- Gansu Analysis and Research Center, Lanzhou, China
The cultivated Echinochloa frumentacea (Roxb.) Link and Echinochloa crusgalli (L.) Beauv. var. mitis (Pursh) Peter are two valuable grass species that are widely used in improving saline-alkali soil. Here we conducted a pot experiment combined with roots morphological analysis to investigate the adaptability of grass roots to saline stress environments, with cultivated E. frumentacea and E. crusgalli being subjected to salt treatments of 0 (CK), 100, 220, and 340 mmoL·L−1. Results indicated that E. frumentacea had longer primary roots with fewer root hairs and lower local branching density than E. crusgalli, with the root volume of E. frumentacea being 1.43 times greater than that of E. crusgalli. The aboveground biomass of both grasses decreased significantly (p < 0.05) with increasing salt concentrations, whereas the root-to-shoot ratio exhibited the opposite trend, suggesting the preferential allocation of photosynthetic products to the roots under salt stress. The total length, surface area, and tip number of fine roots and the growth of coarse roots (d > 2.00 mm) showed significant differences (p < 0.05) between the two grass species. Different concentrations of salt stress had inconsistent effects on the biomass and radial growth of roots for grasses. The cultivated E. frumentacea seems to adopt an adapt strategy of gradually increasing its root thickness, root hairs, and root density under increasing salt stress. E. crusgalli, on the other hand, employed a strategy of increasing root length, maintaining uniform thickness, and developing root hairs.
1 Introduction
Saline-alkali soil is widely distributed worldwide, covering nearly 954 million hectares of global land (Wang et al., 2017), and its extent and area continue to expand due to anthropogenic activities and climate change, etc. (Mukhopadhyay et al., 2021). In China, saline-alkali land occupies 36.9 million hectares (Yang et al., 2022a), and is regarded as a major type of marginal land, and therefore exploring its application plays a crucial role in ensuring China’s food security (Cao et al., 2022). As most crops and forage grasses are salt-sensitive and have limited genetic potentials for salt resistance, and soils with high salt concentrations are detrimental to them by causing osmotic stress, ion toxicity, and oxidative stress. This severely restricts the sustainable development of agriculture and animal husbandry, as well as ecological environment construction (Guo et al., 2020; Guo et al., 2022).
Crop roots are sensitive to soil stress, and saline-alkali soil primarily affects the physiological and morphological changes of roots, with distinct mechanisms for salt and alkali stress (Tong et al., 2012). Research indicates that roots can adapt to adverse environments by altering their morphology and distribution under stress conditions (Jia et al., 2008). In addition, root development determines one of the most direct adaptive characteristics of plants, including root size, adaptation to saline environments, and the absorption and utilization of soil nutrients (Farooq et al., 2015). Therefore, the growth, development, and vitality of roots are crucial for a plant’s ability to tolerate salt stress. Chen et al. (2019) found that low concentrations of neutral salts and alkaline salts are conducive to the increased length, surface area, and volume of fine roots in different salt-tolerant varieties of Sea Island cotton (Gossypium barbadense L.) to varying degrees, with no significant inhibitory effect on medium and coarse roots. Zhang et al. (2014) indicated significant differences in root length, root surface area, root volume, and average diameter of cotton at different salt levels. Furthermore, there are significant genotype differences in both root length and root surface area. Studying the root morphology of Sophora alopecuroides seedlings under salt stress, Zhang et al. (2020) demonstrated that low salt concentration (0.3%) favors the growth of S. alopecuroides roots; however, when the salt concentration reached 0.9%, its growth seemed to be significantly inhibited. In saline-alkali land in arid regions, the root system of forage grass adopts a morphological adaptation strategy to resist saline-alkali stress and enhance its own resistance. This is mainly manifested by the rapid expansion of the root system to deeper soil layers, and within a horizontal distance of 0–15 cm from the plant, the inner roots are dense and show rapidly increasing (Yang et al., 2022b). Furthermore, studies have shown that salt stress significantly alters root hair traits and root surface area in two rapeseed varieties (BARI Sarisha-8 and Binasarisha-5) (Arif et al., 2019). Therefore, different crops exhibit varying levels of stress resistance and adaptability to saline-alkali stress.
Echinochloa frumentacea (Roxb.) Link and Echinochloa crusgalli (L.) Beauv. var. mitis (Pursh) Peter belong to the Poaceae family as members of the Echinochloa genus. They are widely cultivated in the arid and semi-arid regions of northwestern China for improving saline-alkali land. They have high nutritional value and strong reproductive capabilities, with a dry hay yield of 2.29 × 104 kg hm−2 and 2.12 × 104 kg hm−2, respectively, making them important dual-purpose crops (Wu et al., 2002; Zhang et al., 2018). Recent research mainly focused on the effects of saline-alkali stress on seed germination, chlorophyll content, malondialdehyde content, and antioxidant enzyme activity in E. frumentacea (Lu et al., 2021; Lan et al., 2022). Additionally, several studies have investigated its nutrient absorption, productivity, and biological improvement of saline-alkali environments (Jing et al., 2020; Jia et al., 2023). However, limited research has been done on revealing the tolerance and adaptability of the root systems of the two grasses to salt stress. Therefore, this study selected the two Poaceae forage grasses as research subjects, and analyzed the growth status and morphological changes of their root systems under different salt concentrations. The study aims to explore the adaptability of their root systems under salt stress, and is expected to provide a theoretical basis for advancing the breeding of Poaceae forage grasses and better utilizing the widely distributed resources of Poaceae forage grasses.
2 Materials and methods
2.1 Experimental materials
In this study, the experimental materials included two grass species from the Poaceae family, i.e., E. frumentacea and E. crusgalli. The seeds of the two grass species were provided by Ningxia Xibei Agriculture, Forestry, Animal Husbandry, and Ecological Technology Co., Ltd. The thousand-grain weight was 3.82 g for E. frumentacea and 4.17 g for E. crusgalli. The seeds were insect-free, and the seed surfaces were undamaged.
2.2 Experimental design
The experiment adopted a soil salinity control test, designed based on the main salt characteristics of saline-alkali soil in the northern irrigation area of Ningxia, China: NaCl and Na2SO4, as well as the salt tolerance thresholds of E. frumentacea and E. crusgalli: 495.33 mmoL·L−1 and 387.09 mmoL·L−1 respectively. (Lan, 2023). The salt composition was a neutral mixed salt of NaCl + Na2SO4. NaCl and Na2SO4 were prepared in a 1:1 M concentration mixture to simulate salt stress environment. Four concentrations of salt, namely, 0 (CK), 100, 220, and 340 mmoL L−1, were set up for treatment. Potting was carried out in the greenhouse of Ningxia University’s agricultural training base from April to June 2023. The temperature in the greenhouse ranged from 22°C to 27°C. The pots were 2-gallon in size (20 cm high, with top and bottom diameters of 21.3 cm and 16 cm, respectively), filled with 1.2 kg (dry weight) of “PINDSTRUP” substrate soil (0–6 mm; mean 2.3 mm). Thirty fully filled and sterilized seeds were evenly sown in each pot, watered regularly, and the soil moisture content was maintained at 70% ± 5% (Qiu et al., 2016). After all seeds were germinated, 20 seedlings were planted in each pot, and after growing for 22 days (with 4 leaves and 1 core), salt stress treatment was applied. Before treatment, the soil moisture content was controlled at 30% ± 5% to facilitate the diffusion of the salt solution in the soil. Four treatment groups were set up (each with 3 replicates) at concentrations of 0 (CK), 100, 220, and 340 mmoL·L−1. Corresponding salt stress solutions (pure water for the control) were irrigated in four groups to avoid salt shock reactions. The stress solutions were added to pots twice a day (at 9:00 and 18:00, respectively) for 2 days, with 250 mL for each time, totaling 1 L of stress solution per pot. A tray was placed at the bottom of each pot to prevent salt leakage, and any leakage was promptly returned to the pot. After the stress period, the lost moisture was replenished daily using the weighing method to maintain the soil moisture content at 70% ± 5%. Samples were taken for different parameter measurements after 8 days of treatment.
