- 1Department of Civil and Environmental Engineering, Imperial College London, United Kingdom
- 2Bear Valley Ventures, Tarporley, United Kingdom
- 3Biofiltro, Davis, CA, United States
- 4TBF Environmental, Pune, India
- 5Institute for Transformative Technologies, Berkeley, CA, United States
- 6IHE-Delft, Netherlands
- 7Oxfam, Oxford, United Kingdom
- 8Water For People, Denver, CO, United States
Tiger Worm Toilets (TWTs) are a relatively recent innovation in sanitation technology, with the potential to offer affordable and safely managed on-site sanitation for underserved communities in line with Sustainable Development Goal 6. This article, authored by members of the International Worm-Based Sanitation Association (IWBSA), presents the state-of-the-art in TWT construction and operation based on frontier global experiences to-date, stemming from both knowledge from literature and the practical knowledge imparted by the authors, who were involved in the birth of this technology and are still active in the research and deployment of it today. The focus of this paper is on the implementation of TWTs to solve sanitation challenges in least-developed and lower-middle income countries specifically. The design of TWTs can be adapted to a wide range of environmental conditions including high water tables and rocky ground conditions, and in both rural and urban contexts. The challenge for TWTs therefore lies primarily in creating opportunities for scaling the uptake of this promising technology. Our article discusses the key barriers to this, identified to be broadly categorised as user preference, capacity and capability building, and governmental support–and proposes how to overcome these.
Introduction
Tiger Worm Toilets (TWTs) use a worm-based ecosystem to degrade human waste in situ. As illustrated in Figure 1, a TWT consists of a bio-digester tank containing composting worms living in layers of organic bedding material, such as chipped coconut husks or woodchips, which also acts as a primary filter by trapping solids on the surface. Drainage layers beneath, e.g., gravel, ensure the system is aerobic and that the worms can breathe.
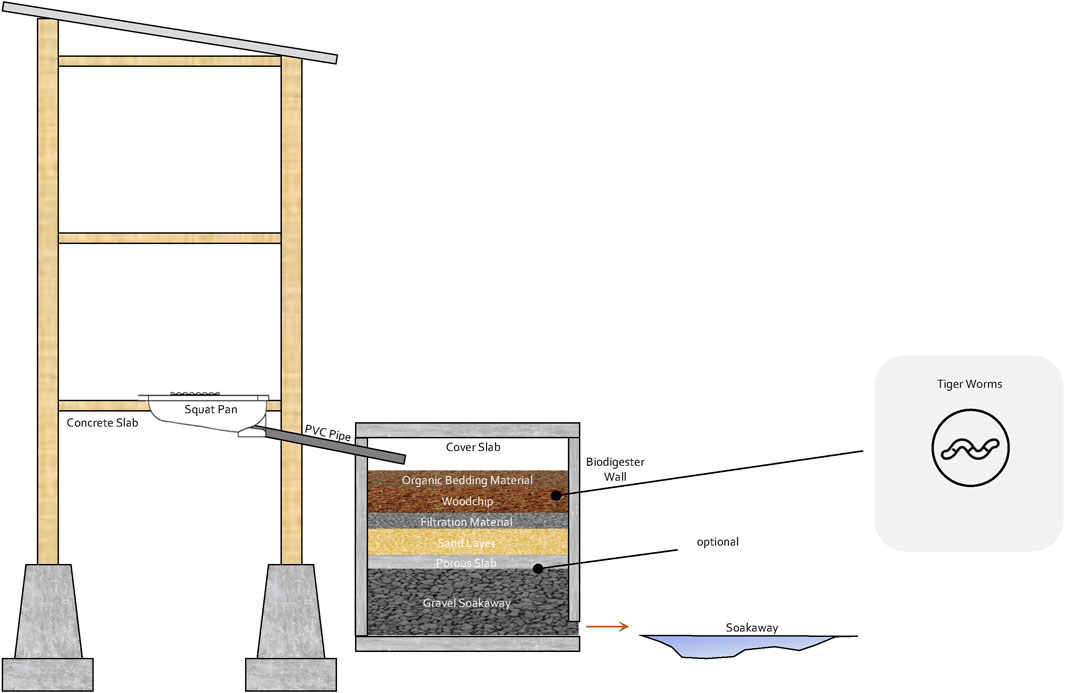
FIGURE 1. An example of a TWT design, adapted from Sioné et al. (2023).
