- Department of Behavioural Biology, University of Vienna, Vienna, Austria
Energy expenditure during winter can be reduced by expressing torpor, which is characterized by decreased metabolic rate and body temperature. In addition to deep, multiday torpor bouts alternating with short arousal episodes during the hibernation period, common hamsters can also enter shallow torpor bouts (STBs), lasting for < 24 h at a minimum body temperature between 30 and 20°C. Food supplements provided shortly before winter have been shown to shorten hibernation in males, but did not affect hibernation duration in females. In the presented study, we analyzed the expression of STBs and compared supplemented to unsupplemented common hamsters. Body temperature during winter was recorded using subcutaneously implanted data loggers (iButtons). The results revealed that supplemented males showed more STBs and thus spent more time in shallow torpor than unsupplemented individuals. The duration of STBs, however, was shorter in supplemented males and both mean and minimum body temperature were significantly higher compared to unsupplemented males. In females, shallow torpor expression did not differ between individuals with and without food supplements. STBs were mainly expressed before the onset of the first deep, multiday torpor bout, but the number of STBs was not related to that of deep torpor bouts. These results indicate that in males, shallow torpor combined with feeding on food stores could be the more appropriate overwintering strategy when sufficient external energy reserves are available. Females generally cache more food than males and are therefore assumed to be less affected by the additional food stores. These results underline the flexibility of the species in the use of heterothermy and could enable adequate and rapid responses to changes in food availability.
Introduction
Several bird and mammalian species have the capability to escape unfavorable environmental conditions by saving energy via reducing metabolic rate and body temperature during so-called torpor bouts. Two distinct types of such heterothermy can be distinguished: daily torpor and hibernation. Daily torpor is characterized by torpor bout durations of < 24 h, a mean minimum metabolic rate during torpor of about 35% of basal metabolic rate, and minimum body temperatures during torpor usually range between 30 and 20°C. In contrast, hibernators are able to express torpor bouts lasting for several days or weeks, minimum metabolic rate during torpor is reduced to 4–6% of basal metabolic rate, and body temperature usually drops close to ambient temperature, usually below 10°C (Geiser, 1988, 2004; Heldmaier and Ruf, 1992; Geiser and Ruf, 1995; Buck and Barnes, 2000; Heldmaier et al., 2004; Ruf and Geiser, 2015). In addition, animals expressing daily torpor usually continue foraging and the torpor-arousal cycle is entrained with the light-dark cycle, while hibernators rely on energy reserves (body fat or food stores) and the circadian clock appears to be strongly suppressed (Ruf and Geiser, 2015).
Common hamsters (Cricetus cricetus), classified as hibernators, terminate above-ground activity in autumn and remain inside their hibernacula until spring (Weinhold and Kayser, 2006; Siutz et al., 2016). During winter, they rely on food stores (Eibl-Eibesfeldt, 1953; Niethammer, 1982), although recent observations of foraging behavior above-ground during the active season indicate sex differences in energy accumulation strategies: females showed much more food caching activities than males, suggesting larger food stores and hence, a stronger reliance on external energy reserves, while males, which were mainly observed feeding above-ground, appeared to have higher body fat proportions than females, indicating that they rather use internal energy reserves for the winter period (Siutz et al., 2012). Moreover, previous studies demonstrated high individual variations in body temperature patterns during winter. In addition to typical deep torpor bouts, common hamsters are known to regularly express short and shallow torpor (Wollnik and Schmidt, 1995; Wassmer and Wollnik, 1997; Siutz and Millesi, 2017; Siutz et al., 2017), which is characterized by less pronounced drops in body temperature (with minimum values ranging between 30 and 20°C) and a duration shorter than 24 h. Several hibernating mammals express short and shallow torpor, primarily before or after the hibernation period, and although this type of torpor seems to be similar to daily torpor, the physiological mechanisms regulating temperature and metabolic rate resemble those of deep torpor bouts (Wilz and Heldmaier, 2000; Geiser, 2004; Sheriff et al., 2012; Ruf and Geiser, 2015). In common hamsters, however, shallow torpor occurred not only prior to or after, but also during the actual hibernation period, i.e., between deep torpor bouts (Wollnik and Schmidt, 1995; Wassmer and Wollnik, 1997). Finally, hamsters kept under laboratory conditions were found to enter shallow torpor primarily in the morning hours, which was significantly later compared to entry into deep torpor. This daily rhythm of torpor entrance, however, was not found under semi-natural conditions, i.e., without exposure to light-dark cycles (Wassmer and Wollnik, 1997; Wassmer, 1998), indicating that shallow torpor expressed by hamsters is functionally distinct from daily torpor.
