- 1Alfred-Wegener-Institut Helmholtz-Zentrum für Polar-und Meeresforschung, Bremerhaven, Germany
- 2Department of Life and Environmental Science, Università di Cagliari, Cagliari, Italy
Plastic production and plastic waste have increased to such an extent that it has become globally ubiquitous. Recent research has highlighted that it has also invaded remote Polar Regions including the Arctic, where it is expected to accumulate over time due to transport from distant sources, rising local anthropogenic activities and increasing fragmentation of existing ocean plastics to microplastics (plastic items <5 mm). While a growing body of research has documented microplastics in the atmosphere, cryosphere, sea surface, water column, sediments and biota, contamination levels on Arctic beaches are poorly known. To fill this knowledge gap, we engaged citizen scientists participating in tourist cruises to sample beach sediments during shore visits on Svalbard, Norway. Following drying, sieving, and visual inspection of samples under a binocular microscope, putative plastic particles ≥1 mm were analysed by attenuated total reflectance Fourier transform infrared (ATR-FTIR) spectroscopy. Plastic particles ≥1 mm were found in two out of 53 samples from 23 beaches (mean: 196.3 particles kg−1 and 147.4 particles L−1). These pollution levels could be due to our focus on plastic particles ≥1 mm as well as the relatively small sample sizes used during this initial phase of the project. In addition, the coarse substrate on most beaches might retain fewer plastic particles. The two samples with plastic particles ≥1 mm contained six polyester-epoxide particles and 4920 polypropylene fibres. The latter likely originated from a fishing net and points to possibly accelerated plastic fragmentation processes on Arctic beaches. Since fisheries-related debris is an important source of plastic on Svalbard, a build-up of microplastic quantities can be expected to burden Arctic ecosystems in addition to climate change unless efficient upstream action is taken to combat plastic pollution.
1 Introduction
Plastic pollution has become a hallmark of the Anthropocene (Waters et al., 2016; Zalasiewicz et al., 2016) because plastic production and pollution have grown exponentially since World War II (Geyer et al., 2017; Geyer, 2020) and are predicted to continue on this exponential path (Borrelle et al., 2020; Lau et al., 2020). Therefore, it is not surprising that plastic pollution has become ubiquitous (Tekman et al., 2022b) and has been documented in the marine (Bergmann et al., 2017a), freshwater (Koutnik et al., 2021), terrestrial (Schwarz et al., 2023) and atmospheric realm (Allen et al., 2022) including remote Polar Regions such as the Arctic (Bergmann et al., 2022a). This irreversible plastic pollution has begun to impact populations, species and ecosystems to varying degrees, running the entire spectrum from interactions causing no detectable harm to severe harm (Bergmann et al., 2015; Puskic et al., 2020; Tekman et al., 2022b), and could lead to irreversible harm unless severe reductions in production and pollution are achieved (Villarrubia-Gomez et al., 2018; MacLeod et al., 2021).
Plastic pollution has also increased in the Arctic (Parga Martínez et al., 2020) with negative impacts on Arctic biota already documented (Baak et al., 2020; Collard and Ask, 2021; Bergmann et al., 2022a; Walther and Bergmann, 2022). Although in recent years more and more studies have documented levels of both macroplastic and microplastic pollution in different regions of the Arctic, many blind spots persist (Grøsvik et al., 2022; Primpke et al., 2022; Walther and Bergmann, 2022; Provencher et al., 2023).
The Svalbard Archipelago is the northernmost landmass of Europe between 74° and 81° N latitude, and comprises three large islands and a number of smaller ones. Because of its natural beauty and wilderness, it has attracted increasing numbers of tourists (Parga Martínez et al., 2020). The rising plastic pollution of Svalbard’s coastline could be due to local people or tourists, but the vast majority is likely transported to Svalbard’s coastline by the surrounding ocean currents, with the debris originating from local and distant fishing activities as well as distant land-based sources (Bergmann et al., 2022a; Meyer et al., 2023 several studies have already investigated the amount and distribution of macroplastic and other large man-made debris along Svalbard’s coastline (Nashoug, 2017; Jaskólski et al., 2018; Węsławski and Kotwicki, 2018; Falk-Andersson and Strietman, 2019; Falk-Andersson et al., 2021; Liutkus et al., 2022), including four studies where at least some of the data were collected by citizen scientists (Bergmann et al., 2017b; Falk-Andersson et al., 2019; Strand et al., 2021; Meyer et al., 2023). While evidence of microplastics along the coasts and fjords of Svalbard has recently emerged (Collard et al., 2021; Choudhary et al., 2022; Lin et al., 2022), baseline information on microplastic pollution levels of its beaches or Arctic beaches in general (Sundet et al., 2016; Sundet et al., 2017; Granberg et al., 2019; Blinovskaya et al., 2020) is still sparse.
Because of the success of recording macrodebris with the help of citizen scientists on Svalbard’s beaches, at the sea surface (Bergmann et al., 2017b; Tekman et al., 2022a; Meyer et al., 2023) and of sampling snow on Svalbard (Bergmann et al., 2019), we used the same setup to collect sediment samples for the analysis of plastic particles ≥1 mm. The goal of this study was to obtain microplastic levels of Svalbard’s beaches by engaging cruise tourists visiting the Svalbard Archipelago in a citizen science sampling project to generate baseline data. In addition, we aimed to compare microplastic pollution levels with those of macroplastic pollution recorded during citizen-scientist campaigns conducted at the time of microplastic sampling (Meyer et al., 2023).
2 Materials and methods
2.1 Sampling
This study was conducted in collaboration with tourist cruise operators during four campaigns to the Svalbard Archipelago, one each in 2016, 2017, 2021, and 2022 (Table 1; Figure 1). Participants were supplied with simple instructions for the collection of sediment samples on beaches, which were visited during shore excursions of tourist cruises around Svalbard. Most of these collections happened in conjunction with a survey of macroplastic pollution during the campaigns of 2016, 2017, and 2021 (Meyer et al., 2023). Hand-held GPS devices (Garmin eTrex 30×) were used to determine the positions of each sampling site. Two different protocols were used to collect sediment samples.
1. For locations 1–21 listed in Table 1 (see also Figure 2), one aluminium container was filled by a metal spoon with sediment from the uppermost 2 cm of a surface area of approximately 1,000 cm2 and sealed with an aluminium lid. The locations were chosen by convenience (middle of beach, no large stones or pieces of wood present, not too wet).
2. For locations 22 and 23, sampling points were determined by laying out a sampling grid over the sampling location. At location 22, the length of the grid was 100 m long and followed the beach’s water edge. The survey width was determined by the width of the beach (Figure 3). At each of the 20 sampling points, a stainless-steel sampling frame of 24 × 24 cm (sampling area of 0.0576 m2) was pushed into the sediment. Using a metal shovel, one tinplate container (195 × 153 × 76 mm, Tinware Direct, https://tinyurl.com/2p87uybd) was filled with sediment from within the sampling frame, which meant that several centimetres of sediment were evenly removed. At location 23, the grid was modified because of the narrow beach width (5 m): Two collection lines (A and B) were established, each with six sampling points (Figure 4). Line A lay within 1 m of the water’s edge and parallel to it, with each of the six sampling points located 5 m apart. Line B ran in parallel, but a further 2 m inland.
