- 1Crawford School of Public Policy, Australian National University, Canberra, ACT, Australia
- 2School of Engineering, Australian National University, Canberra, ACT, Australia
Sustainable, low-emission electricity generation options are needed in the Greater Mekong Subregion, including for cross-border electricity trade. Large-scale investment in solar and wind power, together with off-river pumped hydro energy storage, is identified as a promising way forward. The GMS has many potential off-river pumped hydro sites. Actionable recommendations include greater use of bilateral power purchase agreements for cross-border solar and wind power supply, and potential development of a high-voltage direct current grid. Institutional prioritization and ongoing evaluation are required to ensure desired social, environmental, and economic outcomes from the transition.
1 Introduction
The Greater Mekong Subregion (GMS) comprises Cambodia, Lao People’s Democratic Republic (PDR), Myanmar, Thailand, Vietnam, and Yunnan and Guangxi in China and is home to the longest river in Southeast Asia. With a population of 345 million people in 2021, its real gross domestic product grew at an average annual rate of 6.3% over 1995–2016 (GMS Secretariat, 2022). Annual electricity generation increased at an average rate of about 8.3% per annum over this period, reaching 775 TWh (GMS Secretariat, 2022). With its growing population and as a result of processes including income growth, urbanization, industrialization, and electrification, GMS electricity demand is likely to expand substantially over coming years (Phoumin et al., 2021).
Cross-border electricity trade offers an important way for GMS countries to improve their ability to meet these growing electricity demand pressures. Being able to export and import across borders can be highly economically advantageous (Antweiler, 2016). Indeed, modeling for the GMS shows that power interconnectivity could reduce the present value of electricity supply costs by around one-fifth relative to the situation of having individual electricity systems that are operated independently (Yates, 2021). Regional interconnectivity can also facilitate more stable grid management due to geographical diversification of electricity generation (IRENA and ACE, 2022).
Among the GMS countries, Lao PDR is already a major exporter of electricity, mostly to Thailand given the geographical proximity between the two countries and Thailand’s large demand for electricity. Cross-border electricity trade in other GMS countries has been relatively limited, with the openness index for electricity (imports plus exports scaled by production) of the overall region remaining low (Table 1). Cross-border electricity trade in the GMS has to date been primarily underpinned by hydro and coal generation in the form of large-scale dams and the 1,878 MW Hong Sa coal power plant in Lao PDR (Tran and Suhardiman, 2020).
Large-scale hydro development in the Mekong has been reported to have contributed to inequities, exclusion, and coercive expressions of social injustice (Blake and Barney, 2021). It has also resulted in biodiversity loss, fishery and sediment losses, changes in water temperatures, droughts, and salt intrusions (Grafton et al., 2019; Campbell and Barlow, 2020). The food security of millions of people has been threatened (Sabo et al., 2017). Large-scale hydropower projects are thus often a costly option when all implications are considered (Ansar et al., 2014).
The answer, however, is not coal. Coal power generation contributes to global warming and local and regional air pollution, with serious health consequences. Building additional coal-fired power stations also creates a risk of future stranded assets given the difficulty of reaching net-zero emission goals if unabated thermal coal projects remain in operation (Do and Burke, 2023a). Of concern is that Lao PDR has a project pipeline that would potentially add a further 7,000 MW of coal power capacity, mainly for power exports (Global Energy Monitor, 2023). Some modeling studies have also anticipated highly coal-intensive development pathways in the GMS under business as usual (Phoumin et al., 2021).
Explorations into alternative approaches to powering cross-border electricity trade in the GMS and Southeast Asia have been relatively limited. Previous studies on cross-border trade in the region have focused mainly on institutional, technical, and economic barriers (for example, Asian Development Bank [ADB], 2022; del Barrio-Alvarez and Horii, 2017; Do and Burke, 2023b; IEA, 2019; Shi et al., 2019; Yang et al., 2022). While hydrogen and battery energy storage systems have been identified as potentially important energy storage options (ADB, 2022), off-river pumped hydro energy storage–for which the GMS has vast potential (Australian National University (2021)), is yet to receive prominent focus in the context of facilitating the expansion of cross-border electricity trade among GMS countries.
