- 1School of Environmental and Municipal Engineering, Qingdao University of Technology, Qingdao, China
- 2Shandong Key Laboratory of Coastal Environmental Processes, Yantai Institute of Coastal Zone Research, Chinese Academy of Sciences, Yantai, China
The influence of soil salinization on nitrogen (N) transformation is largely unknown, which impedes the reasonable management of N in saline fields. A comprehensive meta-analysis was thus conducted to evaluate the effects of salinity and relative soil physicochemical properties on net N mineralization and nitrification in upland soils. Results showed that effects of salinity on the net-N mineralization rate (Min) and nitrification rate (Nit) changed with the salinity level and incubation time. Generally, the inhibitory effect of salt on Min and Nit decreased gradually with incubation time. At 14–16 days of soil incubation, significant stimulatory effects on Min were observed in middle-level (ECe: 12–16 dS m-1) and high-level (ECe >16 dS m-1) saline soils, and on Nit in low-level (ECe: 4–12 dS m-1) saline soils. Regression analysis revealed that the effects of soil organic carbon (SOC), total N (TN), C/N, pH, and clay content on Min and Nit were closely related to salinity levels. Nit at 5–7 days of soil incubation first enhanced and then decreased with C/N increase, and the threshold value was 34.7. The effect of pH on Nit changed with salinity levels, and shifted from stimulation to inhibition with increasing pH. Min at 5–7 days of soil incubation in middle-level group first increased with increasing pH, and decreased when pH was higher than 8.1. Salinization deeply affected soil properties, which further influenced N turnover via alteration of the availability of substrates and microbial biomass and activities. Our findings suggest that the influence of salinity on soil N turnover closely related with salinity level, and salinity level should be considered fully when optimizing N management in saline upland fields.
1 Introduction
Soil nitrogen (N) transformation involves a series of microbial processes that control soil N availability and thus ecosystem productivity. Among these, N mineralization and nitrification, which involve the release of active N from organic N and oxidation of ammonium to nitrate, respectively, are associated with the two largest terrestrial N fluxes on Earth (Kuypers et al., 2018). Ammonium and nitrate in soils are the most important forms of the available N for plant and microbial growth, and are thus important for terrestrial net primary production (Wieder et al., 2015). From regional to global scales, the patterns and controlling factors of N mineralization and nitrification in soils have received much attention, and the drivers of both processes vary with ecosystem type (Elrys et al., 2021a; b). However, little information about the characteristics of N mineralization and nitrification in saline soils has been available to date. More than 6% of agricultural land worldwide is affected by salt, and soil salinization is still on the rise (Zhang et al., 2010; Yang et al., 2022). The current security of the world’s food supply is severely challenged by its increasing population. Globally, 86% of saline land provides 11% of the total biomass production on Earth (Wicke et al., 2011), and saline lands thus possess great potential for grain production. N supply plays an irreplaceable role in increasing crop yields; however, excess N easily leads to numerous environmental problems, such as nitrous oxide and NH3 emissions (Ichihashi et al., 2020). Therefore, it is imperative to reveal the features of N mineralization and nitrification, and of their controlling factors, in saline soils.
In soils, N mineralization and nitrification can be influenced by soil properties, microbial characteristics, and climate factors. The rates of N mineralization and nitrification significantly increase with microbial biomass and diversity (Zeng et al., 2014; Li et al., 2020). Bacteria and fungi participate in N mineralization in soil, and ammonia-oxidizing bacteria (AOB) and ammonia-oxidizing archaea (AOA) are participants in autotrophic nitrification (Lang and Jagnow, 1986; Brierley and Wood, 2001; Martens-Habbena et al., 2009). N mineralization and nitrification usually increase with increasing soil organic carbon (SOC) and total N (TN), but decrease with increasing SOC to TN ratio (C/N) (Wang et al., 2016; Cheng et al., 2019; Li et al., 2020). An increase in soil pH can stimulate nitrifying activity and increase N mineralization rates (Nugroho et al., 2007; Wang et al., 2016). Soil physical properties such as soil bulk density are correlated with microbial biomass, activity, and oxygen supply, and ultimately affect N mineralization and nitrification (Elrys et al., 2021a; b).
However, the above findings were mostly observed in non-saline soils. Some studies have reported that the rate of N mineralization increases with increasing soil salinity level (Zhou et al., 2017), whereas other studies have found that N mineralization decreases with salinity levels (Duan et al., 2018), or that salinity has little effect on N mineralization (Laura, 1974). Nitrification has been found to be more sensitive to soil salinization relative to mineralization, as the former can be inhibited completely if soil saturation extract electrical conductivity (ECe) exceeds 26 dS m-1 (McCormick and Wolf, 1980; Pathak and Rao, 1998). However, Magalhaes et al. (2005) revealed that nitrification rates are stimulated when the soil salinity increases from 0 to 15 parts per thousand. Thus, the currently available information about the effect of salinity on N turnover is not consistent. Moreover, the roles of relevant saline soil properties such as SOC, TN, and pH in N transformation were previously ignored, and remain unclear. Therefore, it is essential to discover the general trend of soil salinization and relevant soil properties’ effects on N mineralization and nitrification by synthesizing the available results.
Increases in soil salinity have been found to inhibit plant growth and decrease biomass production significantly (Erickson et al., 2007), further resulting in the reduction of soil organic inputs and SOC (Kamble et al., 2014). SOC is positively correlated with microbial biomass and diversity (Zhang et al., 2019). C/N is an important variable in regulating the balance between N mineralization and immobilization, and mineralization is suppressed with C/N > 18 (Cheng et al., 2017). Thus, we hypothesize that N mineralization and nitrification will be inhibited by soil salinization (hypothesis 1), and that SOC and C/N are important controlling factors under saline conditions (hypothesis 2). With salinization, Ca2+and Mg2+ in soil colloids are replaced by Na+, which causes soil structure destruction, soil compaction, and aeration reduction (Wong et al., 2010). Oxygen supply directly and indirectly affects mineralization and nitrification (Zhou et al., 2017; Elrys et al., 2021b). We thus hypothesize that N mineralization and nitrification will be regulated by the status of soil aeration (hypothesis 3).
Unlike a previous report that studied soil salinization’s effects on N cycling in coastal ecosystems (Zhou et al., 2017), a meta-analysis was conducted to examine the responses of N mineralization and nitrification to soil salinization in upland fields. To the best of our knowledge, this is the first meta-analysis that considers soil salinity and the relevant soil properties’ effects on N mineralization and nitrification in upland soils. The questions examined in this analysis were as follows: i) What are the main features of N mineralization and nitrification in saline upland soils? ii) Are SOC and C/N the key controlling factors of mineralization and nitrification under saline conditions? And iii) Do the effects of salinity on mineralization and nitrification vary with soil texture (clay content)?