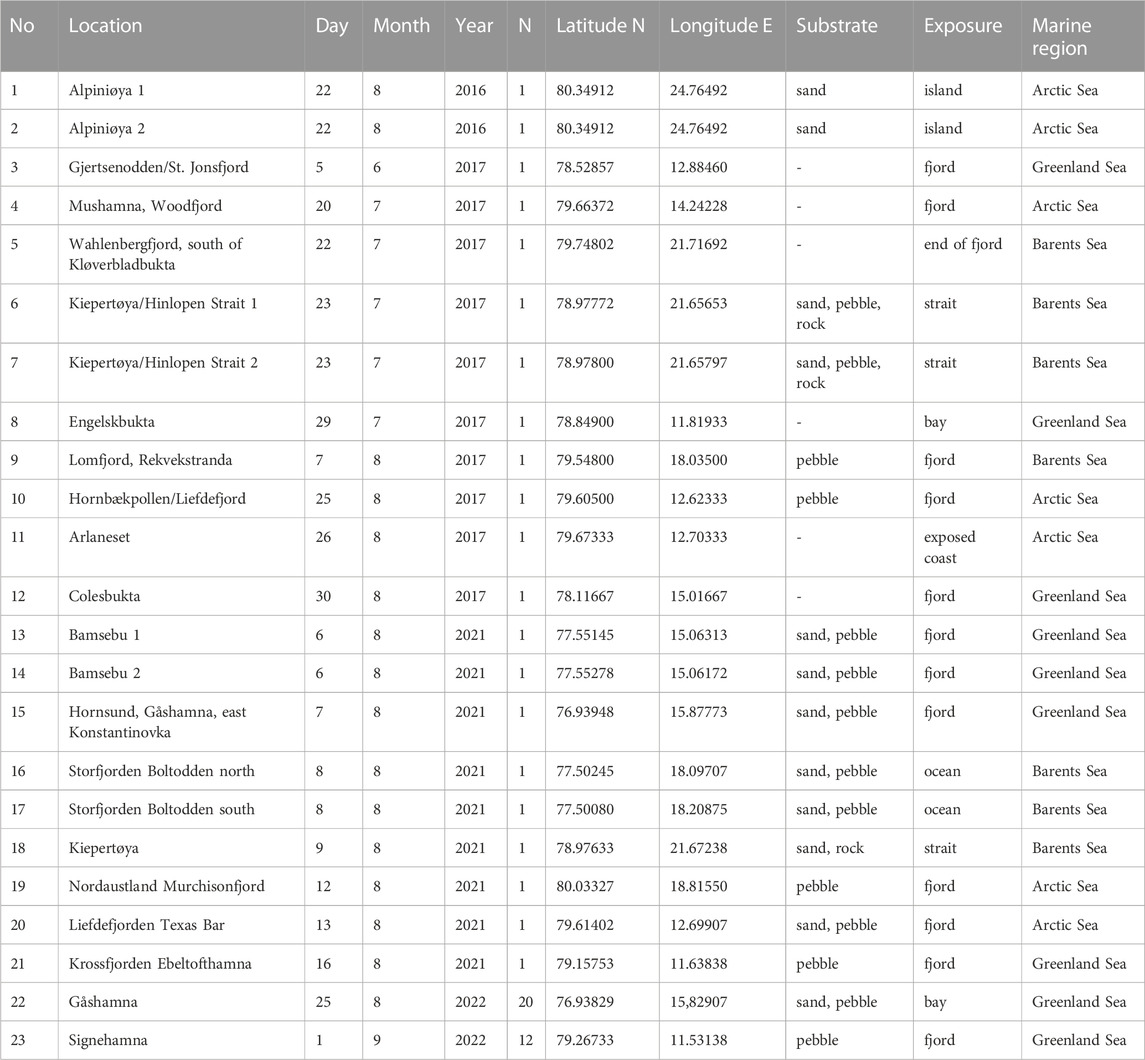
TABLE 1. Characteristics of 23 locations in Svalbard, Norway, where citizens scientists took sediment samples (N = sample size), which were analysed for microplastics. For some locations, the data for substrate were not recorded. Latitude N and longitude E are given in degrees.
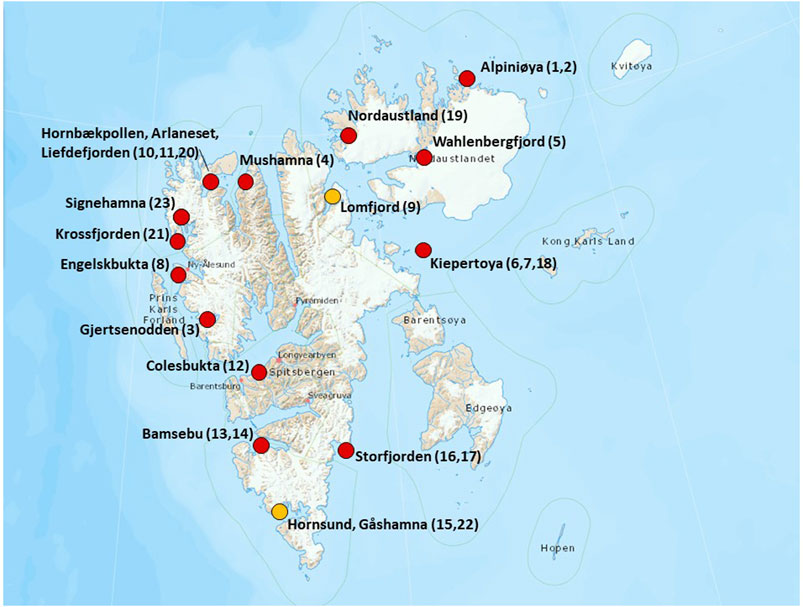
FIGURE 1. Map of all locations of microplastic surveys depicted by dots (yellow: plastic found; red: none found). Numbers inside brackets represent the location number (Table 1) (Map © Norwegian Polar Institute).
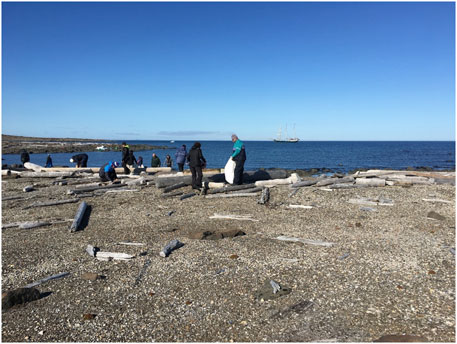
FIGURE 2. Photo of a typical Svalbard beach where citizen scientists assessed macroplastic pollution (Meyer et al., 2023) and took sediment samples for microplastic analyses with the cruise ship in the background (Kiepertøya/Hinlopen Strait, 23 July 2017, credit: Birgit Lutz with permission).
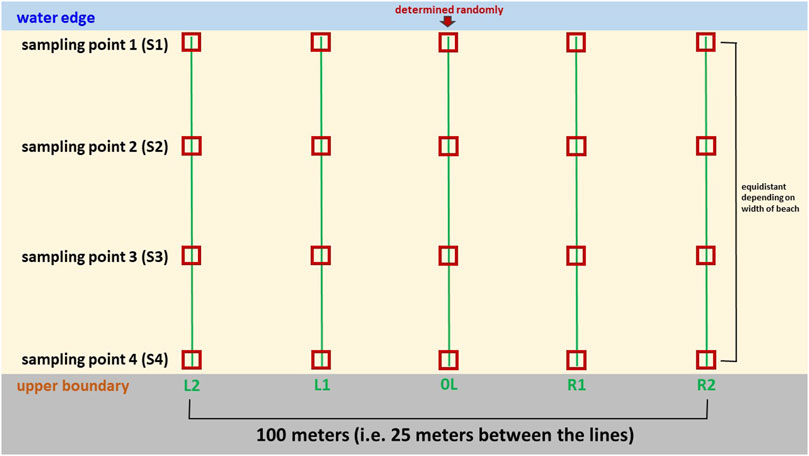
FIGURE 3. Sampling grid used to establish sampling points (dark red squares) for location 22 (Table 1). The location of the first collection line (green line) was determined randomly. The width of the beach was measured along this randomly placed zero line (0L), and four sampling points were placed along this line equidistantly. The other four collection lines were placed parallel to the zero line at 25 m distance along the water’s edge, with two lines to the left (L1 and L2) and two lines to the right (R1 and R2) of the zero line. The upper boundary of the beach was determined by a hard boundary (e.g., the beginning of vegetation, or rock surface, or icy cover).
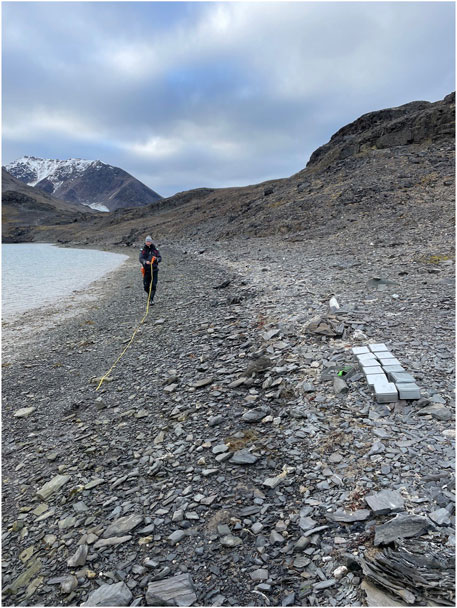
FIGURE 4. Photo of the narrow beach at Signehamna, sampled in 2022 with the 12 sediment samples on the right (credit: Birgit Lutz with permission).
The 53 sample containers (Table 2), protocols with metadata and photos were posted to the Alfred-Wegener-Institut Helmholtz-Zentrum für Polar-und Meeresforschung.
