River mouth morphodynamics and deflection over the short term: effects on spit growth and mangrove dynamics
- Department of Geography, Faculty of Earth and Environmental Sciences, University of Guyana, Georgetown, Guyana
The river mouth shows the influence of the dynamics of sediment deposition and the processes organising the deposits. The river mouths of the Guyana coast, as with other coastal systems in the Guianas, are influenced by the deposition of mud banks from the Amazon River and other rivers. This study analysed and probed the influence of the riverine and marine influences on small river mouth morphological developments. In this study, the morphological dynamics of the system were carried out from satellite images through the comparison of the inter-annual morphodynamics of the river mouths, complemented by data from hydrodynamic processes among others. This study demonstrates that the recent advancement and availability of Synthetic Aperture Radar (SAR), remotely sensed data, allow for the classification of migrating river mouth action and processes; effective monitoring of mudflats development and spit formation; and, the exhibition of river mouth transitioning and infilling. Sentinel-1 images of the Mahaica-Mahaicony river mouth in Guyana were processed and analysed using Google Earth Engine (GEE) and ArcGIS to observe the mud dynamics and its effects on deflecting the river mouth, influencing the development of spit and mangrove dynamics. The results of the analyses show that the dynamics of the mud shoal and river mouth are governed by feedback from various estuarine and hydrodynamic processes resulting from the interactions between the river and ocean. The results have not only highlighted the importance of mud infilling and sediment build-up for spit development and river-mouth deflection but the impact of the sediment morphological dynamics on the ecosystem (mangrove) associated with the river mouth.
1 Introduction
River mouths and estuaries are both bodies of water where freshwater and saltwater mix (Perillo, 1995). However, as river mouths are formed by the flow of freshwater into the ocean, the estuaries are formed by the tidal movement of seawater into a river valley (McLusky and Elliott, 2007; Tagliapietra et al., 2009; Mikhailov annnd Gorin, 2012). River mouths play a great role in coastal water dynamics (Fagherazzi et al., 2015; Sreenivasulu et al., 2016; Taft and Evers, 2016; Li et al., 2022; Ngobeni and Knight, 2023). They are the places where freshwater from rivers mixes with saltwater from the ocean, creating unique ecosystems that support a variety of marine life (McLusky and Elliott, 2004; McLusky and Elliott, 2007; Van Niekerk et al., 2020; Broadley et al., 2022; Cañedo-Argüelles et al., 2023). Additionally, river mouths can also act as sediment traps, affecting coastal erosion and shaping the coastline over time (Dominguez et al., 1987; Anthony, 2015; Özpolat and Demir, 2019; Zhong et al., 2020; Knight, 2023; Vundavilli, 2023).
Mudbanks are one of the major coastal morphological features that have a direct influence and close impact on dynamics in the coastal area (Lefebvre et al., 2004; Parvathy et al., 2015; de Vries et al., 2022; van Bijsterveldt et al., 2023). The river mouth shows the influence of the dynamics of sediments deposition and the processes organising the deposits (Cai et al., 2022; Gardel et al., 2022; Moyano-Paz et al., 2022; Estournel et al., 2023). The morphology of these environments is dynamic and responds to variations in transportation, erosional and depositional of sediments both in space and time (Oyedotun and Burningham, 2019; Turki et al., 2021; Afentoulis et al., 2022). The river mouth environment also responds to a series of dynamics of physical factors and natural processes like changes in river process, tidal and wave energy (Wong et al., 2014; Ge et al., 2021; Ricci et al., 2022), sea level changes (Padmalal et al., 2014; Xia et al., 2020; Haque et al., 2022; Paul and Paul, 2022) or influence of anthropogenic activities (Wang et al., 2015; Manzolli et al., 2022; Syvitski et al., 2022). The morphodynamic response progresses through the significant phases of deposition and/or erosion over short, medium and longer timescales (Luijendijk et al., 2019; Roelvink et al., 2020; Elias et al., 2022).
The river mouths of the Guyana coast, as with other coastal systems in the Guianas, are influenced by the deposition of mud banks from the large and small rivers in the area, including the Amazon River and other rivers (Gardel et al., 2022). The areas in front of the mouth of large river systems have been well and extensively studied and reported in the literature (e.g., Wright and Coleman, 1974; Wang and Liang, 2000; Van Maren, 2007; Zhen et al., 2008; Fagherazzi et al., 2015; Nienhuis et al., 2016; Hashimoto et al., 2021; Polizel and Burningham, 2022; Lakshmanna et al., 2023; Li et al., 2023, etc.). However, there is limited or no study of the dynamics at the mouth of small river systems. This study is there not only aimed at highlighting the importance of mud infilling and sediment build-up at the mouth of large river systems as reported in other studies but to emphasise the dynamics of areas in front of small river systems for sediment accumulation and new landform development, e.g., spit development. This study analysed and probed the influence of the riverine and marine influences on river mouth morphological developments. This study documents the short-term morphological changes at the mouth of the Mahaica River system, Guyana, in response to smaller-scale (local) mud-bank dynamics, as a significant contribution to the understanding of morphodynamics at the mouth of a small river system. Understanding the short-term timescales of muddy movement (for accretion or erosion) and evaluating the pattern of sediment concentration and segregation in this muddy setting as it affects spit development and mangrove dynamics are the two primary objectives of this investigation.
2 Material and methods
2.1 Study area: Guyana coast and Mahaica River
Guyana, a tropical Atlantic country with a geographic area of 214,970 km2, a 430 km coastline and a land mass extent of approximately 724 km (Figure 1) is located in the northeast of the South American continent. About 2% of the country’s coastal land is below 5 m with much of which is 0.5–1 m below mean high tide (Government of Guyana, 2012; Oyedotun and Burningham, 2021). This semi-diurnal coastal system is characterised by a 1–3 m at neap and spring tide respectively (Winterwerp et al., 2020). The coastal plain of Guyana is part of the mud-dominated South America’s Guianas coast (Anthony et al., 2010; 2014; Jolivet et al., 2019; Gardel et al., 2021). Although the two rivers are the largest river system in the region, there are also several small river systems (Anthony et al., 2010). However, the mud supplied by Amazon has dominated the geomorphological and geological development of the coastal terrain of this region (Dominguez, 2009; Anthony et al., 2010; Anthony et al., 2013). The Mahaica River (Figure 1), one of the small river systems in Guyana, is in northern Guyana and one of the principal coastal streams that drain into the Atlantic Ocean, and also serves as a border between Region 4 (Demerara-Mahaica) and Region 5 (Mahaica-Berbice) of Guyana. The Mahaica River’s estimated flow is 1,700 cubic feet (48,138.64 L) per second during the wet season and 700 cubic feet (19,821.79) per second during the dry season (Worts, 1963). The river, whose origin is difficult to determine as it overlaps with lower watersheds of both Demerara and Berbice rivers, has smaller tributaries along its path (Vaughn, 2013) before draining into the Atlantic Ocean at latitude 6°42′26″N and longitude 57°55′14″W. This river is very important for many reasons. It is known for bird-watching activities in addition to serving as a home to many other wildlife animals like river otters, Canje Pheasant (Guyana’s National Bird), and howler monkeys, among others, and used for cultivation of rice farming (Johnson, 2016).
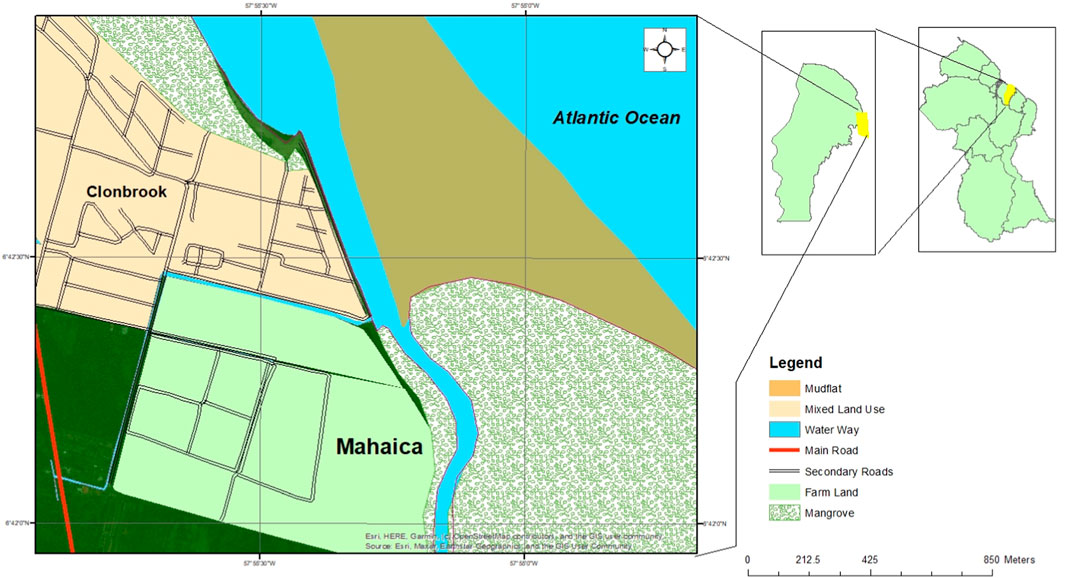
FIGURE 1. Location of the study area (Inset: Map of Guyana showing the region and the map of the region showing the study site).
2.2 Data used
In this study, the morphological dynamics of the system were carried out from satellite images through the comparison of the inter-annual morphodynamics of the river mouths and inlets, complemented by data from hydrodynamic processes among others. Here, this study demonstrates that the recent advancement and availability of Synthetic Aperture Radar (SAR) remotely sensed data (Marghany, 2013) allow for i) classification of migrating river mouth action and processes; ii) effective monitoring of mudflats development and spit formation; and, iii) the exhibition of river mouth transitioning and infilling (after Marghany, 2012; Marghany, 2013; Marghany, 2014; Ouchi, 2013; Matano, 2019; Devrani et al., 2022; Ghanbari et al., 2023). Sentinel-1 images of the Mahaica-Mahaicony river mouth in Guyana were acquired in 2015, 2016, 2017, 2018, 2019, 2020, and 2021 respectively as detailed in Table 1. Sentinels Application Platform (SNAP) was used to pre-process the images through the application of the orbit file to maximise the geolocation quality of the acquired dataset of geocoding. Then the radiometric calibration of the images was done in SNAP through the application of Radiometric Terrian Correction before the radiometric flattening to remove the surface-induced radiometric distortions, after which the geometric terrain correction was applied to compensate for the distortions sensors’ positions’ variations. Speckle filtering was also applied in SNAP to remove the wave interference and also to enable the pre-processing of the images for detection and classification. The pre-processing steps considered in this study are after Lee et al. (1994); Filipponi. (2019); Meyer. (2019); Nedd et al. (2021). The preprocessed images were exported as a geo tiff for further processing and analyses in Google Earth Engine (GEE) and ArcGIS in observing the mud-shoal migration and its effects on deflecting the river mouth, spit evolution/development and mangrove dynamics. The datasets were acquired from the Alaska Satellite Facility (ASF) web platform (www.asf.alaska.edu). Tidal information, especially the tide height data for the study sites was retrieved from the “Tides 4 Fishing” database (https://tides4fishing.com/gy/demerara-mahaica/georgetown) and these covered the study period. The information for the wave height and wind direction was sourced from ABP Marine Environmental Research (MER) Ltd., from its openly available SEASTATES hindcast service (www.seastates.net).
