Assessing biodiversity and regulatory ecosystem services in urban water bodies which serve as aqua-Nature-based Solutions
- 1Department of Biology, University of Antwerp, ECOSPHERE Research Group, Antwerp, Belgium
- 2Leibniz-Institute of Freshwater Ecology and Inland Fisheries, Berlin, Germany
- 3Geography Institute and IRI THESys, Humboldt University of Berlin, Berlin, Germany
In sight of a growing urban population and intensified extreme weather events, cities must integrate in their urban planning elements to both reduce their impact (i.e., air and water pollution, degradation of habitats, loss of biodiversity) and increase their resilience to climate change. In contrast to engineering solutions, which normally not only fail to adequately address these issues but often also exacerbate them, Nature-based Solutions are an efficient strategy which can help cities become more sustainable. Aqua-Nature-based Solutions (aNBS) tackle water-related hazards by enhancing water regulation and mitigating flood and drought impacts. However, under a warming climate, aNBS are expected to often dry-out, changing biodiversity and the ecosystem services they support. The aim of this study is to compare the biodiversity of temporarily and permanently wet urban waterbodies which function as aNBS. We selected two pond complexes with different hydroperiod (i.e., different duration, amplitude and frequency of inundation) and studied the riparian vegetation and aquatic macroinvertebrate biodiversity. The Multimetric Macroinvertebrate Index of Flanders was used to determine the macroinvertebrate biodiversity and to assess water quality of the ponds. Using water stable isotopes and piezometers, the hydrological dynamics were studied in order to identify the water regulating ecosystem services these ponds deliver. The results showed that the selected pond complexes have a high plant biodiversity, particularly in temporary ponds. Water quality ranged from moderate to poor and macroinvertebrate biodiversity tended to be greater in permanent ponds. Plant and macroinvertebrate alien species were also found in the aNBS. Regarding water regulating ecosystem services, the pond complexes enhanced infiltration and groundwater recharge, providing resilience to both flooding and drought. Our findings corroborate previous studies on the need of diversifying urban ponds’ hydroperiod to support biodiversity. Thus, integrating well-designed aNBS into urban planning might be a way to make cities more resilient to water climate-related hazards while enhancing biodiversity.
1 Introduction
It is projected that by 2050 more than two-thirds of the world’s population will live in urban areas (Oertli and Parris, 2019). Built-up space is also expected to triple in surface (Hill et al., 2017). Urbanization creates a diversity of ecological problems including air and water pollution, the degradation and fragmentation of habitats and biodiversity loss, which are exacerbated by an increasingly altered climate and intensified natural disasters. Prolonged periods of drought and intense precipitation are occurring with increasing frequency, posing a significant risk to both water and food security worldwide. In cities, runoff water flows over impervious and compacted surfaces, but also over permeable surfaces which become saturated, increasing flood risks (Oswald et al., 2023).
In addition, the annual worldwide withdrawal of freshwater, estimated more than 10,000 km3 per year (Albert et al., 2021), and the unproper release of 48% of this volume back to the environment as untreated wastewater (Jones et al., 2020), result in declines of groundwater levels and in the degradation of aquatic ecosystems and water resources, respectively (Stefanakis et al., 2021). Freshwater ecosystems are particularly suffering from biodiversity loss due to the altered climate, land use change and unsustainable management practices (Tickner et al., 2020). The UN Global Biodiversity outlook (Secretariat of the Convention on Biological Diversity, 2020) and the Global Assessment Report on Biodiversity and Ecosystem Services by the Intergovernmental Science-Policy Platform on Biodiversity and Ecosystem Services (Brondizio et al., 2019) have claimed that we are failing to decelerate the rate of biodiversity decline and ecosystem degradation. As water is a key factor in the climate crisis, introducing elements in the urban plan which improve water quality and use-efficiency while protecting biodiversity and restoring aquatic ecosystems is of foremost importance.
Nature-based Solutions (NBS) can be used as a tool to create greener and more sustainable cities and help tackle the aforementioned issues (Faivre et al., 2017). The concept of NBS was coined for the first time in 2015 by the European Commission, which defined them as actions inspired by, supported by, or copied from nature, that address environmental, social and economic challenges simultaneously by maximizing the benefits provided by nature (European Commission, 2015). Introducing NBS in urban areas can not only increase the value of ecosystems, but also improve human wellbeing and make cities more climate resilient.
Blue infrastructure, also called aqua-Nature-based Solutions (aNBS), would not only address water-related problems in a sustainable and cost-effective way, but would also provide other ecosystem services (ESS) such as recreation (Ncube and Arthur, 2021), the attenuation of the urban heat island effect via evaporation and shading (Maggiotto et al., 2021), and the enhancement of biodiversity (Oral et al., 2020). Grey infrastructure (e.g., drains, gutters) is often used to counteract disturbances in the water systems (Krauze and Wagner, 2019), but evidence suggest that it leads to the overuse and mismanagement of water (Guerrero et al., 2018), the disturbance of the natural water cycle (Vörösmarty et al., 2010), the enhancement of the urban heat island effect (Stefankis et al., 2021) and the contamination of stormwater and reduction of ESS (e.g., water infiltration and retention) (Prudencio and Null, 2018).
While aNBS offer various ESS, water regulatory ESS are the foundation for a resilient ecosystem, capable of adjusting to extreme precipitation events and droughts. These ESS include water infiltration, retention, interception and evaporation as well as the minimisation of surface run-off. Evidence of how aNBS can help cities increase their resilience to water-related problems was shown in De Vleeschauwer et al. (2014). Rainfall simulations were modelled in the city of Turnhout (Belgium), revealing that if only 1% of the total city runoff area was a combination of open green zones and stormwater retention and infiltration infrastructure, the sewer flood volumes would decrease by 30%–50% for return periods between 10 and 100 years. The support of regulatory ESS is therefore essential for safeguarding freshwater ecosystems functioning and their adaptation to hydrological stress.
Biodiversity is essential for the correct functioning of the ecosystem and the provision of regulating services. Plant roots can decrease soil sealing and enhance water infiltration during intense rainfall events, preventing rapid overland flow (Smith et al., 2015). In fact, it has been shown that hydraulic conductivity is two to four times higher in vegetated areas than in bare ones (Jean-Philippe et al., 2021). In addition, vegetation increases organic carbon in the system and therefore the clay fraction of the soil, which can slow down water drainage and filter water more effectively. Other examples of regulating ESS are the natural water purification by plants (Durance et al., 2016). Despite the influence of biodiversity on ESS, anthropogenic activities together with climate change are contributing to an accelerating biodiversity loss, especially in freshwater ecosystems (Tickner et al., 2020).
The most recent guidelines for the implementation of NBS highlight biodiversity conservation not only as an objective but as a pre-requisite (Faivre et al., 2017; IUCN, 2020; Pettorelli et al., 2021; Seddon et al., 2021). However, previous research found that only 351 out of 976 NBS projects in European cities have explicit biodiversity goals and actions (Xie et al., 2022). In urban freshwater bodies, water purification, flood control, leisure activities or aesthetic value are often prioritized, whilst biodiversity is commonly disregarded. Thus, the conservation of NBS does not always imply the conservation of biodiversity if other ESS are favoured over it. There should be a recognition of the importance of biodiversity as way to support and provide ESS rather than considering it a competitor of the delivery of other ESS.
Blue infrastructure in the urban matrix can provide opportunities to support biodiversity. For instance, a network of small waterbodies can support regional and global biodiversity by favouring the connection with rural habitats (directly or as stepping stones or corridors) and acting as a reservoir for threatened species (Hill et al., 2017). However, unproper management can lead to population extinctions, and the fragmentation of pond networks (i.e., by large roads or long and high buildings) can decrease the movement of species and biologically isolate them. Both in management and in urban planning, emphasis should be put on promoting freshwater ecosystems as habitats for biodiversity and on enhancing their connectivity (e.g., by increasing pond density) in order to create a more climate-resilient system that, with correct management practices, can support biodiversity while also providing other targeted services such as human health and climate change adaptation (Xie et al., 2022).
When aiming to increase our understanding of the effects of aNBS, one needs to consider the hydrological regime of the blue water systems, which includes the duration, amplitude and frequency of inundation of water bodies (Oertli and Parris, 2019). These are often classified as temporary and permanent. Temporary water bodies are defined as periodically or seasonally filled with water, becoming dry during certain periods of the year. They are often reliant on rainfall events or melting snow. Permanent water bodies maintain a consistent water presence throughout the year, regardless of seasonal changes, providing a stable and predictable environment for aquatic organisms. The integration of piezometer data based on groundwater levels together with water stable isotopes can reveal insights into water dynamics, sources and pathways into freshwater bodies (Tetzlaff et al., 2015; Kuhlemann et al., 2022). In particular, water isotopes - that occur naturally in the water cycle and behave conservatively as water moves through a catchment - are useful tools to understand water cycling, partitioning, storage and losses.
Since 2014, the European Commission has developed different programs for the implementation of NBS in cities. Research is needed to promote a systemic adoption of NBS and demonstrate with large-scale projects the benefits of their introduction in the urban planning. This study has the urgency for the adoption of NBS as urban aquatic bodies at its basis, not only for the provisioning of services to mitigate the impacts of natural water-related hazards and climate change, but also for their contribution to biodiversity. Despite the many advantages of NBS, their implementation has been rather limited (Stefanakis et al., 2021). This research provides evidence of how NBS support biodiversity in urban blue ecosystems while delivering water regulation ESS. In this paper, the studied NBS are blue infrastructures (urban ponds) which, according to Eggermont et al. (2015)’s classification of NBS, fall into the category of extensively and intrusively managed ecosystems, including those artificially created. Different freshwater bodies embedded in the municipality of Antwerp (Belgium) were selected and the biodiversity of plants and macroinvertebrates was studied. In sight of the increasing drought periods that cities will face, temporary and permanent ponds were chosen to analyse how biodiversity could shift under a warming climate. Other ESS such as groundwater recharge and infiltration were analysed as well to see the effectiveness of the chosen aNBS on the regulation of water dynamics. The specific objectives were to prove that 1) aNBS have a potential to increase biodiversity and that 2) the hydroperiod (i.e., temporal and permanent ponds) would affect species diversity and therefore the ecosystem services they support.
