PLA case study.
In the current work, the environmental safety aspects of the framework are demonstrated by: 1) conducting integrative ecotoxicity assessment in three environmental compartments while taking into account the leaching potential of bio-based plastics—soil (as primary media, i.e., sink of bio-based plastics when using in agriculture as mulching film), fresh water and marine water (as secondary sinks of bio-based plastics and/or bio-based microplastics); 2) using organisms with different exposure routes to potentially toxic compounds from the bio-based plastics, and/or from different trophic levels within the same compartment; 3) development of different sample preparation procedures and simulation of different exposure scenarios; 4) evaluation of acute and chronic endpoints, and extrapolation of ecotoxicity parameters especially relevant for regulatory risk assessment (NOEC, LOEC); 5) the approach from lower-to higher-tier, where the latter can be applied as an intermediate tool between the laboratory single species standardized bioassays and field studies; 6) evaluation of sublethal endpoints by targeting different levels of biological organisation—from effects on organisms to biochemical-level responses, therefore providing mechanistic understanding and fundamental knowledge on the impact of bio-based plastics/micro-bio-based plastics on non-target organisms. Finally, the results and recommendations of the current study may provide additional support for the certification of the biopolymers and bio-based plastic materials, thus contributing evidence for their safe and sustainable use in line with European strategies and regulations (EU Commission, 2018; EU Commission Eu, 2021c).
5 Conclusion
Overall, organisms’ responses to PLA-based plastics were endpoint- and species-specific. Low-to-no phytotoxicity was observed upon exposure to the soil amended with particles of BPE-AMF-PLA. The exception was the growth reduction effect observed in the roots of dicotyledon plants. This result emphasizes the need to better understand and contextualize the use of bio-based mulching films with different plant/crop species. The earthworms were the most sensitive species tested in the current study, with the reproduction (number of cocoons) as the most sensitive endpoint (LOEC = 0.1% w/w of BPE-AMF-PLA particles), followed by the avoidance of the soil containing BPE-AMF-PLA in the microcosms experiment (2.5% w/w). Toxicity to freshwater crustacean D. magna was possibly linked to the presence of 2-methylnaphthalene, which can be avoided in the material production process. The reproduction response was dependent on the sample preparation method, revealing the reduction in offspring when exposed to the leachate of BPE-AMF-PLA. No adverse effects of BPE-AMF-PLA were observed in the contact assay. Growth of the marine algae was significantly inhibited at the concentration of 25 g BPE-AMF-PLA l−1. Lugworm A. marina ingested both bio-based and conventional, fossil-based microplastics, as shown by the presence of microplastics in the faeces. Despite no adverse effects on the organisms’ biological activity and health were reported in this study, a risk for trophic transfer cannot be excluded. Further contextualization of risks under relevant environmental conditions and range of abiotic and biotic factors remains to be addressed prior to safe use of novel bio-based plastic mulching films. Digestive enzyme activity, namely, lipase, was increased in brine shrimp Artemia nauplii. Although benefits or costs of such response need to be elucidated, the reported results indicate potential degradation of biopolymers within the bio-based plastic film by these organisms. This study represents an early ecotoxicological safety evaluation of the PLA-based plastic film BPE-AMF-PLA, destined to prevent development and production of toxic alternatives. Beyond this, the study approach and results provide a solid platform for the framework development for safe use of novel bio-based materials. A comprehensive risk assessment needs to consider the conditions of product use, the potential release of toxic substances, and their environmental accumulation. Consideration of these factors allows for estimating the Predicted Environmental Concentration (PEC), which can then be tested to target organisms. Additionally, biodegradabilty of biopolymers is a critical property that needs to be contextualized alongside the ecotoxicological approaches and environmentally relevant scenarios. These factors should be considered in follow-up studies from the BIO-PLASTICS EUROPE project, which will be based on a broader knowledge regarding the novel compounds.