Tiger worms (Eisenia fetida) feed on the faeces that enter the biodigester via a pipe or direct drop from the toilet and produce nutrient-rich humus known as vermicompost. This process is known as “vermifiltration” and results in complete degradation of faecal solids (Engineering for Change, 2023). The vermicompost produced by the worms is a dry, odourless solid that accumulates within the digester, whilst the liquid effluent is able to drain away. Vermifiltration research has showed a 98.65% reduction in faecal coliforms over a 24-h period and field tests reported significant removal of chemical oxygen demand (COD), thermotolerant coliforms and total solids from the effluent (Furlong et al., 2014; Furlong et al., 2016). Therefore, the compost is safer to empty and transport than faecal sludge.
Moreover, the vermicompost builds up more slowly than the accumulation of faecal sludge in traditional pit latrines because solids are digested by the composting worms and converted to vermicompost and carbon dioxide which is dissipated into the air. The result is a smaller treatment system, which requires less frequent emptying (Eastman et al., 2001). A vermifilter typically requires emptying once every 6–8 years, whereas a traditional pit latrine may need emptying every 2 years, depending on a range of factors including size, frequency of use, volume of water used for flushing, etc (Burt et al., 2019; Hylton et al., 2022).
This Perspective article first presents an overview the Tiger Worm Toilet technology, including siting, worm requirements and design of the biodigester. A discussion on scaling ensues, touching on cost, the importance of user preferences, strategies to build capacity, and the importance of governmental support.
Overview of the tiger worm technology
Several species of epigeic worms have been used in TWTs, including Eisenia fetida (redworm, tiger worm), Eisenia andrei, and Eudrilus eugeniae (African Night Crawler) (Furlong et al., 2017a; Oxfam, 2019). The Eisenia fetida and Eisenia andrei species are native to Europe and are widely used for vermicomposting there but also in North America and other parts of the world. In particular, Eisenia fetida is now found worldwide, excluding Antarctica. These worms are highly efficient in processing organic waste, reproduce rapidly, and can tolerate a wide range of temperature, acidity, and moisture conditions. Depending on the country’s history of vermicomposting and worm farming, worms for TWTs can be sourced through local farming or importing, as explored in studies conducted in South Africa and India (Furlong, n.d.; Furlong, et al., 2017b).
The quantity of worms needed for the TWT can be determined by estimating the mass of faeces supplied to the biodigester each day and assuming that 1 kg of worms can feed on 1 kg of faeces (Edwards et al., 2010; Sinha et al., 2011; Furlong, 2014; Oxfam, 2020). The estimation of the mass does not need to be precise and can easily be done at household level, by enquiring how many people reside in the house and knowing that a person produces on average around 200 g of faeces daily (Rose et al., 2015). As an example, a household of 10 people generates approximately 2 kg of faeces, requiring 2 kg of worms. It is recommended to provide the system with an extra 20% of worms (in our example, this would be 0.5 kg of worms) to allow for potential losses when the worms are acclimatising (Oxfam, 2019; 2020). As a guideline, the biodigester of a TWT for a 10-person household is recommended to have internal dimensions of 1.2 m in height, 1 m in length, and 1 m in width. This size is determined based on the optimal worm loading of 2 kg/m2.
Advantageously, the biodigester can be positioned either directly below or offset from the toilet, making it suitable for high-density urban areas, where it is most valuable in reducing the prohibitive cost of de-sludging of communal latrines as opposed to in rural areas where its primary purpose is to help avoid open defecation. However, in urban settings there is sometimes not the space to build new TWTs, and so ideally existing latrines could be retrofitted into TWTs to address this issue. Research is needed on how to do this, both from an engineering and a management perspective. Moreover, the biodigester can be built off-set and above or below ground, depending on user preference and environmental conditions, which is particularly useful to avoid high water tables or flood-prone areas, or to avoid having to dig in rocky soil (Furlong, et al., 2017a).