2.3 Measurement of parameters
2.3.1 Biomass
Three randomly selected plants from the replicates were used for biomass determination. The above-ground stems and leaves and the below-ground root systems were separated from the root neck, placed in an oven, and dried at 105°C for 30 min to kill out. Subsequently, they were dried at 75°C to a constant weight, and the dry weight was measured using an electronic balance.
2.3.2 Root morphology
Roots were taken out from the pots, which were then gently washed with running water to remove soil, and water drops on the root surface were removed with absorbent paper. Three evenly grown plant roots were chosen for analysis. The root morphology scanning employed an EPSON Scan 2 Photo Scanner for 8-bit grayscale scanning (resolution of 400 dpi). The obtained grayscale images of the roots were analyzed using the EPSON Expression 12000XL 2.2 V6.2 6.2.2 root analysis system. Data for root-related parameters such as total length, average diameter, total surface area, total volume, root tip number, and crossing number were obtained.
2.3.3 Soil indicators
Soil samples from the pots were collected, air-dried, and passed through a 2 mm sieve. The soluble total salt content was determined by the weight method. Soil electrical conductivity (EC) and pH were measured using a P11 conductivity meter (Shanghai Youke Instrument & Meter Co., Ltd.) and a PHS-3C acidity meter (Shanghai Precision & Scientific Instrument Co., Ltd.), respectively. For EC testing, the ratio of water to soil was 5:1, and for pH testing, the ratio of water to soil was 2.5:1.
2.4 Data analysis
Data analysis and plotting were performed using GraphPad Prism 10. The error bars in the figures represent mean ± standard deviation (mean ± SD). Variance analysis was carried out using ANOVA or 2-way ANOVA, and Tukey’s multiple comparisons test or Šídák’s multiple comparisons test was employed. Correlation analysis and Principal Component Analysis (PCA) were performed on ten variables, including aboveground biomass, root biomass, root-to-shoot ratio, root length, root average diameter, root surface area, root volume, root tip number, branch number, and crossing number, under different concentrations of salt stress for both studied grasses.
3 Results
3.1 Soil electrical conductivity, pH, and soluble salt content under different salt concentrations for two types of Pasture grasses
Under different concentrations of salt stress treatments, the salt content in the soil of root layer of E. frumentacea and E. crusgalli conforms to the increasing concentration of experimental treatments (Table 1). Soluble salt content and electrical conductivity gradually increased, with significant differences observed between the control and each group (p < 0.001). The pH remained neutral or slightly acidic.
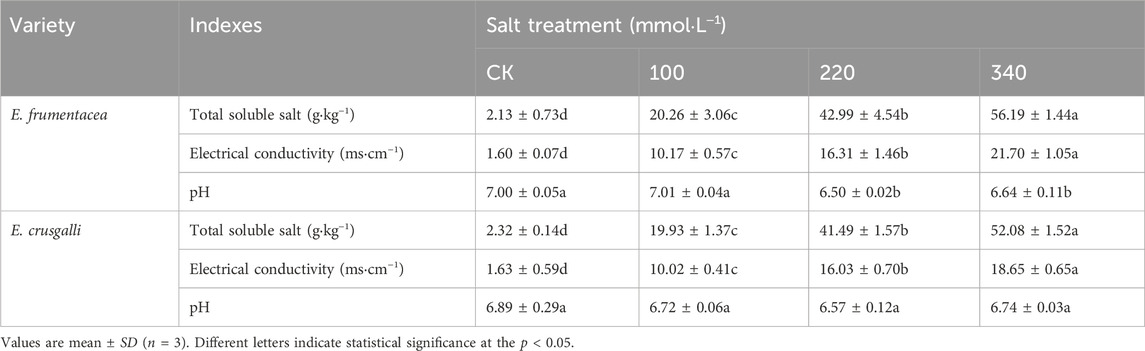
Table 1. Soil conductivity, pH and soluble total salt content of two kinds of forage treated with different concentrations of salt.
3.2 Aboveground and root biomass accumulation of two types of Pasture grasses under different salt stress concentrations
With the increasing of salt treatment concentration, the aboveground biomass of E. frumentacea and E. crusgalli gradually decreased, with significant differences observed between the control and treatment groups (p < 0.05) (Figure 1A,D); there were no significant differences in root biomass between the control and treatment groups; the root-to-shoot ratio showed a gradually increasing trend, with significant differences observed compared to other groups in the 340 mmol L−1 treatment (p < 0.05).
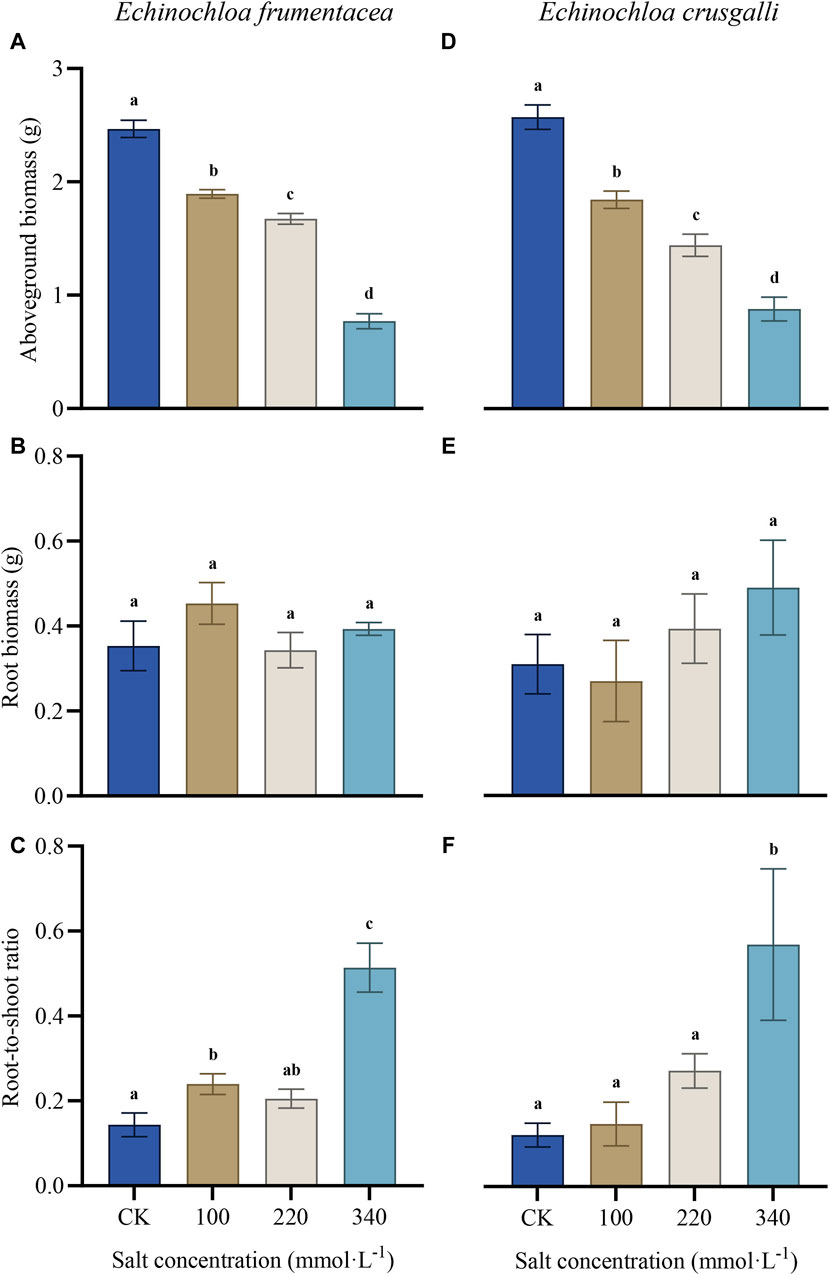
Figure 1. Effects of salt treatment with different concentrations on aboveground biomass, root biomass and root-to-shoot ratio of E. frumentacea and E. crusgalli, respectively. Values are mean ± SD (n = 3). Different letters indicate statistical significance at the p < 0.05 between each group.