2.3 Classification of migrating river mouth action and processes
The spatial-temporal dynamics of the mudbank at the Mahaica River Mouth were achieved by utilising the Jupyter Notebook and the Google Earth Engine (GEE) platform. This analysis covered the period from 2015 to 2021 focusing on the estuary of the Mahaica River. The Jupyter Notebook was used to plot the tide data to visually interpret the lowest tide for each year which coincide with the available Sentinel-1 dataset. First, the tide data for each month of the year was downloaded and displayed on an Excel spreadsheet before being saved as a CSV file. In Jupyter Notebook, the CSV files were imported, then the date and time were combined, resampled every 15 min, and then the mean was interpolated. The results were then plotted on a scattered chart displaying the available Sentinel-1 dataset for each month. The available datasets for each month were then compared against each other to determine which datasets were captured at the lowest tide and were then chosen for further analyses in GEE.
2.3.1 Processes in Google Earth Engine (GEE)
The ee.ImageCollection function was used to import the images for the years being studied. These images were then filtered by area of interest and by the date that recorded the lowest tide for every year. They were further filtered to get the vertical transmit vertical receive (VV) and horizontal transmit vertical receive (VH) dual polarization and look angle (ascending or descending), which is the orbit flight path of the satellite.
2.3.2 Constructing a random forest model
Two classes were defined and merged to run the model; those classes are non-mudflat and mudflat. The Sentinel bands were defined, then included in the model and applied to the image. Next, the samples were assembled for the model and trained using the ee.Classifier.smileCart function. The classification was then applied to the Sentinel 1 composite and clipped to the area of interest. During this stage, the results may be noisy, so a mask was created to mask out unconnected pixels. This created an image that shows the number of pixels each pixel is connected to, then filter out all pixels connected to 4 or fewer. The results were then used to update the classification and add them to the map for visual interpretation. The mudflats that were displayed as a result of the classification for the years were exported for further analysis.
2.3.3 Processes in ArcMap
The exported mudflats for each year were uploaded into ArcMap 10.5 and these were analysed to show how the mudflat eroded and accreted over time.
2.4 Land cover change
To investigate the land cover changes in the study area from 2015 to 2021, a variable dataset was created. This dataset contained all the available Sentinel-1 images for the area of interest within the study period. The study period was further broken down into minor periods which begin from January 1st to December 31st of each year. Another variable was made, and the function (.filterMetadata) was used to give each year’s dataset a cloud coverage assessment of less than 25%. Bands blue (B2), green (B3), red (B4), and near-infrared (B8) were selected. The combination of the colour infrared bands is intended to highlight both healthy and sick vegetation. It is particularly effective at reflecting chlorophyll because it uses the near-infrared (B8) band. The composite image was then further filtered using the function (.filterMetadata) via the Military Grid Reference System tile (MGRS_tile). Then the function (.limit) was used to return the limited collection, which is sorted by “system:time_start” and set to false (descending order). Visual parameters were then applied to the filtered image, clipped to the area of interest, and mapped for visual interpolation. This process was carried out in Google Earth Engine (GEE).
3 Results and discussion
3.1 Short-term morphological changes at the river mouth
Figure 2 depicts the selection of the infra-red output of the Sentinel-1 satellite images of the Mahaica river mouth. The figure illustrates the success of the spatiotemporal contribution of sediment (mostly mud) from the Mahaica River system to the mouth of the system which is contributing to the morphological dynamics of the river mouth, especially in the stability of the spit at the site. One of the most dynamic coastal features is spits and their stability or otherwise are dependent on the delicate balance between the availability of sediments and/or the metocean forcings, especially the hydrodynamic forces (Dan et al., 2011). The progression of the supply of sediments at the mouth of the Mahaica river system (from 2016, Figure 2) has shown that input of sediments from onshore in a zero gradient like this system is an indication that a small river system can be an “equilibrium coastline” that promotes the development of spits (as stated by Delgaard and Fredsøe, 2005). The concept of “equilibrium coastline,” which is hinged on the principles of uniform wave climate condition, the input of sediments from onshore and/or offshore sources, limited or no loss of sediments, zero gradients for the longshore and alongshore movements, etc., is one of the most important concepts that are used in understanding the stability of the dynamic depositional coastal landforms like spits (e.g., Silvester, 1960; Kolb and Schmidt, 1991; Delgaard and Fredsøe, 2005; Dan et al., 2011; Splinter et al., 2014; Deng et al., 2017). In each of the annual images (Figure 2), the continuous effect of northwestward movement of the sediment is noticed (see the river mouth from 2016 to 2021 of the output, Figures 1, 2). This observation corroborates that this system, although a small river system, responds to the regional alongshore transportation or movement of sediments pattern observed for this region (See Gardel et al., 2022).
Figure 3 depicts a spatiotemporal view of the normalized difference vegetation index (NDVI) of the study area. Here the NDVI is used to support and evaluate the coverage, short-time series and medium spatial resolution of the short-term morphodynamics of sediments and mud-bank movements at the river mouth of a small river system. The findings, here, show that on a contemporary timescale, a small river system like the Mahaica River system can divert, to varying extents, significant mud capes, especially in a system with no influence of the bedrock (Gardel et al., 2022). The time series of the dynamics presented in Figure 3 has also shown that the river mouths emerging from a confined onshore environment into an open coastal system can also contribute to the formation of coastal geomorphological dynamics like the displacement of shoreline seaward or alongshore current as variously depicted by the dynamics captured in the images.
River mouths and the coastal systems they produce (e.g., deltas, estuaries, etc.) are very important in many facets as they are mostly known to be fertile, rich in biodiversity and bio-diversified ecosystems, protect against storms, floods and submersion (Hagenlocher et al., 2018; Besset et al., 2019). However, their stability and sustainability depend on the ability of such systems to be sustained with the provision of sediments continuously from their river basins to maintain balance with the metocean forcing working on the system, maintain possible vertical sediment aggradation and promote the seaward advancement of shoreline movement (Besset et al., 2019). These are only possible under the conditions of satisfactory sediment supply in quantity and quality (Anthony, 2016). Whereas the effects of large river basins on sediments supply have received attention in recent years (e.g., Phillips, 1995; Collins et al., 1997; Lu et al., 2003; Ran et al., 2013; Wilkinson et al., 2014; Yang and Lu, 2014; Kondolf et al., 2018; Liu et al., 2018; Mushi et al., 2019; Nilawar and Waikar, 2019; Ranasinghe et al., 2019; Sun et al., 2020; Tian et al., 2020; Föeger et al., 2022; Shrestha et al., 2022; Wang et al., 2022; Kar and Sarkar, 2023; Tangi, 2023). What the findings here have shown is that small rivers in the region contribute sediment on an annual basis to the regional coastal system. However, the alongshore supply and concentrations of mud from the Amazon and the Orinoco exceeding thousands of g/L are so massive as the major source of geological and contemporary mud supply, thereby probably affecting and contributing to the sediment morphodynamics of the smaller river systems of the Guiana (Gratiot et al., 2007; Gardel et al., 2022).
Figure 4 presents the qualitative comparison of recent geomorphological changes at the Mahaica river mouth through the time series display of relatively high-resolution Google Earth Pro satellite imageries showing the dynamics at the river outlets with the observable development and destruction of sand spit at the river mouth from 2014 to 2022. Red arrows (Figures 4A–F) were used to highlight and pinpoint the contemporary and short evolution of the spit barrier at the mouth of this small river system. It is clear that the gradients in the alongshore transport of sediments deposited at the river mouth tend to align the spits evolution and development to a northwestward balance orientation, depending on the Southeast–Northwest (SE-NW) longshore current orientation of the country (Gratiot et al., 2007; Gardel et al., 2022) and not so much of the wind direction nor dominant wave direction (Figure 8). This has, possibly, led to the varied accumulation and erosion of the sand pits at this system. Other possibilities for the short-term dynamics at this site are a result of either the instability of sediment drift (after Ashton et al., 2001; Dan et al., 2011), quasi-parallel waves approaching at incidence angles (Bhattacharya and Giosan, 2003; Sundberg et al., 2013), or alongshore sediment transportation with rapid advancing/migration rates that are induced by alongshore current velocities f 0.2–0.5 m/s northwestward (Winterwerp et al., 2020).
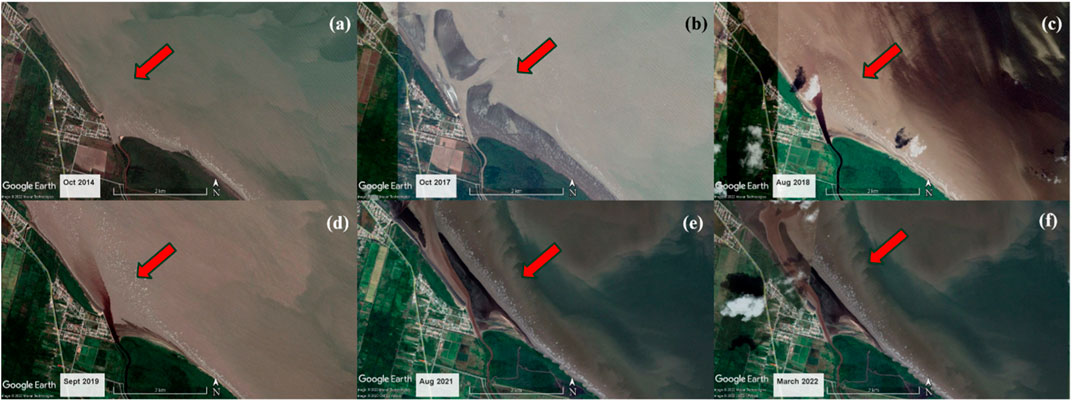
FIGURE 4. Google Earth Pro image and depiction of the idealized spit development and destruction at Mahaica river mouth on a contemporary time scale. Starting from (A) October 2014 when there was no indication of the development, (B) October 2016, (C) August 2018, (D) September 2019, (E) August 2021, and (F) March 2022.