The bedding layer, located approximately 30 cm below the inlet, should have a depth of at least 10 cm and should consist of materials that maintain an aerobic environment and are not easily compressed. Currently known suitable bedding materials include chipped coconut shells, vermicompost, woodchips, or a combination of these but other local materials may also be appropriate and should be explored (Oxfam, 2020). The drainage layer, approximately 30 cm deep, supports the bedding layer and acts as a filter for the effluent, removing suspended solids and organic materials (Oxfam, 2019). It also serves as a safeguard against flooding by providing air spaces that can fill with effluent if the infiltration rate is low. The requirements for the materials composing the drainage layer are loose, other than they should consist of materials of different sizes, with finer materials on top and coarser materials at the bottom (e.g., aggregate).
The effluent from the biodigester can either be discharged into the surrounding soil through direct infiltration or a soakaway if there is space (Figure 1), or collected in an external sump, which may prove to be particularly useful if constructing in higher density areas. Currently, collection in the sump is often adopted by users who use the liquid effluent for fertilization or irrigation of crops, though research is ongoing to determine whether this is safe (Sioné et al., 2023). The size of the soakaway should be based on the expected influent quantity and the soil’s infiltration rate. A higher infiltration rate is desirable for the effluents to permeate through the soil. According to Oxfam (2020), the minimum desired infiltration rate varies according to flush volume and number of flushes per day, and this should be verified against the actual infiltration rate of the pit. If the TWT is located in fragile ecosystems or locations with high water tables, the use of an external sump is preferrable, as nitrification has been observed in vermifilters, which may impact groundwater quality and eutrophication due to the nitrate and phosphorus levels in the effluent (Furlong et al., 2016).
TWTs operate most effectively when the worms are kept moist, but it is also crucial to ensure good drainage to prevent over-saturation, which can be detrimental to the worms, causing them to drown or leave the TWT biodigester (Oxfam, 2020). Therefore, TWTs are unsuitable for cases where no water is introduced into the toilet and they should not be implemented in water scarce regions. This is another pertinent reason to locate the TWT at least 20 m away from the nearest well or borehole (though this also applies to other onsite sanitation systems, to preserve groundwater quality) and should not be constructed in areas that experience water tables that rise higher than the bottom of the filtration material layer (Islam et al., 2016).
Before handing over the TWT system to the household, it is important to communicate the proper operation and maintenance of the system, sensitizing the users to the detrimental effect of harsh chemicals and acidic cleaning substances on the worm population. Moreover, the bedding layer materials (e.g., woodchips) will decompose over time, and may need to be replaced after 2–3 years. In some cases, omitting to replace (or include) a bedding layer may decrease permeability, leading to ponding of flush water. The accumulated vermicompost should be removed once the biodigester is full, which occurs on average every 6–8 years (Hylton et al., 2022). The worms will tend to move to the drainage layer during manipulation of the bedding layer, but it is important to add worms to the system if some are noticed to be removed with the vermicompost or bedding.
Discussion
Scaling any development innovation is challenging and many promising technologies remain small scale (OECD, 2015). Therefore, the aim of this discussion is to explore how to ensure that this is not the fate of TWTs. As a first step towards encouraging scaling, the authors argue that it is important to gain a deeper understanding from the user perspective of the most important impacts of the TWT, so that the scaling effort can be focused around delivering those. This will entail further research in three main areas: user preference, cost effectiveness, and safety.
A study conducted in India assessed user perceptions and preferences of TWTs and found that the majority of participants expressed satisfaction with the performance of the system in terms of odour control, ease of use, and the resulting vermicompost (Furlong et al., 2016). However, more research is needed to gather a comprehensive understanding of user satisfaction across different regions and populations, and also over a more extended period of time. Since user perceptions and satisfaction can vary depending on factors such as cultural preferences, local conditions, and access to sanitation alternatives there is a need for user surveys to explore how TWTs impact the users in terms of accessibility, preference, and equitability coupled with measurements of usage over extended time periods.