2 Materials and methods
2.1 Data compilation
The following terms: “saline soil” or “salinity,” and “nitrogen,” were used in publication searches in the Web of Science and China National Knowledge Infrastructure (CNKI) databases before May 2023. In total, 132 papers were retrieved. Appropriate studies were selected based on the following criteria: 1) net nitrification or mineralization was estimated in the studies; 2) salinization treatments in addition to non-salinization treatments in which soil ECe <4 dS m-1, or salt contents <1 g kg-1, were included in the studies; and 3) surface soils (0–20 cm or 0–10 cm) were used in the studies, and not flooded during experiments. In accordance with the above criteria, 14 papers including 61 observations were screened from the initial 132 publications (Table 1). The variables in the compiled database included the following soil properties: soil salinity, pH, SOC, TN, C/N, clay content, inorganic N content (TIN), net N mineralization rate (Min) and net nitrification rate (Nit). To determine Min or Nit, organic manure or inorganic N were added as a substrate in the studied soils. After substrate addition, Min was calculated at 2–3 days, 5–7 days, 14–16 days, and >21 days in the selected studies, using Eq. 1 (Duan et al., 2018):
where Ct2 andCt1are the sums of the exchangeable NH4+‒N and NO3−‒N concentrations (mg N kg-1 soil) at incubation time t2 and t1, respectively, andt2 and t1 are the incubation times (d).
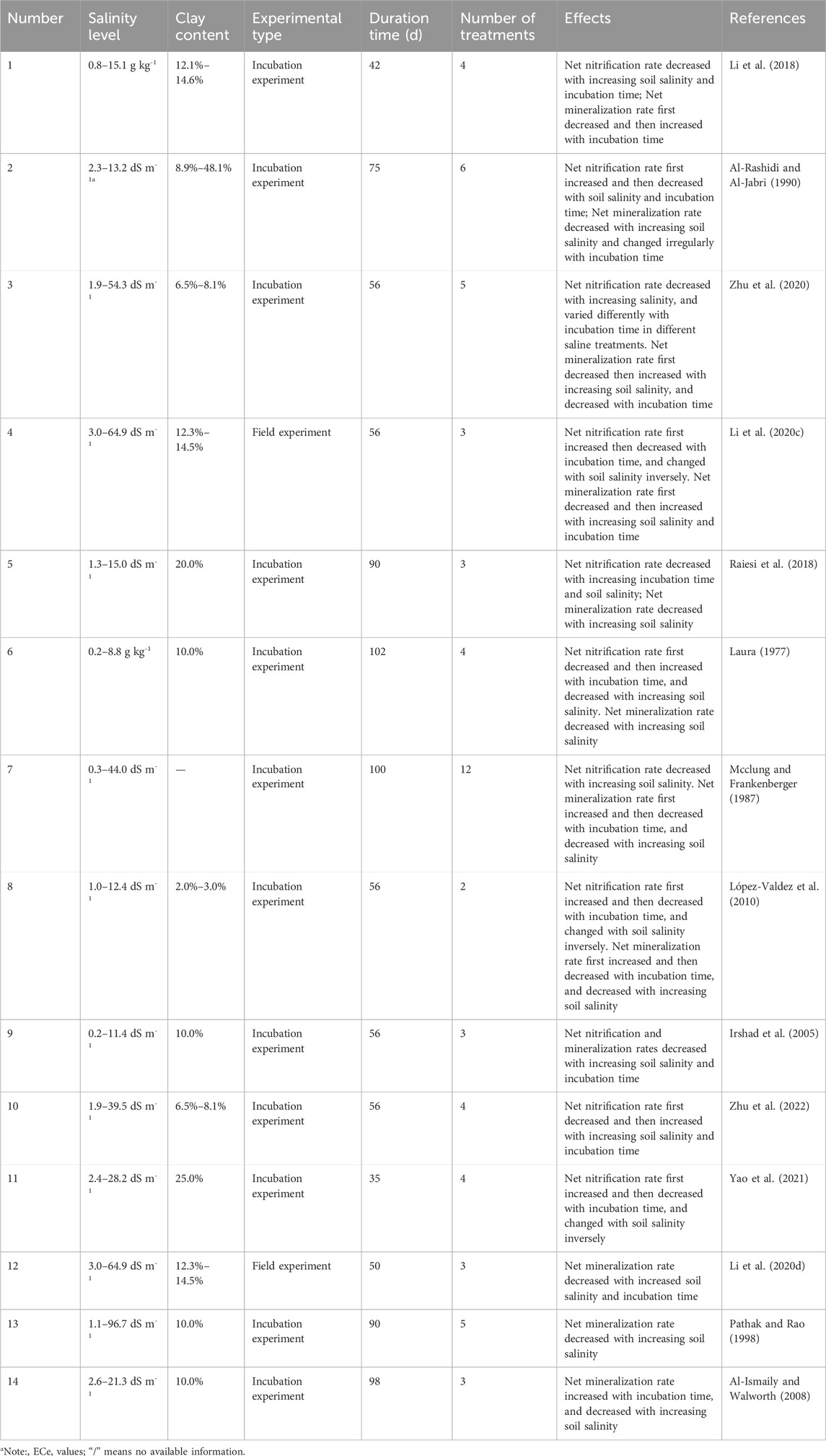
TABLE 1. Soil salinity levels, soil clay contents, experimental type, duration time, and effects in this study.
Similarly, Nit was determined using Eq. 2:
where Dt2 and Dt1 are the exchangeable NO3−‒N concentrations (mg N kg-1 soil) at incubation time t2 and t1, respectively, and t2 and t1 are the incubation times (d).
In each paper, more than one salinity level was used. Subsequently, one salinity level was defined as one treatment in this meta-analysis, and all comparisons were recorded as independent observations (Zhou et al., 2017). For each study, information on soil properties, net N mineralization, and nitrification were extracted. If the data were reported as figures in the original publications, these data were extracted by GetData Graph Digitizer (version 2.24). Soil salinity (ECe level) data were assigned into three salinization categories based on the reports of Wicke et al. (2011) and Bao (2000): 4–12 dS m-1 (low-level), 12–16 dS m-1 (middle-level), and >16 dS m-1 (high-level). Soil salinity EC1:5 data (electrical conductivity of a 1:5 mixture of soil:water) were converted into ECe values (Rengasamy, 2006). Salt contents were converted into ECe values through an experimental equation (Eq. 3), which was developed in our pilot experiment:
where S is the value of soil soluble salt contents (g kg-1).
Clay contents in sandy loam and clay loam soils were assigned as 10% and 20%, respectively, if clay content was not provided in the papers.
2.2 Data analyses
The normality of the data was checked using the Kolmogorov–Smirnov test, and natural logarithm (ln) transformation was used when the data did not exhibit normal distribution, with the exception of the value of pH. To investigate the influences of soil salinity on N mineralization and nitrification, the response ratio (R) was used as a metric of effect size in this study. For a given variable, the natural logarithm of R (lnR) was calculated as the ratio of the value in saline treatment (Xt) to that in the control (non-saline) treatment (Xc) in each study (Eq. 4):
The mean effect size for all observations (lnR+) was calculated using Eq. 5:
where i is the number of observations. Wi is the weight factor in each study, which was calculated using Eq. 6 (Van Groenigen et al., 2014):
where Nt and Nc are the sample sizes for the saline and control treatment groups, respectively.
Bootstrapping procedures within METAWIN 2.1 software (Arizona State University, Tempe, AZ, USA) were used to generate 95% confidence intervals (95%CI) for the weighted effect sizes using 4999 iterations (Rosenberg et al., 2000). The effects of soil salinity were considered significant if the 95%CI values did not overlap with zero. To compare the effects of salinity directly, lnR+ was converted into the mean response ratio (RY), calculated using Eq. 7:
Using SPSS software (12.0), Pearson correlation was conducted to investigate the influence of salinity on soil physicochemical properties. Curvilinear regression analysis was employed to test the relationships between the following soil properties: salinity, pH, SOC, TN, C/N, clay content, and the response ratios (lnR) for N mineralization or nitrification in soils with different salinity levels. If the number of observations was less than five (n < 5), meta and curvilinear regression analysis were negligible.