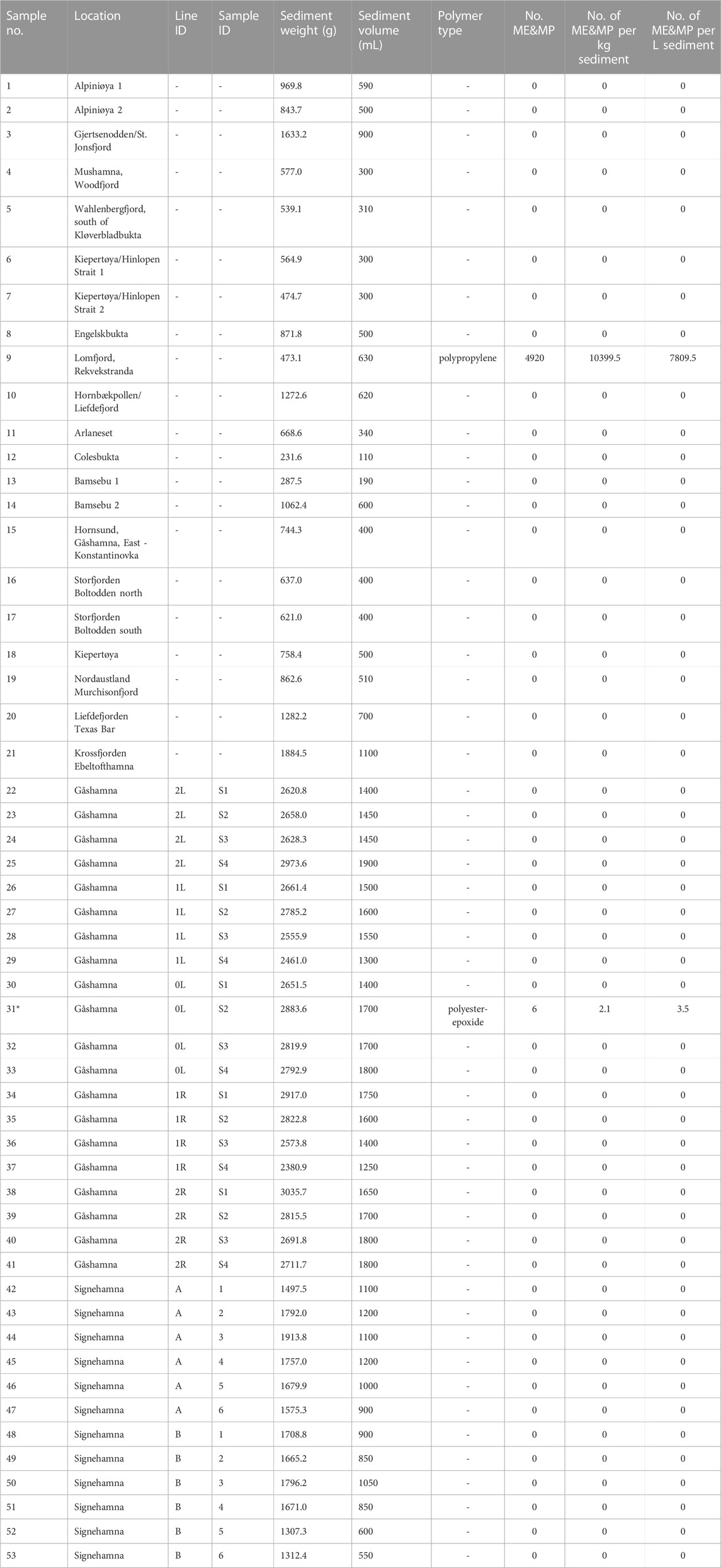
TABLE 2. Details of 53 sediment samples taken at 23 locations in Svalbard (Table 1) including plastic abundance. The sediment’s dry weight and volume were measured in the laboratory for every sample (thus the sample size is N = 53), and the polymers were determined with ATR-FTIR spectroscopy (see Methods). For samples 1–21, the surface area sampled was not determined. However, for the samples 22–53, the surface of the beach sampled was 0.0576 m2 for each sample (thus the sample size is N = 32). *Given that the surface area for sample 31 was 0.0576 m2 and the number of ME&MP particles was six, the number (No.) of ME&MP per m2 was 104.17 for sample 31.
2.2 Laboratory work
Each sediment sample was dried at 50°C in an oven for 1–3 days until the sediment was dried. 50°C is the upper temperature limit for drying recommended by Goli et al. (2022). Temperatures between 50°C and 60°C are commonly used (e.g., Kunz et al., 2016; Chen and Chen, 2020), although temperatures higher than 60°C should be avoided in order to not change the polymers’ chemical and morphological structure (Munno et al., 2018; Pfeiffer and Fischer, 2020; Goli et al., 2022; S. Primpke, in litt. 2023). The dry-weight was determined (Kompakt Waagen Kern EMB-Serie 6000–1, accurate to 0.1 g) as was the volume using a glass beaker. The sediment was then poured into a 5-mm steel sieve stacked on top of a 1-mm steel sieve. All material (almost all of it fine sand) which passed through the sieves was discarded. The contents of the two sieves were then inspected to remove any obvious non-plastic materials such as vegetation, large stones, shells, etc. The remainder was examined thoroughly under a binocular microscope (Olympus SZX16).
To minimise contamination of the samples with airborne microplastics, a high-performance air purifier (DustBox 1000 VSC Hochleistungs-Luftreiniger H14) ran continuously along with the laboratory ventilation system, which also removed dust continuously during working hours. Furthermore, white laboratory coats made of cotton were worn by all laboratory workers, and synthetic textiles avoided. Moreover, all samples were immediately covered by aluminium lids whenever they were not worked on.
In addition, we a priori limited our lower size boundary of microplastics to 1 mm assuming that plastics ≥1 mm do not easily become airborne and should thus rarely contaminate our samples. To test this assumption, we filled a bowl with a surface area of approximately 500 cm2 with Milli-Q water and placed it directly next to the laboratory equipment, so that the water could catch airborne particles. At the end of five different laboratory days of working on sediment samples, we then also filtered the water from the bowl through a 5-μm pore size filter paper using a vacuum pump connected via a rubber tube to a collecting flask (Lee et al., 2019). Since there were no plastic particles on any of the examined filters, we concluded that airborne contamination of our sediment samples with plastic particles ≥1 mm did not occur.
2.3 Visual examination
Although definitions of size categories for plastic pollution vary, the following definitions are most commonly used (Hartmann et al., 2019): megaplastics (>100 cm), macroplastics (2.5–100 cm), mesoplastics (5–25 mm), microplastics (0.0001–5 mm), and nanoplastics (1–100 nm). Since we focussed only on mesoplastic (ME) and microplastic (MP) particles, we calculated amounts for both categories separately and combined (ME&MP).
The contents remaining in the two sieves were first visually examined under a binocular microscope under 2 × or 5 × magnification. For that purpose, we transferred a subsample onto a glass Petri dish and spread it in such a way that the particles formed a single particle layer with no particles lying on top of each other obscuring the view of particles below. We then visually scanned the entire contents for any putative ME and MP. Since >99% of the contents consisted of sand particles and bicarbonate shells (whole or broken into pieces) and organic material (e.g., stems, algae, etc.), it usually took only a few minutes to pick out putative plastic particles, which were transferred with tweezers to a glass container for later analysis by attenuated total reflectance Fourier transform infrared (ATR-FTIR) spectroscopy.
We emphasize here that every suspect particle was checked with ATR-FTIR. Non-suspect particles were all particles which were clearly sand particles or complete or broken shells, which made up >99% of the contents and which can be easily distinguished via visual examination after some visual training (see, e.g., Section 2.3 in Kunz et al., 2016, or Section 2.2. in Bancin et al., 2019). However, if there was any particle which did not clearly belong into the category of non-suspect particle, it was analyzed by ATR-FTIR. In most cases, our initial visual identification turned out to be correct, and putative particles turned out to indeed be plastic particles. In some cases, they turned out to be made of other materials (e.g., bicarbonate, cellulose, glass, coal, or metal). Therefore, we are confident that no plastic particle was missed using this method.
For sample 9, it was not possible to examine the entire sample visually because of the unusually high number of similar-looking fibres (Figure 5). We also observed that many fibres with lengths of both >1 mm and <1 mm were passing through the 1-mm sieve because of their shape. Therefore, we modified the visual counting procedure.
1. We did not discard the contents of the material that had passed through the 1-mm sieve, but included any fibres, which were >1 mm but had passed through the 1-mm sieve. To estimate the total number of fibres, we first placed the contents of the 5-mm sieve, the contents of the 1-mm sieve, and the contents which passed both sieves into separate glass beakers and then determined the weight and volume of those three subsamples.
2. We then placed 10% of each subsample into three separate glass beakers, and counted the greenish fibres (Figure 5). From each subsample, ten fibres were randomly selected and analysed by ATR-FTIR.
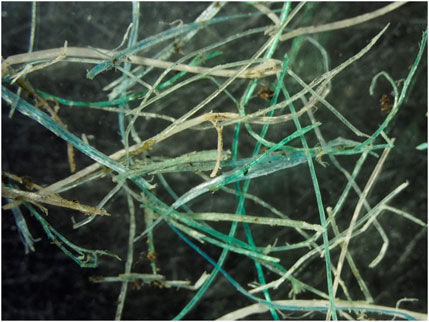
FIGURE 5. Greenish microplastic fibres consisting of polypropylene identified in sample 9 from Lomfjord, Rekvekstranda, Svalbard (credit: Franco Pasolini with permission).
2.4 Polymer identification
An ATR-FTIR device (ALPHA Basis Modul A250/DII, Bruker Optics) was used to identify the polymer type of putative plastic particles in combination with the installed OPUS spectroscopy software and the freeware siMPle software (Primpke et al., 2018; Primpke et al., 2020). We set the hit quality index (HQI) at ≥0.70 (meaning at least a 70% match with the reference spectrum) (Renner et al., 2019). Each particle was analysed by ATR-FTIR only once if the HQI was ≥0.70. At a lower HQI, we measured the particle three times in different places and then calculated the mean of the three measurements. If these extra measurements raised the mean HQI to ≥0.70, then the status of a putative plastic particle was changed to a definite plastic particle.