3.2 Mudbank dynamics at the river mouth
The stability of Guyana’s coastal zone is attributed to the 30 km (approximately) of long mud banks that are migrating alongshore but variously colonised at the landward side by mangroves (Winterwerp et al., 2020). Although the mangrove-mud coast of this section is noted to be generally in good condition (Anthony and Gratiot, 2012; Winterwerp et al., 2020; Gardel et al., 2022), the findings of mudbank dynamics and movements at the mouth of this river system in Figures 5–7 have shown that the mud deposits here experience localised accretion and erosion through the period under consideration (2015–2021). The annual rates of the movement of the mud as either erosion or accretion varies as estimated from the analysis of the Sentinel images. Table 2 presents the yearly estimate of the deposits of the mudbank extent at the mouth of the study area while Table presents the quantification of the yearly movements of these deposits in accretion and deposition.
The change analysis of the repeat remotely sensed surveys at the site (Figures 5–7) show lateral shifts in mud deposit position and the movement of the low amplitude of sand bars dominate the short-term river mouth dynamics. Most of the changes reported here are balanced across this river mouth system with the evidence of erosion at one location in an annual change being balanced with accretion at another year of change evaluation. This pattern of accretion and erosion could be well explained by the migration of sediment across the intertidal channel and alongshore migration. Table 3 presents the estimated volume of the magnitude of the yearly migration of sediments spatially presented in Figures 5–7. What is the sequence of events on the series of change show that the sediments are delivered seaward and these are redistributed northwestward over the 6 years (2015–2021) across the rest of the nearshore. The variable change over the 6 years illustrates the role of migrating and mobile sediments in the organisation and re-organisation of the sediment bars on the flood-delta platform. The happenings at this platform could be forced by diurnal tidal currents (Figure 9) but the supply of sediments into this river mouth drives the shifts in mudbank movements as similar to other environments (Oyedotun and Burningham, 2019).
The contemporary scale of mudbank sedimentary behaviour observed here supports the concept that a coastal-estuarine system tends towards flood dominance until when the process of sediment infilling is completed thereby forcing a switch to ebb dominance through the changes in tidal hydrodynamics (Lincoln and Fitzgerald, 1988; Kang and Jun, 2003; Oyedotun and Burningham, 2019; Eilander et al., 2020; Daramola et al., 2022; Weisscher et al., 2022; Harvey et al., 2023). What the mudbank dynamics suggest here is that shifts in mud deposits are occurring on an annual scale, particularly where areas of accretion are followed by areas of erosion within a year or two later. Sediment migration is viewed as a significant driver of erosion and accretion experienced annually in this study site. What was observed in this study is common to what has been observed in other beach and coastal system dynamics, that are at the mouth of a river system or with proximity to an inlet, with increased yearly migration, sediments movements and morphodynamics (Hicks and Hume, 1996; Van Maren, 2007; Short and Jackson, 2013; Oyedotun and Burningham, 2019; Pan et al., 2020; Glover et al., 2021; Glover et al., 2023; Yunus et al., 2022; Zhang et al., 2022; Romdani et al., 2023). Over the short-term period covered by the Sentinel—1 data considered here, no significant storm surges were recorded which could have been linked as the driver of the sudden changes. The spatiotemporal shifts in mud bank morphological systems reflect that processes operating in the river mouth of a small riverine-estuarine environment could drive a differential range of changes both in frequencies and magnitudes like other large systems already documented in the literature. The findings presented in this section have also shown that river mouth platform could recover rapidly from the either accretional or erosional process, especially if there are no major or continuous storm events that drive permanent change or that are sustained over a long period without allowing sufficient recovery time (Montreuil and Bullard, 2012).
3.3 Forcing factors of short-term morphodynamics
The recent Sentinel-1 data (2015–2021) examined for this study enabled the exploration of the short-term temporal variability of the sediment deposits at the mouth of the smaller river system. The regional climate of Guyana is principally tropical and this is dominated by the northeasterly Trade Winds with the wave direction (Figure 8A) following the same pattern at periods of between 8 and 10 s at the wave height which range from 1 to 1.7 m with the higher wave values noted between November and March (Figure 8B) (Gratiot et al., 2007; Winterwerp et al., 2020). The long mud banks of Guyana’s coast have however made the system to be relatively stable (Winterwerp et al., 2020). The proportion of waves at this study site occurs from the Northeasterly/Easterly (NE/E) position with up to approximately 35% at NE, and 52% from the Easterly direction respectively (Figure 8A). Waves from South-East (SE) are infrequent, with ∼05% and a given reduced fetch. Waves from all other directions (e.g., North, North-West, West, South-West, and South) are very rare (Figure 8A). The significant wave height at the mouth of Mahaica River is ∼0.25 m with mean wave height at 0.4 m, and all are from the Northeasterly at ∼80%.
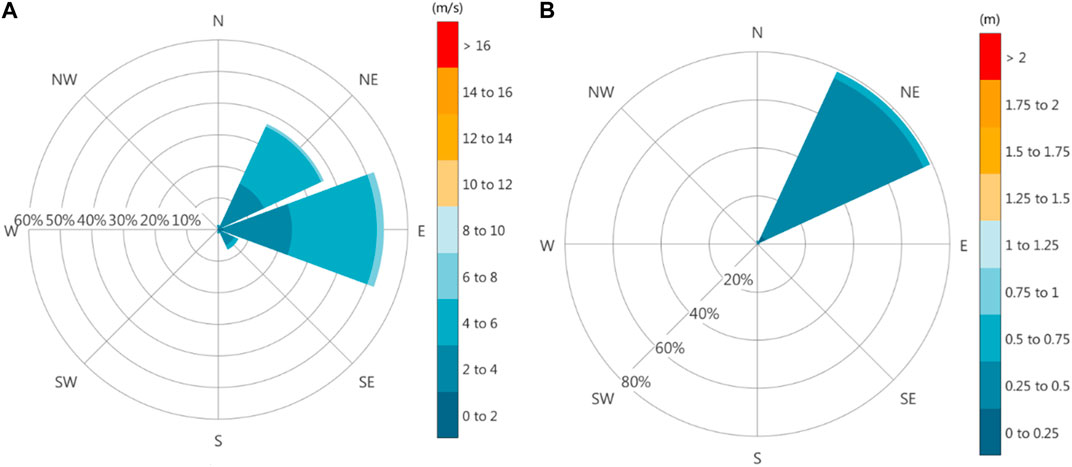
FIGURE 8. Wave roses showing the frequency of wind direction (A) and significant wave height (B) at the mouth of Mahaica River. (Source from AP MER https://www.seastates.net/explore-data/).
The state of the tide is one of the most important coastal forcing factors that drive morphological coastline changes (Oyedotun and Burningham, 2019). The occurrence of high waves at high tide has a greater propensity to cause indelible hazards and significant modification of landforms on the coastline (Summerfield, 2014; Gardner, 2020; Swain, 2022). The semi-diurnal tide at the mouth of Mahaica River ranges from 1 to 3 m at neap and spring tide (Figure 9). Tidal currents here are generally weak (0.2–0.5 ms−1). The lowest monthly water levels at the recorded period are around 0–0.5 m while the monthly maximum is around 3.0–3.5 m (Figure 9).
There was no indication of storm events, storm surges or forcing at the site during the period 2015–2021 of this study. Therefore, the continuous and relative rise in sea level of the Guyana coast (Government of Guyana, 2012), the observed relative constant semi-diurnal tidal conditions and the observed wave parameters are the natural forcing thought to drive the morphological dynamics and small changes at the mouth of Mahaica river reported in this study. Apart from extensive anthropogenic processes, whose impact on this site is limited (Winterwerp et al., 2020), sedimentary characteristics, the geological bedform framework and precursor geomorphology are thought to determine the reported sensitivity of the river mouth planform to changes in the forcing factors observed here as with other documented in published work for other environments (e.g., Blott et al., 2006; Lim et al., 2011; Hunt et al., 2015; Naylor et al., 2017; Oyedotun and Burningham, 2019; Ciarletta et al., 2021; Green et al., 2022; Nanson et al., 2022; Woodroffe et al., 2022; Georgiou et al., 2023).
3.4 Mangroves dynamics at the river mouth
Over the space of approximately a decade (1992–2001), there was a significant reduction in the number of mangroves along the Guyana coast from ∼80,000 to ∼22,000 ha (Conservation International, 2018; Bovell, 2019; Nedd et al., 2021). This significant reduction and depletion are a result of significant anthropogenic (economic) activities (NAREI, 2015; Conservation International, 2018; Bovell, 2019; Nedd et al., 2021) principally, and to a minimal extent, the natural phenomenon (e.g., sea level rise) (Conservation International, 2018). The institution of the Guyana Mangrove Restoration Project (GMRP) among other intervention mechanisms has led to an increase in the number of restoration mangroves along the Guyana coast to approximately 33,362 ha (Guyana Forestry Commission, 2011). Of all the regions of Guyana, the area of the study site is one of the locations noted to be in good condition that was considered to be pristine with limited economic development pressure (Winterwerp et al., 2020). Mud deposits are very important for mangrove growth and sustainability. The migrations of mud-bank involve the spatiotemporal alternations of the bank and their inter-bank phases under the influence of periodic tidal and wave recycling of muddy sediments and/or materials at the yearly/annual timescales (Anthony et al., 2010). Significant mud migration, mud advection, mud deposition and general mud-bank dynamics affect mangroves that depend on mud for their sustenance. Sediment supply is very important for accretion and the prevention of mangrove area loss (Lovelock et al., 2015; Bozi et al., 2021). This section of this study examines how the mangroves in the vicinity of the study area have responded to the yearly mud-bank dynamics.
The spectral and spatial analyses of the mangrove forest cover were conducted to capture the extent of mangrove forest cover changes within the vicinity of the study. The spectral analyses were considered to avoid consideration of other flora and ecosystems, thereby preventing misclassification (after Ranjan et al., 2017; Li et al., 2019; Nedd et al., 2021). The temporal maps presented in Figure 10 illustrate the areas of spatial changes in the mangrove forest cover during the period under consideration. There was a record of differential mangrove gains and losses annually in extent and coverage during the period under consideration. Table 4 presents the estimated annual mangrove forest coverage (in square meter area extent) for the study site while Table 5 presents the estimated extent of gain and loss during the period of this study.