Data availability statement
The raw data supporting the conclusion of this article will be made available by the authors, without undue reservation.
Author contributions
JB: Conceptualization; Project administration; Resources; Supervision; Roles/Writing—original draft; Writing—review and editing. EA: Supervision, Roles/Writing—original draft; Writing—review and editing. C-YC: Data curation; Investigation; Methodology. MG: Conceptualization, Data curation; Formal analysis; Investigation; Methodology; Supervision; Validation. LG: Conceptualization, Data curation; Formal analysis; Funding acquisition; Investigation; Methodology; Project administration; Resources; Supervision; Validation; Writing—review and editing. A-SK: Conceptualization, Data curation; Formal analysis; Investigation; Methodology; Supervision; Validation; Writing—original draft. SDK: Conceptualization; Data curation; Formal analysis; Investigation; Methodology; Supervision; Validation; Writing—review and editing. WLF: Supervision; Validation. EL-B: Data curation; Investigation; Methodology; Roles/Writing—original draft. LM: Conceptualization; Data curation; Formal analysis; Investigation; Methodology; Supervision; Validation; Writing—review and editing. KP: Conceptualization; Data curation; Formal analysis; Methodology; Validation; Writing—original draft. MP: Conceptualization; Methodology; Writing—review and editing. RS: Conceptualization; Formal analysis; Funding acquisition; Investigation; Methodology; Supervision; Validation; Visualization; Writing—original draft. RSR: Methodology; Writing—review and editing. GW: Roles/Writing—original draft; Writing—review and editing. All authors contributed to the article and approved the submitted version.
Funding
This study has received funding from the European Union’s Horizon 2020—Research and Innovation Framework Programme through the research project BIO-PLASTICS EUROPE, under grant agreement No.860407. Thanks are due to financial support to CESAM by FCT/MCTES (UIDP/50017/2020 + UIDB/50017/2020 + LA/P/0094/2020), through Portuguese national funds.
Conflict of interest
The authors declare that the research was conducted in the absence of any commercial or financial relationships that could be construed as a potential conflict of interest.
Publisher’s note
All claims expressed in this article are solely those of the authors and do not necessarily represent those of their affiliated organizations, or those of the publisher, the editors and the reviewers. Any product that may be evaluated in this article, or claim that may be made by its manufacturer, is not guaranteed or endorsed by the publisher.
References
Asiandu, A. P., Wahyudi, A., and Sari, S. W. (2021). A review: plastics waste biodegradation using plastics-degrading bacteria. J. Environ. Treat. Tech. 9 (1), 148–157. doi:10.47277/JETT/9(1)157
Bejgarn, S., MacLeod, M., Bogdal, C., and Breitholtz, M. (2015). Toxicity of leachate from weathering plastics: An exploratory screening study with Nitocra spinipes. Chemosphere 132, 114–119. doi:10.1016/j.chemosphere.2015.03.010
Beltrán-Sanahuja, A., Benito-Kaesbach, A., Sánchez-García, N., and Sanz-Lázaro, C. (2021). Degradation of conventional and biobased plastics in soil under contrasting environmental conditions. Sci. Total Environ. 787, 147678. doi:10.1016/j.scitotenv.2021.147678
Besseling, E., Wegner, A., Foekema, E. M., van den Heuvel-Greve, M. J., and Koelmans, A. A. (2013). Effects of microplastic on fitness and PCB bioaccumulation by the lugworm Arenicola marina (L). Environ. Sci. Technol. 47 (1), 593–600. doi:10.1021/es302763x
Bledzki, A. K., and Jaszkiewicz, A. (2010). Mechanical performance of biocomposites based on PLA and PHBV reinforced with natural fibres – a comparative study to PP. Compos. Sci. Technol. 70, 1687–1696. doi:10.1016/j.compscitech.2010.06.005
Bobra, A. M., Shiu, W. Y., and Mackay, D. (1983). A predictive correlation for the acute toxicity of hydrocarbons and chlorinated hydrocarbons to the water flea. Chemosphere 12, 1121–1129. doi:10.1016/0045-6535(83)90118-2
Bour, A., Hossain, S., Taylor, M., Summer, M., and Almroth, B. C. (2020). Synthetic microfiber and microbead exposure and retention time in model aquatic species under different exposure scenarios. Front. Environ. Sci. 8, 83. doi:10.3389/fenvs.2020.00083
Bråte, I. L., Huwer, B., Thomas, K., Eidsvoll, D., Halsband, C., Almroth, B. C., et al. (2017). Micro-and macro-plastics in marine species from Nordic waters. Copenhagen K, Denmark: The Nordic Council of Minister.