For all positively identified plastic particles in this study, OPUS and siMPle yielded identical polymer identifications with an HQI ≥0.70. For these particles, we also measured their maximum length (i.e., the longest possible distance between two endpoints of the particle).
2.5 Calculation of unweighted and weighted averages
Since the samples (Table 2) were of uneven amount, we used two methods to calculate a mean for the pollution level. The first one is to use each of the 53 samples as one unweighted datapoint (even though the weight and volume of each sample differed). This calculation results in an unweighted average (or mean) because each sample is given the same importance despite the amount of sediment being different between the individual samples (this is the ‘usual’ way of calculating a mean). The second method is to use each of the 53 samples as a weighted datapoint (with the weight of the datapoint depending on the amount of each sample, e.g., the weight of the sediment). This calculation results in a weighted average (or mean) because each sample is given an importance (or weight) according to the amount of the sediment sample.
3 Results
Over a period of 6 years, the citizen scientists collected 53 samples in 23 locations on Svalbard (Tables 1, 2; Figures 1, 2, Figure 4). For all 53 samples, we determined the weight and volume of the sediment, but we only had measurements of the area sampled for only 32 samples because no area was measured for samples 1–21 (Table 2). In total, 91377.7 g and 53200 mL of sediment were collected (sum of all rows in Table 2). The total surface area sampled for samples 22–53 was 1.8432 m2. Plastic particles ≥1 mm were detected in two out of 53 samples (3.8%).
In sample 31, we recorded six pink particles, which all consisted of polyester-epoxide (Figure 6) with a mean length of 2.6 ± 0.7 mm (mean ± standard deviation, range 1.9–3.8 mm). Therefore, for sample 31 only, the number of MP per kilogram was 2.08 MP kg-1, the number of MP per litre was 3.53 MP L-1, and the number of MP per square metre was 104.17 MP m-2.
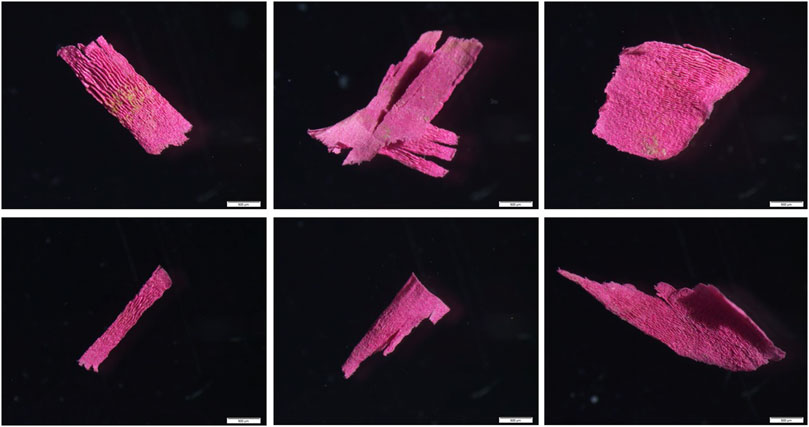
FIGURE 6. Photos of six pink microplastic particles consisting of polyester-epoxide identified in sample 31 from Gåshamna, Svalbard. The maximum lengths of the particles are (clockwise starting in the upper left corner): 2.3, 3.0, 2.7, 3.8, 1.9, and 2.0 mm. The scale bars are 0.5 mm (credit: Lisa Roscher with permission).
Since sample 9 contained hundreds of polypropylene fibres (Figure 5), we took a 10% subsample (see Methods). The number of fibres in the subsample of the contents of the 5-mm sieve was 182 fibres, which by definition were all ME particles. We also found 101 fibres in the 1-mm sieve subsample and 209 fibres in the subsample of the contents that passed through the 1-mm sieve, which by definition were all MP particles. This added up to 492 fibres in total. Multiplication by 10 yielded an estimate of 4920 fibres for the whole sample (1820 ME and 3100 MP). Therefore, for sample 9 only, the number of ME&MP per kg was 10399.49 ME&MP kg-1, and the number of ME&MP per litre was 7809.52 ME&MP L-1 (no surface area was measured for sample 9). From each of the three subsamples, ten fibres were randomly selected and identified as polypropylene. Since all fibres had a similar appearance, we concluded that all fibres likely consisted of polypropylene.
The 4926 particles recovered from two out of 53 samples were then used to calculate mean pollution levels (Table 3). For the mean pollution level per kg sediment and per L sediment, the weighted mean was always lower than the unweighted mean (ranging from 27.4% to 62.9% of the unweighted mean). The mean pollution levels for ME&MP using the unweighted mean were 196.3 ME&MP kg-1, 147.4 ME&MP L-1, and 3.3 ME&MP m-2; and for the weighted mean, they were 53.9 ME&MP kg-1, 92.6 ME&MP L-1, and 3.3 ME&MP m-2 (Table 3).
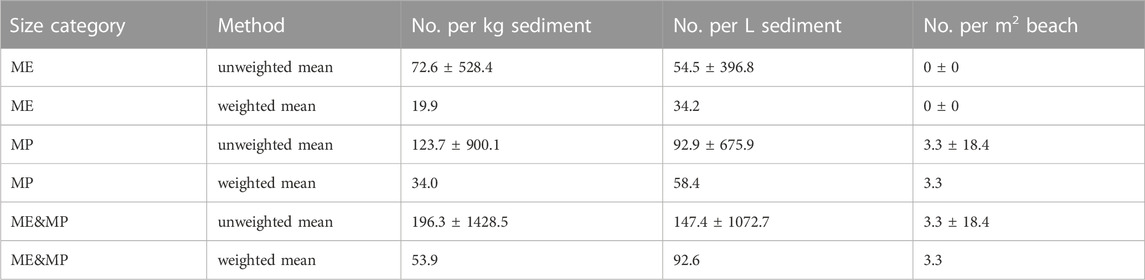
TABLE 3. Mean pollution levels of 53 sediment samples (Table 2). Two methods to calculate a mean for the three types of pollution level were used (see Methods 2.5). The sample size for individual samples was N = 53 for “No. per kg sediment” and “No. per L sediment” and N = 32 for “No. per m2 sediment” (see Table 2 for explanation).
4 Discussion
4.1 Pollution levels of meso- and microplastics
Although only two out of 53 samples from Svalbard beaches contained plastic particles ≥1 mm, this result supports the observation that meso- and microplastics have invaded the remotest places on Earth (Zhang et al., 2016; Evangeliou et al., 2020; Horton and Barnes, 2020; Napper et al., 2020; Trainic et al., 2020; Abel et al., 2021). The mean pollution levels in our samples could be the result of several conditions. One is that we used a lower size limit of 1 mm. Several previous studies have highlighted the importance of smaller microplastic in the Arctic, e.g., microplastics ≤25 μm accounted for 80% of the plastic particles in Arctic deep-sea sediment, 82% in the water column, and 88% in sea ice samples (Bergmann et al., 2017c; Peeken et al., 2018; Tekman et al., 2020). Since we excluded microplastic particles <1 mm, we likely missed smaller plastic particles present at our 23 sampling locations and underestimated contamination levels.
In addition, our sampling design influenced our estimates because from 2017 to 2021, only one sample per beach was taken due to our pilot-study approach. It is well known that sampling larger areas and/or retrieving more samples is a more adequate approach at low and/or patchy pollution levels (e.g., Bancin et al., 2019). Indeed, one of the two beaches where we recorded plastic particles was subject to the highest sampling intensity with 20 samples containing 54.4 kg in total, which of course increases the likelihood of finding plastic particles compared with a site were only one sample was taken (locations 1–21).
Another reason for lower pollution levels in this size range could be the coarse sediment types prevailing at most of the stations, with many being characterised by coarse sand, pebble or rock (Table 1). There is growing evidence that the grain size of sediments affects microplastic accumulation with coarser sediments retaining fewer microplastics (Corcoran et al., 2020; Waldschlager and Schattrumpf, 2020; Vermeiren et al., 2021; Yen et al., 2022).
Furthermore, the remoteness of Svalbard in relation to pollution sources could also explain why most samples did not contain plastic particles ≥1 mm. In highly polluted places, e.g., East Asia, a much higher proportion of samples contained plastic particles ≥1 mm (Kunz et al., 2016; Bancin et al., 2019; Walther et al., 2022). Although larger plastic debris has become widespread on the beaches of Svalbard (Bergmann et al., 2017b; Meyer et al., 2023), plastic particles ≥1 mm have apparently not yet reached the densities reported from more polluted regions.
4.2 Calculation of mean pollution levels
The means for MP per kg and MP per L were larger when we used the unweighted mean than when we used the weighted mean for calculating the mean pollution level (Table 3). The simple reason for that difference is that the sample containing the thousands of polypropylene fibres was the third smallest sample by weight. Thus, assigning it the same importance as the other 52 samples increases its overall effect on the calculated mean. Since a calculated mean is always a somewhat inaccurate estimate of the true mean (Walther and Moore, 2005), both methods of calculating the mean are valid although somewhat inaccurate estimates of the true mean. The only solution to increase accuracy would be to increase the sample size (Bancin et al., 2019). For our study, the true mean for the mean number of plastic particles ≥1 mm per kg is likely somewhere between 50 and 200 ME&MP kg-1, and for the mean number of plastic particles per L somewhere between 90 and 150 ME&MP L-1. However, given the relatively small sample size and the evidently rather patchy distribution of plastic particles among samples, even these ranges should only be considered preliminary estimates, which need to be substantiated with further sampling. We recommend that further sampling adopts the grid method presented in Figure 3 and used in location 22, or, if space is limited, a somewhat re-arranged grid as in location 23.