3.3 Root morphological characteristics of two types of foraging grasses under different salt stress concentrations
Root scanning indicated (Table 2) that with increasing salt concentration, the root length, surface area, tip number, fork number, and crossing number of the root system of E. frumentacea initially increased and then decreased, and significant differences were found between the control and each treatment group (p < 0.05). No significant differences were detected in the average diameter between the control and treatments. For E. crusgalli, the root length, surface area, tip number, and fork number initially decreased and then increased with increasing salt concentration, and significant differences were found between the control and each treatment group (p < 0.05). The average diameter gradually increased with increasing salt concentration, and significant differences were detected between the 340 mmol L−1 salt treatment and other groups (p < 0.05).
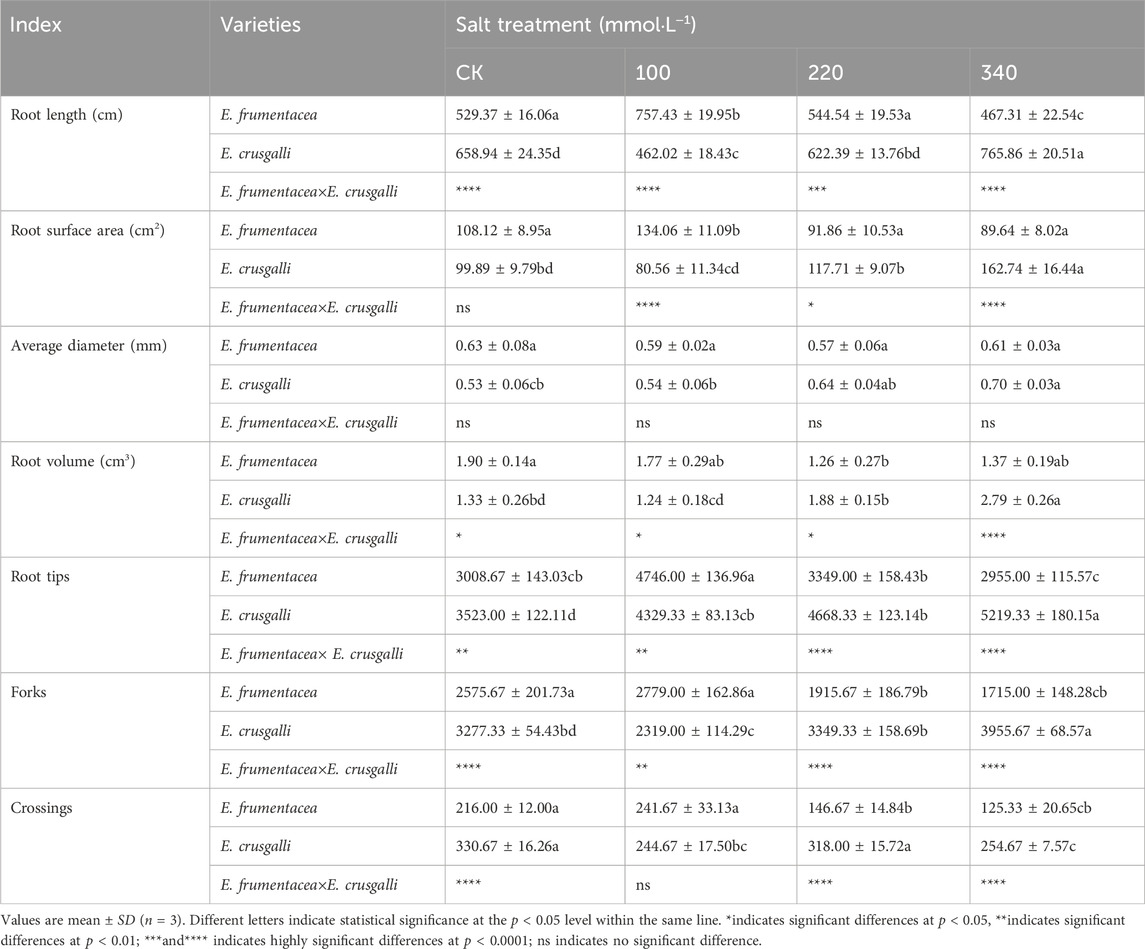
Table 2. Comparison of root length, root surface area, root average diam, root volume, root tips, forks and crossings between E. frumentacea and E. crusgalli under different salt concentrations.
Table 2 made a comparison of root morphology of the two types of forage grasses, and the characteristics obtained from root system image scans were shown in Figure 2. The fibrous root systems of both grasses consisting of main roots and root hairs grew laterally. In the control group, the main roots of E. frumentacea gradually tapered from the stem end to the root tip, exhibiting a longer length, fewer root hairs, and lower local branching density (Figure 2). Additionally, there were significant differences in root volume between the two grasses (p < 0.05), with that of E. frumentacea being 1.43 times larger than E. crusgalli, characterized by fewer but thicker roots (Table 2). The root system of E. crusgalli exhibited shorter growth, with a uniform thickness from the stem apex to the root tip, abundant root hairs, and a high local branching density. The root system of E. crusgalli was well-developed, with a significantly greater root mass than that of E. frumentacea (p < 0.05). The total root length, number of root tips, number of forks, and number of crossings in the root system of E. crusgalli were observed to be 1.24, 1.17, 1.27, and 1.53 times greater than those of E. frumentacea, respectively. (Table 2).