Cadée, G. C. (1976). Sediment reworking by Arenicola marina on tidal flats in the Dutch Wadden Sea. Neth. J. Sea Res. 4, 440–460. doi:10.1016/0077-7579(76)90020-x
Carson, H. S., Colbert, S. L., Kaylor, M. J., and McDermid, K. J. (2011). Small plastic debris changes water movement and heat transfer through beach sediments. Mar. Pollut. Bull. 62 (8), 1708–1713. doi:10.1016/j.marpolbul.2011.05.032
Castro-Aguirre, E., Iñiguez-Franco, F., Samsudin, H., Fang, X., and Auras, R. (2016). Poly(lactic acid) - mass production, processing, industrial applications, and end of life. Adv. Drug Deliv. Rev. 107, 333–366. doi:10.1016/j.addr.2016.03.010
Dahl, M., Bergman, S., Björk, M., Diaz-Almela, E., Granberg, M., Gullström, M., et al. (2021). A temporal record of microplastic pollution in Mediterranean seagrass soils. Environ. Pollut. 273, 116451. doi:10.1016/j.envpol.2021.116451
Di Bartolo, A., Infurna, G., and Dintcheva, N. T. (2021). A review of bioplastics and their adoption in the circular economy. Polymers 13 (8), 1229. doi:10.3390/polym13081229
DIN, (2015). DIN EN ISO 10253:1998-04. Berlin, Germany: Deutsches Institut für Normung.Wachstumshemmtest mit marinen Algen Skeletonema costatum und Phaeodactylum tricornutum
Directive, (2019). DIRECTIVE (EU) 2019/904 Of the European Parliament and of the Council of 5 June 2019 on the reduction of the impact of certain plastic products on the environment. https://eur-lex.europa.eu/legal-content/EN/TXT/PDF/?uri=CELEX:32019L0904.
Eom, H-J., Nam, S-E., and Rhee, J-S. (2020). Polystyrene microplastics induce mortality through acute cell stress and inhibition of cholinergic activity in a brine shrimp. Mol. Cell. Toxicol. 16, 233–243. doi:10.1007/s13273-020-00088-4
European Bioplastics, (2020). BIOPLASTICS facts and figures. Available at:https://docs.european-bioplastics.org/publications/EUBP_Facts_and_figures.pdf.
European Commission, (2018). A European strategy for plastics in a circular economy. Available at: https://ec.europa.eu/environment/circular-economy/pdf/plasticsstrategy-brochure.pdf (Accessed February 24, 2021).
European Commission, (2021a). Communication from the commission to the European parliament, the council, the European economic and social committee and the committee of the regions pathway to a healthy planet for all EU action plan: 'Towards zero pollution for air, water and soil' com/2021/400 final. https://eur-lex.europa.eu/legal-content/EN/ALL/?uri=COM%3A2021%3A400%3AFIN.
European Commission EU, (2021c). Soil strategy for 2030 COM. Available at: https://ec.europa.eu/environment/publications/eu-soil-strategy-2030_en (Accessed October 15, 2022).
European Commission, (2019). The European economic and social committee and the committee of the regions. The European Green Deal. https://eur-lex.europa.eu/legal-content/EN/TXT/?uri=COM%3A2019%3A640%3AFIN.