4.3 Comparison of mean pollution levels
There are few data from Svalbard to compare our data to. Dąbrowska (2021) found microplastics along the beaches of Adventfjorden and Isfjorden but did not provide quantitative data in English. A beach sample taken near the Barentsburg wastewater outlet in the midwest of Svalbard returned 11 microplastics (>20 µm) kg-1, which were made of cellophane. Sundet et al. (2016) reported 0.0–6.3 MP kg-1 in samples from Adventdalen in west Svalbard. At Breibogen in the northwest of Svalbard, beach sediments contained 111 MP L-1 above the high tide mark and 5-8 MP L-1 below the high tide mark (Sundet et al., 2017).
Our estimated mean pollution levels thus appear to be in the higher end of all these results, and it is actually not dissimilar to levels reported from regions usually considered to be more polluted. For example, a review of 11 studies of beach sediments from the ASEAN region (Curren et al., 2021) found similar pollution levels of 192.1 ± 211.1 MP kg-1 (mean ± std. dev., range 2.5–599 MP kg-1). A review of five studies of beach sediments from around the world (Peng et al., 2020) found higher pollution levels of 393.7 ± 753.0 MP kg-1 (mean ± std. dev., range 0.0–2116 MP kg-1), but still within the same order of magnitude. Therefore, it could be argued that our data fit quite well into the global context of beach pollution.
4.4 Plastic polymers and fragmentation processes
The first polymer type, polypropylene, is the second most manufactured polymer after polyethylene and is used in a wide variety of applications, e.g., clothes, carpets, pipes, furniture, medical and laboratory uses, medicine, food and drink containers and packaging, and ropes, which makes it a very common material for fishing nets and other fishing applications (PlasticsEurope, 2022). A quick internet search shows that fishing nets made of green polypropylene fibres are myriad, and the very high concentration of polypropylene fibres from sample 9 strongly suggests that a beached polypropylene net had disintegrated there. Fisheries debris was also suggested as a prime source of microplastics detected in subtidal sediments of the fjords of Svalbard (Lin et al., 2022).
The second polymer type, polyester-epoxide, is used in polyurethane applications and epoxy resins, which, in turn, can be used for adhesives, coatings, elastomers, and other end-use applications (PlasticsEurope, 2022). Therefore, the six polyester-epoxide particles from Gåshamna (sample 31) may have come from such an application, e.g., a colour coating of a ship or some equipment. Hence, we conclude that both larger plastic objects likely were carried onto the beaches via ocean currents (Strand et al., 2021) since these beaches are rarely visited.
In both samples with plastic particles, all plastic particles from each respective beach looked similar and consisted of the same polymer suggesting that they derived from one larger plastic object, which had fragmented into smaller plastic particles over time. Indeed, the extensive thermal cycles in Polar Regions could cause internal stresses and affect the strength of polymer composite materials, which results in tiny cracks in the material, a process intensified by humidity (Kablov and Startsev, 2021). Prolonged exposure to environmental conditions on Arctic beaches such as repeated freeze cycles, wet deposition including fog, and potentially up to 24 h of sunlight during summer could thus accelerate fragmentation processes. Many of the macroplastic items investigated by Meyer et al. (2023) were also very brittle, fragmented when picked up, or had already fragmented whilst on the beach (Figure 7), a process that has also been observed in the Russian Arctic (Ershova et al., 2022).
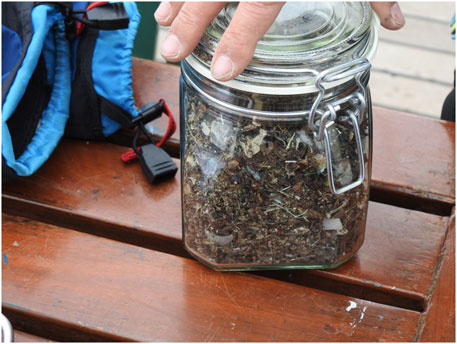
FIGURE 7. Photo of small fragmented plastic particles observed on Lomfjord (location 9) in August 2017 (credit B. Lutz with permission).
Consequently, Arctic beaches could act as a catalyst for fragmentation processes. This supposition is important because the polypropylene fibres from Lomfjord (sample 9) originated almost certainly from a single piece of fishing net that had disintegrated over time. Indeed, fisheries-related plastics, most of which were nets and ropes, accounted for 37% of the marine debris recorded during a parallel macro-debris survey on this beach (Meyer et al., 2023). The same study found that 30% of the analysed nets and ropes were made from polypropylene. Small fragments, which are disintegrating at the sediment surface could potentially also be dispersed more widely by winds (Ershova et al., 2021), which is a common Arctic trait.
Fast fragmentation processes on Arctic beaches could increase microplastic pollution. Consequently, Arctic food webs have been increasingly burdened with plastic pollution, adding to the upheaval due to climate heating, which is four times faster in the Arctic than the global average (Rantanen et al., 2022). Although the impact of plastic pollution on Arctic biota is still only poorly researched (Collard and Ask, 2021; Bergmann et al., 2022a), it is already widespread amongst northern fulmars from Svalbard (Fulmarus glacialis, 87.5% of investigated individuals), a sentinel species used for monitoring purposes (Trevail et al., 2015).
The pressure on Arctic biota will likely worsen as plastic pollution is projected to accumulate in the Nordic Seas due to a buildup via long-range transport from distant sources (van Sebille et al., 2012; Onink et al., 2019) and globally increasing plastic production (Borrelle et al., 2020; Lau et al., 2020), a process that would be worsened by an exponential fragmentation of existing plastics (MacLeod et al., 2021). This stresses the urgent need to implement binding and efficient global measures to combat plastic pollution (Bergmann et al., 2022b).
5 Conclusion
Citizen science is rapidly expanding into more and more areas of scientific investigation. The importance of citizen science to the issue of marine plastic pollution is evident from the fact that at least eight reviews have already been published (Thiel et al., 2014; Cigliano et al., 2015; Hidalgo-Ruz and Thiel, 2015; Zettler et al., 2017; Kawabe et al., 2022; Popa et al., 2022; Severin et al., 2022; Raman et al., 2023) and challenges and opportunities have been evaluated (Nelms et al., 2022). Our study is thus just another example of how collaboration between scientists and citizen scientists can provide useful, insightful and novel results.
One of the main reasons for the importance of our study is the fact that we still know relatively little about pollution levels in the Arctic despite the fact that the Arctic appears to be an accumulation zone for plastic pollution with pollution levels increasing rapidly. We therefore recommend that future citizen-science campaigns monitor macroplastic and take samples for microplastic pollution assessments in the Arctic using systematic sampling approaches as was done for samples 22–53 in this study (Table 2). Citizen scientists are willing and able to quickly learn and conduct various sampling protocols, even relatively difficult ones if the instructions are well-written and well-rehearsed (e.g., Walther et al., 2018; Yen et al., 2022). Consequently, we foresee many productive collaborations between scientists and citizen scientists in the future.
Data availability statement
The original contributions presented in the study are included in the article, further inquiries can be directed to the corresponding author.
Author contributions
MB conceived and designed the study. Laboratory work was conducted by FP and BAW. FP and BAW organized the database. BAW performed the numerical analysis. MB and BAW wrote the manuscript, with input from FP. All authors contributed to the article and approved the submitted version.
Funding
FP was funded by an Erasmus studentship, BW by the Dr. Ernst Weiße-Stiftung and MB by the Helmholtz-Gemeinschaft Deutscher Forschungszentren. This work contributed to the tasks of the Pollution Observatory of the Helmholtz-funded infrastructure programme FRAM (Frontiers in Arctic Marine Research).
Acknowledgments
The authors thank the involved expedition cruise operators (Cape Race Corporation, Tallship Company and Oceanwide Expeditions), the crews and expedition staffs of MS Cape Race, SV Antigua, MV Plancius, SV Rembrandt van Rijn and SV Noorderlicht for technical support and polar bear watches. We are greatly indebted to Birgit Lutz and Janosch Müller-Hillebrand who collected the samples or supervised the participating citizen scientists. We thank Sebastian Primpke for instruction on the ATR-FTIR setup and installation of siMPle. We gratefully acknowledge Tabea Hepfner, Špela Korez, and Sarah Lorenzana for assistance in the laboratory, and Lisa Roscher for the analysis of particles from sample 31 and for additional comments. We also thank two reviewers whose comments substantially improved the manuscript.
Conflict of interest
The authors declare that the research was conducted in the absence of any commercial or financial relationships that could be construed as a potential conflict of interest.
