- Section for Aquatic Biology and Toxicology, Department of Biosciences, University of Oslo, Oslo, Norway
In the last decades, limnic water bodies in the Northern hemisphere have experienced a noticeable browning, i.e., increasing levels of dissolved organic matter (DOM). While the effects on primary producers is usually considered negative (light attenuation), zooplankton is thought to benefit from increased DOM, which absorbs harmful ultraviolet radiation (UVR). However, behavioral alterations due to browning in zooplankton have not yet been studied. We investigated the effects of a DOM gradient, alone and in combination with UVR, on the swimming behavior of Daphnia magna. Making use of a computer-controlled imaging system, we repeatedly filmed individuals over 6 h and analyzed the video material to unravel effects on exploration behavior and other motility patterns. The results show that increasing DOM buffers the detrimental effects of UVR on swimming behavior. This is likely due to attenuation of UVR by DOM. Interestingly, DOM also raised the overall swimming activity independent of UVR exposure. Our findings highlight the importance of DOM in freshwater systems, not only because of its physico-chemical properties, but also due to its higher-level effects on zooplankton communities.
Introduction
In the Anthropocene's world of a changing climate (IPCC, 2014), wildlife throughout ecosystems has to cope with an increasing dissonance of altered bio-, geo-, and photo-chemical realities. In limnic systems of the Northern hemisphere, climate change manifestations are manifold (Adrian et al., 2009; Williamson et al., 2009). Amongst other observable changes, increases of dissolved organic matter (DOM) have led to an increased “browning” of waters in the recent past (Erlandsson et al., 2008; Solomon et al., 2015; Rantala et al., 2016). Reasons for this include climate change (Larsen et al., 2011; de Wit et al., 2016), increased land use (Evans et al., 2012; Arvola et al., 2017), and decreased sulfur deposition (Finstad et al., 2016; Monteith et al., 2017; Strock et al., 2017).
One of the main consequences of rising DOM is the increased attenuation of light. This can have direct negative results for primary producers, which rely on photosynthetically active radiation (Thrane et al., 2014). However, DOM also absorbs harmful ultraviolet radiation (UVR; Williamson et al., 2015). Especially for zooplankton residing in browning waters, the net result of increased DOM levels is thus seen as primarily positive. This is largely due to photoprotection from UVR, which is known to be harmful via a range of mechanisms, including direct DNA damage, protein denaturation and lipid peroxidation (Pellegri et al., 2014; Tartarotti et al., 2014). Interactions between DOM and UVR have been shown to affect growth and survival of zooplankton, as well as inducing DNA damages (Rautio and Korhola, 2002; Geddes, 2009; Wolf et al., 2017).
Zooplankton species are known to rapidly adapt their behavior to changes in the environment. Behavior is one of the first reactions to changes in the environment, having both effects on species interactions as well as individual fitness, and hence influencing the entire ecosystem's ecology (Sih et al., 2010). The behavioral responses of zooplankton to UVR are diverse and have been studied extensively (Rose et al., 2012; Hylander and Hansson, 2013). Exemplary adaptations in zooplankton behavior include predator avoidance (Lampert, 1989), grazing migration (Lampert and Taylor, 1985; Reichwaldt et al., 2004), and flight from UVR (Rhode et al., 2001; Williamson et al., 2001; Hylander and Hansson, 2010). However, behavioral effects of DOM—alone and in combination with UVR—have not been subject to investigation.
In this study, we seek to unravel the behavioral effects of DOM and its interaction with UVR on zooplankton. Daphnia species have a central role in the limnic food web, linking primary production with higher trophic levels, and can be found across all climates ranges. They are directly affected by changes in their environment, e.g., increased DOM and UVR. In natural systems, changes in swimming behavior affect several fitness-related traits, e.g., filter feeding, energy demand, and predator avoidance. We used a battery of endpoints as proxies for swimming activity. In particular, we measured the explored area, average swimming speed, median swimming depth, gross traveled distance, and swimming time. We used a customizable automated imaging solution to record and analyse movies, in order to dissect the effects of DOM, and its interaction with UVR, on zooplankton behavior.
Materials and Methods
Animal Husbandry
For husbandry, Daphnia magna were reared in aerated full silicate glass aquaria with densities not exceeding 20 animals per liter. They were kept in filtered tap water (0.22 μm polyether sulfone filter; Corning, Corning, NY, USA), enriched to 4 mM CaCl2, 4 mM NaHCO3, 12 nM H2SeO3, and supplemented with vitamins B12, d-biotin, and thiamine hydrochloride (4, 2, and 300 nM, respectively). Animals were cultured in a 16:8 h light:dark cycle at a light intensity of 1,500 lux (L 18 W/950 fluorescent lamps; OSRAM, Munich, Germany) and fed Chlamydomonas reinhardtii at an ad libitum diet.
The D. magna clone (DHI strain) of this study was originally obtained from the Norwegian Institute for Water Research (NIVA; Oslo, Norway) and has been cultured in our facilities without observable signs of stress over the last 4 years.
Experimental Setup
Gradients of DOM (2, 2.5, 5, 10, and 20 mg C L−1) were prepared by dissolving freeze-dried Nordic Aquatic Humic Acid Reference (isolated from lake water using reverse osmosis; Gjessing et al., 1999) in D. magna culture medium; 2 mg C L−1 were the background concentration the D. magna culture medium. The concentrations were confirmed using a TOC-VCPH Total Organic Carbon Analyzer (Shimadzu, Kyoto, Japan).