3 Results
3.1 Soil properties
Soil salinization significantly increased TN, and the values of RY for the total observations and low-level group were 53.5% and 142.7%, respectively (Figure 1). SOC was also significantly improved in low-level saline soils (95%CI of RY: 10.7%–271%). However, the effects of salinity on SOC in middle- and high-level saline soils were non-significant. Soil pH, C/N, and TIN did not vary significantly within the total observation, the low-, middle-, and high-level groups.
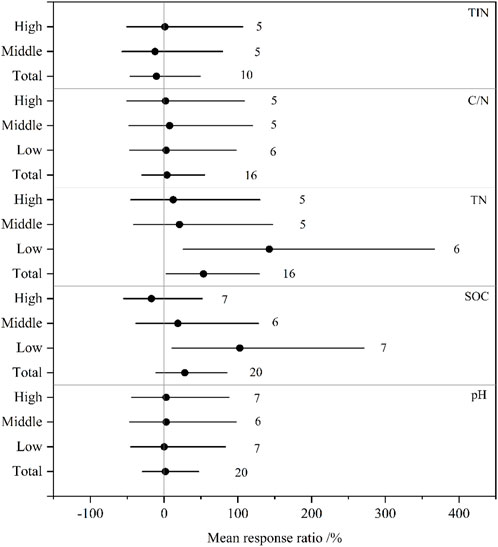
FIGURE 1. The mean effect sizes of salinization on soil properties from the total, low-, middle-, and high-level groups. Error bars represent 95% bootstrapped confidence intervals (CIs). The number of observations for each variable is shown next to the error bars. The effect of salinization was considered significant if the 95% CI of the effect size did not overlap with zero. TIN, soil total inorganic N; C/N, ratio of soil organic C to total N; TN, soil total N; SOC, soil organic C.
3.2 Nitrogen mineralization
The effect of soil salinity on Min changed with incubation time and salinity levels (Figure 2). The effects on Min in total soils significantly decreased by 83.6% at 2–3 days (Min2-3), 37.0% at 5–7 days (Min5-7), and 42.0% after 21 days (Min>21). Inhibitory influences reduced with incubation time, and a significant inhibitory effect was observed in middle- and high-level saline soils after 21 days. A small influence of salinity on Min was observed in low-level saline soils throughout the incubation. Significantly negative effects on Min5-7 were observed in high-level saline soils, and similar inhibitory effects on Min>21 were observed in middle- and high-level saline soils. However, Min at 14–16 days (Min14-16) increased significantly in middle- and high-level saline soils, with RY 95%CI of 34.6%–317.5% and 32.7%–285.7%, respectively.
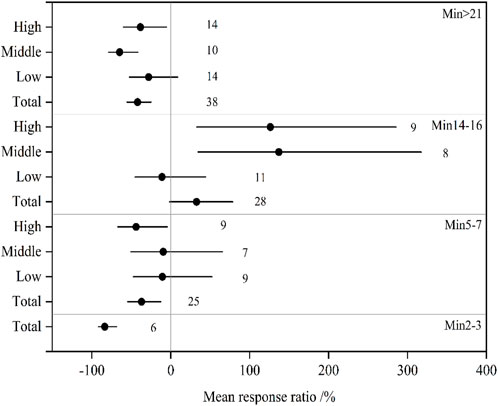
FIGURE 2. The mean effect sizes of salinization on net nitrogen mineralization in the total, low-, middle-, and high-level soils. Error barsrepresent 95% bootstrapped confidence intervals (CIs). The number of observations for each variable is shown next to the error bars. The effect of salinization was considered significant if the 95% CI of the effect size did not overlap with zero. Min2-3, Min5-7, Min14-16, and Min>21 mean the rate of net soil nitrogen mineralization at 2–3 days, 5–7 days, 14–16 days, and after 21 days incubation, respectively.
3.3 Nitrification
Soil salinization effects on Nit are shown in Figure 3. With the exception of Nit at 14–16 days (Nit14-16), Nit significantly decreased by 50.1% at 2–3 days (Nit2-3), 58.8% at 5–7 days (Nit5-7), and 44.5% after 21 days incubation (Nit>21) in the total observations. Thus, the inhibitory effect decreased with incubation time. In middle- and high-level saline soils, Nit5-7 significantly decreased by 56.7% and 75.5%, respectively. Nit>21 in low-, middle-, and high-level saline soils significantly decreased by 39.2%, 49.3%, and 52.7%, respectively. The effects of salinity on Nit14-16 significantly increased by 98.6% in low-level soils, and changed non-significantly by 90.6% and −18.4% in middle- and high-level saline soils. Thereby, the inhibitory effect of salinity on Nit increased with increasing salinity level.
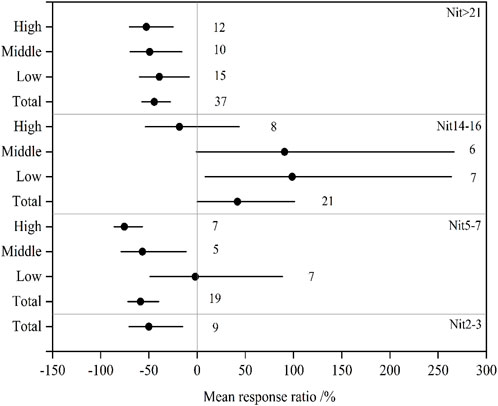
FIGURE 3. The mean effect sizes of salinization on net nitrification in the total, low-, middle-, and high-level soils. Error barsrepresent 95% bootstrapped confidence intervals (CIs). The number of observations for each variable is shown next to the error bars. The effect of salinization was considered significant if the 95% CI of the effect size did not overlap with zero. Nit2-3, Nit5-7, Nit14-16, and Nit>21 mean the rate of net soil nitrification at 2–3 days, 5–7 days, 14–16 days, and after 21 days incubation, respectively.
4 Discussion
4.1 Salinity levels
Overall, the influencing degree of salinity on N mineralization and nitrification depended on salinity level, and the response of mineralization to salinity was different from that of nitrification. The inhibitory effect of salinity on Nit and Min may be explained by the soil microbial biomass, activity, and abundance significantly decreasing with increasing salinity levels (Sun et al., 2016; Xie et al., 2017; Zhu et al., 2022). In the low-level group, Min was little affected by soil salinization throughout the incubation (Figure 2), whereas significant negative influences on Nit>21 were observed (Figure 3). In the middle- and high-level groups, except Min>21, the inhibitory effect of salinity on Min was markedly lower than that on Nit. This suggested that nitrification was more easily affected by salinity than mineralization, which may be due to the higher salt tolerance of the enzymes involved in N mineralization relative to those that catalyze nitrification (Pathak and Rao, 1998; Dendooven et al., 2010). Our results were in accordance with the report of Li et al. (2020), which indicated that N mineralization was little affected by soil salinization if salt content was less than 3.0 g kg-1 (equivalently, ECe <9.2). Furthermore, our results differed from those of previous studies in which nitrification increased in low-level saline soils (salt content <3.0–3.5 g kg-1), or showed no inhibition when salinity was below a threshold (Zeng et al., 2013; Cortes-Lorenzo et al., 2015; Li et al., 2020). In our study, the responses of Nit to salinization varied with salinity level and incubation time, and Nit>21 significantly decreased in total, low-, middle-, and high-level saline soils. The inconsistent conclusions might be caused by the different incubation times in different studies.