2 Methods
2.1 Site description
The study sites are located in the municipality of Antwerp in the Flemish region of Belgium (Figure 1). Antwerp is located in a lowland area of sandy loam soils (Willemen, 2023). Despite the high population density in this area, blue spaces are abundant. This is due its low-altitude and to the abundant rainfall of the region (852.9 mm per year) which is captured by the floodplain, and the low annual mean temperature (11.1°C) (collected data from 1981 to 2010, meteo. be).
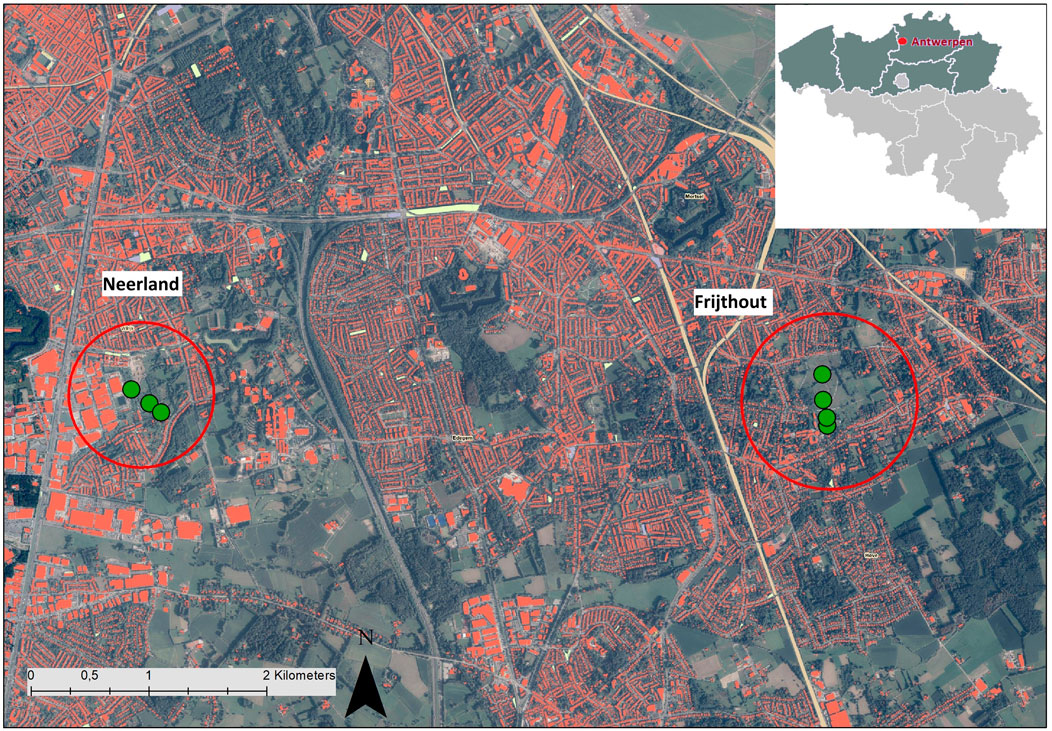
FIGURE 1. Selection of the selected sites. The map represent the built areas (orange) and green areas (green) of the urban periphery of Antwerp.The red circles indicate the two main areas of study (Neerland and Frijthout ). The green dots represent the location of each of the eight selected ponds. Three ponds were selected in Neerland Park and four in Frijthout Park.
Frijthout Park and Neerland Park are recently designed parks developed as aNBS. The main purpose of the construction of these recreational sites was to create water rich parks which collected and infiltrated runoff water while caring for aesthetical quality and biodiversity. These two pond complexes with independent catchment sources and different hydroperiod were chosen as study sites. Seven ponds were studied in total: four in Frijthout Park and three in Neerland Park. The criteria for the classification of the waterbodies are shown in Table 1.
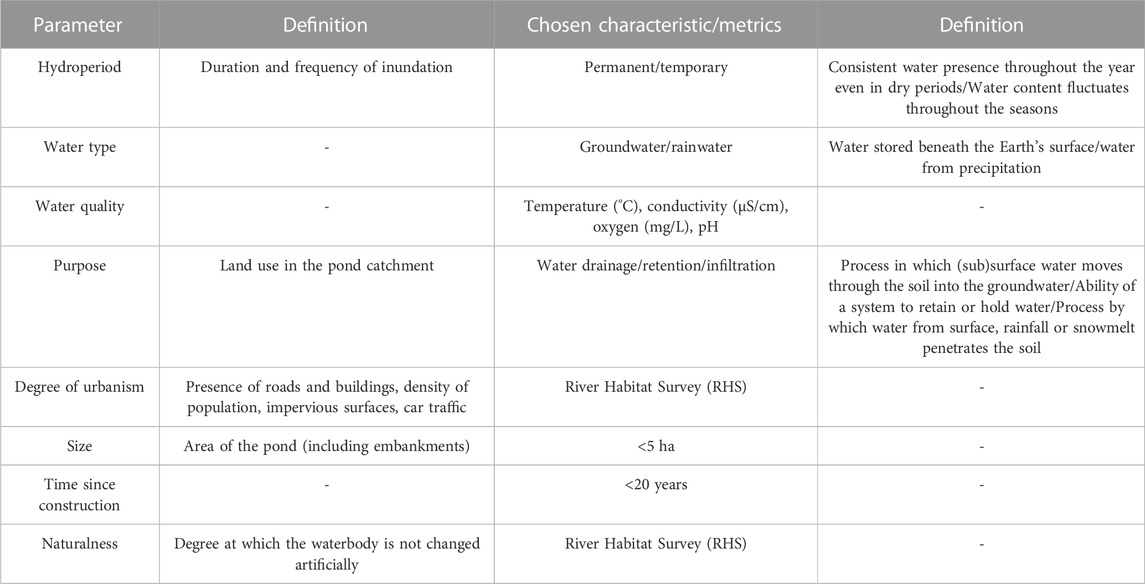
TABLE 1. Criteria for the classification of the selected waterbodies. The parameters that were chosen to classify the waterbodies: hydroperiod, water type, water quality, purpose, degree of urbanism, size, time since construction and naturalness.
2.2 Biodiversity determination
Data collection for plants and macroinvertebrates was performed using methodologies employed by previous studies (Gabriels et al., 2010; Nunes et al., 2015). The habitat quality and naturalness were analysed following a short version of the guideline of the River Habitat Survey (Supplementary Table S1A).
2.2.1 Vegetation biodiversity
Plant relevés were performed in September 2022 using the point-intercept method (Gabriels et al., 2010). Riparian plants were identified to the species level. 20 m linear transects were positioned on the pond margins (either horizontally placed in respect to the pond banks or along the shore of the pond) in which one point every 0.5 m (40 points) was taken (Figure 2). Three transects were studied per pond. Plant species abundance was studied by counting the number of times a species was found in the 20 m linear transect. Species occurrence was then calculated by dividing the abundance of each species by the total number of points taken and expressed in percentage (Supplementary Table S2A).
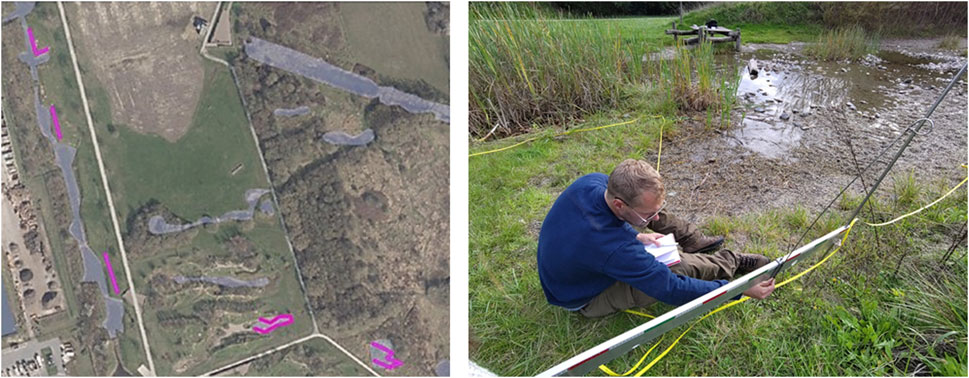
FIGURE 2. Vegetation relevés. (A) Example of transect placement in Neerland Park. (B) Demonstration of the point-intercept method procedure. Every 50 cm along the 20 m linear transect, plants which were intercepted by a pointer placed forming a 90° angle with the ground were identified to the species level.
Variation in plant taxonomic richness between sites was studied by performing a statistical t-test for plant abundance and a Kruskal–Wallis test for occurrence in R Studio 4.3.1. This latter is a non-parametric test, chosen due to the unnormal distribution of the occurrence data.
The Shannon-Wiener Index was also calculated for determining plant biodiversity (Begossi, 1996).
2.2.2 Macroinvertebrate biodiversity and water quality
2.2.2.1 Sampling and identification
Macroinvertebrate kick net sampling was performed in October and November 2022 according to Gabriels et al. (2010). This method was developed for the determination of the water quality and the evaluation of macroinvertebrate biodiversity through the calculation of the biotic index Multimetric Macro-invertebrate Index Flanders (MMIF). The index calculation is a type-specific multimetric system based on five equally weighted metrics, which are taxa richness, number of Ephemeroptera, Plecoptera and Trichoptera taxa, number of other sensitive taxa, the Shannon–Wiener diversity index and the mean tolerance score (Gabriels et al., 2010).