In this study, we manipulated food store availability for the winter period in free-ranging common hamsters by providing individuals with additional food shortly before they immerged into their hibernacula. The analysis of hibernation patterns revealed that males with additional food hibernated for shorter periods and, correspondingly, spent less time in deep torpor compared to unsupplemented hamsters, while deep torpor expression was not affected in females (Siutz et al., 2018). The aim of the presented study was to compare patterns of shallow torpor expression between supplemented and unsupplemented hamsters to gain more insight in different overwintering strategies in this species and investigate potential relationships between deep and shallow torpor.
Methods
Ethical Statement
All procedures were performed in accordance with EU guidelines for the protection of animals used for scientific purposes (Directive 2010/63/EU) and were approved by the ethics committee of the Faculty of Life Sciences, University of Vienna (2015-010), the Austrian Federal Ministry of Education, Science and Research (GZ: BMWFW-66.006/0013-WF/V/3b/2015), and the City of Vienna (MA22-2484/10, MA22-310/11).
Field Techniques
Free-ranging common hamsters, inhabiting urban areas in southern Vienna (Austria), were monitored during their active season (March/April–October/November 2015) by applying capture-mark-recapture techniques. Hamsters were trapped with Tomahawk live traps and released into cone-shaped cotton sacks for investigation allowing animal handling without anesthesia. At first capture, sex and age (adult/juvenile) was determined and, in addition to permanent identification via a subcutaneously implanted transponder (Data Mars), each individual was fur-marked in different, distinguishable patterns for distance recognition. A detailed description of our field methods can be found elsewhere (Franceschini et al., 2007; Franceschini-Zink and Millesi, 2008b; Siutz et al., 2016). All hamsters were monitored until autumn when they terminate above-ground activity and immerge into their hibernaculum (Siutz et al., 2016). This enabled us to define the individual immergence date, which corresponded to the date when an individual was trapped or observed for the last time in autumn and was confirmed by plugged burrows. To detect potential activity during winter, we additionally plugged the burrows with leaves and monitored them at weekly intervals until spring, but we discovered no signs of surface activity. Beginning in March, when hamsters are expected to emerge from their hibernacula (Siutz et al., 2016), we checked the burrows and observed the surrounding area at daily intervals and recaptured active individuals. The individual emergence date was defined as the date when an individual was observed above-ground or trapped for the first time, which coincided with the day when we discovered the removed burrow plug.
Food Supplementation
To provide hamsters with additional food, we placed 500 g sunflower seeds (Dehner Natura, Dehner GmbH, Germany) in front of an individual's hibernaculum and permanently observed the burrow until the hamster had carried all seeds inside, guaranteeing that only the focal individual collected the seeds. We chose sunflower seeds because of their storability and high energetic content (2.45 MJ/100 g, 51.5 g total fat/100 g) (USDA, 2016). Moreover, sunflower seeds are partly available and collected by hamsters, and their nutrient and fatty acid composition is comparable to the natural winter diet of common hamsters consisting of, e.g., beechnuts, black walnuts, hazelnuts, or acorn at our study site (Roswag et al., 2018). Additionally, sunflower seeds are relatively small and can be quickly cached by hamsters, facilitating the experimental procedure. To ensure that the food was used for the winter period, we supplemented hamsters in late autumn (September/October) shortly before immergence into the hibernaculum.