Farah, S., Anderson, D. G., and Langer, R. (2016). Physical and mechanical properties of PLA, and their functions in widespread applications — A comprehensive review. Adv. Drug Deliv. Rev. 107, 367–392. doi:10.1016/j.addr.2016.06.012
Ferreira-Filipe, D. A., Paço, A., Natal-da-Luz, T., SousaSaraiva, J. P. J. A., Duarte, A. C., Rocha-Santos, T., et al. (2022). Are mulch biofilms used in agriculture an environmentally friendly solution? - an insight into their biodegradability and ecotoxicity using key organisms in soil ecosystems. Sci. Total Environ. 828, 154269. doi:10.1016/j.scitotenv.2022.154269
Filiciotto, L., and Rothenberg, G. (2021). Biodegradable plastics: Standards, policies, and impacts. Chem. Sus. Chem. 14 (1), 56–72. doi:10.1002/cssc.202002044
Fuller, S. G., and Gautam, A. (2016). A procedure for measuring microplastics using pressurized fluid extraction. Environ. Sci. Technol. 50, 5774–5780. doi:10.1021/acs.est.6b00816
Gall, S. C., and Thompson, R. C. (2015). The impact of debris on marine life. Mar. Pollut. Bull. 92, 170–179. doi:10.1016/j.marpolbul.2014.12.041
Gambardella, C., Morgana, S., Ferrando, S., Bramini, M., Piazza, V., Costa, E., et al. (2017). Effects of polystyrene microbeads in marine planktonic crustaceans. Ecotoxicol. Environ. Saf. 145, 250–257. doi:10.1016/j.ecoenv.2017.07.036
Green, D. S., Boots, B., Sigwart, J., Jiang, S., and Rocha, C. (2016). Effects of conventional and biodegradable microplastics on a marine ecosystem engineer (Arenicola marina) and sediment nutrient cycling. Environ. Pollut. 208, 426–434. doi:10.1016/j.envpol.2015.10.010
Haave, M., Lorenz, C., Primpke, S., and Gerdts, G. (2019). Different stories told by small and large microplastics in sediment—first report of microplastic concentrations in an urban recipient in Norway. Mar. Pollut. Bull. 141, 501–513. doi:10.1016/j.marpolbul.2019.02.015
Han, X., Zheng, Y., Dai, C., Duan, H., Gao, M., Ali, M. R., et al. (2021). Effect of polystyrene microplastics and temperature on growth, intestinal histology and immune responses of brine shrimp Artemia franciscana. J. Oceanogr. Limnol. 39, 979–988. doi:10.1007/s00343-020-0118-2
Hannam, M. L., Barber, S. D., Galloway, T. S., Moody, A. J., and Jones, M. B. (2010). Immunotoxicity and oxidative stress in the arctic scallop Chlamys islandica: Effects of acute oil exposure. Ecotox Environ Saf 73, 33–36. doi:10.1016/j.molliq.2018.09.109
Henton, D. E., Gruber, P., Lunt, J., Randall, J., and Mohan, A. K. (2005). Natural fibers, biopolymers and biocomposites. Oxfordshire United Kingdom: Taylor & Francis.