Publisher’s note
All claims expressed in this article are solely those of the authors and do not necessarily represent those of their affiliated organizations, or those of the publisher, the editors and the reviewers. Any product that may be evaluated in this article, or claim that may be made by its manufacturer, is not guaranteed or endorsed by the publisher.
References
Abel, S. M., Primpke, S., Int-Veen, I., Brandt, A., and Gerdts, G. (2021). Systematic identification of microplastics in abyssal and hadal sediments of the Kuril Kamchatka trench. Environ. Pollut. 269, 116095. doi:10.1016/j.envpol.2020.116095
Allen, D., Allen, S., Abbasi, S., Baker, A., Bergmann, M., Brahney, J., et al. (2022). Microplastics and nanoplastics in the marine-atmosphere environment. Nat. Rev. Earth Environ. 3, 393–405. doi:10.1038/s43017-022-00292-x
Baak, J. E., Linnebjerg, J. F., Barry, T., Gavrilo, M. V., Mallory, M. L., Price, C., et al. (2020). Plastic ingestion by seabirds in the circumpolar arctic: A review. Environ. Rev. 28, 506–516. doi:10.1139/er-2020-0029
Bancin, L. J., Walther, B. A., Lee, Y. C., and Kunz, A. (2019). Two-dimensional distribution and abundance of micro- and mesoplastic pollution in the surface sediment of Xialiao Beach, New Taipei City, Taiwan. Mar. Pollut. Bull. 140, 75–85. doi:10.1016/j.marpolbul.2019.01.028
Bergmann, M., Almroth, B. C., Brander, S. M., Dey, T., Green, D. S., Gundogdu, S., et al. (2022b). A global plastic treaty must cap production. Science 376, 469–470. doi:10.1126/science.abq0082
Bergmann, M., Collard, F., Fabres, J., Gabrielsen, G. W., Provencher, J. F., Rochman, C. M., et al. (2022a). Plastic pollution in the arctic. Nat. Rev. Earth Environ. 3, 323–337. doi:10.1038/s43017-022-00279-8
Bergmann, M., Lutz, B., Tekman, M. B., and Gutow, L. (2017b). Citizen scientists reveal: Marine litter pollutes Arctic beaches and affects wild life. Mar. Pollut. Bull. 125, 535–540. doi:10.1016/j.marpolbul.2017.09.055
Bergmann, M., Mutzel, S., Primpke, S., Tekman, M. B., Trachsel, J., and Gerdts, G. (2019). White and wonderful? Microplastics prevail in snow from the alps to the arctic. Sci. Adv. 5, eaax1157. doi:10.1126/sciadv.aax1157
Bergmann, M., Tekman, M. B., and Gutow, L. (2017a). Sea change for plastic pollution. Nature 544, 297. doi:10.1038/544297a
Bergmann, M., Wirzberger, V., Krumpen, T., Lorenz, C., Primpke, S., Tekman, M. B., et al. (2017c). High quantities of microplastic in arctic deep-sea sediments from the HAUSGARTEN observatory. Environ. Sci. Technol. 51, 11000–11010. doi:10.1021/acs.est.7b03331
Blinovskaya, Y. Y., Kulikova, O., Mazlova, E., and Gavrilo, M. (2020). Microplastic in the arctic and Russian far East coastal ground. Ecol. Ind. Russ. 24, 16–19. doi:10.18412/1816-0395-2020-4-16-19
Borrelle, S. B., Ringma, J., Law, K. L., Monnahan, C. C., Lebreton, L., McGivern, A., et al. (2020). Predicted growth in plastic waste exceeds efforts to mitigate plastic pollution. Science 369, 1515–1518. doi:10.1126/science.aba3656
Chen, M. C., and Chen, T. H. (2020). Spatial and seasonal distribution of microplastics on sandy beaches along the coast of the Hengchun Peninsula, Taiwan. Mar. Pollut. Bull. 151, 110861. doi:10.1016/j.marpolbul.2019.110861
Choudhary, S., Neelavanan, K., and Saalim, S. M. (2022). Microplastics in the surface sediments of krossfjord-kongsfjord system, svalbard, arctic. Mar. Pollut. Bull. 176, 113452. doi:10.1016/j.marpolbul.2022.113452
Cigliano, J. A., Meyer, R., Ballard, H. L., Freitag, A., Phillips, T. B., and Wasser, A. (2015). Making marine and coastal citizen science matter. Ocean. Coast. Manage. 115, 77–87. doi:10.1016/j.ocecoaman.2015.06.012
Collard, F., and Ask, A. (2021). Plastic ingestion by arctic fauna: A review. Sci. Total Environ. 786, 147462. doi:10.1016/j.scitotenv.2021.147462
Collard, F., Husum, K., Eppe, G., Malherbe, C., Hallanger, I. G., Divine, D. V., et al. (2021). Anthropogenic particles in sediment from an Arctic fjord. Sci. Total Environ. 772, 145575. doi:10.1016/j.scitotenv.2021.145575
Corcoran, P. L., de Haan Ward, J., Arturo, I. A., Belontz, S. L., Moore, T., Hill-Svehla, C. M., et al. (2020). A comprehensive investigation of industrial plastic pellets on beaches across the Laurentia Great Lakes and the factors governing their distribution. Sci. Total Environ. 747, 141227. doi:10.1016/j.scitotenv.2020.141227
Curren, E., Kuwahara, V. S., Yoshida, T., and Leong, S. C. Y. (2021). Marine microplastics in the ASEAN region: A review of the current state of knowledge. Environ. Pollut. 288, 117776. doi:10.1016/j.envpol.2021.117776
Dąbrowska, A. (2021). Marine microplastics in polar region—A spitsbergen case study. Water Air Soil Pollut. 232, 393. doi:10.1007/s11270-021-05346-2
Ershova, A., Makeeva, I., Malgina, E., Sobolev, N., and Smolokurov, A. (2021). Combining citizen and conventional science for microplastics monitoring in the White Sea basin (Russian Arctic). Mar. Pollut. Bull. 173, 112955. doi:10.1016/j.marpolbul.2021.112955
Ershova, A., Vorotnichenko, E., Kotelnikov, V., and Krutikov, I. (2022). Marine litter accumulation patterns in the Russian part of the Barents Sea. Avaliable At: https://www.researchgate.net/publication/365654880_Marine_litter_accumulation_patterns_in_the_Russian_part_of_the_Barents_Sea.
Evangeliou, N., Grythe, H., Klimont, Z., Heyes, C., Eckhardt, S., Lopez-Aparicio, S., et al. (2020). Atmospheric transport is a major pathway of microplastics to remote regions. Nat. Commun. 11, 3381. doi:10.1038/s41467-020-17201-9
Falk-Andersson, J., Berkhout, B. W., and Abate, T. G. (2019). Citizen science for better management: Lessons learned from three Norwegian beach litter data sets. Mar. Pollut. Bull. 138, 364–375. doi:10.1016/j.marpolbul.2018.11.021
Falk-Andersson, J., and Strietman, W. J. (2019). Svalbard beach litter deep dive. Tromsø, Norway: SALT Lofoten AS. Avaliable At: https://www.researchgate.net/publication/332349910_Svalbard_Beach_Litter_Deep_Dive.
Falk-Andersson, J., Tairova, Z., Drægni, T. T., and Haarr, M. L. (2021). Methods for determining the geographical origin and age of beach litter: Challenges and opportunities. Mar. Pollut. Bull. 172, 112901. doi:10.1016/j.marpolbul.2021.112901
Geyer, R. (2020). “A brief history of plastics,” in Mare Plasticum – the plastic sea: Combating plastic pollution through science and art. Editors M. Streit-Bianchi, M. Cimadevila, and W. Trettnak (Cham, Switzerland: Springer), 31–48.
Geyer, R., Jambeck, J. R., and Law, K. L. (2017). Production, use, and fate of all plastics ever made. Sci. Adv. 3, 1700782. doi:10.1126/sciadv.1700782
Goli, V. S. N. S., Paleologos, E. K., Farid, A., Mohamed, A. M. O., O’Kelly, B. C., El Gamal, M. M., et al. (2022). Extraction, characterisation and remediation of microplastics from organic solid matrices. Environ. Geotech. 40, 1–34. doi:10.1680/jenge.21.00072
Granberg, M., von Friesen, L. W., Bach, L., Collard, F., Gabrielsen, G. W., and Strand, J. (2019). Anthropogenic microlitter in wastewater and marine samples from ny-ålesund, Barentsburg and Signehamna, svalbard. Stockholm, Sweden: IVL Swedish Environmental Research Institute. Avaliable At: https://brage.npolar.no/npolar-xmlui/bitstream/handle/11250/2588681/Granberg.pdf?sequence=1.
Grøsvik, B. E., Buhl-Mortensen, L., Bergmann, M., Booth, A. M., Gomiero, A., and Galgani, F. (2022). Status and future recommendations for recording and monitoring litter on the Arctic seafloor. Arct. Sci. 9, 345–355. doi:10.1139/AS-2022-0017
Hartmann, N. B., Huffer, T., Thompson, R. C., Hassellov, M., Verschoor, A., Daugaard, A. E., et al. (2019). Are we speaking the same language? Recommendations for a definition and categorization framework for plastic debris. Environ. Sci. Technol. 53, 1039–1047. doi:10.1021/acs.est.8b05297
Hidalgo-Ruz, V., and Thiel, M. (2015). “The contribution of citizen scientists to the monitoring of marine litter,” in Marine anthropogenic litter. Editors M. Bergmann, L. Gutow, and M. Klages (Berlin: Springer Open), 429–447.