The cost-effectiveness and affordability of TWTs needs to be explored in more depth, to include whole-life costs. A basic comparative analysis of the cost of TWTs and other toilet options locally available in Sierra Leone showed that TWTs were only slightly more costly than pit latrines to install but presented a clear and significant monetary advantage over time, due to the reduced need for pit emptying services (Sioné et al., 2023). Pit emptying costs and frequency is variable, depending on many factors such as location and diet, however assuming a single professional pit empty costs $44, and that pit latrines need emptying on average every 2 years, a TWT beneficiary would save $132 over an 8-year period (Burt et al., 2019; Mubatsi et al., 2021). On the other hand, a survey by Burt et al. (2019) estimated that only 15% of pit owners have recall to professional services, with others choosing to empty their pits non-professionally, by themselves or even waiting for the pits to visibly overflow before resorting to emptying in order to avoid expenses. The construction costs of a TWT system, excluding economies of scale, also varies and depends on the country of implementation, with estimates ranging from £238 in Myanmar (Oxfam, 2018) to £355 in Liberia based on current experiences (Watako et al., 2016). The costs encompass the labour and material expenses for the superstructure, pan, plumbing, biodigester, inner parts, and effluent collector. The material expenses can be reduced by selecting locally available materials. The cost of worms is not included, and varies depending on whether they are imported, supplied by local businesses or collected by community members locally. For example, in Myanmar the cost of worms is relatively high, due to a government monopoly and a need to fly the worms to the desired site, but this could be reduced if policies were developed to encourage worm businesses (Department of Economic and Social Affairs, 2018).
More data on the potential of TWTs to reduce greenhouse gas emissions compared to pit latrines and septic tanks is required to support the development of favourable policies and to encourage investment in the technology. Beyond that, such data would support the creation of novel financing mechanisms for such projects by participating in carbon markets. The current perspective on pit latrines is that they are a major source of emission of the greenhouse gas methane from the anaerobic decomposition of organic matter in pits (McNicol et al., 2020). Globally the contribution of pit latrines to carbon emissions was estimated at about 0.3% in 2019 (Reid et al., 2014; Van Eekert et al., 2019). In contrast, studies of off-site thermophilic composting of toilet waste–simple composting, without tiger worms–showed that this process, if applied globally, could mitigate 13%–44% of sanitation sector CH4 emissions and highlights the potential that vermicomposting might hold (McNicol et al., 2020). In fact, TWTs could reduce greenhouse gas (methane) emissions compared to latrines and septic tanks, not only due to the high rate of decomposition of the faeces which does not produce methane, but also thanks to the reduced need for further waste management. Used on a larger scale, for example, as part of a waste treatment facility, this could also help combat the environmental impacts of different sanitation technologies, though this needs further study.
Moreover, studies have suggested vermicompost to be a potent, multifunctional soil amendment and fertilizer (Joshi et al., 2015), however questions remain regarding the potential presence of Ascaris eggs. Still, interest in the effects of vermicompost on soil health, plant growth and yield has increased significantly over the last decade (Hussain and Abbasi, 2018). Some studies suggest that vermicompost has higher nutritional value than traditional composts due to the increased rate of mineralization and degree of humification (Sharma et al., 2005). TWTs could therefore contribute to the production of fertilizers or soil conditioners that enable more efficient nutrient utilization by plants, reduced pesticides inputs, soil carbon sequestration and reduced need for transport of global synthetic fertilizers, further reducing GHG emissions from transport. This would also promote a circular economy by favouring resource recovery which, when commercialised, would contribute to abating the cost of TWT implementation. However, there is a need for monitoring TWT systems through the cycle of use and vermicompost removal and potential re-use to confirm that they meet the standards for safely managed sanitation.
The research discussed above provides evidence that TWTs are an affordable, desirable, and safely managed form of on-site sanitation for its target households. Delivering this technology at optimal scale will require a substantial, coordinated effort. The International Worm-Based Sanitation Association (IWBSA: www.iwbsa.org), whose aim is to promote and advance the use of TWTs by sharing knowledge, building networks, and advocating for policy and investment support, is well positioned to lead the research on this, as scaling up the TWT technology will involve funders, policymakers, innovators, and implementers. The latter is likely to include businesses, social enterprises, and NGOs. It may also include grassroots, community-led, self-help organisations such as the women cooperatives involved in maintaining the wormeries for the TWT implementation project in Sierra Leone, led by Oxfam (Sioné et al., 2023). Models for scale could include everything from training local communities through to organic growth of small businesses and multi-stakeholder partnerships. The latter will probably prove to be necessary and building such scaling “systems” is a demanding undertaking in itself (McLean and Gargani, 2019). Whatever the model, a key challenge is building capacity and capability at national and local levels. In terms of capacity, worm supply and logistics is a key requirement and would need to be scaled in most countries, though as mentioned previously, worm supply is unlikely to be a limiting factor (Furlong, et al., 2017b). Capacity in terms of other materials is also unlikely to be an issue, especially if the designs can be adapted to suit large-scale manufacture, but building an integrated supply chain is what will be needed to see this technology to scale.