The day before the experiment, adult daphnids carrying their third brood were isolated and left spawning overnight. Shortly before the start of the experiment the next day, individual neonates (not older than 24 h) were placed in 40 ml of the respective DOM-enriched D. magna culture medium in non-coated cell culture flasks (TC Flask T25, Suspension; Sarstedt, Nümbrecht, Germany). The water depth in these flasks was 4 cm. Duplicates of DOM treatments (ten flasks in total) were randomly placed in a row into the filming setup.
Flasks were illuminated from above either using the same D. magna light source as used for husbandry, or in combination with ultraviolet radiation (UVA-340 Lamp; Q-Lab, Westlake, OH, USA). The light source was chosen because it allowed easy manipulation of the photon flux spectrum, and because its waveband peak at 340 nm is sufficiently close to the environmentally relevant UVB waveband (Häder et al., 2011). During the non-UV treatments (“–UV”), daphnids received an intensity of 1,500 lux of regular light (OECD, 2012). The UV treatment (“+UV”) was provided by substituting 60 μmol m−2 s−1 of the total light intensity of 1,500 lux with UVA340 radiation (λ = 340 nm). Light intensities were calibrated with a SpectraPen LM 500-UVIS spectroradiometer (Photon Systems Instruments, Drásov, Czech Republic). The photon flux profiles are given in Supplementary Figure 1.
Each of the 10 bottles was filmed one at a time, using a moveable camera (Raspberry Pi NoIR Camera; Raspberry Pi Foundation, Cambridge, United Kingdom) mounted on a computer-controlled platform (Raspberry Pi; Raspberry Pi Foundation). Each experiment lasted 6 h, with each flask being filmed for 1 min at 26 frames per second every 19 min, resulting in 19 videos per flask. Each experiment was replicated three times with and without UV, resulting in six replicates per treatment combination. A custom Python script (version 2.7.13; available online at: https://www.python.org/; Supplementary Data 1) controlled the connected stepper motor (PhidgetStepper Bipolar HC; Phidgets, Calgary, Canada) and camera. A schematic overview of the experimental design is given in Figure 1.
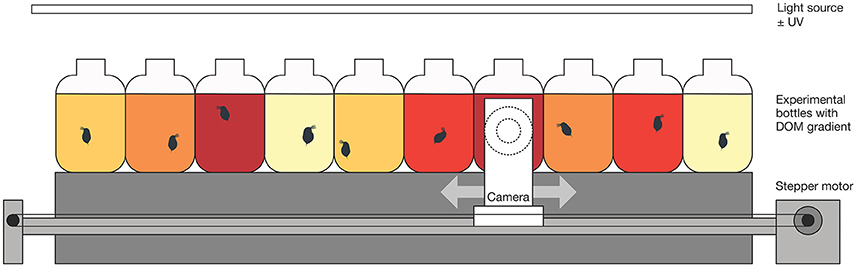
Figure 1. Schematic overview of the experimental design. For each experimental run, every DOM concentration (color gradient) was present in duplicates and randomly placed in front of the camera axis. Each flask contained a single Daphnia magna neonate. For details on the experimental procedure and filming regime, see the Materials and Methods section.
Immediately following the experiments, all animals were post-fixed in 1% (v/v) Lugol's iodine and their size measured as core body length (Ranta et al., 1993) using digital image analysis tool Fiji (version 2.0.0-rc-43/1.51p; Schindelin et al., 2012).
Movie and Tracking Evaluation
The recorded video sequences were analyzed for movement behavior using a custom python script using the Open Source Computer Vision Library (OpenCV; version 3.3.0; available online at: http://opencv.org) to track individual animals. The script identifies the position of the individual Daphnia in the bottle and extracts its position (XY coordinates) for each frame. The annotated script is available in the online version of the article (Supplementary Data 2). The field of view of the camera included the whole waterbody. Due to the use of a rolling average for background subtraction during the feature extraction, individuals were not traced while they remained stationary for a prolonged time (>10 s). Missing positions were thus classified as resting. Position data were then imported into open-source statistical software R (version 3.4.1; R Core Team, 2017) for further analysis (Supplementary Data 3). Tracks shorter than 1 s, and shorter tracks occurring simultaneously to longer tracks were excluded to prevent false detections (e.g., mirror images of daphnids in the bottle walls or false traces) from the data.
From the position data, five behavioral traits were computed to analyse the individuals' behavior. The explored area (mm2) is the area enclosed by the convex envelope of the coordinates and represents a measure of explorative behavior. The swimming speed (mm s−1) is the average of the frame to frame distances over time. The traveled distance (mm) is the gross sum of all traveled distances and was used as a measure of general activity; as was the swimming time (%). The median of the swimming depth (mm) was taken as a measure of UV stress response (Hylander et al., 2014). While these traits can be correlated, they capture different characteristics of an individual's movement (Chapman et al., 2011; Heuschele et al., 2017).
Statistical Analyses
All statistical analyses were carried out using open-source statistical software R (version 3.4.1; R Core Team, 2017) and its add-on packages MASS (version 7.3-47; Venables and Ripley, 2002), MuMIn (version 1.15.6; Barton, 2016), and nlme (version 3.1-131; Pinheiro et al., 2016).
Each of the five behavioral traits were analyzed as independent responses in linear mixed-effects (LME) models. DOM (mg C L−1), UVR (μmol UVA340 m−2 s−1), time (h), and size of the Daphnia (mm) served as fixed-effect parameters, and the initial models allowed for all interactions. Each run (i.e., each round of filming) was treated as random slope, and individuals nested inside the experimental day were the grouping structure. Before analyses, the respective response variables were power-transformed to maximize log-likelihood (Box and Cox, 1964).