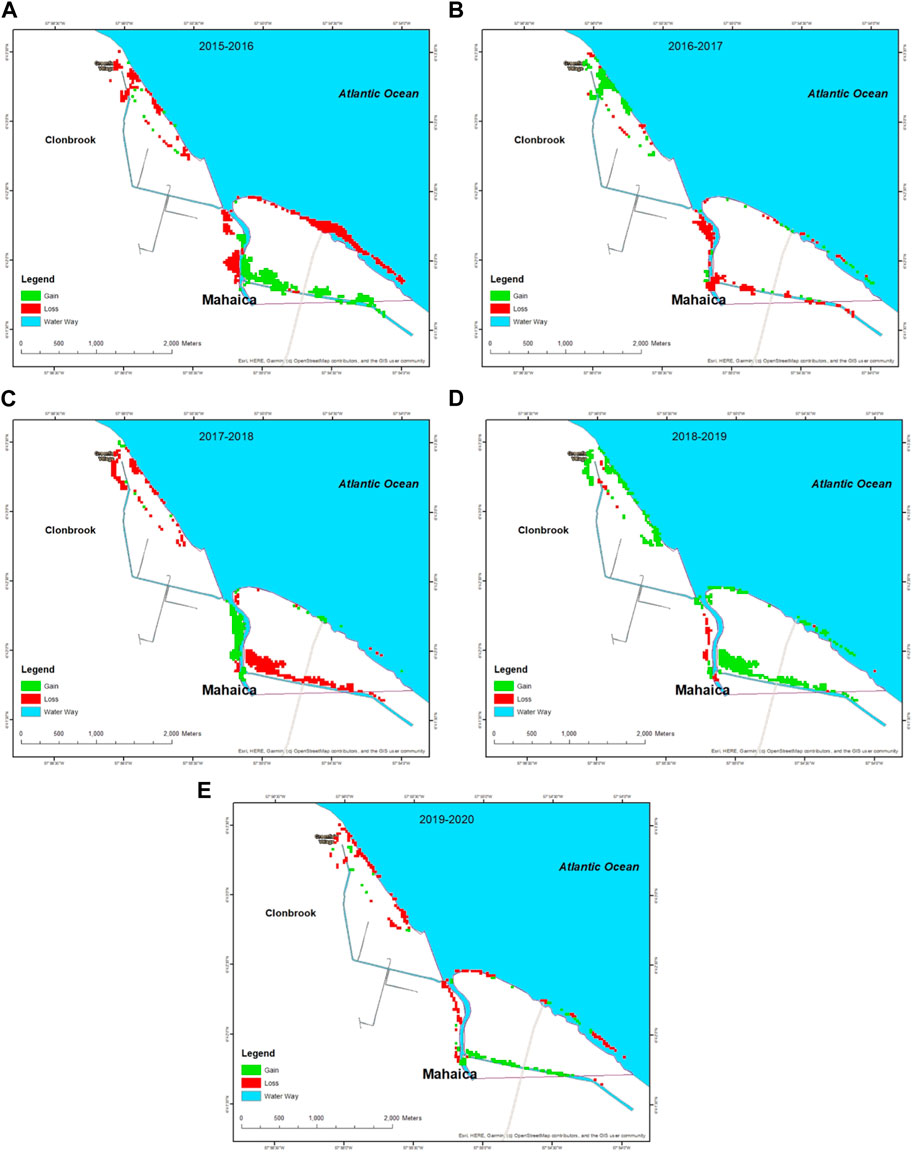
FIGURE 10. Mangrove dynamics in the vicinity of Mahaica River, between 2015 and 2016 (A), 2016 and 2017 (B), 2017 and 2018 (C), 2018 and 2019 (D) and 2019 and 2020 (E) respectively.
Although it appears that mangrove coverage at the study site is being affected by the movement of mud deposits as indicated by the time-series analysis of the mangrove coverage changes. There should be a cautious interpretation of this phenomenon as mangrove forest cover can change under different conditions besides mud-bank migration and movements. The changes observed here could be in combination with other factors that affect other mangrove systems in the region, for example, as a response to relative sea-level rise (Cohen et al., 2009; Cohen et al., 2018; Oyedotun and Johnson-Bhola, 2019), annual average temperature changes that affect the mangrove structure and productivity (Cohen and Lara, 2003), the observed tidal diurnal range and wave action and currents along the coast (Cohen et al., 2005), coastal topography (Castro et al., 2013) and the supply of sediments to the coastal depositional system discussed here. It is, therefore, vital to identify the mangrove dynamics according to many changing variables along these coasts with geomorphological (Augustinus, 1995; Souza et al., 2022), metocean (meteorological and oceanography) particularities (Alfredini et al., 2013; Pezzoli et al., 2013; Reed et al., 2022), climatic dynamics (López-Angarita et al., 2016; Yao et al., 2022), vegetation and pedology changes (Alongi, 2015; Smith and Mayle, 2018; Henriques et al., 2022), mangrove diversity and the influence of mangrove pests, and various in-situ and adjoining anthropogenic activities (Alongi, 2002; Restrepo, 2012; López-Angarita et al., 2016; Thomas et al., 2017; Gorman, 2018; Servino et al., 2018; Pelage et al., 2019; Goldberg et al., 2020; Maina et al., 2021; Wilhelm et al., 2023), etc. as vital factors for consideration in understanding the different and annual dynamic responses presented by this unique ecosystem.
4 Conclusion
The results of the analyses show that the dynamics of the mud shoal and river mouth are governed by feedback from various estuarine and hydrodynamic processes resulting from the interactions between rivers and seas. The results of this study have not only highlighted the importance of mud infilling and sediment build-up for spit development and river-mouth deflection but the influence and morphological dynamics of the sediment (principally mud at the study site) also impact spit development at the river mouth in addition to influencing the marine ecosystem processes, especially mangrove dynamics at the study site. This study which analysed and probed the spatial and temporal structure of the river mouth forms and the mangrove coverage utilised the change detection technique in Google Earth Engine (GEE) on Synthetic Aperture Radar (SAR) images, which allowed for the identification of sediment and mud deposit changes and mangrove forest cover changes of the study area during the short-term period considered. Although there are widely established findings in the literature that indicate the influence of large rivers in modifying the river mouth morphology and dynamics, the findings reported here have shown that the mouth of a small river system also impacts river mouth morphology significantly by the sediment deposited and also by the metocean factors that contribute in the deflection alongshore of deposited sediments (mud) for spit development and mangrove dynamics.
Data availability statement
The original contributions presented in the study are included in the article/Supplementary Material, further inquiries can be directed to the corresponding author.
Author contributions
TDTO: conceptualization, investigation, writing—reviewing and editing; NEDD, GAN: investigation, reviewing. All authors listed have made a substantial, direct, and intellectual contribution to the work and approved it for publication.
Acknowledgments
We appreciate United Nations Development Programme –Indonesia and Archipelagic and Island States (AIS) Forum for the 2021 Archipelagic and Island States Innovation Challenges Award given for ideas in Climate Change Mitigation and Adaptation project which led to the concept and study presented in this paper. The authors would like to fully acknowledge that this study is part of a larger project entitled Guyana Catchment Coastal Observatory which has received financial support through the Greater Guyana Initiative, a joint funding venture between ExxonMobil, Hess Corporation and China National Offshore Oil Corporation. The authors also want to acknowledge through the Office of the Vice Chancellor of the University of Guyana, the secretariat support for the Greater Guyana Initiative provided by the Philanthropy Alumni and Civic Engagement Office of the University of Guyana. TDTO also appreciates and acknowledges the 2022 Association of Commonwealth Climate Resilience Challenge Grants awarded in support of his ongoing research on data integration and analysis for the monitoring of coastal dynamics.
Conflict of interest
The authors declare that the research was conducted in the absence of any commercial or financial relationships that could be construed as a potential conflict of interest.
Publisher’s note
All claims expressed in this article are solely those of the authors and do not necessarily represent those of their affiliated organizations, or those of the publisher, the editors and the reviewers. Any product that may be evaluated in this article, or claim that may be made by its manufacturer, is not guaranteed or endorsed by the publisher.
References
Afentoulis, V., Papadimitriou, A., Belibassakis, K., and Tsoukala, V. (2022). A coupled model for sediment transport dynamics and prediction of seabed morphology with application to 1DH/2DH coastal engineering problems. Oceanologia 64, 514–534. doi:10.1016/j.oceano.2022.03.007
Alfredini, P., Arasaki, E., Pezzoli, A., and Fournier, J. (2013). Impact of climate changes on the santos harbor, são paulo state (Brazil). TransNav Int. J. Mar. Navigation Saf. Sea Transp. 7 (4), 609–617. doi:10.12716/1001.07.04.17
Alongi, D. M. (2002). Present state and future of the world's mangrove forests. Environ. Conserv. 29 (3), 331–349. doi:10.1017/s0376892902000231
Alongi, D. M. (2015). The impact of climate change on mangrove forests. Curr. Clim. Change Rep. 1 (1), 30–39. doi:10.1007/s40641-015-0002-x
Anthony, E. J. (2016). Deltas. Geoscience, Oxford: Oxford University Press, 32. doi:10.1093/OBO/9780199363445-0057
Anthony, E. J., Gardel, A., and Gratiot, N. (2014). Fluvial sediment supply, mud banks, cheniers and the morphodynamics of the coast of South America between the Amazon and Orinoco river mouths. Geol. Soc. Lond. Spec. Publ. 388 (1), 533–560. doi:10.1144/sp388.8
Anthony, E. J., Gardel, A., Gratiot, N., Proisy, C., Allison, M. A., Dolique, F., et al. (2010). The amazon-influenced muddy coast of South America: A review of mud-bank–shoreline interactions. Earth-Science Rev. 103 (3-4), 99–121. doi:10.1016/j.earscirev.2010.09.008
Anthony, E. J., Gardel, A., Proisy, C., Fromard, F., Gensac, E., Peron, C., et al. (2013). The role of fluvial sediment supply and riverriver-mouth hydrology in the dynamics of the muddy, amazon-dominated amapá–guianas coast, South America: A three-point research agenda. J. S. Am. Earth Sci. 44, 18–24. doi:10.1016/j.jsames.2012.06.005
Anthony, E. J., and Gratiot, N. (2012). Coastal engineering and large-scale mangrove destruction in Guyana, South America: Averting an environmental catastrophe in the making. Ecol. Eng. 47, 268–273. doi:10.1016/j.ecoleng.2012.07.005
Anthony, E. J. (2015). Wave influence in the construction, shaping and destruction of river deltas: A review. Mar. Geol. 361, 53–78. doi:10.1016/j.margeo.2014.12.004
Ashton, A., Murray, A. B., and Arnoult, O. (2001). Formation of coastline features by large-scale instabilities induced by high-angle waves. Nature 414, 296–300. doi:10.1038/35104541
Augustinus, P. G. (1995). Geomorphology and sedimentology of mangroves. Dev. sedimentology 53, 333–357.