Regression analysis indicated that the lnR of Nit5-7 was significantly negatively correlated with salinity in the total (p < 0.01) and high-level (p < 0.05) groups (Table 2), indicating that after 5–7 days of substrate addition, Nit significantly decreased with increasing salinity level. This was consistent with the results of Dendooven et al. (2010), which showed that concentrations of NO3− or Nit decreased with salinity level increases. The relation between the lnR of Nit14-16 and salinity in the total soils fitted the quadratic equation well (p < 0.05), and the lnR of Nit14-16 increased when ECe <10.4 dS m-1, and decreased when ECe >10.4 dS m-1. This could be explained by the high substrate (NH4+) accumulation due to nitrification inhibition by salinity at 14–16 days, which in turn increased Nit. However, excessively high salinity can increase NH3 loss and decrease microbial activities in soil (Mandal et al., 2016; Zhu et al., 2020), and leads to Nit decrease. An ECe value of 10.4 dS m-1 can be used as a threshold value to estimate the nitrifying process after 14–16 days of N addition in saline soils. In contrast to the observed stimulatory effect on Min by increasing salinity in coastal ecosystems (Zhou et al., 2017), no significant relation was observed between Min and salinity in the present study. This is probably due to the complex mechanisms of N mineralization in soils, which is regulated by microbial N immobilization (Kooijman et al., 2009), microbial community composition (Wong et al., 2008), and ecosystem type (Bai et al., 2012).
4.2 Incubation time
The total inhibitory effect of salinity on N mineralization and nitrification reduced with time in general, and the stimulatory effect of salinity on Nit14-16/Min14-16 was recorded (Figure 2; Figure 3). The stimulatory effect of salinity on N transformation, especially at 14–16 days after substrate addition, was mostly caused by the residence time of substrates (organic nitrogen and NH4+) increase. Awadelkarim et al. (1995) found that urea hydrolysis significantly decreased when salt concentration rose from 40 meq L-1–200 meq L-1. Under high saline conditions, NH4+ transformation was found to be delayed by approximately 2 days (Tao et al., 2020), and Nit14-16 still remained at a relatively high level, compared with that in low- and mid-level soils. The accumulation of substrates in saline soils increased Min and Nitto a different extent. Therefore, to discover the influence of salinity on N transformation in soil, the incubation time must be considered carefully.
4.3 Saline soil physicochemical properties
4.3.1 SOC, TN, and C/N
SOC, TN, and C/N significantly influenced N mineralization and nitrification in saline soils (Table 2; Table 3). Soil microbial activity and biomass increase with increasing SOC (Elrys et al., 2021a), and soil nitrifying enzyme activity is positively correlated with SOC (Silva et al., 2012). Thus, the lnR of Nit5-7 and Nit14-16 in the high-level group was significantly positively correlated with SOC (p < 0.05). However, the relation between the lnR of Nit5-7 and SOC in the total group fitted the quadratic equation (p < 0.05), and Nit5-7 first increased and then decreased with increasing SOC. This could be because more ammonium was preferentially immobilized by the microbial community under high SOC conditions (Cheng et al., 2017), further causing the decrease of substrate for nitrifiers. This was supported by the relation between the lnR ofNit5-7 and C/N fitting well with the curves of the second-order equation in the total (p < 0.05) and mid-level groups (p < 0.01). The threshold values of C/N were 34.7 and 35.5, which were much higher than the 18 reported by Cheng et al. (2017) in non-saline soils, suggesting microbes require more C source to produce energy and osmoregulatory compounds to withstand the low osmotic potential stress (Elmajdoub and Marschner, 2013). The relation between TN and Nit in low-level saline soils changed with incubation time, showing a positive correlation with the lnR ofNit5-7 (p < 0.01) and a negative correlation with the lnR of Nit14-16 (p < 0.05). Soil TN, which is mostly constituted of organic N, can be directly or indirectly used as a substrate for nitrification (Corre et al., 2010). Simultaneously, soils with a higher TN usually have high microbial biomass and abundance (Elrys et al., 2021b; Cai et al., 2021). Thus, the lnR of Nit5-7significantly increased with increasing TN. The decrease of Nit14-16 with increasing TN maybe caused by the faster consumption of substrates during 0–14/16 days incubation in soils with high TN, and substrate concentrations thereby decreasing with increasing TN, ultimately leading to the decrease of Nit14-16.
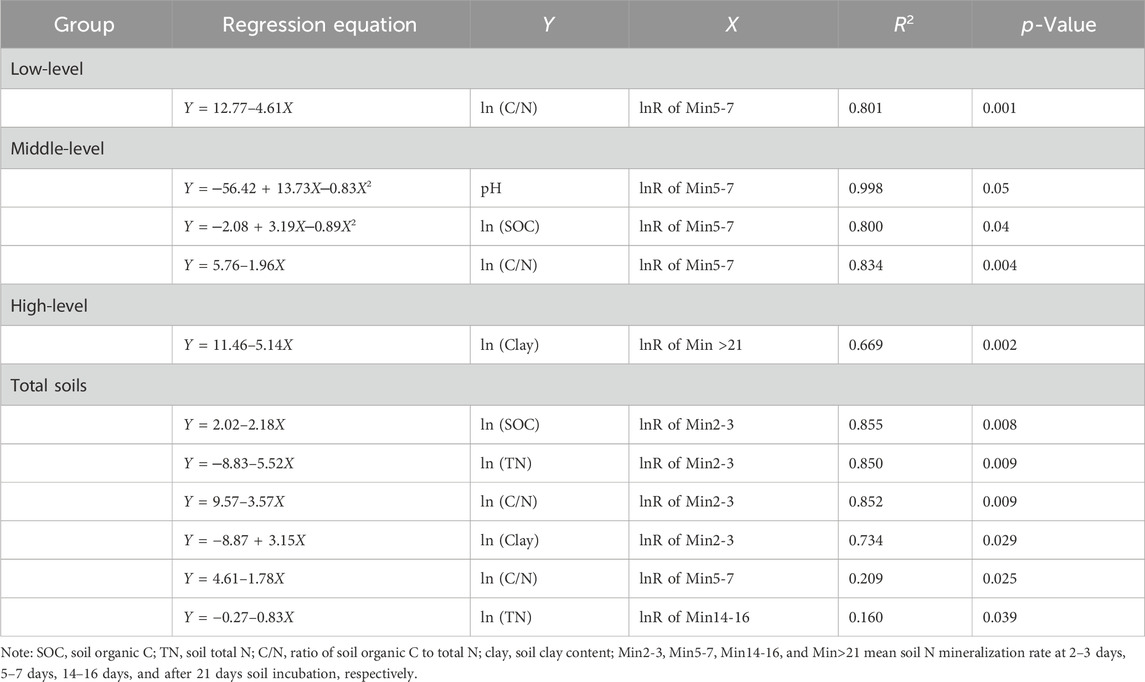
TABLE 3. Curvilinear regression analysis between the net nitrogen mineralization and saline soil properties.