Common hamsters are strictly protected by the Fauna-Flora-Habitat directives (Appendix IV) and the Bern Convention (Appendix II) and implantation of temperature data loggers (see methods section “hibernation patterns”) was permitted for 20 individuals (GZ BMWFW-66.006/0013-WF/V/3b/2015). By monitoring hamsters throughout the active season, we could certainly identify the hibernacula of 18 individuals in autumn. Considering this relatively small sample size and a rather high overwinter mortality rate in this species (on average about 60 %; Franceschini-Zink and Millesi, 2008a), we supplemented all 18 individuals and used hibernation data collected in unsupplemented hamsters in previous years as control (13 females, 13 males). Although this might be a limiting factor of the study, analyses of patterns of deep torpor expression in unsupplemented hamsters, which were already published, revealed no significant effect of year, neither on hibernation performance nor seasonal timing (Siutz et al., 2016). Furthermore, ambient temperatures recorded at our study site during autumn, winter, and spring, as well as, food availability and snow cover showed no inter-year variations and were similar in the season of food supplementation compared to previous years (Siutz et al., 2018). In addition, body mass was similar in males (335.6 ± 13.4 g, n = 19) and females (319 ± 16.8 g, n = 19) at immergence (Student's t-test: t = 0.776, p = 0.443) and did not differ between unsupplemented (332.1 ± 13.8 g, n = 26) and supplemented individuals (317 ± 16.1 g, n = 12; Student's t-test: t = 0.712, p = 0.483). Finally, the data set included different age classes (adults and subadults), but these were similarly distributed between years, sexes, and groups.
Body Temperature Recording
We recorded individual body temperature during winter at 90-min intervals using temperature data loggers (iButtons, DS1922L-F5#, range: −40 to +85°C, accuracy: ±0.5°C, Maxim Integrated Products International, Dublin, Ireland). Using iButtons to detect changes in body temperature is an approved method and was previously successfully applied in common hamsters (Siutz et al., 2016, 2017; Siutz and Millesi, 2017). Moreover, by using iButtons we could demonstrate that the time a hibernator spent inside its hibernaculum does not necessarily reflect the actual hibernation period (Siutz et al., 2016). The iButtons were coated in a mixture of resin (Elvax ethylene vinyl acetate, DuPont) and paraffin (potted mass: ~4.5 g) and were gas-sterilized before implantation. Hamsters were trapped at our field site in the morning and immediately transported to a veterinary clinic (~20 min) where the iButtons were implanted subcutaneously in the neck region (dorsal, between the scapulae) under isoflurane anesthesia. When the animals had recovered from anesthesia, they were returned to the field site (1–2 h after trapping) and released at their burrows, which were blocked in the meantime to prevent other hamsters from entering the burrows. The implantation was done several weeks before autumnal immergence to avoid potential surgery effects on the timing of hibernation. The iButtons were removed in spring using the same technique and was applied to all individuals used in the analyses. We were able to recover iButtons of 12 supplemented individuals (6 females, 6 males).