Besset, M., Anthony, E. J., and Bouchette, F. (2019). Multi-decadal variations in delta shorelines and their relationship to river sediment supply: An assessment and review. Earth-science Rev. 193, 199–219. doi:10.1016/j.earscirev.2019.04.018
Bhattacharya, J. P., and Giosan, L. (2003). Wave-influenced deltas: Geomorphological implications for facies reconstruction. Sedimentology 50 (1), 187–210. doi:10.1046/j.1365-3091.2003.00545.x
Blott, S. J., Pye, K., van der Wal, D., and Neal, A. (2006). Long-term morphological change and its causes in the mersey estuary, NW england. Geomorphology 81, 185–206. doi:10.1016/j.geomorph.2006.04.008
Bovell, O. (2019). “Setting the foundations for zero net loss of the mangroves that underpin human wellbeing in the north Brazil shelf LME: Review of the effectiveness of existing coastal restoration efforts in Guyana,” in Conservation international and the national agricultural research and extension Institute (Washington DC: Georgetown).
Bozi, B. S., Figueiredo, B. L., Rodrigues, E., Cohen, M. C., Pessenda, L. C., Alves, E. E., et al. (2021). Impacts of sea-level changes on mangroves from southeastern Brazil during the Holocene and Anthropocene using a multi-proxy approach. Geomorphology 390, 107860. doi:10.1016/j.geomorph.2021.107860
Broadley, A., Stewart-Koster, B., Burford, M. A., and Brown, C. J. (2022). A global review of the critical link between river flows and productivity in marine fisheries. Rev. Fish Biol. Fish. 32 (3), 805–825. doi:10.1007/s11160-022-09711-0
Cai, M., Colin, C., Xu, Z., Wang, W., Cui, Y., Yu, Z., et al. (2022). Climate and sea level forcing of terrigenous sediments input to the eastern Arabian Sea since the last glacial period. Mar. Geol. 450, 106860. doi:10.1016/j.margeo.2022.106860
Cañedo-Argüelles, M., Brito, A. C., Sen, I., and Roy, R. (2023). Human impacts on river catchments and coastal ecosystems: A meta-ecosystem perspective. Front. Mar. Sci. 10, 470.
Castro, D. F., Rossetti, D. F., Cohen, M. C. L., Pessenda, L. C. R., and Lorente, F. L. (2013). The growth of the Doce River Delta in northeastern Brazil indicated by sedimentary facies and diatoms. Diatom Res. 28, 455–466. doi:10.1080/0269249X.2013.841100
Ciarletta, D. J., Miselis, J. L., Shawler, J. L., and Hein, C. J. (2021). Quantifying thresholds of barrier geomorphic change in a cross-shore sediment-partitioning model. Earth Surf. Dyn. 9 (2), 183–203. doi:10.5194/esurf-9-183-2021
Cohen, M. C. L., Behling, H., and Lara, R. J. (2005). Amazonian mangrove dynamics during the last millennium: The relative seasea-level and the little ice age. Rev. Palaeobot. Palynol. 136, 93–108. doi:10.1016/j.revpalbo.2005.05.002
Cohen, M. C. L., Behling, H., Lara, R. J., Smith, C. B., Matos, H. R. S., and Vedel, V. (2009) Impact of sealevel and climatic changes on the Amazon coastal wetlands during the late Holocene. Veg. Hist. Archaeobot. 18, 425–439. doi:10.1007/s00334-008-0208-0
Cohen, M. C. L., de Souza, A. V., Rossetti, D. F., Pessenda, L. C. R., and França, M. C. (2018). Decadal scale dynamics of an amazonian mangrove caused by climate and sea level changes: Inferences from spatial-temporal analysis and digital elevation models. Earth Surf. Process. Landforms 43, 2876–2888. doi:10.1002/esp.4440
Cohen, M. C. L., and Lara, R. J. (2003). Temporal changes of mangrove vegetation boundaries in Amazonia: Application of GIS and remote sensing techniques. Wetl. Ecol. Manag. 11, 223–231. doi:10.1023/A:1025007331075
Collins, A. L., Walling, D. E., and Leeks, G. J. (1997). Fingerprinting the origin of fluvial suspended sediment in larger river basins: Combining assessment of spatial provenance and source type. Geogr. Ann. Ser. A, Phys. Geogr. 79 (4), 239–254. doi:10.1111/1468-0459.00020
Conservation International (2018). “Setting the foundations for zero net loss of the mangroves that underpin human wellbeing in the north Brazil shelf LME: State of mangroves in Guyana: An analysis of research gaps, and recommendations,” in Conservation international (Georgetown: Georgetown University).
Dan, S., Walstra, D. J. R., Stive, M. J., and Panin, N. (2011). Processes controlling the development of a river mouth spit. Mar. Geol. 280 (1-4), 116–129. doi:10.1016/j.margeo.2010.12.005
Daramola, S., Li, H., Omonigbehin, O., Faruwa, A., and Gong, Z. (2022). Recent retreat and flood dominant areas along the muddy Mahin coastline of Ilaje, Nigeria. Regional Stud. Mar. Sci. 52, 102272. doi:10.1016/j.rsma.2022.102272
de Vries, J., van Maanen, B., Ruessink, G., Verweij, P. A., and de Jong, S. M. (2022). Multi-decadal coastline dynamics in Suriname controlled by migrating subtidal mudbanks. Earth Surf. Process. Landforms 47, 2500–2517. doi:10.1002/esp.5390
Deigaard, R., and Fredsøe, J. (2005). “The shape of equilibrium coastlines,” in Proceedings of international coastal symposium (Höfn, Iceland: Innovation Center of East-Iceland), 134–149.
Deng, J., Harff, J., Zhang, W., Schneider, R., Dudzińska-Nowak, J., Giza, A., et al. (2017). “The dynamic equilibrium shore model for the reconstruction and future projection of coastal morphodynamics,” in Coastline changes of the baltic sea from South to east (Cham: Springer), 87–106.
Devrani, R., Srivastava, P., Kumar, R., and Kasana, P. (2022). Characterization and assessment of flood inundated areas of lower brahmaputra river basin using multitemporal synthetic aperture radar data: A case study from NE India. Geol. J. 57 (2), 622–646. doi:10.1002/gj.4365
Dominguez, J. M., Martin, L., and Bittencourt, A. C. (1987). “Sea-Level history and quaternary evolution of River mouth–associated beachridge plains along the east–southeast Brazilian coast: A summary,” in The Society of Economic Paleontologists and Mineralogists.
Dominguez, J. M. (2009). “The coastal zone of Brazil,” in Geology and geomorphology of holocene coastal barriers of Brazil (Berlin, Heidelberg: Springer), 17–51.
Eilander, D., Couasnon, A., Ikeuchi, H., Muis, S., Yamazaki, D., Winsemius, H. C., et al. (2020). The effect of surge on riverine flood hazard and impact in deltas globally. Environ. Res. Lett. 15 (10), 104007. doi:10.1088/1748-9326/ab8ca6
Elias, E. P., Pearson, S. G., van der Spek, A. J., and Pluis, S. (2022). Understanding meso-scale processes at a mixed-energy tidal inlet: Ameland Inlet, The Netherlands–Implications for coastal maintenance. Ocean Coast. Manag. 222, 106125. doi:10.1016/j.ocecoaman.2022.106125
Estournel, C., Mikolajczak, G., Ulses, C., Bourrin, F., Canals, M., Charmasson, S., et al. (2023). Sediment dynamics in the Gulf of Lion (NW Mediterranean Sea) during two autumn–winter periods with contrasting meteorological conditions. Prog. Oceanogr. 210, 102942. doi:10.1016/j.pocean.2022.102942
Fagherazzi, S., Edmonds, D. A., Nardin, W., Leonardi, N., Canestrelli, A., Falcini, F., et al. (2015). Dynamics of river mouth deposits. Rev. Geophys. 53 (3), 642–672. doi:10.1002/2014rg000451
Filipponi, F. (2019). Sentinel-1 GRD preprocessing workflow. Proceedings 18, 11. doi:10.3390/ECRS-3-06201
Föeger, L. B., Buarque, D. C., Pontes, P. R. M., de Oliveira Fagundes, H., and Fan, F. M. (2022). Large-scale sediment modeling with inertial flow routing: Assessment of Madeira river basin. Environ. Model. Softw. 149, 105332. doi:10.1016/j.envsoft.2022.105332
Gardel, A., Anthony, E. J., Dos Santos, V. F., Huybrechts, N., Lesourd, S., Sottolichio, A., et al. (2021). Fluvial sand, Amazon mud, and sediment accommodation in the tropical Maroni River estuary: Controls on the transition from estuary to delta and chenier plain. Regional Stud. Mar. Sci. 41, 101548. doi:10.1016/j.rsma.2020.101548
Gardel, A., Anthony, E. J., Santos, V. F., Huybrechts, N., Lesourd, S., Sottolichio, A., et al. (2022). A remote sensing-based classification approach for river mouths of the Amazon-influenced Guianas coast. Reg. Environ. Change 22 (2), 65–12. doi:10.1007/s10113-022-01913-3
Gardner, J. (2020). How water, wind, waves and ice shape landscapes and landforms: Historical contributions to geomorphic science. Geomorphology 366, 106687. doi:10.1016/j.geomorph.2019.02.031
Ge, J., Zhang, J., Chen, C., and Ding, P. (2021). Impacts of fluvial flood on physical and biogeochemical environments in estuary–shelf continuum in the East China Sea. J. Hydrology 598, 126441. doi:10.1016/j.jhydrol.2021.126441
Georgiou, I., Foster-Martinez, M., Fitzpatrick, C., Jarrell, E., Bridgeman, J., Lee, D., et al. (2023). Coastal master plan: Barrier island model improvements. Version I, 42.