The identification was performed immediately upon arrival in the laboratory after the sampling. The bottom of a sorting tray was divided into squares to facilitate the systematic examination of the collected material. After the sorting of the organisms, macroinvertebrates were transferred to plastic bottles and preserved with ethanol 70%. Identification of the selected taxonomic groups (Table 2) took place using a BX50 microscope (Olympus) and SteREO Discovery V12 optical-light microscope (ZEISS) using the handbook by De Pauw and Vanhooren. (1983) as a guideline. Macroinvertebrates were identified either to the genus or family level, depending on the requirements of the MMIF for each species (Table 2). For Chironomidae, macroinvertebrates were divided further into the group of thummi-plumosus and non-thummi-plumosus due to their different tolerance to pollution. Importantly, a species represented in the sample by only a single individual was not considered in the calculation of the biotic index; as Verneaux and Tuffery (1968) remark its occurrence could be accidental.
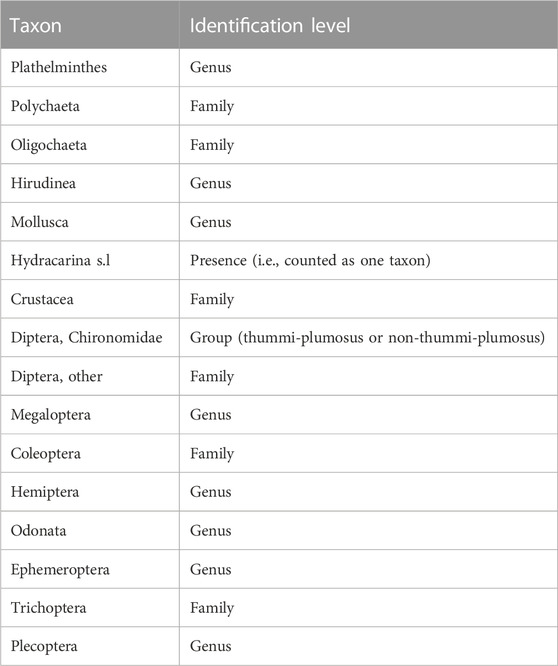
TABLE 2. Taxonomic identification level of macroinvertebrates. Retrieved from (Gabriels et al., 2010).
2.2.2.2 MMIF calculation
The MMIF was used to assess both the quality of the water and the macroinvertebrate biodiversity of the selected sites. The MMIF uses macroinvertebrates as indicators of water and ecosystem quality status based on the assumption that ecological integrity would be reduced in a disturbed system. Environmental data of the water (i.e., temperature, pH, conductivity, oxygen) were recorded with a multimeter (WTW Multi 3430 SET F) after the collection of macroinvertebrates to assess the water quality. The selected ponds were classified according to these features into alkaline (A) when pH ≥ 7.5, and circumneutral (C) when 7.5 < pH ≥ 6.5 according to Gabriels et al. (2010) classification. Acid ponds (pH < 6.5) are not present in the study area. The alkalinity classification of the sites and their abiotic values are shown in Supplementary Table S3A.
The index compares the observations to reference conditions of good water status using the Ecological Quality Ratio (EQR) and divides the water bodies into five categories which reflect a bad (0.00–0.19), poor (0.20–0.39), moderate (0.40–0.59), good (0.60–0.79) and high (0.80–1.00) water quality status.
To study if the sites differed significantly in the MMIF a t-test was performed in RStudio 4.3.1.
2.3 Hydrological characterisation
Piezometers with groundwater level divers were installed in a straight transect which followed the topography at 5 locations in each park in February 2023 (Figure 1A; Supplementary Figure S2A). Two of them were installed inside the ponds Neerland 1 and Neerland 3. Groundwater level at each site was measured hourly with a Rugged TROLL Data Logger 100 (662,226) (accuracy >0.1%; United States) with external correction for atmospheric pressure. Groundwater levels are expressed in reference to the water at the sea level (TAW) and represented as meters above this reference level (mTAW).
Groundwater piezometers and surface water ponds were sampled and analysed for water isotope composition. Liquid reference samples were taken from a rain collection device installed at the Antwerp University campus of Drie Eiken (51.155,620, 4.408,859) and protected from evaporation. Samples were membrane filtered (0.2 μm cellulose acetate) into 1.5 mL vials (LLG Labware) and sent to the Leibniz Institute of Freshwater Ecology and Inland Fisheries (IGB) in Berlin for isotope analyses. The ratio of the heavy stable isotopes of oxygen (18O) and hydrogen (2H) were analysed using cavity ringdown spectroscopy (Picarro L-2130i, Picarro Inc., CA, United States) relative to the Vienna Standard Mean Ocean Water (VSMOW) isotope standard. After quality-checking, the averaged results for each sample were expressed in δ-notation and reported on the VSMOW scale. Analytical precision was 0.04‰ standard deviation (SD) for δ18O and 0.14‰ for δ2H.
The combined information from the piezometer water dynamics and the isotope composition was interpreted to reveal the water source of the ponds (i.e., rain, ground water, mixed).
3 Results
3.1 Biodiversity determination
3.1.1 Vegetation biodiversity
Significant differences in the plant species abundance between hydroperiod (p-value = 0.0013) were found where temporary ponds had an average of 10.20 ± 2.69 more species than those with larger hydroperiod (Figure 3). However, the occurrence of species was not different between sites (p-value = 0.6106). These findings suggest that temporary waterbodies have higher plant biodiversity, although in both temporary and permanent ponds individual species were found in the same ratio compared to the total number of species.
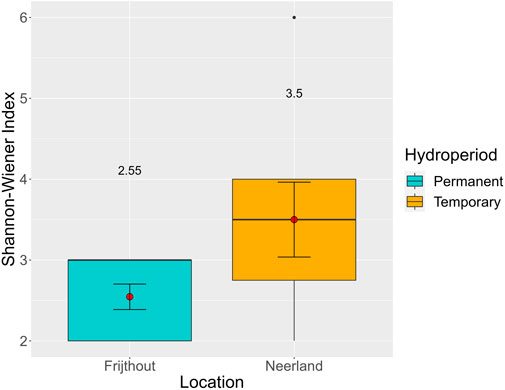
FIGURE 3. Average plant species abundance in each aNBS. Permanent (blue) and temporary (orange) aNBS are shown separately for Frijthout (n = 480) and Neerland (n = 360). The average species abundance is represented by a red dot. The standard error bars and median line are also shown.
The Shannon-Wiener Index scores were 2.55 for Frijthout Park and 3.50 for Neerland Park. Generally, a Shannon-Wiener Index greater than 2 indicates a relatively high level of biodiversity, therefore, our results suggest that the studied aNBS have a high plant biodiversity. Interestingly, between the two parks, temporary ponds showed a Shannon Index of 0.95 ± 0.43 units higher than the permanent ponds (p-value = 0.0018) (Figure 4).
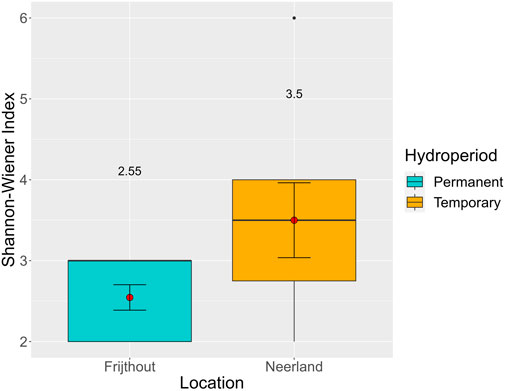
FIGURE 4. Average Shannon-Wiener Index in each aNBS. Permanent (blue) and temporary (orange) aNBS are shown separately for Frijthout (n = 480) and Neerland (n = 360). The average Shannon-Wiener Index is represented by a red dot. The standard error bars and median line are also shown.
In addition, two alien plant species, allochtonous from the United States and South Canada, were observed in the ponds of Frijthout Park, namely, Eleocharis engelmannii and Carex scoparia.
3.1.2 Macroinvertebrate biodiversity and water quality
When measuring the abiotic parameters of the ponds, the oxygen value in pond Neerland 3 was 3.74 ± 1.03 mg O2/L, thus on the verge of hypoxia (Supplementary Table S3A). The rest of the ponds showed oxygen levels above 5 mg/l.
The obtained values of the MMIF calculation are shown in Table 3. Moderate to poor water quality was observed in the water rich parks, suggesting a low ecological quality probably due to their urban location. However, differences in the MMIF between hydroperiod could not be observed (p-value = 0.637) (Figure 5).
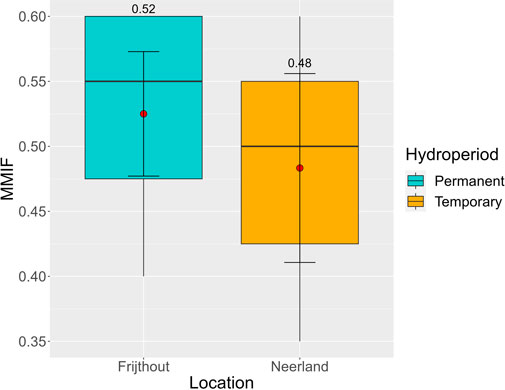
FIGURE 5. Average MMIF in each aNBS. Permanent (blue) and temporary (orange) aNBS are shown separately for Frijthout (n = 4) and Neerland (n = 3). The average MMIF is represented by a red dot. The standard error bars and median line are also shown.