The net N mineralization resulted from two concurrent and opposite microbial processes: gross N mineralization and microbial immobilization. Significantly negative correlations were found between the lnR of Min2-3 and SOC or TN (p < 0.01), and between the lnR of Min14-16 and TN (p < 0.05) in the total group (Table 3), indicating that N immobilization was greater than N mineralization, and rose with increasing SOC and TN. Due to the reduction of exogenous organic matter input, soil salinization is usually coupled with low SOC and TN contents, and the shortage of C and N sources are key factors limited microbial growth (Kamble et al., 2014). Therefore, microbial growth and reproduction can be boosted when the available C and N increase, ultimately reducing Min. The lnR of Min2-3 in the total group and the lnR of Min5-7 in the low-level, mid-level, and total groups were significantly negatively correlated with C/N. Similarly to the nitrification discussed above, more N would have been immobilized with increasing C/N to meet microbial requirements (Wang et al., 2016; Cheng et al., 2019). This also could be confirmed by the relation of the quadratic equation between the lnR of Min5-7and SOC in the mid-level group (p < 0.05), with Min showing an initial increase and then decreasing with increasing SOC.
4.3.2 pH
Interestingly, the effect of pH on nitrification changed with salinity levels (Table 2). In the low-level group, the lnR of Nit14-16 was significantly positively correlated with pH (p < 0.05). In the middle-level group, the relation between the lnR of Nit14–16 and pH showed aparabolic trend (p < 0.05), and the threshold value was 9.1. In the high-level group, the lnR of Nit5-7 was significantly negatively correlated with pH (p < 0.01). Thereby, the effect of pH on nitrification generally shifted from stimulation to inhibition with increasing pH. The positive effect was in line with the finding of Elrys et al. (2021a) in non-saline soils, and might be due to the positive effect of pH on ammonia-oxidizing bacterial abundance and potential nitrification (Zhang et al., 2017). However, a threshold effect was observed in the relation between pH and nitrification, whereby nitrification suppression occurred if the pH exceeded a threshold value, such as 9.1. The potential reasons for nitrification suppression under high pH conditions are: i) higher pH reduced the relative abundance of bacterial genes related to the process of nitrification (Li et al., 2022); ii) soil pH was significantly positively correlated with salinity (r = 0.473, p = 0.013), and salinity increase led to the accumulation of ammonium and high pH, which resulted in a significant increase in NH3 volatilization (Mandal et al., 2016; Zhu et al., 2020), ultimately reducing substrate concentration and Nit.
A threshold effect may also exist in the relationship between N mineralization and soil pH, as supported by the relation of the quadratic equation between the lnR of Min5-7 and pH in the middle-level group (p = 0.05; Table 3). With increasing pH, Min5-7 first increased, and decreased thereafter. The threshold value was 8.1, which was highly similar to the results of Elrys et al. (2021b), where gross N mineralization significantly decreased when pH was higher than 8.0. Under conditions of pH < 8.1, the positive effect of pH on Min might be caused by an increase in the availability of microbial C source (Curtin et al., 1998). The negative effect of high pH on Min may be due to high pH decreasing the enzymatic activities that directly regulate N mineralization, as observed urease activity was negatively influenced by pH in saline soils (Zhang et al., 2014).
4.3.3 Clay
Clay contents in saline soils could influence nitrification mainly related to NH4+ adsorption, and microbial activity and diversity. In the low-level group, the lnR of Nit14-16 was significantly negatively correlated with soil clay content (p < 0.05; Table 2), because fine-textured soils (i.e., those with high clay content) possessed a high cation exchange capacity and adsorbed more NH4+ (Jarecki et al., 2008), leading to the reduction of substrate availability for nitrification. However, the lnR of Nit>21 was significantly positively correlated with soil clay content (p < 0.05). This was likely due to more NH4+being released into soil solution when the substrate concentration decreased, further increasing Nit>21. In addition, the fine-textured saline soil resulted in high microbial biomass and diversity (Zhu et al., 2021), which in turn increased N mineralization and increased the availability of NH4+ (Elrys et al., 2021b). In the high-level group, the lnR of Nit5-7 was significantly positively correlated with soil clay content, suggesting that low microbial activity was the key factor constraining nitrification in severely saline soils.
Soil microbial biomass and compaction play significant roles in controlling N mineralization (Elrys et al., 2021b). In this study, the lnR of Min2-3 in the total group was significantly positively correlated with soil clay content (p < 0.05, Table 3), mainly due to the high microbial biomass and activity in fine-textured saline soils (Zhu et al., 2021). Nevertheless, in the high-level group, a significant negative relationship was observed between clay content and the lnR of Min>21 (p < 0.01). Soil oxygen availability can be reduced with increasing salinity level (Noe et al., 2013), and this would be even more pronounced in soils with high clay content. Ishak et al. (2016) reported that soil microbial activity was reduced by 50%–60% with decreasing soil oxygen and increasing compaction. Meanwhile, soil enzymatic activity, e.g., mineralization-related protease levels, also decreased with increasing compaction (Mishra et al., 2005). Therefore, coarse soil texture was conducive to N mineralization by improving soil aeration in severely saline conditions.
4.4 Limitations and future perspectives
N mineralization and nitrification are vital transformative processes in agricultural soil ecosystems. There is far less information available about N transformation in the context of saline soils compared to non-saline soils, and the gross N turnover (i.e., mineralization, nitrification, denitrification, and immobilization) in saline soils has not been reported thus far. Net mineralization and nitrification involve production and consumption of NO3− and NH4+ concurrently. Thus, this study contained some limitations that impeded elucidation of the direct mechanisms of salinity’s effects on N turnover. Even so, our findings clearly indicated that the effects of salinity on the availability of NO3− (nitrification) and NH4+ (mineralization) changed with incubation time and salinity levels. Such knowledge is greatly important for optimizing N management in saline fields.
According to our results, the residence time of substrates for N mineralization and nitrification clearly increased under saline conditions. Except for the negative influences, stimulatory effects on Min and Nit were found 14–16 days after substrate addition. The differences in N turnover from non-saline soils can guide us to optimize fertilization times for saline soils. In effect, N management plans for saline soils are rare at present, mainly due to the knowledge gap regarding the responses of N transformation to salinization. Besides salinity, C/N, pH, and clay content are also important factors controlling N mineralization and nitrification in saline soils. These factors work by influencing microbial activities and/or substrate availability. Nevertheless, due to lack of sufficient data, soil microbial biomass, diversity, and community composition were not included in this study. Thus, the direct relationships between saline soil biological properties and N turnover remain unclear. Further research is needed on gross N transformation as well as its biomechanisms under different salinity level conditions.
5 Conclusion
This study provides a comprehensive evaluation of salinity and relevant soil variables’ effects on N mineralization and nitrification in upland fields. The findings revealed that the influences of salinity on N turnover changed with salinity level and incubation time. Soil salinization and other easily available variables, such as SOC, TN, C/N, pH, and clay content, might have affected N mineralization and nitrification via directly or indirectly altering microbial activity and substrate availability. The residence time of substrates for N transformation in saline soils significantly increased relative to non-saline soils, and transformation rates changed with salinity level and time, suggesting that N-fertilization time, supply rate, and fertilizer type must be considered seriously when developing N management plans. Further research on gross N transformation and its mechanisms in saline soils should be conducted in future. Such knowledge can help us implement sustainable agricultural production while minimizing N loss in saline fields.