Ghanbari, M., Xu, L., and Clausi, D. A. (2023). Local and global spatial information for land cover semi-supervised classification of complex polarimetric SAR data. IEEE J. Sel. Top. Appl. Earth Observations Remote Sens. 16, 3892–3904. doi:10.1109/jstars.2023.3264452
Glover, H. E., Ogston, A. S., Fricke, A. T., Nittrouer, C. A., Aung, C., Naing, T., et al. (2021). Connecting sediment retention to distributary-channel hydrodynamics and sediment dynamics in a tide-dominated delta: The ayeyarwady delta, Myanmar. J. Geophys. Res. Earth Surf. 126 (3), e2020JF005882. doi:10.1029/2020jf005882
Glover, H. E., Ogston, A. S., Fricke, A. T., Nittrouer, C. A., Aung, C., Naing, T., et al. (2023). Pathways for sediment transport and retention in a vegetated, mid-channel island: Connecting sediment dynamics to morphology in Meinmahla Island, Ayeyarwady Delta, Myanmar. Sedimentology 70 (1), 214–234. doi:10.1111/sed.13036
Goldberg, L., Lagomasino, D., Thomas, N., and Fatoyinbo, T. (2020). Global declines in human-driven mangrove loss. Glob. change Biol. 26 (10), 5844–5855. doi:10.1111/gcb.15275
Gorman, D. (2018). “Historical losses of mangrove systems in South America from human-induced and natural impacts,” in Threats to mangrove forests (Cham: Springer), 155–171.
Government of Guyana (2012). Guyana second national communication to the UNFCCC. Avaliable At: https://unfccc.int/resource/docs/natc/guync2.pdf.
Gratiot, N., Gardel, A., and Anthony, E. .J. (2007). Trade-wind waves and mud dynamics on the French Guiana coast, South America: Input from ERA-40 wave data and field investigations. Mar. Geol. 236, 15–26. doi:10.1016/j.margeo.2006.09.013
Green, A. N., Humphries, M. S., Cooper, J. A. G., Strachan, K. L., Gomes, M., and Dladla, N. N. (2022). The Holocene evolution of Lake St Lucia, Africa's largest estuary: Geological implications for contemporary management. Estuar. Coast. Shelf Sci. 266, 107745. doi:10.1016/j.ecss.2022.107745
Hagenlocher, M., Renaud, F. G., Haas, S., and Sebesvari, Z. (2018). Vulnerability and risk of deltaic social ecological systems exposed to multiple hazards. Sci. Total Environ. 631-632, 71–80. doi:10.1016/j.scitotenv.2018.03.013
Haque, M. M., Ghosh, M. K., and Hoyanagi, K. (2022). Coastal development in southwestern Bangladesh: Understanding the interplay between storms and sea level rise. Prog. Phys. Geogr. Earth Environ. 46 (3), 331–356. doi:10.1177/03091333211046189
Harvey, M. E., Giddings, S. N., Pawlak, G., and Crooks, J. A. (2023). Hydrodynamic variability of an intermittently closed estuary over interannual, seasonal, fortnightly, and tidal timescales. Estuaries Coasts 46 (1), 84–108. doi:10.1007/s12237-021-01014-0
Hashimoto, K., Shimozono, T., Matsuba, Y., and Okabe, T. (2021). Unmanned aerial vehicle depth inversion to monitor river-mouth bar dynamics. Remote Sens. 13 (3), 412. doi:10.3390/rs13030412
Henriques, R. J., de Oliveira, F. S., Schaefer, C. E. G. R., Francelino, M. R., Lopes, P. R. C., Senra, E. O., et al. (2022). Soils and landscapes of Marajó island, Brazilian Amazonia: Holocene evolution, geoarchaeology and climatic vulnerability. Environ. Earth Sci. 81 (9), 254–325. doi:10.1007/s12665-022-10310-2
Hicks, D. M., and Hume, T. M. (1996). Morphology and size of ebb tidal deltas at natural inlets on open-sea and pocket-bay coasts, North Island, New Zealand. J. Coast. Res. 12 (1), 47–63.
Hunt, S., Bryan, K. R., and Mullarney, J. C. (2015). The influence of wind and waves on the existence of stable intertidal morphology in meso-tidal estuaries. Geomorphology 228, 158–174. doi:10.1016/j.geomorph.2014.09.001
Johnson, D. (2016). Wildlife lures nature lovers along Mahaica River. Stabroek News. 2016-09-24 to 2021-01-18.
Jolivet, M., Gardel, A., and Anthony, E. J. (2019). Multi-decadal changes on the mud-dominated coast of Western French Guiana: Implications for mesoscale shoreline mobility, river-mouth deflection, and sediment sorting. J. Coast. Res. 88 (SI), 185–194. doi:10.2112/si88-014.1
Kang, J. W., and Jun, K. S. (2003). Flood and ebb dominance in estuaries in Korea. Estuar. Coast. Shelf Sci. 56 (1), 187–196. doi:10.1016/s0272-7714(02)00156-7
Kar, R., and Sarkar, A. (2023). “Impact of anthropocene on the fluvial sediment supply: The mahanadi river basin perspective,” in River dynamics and flood hazards (Singapore: Springer), 241–282.
Knight, J. (2023). Nature-based solutions for coastal resilience in South Africa. South Afr. Geogr. J. 2023, 1–30. doi:10.1080/03736245.2023.2193565
Kolb, W., and Schmidt, H. (1991). Depositional sequences associated with equilibrium coastlines in the Neogene of South-Western Nicaragua. Sediment. Tect. eustasy sea-level changes A. T. Act. margins 12, 259–272.
Kondolf, G. M., Schmitt, R. J., Carling, P., Darby, S., Arias, M., Bizzi, S., et al. (2018). Changing sediment budget of the Mekong: Cumulative threats and management strategies for a large river basin. Sci. total Environ. 625, 114–134. doi:10.1016/j.scitotenv.2017.11.361
Lakshmanna, B., Jayaraju, N., Sreenivasulu, G., Prasad, T. L., Nagalakshmi, K., Kumar, M. P., et al. (2023). “A study on dynamics of krishna River Mouth, east coast of India: A geospatial approach,” in Coasts, estuaries and lakes: Implications for sustainable development (Cham: Springer International Publishing), 459–469.
Lee, J., Jurkevich, L., Dewaele, P., Wambacq, P., and Oosterlinck, A. (1994). Speckle filtering of synthetic aperture radar images: A review. Remote Sens. Rev. 8, 313–340. doi:10.1080/02757259409532206
Lefebvre, J. P., Dolique, F., and Gratiot, N. (2004). Geomorphic evolution of a coastal mudflat under oceanic influences: An example from the dynamic shoreline of French Guiana. Mar. Geol. 208 (2-4), 191–205. doi:10.1016/j.margeo.2004.04.008
Li, G., Mei, L., Ye, Q., Pang, X., Zheng, J., and Li, W. (2023). Post-rift faulting controlled by different geodynamics in the pearl River Mouth basin, northern South China sea margin. Earth-Science Rev. 237, 104311. doi:10.1016/j.earscirev.2022.104311
Li, J., Roughan, M., Kerry, C., and Rao, S. (2022). Impact of mesoscale circulation on the structure of River plumes during large rainfall events inshore of the east Australian current. Front. Mar. Sci. 9, 156. doi:10.3389/fmars.2022.815348
Li, W., El-Askary, H., Qurban, M. A., Li, J., ManiKandan, K., and Piechota, T. (2019). Using multi-indices approach to quantify mangrove changes over the Western Arabian Gulf along Saudi Arabia coast. Ecol. Indic. 102, 734–745. doi:10.1016/j.ecolind.2019.03.047
Lim, M., Rosser, N. J., Petley, D. N., and Keen, M. (2011). Quantifying the controls and influence of tide and wave impacts on coastal rock cliff erosion. J. Coast Res. 27, 46–56. doi:10.2112/jcoastres-d-09-00061.1
Lincoln, J. M., and Fitzgerald, D. M. (1988). Tidal distortions and flood dominance at five small tidal inlets in southern Maine. Mar. Geol. 82 (3-4), 133–148. doi:10.1016/0025-3227(88)90137-5
Liu, C., Walling, D. E., and He, Y. (2018). Review: The International Sediment Initiative case studies of sediment problems in river basins and their management. Int. J. Sediment Res. 33 (2), 216–219. doi:10.1016/j.ijsrc.2017.05.005
López-Angarita, J., Roberts, C. M., Tilley, A., Hawkins, J. P., and Cooke, R. G. (2016). Mangroves and people: Lessons from a history of use and abuse in four Latin American countries. For. Ecol. Manag. 368, 151–162. doi:10.1016/j.foreco.2016.03.020
Lovelock, C. E., Cahoon, D. R., Friess, D. A., Guntenspergen, G. R., Krauss, K. W., Reef, R., et al. (2015). The vulnerability of Indo-Pacific mangrove forests to sea-level rise. Nature 526, 559–563. doi:10.1038/nature15538
Lu, X. X., Ashmore, P., and Wang, J. (2003). Sediment yield mapping in a large river basin: The upper yangtze, China. Environ. Model. Softw. 18 (4), 339–353. doi:10.1016/s1364-8152(02)00107-x
Luijendijk, A. P., de Schipper, M. A., and Ranasinghe, R. (2019). Morphodynamic acceleration techniques for multi-timescale predictions of complex sandy interventions. J. Mar. Sci. Eng. 7 (3), 78. doi:10.3390/jmse7030078
Maina, J. M., Bosire, J. O., Kairo, J. G., Bandeira, S. O., Mangora, M. M., Macamo, C., et al. (2021). Identifying global and local drivers of change in mangrove cover and the implications for management. Glob. Ecol. Biogeogr. 30 (10), 2057–2069. doi:10.1111/geb.13368
Manzolli, R. P., Portz, L., Villate-Daza, D., Pulido-Nossa, D., García-Becerra, D., and Alcántara-Carrió, J. (2022). Recent geomorphological evolution of bar channels in Magdalena River (Colombia) due to natural and anthropogenic interferences. Estuar. Coast. Shelf Sci. 275, 10795. doi:10.1016/j.ecss.2022.107959
Marghany, M. (2013). DInSAR technique for three-dimensional coastal spit simulation from radarsat-1 fine mode data. Acta Geophys. 61, 478–493. doi:10.2478/s11600-012-0061-5
Marghany, M. (2014). Simulation of three-dimensional of coastal erosion using differential interferometric synthetic aperture radar. Glob. NEST J. 16 (1), 80–86.
Marghany, M. (2012). Three-dimensional coastal geomorphology deformation modelling using differential synthetic aperture interferometry. Zeitschrift fur Naturforschung A-Journal Phys. Sci. 67 (6), 419.
Matano, F. (2019). Analysis and classification of natural and human-induced ground deformations at regional scale (Campania, Italy) detected by satellite synthetic-aperture radar interferometry archive datasets. Remote Sens. 11 (23), 2822. doi:10.3390/rs11232822
McLusky, D. S., and Elliott, M. (2004). The estuarine ecosystem: Ecology, threats and management. Oxford: OUP.