One alien coleoptera species (Proasellus coxalis) allochthonous from the Mediterranean region was found in Neerland Park.
3.2 Hydrological dynamics and patterns
In Frijthout Park, groundwater levels were 0.95 mTAW (piezometer 1), 0.25 mTAW (piezometer 2), 0.80 mTAW (piezometer 3), 0.22 mTAW (piezometer 4), and 0.26 mTAW (piezometer 5) In Neerland Park, groundwater levels were 0.06 mTAW (piezometer 2), 0.02 mTAW (piezometer 3) and 0.07 mTAW (piezometer 4) (Supplementary Figure S3A, S4A).
The data from the piezometers 1, 2 and 3 Frijthout Park showed a similar pattern (Figure 6). February 2023 was an extremely dry month (<9 mm), with very few precipitation events. During the preceding 3 months, the total precipitation was approximately 260 mm. Therefore, we can assume that groundwater levels would have been normal for the time of the year. The period in February without precipitation and low evapotranspiration provided excellent conditions to analyse spatial and temporal responses of the water system. March was again a wet month (118 mm), which allowed us to see the response of the system to precipitation surplus. From the 24th of February to the second of March, the groundwater recession was on average 0.013 ± 0.008 m per day. In the beginning of March, a rainfall event took place from the fifth to the 10th of March (approx. 55 mm of precipitation in 5 days). The average daily increase in the groundwater level during this rainfall event recorded by piezometers 1, 2 and 3 was 0.149 ± 0.015 m per day. The piezometer 5 in Frijthout Park showed a different, more dynamic pattern with a groundwater increase after the rainfall of the fifth to the 10th of March of 0.450 m, and a recession rate of 0.08 m per day during the selected days before the precipitation event.
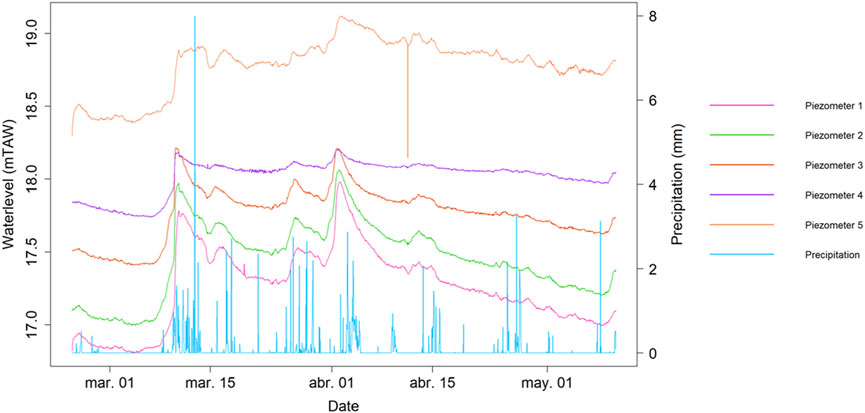
FIGURE 6. Frijthout Park piezometer data for the months of February to May. Collected data for the piezometers in Frijthout Park is shown. Data is shown in pink, green, red, purple and orange for piezometers 1, 2, 3, 4 and 5, respectively. Precipitation data is shown in blue. The groundwater levels are shown taking as reference the water at the sea level (TAW) and represented as meters above this reference level (mTAW). There is a sudden drop in piezometer 5 data on the 12th of April due to vandalism.
Piezometers 2, 3 and 4 at Neerland Park showed fluctuating patterns particularly piezometers 2 and 4 (Figure 7). As in Frijthout Park, the precipitation event in March increased groundwater levels on average 0.150 ± 0.04 m increase per day. The recession was 0.005 ± 0.0001 m per day before the first rains in March. The piezometers installed in the ponds of Neerland 1 (piezometer 1) and Neerland 3 (piezometer 5) showed water fluctuations of the ponds (Figure 8). For Neerland 1, which is located in the infiltration zone (19.387 mTAW), water fluctuations followed precipitation events, although dampened in their response. Neerland 3, however, had steeper peaks. Neerland 3 pond is located much lower in the landscape (9.92 mTAW) and infiltration cannot take place due to the shallow ground water levels.
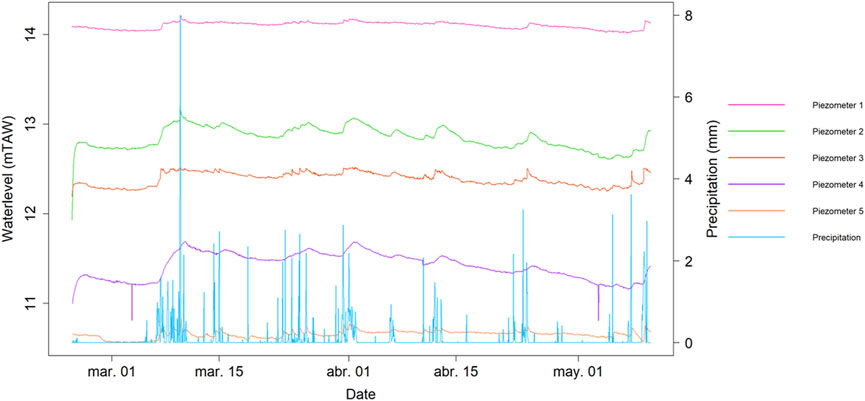
FIGURE 7. Neerland Park piezometer data for the months of February to May. Collected data for the piezometers in Neerland Park is shown. Data is shown in blue, orange, green, red and purple for piezometers 1, 2, 3, 4 and 5, respectively. The groundwater levels are shown taking as reference the water at the sea level (TAW) and represented as meters above this reference level (mTAW). Piezometers 2, 3 and 4 show initially a steep increase as in at that time groundwater was extracted for the isotope analysis. There are two sudden drops on the piezometer 4 due to vandalism.
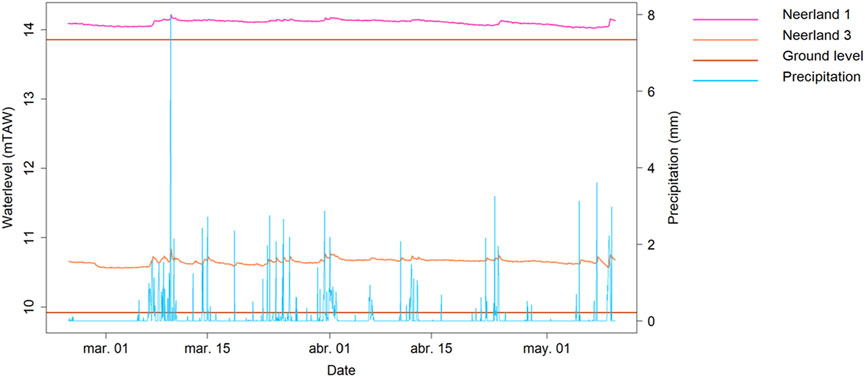
FIGURE 8. Neerland Park piezometer data of Neerland ponds 1 and 3. Ground level (orange) and groundwater level in meters taken as reference the sea level (TAW) (mTAW) (blue) are shown for the ten different piezometers in Frijhout during the time of the study.
Isotope data plotted in dual isotope space (Figure 9) revealed noticeable differences among ponds and across seasons. Data showed a clear distinction between spring and winter samples with warmer periods more enriched (less negative isotopic values) than those in the wet period due to evaporation fractionation processes. The allocated water source of each pond of the different sites are listed in Table 4.
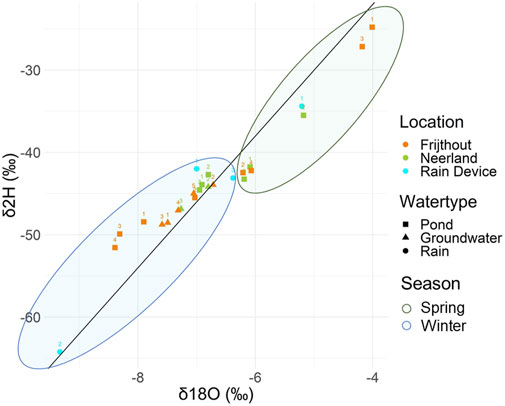
FIGURE 9. Dual isotope plot. Oxygen isotopes (18O) and hydrogen isotopes (2H) are plotted as permill (‰). Data is arranged by location (Frijthout = orange; Neerland = green; Rain Device = blue), watertype (Pond = square, Groundwater = triangle, Rain = circle) and season (Spring = green circle; Winter = blue circle). Each dot has a number above indicating the number of the piezometer labelling shown in Supplementary Figure S1A and Supplementary Figure S2A in the Supplementary Material. The Global Meteoric Water Line (GMWL) is shown as a solid black line.