Building capability is perhaps a greater challenge. Although much of the knowledge of these systems is freely available, and Oxfam in particular has been active in publishing freely available manuals on how to construct TWTs, scaling will require extensive training no matter what model is used (Oxfam, 2018; Oxfam, 2019; Oxfam, 2020). Planning, marketing, distributing, and installing all require people with the right skills and knowledge. Finding the optimal ways to do this effectively is going to be vital, and both innovators and current implementers will have important roles to play. Capability also includes a replicable growth model defining, for example, demand generation and what a large-scale distribution network for components and finished products looks like. For distribution, in some countries it may be possible to “piggyback” on existing networks such as builders’ merchants, but there will be work to be done to integrate this effectively with worm supply. Local “aggregators”, who bring together all the components needed for construction, have proved effective in previous studies of market development in sanitation (Singh et al., 2017).
It is possible that in some settings government regulations may prevent the introduction of new technologies for sanitation, or that approval may need to be sought if they are to be paid for by government subsidy. This is not foreseen to be a major obstacle, as some countries have already included vermicomposting or other forms of ecological sanitation in their strategies, which could potentially include TWT technology. For example, in India, TWTs have already been put forward for use under the Swachh Bharat Mission on the basis of the evidence already available. Other countries have included composting or other forms of ecological sanitation in their strategies, which could potentially include TWT technology, including Uganda (The National Sanitation Task Force, 1997) and Nepal (Government of Nepal, 2011).
So far, local communities, non-governmental organizations (NGOs) and start-up ventures have taken the lead in promoting and implementing support for TWTs. Notably, there have been major efforts in India between Bear Valley Ventures, the Institute for Transformative Technologies and PriMove Infrastructure Development Consultants Pvt Ltd. to commercialise TWTs, with 5000 toilets sold since the first market test in 2015. The venture was also part of the Toilet Board Coalition accelerator programme which helps start-ups to take their technology forward and gained visibility at the Global Innovation Week 2017 (Global Innovation Week, 2017). The UN’s Toilet Accelerator programme, a bespoke mentorship programme pairing sanitation entrepreneurs serving low-income markets with experts and advisors in multinational corporations to offer business learning and enable acceleration to scale, has also been helpful in supporting scaling in TWTs since 2018 (UN Department of Economic and Social Affairs, 2018).
Finally, involving governments, who play a major role in many developing countries in sanitation provision, is essential of course. Efforts should be made to incite government buy-in at national and local/district levels, and this can come from companies but also from NGOs and foreign governments, which can include this in the scope of their foreign policy, as the United Kingdom’s Department for International Development did to stimulate such buy-in in Ghana (Gould and Brown, 2020).
Data availability statement
The original contributions presented in the study are included in the article/Supplementary material, further inquiries can be directed to the corresponding author.
Author contributions
LS: Writing–original draft. WG: Writing–original draft. PB: Writing–original draft. AO: Writing–review and editing. RS: Writing–review and editing. CF: Writing–review and editing. AB: Writing–review and editing. CB: Writing–review and editing. MT: Conceptualization, Supervision, Writing–review and editing.
Funding
The author(s) declare financial support was received for the research, authorship, and/or publication of this article. This project was supported by the Royal Academy of Engineering under the Research Chairs and Senior Research Fellowships programme. Open access publication of this paper was funded by the Bill & Melinda Gates Foundation, who also funded the early development of Tiger Worm Toilets under grant number OPP52641.
Conflict of interest
Author WG was employed by Bear Valley Ventures (United Kingdom). Author PB is employed by Biofiltro (United States). Author AO is employed by TBF Environmental Solutions Pvt. Ltd. (India).
The remaining authors declare that the research was conducted in the absence of any commercial or financial relationships that could be construed as a potential conflict of interest.
Publisher’s note
All claims expressed in this article are solely those of the authors and do not necessarily represent those of their affiliated organizations, or those of the publisher, the editors and the reviewers. Any product that may be evaluated in this article, or claim that may be made by its manufacturer, is not guaranteed or endorsed by the publisher.