Recent modeling efforts increasingly conclude there is a large role for solar and wind power in the electricity mixes of GMS countries under sustainable development pathways. The study of Handayani et al. (2022), for example, concludes that solar plus wind will make majority contributions to power generation in countries including Thailand and Vietnam by 2050 under a net zero emission scenario. The authors did not incorporate growth in cross-border power trade into their modeling, however.
This policy brief has the objective of exploring the potential for a new approach to cross-border electricity trade in the GMS based on trade in solar and wind power. It makes the case that a focus on solar and wind projects together with off-river pumped hydro energy storage is a highly promising way forward. We also point to initial signs of movements in this direction. The brief also highlights the importance of ensuring that projects are designed to achieve the best social, environmental, and economic outcomes possible and are subject to ongoing evaluation.
2 Policy options and implications
As of 2021, the five GMS nation states had about 20 GW of combined installed solar PV capacity and about 6 GW of installed wind capacity. This equalled about 17% of their total power generation capacity, although solar and wind installations typically operate at lower capacity factors than most other generators. The majority of this solar and wind capacity was in Vietnam. China had over 630 GW of installed solar PV and wind power in 2021, equal to about 28% of total power generation capacity (Ember, 2023).
Relative to their potentials, however, solar and wind uptake in the GMS remains low. In the best locations, solar and wind are now competitive with new hydropower and coal power, even before considering the likely lower environmental and social costs. Specifically, the levelized costs of electricity (LCOEs) for solar photovoltaic (PV) and onshore wind power in the GMS were estimated to be about US$46–75/MW h and US$49–76/MWh respectively in 2021 (IRENA and ACE, 2022). These are projected to continue to fall as the technologies improve and the industry matures. The LCOE for hydropower is about US$36/MWh, but this is tending to increase over time for reasons including that the best sites are utilized first (IRENA, 2021). Coal power has an estimated LCOE of about US$60–90/MWh in the GMS (Lu et al., 2021a). It is a mature technology and is losing competitiveness. Globally, the majority of new power generation capacity is either solar or wind (IRENA, 2023).
GMS countries have ample solar and wind resources (Figures 1A, B). The potentials for solar PV and onshore wind power at onshore sites with an LCOE of less than US$150/MWh have been estimated to be about 25,500 GW and 1,100 GW respectively in the Mekong countries as of 2018 (Lee et al., 2020). This far exceeds the current installed generation capacity of all technology types in the GMS of about 140 GW as of 2017 (ASEAN Centre for Energy, 2020; Statista, 2018). This potential is augmented by sizable offshore wind potential in countries such as Vietnam (Do et al., 2022). The GMS would benefit from developing solar and wind power concurrently, as wind resources are complementary to solar. Solar and wind energy are also complementary on a seasonal basis given the winter monsoon and other seasonal variation.
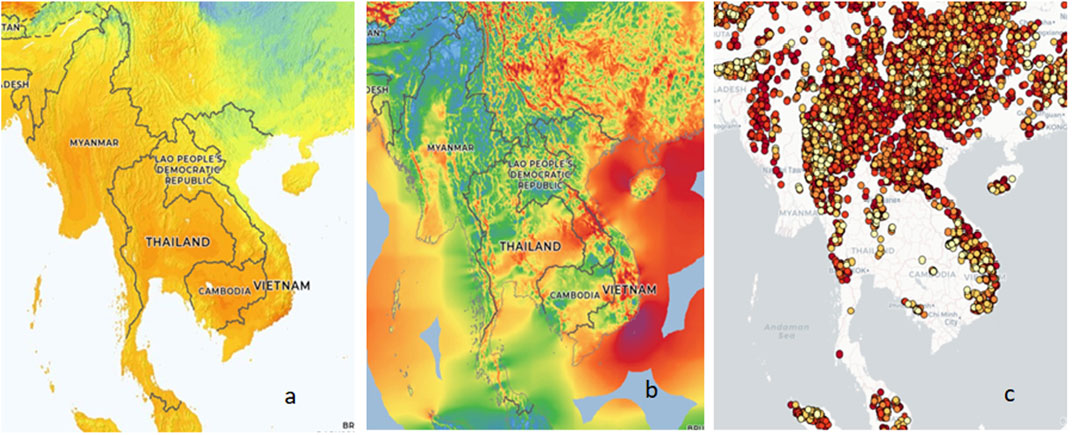
FIGURE 1. Solar, wind, and pumped hydro energy storage potential in the GMS: (A) Global horizontal irradiance as denoted by the blue-yellow-orange-red colour scheme (low to high solar radiation). (B) Mean wind speed at 150 m height with the excellent wind energy resources (>8 m/s) highlighted in red. (C) Potential sites for off-river pumped hydro, classified into A (dark red), B (red), C (orange), D (yellow), and E (light yellow) based on construction costs (low to high). Image source: Figure 1A was sourced from the Global Solar Atlas 2.5, a free, web-based application developed and operated by Solargis (2021) on behalf of the World Bank Group. Figure 1B uses wind resource data from Global Wind Atlas 3.1, by the Technical University of Denmark (2021). Figure 1C is from the Australian National University (2021) Global Pumped Hydro Atlas.