Hübner, L., Pennings, S. C., and Zimmer, M. (2015). Sex- and habitat-specific movement of an omnivorous semi-terrestrial crab controls habitat connectivity and subsidies: A multi-parameter approach. Oecologia 178, 999–1015. doi:10.1007/s00442-015-3271-0
Johnston, D., and Freeman, J. (2005). Dietary preference and digestive enzyme activities as indicators of trophic resource utilization by six species of crab. Biol. Bull. 208, 36–46. doi:10.2307/3593099
Jones, D. A., Kumlu, M., Le Vay, L., and Fletcher, D. J. (1997). The digestive physiology of herbivorous, omnivorous and carnivorous crustacean larvae: A review. Aquaculture 155, 285–295. doi:10.1016/S0044-8486(97)00129-4
Kokalj, A. J., Kunej, U., and Skalar, T. (2018). Screening study of four environmentally relevant microplastic pollutants: Uptake and effects on Daphnia magna and Artemia franciscana. Chemosphere 208, 522–529. doi:10.1016/j.chemosphere.2018.05.172
Korez, Š., Gutow, L., and Saborowski, R. (2019). Feeding and digestion of the marine isopod Idotea emarginata challenged by poor food quality and microplastics. Comparative Biochemistry and Physiology. Toxicol. Pharmacol. CBP 226, 108586. doi:10.1016/j.cbpc.2019.108586
Koul, B., Yakoob, M., and Shah, M. P. (2022). Agricultural waste management strategies for environmental sustainability. Environ. Res. 206, 112285. doi:10.1016/j.envres.2021.112285
Koussoroplis, A-M., Schwarzenberger, A., and Wacker, A. (2017). Diet quality determines lipase gene expression and lipase/esterase activity in Daphnia pulex. Biol. Open 6, 210–216. doi:10.1242/bio.022046
Kreibich, T., Saborowski, R., Hagen, W., and Niehoff, B. (2008). Short-term variation of nutritive and metabolic parameters in Temora longicornis females (Crustacea, Copepoda) as a response to diet shift and starvation. Helgol. Mar. Res. 62, 241–249. doi:10.1007/s10152-008-0112-0
Lesser, M. P. (2006). Oxidative stress in marine environments: Biochemistry and physiological ecology. Ann. Rev. Physiol. 68, 253–278. doi:10.1146/annurev.physiol.68.040104.110001
Lithner, D., Damberg, J., Dave, G., and Larsson, K. (2009). Leachates from plastic consumer products-screening for toxicity with Daphnia magna. Chemosphere 74, 1195–1200. doi:10.1016/j.chemosphere.2008.11.022
Lithner, D., Nordensvan, I., and Dave, G. (2011). Comparative acute toxicity of leachates from plastic products made of polypropylene, polyethylene, PVC, acrylonitrile-butadiene-styrene, and epoxy to Daphnia magna. Environ. Sci. Pollut. Res. Int. 19, 1763–1772. doi:10.1007/s11356-011-0663-5
Liwarska-Bizukojć, E. (2022b). Application of a small scale-terrestrial model ecosystem (STME) for assessment of ecotoxicity of bio-based plastics. Sci. Total Environ. 828, 154353. doi:10.1016/j.scitotenv.2022.154353
Liwarska-Bizukojc, E. (2022a). Phytotoxicity assessment of biodegradable and non-biodegradable plastics using seed germination and early growth tests. Chemosphere 289, 133132. doi:10.1016/j.chemosphere.2021.133132
Luo, H., Xiang, Y., He, D., Li, Y., Zhao, Y., Wang, S., et al. (2019). Leaching behavior of fluorescent additives from microplastics and the toxicity of leachate to Chlorella vulgaris. Sci. Total Environ. 678, 1–9. doi:10.1016/j.scitotenv.2019.04.401
Luyt, A. S., and Malik, S. S. (2019). “Can biodegradable plastics solve plastic solid waste accumulation?,” in Plastics to energy (New York, NY, USA: Elsevier), 403–423.