Horton, A. A., and Barnes, D. K. (2020). Microplastic pollution in a rapidly changing world: Implications for remote and vulnerable marine ecosystems. Sci. Total Environ. 738, 140349. doi:10.1016/j.scitotenv.2020.140349
Jaskólski, M., Pawlowski, Ł., Strzelecki, M., Zagórski, P., and Lane, T. P. (2018). Trash on arctic beach: Coastal pollution along calypsostranda, bellsund, svalbard. Pol. Polar Res. 39, 211–224. doi:10.24425/118746
Kablov, E., and Startsev, V. (2021). The influence of internal stresses on the aging of polymer composite materials: A review. Mech. Compos. Mat. 57, 565–576. doi:10.1007/s11029-021-09979-6
Kawabe, L., Ghilardi-Lopes, N., Turra, A., and Wyles, K. (2022). Citizen science in marine litter research: A review. Mar. Pollut. Bull. 182, 114011. doi:10.1016/j.marpolbul.2022.114011
Koutnik, V. S., Leonard, J., Alkidim, S., DePrima, F. J., Ravi, S., Hoek, E. M., et al. (2021). Distribution of microplastics in soil and freshwater environments: Global analysis and framework for transport modeling. Environ. Pollut. 274, 116552. doi:10.1016/j.envpol.2021.116552
Kunz, A., Walther, B. A., Löwemark, L., and Lee, Y. C. (2016). Distribution and quantity of microplastic on sandy beaches along the northern coast of Taiwan. Mar. Pollut. Bull. 111, 126–135. doi:10.1016/j.marpolbul.2016.07.022
Lau, W. W., Shiran, Y., Bailey, R. M., Cook, E., Stuchtey, M. R., Koskella, J., et al. (2020). Evaluating scenarios toward zero plastic pollution. Science 369, 1455–1461. doi:10.1126/science.aba9475
Lee, H., Kunz, A., Shim, W. J., and Walther, B. A. (2019). Microplastic contamination of table salts from Taiwan, including a global review. Sci. Rep. 9, 10145. doi:10.1038/s41598-019-46417-z
Lin, Y., Cen, Z., Peng, J., Yu, H., Huang, P., Huang, Q., et al. (2022). Occurrence and sources of microplastics and polycyclic aromatic hydrocarbons in surface sediments of Svalbard, Arctic. Mar. Pollut. Bull. 184, 114116. doi:10.1016/j.marpolbul.2022.114116
Liutkus, M., Olenin, S., Węsławski, J. M., and Kotwicki, L. (2022). Wave fetch and distance from the ocean determine the distribution of macroplastics in the intertidal zone of central Spitsbergen, Arctic. Mar. Pollut. Bull. 177, 113516. doi:10.1016/j.marpolbul.2022.113516
MacLeod, M., Arp, H. P. H., Tekman, M. B., and Jahnke, A. (2021). The global threat from plastic pollution. Science 373, 61–65. doi:10.1126/science.abg5433
Meyer, A. N., Lutz, B., and Bergmann, M. (2023). Where does arctic beach debris come from? Analyzing debris composition and provenance on svalbard aided by citizen scientists. Front. Mar. Sci. 10, 1092939. doi:10.3389/fmars.2023.1092939
Munno, K., Helm, P. A., Jackson, D. A., Rochman, C., and Sims, A. (2018). Impacts of temperature and selected chemical digestion methods on microplastic particles. Environ. Toxicol. Chem. 37, 91–98. doi:10.1002/etc.3935
Napper, I. E., Davies, B. F. R., Clifford, H., Elvin, S., Koldewey, H. J., Mayewski, P. A., et al. (2020). Reaching new heights in plastic pollution—Preliminary findings of microplastics on mount everest. One Earth 3, 621–630. doi:10.1016/j.oneear.2020.10.020
Nashoug, B. F. (2017). “Sources of marine litter –workshop report,” in Svalbard 4th -6th september 2016 (Svolvær, Norway: SALT: Norwegian Research Council). Avaliable At: https://pame.is/document-library/desktop-study-on-marine-litter-library/marine-litter-sources/577-nashoug-2017-sources-of-marine-litter-worksh/file.
Nelms, S. E., Easman, E., Anderson, N., Berg, M., Coates, S., Crosby, A., et al. (2022). The role of citizen science in addressing plastic pollution: Challenges and opportunities. Environ. Sci. Policy 128, 14–23. doi:10.1016/j.envsci.2021.11.002
Onink, V., Wichmann, D., Delandmeter, P., and van Sebille, E. (2019). The role of Ekman currents, geostrophy, and Stokes drift in the accumulation of floating microplastic. J. Geophys. Res. Oceans 124, 1474–1490. doi:10.1029/2018JC014547
Parga Martínez, K. B., Tekman, M. B., and Bergmann, M. (2020). Temporal trends in marine litter at three stations of the HAUSGARTEN observatory in the Arctic deep sea. Front. Mar. Sci. 7, 321. doi:10.3389/fmars.2020.00321
Peeken, I., Primpke, S., Beyer, B., Gütermann, J., Katlein, C., Krumpen, T., et al. (2018). Arctic sea ice is an important temporal sink and means of transport for microplastic. Nat. Commun. 9, 1505. doi:10.1038/s41467-018-03825-5
Peng, L. C., Fu, D. D., Qi, H. Y., Lan, C. Q., Yu, H. M., and Ge, C. J. (2020). Micro- and nano-plastics in marine environment: Source, distribution and threats - a review. Sci. Total Environ. 698, 134254. doi:10.1016/j.scitotenv.2019.134254
Pfeiffer, F., and Fischer, E. K. (2020). Various digestion protocols within microplastic sample processing—Evaluating the resistance of different synthetic polymers and the efficiency of biogenic organic matter destruction. Front. Environ. Sci. 8, 572424. doi:10.3389/fenvs.2020.572424
PlasticsEurope (2022). Plastics – the facts 2022. (Wemmel, Belgium: PlasticsEurope). Avaliable At: https://plasticseurope.org/wp-content/uploads/2022/12/PE-PLASTICS-THE-FACTS_FINAL_DIGITAL.pdf.
Popa, C. L., Dontu, S. I., Savastru, D., and Carstea, E. M. (2022). Role of citizen scientists in environmental plastic litter research—A systematic review. Sustainability 14, 13265. doi:10.3390/su142013265
Primpke, S., Booth, A. M., Gerdts, G., Gomiero, A., Kögel, T., Lusher, A., et al. (2022). Monitoring of microplastic pollution in the arctic: Recent developments in polymer identification, quality assurance and control, and data reporting. Arct. Sci. 9, 176–197. doi:10.1139/as-2022-0006
Primpke, S., Cross, R. K., Mintenig, S. M., Simon, M., Vianello, A., Gerdts, G., et al. (2020). Toward the systematic identification of microplastics in the environment: Evaluation of a new independent software tool (siMPle) for spectroscopic analysis. Appl. Spectrosc. 74, 1127–1138. doi:10.1177/0003702820917760
Primpke, S., Wirth, M., Lorenz, C., and Gerdts, G. (2018). Reference database design for the automated analysis of microplastic samples based on Fourier transform infrared (FTIR) spectroscopy. Anal. Bioanal. Chem. 410, 5131–5141. doi:10.1007/s00216-018-1156-x
Provencher, J. F., Aliani, S., Bergmann, M., Bourdages, M., Buhl-Mortensen, L., Galgani, F., et al. (2023). Future monitoring of litter and microplastics in the arctic—Challenges, opportunities, and strategies. Arct. Sci. 9, 209–226. doi:10.1139/as-2022-0011
Puskic, P. S., Lavers, J. L., and Bond, A. L. (2020). A critical review of harm associated with plastic ingestion on vertebrates. Sci. Total Environ. 743, 140666. doi:10.1016/j.scitotenv.2020.140666
Raman, N. V., Dubey, A., Millar, E., Nava, V., Leoni, B., and Gallego, I. (2023). Monitoring contaminants of emerging concern in aquatic systems through the lens of citizen science. Sci. Total Environ. 874, 162527. doi:10.1016/j.scitotenv.2023.162527
Rantanen, M., Karpechko, A. Y., Lipponen, A., Nordling, K., Hyvärinen, O., Ruosteenoja, K., et al. (2022). The Arctic has warmed nearly four times faster than the globe since 1979. Commun. Earth Environ. 3, 168. doi:10.1038/s43247-022-00498-3
Renner, G., Nellessen, A., Schwiers, A., Wenzel, M., Schmidt, T. C., and Schram, J. (2019). Data preprocessing and evaluation used in the microplastics identification process: A critical review and practical guide. Trends Anal. Chem. 111, 229–238. doi:10.1016/j.trac.2018.12.004
Schwarz, A., Lensen, S., Langeveld, E., Parker, L., and Urbanus, J. H. (2023). Plastics in the global environment assessed through material flow analysis, degradation and environmental transportation. Sci. Total Environ. 875, 162644. doi:10.1016/j.scitotenv.2023.162644
Severin, M. I., Hooyberg, A., Everaert, G., and Catarino, A. (2022). “Using citizen science to understand plastic pollution: Implications for science and participants,” in Living in the plastic age. perspectives from humanities, social sciences and environmental sciences. Editors J. Kramm, and C. Völker (Berlin: Spinger), 133–168.