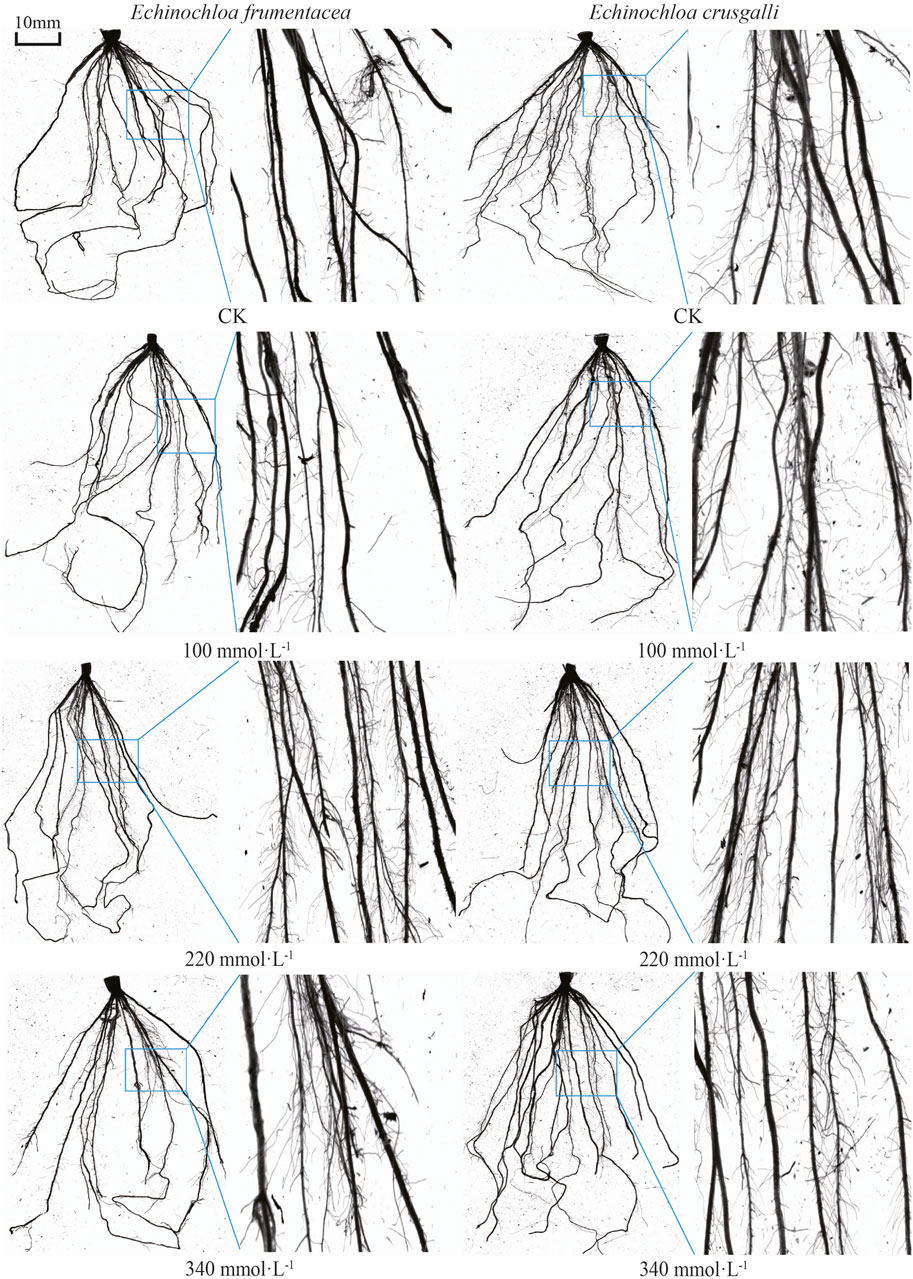
Figure 2. Root scanning images of two forage species under different concentration of salt treatment.
With increasing salt concentration, the root system of E. frumentacea gradually thickened, and the number of root hairs increased, resulting in increased root system density (Figure 2; E. frumentacea at 100, 220 and 340 mmol L-1). The root system of E. crusgalli became longer and thinner, maintaining uniform thickness, but with little change in the root hair zone. The density of root hairs remained high and well-developed under different concentrations of salt stress (Figure 2; E. crusgalli at 100, 220 and 340 mmol L−1). The interaction between grass species and salt stress had no significant effect on the average root diameter, but exerted a significant impact on root total length, root surface area, root volume, tip number, and fork number (p < 0.05) (Table 2).
The root systems of both grasses were classified based on average root diameter (mm) into five categories: (0.00, 0.50], (0.50, 1.00], (1.00, 1.50], (1.50, 2.00], and d > 2.00. A classification analysis was then conducted for total root length, root surface area, root volume, and total root tip number (Figure 3). With the increasing salt concentration, significant differences were observed between the control and treatment groups for the total root length in the (0.00, 0.50], (0.50, 1.00], and (1.00, 1.50] mm diameter categories for both E. frumentacea and E. crusgalli (p < 0.05). Significant differences were also observed in root surface area between the (0.50, 1.00] and (1.00, 1.50] mm diameter categories (p < 0.05). Significant differences in root volume were found in >2.00 mm diameter category between the treatment groups (p < 0.05), and significant differences in root tip number were observed in the (0.00, 0.50] mm diameter category among the treatment groups (p < 0.05).
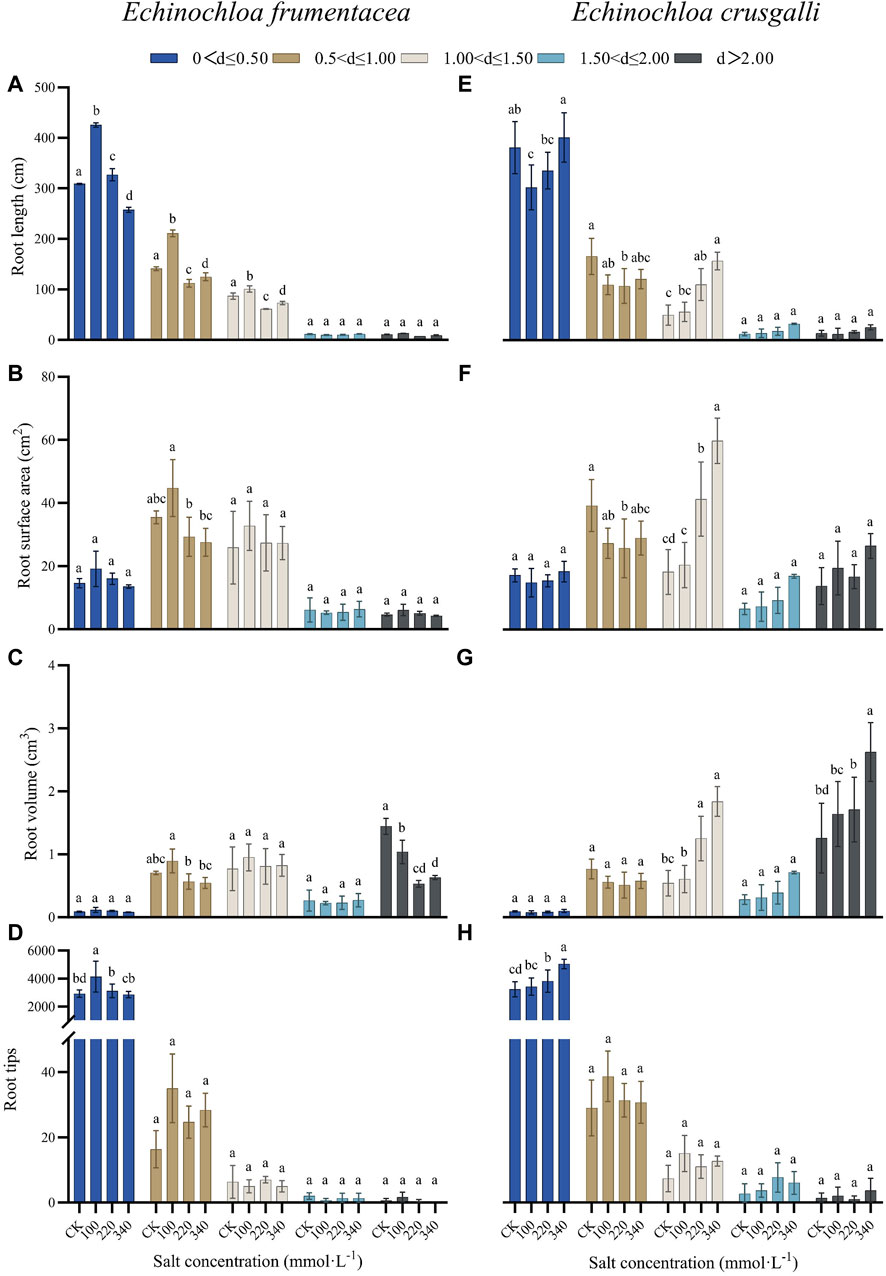
Figure 3. Effects of different concentration of salt treatment on root length, root surface area, root volume and root tips of different diameter roots of E. frumentacea and E. crusgalli. Values are mean ± SD (n = 3). Different letters indicate statistical significance at the p < 0.05 level between each group.