GMS countries have relatively high potential solar PV capacity factors-at 16%–18% for much of the region (Solargis, 2021). Seasonal variation in the solar resource is relatively low, with the maximum:minimum ratio for the monthly average solar energy resource typically below two. This means that the need for seasonal energy storage would be relatively modest, especially if domestic and regional electricity transmission interconnectivity is upgraded (Do et al., 2020; Do et al., 2021). Transmission connections would help to diversify supply and allow access to the lowest-cost generation sites in the region.
Off-river pumped hydro would be an ideal solution for the energy storage needs of both power importers and exporters. Pumped hydro is known to be the most economical option for large-scale energy storage on timescales ranging from hours to a few days (Schmidt et al., 2019). Unlike conventional on-river hydro, off-river pumped hydro can have relatively low environmental impacts, especially if located on brownfield sites such as former mines (Gilfillan and Pittock, 2022). The Lower Mekong countries have about 27,300 potential off-river pumped hydro sites with a combined storage capacity of over 896,000 GWh (Figure 1C) (Stocks et al., 2021). This is ample to support 100% renewable energy systems in the region.
Off-river pumped hydro has substantial potential to provide the dispatchability services that are currently often provided by hydropower, and without disrupting river systems (Waldman et al., 2019). It could also supply ancillary services such as frequency control. To facilitate cross-border trade flows, the pumped hydro sites could be either on the exporting or importing side of the border, depending on the context. Other storage solutions and smart grid management technologies will also become increasingly important as the share of variable energy sources rises (Schmitt et al., 2019). The availability of high quality predictive data on solar and wind resource availability is also important for system management and cost mitigation (Djaafari et al., 2022).
Adoption of solar and wind power plus off-river pumped hydro energy storage in the GMS could bring substantial benefits. The approach would reduce the risks of insufficient water availability for dam operations in the dry season (Chowdhury et al., 2021). At the regional scale, modelling suggests that a solar- and wind-dominated 100% renewable electricity system could be adopted in GMS countries at a highly competitive LCOE of about US$55–110/MWh in 2020 dollars (Lu et al., 2021a; Yates, 2021).
By avoiding the need for new large-scale hydro and thermal power projects, solar and wind power plus off-river pumped hydro energy storage could mitigate social and environmental impacts for local communities, especially if installations are well sited and community consultation and participation are strong (Do et al., 2021). Floating or fixed water-mounted solar PV projects are one way to reduce land impacts and can also potentially boost the energy output of the panels via a cooling effect. These installations can also help to reduce evaporation, thus providing potential benefits for agricultural and other users of water (Alhejji et al., 2021). Ecological impacts on waterbodies need to be minimized, however (Yang et al., 2019). Lao PDR is currently building its first utility-scale solar project, with a floating PV project on an existing dam also planned (IEEFA, 2022).
3 Actionable recommendations
Efforts to overcome institutional and technical barriers to greater cross-border electricity trade in the GMS are multifaceted (ADB, 2022). Here we outline several key strategies.
3.1 Mechanisms to promote solar, wind, and energy storage power adoption
Government policy instruments such as feed-in tariffs and reverse auctions will be crucial in boosting overall adoption of solar and wind power in GMS countries (Vakulchuk et al., 2023). These mechanisms should ideally be open to cross-border suppliers. The renewable portfolio standard approach, as used in, for example, Australia and California, is also a highly promising approach (Burke and Do, 2021). Auctions and certificate mechanisms can also be used to target the provision of short- and long-term energy storage services, or firmed supply. Improvements in grid capacity and management are also important, as it is highly desirable to avoid the type of solar and wind output curtailment experienced in Vietnam in its early stage of adoption (Do et al., 2021).