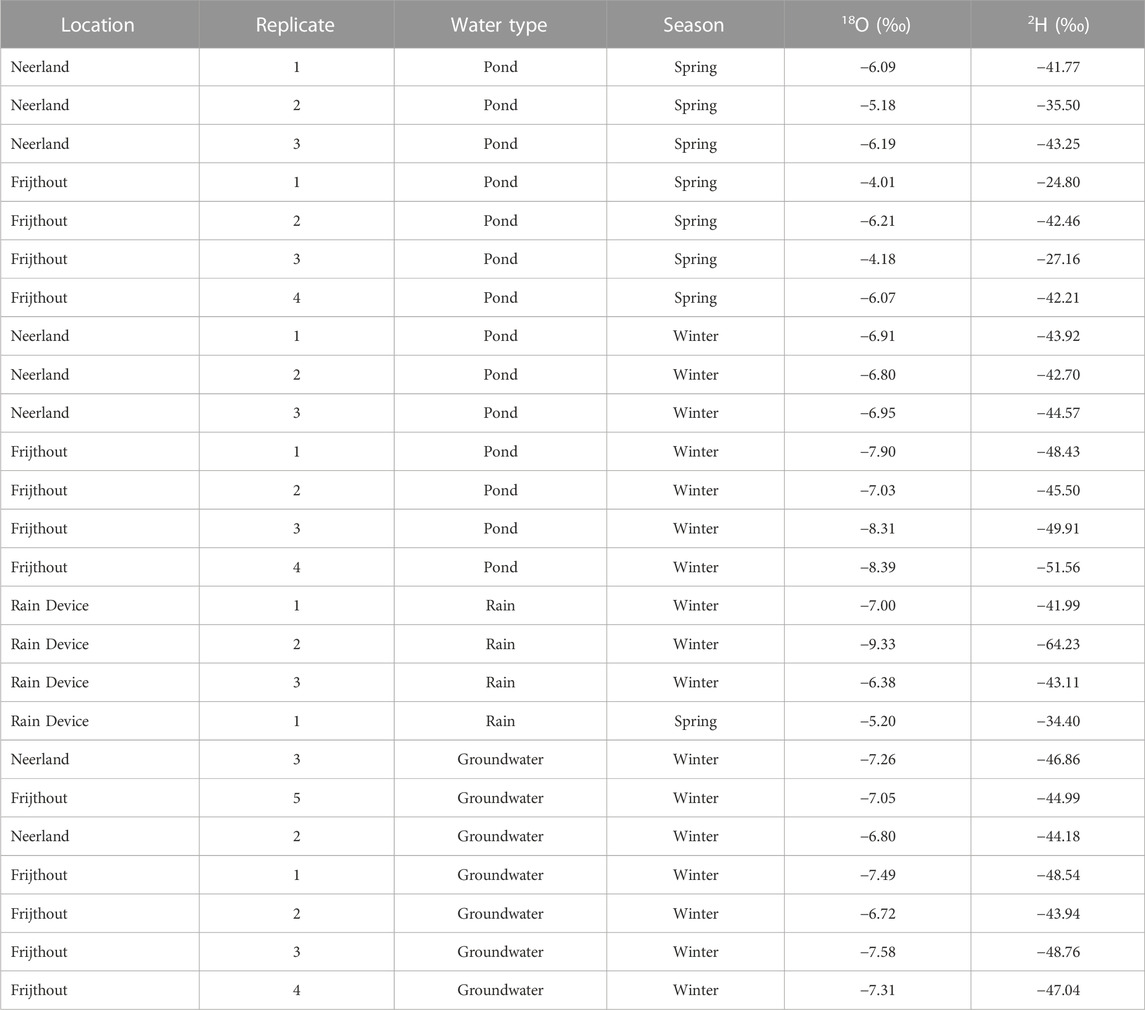
TABLE 4. Summary of water isotope data. Ponds were classified according to their location (Neerland, Frijthout and Rain Device), the water type (pond, rain or groundwater) and season (spring or winter).
When rainwater infiltrates into the ground and recharges groundwater, it carries its isotopic signature with it. Therefore, the isotopic values of 18O and 2H in groundwater are often reflective of the local precipitation from which it was derived. However, during infiltration and subsequent movement through the subsurface, additional processes like mixing with other water sources, evaporation, and isotopic fractionation can occur, altering the isotopic composition of the groundwater (Bowen et al., 2019).
Groundwater, being a more stable and long-term reservoir, tends to exhibit more negative isotopic values compared to rainwater due to these processes. Thus, groundwater-fed ponds have less negative values of heavy isotopes compared to rain-fed ponds. The four ponds in Frijthout Park showed different isotopic signatures both in spring and winter. In spring, ponds 1 and 3 were rainfed and experienced evaporative fractionation reflected by the more enriched values, while 2 and 4 are groundwater-fed explained by their more negative values. In winter, groundwater samples (Average 18O (‰) = −7.17 ± 0.33, Average 2H (‰) = −46.33 ± 1.98) had similar values to precipitation values (Average 18O (‰) = −7.57 ± 1.55, Average 2H (‰) = −49.77 ± 12.53), suggesting that there was a constant groundwater recharge from rain infiltration due to the high precipitation rate in these sites during this season. The isotopic composition of pond 2 of Frijhout Park in winter was similar to groundwater, suggesting that the primary water source of this pond was indeed groundwater. Pond 4 in the winter season had closer values to precipitation, however, this does not consequently imply it is not groundwater-fed due to the similarities of both water sources in this season. Ponds 1 and 3 showed similar values to their counterparts in winter as well.
Neerland Park’s ponds 1 and 3 showed values similar to Frijthout Park’s ponds 2 and 4, both in spring and in winter, suggesting they were groundwater-fed. Pond number 2 in Neerland Park could be rainfed, as both in spring and in winter had similar values to precipitation.
4 Discussion
Aqua-Nature based Solutions refer to approaches, techniques and systems that utilize and mimic natural processes to address water-related challenges, promoting water infiltration, drought and flood mitigation and groundwater recharge. By creating water-rich habitats, aNBS can support biodiversity and enhance ecological functions. Given the adverse environmental effects of large-scale grey infrastructure, aNBS are increasingly being consider as an approach to tackle hydrological challenges in cities. However, it is important to highlight that NBS interventions might need to work hand by hand with grey interventions (i.e., hybrid solutions). The enhancement of synergies between aNBS and grey urban infrastructures in the urban planning (e.g., mobility, water drainage) could be one way to create resilient, just and sustainable urban environments (Stefanakis et al., 2021). The selected parks are the result of bargaining between developers and the permitting authorities, showing that urban development can go hand in hand with the creation of new green infrastructure, especially at the periphery of cities, creating space for people, water and biodiversity.
4.1 Vegetation biodiversity
Our findings revealed that both studied aNBS had a relatively high plant biodiversity. However, temporary ponds showed a significantly higher species abundance and Shannon-Wiener Index. Temporary ponds were indeed expected to have higher plant biodiversity due to the differentiation of various habitats, increasing the number of niches that can be filled and thus supporting significantly more biodiversity than those with permanent water (Fernández-Zamudio et al., 2016). These different zonations allow for the establishment of different plant species. Moreover, ephemeral and shallow-marsh zones are crucial for seed germination; thus, extended periods of inundation and deep water levels can be detrimental for plant species’ colonization and survival (Greenway et al., 2007). This supports our findings, as Neerland’s temporary ponds showed higher biodiversity than Frijthout’s permanent ponds. Importantly, Frijthout Park ponds, although classified as permanent due to the presence of water all year long, were seen to increase or decrease the water level depending on the season, varying their margins. This might as well benefit biodiversity, as the margins of a pond can also provide with a variety of habitats where vegetation can thrive (Oertli and Parris, 2019), and even support threatened species (Fernández-Zamudio et al., 2016). Therefore, a pond design with greater habitat heterogeneity has been demonstrated repeatedly to enhance plant biodiversity (Oertli and Parris, 2019; Jean-Philippe et al., 2021).
Urban environments are known to have a higher prevalence of alien and invasive plant species, as these ecosystems are often nutrient-rich and disturbed habitats that promote their establishment (Sinclair et al., 2020). In fact, in one of our study sites we found two exotic plants, E. engelmannii and C. scoparia. This is not uncommon in urbanized landscapes, where human activities favour the dispersal of alien species. These anthropogenic activities have been summarized by Ehrenfeld (2008) and include i) modification of channels and banks; ii) presence of paths for walking and biking; iii) presence of pet animals which can disperse the seeds or be predators of seed dispersers; iv) dumping rubbish; v) other physical disturbances (e.g., excavations, Earth mounds); vi) erosion due to stream channel elevation; vii) soil enrichment or loss of nutrients due to unproper management. In Frijthout Park, where these plant species were found, most of these activities are omnipresent. As a consequence, we might expect the invasion of alien species, and urban waterbodies are particularly prone to support them (Oertli and Parris, 2019). This can be detrimental to biodiversity if the introduced species eventually dominate the area and hinder the growth and dispersal of native species. E. engelmannii is a North American species that grows in fresh shores and disturbed places and which relies on the recession of spring water levels for its germination and development (Batten, 2014). With longer dry periods due to a warming climate, it is possible that this species might eventually dominate the area due to an early germination, so management should be performed to monitor its spread. For example, cutting the mature achenes in the late summer would prevent its dispersal with the waterflow during the winter period. However, although future naturalization seems likely, currently this species is instead considered to be critically endangered in Belgium (Verloove, 2015) and therefore these measures should be avoided at present. On the other hand, C. scoparia grows in a wide range of wetland types (i.e., wet or dry acidic sandy soils) and shows high tolerance to disturbance (Wallnöfer and Essl, 2016). The lack of surrounding suitable habitats can hamper the dispersal of this species, like it has been seen in Slovakia, although the dispersal of this plant might be favourable, as it has been reported that other Carex species (e.g., Carex acutiformis, Carex appropinquata, Carex lasiocarpa, Carex rostrata) have a high potential for nitrogen and phosphorus uptake, which might benefit the pond’s health by counteracting eutrophication (Hinzke et al., 2021). Previous studies have shown that alien species do not necessarily have a detrimental impact and even some have been reported to have positive environmental and socioeconomic effects (Milanović et al., 2020; Kourantidou et al., 2022). Nevertheless, attention should be taken to the domination of alien species. Invasive plant species can alter ecosystem interactions and potentially increase the transmission of disease vectors, such as mosquitoes, which might not only affect other animals (i.e., amphibians) but also humans. Therefore, a thorough monitoring of invasive species’ dispersal is highly recommended in aNBS.