3.4 Correlation between biomass and root morphological variables of E. frumentacea and E. crusgalli under salt stress
A negative correlation existed between aboveground biomass and root biomass, root-to-shoot ratio, root total length, surface area, average diameter, and volume (Table 3). Specifically, a significant negative correlation was observed with the root-to-shoot ratio (p < 0.01). Root biomass showed a significantly positive correlation with root growth variables (root length, surface area, volume, tip number, and fork number) (p < 0.05 or p < 0.01). Variables reflecting root quantity, such as root length, surface area, tip number, fork number, and crossing number, exhibited significantly positive correlations among themselves (p < 0.05). Additionally, the average diameter, reflecting root size, showed a significantly positive correlation with variables reflecting root quantity, including root length, surface area, tip number, and fork number (p < 0.05) (Table 3).
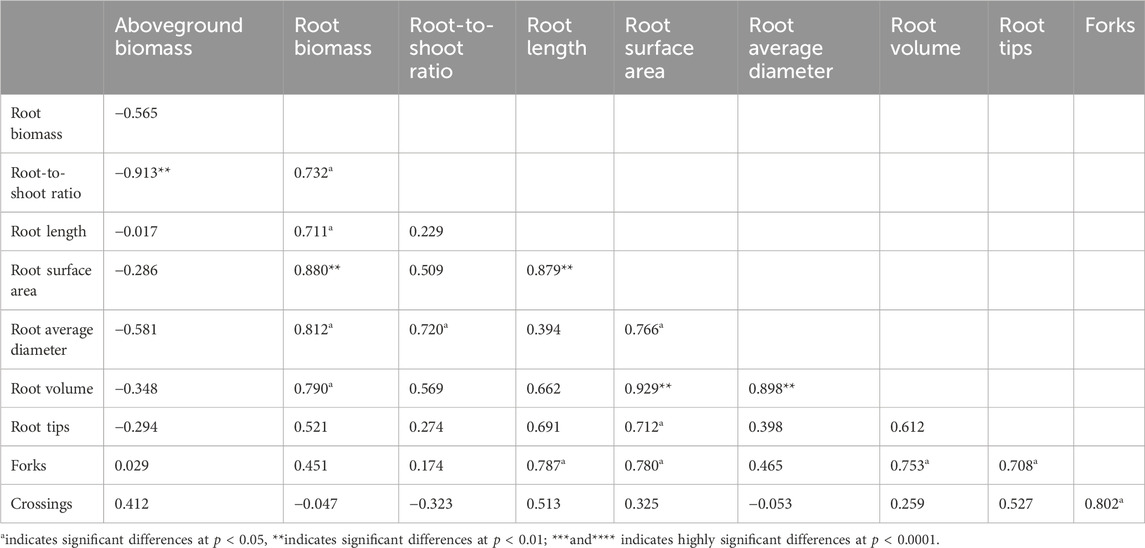
Table 3. Correlation between variables of root biomass and root morphology of E. frumentacea and E. crusgalli.
3.5 Principal Component Analysis of biomass and root morphological variables of E. frumentacea and E. crusgalli
PCA revealed that two principal components explained 80.32% of the variability in the 10 variables, with PC1 (the first principal component) accounting for 49.16% and PC2 (the second principal component) accounting for 31.16% (Table 4). Based on the reported results of the characteristic vectors of the 10 variables (Table 5), the estimates of the first two principal components can be expressed as: PC1 = −0.822 × Aboveground biomass + 0.749 × Root biomass + 0.930 × Root top ratio + 0.444 × Root length + 0.697 × Root surface area + 0.718 × Root average diameter + 0.863 × Root volume + 0.843 × Root tips + 0.362 × Forks - 0.173 × Crossings.

Table 4. Eigenvalues of the correlation matrix of 10 variables of biomass and root morphology in principal component analysis of E. frumentacea and E. crusgalli.
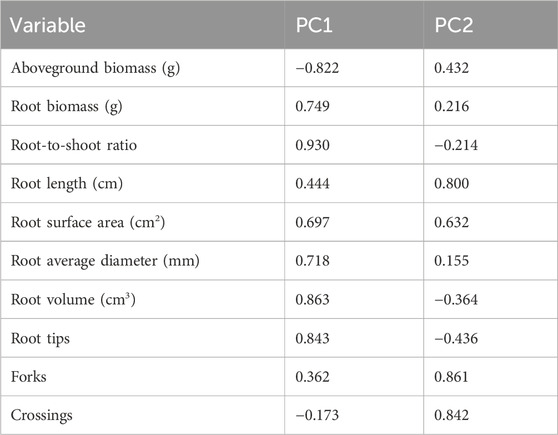
Table 5. Eigenvector estimation results of 10 variables of biomass and root morphology in principal component analysis of E. frumentacea and E. crusgalli.
PC2 = 0.432 × Aboveground biomass + 0.216 × Root biomass - 0.214 × Root top ratio + 0.800 × Root length + 0.632 × Root surface area + 0.155 × Root average diameter - 0.364 × Root volume - 0.436 × Root tips + 0.861 × Forks + 0.842 × Crossings.
PC1 was positively correlated with root biomass and root morphology variables, with factor loadings ranging from 0.697 to 0.930; it had a negative correlation with aboveground biomass, with a factor loading of −0.822, reflecting the distribution of belowground and aboveground biomass. PC2 was positively correlated with root length, number of branches, and number of crossings, with relatively large factor loadings (0.800–0.861), reflecting the complexity of grass root systems. Figure 4 was a scatter plot of the scores of the two principal components for the 10 variables, and ANOVA of the estimated values of PC1 and PC2 for all observed variables were illustrated in Table 6. PC1, reflected significant differences between the two grass species and under salt stress (Table 6, p ≤ 0.0001). E. frumentacea and E. crusgalli were positively correlated with aboveground biomass and root variables under different concentrations of salt treatment (Figure 4), which, combined with the ANOVA results, indicated that 49.16% of the variation in these 10 variables was attributed to differences in grass species and salt treatment concentrations (Table 6). PC2, reflected significant differences in aboveground biomass and root growth among grass species under different concentrations of salt treatment (Table 6).
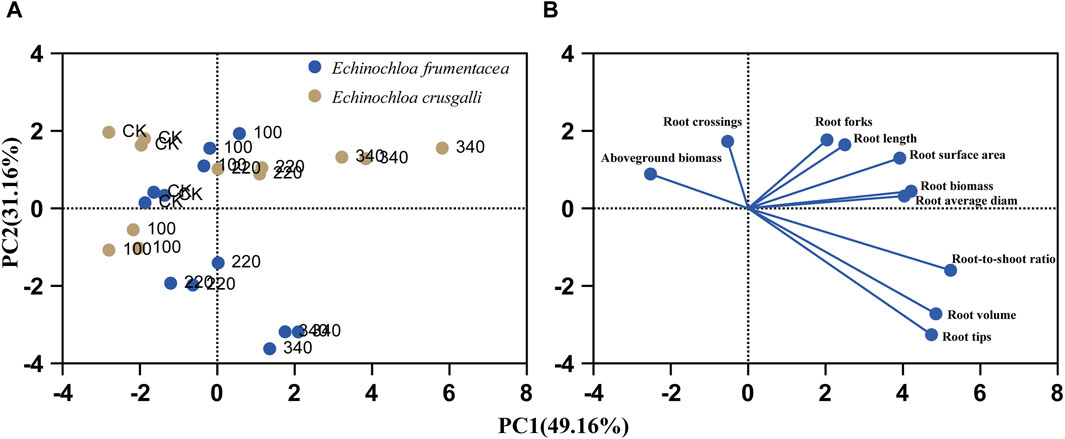
Figure 4. Scatter plots of the first and second principal components of 10 variables of biomass and root morphology E. frumentacea and E. crusgalli. (A) PC scores; (B) Biplot.