Data availability statement
The original contributions presented in the study are included in the article/Supplementary material, further inquiries can be directed to the corresponding author.
Author contributions
YT: Data curation, Writing–original draft. WX: Conceptualization, Data curation, Writing–review and editing. LX: Data curation, Writing–review and editing. LZ: Data curation, Writing–review and editing. GW: Data curation, Writing–review and editing. XW: Writing–review and editing. CS: Writing–review and editing.
Funding
The author(s) declare financial support was received for the research, authorship, and/or publication of this article. This research was financially supported by the National Natural Science Foundation of China (41877101), the Talent Introduction Program of Qingdao University of Technology (JC2022-003), and the Science and Technology Specific Projects in Agricultural High-tech Industrial Demonstration Area of the Yellow River Delta (2022SZX01).
Conflict of interest
The authors declare that the research was conducted in the absence of any commercial or financial relationships that could be construed as a potential conflict of interest.
Publisher’s note
All claims expressed in this article are solely those of the authors and do not necessarily represent those of their affiliated organizations, or those of the publisher, the editors and the reviewers. Any product that may be evaluated in this article, or claim that may be made by its manufacturer, is not guaranteed or endorsed by the publisher.
References
Al-Rashidi, R. K., and Al-Jabri, M. M. (1990). Nitrification and urea hydrolysis in amended calcareous saline soils. Arid. Soil Res. Rehabil. 4 (4), 243–252. doi:10.1080/15324989009381255
Awadelkarim, A. H., Gorashi, Y. E., and Ei-Tilip, A. M. A. (1995). Effect of soil salinity and sodicity on urea hydrolysis in three soil orders. U. K. J. Agric. Sci. 3 (1), 60–74.
Bai, J., Gao, H., Xiao, R., Wang, J., and Huang, C. (2012). A review of soil nitrogen mineralization as affected by water and salt in coastal wetlands: issues and methods. Clean-Soil Air Water 40 (10), 1099–1105. doi:10.1002/clen.201200055
Brierley, E. D. R., and Wood, M. (2001). Heterotrophic nitrification in an acid forest soil: isolation and characterisation of a nitrifying bacterium. Soil Biol. Biochem. 33 (10), 1403–1409. doi:10.1016/S0038-0717(01)00045-1
Cai, F., Luo, P., Yang, J., Irfan, M., Zhang, S., An, N., et al. (2021). Effect of long-term fertilization on ammonia-oxidizing microorganisms and nitrification in brown soil of northeast China. Front. Microbiol. 11, 622454. doi:10.3389/fmicb.2020.622454
Cheng, Y., Wang, J., Chang, S. X., Cai, Z., Müller, C., and Zhang, J. (2019). Nitrogen deposition affects both net and gross soil nitrogen transformations in forest ecosystems: a review. Environ. Pollut. 244, 608–616. doi:10.1016/j.envpol.2018.10.054
Cheng, Y., Wang, J., Wang, J., Chang, S. X., and Wang, S. (2017). The quality and quantity of exogenous organic carbon input control microbial NO3− immobilization: a meta-analysis. Soil Biol. Biochem. 115, 357–363. doi:10.1016/j.soilbio.2017.09.006
Corre, M. D., Veldkamp, E., Arnold, J., and Wright, S. J. (2010). Impact of elevated N input on soil N cycling and losses in old-growth lowland and montane forests in Panama. Ecology 91 (6), 1715–1729. doi:10.1890/09-0274.1
Cortes-Lorenzo, C., Rodriguez-Diaz, M., Sipkema, D., Juarez-Jimenez, B., Rodelas, B., Smidt, H., et al. (2015). Effect of salinity on nitrification efficiency and structure of ammonia-oxidizing bacterial communities in a submerged fixed bed bioreactor. Chem. Eng. J. 266, 233–240. doi:10.1016/j.cej.2014.12.083
Curtin, D., Campbell, C., and Jalil, A. (1998). Effects of acidity on mineralization: pH-dependence of organic matter mineralization in weakly acidic soils. Soil Biol. Biochem. 30 (1), 57–64. doi:10.1016/S0038-0717(97)00094-1
Dendooven, L., Alcantara-Hernandez, R. J., Valenzuela-Encinas, C., Luna-Guido, M., Perez-Guevara, F., and Marsch, R. (2010). Dynamics of carbon and nitrogen in an extreme alkaline saline soil: a review. Soil Biol. Biochem. 42 (6), 865–877. doi:10.1016/j.soilbio.2010.02.014
Duan, M., House, J., Liu, Y., and Chang, S. (2018). Contrasting responses of gross and net nitrogen transformations to salinity in a reclaimed boreal forest soil. Biol. Ferti. Soils 54, 385–395. doi:10.1007/s00374-018-1268-7
Elmajdoub, B., and Marschner, P. (2013). Salinity reduces the ability of soil microbes to utilise cellulose. Biol. Ferti. Soils 49 (4), 379–386. doi:10.1007/s00374-012-0734-x
Elrys, A. S., Ali, A., Zhang, H., Cheng, Y., Zhang, J., Cai, Z. C., et al. (2021b). Patterns and drivers of global gross nitrogen mineralization in soils. Glob. Chang. Biol. 27 (22), 5950–5962. doi:10.1111/gcb.15851
Elrys, A. S., Wang, J., Metwally, M. A. S., Cheng, Y., Zhang, J. B., Cai, Z. C., et al. (2021a). Global gross nitrification rates are dominantly driven by soil carbon-to-nitrogen stoichiometry and total nitrogen. Glob. Chang. Biol. 27 (24), 6512–6524. doi:10.1111/gcb.15883
Erickson, J. E., Megonigal, J. P., Peresta, G., and Drake, B. G. (2007). Salinity and sea level mediate elevated CO2 effects on C3-C4 plant interactions and tissue nitrogen in a Chesapeake Bay tidal wetland. Glob. Chang. Biol. 13 (1), 202–215. doi:10.1111/j.1365-2486.2006.01285.x
Ichihashi, Y., Date, Y., Shino, A., Shimizu, T., Shibata, A., Kumaishi, K., et al. (2020). Multi-omics analysis on an agroecosystem reveals the significant role of organic nitrogen to increase agricultural crop yield. Proc. Nat. Acad. Sci. 117 (25), 14552–14560. doi:10.1073/pnas.1917259117
Irshad, M., Honna, T., Yamamoto, S., Eneji, A. E., and Yamasaki, N. (2005). Nitrogen mineralization under saline conditions. Commun. Soil Sci. Plant Anal. 36 (11-12), 1681–1689. doi:10.1081/css-200059116
Ishak, L., McHenry, M. T., and Brown, P. H. (2016). Soil compaction and its effects on soil microbial communities in Capsicum growing soil. Acta Hortic. 1123, 123–130. doi:10.17660/ActaHortic.2016.1123.17