4.2 Macroinvertebrate biodiversity and water quality
It is noteworthy to highlight that one pond in Neerland Park (pond Neerland 3) showed very low oxygen levels (down to 3.74 ± 1.03 mg O2/L), just two units above degraded freshwater bodies according to the Environmental Protection Agency (EPA). Oxygen is crucial for biodiversity but increasing temperatures are warming the surface of waterbodies, especially shallow ones, decreasing oxygen solubility and putting urban ponds on the verge of hypoxia (USEPA, 2003). This pond showed the highest temperature of all, so considering the increasing global warming, it will probably enter a hypoxic state soon. High temperatures not only enhance bacterial decomposition but also contribute to the accelerated depletion of oxygen. The relationship between oxygen, temperature and species richness is also well reflected in our MMIF results, as pond Neerland 3 showed the lowest values of all the aNBS (Table 2).
The MMIF uses macroinvertebrates as indicators of water and ecosystem quality status based on the assumption that ecological integrity would be reduced in a disturbed system. Indeed, studies have demonstrated that macroinvertebrates are particularly sensitive to metal contamination and thus, they are good indicators of urban pollution (Carlisle and Clements, 1999). The MMIF can therefore be used as an EQR scale (Gabriels et al., 2010). Our results indicate that the selected sites in the municipality of Antwerp have a water quality from moderate to poor (Table 2). MMIF values are normally positively correlated with oxygen concentration and negatively correlated with nitrogen, ammonium ion, nitrate ion, phosphorous, phosphate ion and biochemical and chemical oxygen demand (Gabriels et al., 2010). As oxygen levels were within the EPA standard levels (except for N3), we can deduce that our ponds are suffering from other stressors (i.e., pollution). This is not surprising, as urban waters tend to be heavily contaminated (Haase, 2015). The chosen ponds are indeed completely embedded in the urban matrix, surrounded by roads, residential buildings and parking places. Runoff water can carry road salts, heavy metals from automobile brakes and tires, polycyclic aromatic hydrocarbons (PAH) from road surfaces and fuel combustion to stormwater ponds (Gallagher et al., 2014). The MMIF values of the water-rich parks were mostly moderate, reflecting that despite the proximity to cities and its pollutants, urban ponds can increase biodiversity with correct management and design (e.g., planting of phytoremediation plants such as reed beds and willow bushes like in Frijthout Park). It is important to acknowledge that connectivity might decrease biodiversity by introducing polluted waters (Jackson and Pringle, 2010). In fact, in order to boost amphibian biodiversity, some studies have suggested that the stormwater ponds which are expected to accumulate significant levels of pollutants should be placed to minimize connectivity, managed as temporary ponds and frequently mowed so to deter amphibian use, but those with lower levels of pollutants should be connected to the wetlands and managed to promote amphibian reproductive success by increasing the wet duration and allowing aquatic vegetation to grow (Gallagher et al., 2014; Gołdyn et al., 2015). Therefore, hydrological connectivity is a crucial parameter to integrate in urban planning, and a previous study of the water flow and surroundings is needed to correctly locate, design and manage urban ponds.
Regarding the second hypothesis, where the hydroperiod was assumed to affect biodiversity, sites presenting different hydroperiod did not show significant differences on their MMIF results. These results contrast to those of Oertli and Parris (2019), who found that the hydroperiod determined macroinvertebrate assemblages of several urban ponds in United Kingdom (Sanderson et al., 2005) and South Africa (Apinda Legnouo et al., 2014). Despite these findings, the Frijthout permanent ponds had higher MMIF than the Neerland temporary ponds (Figure 5). Della Bella et al. (2005) also found that species richness of aquatic macroinvertebrates tended to increase with the length of the wet phase. Other studies support our finding, as temporary ponds might fail to support larval life cycles of macroinvertebrates (Chester and Robson, 2013).
Interestingly, it could be discussed that despite the generally low water quality, the plant diversity (i.e., Shannon-Wiener Index) was high. This might be because macroinvertebrates are generally more sensitive to disturbance, and therefore few tolerant species would thrive in urban pond ecosystems. On the other hand, many plants have mechanisms to cope with high levels of pollution, thriving in disturbed, ecologically poor habitats. Nutrient-rich ponds containing agricultural runoff waters can lead to extremely high productivity in urban ponds used for water management (Hassall, 2014). The vegetation in these sites might as well be introduced, creating a high diverse plant community which flourish on disturbed ecosystems. A previous study showed that although urbanization homogenizes invertebrates and birds’ biological communities, plants do not show a decline in species richness (Hassall, 2014).
An exotic coleoptera species, P. coxalis, was found in Neerlandpark. This species is native in southern Europe and has probably migrated north due to the increasing temperatures of the past years. Geographical shifts from warmer regions can increase the introduction of species, some of them are nuisance species such as mosquitoes. Mosquitoes are vectors of diseases and due to climate change, an increase in their transmission in Europe is expected. Several measures (e.g., steepening of banks, removal of vegetation and increased water depth, decrease shade) can reduce their abundance (Gingrich et al., 2006) but these might also decrease the overall biodiversity. Instead, introducing mosquitoes predators would succeed to decline this species while increasing the biodiversity (Gingrich et al., 2006). A thorough understanding of the ecological functioning on pond ecosystems is needed to combine design and species management to deliver all the required ESS aNBS are meant to provide while minimizing the potential disservices that can occur.
4.3 Hydrological dynamics and ecosystem services
It is worth to highlight that the classification of the ponds as temporary or permanent was based on a general observation and that this dichotomy should instead incorporate a gradient of states in the water level which can occur along the year (as it was observed in Frijthout Park). Therefore, monitoring of both the frequency and amplitude of the dry and wet states should be performed to better categorize the ponds according to their hydroperiod. The utilization of groundwater data was key in this determination. However, this data was collected only in the wet period and future monitoring during the dry period is required to better understand the water regulation and the ecosystem services that could be delivered.
In Frijthout Park, it was observed that across the park there are both infiltration and seepage areas, which correspond to the locations with deep or shallow groundwater levels, respectively. The piezometer data also suggested that water flowed from south to north, according to the topography. In Neerland Park, however, the groundwater was near the ground surface, meaning that the recharge from groundwater to the ponds is predominant. The flow also followed the topography.
Frijhout presented two clear zones with different water dynamics. In the north, further from the residential area, higher infiltration and deep groundwater recharge rates were found. Therefore, flooding events will be better mitigated in this zone. The MMIF values in the ponds selected in this zone were also higher (Frijthout 2 = 0.6; Frijthout 4 = 0.6) than in the ponds in the south of the site (Frijthout 1 = 0.5; Frijthout 3 = 0.4), suggesting that macroinvertebrates might play a role in the support of water regulation ESS and filtration of the water. For example, detrivores decrease litter quantity and burrowing invertebrates (e.g., earthworms) increase soil porosity, enhancing porewater turnover and increasing the water in the sediments (Prather et al., 2013). This encourages the implementation of a pond design that cover, not only water-related problems, but also the increase of biodiversity.
This different zonation in this site coincides with the isotope data. These data revealed that, in spring, ponds in the north were groundwater-fed while ponds in the south were more likely to depend on precipitation. Higher variability in piezometer 5, located in between the ponds located in the south, confirms this data, suggesting that they fluctuate according to the runoff from precipitation from the residential area. The income of polluted runoff (i.e., from the parking lot or houses) might also explain the lower MMIF, compared to the other two ponds. In addition, ponds in the north, due to their groundwater source, would provide a more stable habitat from which macroinvertebrate biodiversity could benefit, thus increasing their MMIF.
The area of Frijthout Park could be described as valuable on the delivery of water regulating ecosystem services. The high infiltration and drainage rates might provide buffering against flooding events after extreme precipitation events. Also, by the end of March 2022, the groundwater levels had not yet reached the low values from February of that year, suggesting a long retention time of groundwater in this park. This is crucial for the maintenance of the water table and the mitigation of drought events in the summer.
On the other hand, Neerland showed variable hydrodynamics. Its high groundwater levels indicate seeping zones. Despite the high infiltration and low drainage on the locations where the land piezometers were located, the high water fluctuations (indicative of low groundwater stability), showed that it is possible that droughts events might not be palliated due to the reliance on precipitation for the groundwater recharge. This should be confirmed with a follow-up study on the piezometer data after the summer period.
This research has therefore assessed the water regulating ESS of both pond complexes, although further study on the provisioning of other ESS (e.g., recreation, pollution sequestration, pest control, pollination) is needed to understand holistically how these aNBS can benefit society and to fully acknowledge the value of these ecosystems.
5 Conclusion
The research aimed to study the potential contribution of aNBS on increasing biodiversity while providing ESS that benefit human societies (i.e., water regulation). Our findings have provided evidence of the essential role of these ecosystems on supporting biological communities in cities, namely, vegetation communities, as these are able to thrive in disturbed ecosystems and are being introduced regularly in urban environments. Macroinvertebrate communities, on the other hand, are more sensitive to pollution and more likely to suffer the impacts of urbanization. Attention should be drawn on the invasiveness of the introduced species and the potential (dis)services these might provide. The water quality of the ponds was also examined with the MMIF and was found to be on the lower end in all the study ponds. Comparison between ponds with different hydroperiods was performed, and the results showed that temporary hydroperiods were better for plant biodiversity, while longer wet duration enhanced macroinvertebrate biodiversity. This finding encourages urban designers to implement aNBS with different hydrological characteristics that can support a diverse groups of organisms. However, the classification of ponds as temporary or permanent with the current definitions might not capture the whole complexity of the water dynamics. This was proven through the analysis of piezometer data. The hydrological characteristics of the ponds were studied and the findings revealed that the studied parks provided ESS related to water regulation such as infiltration, groundwater recharge and buffering against floods and droughts. However, a follow up study of the dry seasons should be performed. The correct design of water bodies is essential for the provisioning of all the required ESS, from water regulation to pollution sequestration, including recreation, the enhancement of biodiversity and the management of invasive species. Further research should account on the delivery of these other ESS. A holistic approach should be taken when integrating aNBS in the urban matrix, where grey infrastructure currently predominates. Introducing aNBS in the city plans is crucial as these are a key strategy to mitigate the impacts of urbanization and increase resilience against climate change-related risks while supporting biodiversity.