While investing in new solar and wind projects, transitioning away from coal projects is also of foremost importance so as not to be adding to the unsustainable trend in GMS countries. There is currently substantial international support available for the phase out of coal power and bringing in of renewables. For example, in 2022 Vietnam entered a US$15.5 billion Just Energy Transition Partnership with international partners that focuses on substitution away from thermal coal (Do and Burke, 2023a).
3.2 Focusing on bilateral cross-border electricity trade
Deep integration of wholesale electricity markets is unlikely in the GMS in the foreseeable future. Instead, it is likely that bilateral or trilateral cross-border power purchase agreements (PPAs) will continue to be the key facilitating mechanism for cross-border electricity trade (Do and Burke, 2023b). Such agreements can be de-risked to some extent via sovereign guarantees and other approaches (IRENA, 2020).
Positive signs have recently been seen in this direction. In early 2023, Vietnam Oil and Gas Group and Singapore’s Sembcorp Industries signed an agreement enabling Vietnam to export about 2.3 GW of offshore wind power to Singapore (Reuters, 2023). The ADB also signed a US$692 million project financing package for the building of a 600 MW wind power project in Lao PDR for exports to Vietnam (ADB, 2023). Cambodia also signed a memorandum of understanding for the export of clear power to Singapore (Khmer Times, 2023).
3.3 High-voltage direct current super grid
One promising approach would be for a high-voltage direct current (HVDC) super grid to be built to interconnect the member countries. Modern HVDC technology enables long-distance, bulk electricity transmission with relatively low energy loss (about 3% per 1,000 km) (Lu et al., 2021b). An HVDC super grid has been modeled in various exercises. For example, the study of Yates (2021) found that it could help to enable a 97% renewables share in the GMS electricity mix at a competitive system LCOE of about $US66/MWh. An HVDC super grid could also be extended to connect with other provinces in China, other Southeast Asian nations, and countries such as Bangladesh, India, and Australia (Do and Burke, 2023b). Such an interconnected system could be decoupled from the alternate current grids to reduce the chance of a failure pass-through (Lu et al., 2021b). The grid would need to be built in a staged manner, ideally with a view to providing broad regional interconnections in the long run.
3.4 Institutional and financing arrangements
Institutionally, prioritization of a solar- and wind-powered vision for GMS power trade by the GMS Regional Power Trade Coordination Committee would be a useful step. This committee comprises representatives from the six national energy ministries (ADB, 2022). The Mekong River Commission and the Association of Southeast Asian Nations (ASEAN) also have key regional roles (Dombrowsky and Hensengerth, 2018). There is also a potential role for new institutions to monitor and evaluate progress in the energy transition in the GMS, drawing lessons for subsequent stages.
Sizable investment in power generation and transmission capacities will be needed, although this would also be the case under either an emission-intensive or a hydro-intensive development pathway. We estimate that the capital costs for a well-connected HVDC super grid in the GMS would likely run into the hundreds of billions of dollars (Lu et al., 2021a; Yates, 2021). Substantial investment will also be needed for the overall move to a net-zero economy, including for the electrification of sectors such as road transport (DEA and EREA, 2022). Given technology learning and maturity effects, the extent to which local solar and wind costs are expected to decline over coming years is itself a function of adoption levels in the region.
Multilateral development banks such as the Asian Development Bank, the Asian Infrastructure Investment Bank, and the World Bank have key financing roles, and bilateral partners are also important (Feng et al., 2020). The ADB (2021), for example, has announced it will provide US$100 billion in climate financing across Asia and the Pacific over 2019–2030 and has specifically ruled out support for new coal mining or power projects. GMS countries could also mobilize domestic resources for expanding transmission grids via mechanisms such as carbon pricing (Do and Burke, 2021).