While regular, deep torpor bouts last for several days and body temperature drops close to ambient temperature (Wollnik and Schmidt, 1995; Wassmer and Wollnik, 1997; Wassmer, 2004; Siutz and Millesi, 2017; Siutz et al., 2017), distinctive shallow torpor bouts (STB) were, according to Wassmer and Wollnik (1997), defined as periods of body temperature between 30 and 20°C and a duration of ≤ 24 h (Figure 1). By combining both minimum body temperature and torpor bout duration, these two types of torpor can be well distinguished as no overlaps were detected (Figure 2). Shallow torpor bout duration ranged between 3 and 24 h, although most bouts ranged between 3 and 15 h (only 2 unsupplemented males showed one bout of 16 and 24 h, respectively; Figure 2). Previous studies documented another, intermediate type of torpor, which is also characterized by a duration shorter than 24 h, but body temperature drops below 20°C (Wassmer and Wollnik, 1997; Siutz and Millesi, 2017). In the presented study, this torpor type was expressed only in 3 out of 12 supplemented individuals (1 male with 1 bout and 2 females with 1 and 3 bouts, respectively), and in 3 out of 26 unsupplemented individuals (1 male and 2 females with 1 bout each). Due to this low number we excluded this torpor type from analyses. Shallow torpor was expressed by 75% of supplemented (9 out of 12; 5 males, 4 females) and 77 % of unsupplemented individuals (20 out of 26; 11 males, 9 females). Individuals which did not show STBs were evenly distributed among age and sex classes, and their immergence body mass was well within the range of those expressing shallow torpor.
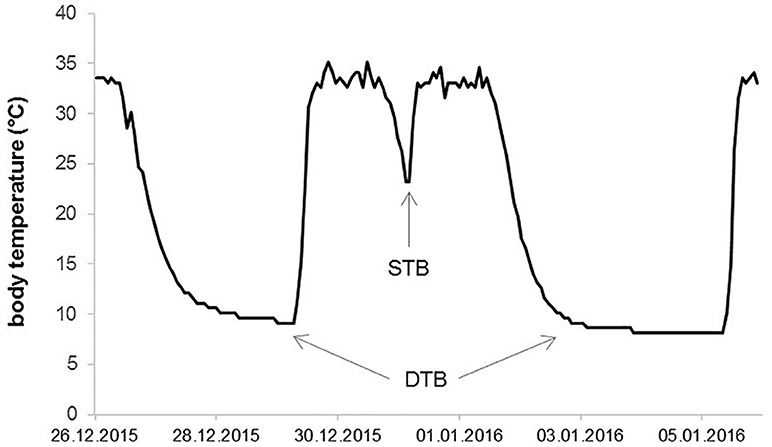
Figure 1. Representative section of a free-ranging common hamster's body temperature pattern demonstrating a shallow torpor bout (STB) between two regular, deep torpor bouts (DTB).
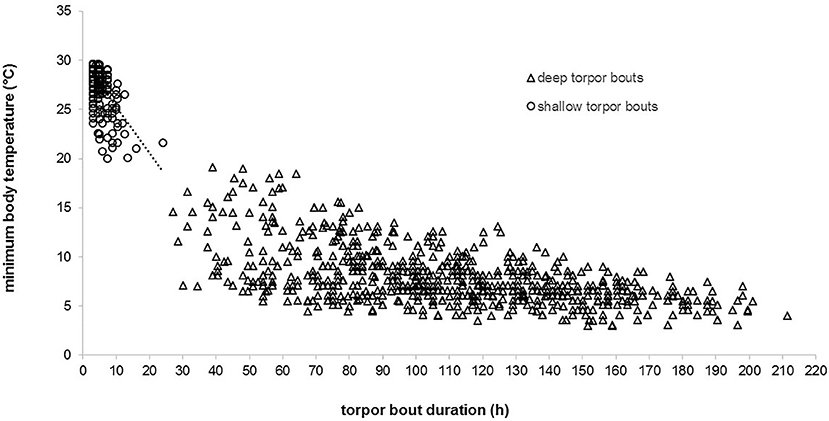
Figure 2. Minimum body temperature and torpor bout duration of every shallow and deep torpor bout expressed by individuals sampled in this study. Shallow torpor bout regression line: y = −0.4728x + 30.048, R2 = 0.2978.