Madadi, R., Maljaee, H., Serafim, L. S., and Ventura, S. P. M. (2021). Microalgae as contributors to produce biopolymers. Mar. Drugs 19 (8), 466. doi:10.3390/md19080466
Miksch, L., Köck, M., Gutow, L., and Saborowski, R. (2022). Bioplastics in the sea: Rapid in-vitro evaluation of degradability and persistence at natural temperatures. Front. Mar. Sci. 9, 920293. doi:10.3389/fmars.2022.920293
Mohee, R., and Unmar, G. (2007). Determining biodegradability of plastic materials under controlled and natural composting environments. Waste Manag. 27 (11), 1486–1493. doi:10.1016/j.wasman.2006.07.023
Moliner, C., Finocchio, E., Arato, E., Ramis, G., and Lagazzo, A. (2020). Influence of the degradation medium on water uptake, morphology, and chemical structure of Poly(Lactic Acid)-Sisal bio-composites. Materials 13 (18), 3974. doi:10.3390/ma13183974
Narancic, T., and O’Connor, K. E. (2019). Plastic waste as a global challenge: Are biodegradable plastics the answer to the plastic waste problem?. Microbiology 165 (2), 129–137. doi:10.1099/mic.0.000749
Nunes, B. S., Carvalho, F. D., Guilhermino, L. M., and Van Stappen, G. (2006). Use of the genus Artemia in ecotoxicity testing. Environ. Pollut. 144 (2), 453–462. doi:10.1016/j.envpol.2005.12.037
OECD, (2004). 202Daphnia sp., Acute Immobilisation Test. OECD guideline for testing of chemicals. Paris, France: OECD.
OECD, (1984). 207 Earthworm, acute toxicity tests. OECD guideline for testing of chemicals. Paris, France: OECD.
OECD, (2012). 211 Daphnia magna Reproduction Test. OECD guideline for testing of chemicals. Paris, France: OECD.
Piehl, S., Leibner, A., Löder, M. G. J., Dris, R., Bogner, C., and Laforsch, C. (2018). Identification and quantification of macro- and microplastics on an agricultural farmland. Sci. Rep. Dec. 18 8 (1), 17950. doi:10.1038/s41598-018-36172-y
Ratte, M., Heseding, J., and Floeter, C. (2016). Round robin test of the use of microwell plates and Erlenmeyer flasks in the marine algae test with Phaeodactylum tricornutum according to DIN EN ISO 10253. Alsdorf, Germany: ToxRat Solutions GmbH & Co.
Reddy, M. M., Vivekanandhan, S., Misra, M., Bhatia, S. K., and Mohanty, A. K. (2013). Biobased plastics and bionanocomposites: Current status and future opportunities. Prog. Polym. Sci. 38 (10–11), 1653–1689. doi:10.1016/j.progpolymsci.2013.05.006
Rezvani Ghomi, E. R., Khosravi, F., Saedi Ardahaei, A. S., Dai, Y., Neisiany, R. E., Foroughi, F., et al. (2021). The life cycle assessment for polylactic acid (PLA) to make it a low-carbon material. Polymers 13 (11), 1854. doi:10.3390/polym13111854
Rivera-Pérez, C., García-Carreño, F. L., and Saborowski, R. (2011). Purification and biochemical characterization of digestive lipase in Whiteleg shrimp. Mar. Biotechnol. 13, 284–295. doi:10.1007/s10126-010-9298-7
Romano, N., Ashikin, M., Teh, J. C., Syukri, F., and Karami, A. (2018). Effects of pristine polyvinyl chloride fragments on whole body histology and protease activity in silver barb Barbodes gonionotus fry. Environ. Pollut. (Barking, Essex 1987) 237, 1106–1111. doi:10.1016/j.envpol.2017.11.040
Scheurer, M., and Bigalke, M. (2018). Microplastics in Swiss floodplain soils. Environ. Sci. Technol. 52, 3591–3598. doi:10.1021/acs.est.7b06003
Schrank, I., Trotter, B., Dummert, J., Scholz-Böttcher, B. M., Löder, M. G., and Laforsch, C. (2019). Effects of microplastic particles and leaching additive on the life history and morphology of Daphnia magna. Environ. Pollut. 255, 113233. doi:10.1016/j.envpol.2019.113233