Data availability statement
The original contributions presented in the study are included in the article/Supplementary Material, further inquiries can be directed to the corresponding author.
Ethics statement
The manuscript presents research on animals that do not require ethical approval for their study.
Author contributions
SM: Conceptualization, Data curation, Formal Analysis, Investigation, Methodology, Software, Visualization, Writing–original draft, Writing–review and editing. RD: Conceptualization, Data curation, Formal Analysis, Investigation, Methodology, Software, Supervision, Writing–review and editing. JoS: Conceptualization, Funding acquisition, Resources, Supervision, Validation, Writing–review and editing. DT: Data curation, Methodology, Supervision, Writing–review and editing. MW: Data curation, Methodolog, Writing–original draft. JaS: Conceptualization, Funding acquisition, Methodology, Project administration, Resources, Supervision, Validation, Visualization, Writing–original draft, Writing–review and editing.
Funding
The author(s) declare financial support was received for the research, authorship, and/or publication of this article. This research was developed within the project BiNatUr: Bringing nature back–biodiversity-friendly nature-based solutions in cities and funded through the 2020–2021 Biodiversa and Water JPI joint call for research projects, under the BiodivRestore ERA-NET Cofund (GA N°101003777), with the EU and the funding organisations Academy of Finland (Finland), Bundesministerium für Bildung und Forschung (BMBF, Germany), Federal Ministry of Education and Research (Germany), National Science Centre (Poland), Research Foundation Flanders (fwo, Belgium) and Fundação para a Ciência e Tecnologia (Portugal).
Acknowledgments
I would like to express my deepest gratitude to the individuals who have contributed significantly to the completion of this paper. Namely JS, JS and DT for their guidance and support, and RD and MW for their willingness to share their expertise with me. Your help has been invaluable.
Conflict of interest
The authors declare that the research was conducted in the absence of any commercial or financial relationships that could be construed as a potential conflict of interest.
Publisher’s note
All claims expressed in this article are solely those of the authors and do not necessarily represent those of their affiliated organizations, or those of the publisher, the editors and the reviewers. Any product that may be evaluated in this article, or claim that may be made by its manufacturer, is not guaranteed or endorsed by the publisher.
Supplementary material
The Supplementary Material for this article can be found online at: https://www.frontiersin.org/articles/10.3389/fenvs.2023.1304347/full#supplementary-material
References
Albert, J. S., Destouni, G., Duke-Sylvester, S. M., Magurran, A. E., Oberdorff, T., Reis, R. E., et al. (2021). Scientists’ warning to humanity on the freshwater biodiversity crisis. Ambio 50, 85–94. doi:10.1007/s13280-020-01318-8
Apinda Legnouo, E. A., Samways, M. J., and Simaika, J. P. (2014). Value of artificial ponds for aquatic beetle and bug conservation in the Cape Floristic Region biodiversity hotspot. Aquatic Conservation Mar. Freshw. Ecosyst. 24 (4), 522–535. doi:10.1002/aqc.2413
Batten, R. (2014). Engelmann’s spike-rush (Eleocharis engelmannii) cyperaceae (sedge family). Maryland: Engelmann's Spikerush in Dorchester Co.
Begossi, A. (1996). Use of ecological methods in ethnobotany: diversity indices. Econ. Bot. 50 (3), 280–289. doi:10.1007/bf02907333
Bowen, G. J., Cai, Z., Fiorella, R. P., and Putman, A. L. (2019). Isotopes in the water cycle: regional- to global-scale patterns and applications. Annual Review of Earth and Planetary Sciences 47 (1), 453–479. doi:10.1146/annurev-earth-053018-060220
Brondizio, E., Diaz, S., Settele, J., and Ngo, H. T. (2019). Global assessment report on biodiversity and ecosystem services of the intergovernmental science-policy Platform on biodiversity and ecosystem services. Bonn, Germany: IPBES secretariat.
Carlisle, D. M., and Clements, W. H. (1999). Sensitivity and variability of metrics used in biological assessments of running waters. Environ. Toxicol. Chem. 18 (2), 285–291. doi:10.1002/etc.5620180227
Chester, E. T., and Robson, B. J. (2013). Anthropogenic refuges for freshwater biodiversity: their ecological characteristics and management. Biol. Conserv. 166, 64–75. doi:10.1016/j.biocon.2013.06.016
Della Bella, V., Bazzanti, M., and Chiarotti, F. (2005). Macroinvertebrate diversity and conservation status of Mediterranean ponds in Italy: water permanence and mesohabitat influence. Aquatic Conservation Mar. Freshw. Ecosyst. 15 (6), 583–600. doi:10.1002/aqc.743
De Pauw, N., and Vanhooren, G. (1983). Method for biological quality assessment of watercourses in Belgium. Hydrobiologia 100 (1), 153–168. doi:10.1007/BF00027428
De Vleeschauwer, K., Weustenraad, J., Nolf, C., Wolfs, V., De Meulder, B., Shannon, K., et al. (2014). Green–blue water in the city: quantification of impact of source control versus end-of-pipe solutions on sewer and river floods. Water Sci. Technol. 70 (11), 1825–1837. doi:10.2166/wst.2014.306
Durance, I., Bruford, M. W., Chalmers, R., Chappell, N. A., Christie, M., Cosby, B. J., et al. (2016). The challenges of linking ecosystem services to biodiversity. Adv. Ecol. Res. 54, 87–134. doi:10.1016/bs.aecr.2015.10.003
Eggermont, H., Balian, E., Azevedo, J. M. N., Beumer, V., Brodin, T., Claudet, J., et al. (2015). Nature-based solutions: new influence for environmental management and research in Europe. GAIA - Ecol. Perspect. Sci. Soc. 24 (4), 243–248. doi:10.14512/gaia.24.4.9
Ehrenfeld, J. G. (2008). Exotic invasive species in urban wetlands: environmental correlates and implications for wetland management. J. Appl. Ecol. 45 (4), 1160–1169. doi:10.1111/j.1365-2664.2008.01476.x
European Commission (2015). The EU and nature-based solutions. Avaliable at: https://research-and-innovation.ec.europa.eu/research-area/environment/nature-based-solutions_en (Accessed October 23, 2023).
Faivre, N., Fritz, M., Freitas, T., de Boissezon, B., and Vandewoestijne, S. (2017). Nature-Based Solutions in the EU: innovating with nature to address social, economic and environmental challenges. Environ. Res. 159, 509–518. doi:10.1016/j.envres.2017.08.032
Fernández-Zamudio, R., García-Murillo, P., and Díaz-Paniagua, C. (2016). Aquatic plant distribution is driven by physical and chemical variables and hydroperiod in a mediterranean temporary pond network. Hydrobiologia 774 (1), 123–135. doi:10.1007/s10750-016-2701-2
Gabriels, W., Lock, K., De Pauw, N., and Goethals, P. L. M. (2010). Multimetric macroinvertebrate index Flanders (MMIF) for biological assessment of rivers and lakes in Flanders (Belgium). Limnologica - Ecol. Manag. Inland Waters 40 (3), 199–207. doi:10.1016/j.limno.2009.10.001
Gallagher, M. T., Snodgrass, J. W., Brand, A. B., Casey, R. E., Lev, S. M., and Van Meter, R. J. (2014). The role of pollutant accumulation in determining the use of stormwater ponds by amphibians. Wetl. Ecol. Manag. 22 (5), 551–564. doi:10.1007/s11273-014-9351-9
Gingrich, J. B., Anderson, R. D., Williams, G. M., O'Connor, L. I. N. D. A., and Harkins, K. (2006). Stormwater ponds, constructed wetlands, and other best management practices as potential breeding sites for West Nile virus vectors in Delaware during 2004. J. Am. Mosquito Control Assoc. 22 (2), 282–291. doi:10.2987/8756-971X(2006)22[282:SPCWAO]2.0.CO;2
Gołdyn, B., Chudzińska, M., Barałkiewicz, D., and Celewicz-Gołdyn, S. (2015). Heavy metal contents in the sediments of astatic ponds: influence of geomorphology, hydroperiod, water chemistry and vegetation. Ecotoxicol. Environ. Saf. 118, 103–111. doi:10.1016/j.ecoenv.2015.04.016
Greenway, M., Jenkins, G., and Polson, C. (2007). Macrophyte zonation in stormwater wetlands: getting it right! A case study from subtropical Australia. Water Sci. Technol. 56 (3), 223–231. doi:10.2166/wst.2007.494
Guerrero, P., Haase, D., and Albert, C. (2018). Locating spatial opportunities for nature-based solutions: a river landscape application. Water 10 (12), 1869. doi:10.3390/w10121869
Haase, D. (2015). Reflections about blue ecosystem services in cities. Sustain. Water Qual. Ecol. 5, 77–83. doi:10.1016/j.swaqe.2015.02.003
Hassall, C. (2014). The ecology and biodiversity of urban ponds. WIREs Water 1 (2), 187–206. doi:10.1002/wat2.1014
Hill, M. J., Biggs, J., Thornhill, I., Briers, R. A., Gledhill, D. G., White, J. C., et al. (2017). Urban ponds as an aquatic biodiversity resource in modified landscapes. Glob. Change Biol. 23 (3), 986–999. doi:10.1111/gcb.13401
Hinzke, T., Tanneberger, F., Aggenbach, C., Dahlke, S., Knorr, K. H., Kotowski, W., et al. (2021). Can nutrient uptake by Carex counteract eutrophication in fen peatlands? Sci. Total Environ. 785, 147276. doi:10.1016/j.scitotenv.2021.147276
IUCN (2020). “Global standard for nature-based solutions,” in A user-friendly framework for the verification, design and scaling up of NbS. 1st Edn (Gland, Switzerland: IUCN).