References
Burt, Z., Sklar, R., and Murray, A. (2019). Costs and willingness to pay for pit latrine emptying services in kigali, Rwanda. Int. J. Environ. Res. Public Health 16 (23), 4738. doi:10.3390/IJERPH16234738
Department of Economic and Social Affairs (2018). Progress report for Toilet Board Coalition. Avaliable at: https://sdgs.un.org/partnership-progress/partnership-progress-2018-04-26 (Retrieved June 11, 2023).
Eastman, B. R., Kane, P. N., Edwards, C. A., Trytek, L., Gunadi, B., Stermer, A. L., et al. (2001). The effectiveness of vermiculture in human pathogen reduction for USEPA biosolids stabilization. Compost Sci. Util. 9 (1), 38–49. doi:10.1080/1065657X.2001.10702015
Edwards, C. A., Clive, A., Arancon, N. Q., and Sherman, R. L. (2010). Vermiculture technology: earthworms, organic wastes, and environmental management. CRC Press.
Engineering for Change (2023). Tiger toilet. Avaliable at: https://www.engineeringforchange.org/solutions/product/tiger-toilet/ (Accessed May 30, 2023).
Furlong, C. (2014). Worm Farming in South Africa: assessing the potential to supply composting worms for the humanitarian sector in Africa. IWA Publishing.
Furlong, C., Gibson, W. T., Oak, A., Thakar, G., Kodgire, M., and Patankar, R. (2016). Technical and user evaluation of a novel worm-based, on-site sanitation system in rural India. Waterlines 35 (2), 148–162. doi:10.3362/1756-3488.2016.013
Furlong, C., Lamb, J., and Bastable, A. (2017a). “Learning from oxfam’s tiger worm toilets projects,” in 40th WEDC international conference (Loughborough, UK: WEDC).
Furlong, C., Rajapaksha, N. S., Butt, K. R., and Gibson, W. T. (2017b). Is composting worm availability the main barrier to large-scale adoption of worm-based organic waste processing technologies? J. Clean. Prod. 164, 1026–1033. doi:10.1016/J.JCLEPRO.2017.06.226
Furlong, C., Templeton, M. R., and Gibson, W. T. (2014). Processing of human faeces by wet vermifiltration for improved on-site sanitation. J. Water Sanitation Hyg. Dev. 4 (2), 231–239. doi:10.2166/WASHDEV.2014.107
Global Innovation Week (2017). Bear Valley ventures: vermifiltration-based tiger toilet innovations. Avaliable at: https://giw2017.org/innovations/bear-valley-ventures-vermifiltration-based-tiger-toilet.
Gould, C., and Brown, C. (2020). The sanitation challenge for Ghana: making urban sanitation a political priority. Avaliable at: https://assets.publishing.service.gov.uk/media/5eba7e8d86650c2791ec710d/Sanitation_Challenge_for_Ghana_summary.pdf.
Government of Nepal (2011). Sanitation and hygiene master plan: steering committee for national sanitation action 2011. Government of Nepal.
Hussain, N., and Abbasi, S. A. (2018). Efficacy of the vermicomposts of different organic wastes as “clean” fertilizers: state-of-the-art. Sustain. Switz. 10 (4), 1205. doi:10.3390/su10041205
Hylton, E., Noad, L., Templeton, M. R., and Sule, M. N. (2022). The rate of vermi-compost accumulation within ‘Tiger Toilets’ in India. Environ. Technol. (United Kingdom) 43, 376–385. doi:10.1080/09593330.2020.1789750
Islam, M. S., Mahmud, Z. H., Islam, M. S., Saha, G. C., Zahid, A., Ali, A. Z., et al. (2016). Safe distances between groundwater-based water wells and pit latrines at different hydrogeological conditions in the Ganges Atrai floodplains of Bangladesh. J. Health, Popul. Nutr. 35 (1), 26. doi:10.1186/s41043-016-0063-z
Joshi, R., Singh, J., and Vig, A. P. (2015). Vermicompost as an effective organic fertilizer and biocontrol agent: effect on growth, yield and quality of plants. Rev. Environ. Sci. Biotechnol. 14 (1), 137–159. doi:10.1007/s11157-014-9347-1
McLean, R., and Gargani, J. (2019). Scaling impact: innovation for the public good. Routledge, IDRC. Avaliable at: https://idl-bnc-idrc.dspacedirect.org/bitstream/handle/10625/57605/Scaling%20impact.pdf?sequence=2&isAllowed=y.