3.5 Strengthening political and community support
Political support will be crucial for the prospects for sustainable cross-border electricity trade in the GMS (Puka and Szulecki, 2014), and indeed was a key requisite for the initial establishment of cross-border electricity trade between Lao PDR and Thailand (del Barrio-Alvarez and Horii, 2017). Ensuring strong community participation in decision making and benefit sharing is also crucially important (Wyrwoll et al., 2018). Stringent efforts to minimize the environmental and social footprints of solar and wind projects and related transmission and energy storage infrastructure will be essential for environmental sustainability and maintaining public support (Opperman et al., 2023). It is vital that infrastructure is sited with an eye to minimizing adverse impacts.
4 Conclusion
Solar and wind power combined with off-river pumped hydro storage have emerged as a promising alternative to new large-scale hydro or thermal power in the GMS in an era in which low-emission, sustainable options are highly needed. Resource variation between countries creates important cross-border trade opportunities, for example, the export of offshore wind power from Vietnam, onshore wind power from Lao PDR, or solar power from Cambodia, among other potentials. However there is much to do to realize this more sustainable model of cross-border power trade for the region. Substantial capital flows will be needed.
Short-term actions to ramp up investment in solar and wind power and needed transmission infrastructure–both domestic and cross-border–are important. Environmental and social safeguards will also be needed for all solar, wind, storage, and transmission projects. Regional cooperation and trust will be crucial. A deep form of wholesale power market integration is unlikely in the near term, with continuing reliance on bilateral and trilateral agreements for cross-border electricity supply and offtake likely to remain as the mainstay model.
There is substantial scope for future research on specific engineering designs, project costings, and models for community participation in clean energy projects in the context of the GMS. Ongoing evaluation along social, environmental, and economic dimensions will also be crucial. Lessons learned along the way will be informative for ensuring the long-run delivery of sustainable development outcomes in the GMS in the new era of solar and wind power.
Author contributions
TD: Conceptualization, investigation, writing–original draft, writing–review and editing. PB: Investigation, writing–original draft, writing–review and editing. BL: Investigation, visualization, writing–review and editing. All authors contributed to the article and approved the submitted version.
Funding
This policy brief receives support from the Australian National University Zero-Carbon Energy for the Asia-Pacific Grand Challenge.
Acknowledgments
We are grateful to the reviewers for comment and the Australian National University’s Zero-Carbon Energy for the Asia-Pacific initiative.
Conflict of interest
The authors declare that the research was conducted in the absence of any commercial or financial relationships that could be construed as a potential conflict of interest.
Publisher’s note
All claims expressed in this article are solely those of the authors and do not necessarily represent those of their affiliated organizations, or those of the publisher, the editors and the reviewers. Any product that may be evaluated in this article, or claim that may be made by its manufacturer, is not guaranteed or endorsed by the publisher.
Abbreviations
ACE, ASEAN Centre for Energy; ADB, Asian Development Bank; DEA, Danish Energy Agency; EIA, Energy Information Administration; EREA, Electricity and Renewable Energy Agency; ESMAP, Energy Sector Management Assistance Program; GMS, Greater Mekong Subregion; GW, gigawatts; GWh, gigawatt hours; HVDC, high-voltage direct current; IEA, International Energy Agency; IEEFA, Institute for Energy Economics and Financial Analysis; IRENA, International Renewable Energy Agency; LCOE, levelized cost of electricity; MW, megawatt; MWh, megawatt hour; PDR, People’s Democratic Republic; PPA, power purchase agreement; PV, photovoltaic; TWh, terawatt hours; US, United States.
References
ADB (2023). ADB signs loan for first cross-border wind power project in Asia, first plant in Lao PDR and largest in Southeast Asia. https://www.adb.org/news/adb-signs-loan-first-cross-border-wind-power-project-asia-first-plant-lao-pdr-and-largest.
ADB (2021). “Energy policy: Supporting low-carbon transition in Asia and the Pacific,” in Policy paper (Manila, Philippines: Asian Development Bank).
ADB (2022). Facilitating power trade in the Greater Mekong Subregion: Establishing and implementing a regional grid code. Manila, Philippines: Asian Development Bank.