Jarecki, M. K., Parkin, T. B., Chan, A. S. K., Hatfield, J. L., and Jones, R. (2008). Greenhouse gas emissions from two soils receiving nitrogen fertilizer and swine manure slurry. J. Environ. Qual. 37 (4), 1432–1438. doi:10.2134/jeq2007.0427
Kamble, P. N., Gaikwad, V. B., Kuchekar, S. R., and Bååth, E. (2014). Microbial growth, biomass, community structure and nutrient limitation in high pH and salinity soils from Pravaranagar (India). Eur. J. Soil Biol. 65, 87–95. doi:10.1016/j.ejsobi.2014.10.005
Kooijman, A. M., Van Mourik, M., and Schilder, M. L. M. (2009). The relationship between N mineralization or microbial biomass N with micromorphological properties in beech forest soils with different texture and pH. Biol. Ferti. Soils 45 (5), 449–459. doi:10.1007/s00374-009-0354-2
Kuypers, M. M. M., Marchant, H. K., and Kartal, B. (2018). The microbial nitrogen-cycling network. Nat. Rev. Microbiol. 16, 263–276. doi:10.1038/nrmicro.2018.9
Lang, E., and Jagnow, G. (1986). Fungi of a forest soil nitrifying at low pH values. FEMS Microbiol. Ecol. 38 (5), 257–265. doi:10.1016/0378-1097(86)90001-7
Laura, R. D. (1974). Effects of neutral salts on carbon and nitrogen mineralisation of organic matter in soil. Plant Soil 41, 113–127. doi:10.1007/BF00017949
Laura, R. D. (1977). Salinity and nitrogen mineralization in soil. Soil Biol. biochem. 9 (5), 333–336. doi:10.1016/0038-0717(77)90005-0
Li, H., Yao, R., Yang, J., Wang, X., Zheng, F., Chen, Q., et al. (2020b). Influencing mechanism of soil salinization on nitrogen transformation processes and efficiency improving methods for high efficient utilization of nitrogen in salinized farmland. Chin. J. Appl. Ecol. 31 (11), 3915–3924. (in Chinese). doi:10.13287/j.1001-9332.202011.023
Li, Y., Hu, Y., Yan, C., Xiong, J., and Qiu, Q. (2022). pH and salinity are the dominant limiting factors for the application of mariculture sludge to paddy soil. Appl. Soil Ecol. 175, 104463. doi:10.1016/j.apsoil.2022.104463
Li, Y., Xu, J., Liu, S., Qi, Z., Wang, H., Wei, Q., et al. (2020d). Salinity-induced concomitant increases in soil ammonia volatilization and nitrous oxide emission. Geoderma 361, 114053. doi:10.1016/j.geoderma.2019.114053
Li, Y., Xu, J., Liu, X., Qi, Z., Wang, H., Li, Y., et al. (2020c). Nitrification inhibitor DMPP offsets the increase in N2O emission induced by soil salinity. Biol. Fertil. Soils 56 (8), 1211–1217. doi:10.1007/s00374-020-01490-9
Li, Y., Xu, J., Wei, Q., Bai, W., Li, K., and Liu, X. (2018). Soil nitrification process under different soil moisture and salinity conditions. J. Drain. Irrig. Mach. Eng. 36 (9), 909–913. (in Chinese). doi:10.3969/j.issn.1674-8530.18.1063
Li, Z., Zeng, Z., Tian, D., Wang, J., Fu, Z., Zhang, F., et al. (2020a). Global patterns and controlling factors of soil nitrification rate. Glob. Chang. Biol. 26 (7), 4147–4157. doi:10.1111/gcb.15119
López-Valdez, F., Fernández-Luquenñ, O. F., Luna-Guido, M. L., Marsch, R., Olalde-Portugal, V., and Dendooven, L. (2010). Microorganisms in sewage sludge added to an extreme alkaline saline soil affect carbon and nitrogen dynamics. Appl. Soil Ecol. 45 (3), 225–231. doi:10.1016/j.apsoil.2010.04.009
Magalhaes, C. M., Joye, S. B., Moreira, R. M., Wiebe, W. J., and Bordalo, A. A. (2005). Effect of salinity and inorganic nitrogen concentrations on nitrification and denitrification rates in intertidal sediments and rocky biofilms of the Douro River estuary, Portugal. Water Res. 39 (9), 1783–1794. doi:10.1016/j.watres.2005.03.008
Mandal, S., Thangarajan, R., Bolan, N. S., Sarkar, B., Khan, N., Ok, Y. S., et al. (2016). Biochar-induced concomitant decrease in ammonia volatilization and increase in nitrogen use efficiency by wheat. Chemosphere 142, 120–127. doi:10.1016/j.chemosphere.2015.04.086
Martens-Habbena, W., Berube, P. M., Urakawa, H., De La Torre, J. R., and Stahl, D. A. (2009). Ammonia oxidation kinetics determine niche separation of nitrifying Archaea and Bacteria. Nature 461 (7266), 976–979. doi:10.1038/nature08465
Mcclung, G., and Frankenberger, W. T. (1987). Nitrogen mineralization rates in salinevs. salt-amended soils. Plant Soil 104, 13–21. doi:10.1007/BF02370619
McCormick, R. W., and Wolf, D. C. (1980). Effect of sodium chloride on CO2 evolution, ammonification, and nitrification in a Sassafras Sandy loam. Soil Biol. Biochem. 12 (2), 153–157. doi:10.1016/0038-0717(80)90052-8
Mishra, S., Di, H. J., Cameron, K. C., Monaghan, R., and Carran, A. (2005). Gross nitrogen mineralisation rates in pastural soils and their relationships with organic nitrogen fractions, microbial biomass and protease activity under glasshouse conditions. Biol. Ferti. Soils 42 (1), 45–53. doi:10.1007/s00374-005-0863-6
Noe, G. B., Krauss, K. W., Lockaby, B. G., Conner, W. H., and Hupp, C. R. (2013). The effect of increasing salinity and forest mortality on soil nitrogen and phosphorus mineralization in tidal freshwater forested wetlands. Biogeochemistry 114 (1-3), 225–244. doi:10.1007/s10533-012-9805-1
Nugroho, R. A., Roling, W. F. M., Laverman, A. M., and Verhoef, H. A. (2007). Low nitrification rates in acid Scots pine forest soils are due to pH-related factors. Microb. Ecol. 53 (1), 89–97. doi:10.1007/s00248-006-9142-9
Pathak, H., and Rao, D. L. N. (1998). Carbon and nitrogen mineralization from added organic matter in saline and alkali soils. Soil Biol. Biochem. 30 (6), 695–702. doi:10.1016/s0038-0717(97)00208-3
Raiesi, F., Razmkhah, M., and Kiani, S. (2018). Salinity stress accelerates the effect of cadmium toxicity on soil N dynamics and cycling: does joint effect of these stresses matter? Ecotoxicol. Environ. Saf. 153, 160–167. doi:10.1016/j.ecoenv.2018.01.035
Rengasamy, P. (2006). “Soil salinity and sodicity,” in Growing crops with reclaimed wastewater. Editor D. Stevens (Victoria: CSIRO), 125–138.
Rosenberg, M. S., Adams, D. C., and Gurevitch, J. (2000). MetaWin: statistical software for meta analysis. Sunderland, MA, USA: Sinauer Associates. Version 2.