McNicol, G., Jeliazovski, J., François, J. J., Kramer, S., and Ryals, R. (2020). Climate change mitigation potential in sanitation via off-site composting of human waste. Nat. Clim. Change 10 (6), 545–549. doi:10.1038/S41558-020-0782-4
Mubatsi, J. B., Wafula, S. T., Etajak, S., Ssekamatte, T., Isunju, J. B., Kimbugwe, C., et al. (2021). Latrine characteristics and maintenance practices associated with pit latrine lifetime in an informal settlement in kampala, Uganda. J. Water Sanitation Hyg. Dev. 11 (4), 657–667. doi:10.2166/washdev.2021.032
Oxfam (2018). Tiger worm toilet manual: globally relevant learnings from Myanmar. Avaliable at: https://www.researchgate.net/publication/335749958_Tiger_Worm_Toilet_Manual.
Oxfam (2019). Design, construction and maintenance of a tiger worm toilet. Avaliable at: http://agracultura.org/wp-content/uploads/2019/12/Tiger-worm-toilet.pdf.
Oxfam (2020). Tiger worm toilets: design manual. Avaliable at: https://www.elrha.org/wp-content/uploads/2020/11/TWT-Manual-Different-designs-PART-2.pdf.
Reid, M. C., Guan, K., Wagner, F., and Mauzerall, D. L. (2014). Global methane emissions from pit latrines. Environ. Sci. Technol. 48 (15), 8727–8734. doi:10.1021/es501549h
Rose, C., Parker, A., Jefferson, B., and Cartmell, E. (2015). The characterization of feces and urine: a review of the literature to inform advanced treatment technology. Crit. Rev. Environ. Sci. Technol. 45 (17), 1827–1879. doi:10.1080/10643389.2014.1000761
Sharma, S., Pradhan, K., Satya, S., and Vasudevan, P. (2005). Potentiality of earthworms for waste management and in other uses-A review. J. Am. Sci. 1, 1.
Singh, S., Singh, A., Sharma, V., and Sinha, B. (2017). Building a sustainable market for toilets: lessons learned from rural Bihar. India: Nova Publishers.
Sinha, R., Valani, D., Soni, B., and Valani, D. (2011). Earthworm vermicompost: a sustainable alternative to chemical fertilizers for organic farming. Nova Publishers.
Sioné, L., Bastable, A., Ampilan, A., and Templeton, M. R. (2023). Lessons learned from a tiger worm toilet implementation project in Sierra Leone. J. Water 00, 604–609. doi:10.2166/WASHDEV.2023.134
The National Sanitation Task Force (1997). The national sanitation policy for Uganda. Government of Uganda.
UN Department of Economic and Social Affairs (2018). Progress report for toilet board coalition partnership progress.
Van Eekert, M. H. A., Gibson, W. T., Torondel, B., Abilahi, F., Liseki, B., Schuman, E., et al. (2019). Anaerobic digestion is the dominant pathway for pit latrine decomposition and is limited by intrinsic factors. Water Sci. Technol. 79 (12), 2242–2250. doi:10.2166/wst.2019.220
Keywords: tiger worm toilet, vermicompost, vermifiltration, on-site sanitation, faecal sludge
Citation: Sioné L, Gibson W, Beckett P, Oak A, Sathre R, Furlong C, Bastable A, Batarda C and Templeton MR (2023) Taking Tiger Worm Toilets to scale: opportunities and challenges. Front. Environ. Sci. 11:1278087. doi: 10.3389/fenvs.2023.1278087
Received: 15 August 2023; Accepted: 16 October 2023;
Published: 02 November 2023.
Edited by:
Maria Elisa Magri, Federal University of Santa Catarina, BrazilReviewed by:
Chidozie Charles Nnaji, University of Nigeria, Nsukka, NigeriaCopyright © 2023 Sioné, Gibson, Beckett, Oak, Sathre, Furlong, Bastable, Batarda and Templeton. This is an open-access article distributed under the terms of the Creative Commons Attribution License (CC BY). The use, distribution or reproduction in other forums is permitted, provided the original author(s) and the copyright owner(s) are credited and that the original publication in this journal is cited, in accordance with accepted academic practice. No use, distribution or reproduction is permitted which does not comply with these terms.
*Correspondence: Laure Sioné, bGF1cmUuc2lvbmUwOUBpbXBlcmlhbC5hYy51aw==