Parameter selection for the final model was based on the Akaike's corrected information criterion (AICc; Akaike, 1974; Burnham and Anderson, 2004). For this, the AICc of models of all possible parameter combinations were determined using maximum likelihood. All models within a Δ of 2 of the lowest AICc were updated using restricted maximum likelihood. The number of models fulfilling this criterion varied between behavioral traits, as the treatments did not influence all aspects of swimming behavior equally strong. Model averaging of the full model was then used to summarize the information contained in the competing “best” models and to determine the final set of parameters (Burnham and Anderson, 2002).
After parameter selection and model averaging based on information criteria, the fixed-effect parameters of the final full models were analyzed using Wald's F-tests with marginal (type III) sum-of-squares (Pinheiro and Bates, 2000; Li and Redden, 2015), and were considered significant for P < 0.05. This served as proxy for the effect strength of the retained fixed-effect parameters on the swimming behavior of D. magna. All results are given as mean ± standard deviation. The annotated script for statistical analyses can be found in the online version of this article (Supplementary Data 4).
Results
The explored area was most prominently influenced by the presence of UVR, which had an overall negative effect (Figure 2A). Without UVR, the explored area fluctuated between 653.7 ± 351.3 and 713.5 ± 351.5 mm2, without a clear DOM effect. In the presence of UVR, the explored area increased along the DOM gradient, from 64.7 ± 65.8 to 190.3 ± 156.6 mm2. The final LME model was averaged from two models and contained three variables with two interactions. UVR had a negative effect, and time as well as the DOM × UVR interaction had a positive effect.
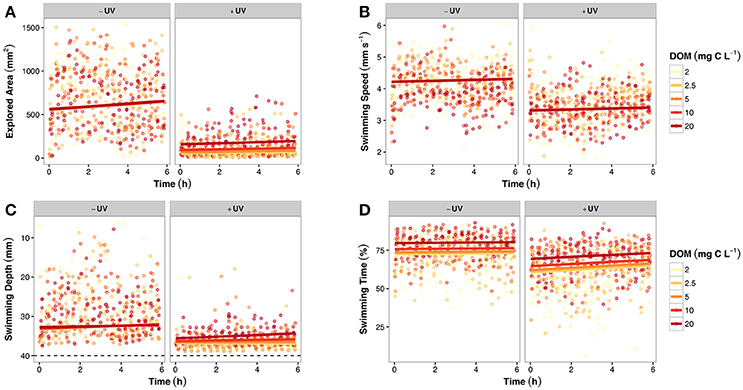
Figure 2. Overview of four different swimming behavior proxies over time, separated by UVR presence and differentiated by DOM concentrations (color gradient). Points are raw data and solid lines are the predictions of the best-fit LME models (see the Materials and Methods section for details). (A) Development of explored area (mm2). Note the strong inhibiting effect of UVR, as well as the monotonous increase along the DOM gradient. (B) Overview of the swimming speed (mm s−1) of daphnids. Note the overall negative influence of UVR and the lack of any DOM-related effects. (C) Overview of the swimming depth (mm). Note the severe flight behavior under UVR treatment, as well as the concentration-dependent DOM effect. The dashed black line indicates the bottom of the flask, i.e., the physical limitation to the swimming depth. (D) Overview of the development of the swimming time (%). Compared to the other swimming behavior proxies, the effect of UVR seems to be moderate, while there is a strong influence of DOM.
The swimming speed of daphnids was mostly influenced by the presence of UVR, but apparently not by DOM (Figure 2B). Without UVR, the swimming speed ranged from 4.0 ± 0.6 to 4.3 ± 0.5 mm s−1; under UVR exposure, it ranged from 3.3 ± 0.4 to 3.6 ± 0.6 mm s−1. In both cases, DOM did not seem to exert an effect. The final LME model was averaged from five models and contained four parameters with three interactions. UVR had a negative effect and time a positive influence on the swimming speed.
The swimming depth, again, was mostly influenced by the presence of UVR; however, a DOM-influence was visible under the UVR treatment (Figure 2C). Without UVR, the swimming depth ranged from 28.2 ± 7.0 to 29.9 ± 6.1 mm, while it increased along the DOM gradient from 37.4 ± 1.8 to 34.9 ± 2.1 mm under UVR exposure. The final LME model was averaged from 16 models and contained four parameters with six interactions. UVR and the UVR × time interaction increased the swimming depth, while the DOM × UVR × time triple interaction had a decreasing effect.
The traveled distance during the experiment, again, was mostly influenced by the presence of UVR, but not visibly by DOM; however, a temporal trend was observable (Supplementary Figure 2). Without UVR, the traveled distance ranged from 176.0 ± 33.9 to 195.3 ± 29.9 mm, without a clear DOM effect. Under UVR treatment, it increased along the DOM gradient from 118.3 ± 42.9 to 141.2 ± 31.1 mm. The final LME model was averaged from 11 models and had four parameters with four interactions. UVR had a negative effect, and the DOM × UVR interaction a positive effect. DOM had a positive effect and the DOM × size interaction had a negative influence.
In contrast to the previous parameters, the swimming time appeared to be mostly influenced by DOM, but also contrastingly by UVR (Figure 2D). Without UVR, the swimming time increased along the DOM gradient from 68.6 ± 10.9 to 80.4 ± 6.4%. The same pattern could be observed under UVR exposure, as swimming time increased from 55.0 ± 14.8 to 68.6 ± 10.0% along the DOM gradient. The final LME model was averaged from four models and contained four parameters with two interactions. DOM had a positive effect, whereas UVR had a negative influence on the swimming time. The interaction between time and UVR had a positive effect.
Details on the respective LME model parameters and parameter significances can be found in Table 1, and summary statistics for all endpoints and treatments are given in Supplementary Table 1.