We analyzed the number of STBs, the time spent in shallow torpor (total duration of all torpor bouts; calculated in hours, expressed as days), STB duration (beginning from the sampling interval when body temperature decreased below 30°C until it had reached 30°C again; calculated in hours, expressed as days), minimum body temperature during STB (lowest value of body temperature during a torpor bout), and mean body temperature during STB (beginning from the sampling interval when body temperature decreased below 30°C until it had reached 30°C again). Additionally, we analyzed the temporal (seasonal) pattern of shallow torpor occurrence, i.e., whether STBs were expressed before, during or after the actual hibernation period (defined as the period between the first and last deep torpor bout), but during the period without surface activity (from autumnal immergence to vernal emergence). For that, we compared the number of STBs during the pre-hibernation period (from autumnal immergence until entering the first deep torpor bout), the actual hibernation period (from onset of the first until termination of the last deep torpor bout), and the post-hibernation period (from termination of the last deep torpor bout, until vernal emergence).
Statistics
Statistical analyses were conducted in R (R Core Team, 2015) by using the packages “car” (Fox and Weisberg, 2011) for linear models, “nlme” (Pinheiro et al., 2015) for linear mixed models (LMEs), and “phia” (De Rosario-Martinez, 2015) for post-hoc analyses of interaction effects. The parameters number of STBs and time spent in shallow torpor were analyzed by linear models, including the parameters sex, group (supplemented/unsupplemented), and their interaction as predictor variables. In addition, we calculated the same model for these two parameters including individuals without shallow torpor expression. We applied LMEs for the parameters STB duration, minimum body temperature, and mean body temperature and included the parameters sex, group (supplemented/unsupplemented), and their interaction as fixed effects and individual identity as a random effect to correct for repeated measurements. The initial models for all response variables mentioned above included the parameters age (adult/subadult) and immergence body mass as predictor variables, but were removed from the final models as this contributed to AIC (Akaike's information criterion) reduction (Table S1). The parameter seasonal occurrence of STBs was analyzed by an LME with the parameters period (pre-hibernation/hibernation/post-hibernation) and group (supplemented/unsupplemented), as well as, their interaction as fixed effects and individual identity as a random effect. We tested model residuals for normality by Shapiro–Wilk tests and for homoscedasticity using Levene-tests and visually controlled residual and fitted value plots. Statistics were obtained from ANOVA (Type III) tables and all post-hoc analyses were Bonferroni-corrected. To analyze relationships between the number of STBs and the duration of the pre-hibernation period, the number of deep torpor bouts, as well as, body mass change over winter, we applied Spearman rank correlations. Significance level was set at p ≤ 0.05 and results are presented as means ± SE.
Results
Effects of Food Supplementation
We found sex-specific effects of food supplementation on shallow torpor expression (Table 1). Supplemented males showed more STBs [F(1, 25) = 11.640, p = 0.004], although of shorter duration (χ2 = 6.004, p = 0.029), and spent more time in shallow torpor than unsupplemented ones [F(1, 25) = 9.236, p = 0.011; Figure 3]. Even when including individuals without STBs in the analyses, the number of STBs [F(1, 34) = 7.729, p = 0.018] and time spent in shallow torpor [F(1, 34) = 5.574, p = 0.048] in supplemented males significantly exceeded that of unsupplemented ones. Additionally, minimum and mean body temperature during STBs were higher in supplemented than unsupplemented males (minimum: χ2 = 12.065, p = 0.001; mean: χ2 = 11.770, p = 0.001; Figure 4). In contrast to males, shallow torpor expression did not differ between females with and without additional food (p > 0.412 in all cases; Figures 3, 4).