4 Discussion
4.1 Adaptive changes of biomass accumulation in response to salt stress
The accumulation and allocation of biomass in plants under different environmental stresses can reflect their survival strategies and adaptive mechanisms. The distribution of biomass between aboveground and root systems also undergoes changes (Singh Grewal, 2010; He et al., 2021). In this study, significant differences were observed in the aboveground biomass accumulation of E. frumentacea and E. crusgalli under different concentrations of salt stress. However, there were no significant differences in the accumulation of root biomass between two species, and the root-to-shoot ratio showed no significant interspecific or intergroup variations. This finding differs from previous research, where foxtail millet (Chenopodium quinoa) seedlings under different mixed salt-alkali conditions increased their root-to-shoot ratio to resist the harm caused by salt-alkali stress (Xu et al., 2023). Salt stress significantly reduced biomass allocation in cotton leaves and stems. Under moderate salt stress, cotton biomass allocation may lead to sustained yield under lower water consumption conditions (Ma et al., 2021). When comparing the salt tolerance of three varieties of proso millet (Blackwell, Cave-in-rock, and Sunburst) with Leymus chinensis, it was found that under low salt (NaCl) conditions (≤2 g kg−1), the biomass of each of the three proso millet varieties was higher than that of Leymus chinensis. However, under higher salt concentrations, their biomass significantly decreased (Zhuo et al., 2015). Zhao et al. (2023) indicated that wheat must coordinate the allocation of biomass between its belowground and aboveground parts, as well as nutrient utilization, to achieve growth compromise under the influence of changes in phosphorus supply and salt levels. Root-to-shoot ratio usually reflects investment differences between aboveground and root biomass caused by biotic or abiotic factors. In line with previous studies, our results showed that the root-to-shoot ratio of two grass species under salt stress exhibited an increasing trend, and the difference was significant under high salt concentration (340 mmol·L−1) treatment. This implies that under salt stress, the two grass species increase the root-to-shoot ratio through biomass allocation to adapt to adversity.
The Optimal Partitioning Theory (OPT) suggests that when a resource becomes limiting, plants prioritize allocating metabolites to organs that can obtain the limiting resource, indicating that they allocate more biomass to the parts obtaining the scarcest resource (Gargaglione et al., 2010; Kobe et al., 2010). If the growth of plants is more strongly influenced by belowground factors (such as soil salinity, moisture, nutrients, physicochemical properties, etc.), plants allocate relatively more biomass to the roots (Poorter et al., 2012). Conversely, when plant growth is more strongly influenced by above-ground factors (temperature, light, humidity, CO2 concentration, etc.), plants allocate relatively more biomass to the aboveground parts (Yang et al., 2018). In this study, 20 individuals of the same grass species grew in limited space and underwent 8 days of salt stress treatment, experiencing strong intraspecific competition. Whether it was the underground roots competing for water and nutrients or the above-ground stems and leaves competing for sunlight, the competition was intense, indicating that under salt stress conditions, both grass species equally and urgently require underground water and aboveground sunlight, thereby affecting biomass accumulation. Results of biomass accumulation in both grass species further demonstrate that plant organs can produce relatively suitable phenotypic potentials under adverse conditions. As the most sensitive organs sensing changes in soil salinity, moisture, and nutrient availability, plant roots exhibit strong phenotypic plasticity in their growth mechanisms (He and Li, 2008; Chen et al., 2016). Therefore, the growth of roots can promptly adapt to changes in soil conditions.
4.2 Changes in the root system morphology of grass under salt stress
Under salt stress, plants can resist the salt environment by altering root system morphology. In this study, under 100 mmol L−1 salt stress, the total length, surface area, tip number, fork number, and crossing number of E. frumentacea roots significantly increased, while under 220 and 340 mmol L−1 salt stress, the root system’s total length, surface area, tip number, fork number, and crossing number significantly decreased. This is consistent with previous studies on the root system of Robinia pseudoacacia seedlings, indicating that the root system adapts to low salt levels but their adaptation to high salt stress is relatively weak. (Mao et al., 2016). Low salt (2 g kg−1) treatment promoted buckwheat root growth, while high salt treatment showed inhibition (Zhang et al., 2023). However, different plants exert different responses to salt stress. Salt-tolerant plants, under high salt conditions, reduce root diameter, increase root length, root surface area in response to salt stress (Wang et al., 2021). This is consistent with our results that the total root length and surface area of E. crusgalli increased under 340 mmol L−1 salt stress. Despite the significant decrease in total root length of E. frumentacea with increasing salt concentration, the effect on root diameter was different. High salt concentration (340 mmol L−1) promoted the thickening of E. crusgalli roots but had no significant effect on the root diameter of E. frumentacea (Table 2). Combining with PCA results, E. crusgalli at 220 and 340 mmol L−1 treatment showed a positive correlation with the average root diameter (Figure 4). Therefore, it is speculated that the lateral growth of E. crusgalli roots is a positive response to adapting to high salt conditions.
The total root length reflects the growth and elongation ability of the root system; the more the number of forks, the wider the distribution range of the root system in the soil; root tips reflect the physiological metabolic ability of the root system; and root surface area is an important indicator of the root system’s absorption of water and nutrients (Tang et al., 2020). In this study, the root scanning showed that under 100 mmol L−1 salt stress, the total length, surface area, volume, tip number, and fork number of E. frumentacea roots were significantly higher than those of E. crusgalli. Therefore, under low salt stress, The higher growth capacity of the root system in E. frumentacea compared to E. crusgalli may be advantageous for E. frumentacea in acquiring water and nutrients. Under 220 and 340 mmol L−1 salt stress, the single plant root length, total root surface area, tip number, fork number, and crossing number of E. crusgalli were significantly higher than those of E. frumentacea (Table 2, p < 0.01). The root system of E. crusgalli is well-developed, exhibiting more complexity than E. frumentacea. The growth range of the root system of E. crusgalli may also be wider than that of E. frumentacea, thus having a higher opportunity to compete for water and nutrients under high salt stress.
Root hairs are tubular cells that extend from the surface of the root to the soil. Root hairs anchor the root system to the growing medium, interact with the soil microbial community, and absorb inorganic nutrients and water from the soil (Dolan, 2017). The root hairs of E. crusgalli are dense and long, with minimal influence from salt, while the root hairs of E. frumentacea are more affected by salt, increasing gradually with the increase in salt concentration (Figure 2). The proliferation of root hairs greatly increases the total surface area of the root system, correspondingly increasing the contact area between the root system and the soil, This is conducive to a better acquisition of water and nutrients under salt stress conditions for the root systems of both grass species under salt stress.