McLusky, D. S., and Elliott, M. (2007). Transitional waters: A new approach, semantics or just muddying the waters? Estuar. Coast. Shelf Sci. 71 (3-4), 359–363. doi:10.1016/j.ecss.2006.08.025
Meyer, F. (2019). “Spaceborne synthetic aperture radar: Principles, data access, and basic processing techniques,” in The SAR handbook (Huntsville: SERVIR Global Science). doi:10.25966/nr2c-s697
Mikhailov, V. N., and Gorin, S. L. (2012). New definitions, regionalization, and typification of river mouth areas and estuaries as their parts. Water Resour. 39, 247–260. doi:10.1134/s0097807812030050
Montreuil, A. L., and Bullard, J. E. (2012). A 150-year record of coastline dynamics within a sediment cell: Eastern England. Geomorphology 179, 168–185. doi:10.1016/j.geomorph.2012.08.008
Moyano-Paz, D., Isla, M. F., MacEachern, J. A., Richiano, S., Gómez-Dacal, A. R., Varela, A. N., et al. (2022). Evolution of an aggradational wave-dominated delta: Sediment balance and animal-substrate dynamics (Upper cretaceous La Anita Formation, Southern Patagonia). Sediment. Geol. 437, 106193. doi:10.1016/j.sedgeo.2022.106193
Mushi, C. A., Ndomba, P. M., Trigg, M. A., Tshimanga, R. M., and Mtalo, F. (2019). Assessment of basin-scale soil erosion within the Congo river basin: A review. Catena 178, 64–76. doi:10.1016/j.catena.2019.02.030
Nanson, R., Bishop-Taylor, R., Sagar, S., and Lymburner, L. (2022). Geomorphic insights into Australia's coastal change using a national dataset derived from the multi-decadal Landsat archive. Estuar. Coast. Shelf Sci. 265, 107712. doi:10.1016/j.ecss.2021.107712
NAREI (2015). Mangrove restoration and management department, annual report. Mon Repos, Guyana: National Agriculture Research and Extension Institute.
Naylor, L. A., Spencer, T., Lane, S. N., Darby, S. E., Magilligan, F. J., Macklin, M. G., et al. (2017). Stormy geomorphology: Geomorphic contributions in an age of climate extremes. Earth Surf. Process. Landforms 42 (1), 166–190. doi:10.1002/esp.4062
Nedd, G. A., Oyedotun, T. D. T., and Simard, M. (2021). Evaluation of spatio-temporal dynamics of Guyana’s mangroves using SAR and GEE. Earth Syst. Environ. 2021, 1–16. doi:10.1007/s41748-021-00277-8
Ngobeni, D., and Knight, J. (2023). Evaluation of river mouth dynamics along the Eastern Cape coastline. South Africa: Transactions of the Royal Society of South Africa, 1–14.
Nienhuis, J. H., Ashton, A. D., Nardin, W., Fagherazzi, S., and Giosan, L. (2016). Alongshore sediment bypassing as a control on river mouth morphodynamics. J. Geophys. Res. Earth Surf. 121 (4), 664–683. doi:10.1002/2015jf003780
Nilawar, A. P., and Waikar, M. L. (2019). Impacts of climate change on streamflow and sediment concentration under rcp 4.5 and 8.5: A case study in purna river basin, India. Sci. total Environ. 650, 2685–2696. doi:10.1016/j.scitotenv.2018.09.334
Ouchi, K. (2013). Recent trend and advance of synthetic aperture radar with selected topics. Remote Sens. 5 (2), 716–807. doi:10.3390/rs5020716
Oyedotun, T. D. T., and Burningham, H. (2019). Comparable short-term morphodynamics of three estuarine–coastal systems in the southwest coastal region of England, UK. Regional Stud. Mar. Sci. 31, 100749. doi:10.1016/j.rsma.2019.100749
Oyedotun, T. D. T., and Burningham, H. (2021). The need for data integration to address the challenges of climate change on the Guyana coast. Geogr. Sustain. 2 (4), 288–297. doi:10.1016/j.geosus.2021.11.003
OyedotunJohnson-Bhola, T. D. T. L. (2019). Shoreline change identification and analysis along the coast of Guyana, South America. Interdiscip. Environ. Rev. 20 (1), 7–31. doi:10.1504/ier.2019.10019703
Özpolat, E., and Demir, T. (2019). The spatiotemporal shoreline dynamics of a delta under natural and anthropogenic conditions from 1950 to 2018: A dramatic case from the eastern mediterranean. Ocean Coast. Manag. 180, 104910. doi:10.1016/j.ocecoaman.2019.104910
Padmalal, D., Kumaran, K. P. N., Nair, K. M., Limaye, R. B., Mohan, S. V., Baijulal, B., et al. (2014). Consequences of sea level and climate changes on the morphodynamics of a tropical coastal lagoon during holocene: An evolutionary model. Quat. Int. 333, 156–172. doi:10.1016/j.quaint.2013.12.018
Pan, D., Wang, Z., Zhan, Q., Saito, Y., Wu, H., Yang, S., et al. (2020). Organic geochemical evidence of past changes in hydro-and sediment-dynamic processes at river mouths: A case study of holocene sedimentary records in the changjiang river delta, China. Cont. Shelf Res. 204, 104189. doi:10.1016/j.csr.2020.104189
Parvathy, K. G., Noujas, V., Thomas, K. V., and Ramesh, H. (2015). Impact of mudbanks on coastal dynamics. Aquat. Procedia 4, 1514–1521. doi:10.1016/j.aqpro.2015.02.196
Paul, A. K., and Paul, A. (2022). “Adjustment of the coastal communities in response to climate variability and sea level rise in the sundarban, West Bengal, India,” in Climate change, disaster and adaptations (Cham: Springer), 201–217.
Pelage, L., Domalain, G., Lira, A. S., Travassos, P., and Frédou, T. (2019). Coastal land use in northeast Brazil: Mangrove coverage evolution over three decades. Trop. Conservation Sci. 12, 194008291882241. doi:10.1177/1940082918822411
Perillo, G. M. (1995). Definitions and geomorphologic classifications of estuaries. Dev. sedimentology 53, 17–47.
Pezzoli, A., Alfredini, P., Arasaki, E., Rosso, M., and de Sousa, W. C. (2013). Impacts of climate changes on management policy of the harbors, land areas and wetlands in the São Paulo State Coastline (Brazil). J. Climatol. & Weather Forecast. 1, 101. doi:10.4172/jcwf.1000101
Phillips, J. D. (1995). Decoupling of sediment sources in large river basins. IAHS Publications-Series Proc. Reports-Intern Assoc Hydrological Sci. 226, 11–16.
Polizel, S., and Burningham, H. (2022). “Doce River mouth dynamics and morphological changes over recent years,” in 10th international conference on geomorphology, Coimbra, Portugal, 12–16 September 2022. ICG2022-603. doi:10.5194/icg2022-603
Ran, L., Lu, X. X., Xin, Z., and Yang, X. (2013). Cumulative sediment trapping by reservoirs in large river basins: A case study of the yellow river basin. Glob. Planet. Change 100, 308–319. doi:10.1016/j.gloplacha.2012.11.001
Ranasinghe, R., Wu, C. S., Conallin, J., Duong, T. M., and Anthony, E. J. (2019). Disentangling the relative impacts of climate change and human activities on fluvial sediment supply to the coast by the world’s large rivers: Pearl River Basin, China. Sci. Rep. 9 (1), 9236–9310. doi:10.1038/s41598-019-45442-2
Ranjan, A., Sivathanu, V., Verma, S., Murmu, L., and Kumar, P. (2017). Spatiotemporal variation in Indian part of Sundarban Delta over the years 1990–2016 using geospatial technology. Int. J. Geomat. Geosci. 7, 275–292.
Reed, A., TajalliBakhsh, T., Klebaner, L., Suman, D. O., and Brooker, J. P. (2022). Coastal impacts of climate change. Env't L. Rep. 52, 10169.
Restrepo, A. J. D. (2012). Assessing the effect of sea-level change and human activities on a major delta on the Pacific coast of northern South America: The Patía River. Geomorphology 151, 207–223. doi:10.1016/j.geomorph.2012.02.004
Ricci, F., Capellacci, S., Campanelli, A., Grilli, F., Marini, M., and Penna, A. (2022). Long-term dynamics of annual and seasonal physical and biogeochemical properties: Role of minor river discharges in the North-Western Adriatic coast. Estuar. Coast. Shelf Sci. 272, 107902. doi:10.1016/j.ecss.2022.107902
Roelvink, D., Huisman, B., Elghandour, A., Ghonim, M., and Reyns, J. (2020). Efficient modeling of complex sandy coastal evolution at monthly to century time scales. Front. Mar. Sci. 7, 535. doi:10.3389/fmars.2020.00535
Romdani, A., Chen, J. L., Chien, H., Lin, J. H., Liao, C. Y., and Hou, C. C. (2023). Case study downdrift port siltation adjacent to a River Mouth: Effects of mesotidal conditions and typhoon. J. Waterw. Port, Coast. Ocean Eng. 149 (2), 05022007.
Servino, R. N., de Oliveira Gomes, L. E., and Bernardino, A. F. (2018). Extreme weather impacts on tropical mangrove forests in the Eastern Brazil Marine Ecoregion. Sci. Total Environ. 628, 233–240. doi:10.1016/j.scitotenv.2018.02.068
Short, A. D., and Jackson, D. W. T. (2013). Beach morphodynamics. Treatise Geomorphol. 2013, 106–129.
Shrestha, S., Bhatta, B., Talchabhadel, R., and Virdis, S. G. P. (2022). Integrated assessment of the landuse change and climate change impacts on the sediment yield in the Songkhram River Basin, Thailand. Catena 209, 105859. doi:10.1016/j.catena.2021.105859
Silvester, R. (1960). Stabilization of sedimentary coastlines. Nature 188 (4749), 467–469. doi:10.1038/188467a0
Smith, R. J., and Mayle, F. E. (2018). Impact of mid-to late holocene precipitation changes on vegetation across lowland tropical South America: A paleo-data synthesis. Quat. Res. 89 (1), 134–155. doi:10.1017/qua.2017.89
Souza, A. D. O., Lämmle, L., Filho, A. P., and Donadio, C. (2022). Recent geomorphological changes in the paraiba do sul delta, South America east coast. Prog. Phys. Geogr. Earth Environ. 46 (4), 566–588. doi:10.1177/03091333221077614
Splinter, K. D., Turner, I. L., Davidson, M. A., Barnard, P., Castelle, B., and Oltman-Shay, J. (2014). A generalized equilibrium model for predicting daily to interannual shoreline response. J. Geophys. Res. Earth Surf. 119 (9), 1936–1958. doi:10.1002/2014jf003106
Sreenivasulu, G., Jayaraju, N., Reddy, B. S. R., Prasad, T. L., Lakshmanna, B., Nagalakshmi, K., et al. (2016). River mouth dynamics of Swarnamukhi estuary, Nellore coast, southeast coast of India. Geodesy Geodyn. 7 (6), 387–395. doi:10.1016/j.geog.2016.09.003
Sun, P., Wu, Y., Gao, J., Yao, Y., Zhao, F., Lei, X., et al. (2020). Shifts of sediment transport regime caused by ecological restoration in the Middle Yellow River Basin. Sci. Total Environ. 698, 134261. doi:10.1016/j.scitotenv.2019.134261
Sundberg, T., Boardsen, S. A., Slavin, J. A., Uritsky, V. M., Anderson, B. J., Korth, H., et al. (2013). Cyclic reformation of a quasi-parallel bow shock at mercury: MESSENGER observations. J. Geophys. Res. Space Phys. 118 (10), 6457–6464. doi:10.1002/jgra.50602
Swain, D. (2022). “Tropical cyclones and coastal vulnerability: Assessment and mitigation,” in Geospatial technologies for land and water resources management (Cham: Springer), 587–621.