Jackson, C. R., and Pringle, C. M. (2010). Ecological benefits of reduced hydrologic connectivity in intensively developed landscapes. BioScience 60 (1), 37–46. doi:10.1525/bio.2010.60.1.8
Jean-Philippe, B., Marie, H., Muriel, S., and Laurent, L. (2021). Are acts of selective planting and maintenance drivers for vegetation change in stormwater systems? A case study of two infiltration basins. Ecol. Eng. 172, 106400. doi:10.1016/j.ecoleng.2021.106400
Jones, E. R., van Vliet, V., Michelle, T., Qadir, M., and Bierkens, M. F. P. (2020). Spatially-explicit estimates of global wastewater production, collection, treatment and re-use. Earth Syst. Sci. Data doi:10.5194/essd-2020-156
Kourantidou, M., Haubrock, P. J., Cuthbert, R. N., Bodey, T. W., Lenzner, B., Gozlan, R. E., et al. (2022). Invasive alien species as simultaneous benefits and burdens: trends, stakeholder perceptions and management. Biol. Invasions 24 (7), 1905–1926. doi:10.1007/s10530-021-02727-w
Krauze, K., and Wagner, I. (2019). From classical water-ecosystem theories to nature-based solutions — contextualizing nature-based solutions for sustainable city. Sci. Total Environ. 655, 697–706. doi:10.1016/j.scitotenv.2018.11.187
Kuhlemann, L. M., Tetzlaff, D., Marx, C., and Soulsby, C. (2022). The imprint of hydroclimate, urbanization and catchment connectivity on the stable isotope dynamics of a large river in Berlin, Germany. J. Hydrology 613, 128335. doi:10.1016/j.jhydrol.2022.128335
Maggiotto, G., Miani, A., Rizzo, E., Castellone, M. D., and Piscitelli, P. (2021). Heat waves and adaptation strategies in a mediterranean urban context. Environ. Res. 197, 111066. doi:10.1016/j.envres.2021.111066
Milanović, M., Knapp, S., Pyšek, P., and Kühn, I. (2020). Linking traits of invasive plants with ecosystem services and disservices. Ecosyst. Serv. 42, 101072. doi:10.1016/j.ecoser.2020.101072
Ncube, S., and Arthur, S. (2021). Influence of blue-green and grey infrastructure combinations on natural and human-derived capital in urban drainage planning. Sustainability 13 (5), 2571. doi:10.3390/su13052571
Nunes, A., Tápia, S., Pinho, P., Correia, O., and Branquinho, C. (2015). Advantages of the point-intercept method for assessing functional diversity in semi-arid areas. IForest - Biogeosciences For. 8 (4), 471–479. doi:10.3832/ifor1261-007
Oertli, B., and Parris, K. M. (2019). Review: toward management of urban ponds for freshwater biodiversity. Ecosphere 10 (7), e02810. doi:10.1002/ecs2.2810
Oral, H. V., Carvalho, P., Gajewska, M., Ursino, N., Masi, F., Hullebusch, E. D. V., et al. (2020). A review of nature-based solutions for urban water management in European circular cities: a critical assessment based on case studies and literature. Blue-Green Syst. 2 (1), 112–136. doi:10.2166/bgs.2020.932
Oswald, C. J., Kelleher, C., Ledford, S. H., Hopkins, K. G., Sytsma, A., Tetzlaff, D., et al. (2023). Integrating urban water fluxes and moving beyond impervious surface cover: a review. J. Hydrology 618, 129188. doi:10.1016/j.jhydrol.2023.129188
Pettorelli, N., Graham, N. A. J., Seddon, N., Maria da Cunha Bustamante, M., Lowton, M. J., Sutherland, W. J., et al. (2021). Time to integrate global climate change and biodiversity science-policy agendas. J. Appl. Ecol. 58 (11), 2384–2393. doi:10.1111/1365-2664.13985
Prather, C. M., Pelini, S. L., Laws, A., Rivest, E., Woltz, M., Bloch, C. P., et al. (2013). Invertebrates, ecosystem services and climate change. Biol. Rev. 88 (2), 327–348. doi:10.1111/brv.12002
Prudencio, L., and Null, S. E. (2018). Stormwater management and ecosystem services: a review. Environ. Res. Lett. 13, 033002. doi:10.1088/1748-9326/aaa81a
Sanderson, R. A., Eyre, M. D., and Rushton, S. P. (2005). Distribution of selected macroinvertebrates in a mosaic of temporary and permanent freshwater ponds as explained by autologistic models. Ecography 28 (3), 355–362. doi:10.1111/j.0906-7590.2005.04093.x
Secretariat of the Convention on Biological Diversity (2020). Global Biodiversity Outlook 5. Montrea
Seddon, N., Smith, A., Smith, P., Key, I., Chausson, A., Girardin, C., et al. (2021). Getting the message right on nature-based solutions to climate change. Glob. Change Biol. 27 (8), 1518–1546. doi:10.1111/gcb.15513
Sinclair, J. S., Reisinger, A. J., Bean, E., Adams, C. R., Reisinger, L. S., and Iannone, B. V. (2020). Stormwater ponds: an overlooked but plentiful urban designer ecosystem provides invasive plant habitat in a subtropical region (Florida, USA). Sci. Total Environ. 711, 135133. doi:10.1016/j.scitotenv.2019.135133
Smith, P., Cotrufo, M. F., Rumpel, C., Paustian, K., Kuikman, P. J., Elliott, J. A., et al. (2015). Biogeochemical cycles and biodiversity as key drivers of ecosystem services provided by soils. SOIL 1 (2), 665–685. doi:10.5194/soil-1-665-2015
Stefanakis, A. I., Calheiros, C. S. C., and Nikolaou, I. (2021). Nature-based solutions as a tool in the new circular economic model for climate change adaptation. Circular Econ. Sustain. 1 (1), 303–318. doi:10.1007/s43615-021-00022-3
Tetzlaff, D., Buttle, J., Carey, S. K., McGuire, K., Laudon, H., and Soulsby, C. (2015). Tracer-based assessment of flow paths, storage and runoff generation in northern catchments: a review. Hydrol. Process. 29, 3475–3490. doi:10.1002/hyp.10412
Tickner, D., Opperman, J. J., Abell, R., Acreman, M., Arthington, A. H., Bunn, S. E., et al. (2020). Bending the curve of global freshwater biodiversity loss: an emergency recovery plan. BioScience 70 (4), 330–342. doi:10.1093/biosci/biaa002
USEPA (U.S. Environmental Protection Agency) (2003). Ambient water quality criteria for dissolved oxygen, water clarity and chlorophyll a for the chesapeake bay and its tidal tributaries (Regional Criteria Guidance). Annapolis, MD: EPA 903-R-03-002 Region III Chesapeake Bay Program Office.
Verloove, F. (2015). Eleocharis engelmannii and E. obtusa (Cyperaceae), two recent acquisitions from series Ovatae in Belgium. Dumortiera 107, 25–30.
Verneaux, J., and Tuffery, G. (1968). “Methods de determination de La qualite biologique des eaux de La fauna du fond. Minestere de l’agriculture (France),” in Centre National d’edtudes techniques et de recherches technologiques por l’agricul de l’Agriculture (France). Centre national d’edtudes techniques et de recherches technologiques por l’agriculture, 1968.
Vörösmarty, C. J., McIntyre, P. B., Gessner, M. O., Dudgeon, D., Prusevich, A., Green, P., et al. (2010). Global threats to human water security and river biodiversity. Nature 467 (7315), 555–561. doi:10.1038/nature09440
Wallnöfer, B., and Essl, F. (2016). Overview on alien Carex species of section cyperoideae (including ovales) in Europe and the discovery of Carex scoparia in Austria. Annalen des naturhistorischen museums in wien. Ser. B Für Bot. Und Zool. 118, 115–127. Avaliable at: http://www.jstor.org/stable/43922687.
Willemen, S. (2023). Landscape design strategies to improve ground water levels in Flanders. Master’s thesis. Antwerpen: University Antwerpen.
Keywords: ecosystem services, nature-based solutions, biodiversity, climate change, urban ponds, urban planning, water regulation, hydroperiod
Citation: Martín Muñoz S, Schoelynck J, Tetzlaff D, Debbaut R, Warter M and Staes J (2024) Assessing biodiversity and regulatory ecosystem services in urban water bodies which serve as aqua-Nature-based Solutions. Front. Environ. Sci. 11:1304347. doi: 10.3389/fenvs.2023.1304347
Received: 29 September 2023; Accepted: 08 December 2023;
Published: 05 January 2024.
Edited by:
Fátima Alves, Universidade Aberta, PortugalReviewed by:
Rui Manuel Vitor Cortes, University of Trás-os-Montes and Alto Douro, PortugalPedro Pereira, Universidade Aberta, Portugal
Luciana Souza Bragança, Federal University of Minas Gerais, Brazil
Copyright © 2024 Martín Muñoz, Schoelynck, Tetzlaff, Debbaut, Warter and Staes. This is an open-access article distributed under the terms of the Creative Commons Attribution License (CC BY). The use, distribution or reproduction in other forums is permitted, provided the original author(s) and the copyright owner(s) are credited and that the original publication in this journal is cited, in accordance with accepted academic practice. No use, distribution or reproduction is permitted which does not comply with these terms.
*Correspondence: Jan Staes, jan.staes@uantwerpen.be