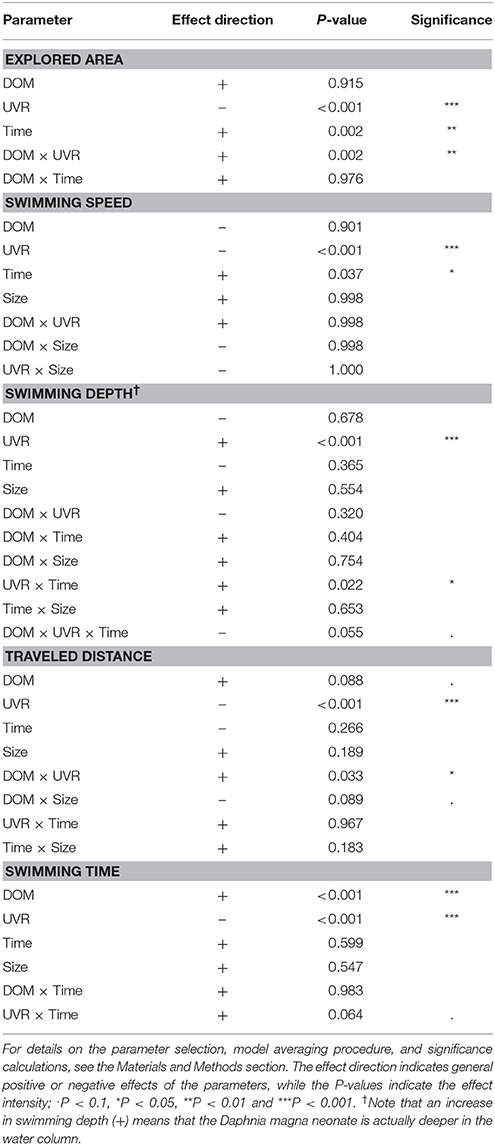
Table 1. Overview of the results from model averaging for the five different behavioral parameters: explored area, swimming speed, swimming depth, traveled distance, and swimming time.
Discussion
In the present study, we demonstrate the interactive effects of DOM and UVR on zooplankton swimming behavior. For our model organism D. magna, DOM mostly altered swimming behavior under UVR exposure, seemingly relieving the detrimental UVR effects. Individuals explored a larger area and traveled more when “protected” from UVR. This can be interpreted as a direct effect of UVR-absorption by DOM molecules, i.e., shading.
Without UVR exposure, no differences in motility were measurable along the DOM gradient, with the exception of the swimming time (Figure 2D), which was positively correlated to DOM independent of UVR exposure. This indicates that DOM itself has the potential to alter zooplankton behavior. A possible explanation could be a change in water-borne oxygen concentration due to chemical consumption in oxidation processes (Asakura et al., 2010; Ylla et al., 2012).
Increased swimming activity under higher DOM levels would allow individuals to increase filter feeding. Likely, the increase in DOM-associated free nutrients (e.g., carbon, nitrogen, and phosphorus; Qualls et al., 1991; Williams and Edwards, 1993) would also benefit bacterial growth (Anesio et al., 2005; Buchan et al., 2014), which could increase food source availability for daphnids.
The singular effects of UVR on the swimming behavior of Daphnia species have repeatedly been studied elsewhere and our findings corroborate these studies. As daphnids actively recognize the presence of harmful UVR through their compound eyes (Smith and Macagno, 1990), their first initial behavioral response is the flight into deeper waters (Figure 2C; Leech and Williamson, 2001; Rhode et al., 2001; Fischer et al., 2006; Williamson et al., 2011). By doing so, they effectively eliminate potential metabolic harm from UVR (Häder and Sinha, 2005). If not constraint by the size of the culture flasks, the daphnids in our experiments likely would have swum several decimeters deeper (Hylander et al., 2014), until the UVR intensity was below their perception threshold. The intensity is modulated by the strong light attenuation of DOM in the UVR waveband (Thrane et al., 2014; Wolf et al., 2018), so daphnids in browner water would reside higher up in the water column in the presence of UVR. The influence of UVR on the explored area in our experiment is a direct result of this “flight” behavior, as daphnids were mostly residing in the bottom of the flask and could not swim deeper.
The decrease in swimming speed due to UVR could point toward a shift in energy allocation, from motility to oxidative stress defense and repair mechanisms. The negative UVR impact on traveled distance can be explained either as an artifact of the flask depth, or as a result of activated defense and repair mechanisms, which seemingly calm down daphnids (Hylander et al., 2014). Over time, swimming behavior generally increased, which in this context could indicate successful defense mechanisms (Rautio and Tartarotti, 2010; Lushchak, 2011).
While DOM and UVR were the dominant factors affecting swimming behavior (Figure 2, Table 1), both the duration of the experiments and size of the daphnids had some influence as well. Although the size of daphnids did not have strong effects on any observed endpoint, larger specimen seemingly covered more distance than their smaller peers. The experimental duration altered both the explored area and the swimming speed. This indicates acclimation processes of daphnids to the experimental conditions and makes a strong case for our approach of repeated measurements of activity patterns over a reasonable time scale (Hansson et al., 2016). Our approach is especially well-suited to detect sublethal effects of environmental changes on behavior, well-before detrimental physiological or genetic damages are detectable (Lima, 1998; Pan et al., 2017).
In our study, we focused on the interaction between DOM and UVR on swimming behavior. However, both factors alter several other environmental factors relevant for zooplankton fitness. In nature, most of the UVR photons will be absorbed within the first meter of the water column (Thrane et al., 2014). However, daphnids often inhabit shallow ponds, with—in extreme cases—only a few centimeters of water depth. Under these conditions, behavioral reactions alone are insufficient and additional physiological protection is needed, which comes at energetic costs (Leech et al., 2005; Wolf et al., 2017). Because of the immensely negative effects of UVR, the filtration efficiency of UVR-exposed Daphnia is reduced (Fernández and Rejas, 2017), which can further deplete energy reserves.