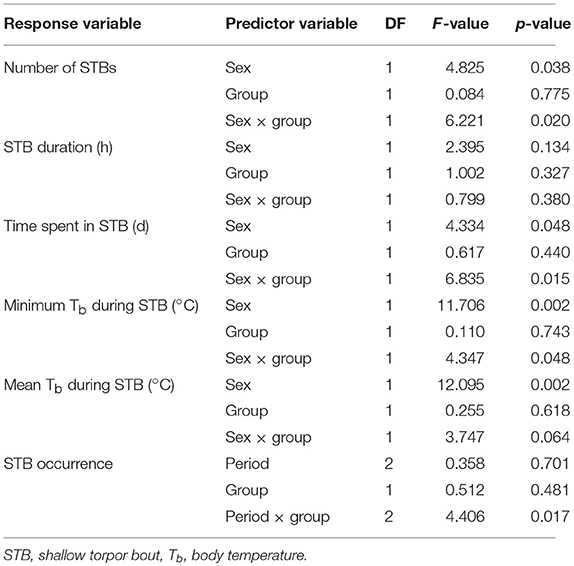
Table 1. ANOVA (Type III) table representing effects of sex and group (supplemented/unsupplemented) on shallow torpor expression, as well as, effects of period (before, during, after the actual hibernation period) and group (supplemented/unsupplemented) on shallow torpor occurrence in common hamsters.
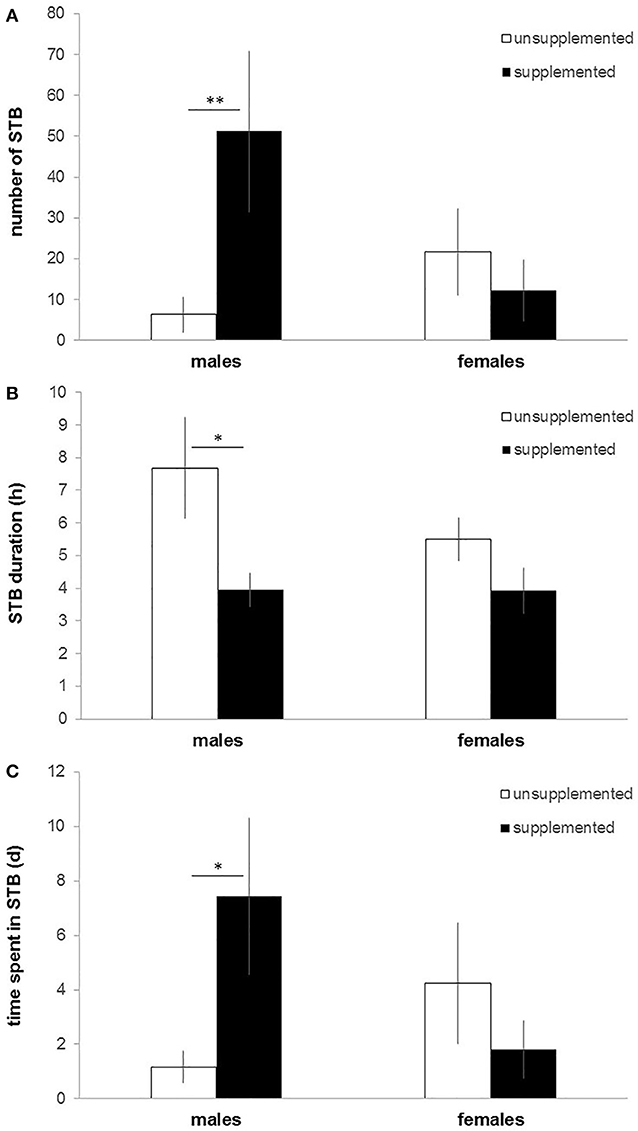
Figure 3. (A) Number of shallow torpor bouts (STB), (B) mean duration of STBs, and (C) time spent in shallow torpor in supplemented and unsupplemented males and females. *p ≤ 0.05, **p ≤ 0.01.