Syvitski, J., Ángel, J. R., Saito, Y., Overeem, I., Vörösmarty, C. J., Wang, H., et al. (2022). Earth’s sediment cycle during the Anthropocene. Nat. Rev. Earth Environ. 3 (3), 179–196. doi:10.1038/s43017-021-00253-w
Taft, L., and Evers, M. (2016). A review of current and possible future human–water dynamics in Myanmar's river basins. Hydrology Earth Syst. Sci. 20 (12), 4913–4928. doi:10.5194/hess-20-4913-2016
Tagliapietra, D., Sigovini, M., and Ghirardini, A. V. (2009). A review of terms and definitions to categorise estuaries, lagoons and associated environments. Mar. Freshw. Res. 60 (6), 497–509. doi:10.1071/mf08088
Tangi, M. (2023). “Dynamic sediment connectivity modelling for strategic river basin planning,” in Special topics in information Technology (Cham: Springer), 27–37.
Thomas, N., Lucas, R., Bunting, P., Hardy, A., Rosenqvist, A., and Simard, M. (2017). Distribution and drivers of global mangrove forest change, 1996–2010. PloS one 12 (6), e0179302. doi:10.1371/journal.pone.0179302
Tian, P., Lu, H., Feng, W., Guan, Y., and Xue, Y. (2020). Large decrease in streamflow and sediment load of qinghai–Tibetan plateau driven by future climate change: A case study in lhasa river basin. Catena 187, 104340. doi:10.1016/j.catena.2019.104340
Turki, I., Le Bot, S., Lecoq, N., Shafiei, H., Michel, C., Deloffre, J., et al. (2021). Morphodynamics of intertidal dune field in a mixed wave-tide environment: Case of Baie de Somme in Eastern English Channel. Mar. Geol. 431, 106381. doi:10.1016/j.margeo.2020.106381
van Bijsterveldt, C. E., van der Wal, D., Mancheño, A. G., Fivash, G. S., Helmi, M., and Bouma, T. J. (2023). Can cheniers protect mangroves along eroding coastlines?–The effect of contrasting foreshore types on mangrove stability. Ecol. Eng. 187, 106863. doi:10.1016/j.ecoleng.2022.106863
Van Maren, D. S. (2007). Water and sediment dynamics in the Red River mouth and adjacent coastal zone. J. Asian Earth Sci. 29 (4), 508–522. doi:10.1016/j.jseaes.2006.03.012
Van Niekerk, L., Adams, J. B., James, N. C., Lamberth, S. J., MacKay, C. F., Turpie, J. K., et al. (2020). An Estuary Ecosystem Classification that encompasses biogeography and a high diversity of types in support of protection and management. Afr. J. Aquatic Sci. 45 (1-2), 199–216. doi:10.2989/16085914.2019.1685934
Vaughn, S. E. (2013). Between a promise and a trench: Citizenship, vulnerability, and climate change in Guyana. Doctoral dissertation. Columbia University.
Vundavilli, V. S. S. R. H. (2023). The interaction of buoyant river plumes with vegetation and consequences for sediment transport and deposition in coastal regions. Doctoral dissertation. Waikato: The University of Waikato.
Wang, J., Shi, B., Zhao, E., Yuan, Q., and Chen, X. (2022). The long-term spatial and temporal variations of sediment loads and their causes of the Yellow River Basin. CATENA 209, 105850. doi:10.1016/j.catena.2021.105850
Wang, Z. B., Van Maren, D. S., Ding, P. X., Yang, S. L., Van Prooijen, B. C., De Vet, P. L. M., et al. (2015). Human impacts on morphodynamic thresholds in estuarine systems. Cont. Shelf Res. 111, 174–183. doi:10.1016/j.csr.2015.08.009
Wang, Z. Y., and Liang, Z. Y. (2000). Dynamic characteristics of the yellow River mouth. Earth Surf. Process. Landforms J. Br. Geomorphol. Res. Group 25 (7), 765–782. doi:10.1002/1096-9837(200007)25:7<765::aid-esp98>3.0.co;2-k
Weisscher, S. A., Baar, A. W., van Belzen, J., Bouma, T. J., and Kleinhans, M. G. (2022). Transitional polders along estuaries: Driving land-level rise and reducing flood propagation. Nature-Based Solutions 2, 100022. doi:10.1016/j.nbsj.2022.100022
Wilhelm, M. M., Cabral, A. C., Dauner, A. L. L., Garcia, M. R., Figueira, R. C., and Martins, C. C. (2023). Variability of sedimentary organic matter in subtropical estuarine systems due to anthropogenic and climatic events. Environ. Earth Sci. 82 (1), 22–16. doi:10.1007/s12665-022-10704-2
Wilkinson, S. N., Dougall, C., Kinsey-Henderson, A. E., Searle, R. D., Ellis, R. J., and Bartley, R. (2014). Development of a time-stepping sediment budget model for assessing land use impacts in large river basins. Sci. Total Environ. 468, 1210–1224. doi:10.1016/j.scitotenv.2013.07.049
Winterwerp, J. C., Albers, T., Anthony, E. J., Friess, D. A., Mancheño, A. G., Moseley, K., et al. (2020). Managing erosion of mangrove-mud coasts with permeable dams–lessons learned. Ecol. Eng. 158, 106078. doi:10.1016/j.ecoleng.2020.106078
Wong, P. P., Losada, I. J., Gattuso, J. P., Hinkel, J., Khattabi, A., McInnes, K. L., et al. (2014). “Coastal systems and low-lying areas,” in Climate change 2014: Impacts, adaptation, and vulnerability. Part A: Global and sectoral aspects. Contribution of working group II to the fifth assessment report of the intergovernmental panel on climate change. Editors C. B. Field, V. R. Barros, D. J. Dokken, K. J. Mach, M. D. Mastrandrea, T. E. Biliret al. (Cambridge, UK and New York, NY, USA: Cambridge University Press), 61–409.
Woodroffe, C. D., Carvalho, R. C., Oliver, T. S., and Thom, B. G. (2022). Sediment dynamics at different timescales on an embayed coast in southeastern Australia. J. Coast. Conservation 26 (3), 21–19. doi:10.1007/s11852-022-00867-2
Worts, G. F. (1963). A brief appraisal of ground-water conditions of the coastal artesian basin of British Guiana. South Americ: United States Geological Survey. Avaliable At: https://pubs.usgs.gov/wsp/1663b/report.pdf.
Wright, L. D., and Coleman, J. M. (1974). Mississippi River mouth processes: Effluent dynamics and morphologic development. J. Geol. 82 (6), 751–778. doi:10.1086/628028
Xia, S., Li, X., Deng, J., Luo, D., Shu, L., Wang, S., et al. (2020). Sequence architecture and depositional evolution of the late Palaeogene in southwestern slope of Lufeng Depression, Pearl River Mouth Basin: Responses to tectonic processes and base-level changes. Geol. J. 55 (2), 1468–1492. doi:10.1002/gj.3504
Yang, X., and Lu, X. X. (2014). Estimate of cumulative sediment trapping by multiple reservoirs in large river basins: An example of the Yangtze River basin. Geomorphology 227, 49–59. doi:10.1016/j.geomorph.2014.01.014
Yao, Q., Cohen, M., Liu, K. B., Fan, D., Rodrigues, E., Maiti, K., et al. (2022). Mangrove expansion at poleward range limits in North and South America: Late-Holocene climate variability or anthropocene global warming? Catena 216, 106413. doi:10.1016/j.catena.2022.106413
Yunus, A. P., Masago, Y., Boulange, J., and Hijioka, Y. (2022). Natural and anthropogenic forces on suspended sediment dynamics in Asian estuaries. Sci. Total Environ. 836, 155569. doi:10.1016/j.scitotenv.2022.155569
Zhang, D., Xie, W., Shen, J., Guo, L., Chen, Y., and He, Q. (2022). Sediment dynamics in the mudbank of the Yangtze River Estuary under regime shift of source and sink. Int. J. Sediment Res. 37 (1), 97–109. doi:10.1016/j.ijsrc.2021.07.005
Zhen, S. U. N., Zhihong, Z., Di, Z. H. O. U., Xiong, P. A. N. G., Chunju, H. U. A. N. G., Changmin, C. H. E. N., et al. (2008). Dynamics analysis of the baiyun sag in the pearl River Mouth basin, north of the South China sea. Acta Geol. Sinica-English Ed. 82 (1), 73–83. doi:10.1111/j.1755-6724.2008.tb00326.x
Keywords: accretion, Google Earth Engine, Guyana, mud shoal morphodynamics, remote sensing of river mouth, spit development, synthetic aperture radar (SAR)
Citation: Oyedotun TDT and Nedd GA (2023) River mouth morphodynamics and deflection over the short term: effects on spit growth and mangrove dynamics. Front. Environ. Sci. 11:1181627. doi: 10.3389/fenvs.2023.1181627
Received: 07 March 2023; Accepted: 09 May 2023;
Published: 18 May 2023.
Edited by:
Antonio Pepe, National Research Council (CNR), ItalyReviewed by:
Xiankun Yang, Guangzhou University, ChinaMaged Marghany, Syiah Kuala University, Indonesia
Copyright © 2023 Oyedotun and Nedd. This is an open-access article distributed under the terms of the Creative Commons Attribution License (CC BY). The use, distribution or reproduction in other forums is permitted, provided the original author(s) and the copyright owner(s) are credited and that the original publication in this journal is cited, in accordance with accepted academic practice. No use, distribution or reproduction is permitted which does not comply with these terms.
*Correspondence: Temitope D. Timothy Oyedotun, temitope.oyedotun@uog.edu.gy