On an ecosystem level, the effects of water browning on primary production point toward a negative impact due to increased light attenuation (Buchan et al., 2014; Kissman et al., 2017). Besides affecting physiological processes of organisms, interactions between DOM and UVR also affects species interactions in ecosystems, e.g., UVR photochemically degrades kairomones, rendering them ineffective (Sterr and Sommaruga, 2008). Water browning could thus increase the effect duration of kairomones in water. This could be beneficial for zooplankton, by prolonging alertness levels to predator presence (Hylander et al., 2009). The light regime also influences predator escape efficiency, mostly through visual predator avoidance (Brewer et al., 1999). In this case, water browning should decrease the chances of spotting a predator—or, vice versa, prey (Ranåker et al., 2012; Lindholm et al., 2016). In our experiments, D. magna individuals generally increased exploration activity with increasing DOM levels, suggesting that encounter rates with predators could increase. However, whether predator or prey will benefit from water browning will also depend on the specific combination of the visual sensitivities and reaction distances in both organisms (Utne-Palm, 2010).
In summary, we demonstrate that increased DOM can alleviate the negative effects of UVR on zooplankton swimming behavior. The multitude and complexity of pathways and interactions between DOM, UVR, and other factors makes it difficult to predict ecosystem responses. It is clear that the continued trend of water browning in freshwater systems of the Northern hemisphere will challenge biota throughout ecosystems, providing both beneficial, but also detrimental new circumstances to cope with. Besides freshwater systems, also coastal ecosystems are affected by increased water browning (Aksnes et al., 2009). Hence, our findings are likely also relevant for marine zooplankton. Given that organisms first react to changing environmental conditions by altering their behavior, our experimental approach can be used to investigate short-term effects of multiple stressors in small organisms, not only in the context of water browning, but also other environmental challenges.
Author Contributions
RW conceived the study and both authors designed the experiment. JH was responsible for the technical setup and RW carried out the experiment. JH developed the movie and tracking evaluation, and both authors worked on the statistical analysis, figures, and tables. RW wrote the manuscript with input from JH.
Conflict of Interest Statement
The authors declare that the research was conducted in the absence of any commercial or financial relationships that could be construed as a potential conflict of interest.
Acknowledgments
We thank Tom Andersen for his valuable input regarding the statistical analyses. JH was financed by the prioritized research group LUMS at the Department of Biosciences, Faculty of Mathematics and Natural Sciences, University of Oslo, Norway. The publication cost was covered by the Open Access Publishing Fund of the University of Oslo Library.
Supplementary Material
The Supplementary Material for this article can be found online at: https://www.frontiersin.org/articles/10.3389/fevo.2018.00026/full#supplementary-material
References
Adrian, R., O'Reilly, C. M., Zagarese, H., Baines, S. B., Hessen, D. O., Keller, W., et al. (2009). Lakes as sentinels of climate change. Limnol. Oceanogr. 56, 2283–2297. doi: 10.4319/lo.2009.54.6_part_2.2283
Akaike, H. (1974). A new look at the statistical model identification. IEEE Trans. Autom. Contr. 19, 716–723. doi: 10.1109/TAC.1974.1100705
Aksnes, D. L., Dupont, N., Staby, A., Fiksen, Ø., Kaartvedt, S., and Aure, J. (2009). Coastal water darkening and implications for mesopelagic regime shifts in Norwegian fjords. Mar. Ecol. Prog. Ser. 387, 39–49. doi: 10.3354/meps08120
Anesio, A. M., Granéli, W., Aiken, G. R., Kieber, D. J., and Mopper, K. (2005). Effect of humic substance photodegradation on bacterial growth and respiration in lake water. Appl. Environ. Microbiol. 71, 6267–6275. doi: 10.1128/AEM.71.10.6267-6275.2005
Arvola, L., Leppäranta, M., and Äijälä, C. (2017). CDOM variations in Finnish lakes and rivers between 1913 and 2014. Sci. Tot. Environ. 601–602, 1638–1648. doi: 10.1016/j.scitotenv.2017.06.034
Asakura, H., Endo, K., Yamada, M., Inoue, Y., Ono, Y., and Ono, Y. (2010). Influence of oxygen flow rate on reaction rate of organic matter in leachate from aerated waste layer containing mainly incineration ash. Waste Manage. 30, 2185–2193. doi: 10.1016/j.wasman.2010.06.001
Barton, K. (2016). MuMIn: Multi-Model Interference. R Package Version 1.15.6. Available online at: https://CRAN.R-project.org/package=MuMIn
Box, G. E. P., and Cox, D. R. (1964). An analysis of transformations. J. R. Stat. Soc. Ser. B Stat. Methodol. 26, 211–252.
Brewer, M. C., Dawidowicz, P., and Dodson, S. I. (1999). Interactive effects of fish kairomone and light on Daphnia escape behavior. J. Plankton Res. 21, 1317–1335. doi: 10.1093/plankt/21.7.1317
Buchan, A., LeCleir, G. R., Gulvik, C. A., and González, J. M. (2014). Master recyclers: features and functions of bacteria associated with phytoplankton blooms. Nat. Rev. Microbiol. 12, 686–698. doi: 10.1038/nrmicro3326
Burnham, K. P., and Anderson, D. R. (2002). Model Selection and Multimodel Inference: A Practical Information-Theoretic Approach. New York, NY: Springer.