5 Conclusion
The aboveground biomass of E. frumentacea and E. crusgalli decreased significantly (p < 0.05) with increasing salt stress concentrations. The root-to-shoot ratio showed an increasing trend, and significant differences were observed in root growth and morphology (p < 0.05). This suggests that photosynthetic products were preferentially allocated to the roots under salt stress. The total root length, root surface area, root tip number of fine roots as well as the growth of coarse roots (d > 2.00 mm) showed significant differences (p < 0.05) between the two grass species. Different concentrations of salt stress had inconsistent effects on the biomass and radial growth of the roots for the two grass species. E. frumentacea seems to adopt the strategy of gradually increasing its root thickness, root hairs, and root density to adapt to salt stress. Meanwhile, E. crusgalli employed a strategy of increasing its root length, maintaining uniform thickness of root, and developing root hairs.
Data availability statement
The raw data supporting the conclusion of this article will be made available by the authors, without undue reservation.
Author contributions
YZ: Conceptualization, Data curation, Formal Analysis, Funding acquisition, Investigation, Methodology, Project administration, Resources, Software, Supervision, Validation, Visualization, Writing–original draft, Writing–review and editing. YZ: Conceptualization, Data curation, Formal Analysis, Investigation, Methodology, Project administration, Resources, Software, Supervision, Validation, Visualization, Writing–review and editing. RZ: Conceptualization, Data curation, Formal Analysis, Funding acquisition, Investigation, Methodology, Project administration, Software, Supervision, Validation, Writing–review and editing. YS: Conceptualization, Data curation, Formal Analysis, Investigation, Methodology, Software, Writing–review and editing. GL: Conceptualization, Data curation, Investigation, Methodology, Software, Supervision, Writing–review and editing. YS: Formal Analysis, Project administration, Resources, Supervision, Validation, Visualization, Writing–review and editing. GM: Formal Analysis, Methodology, Project administration, Resources, Validation, Visualization, Writing–review and editing. HD: Formal Analysis, Methodology, Project administration, Resources, Software, Validation, Visualization, Writing–review and editing.
Funding
The author(s) declare that financial support was received for the research, authorship, and/or publication of this article. Natural Science Foundation of Gansu Province (grant nos. 21JR7RA760, 22JR5RA796).
Acknowledgments
The authors wish to thank Yunlong Chen for his assistance in the laboratory.
Conflict of interest
The authors declare that the research was conducted in the absence of any commercial or financial relationships that could be construed as a potential conflict of interest.
Publisher’s note
All claims expressed in this article are solely those of the authors and do not necessarily represent those of their affiliated organizations, or those of the publisher, the editors and the reviewers. Any product that may be evaluated in this article, or claim that may be made by its manufacturer, is not guaranteed or endorsed by the publisher.
References
Arif, M. R., Islam, M. T., and Robin, A. H. K. (2019). Salinity stress alters root morphology and root hair traits in Brassica napus. Plants 8, 192. doi:10.3390/plants8070192
Cao, L. W., Lu, R., Fan, J. B., Hu, L. X., and Chen, L. (2022). The fundamental biological problems in the development and utilization of new forage grass. Chin. Bull. Bot. 57 (06), 826–836. doi:10.11983/CBB22159
Chen, G. P., Zhao, W. Z., He, S. X., and Fu, X. (2016). Biomass allocation and allometric relationship in aboveground components of salix psammophila branches. J. Desert Res. 36 (2), 357–363. doi:10.7522/j.issn.1000-694X.2015.00157
Chen, Z., Zhang, J. S., Yan, Q. Q., Yang, P., and Zhang, W. T. (2019). Responses of root morphological parameters to complex saline-alkali stress among Island cotton varieties with different genotypes. Xinjiang Agric. Sci. 56 (11), 1961–1970. doi:10.6048/j.issn.1001-4330.2019.11.001
Dolan, L. (2017). Root hair development in grasses and cereals (Poaceae). Curr. Opin. Genet. Dev. 45, 76–81. doi:10.1016/j.gde.2017.03.009
Farooq, M., Hussain, M., Wakeel, A., and Siddique, K. H. (2015). Salt stress in maize: effects, resistance mechanisms, and management. A review. Agron. Sustain. Dev. 35, 461–481. doi:10.1007/s13593-015-0287-0
Gargaglione, V., Peri, P. L., and Rubio, G. (2010). Allometric relations for biomass partitioning of Nothofagus Antarctica trees of different crown classes over a site quality gradient. For. Ecol. Manag. 259 (6), 1118–1126. doi:10.1016/j.foreco.2009.12.025
Guo, J. C., Wang, M. G., Geng, R., Li, X., Yin, X. H., and Wei, G. N. (2021). Salinity characteristics analysis of saline alkali soil in yinbei irrigation district of Ningxia. Chin. Agric. Sci. Bull. 37 (5), 38–42.
Guo, Q., Han, J., Li, C., Hou, X., Zhao, C., Wang, Q., et al. (2022). Defining key metabolic roles in osmotic adjustment and ROS homeostasis in the recretohalophyte Karelinia caspia under salt stress. Physiol. Plant. 174 (2), e13663. doi:10.1111/ppl.13663
Guo, Q., Meng, L., Han, J., Mao, P., Tian, X., Zheng, M., et al. (2020). SOS1 is a key systemic regulator of salt secretion and K+/Na+ homeostasis in the recretohalophyte Karelinia caspia. Environ. Exp. Bot. 177, 104098. doi:10.1016/j.envexpbot.2020.104098
He, H. B., and Li, Y. (2008). Study on measures of biomass allocation of two desert halophyte species under drought and salt stress: study on measures of biomass allocation of two desert halophyte species under drought and salt stress. Arid Zone Res. 25 (02), 242–247. doi:10.3724/sp.j.1148.2008.00242
He, W. J., Han, G. X., Yan, K., Guan, B., Wang, G. M., Lu, F., et al. (2021). Effects of microtopography on plant biomass and the distribution of both soil water and salinity in coastal saline-alkali land. Chin. J. Ecol. 40 (11), 3585–3597. doi:10.13292/j.1000-4890.202111.002
Jia, X. H., Zhang, F. J., Xie, X. W., Wang, B., Wang, T. F., Ni, W., et al. (2023). Effects of different nitrogen fertilizers on performance and nutrient uptake of Echinochloa frumentacea in saline-alkali land. Acta Agrestia Sin. 31 (1), 272–279. doi:10.13292/j.1000-4890.202111.002
Jia, Y. B., Yang, X. E., Feng, Y., and Jilani, G. (2008). Differential response of root morphology to potassium deficient stress among rice genotypes varying in potassium efficiency. J. Zhejiang Univ. Sci. B 9, 427–434. doi:10.1631/jzus.b0710636
Jing, Y. P., Lin, C. Y., Zhao, P. Y., Li, X. P., Zhao, Q., Liu, Y. J., et al. (2020). Salinized soil improvement by planting 5 plant species in Hetao irrigation area. Soils Crops 9 (2), 114–125. doi:10.11689/j.issn.2095-2961.2020.02.002
Kobe, R. K., Iyer, M., and Walters, M. B. (2010). Optimal partitioning theory revisited: nonstructural carbohydrates dominate root mass responses to nitrogen. Ecology 91 (1), 166–179. doi:10.1890/09-0027.1
Lan, Y. (2022) Physiological responses of three Echinochloa forages to saline-alkaline stress and comprehensive evaluation for their saline-alkaline tolerance. Master of Thesis. Ningxia, China: Ningxia University, 3.