Silva, M. C. P. E., Poly, F., Guillaumaud, N., Van Elsas, J. D. V., and Salles, J. F. (2012). Fluctuations in ammonia oxidizing communities across agricultural soils are driven by soil structure and pH. Front. Microbiol. 3 (77), 77. doi:10.3389/fmicb.2012.00077
Sun, H., Zhang, J., Xu, H., Chen, G., and Wang, L. (2016). Variations of soil microbial community composition and enzyme activities with different salinities on Yuyao coast, Zhejiang, China. Chin. J. Appl. Ecol. 27 (10), 3361–3370. (in Chinese). doi:10.13287/j.1001-9332.201610.034
Tao, J., Yang, J., Yao, R., Wang, X., Liu, G., and Chen, Q. (2020). Effects of soil salinity on nitrogen transformation in hetao irrigation district of inner Mongolia, China. Soils 52 (4), 802–810. (in Chinese). doi:10.13758/j.cnki.tr.2020.04.021
Van Groenigen, K. J., Qi, X., Osenberg, C. W., Luo, Y. Q., and Hungate, B. A. (2014). Faster decomposition under increased atmospheric CO2 limits soil carbon storage. Science 344 (6183), 508–509. doi:10.1126/science.1249534
Wang, J., Cheng, Y., Zhang, J. B., Müller, C., and Cai, Z. C. (2016). Soil gross nitrogen transformations along a secondary succession transect in the north subtropical forest ecosystem of southwest China. Geoderma 280, 88–95. doi:10.1016/j.geoderma.2016.06.016
Wicke, B., Smeets, E., Dornburg, V., Vashev, B., Gaiser, T., Turkenburg, W., et al. (2011). The global technical and economic potential of bioenergy from salt-affected soils. Energy Environ. Sci. 4 (8), 2669–2681. doi:10.1039/c1ee01029h
Wieder, W. R., Cleveland, C. C., Smith, W. K., and Todd-Brown, K. (2015). Future productivity and carbon storage limited by terrestrial nutrient availability. Nat. Geosci. 8 (6), 441–444. doi:10.1038/ngeo2413
Wong, V. N. L., Dalal, R. C., and Greene, R. S. B. (2008). Salinity and sodicity effects on respiration and microbial biomass of soil. Biol. Fertil. Soils 44 (7), 943–953. doi:10.1007/s00374-008-0279-1
Wong, V. N. L., Greene, R. S. B., Dalal, R. C., and Murphy, B. W. (2010). Soil carbon dynamics in saline and sodic soils: a review. Soil Use Manag. 26 (1), 2–11. doi:10.1111/j.1475-2743.2009.00251.x
Xie, W., Wu, L., Wang, J., Zhang, Y., and Ouyang, Z. (2017). Effect of salinity on the transformation of wheat straw and microbial communities in a saline soil. Commun. Soil Sci. Plant Anal. 48 (12), 1455–1461. doi:10.1080/00103624.2017.1373787
Yang, J., Yao, R., Wang, X., Xie, W., Zhang, X., Zhu, W., et al. (2022). Research on salt-affected soils in China: history, status and prospect. Acta Pedol. Sin. 59 (1), 10–27. (in Chinese). doi:10.11766/trxb202110270578
Yao, R., Li, H., Yang, J., Yin, C., Wang, X., Xie, W., et al. (2021). Interactive Effects of amendment materials and soil salinity on net rates of urea hydrolysis and nitrification in salt-affected soil. J. Soil Sci. Plant Nutr. 21 (7), 3414–3427. doi:10.1007/s42729-021-00616-8
Zeng, W., Xu, C., Wu, J., Huang, J., and Tao, M. (2013). Effect of salinity on soil respiration and nitrogen dynamics. Ecol. Chem. Eng. S. 20 (3), 519–530. doi:10.2478/eces-2013-0039
Zeng, Y., Xiang, W., Deng, X., Fang, X., Liu, C., and Peng, C. (2014). Soil N forms and gross transformation rates in Chinese subtropical forests dominated by different tree species. Plant Soil 384 (1–2), 231–242. doi:10.1007/s11104-014-2206-6
Zhang, H. X., Irving, L. J., McGill, C., Matthew, C., Zhou, D. W., and Kemp, P. (2010). The effects of salinity and osmotic stress on barley germination rate: sodium as an osmotic regulator. Ann. Bot-london 106 (6), 1027–1035. doi:10.1093/aob/mcq204
Zhang, J. B., Sun, W. J., Zhong, W. H., and Cai, Z. C. (2014). The substrate is an important factor in controlling the significance of heterotrophic nitrification in acidic forest soils. Soil Biol. Biochem. 76, 143–148. doi:10.1016/j.soilbio.2014.05.001
Zhang, M. M., Alves, R. J. E., Zhang, D. D., Han, L. L., He, J. Z., and Zhang, L. M. (2017). Time-dependent shifts in populations and activity of bacterial and archaeal ammonia oxidizers in response to liming in acidic soils. Soil Biol. Biochem. 112, 77–89. doi:10.1016/j.soilbio.2017.05.001
Zhang, W., Wang, C., Xue, R., and Wang, L. (2019). Effects of salinity on the soil microbial community and soil fertility. J. Integr. Agric. 18 (6), 1360–1368. doi:10.1016/s2095-3119(18)62077-5
Zhou, M. H., Butterbach-Bahl, K., Vereecken, H., and Bruggemann, N. (2017). A meta-analysis of soil salinization effects on nitrogen pools, cycles and fluxes in coastal ecosystems. Glob. Chang. Biol. 23 (3), 1338–1352. doi:10.1111/gcb.13430
Zhu, H., Yang, J., Li, Y., Liu, X., Jin, H., Li, J., et al. (2022). Effects of soil salinity on nitrification and ammonia-oxidizing microorganisms in coastal reclaimed farmland soil. J. Soil Sci. Plant Nutr. 22 (2), 2743–2754. doi:10.1007/s42729-022-00841-9
Zhu, H., Yang, J., Yao, R., Wang, X., Xie, W., Zhu, W., et al. (2020). Interactive effects of soil amendments (biochar and gypsum) and salinity on ammonia volatilization in coastal saline soil. Catena 190, 104527. doi:10.1016/j.catena.2020.104527
Keywords: salinity level, N turnover, soil properties, N management, meta-analysis
Citation: Tao Y, Xie W, Xu L, Zhang L, Wang G, Wang X and Shi C (2024) The characteristics of soil salinization effects on nitrogen mineralization and nitrification in upland fields. Front. Environ. Sci. 12:1369554. doi: 10.3389/fenvs.2024.1369554
Received: 12 January 2024; Accepted: 05 February 2024;
Published: 14 February 2024.
Edited by:
Jifu Ma, Yan’an University, ChinaReviewed by:
Fayuan Wang, Qingdao University of Science and Technology, ChinaJiakun Yan, Yulin University, China
Shihong Yang, Hohai University, China
Copyright © 2024 Tao, Xie, Xu, Zhang, Wang, Wang and Shi. This is an open-access article distributed under the terms of the Creative Commons Attribution License (CC BY). The use, distribution or reproduction in other forums is permitted, provided the original author(s) and the copyright owner(s) are credited and that the original publication in this journal is cited, in accordance with accepted academic practice. No use, distribution or reproduction is permitted which does not comply with these terms.
*Correspondence: Wenjun Xie, eGlld2VuanVuQHF1dC5lZHUuY24=, eHdqZXJpY0AxNjMuY29t