Burnham, K. P., and Anderson, D. R. (2004). Multimodel interference: understanding AIC and BIC in model selection. Sociol. Method. Res. 33, 261–304. doi: 10.1177/0049124104268644
Chapman, B. B., Hulthén, K., Blomqvist, D. R., Hansson, L.-A., Nilsson, J.-Å., Brodersen, J., et al. (2011). To boldly go: individual differences in boldness influence migratory tendency. Ecol. Lett. 14, 871–876. doi: 10.1111/j.1461-0248.2011.01648.x
de Wit, H. A., Valinia, S., Weyhenmeyer, G. A., Futter, M. N., Kortelainen, P., Austnes, K., et al. (2016). Current browning of surface waters will be further promoted by wetter climate. Environ. Sci. Technol. Lett. 3, 430–435. doi: 10.1021/acs.estlett.6b00396
Erlandsson, M., Buffam, I., Fölster, J., Hjalmar, L., Temnerud, J., Weyhenmeyer, G. A., et al. (2008). Thirty-five years of synchrony in the organic matter concentrations of Swedish rivers explained by variation in flow and sulphate. Glob. Chang. Biol. 14, 1191–1198. doi: 10.1111/j.1365-2486.2008.01551.x
Evans, C. D., Jones, T. G., Burden, A., Ostle, N., Zielinski, P., Cooper, M. D. A., et al. (2012). Acidity controls on dissolved organic carbon mobility in organic soils. Glob. Chang. Biol. 18, 3317–3331. doi: 10.1111/j.1365-2486.2012.02794.x
Fernández, C. E., and Rejas, D. (2017). Effects of UVB radiation on grazing of two cladocerans from high-altitude Andean lakes. PLoS ONE 12:e0174334. doi: 10.1371/journal.pone.0174334
Finstad, A. G., Andersen, T., Larsen, S., Tominaga, K., Blumentrath, S., de Wit, H. A., et al. (2016). From greening to browning: catchment vegetation development and reduced S-deposition promote organic carbon load on decadal time scales in Nordic lakes. Sci. Rep. 6:31944. doi: 10.1038/srep31944
Fischer, J. M., Nicolai, J. L., Williamson, C. E., Persaud, A. D., and Lockwood, R. S. (2006). Effects of ultraviolet radiation on diel vertical migration of crustacean zooplankton: an in situ mesocosm experiment. Hydrobiologia 563, 217–224. doi: 10.1007/s10750-005-0007-x
Geddes, P. (2009). Decoupling carbon effects and UV protection from terrestrial subsidies on pond zooplankton. Hydrobiologia 628, 47–66. doi: 10.1007/s10750-009-9745-5
Gjessing, E. T., Egeberg, P. K., and Håkedal, J. (1999). Natural organic matter in drinking water—the “NOM-Typing Project”, background and basic characteristics of original water samples and NOM isolates. Environ. Int. 25, 145–159. doi: 10.1016/S0160-4120(98)00119-6
Häder, D.-P., Helbling, E. W., Williamson, C. E., and Worrest, R. C. (2011). Effects of UV radiation on aquatic ecosystems and interactions with climate change. Photochem. Photobiol. Sci. 10, 242–260. doi: 10.1039/c0pp90036b
Häder, D.-P., and Sinha, R. P. (2005). Solar ultraviolet radiation-induced DNA damage in aquatic organisms: potential environmental impact. Mutat. Res. 571, 221–233. doi: 10.1016/j.mrfmmm.2004.11.017
Hansson, L.-A., Bianco, G., Ekvall, M., Heuschele, J., Hylander, S., and Yang, X. (2016). Instantaneous threat escape and differentiated refuge demand among zooplankton taxa. Ecology 97, 279–285. doi: 10.1890/15-1014.1
Heuschele, J., Ekvall, M. T., Bianco, G., Hylander, S., and Hansson, L.-A. (2017). Context-dependent individual behavioral consistency in Daphnia. Ecosphere 8:e01679. doi: 10.1002/ecs2.1679
Hylander, S., Ekvall, M. T., Bianco, G., Yang, X., and Hansson, L.-A. (2014). Induced tolerance expressed as relaxed behavioural threat response in millimetre-sized aquatic organisms. Proc. Biol. Sci. 281:20140364. doi: 10.1098/rspb.2014.0364
Hylander, S., and Hansson, L.-A. (2010). Vertical migration mitigates UV effects on zooplankton community composition. J. Plankton Res. 32, 971–980. doi: 10.1093/plankt/fbq037
Hylander, S., and Hansson, L.-A. (2013). Vertical distribution and pigmentation of Antarctic zooplankton determined by a blend if UV radiation, predation and food availability. Aquat. Ecol. 47, 467–480. doi: 10.1007/s10452-013-9459-7
Hylander, S., Larsson, N., and Hansson, L.-A. (2009). Zooplankton vertical migration and plasticity of pigmentation arising from simultaneous UV and predation threats. Limnol. Oceanogr. 54, 483–491. doi: 10.4319/lo.2009.54.2.0483
IPCC (2014). Climate Change 2014: Synthesis Report. Contribution of Working Groups I, II and III to the Fifth Assessment Report of the Intergovernmental Panel on Climate Change. Geneva: IPCC. Available online at: https://www.ipcc.ch/report/ar5/syr/
Kissman, C. E. H., Williamson, C. E., Rose, K. C., and Saros, J. E. (2017). Nutrients associated with terrestrial dissolved organic matter drive changes in zooplankton:phytoplankton biomass ratios in an alpine lake. Freshw. Biol. 62, 40–51. doi: 10.1111/fwb.12847
Lampert, W. (1989). The adaptive significance of diel vertical migration of zooplankton. Funct. Ecol. 3, 21–27. doi: 10.2307/2389671