Alhejji, A., Kuriqi, A., Jurasz, J., and Abo-Elyousr, F. K. (2021). Energy harvesting and water saving in arid regions via solar PV accommodation in irrigation canals. Energies 14 (9), 2620. doi:10.3390/en14092620
Ansar, A., Flyvbjerg, B., Budzier, A., and Lunn, D. (2014). Should we build more large dams? The actual costs of hydropower megaproject development. Energy Policy 69, 43–56. doi:10.1016/j.enpol.2013.10.069
Antweiler, W. (2016). Cross-border trade in electricity. J. Int. Econ. 101, 42–51. doi:10.1016/j.jinteco.2016.03.007
Australian National University (2021). Global pumped hydro atlas. https://www.nationalmap.gov.au/#share=s-py9ofDCNEwqsrfGGkptS5dJ9wSq.
Blake, D. J. H., and Barney, K. (2021). Impounded rivers, compounded injustice: Contesting the social impacts of hydraulic development in Laos. Int. J. Water Resour. 38 (1). doi:10.1080/07900627.2021.1920373
Burke, P. J., and Do, T. N. (2021). Greening Asia’s economic development. Asia Econ. Policy Rev. 16 (1), 22–39. doi:10.1111/aepr.12316
Campbell, I., and Barlow, C. (2020). Hydropower development and the loss of fisheries in the Mekong River basin. Front. Environ. Sci. 8. doi:10.3389/fenvs.2020.566509
Chowdhury, A. F. M. K., Dang, T. D., Nguyen, H. T. T., Koh, R., and Galelli, S. (2021). The Greater Mekong's climate-water-energy nexus: How ENSO-triggered regional droughts affect power supply and CO2 emissions. Earth’s Future 9, e2020EF001814. doi:10.1029/2020EF001814
DEA and EREA, (2022). Vietnam Energy Outlook Report 2021. https://ens.dk/en/our-responsibilities/global-cooperation/country-cooperation/vietnam.
del Barrio-Alvarez, D., and Horii, H. (2017). Energy security and regional power sector cooperation in the Greater Mekong Sub-Region: Past developments and near-term challenges. Asian J. Public Aff. 9 (2), e2. doi:10.18003/ajpa.20174
Djaafari, A., Ibrahim, A., Bailek, N., Bouchouicha, K., Hassan, M. A., Kuriqi, A., et al. (2022). Hourly predictions of direct normal irradiation using an innovative hybrid LSTM model for concentrating solar power projects in hyper-arid regions. Energy Rep. 8, 15548–15562. doi:10.1016/j.egyr.2022.10.402
Do, T. N., Burke, P. J., Baldwin, K. G. H., and Nguyen, C. T. (2020). Underlying drivers and barriers for solar photovoltaics diffusion: The case of Vietnam. Energy Policy 144, 111561. doi:10.1016/j.enpol.2020.111561
Do, T. N., and Burke, P. J. (2021). Carbon pricing in Vietnam: Options for adoption. Energy Clim. Change 2, 100058. doi:10.1016/j.egycc.2021.100058
Do, T. N., Burke, P. J., Hughes, L., and Ta, D. T. (2022). Policy options for offshore wind power in Vietnam. Mar. Policy 141, 105080. doi:10.1016/j.marpol.2022.105080
Do, T. N., and Burke, P. J. (2023b). Is ASEAN ready to move to multilateral cross-border electricity trade? Asia Pac. Viewp. 64 (1), 110–125. doi:10.1111/apv.12343
Do, T. N., Burke, P. J., Nguyen, N. H., Overland, I., Suryadi, B., Swandaru, A., et al. (2021). Vietnam’s solar and wind power success: Policy implications for the other ASEAN countries. Energy Sustain. Dev. 65, 1–11. doi:10.1016/j.esd.2021.09.002
Do, T. N., and Burke, P. J. (2023a). Phasing out coal power in a developing country context: Insights from Vietnam. Energy Policy 176, 113512. doi:10.1016/j.enpol.2023.113512
Dombrowsky, I., and Hensengerth, O. (2018). Governing the water-energy-food nexus related to hydropower on shared rivers the role of regional organizations. Front. Environ. Sci. 6. doi:10.3389/fenvs.2018.00153
Ember, (2023). Data. https://ember-climate.org/data.