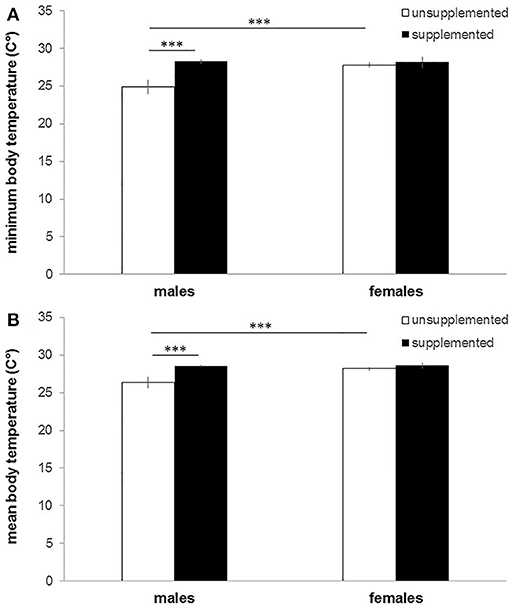
Figure 4. (A) Minimum body temperature and (B) mean body temperature during STBs in supplemented and unsupplemented males and females. ***p ≤ 0.001.
Irrespective of sex, supplemented individuals expressed more STBs during the pre-hibernation period (from autumnal immergence until entering the first deep torpor bout) than unsupplemented ones (χ2 = 11.074, p = 0.003), while no differences between the groups were found during the hibernation (from onset of the first until termination of the last deep torpor bout; χ2 = 0.550, p > 0.999) and post-hibernation period (from termination of the last deep torpor bout, until vernal emergence; χ2 = 0.033, p > 0.999; Figure 5). Supplemented hamsters showed more STBs during the pre- than during the post-hibernation period (χ2 = 9.176, p = 0.015; Figure 5), while the number of STBs did not differ between the other periods (pre-hibernation vs. hibernation: χ2 = 5.277, p = 0.130; hibernation vs. post-hibernation: χ2 = 0.536, p > 0.999; Figure 5). STB occurrence was similar in all three periods in unsupplemented individuals (p > 0.999 in all cases; Figure 5).
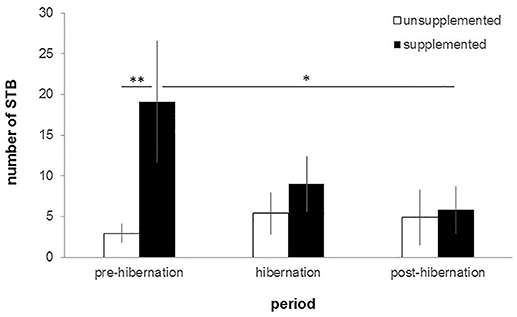
Figure 5. Number of STBs during pre-hibernation (period from immergence into the hibernaculum until hibernation onset, i.e., date of the first deep torpor onset), hibernation (period between onset of the first and termination of the last deep torpor bout), and post-hibernation (period from hibernation end until emergence from the hibernaculum) in supplemented and unsupplemented males and females. *p ≤ 0.05, **p ≤ 0.01.
Sex Differences
We found no sex differences among supplemented individuals (p > 0.184 in all cases; Figures 3, 4). Among unsupplemented hamsters, the number of STBs [F(1, 25) = 4.825, p = 0.075], STB duration (χ2 = 2.412, p = 0.241), and the time spent in shallow torpor [F(1, 25) = 4.334, p = 0.096] were similar between males and females (Figure 3). Unsupplemented females, however, expressed shallow torpor at higher minimum and mean body temperatures than unsupplemented males (minimum: χ2 = 11.788, p = 0.001; mean: χ2 = 12.180, p = 0.001; Figure 4).