Lampert, W., and Taylor, B. E. (1985). Zooplankton grazing in a eutrophic lake: implications of diel vertical migration. Ecology 66, 68–82. doi: 10.2307/1941307
Larsen, S., Andersen, T., and Hessen, D. O. (2011). Climate change predicted to cause severe increase of organic carbon in lakes. Glob. Chang. Biol. 17, 1186–1192. doi: 10.1111/j.1365-2486.2010.02257.x
Leech, D. M., Padeletti, A., and Williamson, C. E. (2005). Zooplankton behavioral responses to solar UV radiation vary within and among lakes. J. Plankton Res. 27, 461–471. doi: 10.1093/plankt/fbi020
Leech, D. M., and Williamson, C. E. (2001). In situ exposure to ultraviolet radiation alters the depth distribution of Daphnia. Limnol. Oceanogr. 46, 416–420. doi: 10.4319/lo.2001.46.2.0416
Li, P., and Redden, D. T. (2015). Comparing denominator degrees of freedom approximations for the generalized linear mixed model in analyzing binary outcome in small sample cluster-randomized trials. BMC Med. Res. Methodol. 15:38. doi: 10.1186/s12874-015-0026-x
Lima, S. L. (1998). Nonlethal effects in the ecology of predator—prey interactions: what are the ecological effects of anti-predator decision-making? Bioscience 48, 25–34. doi: 10.2307/1313225
Lindholm, M., Wolf, R., Finstad, A., and Hessen, D. O. (2016). Water browning mediates predatory decimation of the Arctic fairy shrimp Branchinecta paludosa. Freshw. Biol. 61, 340–347. doi: 10.1111/fwb.12712
Lushchak, V. I. (2011). Environmentally induced oxidative stress in aquatic animals. Aquat. Toxicol. 101, 12–30. doi: 10.1016/j.aquatox.2010.10.006
Monteith, D. T., Stoddard, J. L., Evans, C. D., de Wit, H. A., Forsius, M., Høgåsen, T., et al. (2017). Dissolved organic carbon trends resulting from changes in atmospheric deposition chemistry. Nature 450, 537–540. doi: 10.1038/nature06316
OECD (2012). OECD Guideline for the Testing of Chemicals No. 211: Daphnia magna Reproduction Test. Paris: OECD Publishing.
Pan, Y., Yan, S.-W., Li, R.-Z., Hu, Y.-W., and Chang, X.-X. (2017). Lethal/sublethal responses of Daphnia magna to acute norfloxacin contamination and changes in phytoplankton-zooplankton interactions induced by this antibiotic. Sci. Rep. 7:40385. doi: 10.1038/srep40385
Pellegri, V., Gorbi, G., and Buschini, A. (2014). Comet assay on Daphnia magna in eco-genotoxicity testing. Aquat. Toxicol. 155, 261–268. doi: 10.1016/j.aquatox.2014.07.002
Pinheiro, J., Bates, D., DebRoy, S., Sarkar, D., and R Core Team (2016). nlme: Linear and Nonlinear Mixed Effects Models. R Package Version 3.1-128. Available online at: https://CRAN.R-project.org/package=nlme
Qualls, R. G., Haines, B. L., and Swank, W. T. (1991). Fluxes of dissolved organic nutrients and humic substances in a deciduous forest. Ecology 72, 254–266. doi: 10.2307/1938919
Ranåker, L., Jönsson, M., Nilsson, P. A., and Brönmark, C. (2012). Effects of brown and turbid water on piscivore–prey fish interactions along a visibility gradient. Freshw. Biol. 57, 1761–1768. doi: 10.1111/j.1365-2427.2012.02836.x
Ranta, E., Bengtsson, J., and McManus, J. (1993). Growth, size and shape of Daphnia longispina, D. magna and D. pulex. Ann. Zool. Fennici 30, 299–311.
Rantala, M. V., Nevalainen, L., Rautio, M., Galkin, A., and Luoto, T. P. (2016). Sources and controls of organic carbon in lakes across the subarctic treeline. Biogeochemistry 129, 235–253. doi: 10.1007/s10533-016-0229-1
Rautio, M., and Korhola, A. (2002). Effects of ultraviolet radiation and dissolved organic carbon on the survival of subarctic zooplankton. Polar Biol. 25, 460–468. doi: 10.1007/s00300-002-0366-y
Rautio, M., and Tartarotti, B. (2010). UV radiation and freshwater zooplankton: damage, protection and recovery. Freshw. Rev. 3, 105–131. doi: 10.1608/FRJ-3.2.157
R Core Team (2017). R: A Language and Environment for Statistical Computing. Available online at: https://www.R-project.org/
Reichwaldt, E. S., Wolf, I. D., and Stibor, H. (2004). The effect of different zooplankton grazing patterns resulting from diel vertical migration on phytoplankton growth and composition: a laboratory experiment. Oecologia 141, 411–419. doi: 10.1007/s00442-004-1645-9
Rhode, S. C., Pawlowski, M., and Tollrian, R. (2001). The impact of ultraviolet radiation on the vertical distribution of zooplankton of the genus Daphnia. Nature 412, 69–72. doi: 10.1038/35083567
Rose, K. C., Williamson, C. E., Fischer, J. M., Connelly, S. J., Olson, M., Tucker, A. J., et al. (2012). The role of ultraviolet radiation and fish in regulating the vertical distribution of Daphnia. Limnol. Oceanogr. 57, 1867–1876. doi: 10.4319/lo.2012.57.6.1867
Schindelin, J., Arganda-Carreras, I., Frise, E., Kaying, V., Longair, M., Pietzsch, T., et al. (2012). Fiji: an open-source platform for biological-image analysis. Nat. Methods 9, 676–682. doi: 10.1038/nmeth.2019