Feng, T.-t., Gong, X.-l., Guo, Y.-h., Yang, Y.-s., Pan, B.-b., Li, S.-p., et al. (2020). Electricity cooperation strategy between China and ASEAN countries under ‘The Belt and Road. Energy Strategy Rev. 30, 100512. doi:10.1016/j.esr.2020.100512
Gilfillan, D., and Pittock, J. (2022). Pumped storage hydropower for sustainable and low-carbon electricity grids in Pacific Rim economies. Energies 15 (9), 3139. doi:10.3390/en15093139
Global Energy Monitor (2023). Global coal power plant tracker. https://globalenergymonitor.org/projects/global-coal-plant-tracker.
Grafton, R. Q., Garrik, D., Manero, A., and Do, T. N. (2019). The Water Governance Reform Framework: Overview and Applications to Australia, Mexico, Tanzania, U.S.A and Vietnam. Water 11 (1), 137. doi:10.3390/w11010137
Handayani, K., Anugrah, P., Goembira, F., Overland, I., Suryadi, B., and Swandaru, A. (2022). Moving beyond the NDCs: ASEAN pathways to a net-zero emissions power sector in 2050. Appl. Energy 311, 118580. doi:10.1016/j.apenergy.2022.118580
IEA (2019). Establishing multilateral power trade in ASEAN. Paris, France: International Energy Agency.
IEEFA (2022). Construction starts on first large-scale solar farm in Laos. Abu Dhabi, United Arab Emirates: Institute for Energy Economics and Financial Analysis.
IRENA and ACE (2022). Renewable Energy Outlook for ASEAN: Towards a regional energy transition. Abu Dhabi, United Arab Emirates: International Renewable Energy Agency.
IRENA (2023). Renewable capacity statistics 2023. Abu Dhabi, United Arab Emirates: International Renewable Energy Agency.
IRENA (2020). Renewable energy finance: Sovereign guarantees. Abu Dhabi, United Arab Emirates: International Renewable Energy Agency.
IRENA (2021). Renewable power generation costs in 2020. Abu Dhabi, United Arab Emirates: International Renewable Energy Agency.
Khmer Times, (2023). Cambodia to export renewable electricity to Singapore. https://www.khmertimeskh.com/501256436/cambodia-to-export-renewable-electricity-to-singapore.
Lee, N., Flores-Espino, F., Oliveira, R., Roberts, B., Bowen, T., and Katz, J. (2020). Exploring renewable energy opportunities in select Southeast Asian countries: A geospatial Analysis of the levelized cost of energy of utility-scale wind and solar photovoltaics. Washington, DC, USA: National Renewable Energy Laboratory.
Lu, B., Blakers, A., Stocks, M., Cheng, C., and Nadolny, A. (2021b). A zero-carbon, reliable and affordable energy future in Australia. Energy 220, 119678. doi:10.1016/j.energy.2020.119678
Lu, B., Blakers, A., Stocks, M., and Do, T. N. (2021a). Low-cost, low-emission 100% renewable electricity in Southeast Asia supported by pumped hydro storage. Energy 236, 121387. doi:10.1016/j.energy.2021.121387
Opperman, J. J., Carvallo, J. P., Kelman, R., Schmitt, R. J. P., Almeida, R., Chapin, E., et al. (2023). Balancing renewable energy and river resources by moving from individual assessments of hydropower projects to energy system planning. Front. Environ. Sci. 10, 1036653. doi:10.3389/fenvs.2022.1036653
Phoumin, H., Meas, S., and An, H. P. (2021). Sustainable energy-related infrastructure development in the Mekong Subregion: Key drivers and policy implications. Sustainability 13 (10), 5720. doi:10.3390/su13105720
Puka, L., and Szulecki, K. (2014). The politics and economics of cross-border electricity infrastructure: A framework for analysis. Energy Res. Soc. Sci. 4, 124–134. doi:10.1016/j.erss.2014.10.003
Reuters, (2023). Vietnam in talks with Sembcorp to build power line linking to Singapore. https://www.reuters.com/business/energy/vietnam-talks-with-sembcorp-build-power-line-linking-singapore-2023-02-10.