Lan, Y., Zhu, L., Wang, T. T., Jing, Q. F., Zhang, Y., and Zhao, X. L. (2022). Effect of mixed saline-alkail strees on seed germination of three kinds of Echinochloa gass. Seed 41 (03), 37–44. doi:10.16590/j.cnki.1001-4705.2022.03.037
Lu, A. Q., Zhang, F. J., Xu, X., Wang, X. Q., and Yao, S. (2021). Effects of salt stress on growth and physiological characteristics of Echinochloa frumentacea seedlings. Acta Prataculturae Sin. 30 (5), 84–93. doi:10.11686/cyxb20205209
Ma, Y., Wei, Z., Liu, J., Liu, X., and Liu, F. (2021). Growth and physiological responses of cotton plants to salt stress. J. Agron. Crop Sci. 207 (3), 565–576. doi:10.1111/jac.12484
Mao, P. L., Zhang, Y. J., Cao, B. H., Guo, L. M., Shao, H. B., Cao, Z. Y., et al. (2016). Effects of salt stress on eco-physiological characteristics in Robinia pseudoacacia based on salt-soil rhizosphere. Sci. Total Environ. 568, 118–123. doi:10.1016/j.scitotenv.2016.06.012
Mukhopadhyay, R., Sarkar, B., Jat, H. S., Sharma, P. C., and Bolan, N. S. (2021). Soil salinity under climate change: challenges for sustainable agriculture and food security. J. Environ. Manag. 280, 111736. doi:10.1016/j.jenvman.2020.111736
Poorter, H., Niklas, K. J., Reich, P. B., Oleksyn, J., Poot, P., and Mommer, L. (2012). Biomass allocation to leaves, stems and roots: meta-analyses of interspecific variation and environmental control. New Phytol. 193 (1), 30–50. doi:10.1111/j.1469-8137.2011.03952.x
Qiu, S. F., Yang, L. Q., Zhao, H., Huang, D. F., and Tang, D. Q. (2016). Effects of water holding capacity in substrate on growth characters and comprehensive quality of Brassica campestris L. ssp. Chinensis cv. Ziyoucai. Acta Agric. Zhejiangensis 28 (2), 195–199. doi:10.3969/j.issn.1004-1524.2016.02.03
Singh Grewal, H. (2010). Response of wheat to subsoil salinity and temporary water stress at different stages of the reproductive phase. Plant soil 330, 103–113. doi:10.1007/s11104-009-0179-7
Tang, Z. Q., Chen, Y. J., Hu, J., Wang, H., Lei, Y. X., Shuai, L. L., et al. (2020). Analysis of root architecture and rhizosheath characteristics of seven forage species in desertified grassland of northwest sichuan. Chin. J. Grassl. 42 (2), 22–31. doi:10.16742/j.zgcdxb.20190315
Tong, H., Sun, J., Guo, S. R., and Zhang, Z. X. (2012). Effects of iso-osmotic Ca(NO3)2 and NaCl stress on root morphology and activity of cucumber seedlings. J. Nanjing Agric. Univ. 35 (3), 37–41.
Wang, S., Zhao, Z., Ge, S., Peng, B., Zhang, K., Hu, M., et al. (2021). Root morphology and rhizosphere characteristics are related to salt tolerance of Suaeda salsa and Beta vulgaris L. Front. Plant Sci. 12, 677767. doi:10.3389/fpls.2021.677767
Wang, S. J., Chen, Q., Li, Y., Zhuo, Y. Q., and Xu, L. Z. (2017). Research on saline-alkali soil amelioration with FGD gypsum. Resour. Conservation Recycl. 121, 82–92. doi:10.1016/j.resconrec.2016.04.005
Wu, S. Q., Yang, P., Fan, Z. J., Ma, G. S., Chen, L. X., and Gen, B. R. (2002). Study on Echinochloa crusgalli (L.) Beauv. Var. mitis (pursh) peter. Pratacultural Sci. 19 (2), 33–36.
Xu, H. Y., Zhao, Y., Ruan, Q., Zhu, X. L., Wang, B. Q., and Wei, X. H. (2023). Resistance of quinoa seedlings under different salt-alkali stress levels. Acta Prataculturae Sin. 32 (1), 122–130. doi:10.11686/cyxb2021500
Yang, J. S., Yao, R. J., Wang, X. P., Xie, W. P., Zhang, X., Zhu, W., et al. (2022a). Research on salt-affected soils in China: history, status quo and prospect. Acta Pedol. Sin. 59 (1), 10–27. doi:10.11766/trxb202110270578
Yang, Y., Dou, Y., An, S., and Zhu, Z. (2018). Abiotic and biotic factors modulate plant biomass and root/shoot (R/S) ratios in grassland on the Loess Plateau, China. Sci. Total Environ. 636, 621–631. doi:10.1016/j.scitotenv.2018.04.260
Yang, Z. X., Zhen, X., Chen, L. B., Yu, Y. X., Zhang, F. H., Li, L. H., et al. (2022b). Morphological adaptation strategies of Rumex hanus planted in saline-alkali land of arid areas. Acta Prataculturae Sin. 31 (7), 15–27. doi:10.11686/cyxb2021231
Zhang, J. P., Gao, Y., Dang, X. H., Zhang, G., and Wang, T. (2020). Responses of root morphology of Amorpha fruticosa NaCl saline stress. Bull. Soil Water Conservation 40 (2), 54–58. doi:10.13961/j.cnki.stbctb.2020.02.008
Zhang, L., Ma, H., Chen, T., Pen, J., Yu, S., and Zhao, X. (2014). Morphological and physiological responses of cotton (Gossypium hirsutum L.) plants to salinity. PLoS One 9 (11), e112807. doi:10.1371/journal.pone.0112807
Zhang, W. R., Wu, S. J., and Wang, X. P. (2018). Research advances in germplasm resources and applications of Echinochloa. Pratacultural Sci. 35 (1), 165–175. doi:10.11829/j.issn.1001-0629.2017-0277
Zhang, X., He, P., Guo, R., Huang, K., and Huang, X. (2023). Effects of salt stress on root morphology, carbon and nitrogen metabolism, and yield of Tartary buckwheat. Sci. Rep. 13 (1), 12483. doi:10.1038/s41598-023-39634-0
Zhao, D. Y., Zhang, X. L., Zhao, S. P., Liu, G. L., Zhang, Z. W., Zhao, W. F., et al. (2023). Biomass allocation and nutrients utilization in wheat as affected by phosphorus placement and salt stress. Agronomy 13 (6), 1570. doi:10.3390/agronomy13061570
Keywords: Echinochloa frumentacea, Echinochloa crusgalli, salt stress, biomass, root morphology
Citation: Zhang Y, Zhang Y, Zhang R, Song Y, Li G, Song Y, Ma G and Guo H (2024) Adaptability of root morphology and growth of two forage grass species in response to salt stress. Front. Environ. Sci. 12:1406778. doi: 10.3389/fenvs.2024.1406778
Received: 25 March 2024; Accepted: 18 April 2024;
Published: 06 May 2024.
Edited by:
Ning Chen, Lanzhou University, ChinaReviewed by:
Ke Zhang, Zhengzhou University of Light Industry, ChinaHu Rui, Chinese Academy of Sciences (CAS), China
Copyright © 2024 Zhang, Zhang, Zhang, Song, Li, Song, Ma and Guo. This is an open-access article distributed under the terms of the Creative Commons Attribution License (CC BY). The use, distribution or reproduction in other forums is permitted, provided the original author(s) and the copyright owner(s) are credited and that the original publication in this journal is cited, in accordance with accepted academic practice. No use, distribution or reproduction is permitted which does not comply with these terms.
*Correspondence: Yang Zhang, zysdy1316@foxmail.com
†These authors have contributed equally to this work