Sih, A., Stamps, J., Yang, L. H., McElreath, R., and Ramenofsky, M. (2010). Behaviour as a key component of integrative biology in a human-altered world. Integr. Comp. Biol. 50, 934–944. doi: 10.1093/icb/icq148
Smith, K. C., and Macagno, E. R. (1990). UV photoreceptors in the compound eye of Daphnia magna (Crustacea, Branchiopoda). A fourth spectral class in single ommatidia. J. Comp. Physiol. A 166, 597–606. doi: 10.1007/BF00240009
Solomon, C. T., Jones, S. E., Weidel, B. C., Buffam, I., Fork, M. L., Karlsson, J., et al. (2015). Ecosystem consequences of changing inputs of terrestrial dissolved organic matter to lakes: current knowledge and future challenges. Ecosystems 18, 376–389. doi: 10.1007/s10021-015-9848-y
Sterr, B., and Sommaruga, R. (2008). Does ultraviolet radiation alter kairomones? An experimental test with Chaborus obscuripes and Daphnia pulex. J. Plankton Res. 30, 1343–1350. doi: 10.1093/plankt/fbn087
Strock, K. E., Theodore, N., Gawley, W. G., Ellsworth, A. C., and Saros, J. E. (2017). Increasing dissolved organic carbon concentrations in northern boreal lakes: implications for lake water transparency and thermal structure. J. Geophys. Res. Biogeosci. 122, 1022–1035. doi: 10.1002/2017JG003767
Tartarotti, B., Saul, N., Chakrabarti, S., Trattner, F., Steinberg, C. E. W., and Sommaruga, R. (2014). UV-induced DNA damage in Cyclops abyssorum tatricus populations from clear and turbid alpine lakes. J. Plankton Res. 36, 557–566. doi: 10.1093/plankt/fbt109
Thrane, J.-E., Hessen, D. O., and Andersen, T. (2014). The absorption of light in lakes: negative impact of dissolved organic carbon on primary productivity. Ecosystems 17, 1040–1052. doi: 10.1007/s10021-014-9776-2
Utne-Palm, A. C. (2010). Visual feeding of fish in a turbid environment: physical and behavioural aspects. Mar. Freshw. Behav. Physiol. 35, 111–128. doi: 10.1080/10236240290025644
Venables, W. N., and Ripley, B. D. (2002). Modern Applied Statistics With S-Plus. New York, NY: Springer.
Williams, B. L., and Edwards, A. C. (1993). Processes influencing dissolved nitrogen, phosphorus, and sulphur in soils. Chem. Ecol. 8, 203–215. doi: 10.1080/02757549308035309
Williamson, C. E., Fischer, J. M., Bollens, S. M., Overholt, E. P., and Breckenridge, J. K. (2011). Toward a more comprehensive theory of zooplankton diel vertical migration: integrating ultraviolet radiation and water transparency into the biotic paradigm. Limnol. Oceanogr. 56, 1603–1623. doi: 10.4319/lo.2011.56.5.1603
Williamson, C. E., Olson, O. G., Lott, S. E., Walker, N. D., Engstrom, D. R., and Hargreaves, B. R. (2001). Ultraviolet radiation and zooplankton community structure following deglaciation in Glacier Bay, Alaska. Ecology 82, 1748–1760. doi: 10.1890/0012-9658(2001)082[1748:URAZCS]2.0.CO;2
Williamson, C. E., Overholt, E. P., Pilla, R. M., Leach, T. H., Brentrup, J. A., Knoll, L. B., et al. (2015). Ecological consequences of long-term browning in lakes. Sci. Rep. 5:18666. doi: 10.1038/srep18666
Williamson, C. E., Saros, J. E., and Schindler, D. W. (2009). Sentinels of change. Science 323, 887–888. doi: 10.1126/science.1169443
Wolf, R., Andersen, T., Hessen, D. O., and Hylland, K. (2017). The influence of dissolved organic carbon and ultraviolet radiation on the genomic integrity of Daphnia magna. Funct. Ecol. 31, 848–855. doi: 10.1111/1365-2435.12730
Wolf, R., Thrane, J.-E., Hessen, D. O., and Andersen, T. (2018). Modelling ROS formation in boreal lakes from interactions between dissolved organic matter and absorbed solar photon flux. Water Res. 132, 331–339. doi: 10.1016/j.watres.2018.01.025
Keywords: behavioral traits, Daphnia magna, dissolved organic matter, ultraviolet radiation, video imaging
Citation: Wolf R and Heuschele J (2018) Water Browning Influences the Behavioral Effects of Ultraviolet Radiation on Zooplankton. Front. Ecol. Evol. 6:26. doi: 10.3389/fevo.2018.00026
Received: 15 November 2017; Accepted: 01 March 2018;
Published: 14 March 2018.
Edited by:
Jordi Figuerola, Estación Biológica de Doñana (CSIC), SpainReviewed by:
Karen Carleton, University of Maryland, College Park, United StatesLydia M. Mathger, Marine Biological Laboratory (MBL), United States
Copyright © 2018 Wolf and Heuschele. This is an open-access article distributed under the terms of the Creative Commons Attribution License (CC BY). The use, distribution or reproduction in other forums is permitted, provided the original author(s) and the copyright owner are credited and that the original publication in this journal is cited, in accordance with accepted academic practice. No use, distribution or reproduction is permitted which does not comply with these terms.
*Correspondence: Raoul Wolf, cmFvdWwud29sZkBpYnYudWlvLm5v