Strand, K. O., Huserbråten, M., Dagestad, K. F., Mauritzen, C., Grøsvik, B. E., Nogueira, L. A., et al. (2021). Potential sources of marine plastic from survey beaches in the Arctic and Northeast Atlantic. Sci. Total Environ. 790, 148009. doi:10.1016/j.scitotenv.2021.148009
Sundet, J. H., Herzke, D., and Jenssen, M. (2017). Forekomst av mikroplastikk i sjøvann, bunnsedimenter, fjæresediment og i filtrerende bunnorganismer i nære kystområder på Svalbard. RIS-prosjekt nr 10495. Svalbards Miljøvernfond). Avaliable At: https://pame.is/document-library/desktop-study-on-marine-litter-library/additional-documents/annexes-literature-from-the-desktop-study/table-2-2-abundance-of-plastics-observed-in-sea-ice-and-seawater/509-sundet-2017-forekomst-av-mikroplastikk-i-sjova/file.
Sundet, J. H., Herzke, D., and Jenssen, M. (2016). Forekomst og kilder av mikroplastikk i sediment, og konsekvenser for bunnlevende frisk og evertebrater på Svalbard RIS-prosjekt nr 10495 Svalbards Miljøvernfond). Avaliable At: https://pame.is/document-library/desktop-study-on-marine-litter-library/additional-documents/annexes-literature-from-the-desktop-study/table-2-4-abundance-of-microplastics-observed-in-sediments/508-sundet-2016-forekomst-og-kilder-av-mikroplasti/file.
Tekman, M. B., Gutow, L., and Bergmann, M. (2022a). Marine debris floating in Arctic and temperate Northeast Atlantic waters. Front. Mar. Sci. 9, 933768. doi:10.3389/fmars.2022.933768
Tekman, M. B., Walther, B. A., Peter, C., Gutow, L., and Bergmann, M. (2022b). Impacts of plastic pollution in the oceans on marine species, biodiversity and ecosystems. Berlin, Germany: WWF Germany. Avaliable At: https://www.wwf.de/fileadmin/fm-wwf/Publikationen-PDF/Plastik/WWF-Impacts_of_plastic_pollution_in_the_ocean_on_marine_species__biodiversity_and_ecosystems.pdf.
Tekman, M. B., Wekerle, C., Lorenz, C., Primpke, S., Hasemann, C., Gerdts, G., et al. (2020). Tying up loose ends of microplastic pollution in the Arctic: Distribution from the sea surface through the water column to deep-sea sediments at the HAUSGARTEN observatory. Environ. Sci. Technol. 54, 4079–4090. doi:10.1021/acs.est.9b06981
Thiel, M., Penna-Díaz, M. A., Luna-Jorquera, G., Sala, S., Sellanes, J., and Stotz, W. (2014). Citizen scientists and marine research: Volunteer participants, their contributions and projection for the future. Oceanogr. Mar. Biol. Ann. Rev. 52, 257–314. doi:10.1201/b17143-6
Trainic, M., Flores, J. M., Pinkas, I., Pedrotti, M. L., Lombard, F., Bourdin, G., et al. (2020). Airborne microplastic particles detected in the remote marine atmosphere. Commun. Earth Environ. 1, 64. doi:10.1038/s43247-020-00061-y
Trevail, A. M., Gabrielsen, G. W., Kühn, S., and Van Franeker, J. A. (2015). Elevated levels of ingested plastic in a high Arctic seabird, the northern fulmar (Fulmarus glacialis). Polar Biol. 38, 975–981. doi:10.1007/s00300-015-1657-4
van Sebille, E., England, M. H., and Froyland, G. (2012). Origin, dynamics and evolution of ocean garbage patches from observed surface drifters. Environ. Res. Lett. 7, 044040. doi:10.1088/1748-9326/7/4/044040
Vermeiren, P., Lercari, D., Munoz, C. C., Ikejima, K., Celentano, E., Jorge-Romero, G., et al. (2021). Sediment grain size determines microplastic exposure landscapes for sandy beach macroinfauna. Environ. Pollut. 286, 117308. doi:10.1016/j.envpol.2021.117308
Villarrubia-Gomez, P., Cornell, S. E., and Fabres, J. (2018). Marine plastic pollution as a planetary boundary threat - the drifting piece in the sustainability puzzle. Mar. Policy 96, 213–220. doi:10.1016/j.marpol.2017.11.035
Waldschlager, K., and Schuttrumpf, H. (2020). Infiltration behavior of microplastic particles with different densities, sizes, and shapes—From glass spheres to natural sediments. Environ. Sci. Technol. 54, 9366–9373. doi:10.1021/acs.est.0c01722
Walther, B. A., and Bergmann, M. (2022). Plastic pollution of four understudied marine ecosystems: A review of mangroves, seagrass meadows, the arctic ocean and the deep seafloor. Emerg. Top. Life Sci. 6, 371–387. doi:10.1042/ETLS20220017
Walther, B. A., Kunz, A., and Hu, C. S. (2018). Type and quantity of coastal debris pollution in taiwan: A 12-year nationwide assessment using citizen science data. Mar. Pollut. Bull. 135, 862–872. doi:10.1016/j.marpolbul.2018.08.025
Walther, B. A., Kusui, T., Yen, N., Hu, C. S., and Lee, H. (2022). “Plastic pollution in East Asia: Macroplastics and microplastics in the aquatic environment and mitigation efforts by various actors,” in Plastics in the aquatic environment - Part I: Current status and challenges. Editors F. Stock, G. Reifferscheid, N. Brennholt, and E. Kostianaia (Cham, Switzerland: Springer), 353–403.
Walther, B. A., and Moore, J. L. (2005). The concepts of bias, precision and accuracy, and their use in testing the performance of species richness estimators, with a literature review of estimator performance. Ecography 28, 815–829. doi:10.1111/j.2005.0906-7590.04112.x
Waters, C. N., Zalasiewicz, J., Summerhayes, C., Barnosky, A. D., Poirier, C., Gałuszka, A., et al. (2016). The Anthropocene is functionally and stratigraphically distinct from the Holocene. Science 351, 2622. doi:10.1126/science.aad2622
Węsławski, J. M., and Kotwicki, L. (2018). Macro-plastic litter, a new vector for boreal species dispersal on Svalbard. Pol. Polar Res. 39, 165–174. doi:10.24425/118743
Yen, N., Hu, C. S., Chiu, C. C., and Walther, B. A. (2022). Quantity and type of coastal debris pollution in taiwan: A rapid assessment with trained citizen scientists using a visual estimation method. Sci. Total Environ. 822, 153584. doi:10.1016/j.scitotenv.2022.153584
Zalasiewicz, J., Waters, C. N., do Sul, J. A. I., Corcoran, P. L., Barnosky, A. D., Cearreta, A., et al. (2016). The geological cycle of plastics and their use as a stratigraphic indicator of the Anthropocene. Anthropocene 13, 4–17. doi:10.1016/j.ancene.2016.01.002
Zettler, E., Takada, H., Monteleone, B., Mallos, N., Eriksen, M., and Amaral-Zettler, L. (2017). Incorporating citizen science to study plastics in the environment. Anal. Meth. 9, 1392–1403. doi:10.1039/C6AY02716D
Keywords: Arctic, beach debris, citizen science, derelict fishing gear, marine litter, plastic pollution, microplastics, Polar Regions
Citation: Pasolini F, Walther BA and Bergmann M (2023) Citizen scientists reveal small but concentrated amounts of fragmented microplastic on Arctic beaches. Front. Environ. Sci. 11:1210019. doi: 10.3389/fenvs.2023.1210019
Received: 24 April 2023; Accepted: 26 June 2023;
Published: 21 July 2023.
Edited by:
Nsikak U. Benson, Université Claude Bernard Lyon 1, FranceReviewed by:
Ana Virginia Filgueiras, Spanish Institute of Oceanography (IEO), SpainJames Kar-Hei Fang, Hong Kong Polytechnic University, Hong Kong SAR, China
Copyright © 2023 Pasolini, Walther and Bergmann. This is an open-access article distributed under the terms of the Creative Commons Attribution License (CC BY). The use, distribution or reproduction in other forums is permitted, provided the original author(s) and the copyright owner(s) are credited and that the original publication in this journal is cited, in accordance with accepted academic practice. No use, distribution or reproduction is permitted which does not comply with these terms.
*Correspondence: Bruno Andreas Walther, YmF3YWx0aGVyMjAwOUBnbWFpbC5jb20=