Serrano-Ruiz, H., Martin-Closas, L., and Pelacho, A. M. (2021). Biodegradable plastic mulches: Impact on the agricultural biotic environment. Sci. Total Environ. 750, 141228. doi:10.1016/j.scitotenv.2020.141228
Simon, M., Hartmann, N. B., and Vollertsen, J. (2021). Accelerated weathering increases the release of toxic leachates from microplastic particles as demonstrated through altered toxicity to the green algae Raphidocelis subcapitata. Toxics 9, 185. doi:10.3390/toxics9080185
Spierling, S., Knüpffer, E., Behnsen, H., Mudersbach, M., Krieg, H., Springer, S., et al. (2018). Bio-based plastics - a review of environmental, social and economic impact assessments. J. Clean. Prod. 185, 476–491. doi:10.1016/j.jclepro.2018.03.014
Stibany, F., Schmidt, S. N., Schäffer, A., and Mayer, P. (2017). Aquatic toxicity testing of liquid hydrophobic chemicals - Passive dosing exactly at the saturation limit. Chemosphere 167, 551–558. doi:10.1016/j.chemosphere.2016.10.014
Tan, Y., Henehan, G. T., Kinsella, G. K., and Ryan, B. J. (2021). An extracellular lipase from Amycolatopsis mediterannei is a cutinase with plastic degrading activity. Comput. Struct. Biotechnol. J. 19, 869–879. doi:10.1016/j.csbj.2021.01.019
Trestrail, C., Walpitagama, M., Miranda, A., Nugegoda, D., and Shimeta, J. (2021). Microplastics alter digestive enzyme activities in the marine bivalve, Mytilus galloprovincialis. Sci. Total Environ. 779, 146418. doi:10.1016/j.scitotenv.2021.146418
Vasile, C., Pamfil, D., Râpă, M., Darie-Niţă, R. N., Mitelut, A. C., Popa, E. E., et al. (2018). Study of the soil burial degradation of some PLA/CS biocomposites. Compos. Part B Eng. 142, 251–262. doi:10.1016/j.compositesb.2018.01.026
Wright, S. L., Rowe, D., Thompson, R. C., and Galloway, T. S. (2013). Microplastic ingestion decreases energy reserves in marine worms. Curr. Biol. 23, 1031–1033. doi:10.1016/j.cub.2013.10.068
Yu, M., Zheng, Y., and Tian, J. (2020). Study on the biodegradability of modified starch/polylactic acid (PLA) composite materials. RSC Adv. 10 (44), 26298–26307. doi:10.1039/D0RA00274G
Keywords: toxicity, bio-based plastics, mulch films, bioassays, PLA, environmental toxicity
Citation: Barbir J, Arato E, Chen C-Y, Granberg M, Gutow L, Krång A-S, Kröger SD, Leal Filho W, Liwarska-Bizukojc E, Miksch L, Paetz K, Prodana M, Saborowski R, Silva Rojas R and Witt G (2023) Assessing ecotoxicity of an innovative bio-based mulch film: a multi-environmental and multi-bioassay approach. Front. Environ. Sci. 11:1171261. doi: 10.3389/fenvs.2023.1171261
Received: 21 February 2023; Accepted: 27 June 2023;
Published: 07 July 2023.
Edited by:
Zhenming Zhang, Guizhou University, ChinaCopyright © 2023 Barbir, Arato, Chen, Granberg, Gutow, Krång, Kröger, Leal Filho, Liwarska-Bizukojc, Miksch, Paetz, Prodana, Saborowski, Silva Rojas and Witt. This is an open-access article distributed under the terms of the Creative Commons Attribution License (CC BY). The use, distribution or reproduction in other forums is permitted, provided the original author(s) and the copyright owner(s) are credited and that the original publication in this journal is cited, in accordance with accepted academic practice. No use, distribution or reproduction is permitted which does not comply with these terms.
*Correspondence: J. Barbir, amVsZW5hLmJhcmJpckBoYXctaGFtYnVyZy5kZQ==