Sabo, J. L., Ruhi, A., Holtgrieve, G. W., Elliott, V., Arias, M. E., Ngor, P. B., et al. (2017). Designing river flows to improve food security futures in the Lower Mekong Basin. Science 358 (6368), eaao1053. doi:10.1126/science.aao1053
Schmidt, O., Melchior, S., Hawkes, A., and Staffell, I. (2019). Projecting the Future Levelized Cost of Electricity Storage Technologies. Joule 3, 81–100. doi:10.1016/j.joule.2018.12.008
Schmitt, R. J. P., Kittner, N., Kondolf, G. M., and Kammen, D. M. (2019). Deploy diverse renewables to save tropical rivers. Nature 569, 330–332. doi:10.1038/d41586-019-01498-8
GMS Secretariat, (2022). The Greater Mekong Subregion Statistical Database. http://www.greatermekong.org/stats/index-static.php.
Shi, X., Yao, L., and Jiang, H. (2019). Regional power connectivity in Southeast Asia: the role of regional cooperation. Glob. Energy Interconnect. 2, 444–456. doi:10.1016/j.gloei.2019.11.020
Solargis, (2021). Global Solar Atlas 2.5. https://globalsolaratlas.info.
Statista, (2018). China electricity by region. https://www.statista.com/statistics/302317/china-power-generation-installed-capacity-by-region.
Stocks, M., Stocks, R., Lu, B., Cheng, C., and Blakers, A. (2021). Global Atlas of Closed-Loop Pumped Hydro Energy Storage. Joule 5 (1), 270–284. doi:10.1016/j.joule.2020.11.015
Technical University of Denmark (2021). Global Wind Atlas 3.1. https://globalwindatlas.info.
Tran, T. A., and Suhardiman, D. (2020). Laos’ hydropower development and cross-border power trade in the Lower Mekong Basin: A discourse analysis. Asia Pac. Viewp. 61 (2), 219–235. doi:10.1111/apv.12269
U.S. EIA, (2022). International Energy Statistics. https://www.eia.gov/international/data/world.
Vakulchuk, R., Overland, I., and Suryadi, B. (2023). ASEAN’s energy transition: How to attract more investment in renewable energy. Energy, Ecol. Environ. 8 (1), 1–16. doi:10.1007/s40974-022-00261-6
Waldman, J., Sharma, S., Afshari, S., and Fekete, B. (2019). Solar-power replacement as a solution for hydropower foregone in US dam removals. Nat. Sustain. 2 (9), 872–878. doi:10.1038/s41893-019-0362-7
Wyrwoll, P, R., Grafton, R. Q., Daniell, K. A., Chu, L., Le, T. H. L., Dang, K. K., et al. (2018). Decision-making for systemic water risks: Insights from a participatory risk assessment process in Vietnam. Earth’s Future 6, 543–564. doi:10.1002/2017EF000777
Yang, M., Sharma, D., Shi, X., Mamaril, K., Jiang, H., and Candlin, A. (2022). Power connectivity in the Greater Mekong Subregion (GMS) – The need for a wider discourse. Energy Policy 165, 112994. doi:10.1016/j.enpol.2022.112994
Yang, P., Chua, L. H. C., Irvine, K. N., Nguyen, M. T., and Low, E. W. (2019). Impacts of a floating photovoltaic system on temperature and water quality in a shallow tropical reservoir. Limnology 23, 441–454. doi:10.1007/s10201-022-00698-y
Keywords: electricity trade, Mekong, pumped hydro energy storage, solar, wind
Citation: Do TN, Burke PJ and Lu B (2023) Harnessing solar and wind for sustainable cross-border electricity trade in the Greater Mekong Subregion. Front. Environ. Sci. 11:1188335. doi: 10.3389/fenvs.2023.1188335
Received: 17 March 2023; Accepted: 30 May 2023;
Published: 08 June 2023.
Edited by:
Xander Wang, University of Prince Edward Island, CanadaReviewed by:
Nathan Moore, Michigan State University, United StatesAlban Kuriqi, University of Lisbon, Portugal
Copyright © 2023 Do, Burke and Lu. This is an open-access article distributed under the terms of the Creative Commons Attribution License (CC BY). The use, distribution or reproduction in other forums is permitted, provided the original author(s) and the copyright owner(s) are credited and that the original publication in this journal is cited, in accordance with accepted academic practice. No use, distribution or reproduction is permitted which does not comply with these terms.
*Correspondence: Thang Nam Do, dGhhbmcuZG9AYW51LmVkdS5hdQ==