Relationships Between Shallow and Deep Torpor Expression
In both unsupplemented and supplemented individuals, we found no relationships between the number of STBs and the duration of the pre-hibernation period (unsupplemented: rs = 0.161, p = 0.498, n = 20; supplemented: rs = 0.469, p = 0.203, n = 9), the number of deep torpor bouts (unsupplemented: rs = −0.320, p = 0.170, n = 20; supplemented: rs = −0.601, p = 0.087, n = 9), or body mass change over winter (unsupplemented: rs = −0.074, p = 0.778, n = 17; supplemented: rs = 0.393, p = 0.295, n = 9). Similar results were found in unsupplemented hamsters when including individuals without STB expression (STB number/pre-hibernation duration: rs = −0.048, p = 0.816, n = 26; STB number/number of deep torpor bouts: rs = −0.057, p = 0.781, n = 26; STB number/body mass change: rs = −0.041, p = 0.816, n = 21). Among supplemented hamsters, however, the number of STBs significantly increased with the duration of the pre-hibernation period (rs = 0.678, p = 0.015, n = 12), while no relationships were found between the number of STBs and the number of deep torpor bouts (rs = −0.508, p = 0.092, n = 12) and body mass change over winter (rs = 0.356, p = 0.256, n = 12).
Discussion
Supplemented males showed much more STBs when provided with additional food before winter compared to unsupplemented males while no differences were found among females. Previous analyses of deep torpor expression in the same individuals revealed that supplemented males showed a substantially delayed hibernation onset and entered the first deep torpor bout between December 28 and January 30, demonstrating that (depending on the date of immergence) they remained euthermic inside their burrows for 6–13 weeks (Siutz et al., 2018). The results of the presented study showed that this extended euthermic period was frequently interrupted by STBs as this type of torpor was primarily expressed before the individuals entered the first deep torpor bout. Shallow torpor bouts prior to hibernation onset have been documented in some hibernating species and were traditionally thought to be preparatory to deep torpor (Strumwasser, 1958), but more recent studies demonstrated that shallow torpor is not necessary to enter hibernation (e.g., Sheriff et al., 2012) and can be expressed even year-round (Wilz and Heldmaier, 2000). Common hamsters, however, seem to differ from other hibernators as particularly the number of STBs but also the associated minimum body temperatures are relatively high. In the presented study, the number of STBs appeared to be positively related to the duration of the pre-hibernation period in supplemented hamsters. One explanation could be that individuals with lower body mass at autumnal immergence required more time to improve body condition before entering hibernation; however, we found no effects of immergence body mass on shallow torpor expression. This indicates that the longer the hamsters delayed hibernation onset, the more STBs are needed to keep the balance between energy expenditure and food availability. We found, however, no relationship between shallow and deep torpor expression. Hence, not only hamsters with a short hibernation period increased the use of shallow torpor, indicating that these are not alternative strategies, which has also been demonstrated under laboratory conditions (Siutz and Millesi, 2017).
The pronounced expression of shallow torpor during the pre-hibernation period in supplemented males rather indicates that the energy expenditure caused by delayed hibernation onset can be, at least partly, compensated. Shallow torpor also represents reduced energy expenditure, albeit not to the same extent as deep torpor bouts with lower metabolic rates reflected by lower body temperatures. It seems, therefore, unlikely that energy savings by shallow torpor even come close to that of hibernation and individuals had to complement this deficit with feeding. In contrast to fat-storing hibernators, which usually show a marked atrophy of the gastrointestinal tract during the hibernation period (Carey, 1990; Hume et al., 2002), food-storing hibernators were found to maintain their intestinal morphology during hibernation and continue to digest when torpid (Humphries et al., 2001; Weitten et al., 2013) and a recent study in common hamsters demonstrated a full maintenance of nutrient assimilation capacities (Weitten et al., 2016). Although individuals were regularly observed feeding during longer euthermic periods between deep torpor bouts under laboratory conditions (Siutz C., personal observation), this could be rather disadvantageous for digestive processes during normal arousals which usually only last for a few hours. The better option, therefore, might be to consume hoarded food mainly during the pre-hibernation period and additionally make use of shallow torpor expression, which would also explain the shorter duration and higher body temperatures of STBs as this might be more beneficial for digestive processes. On the other hand, the higher body temperatures of shallow torpor in supplemented males might simply have resulted from shorter durations of these bouts. It seems, however, unlikely that these findings resulted from inaccurate measurements due to the relatively long sampling interval because only a few individuals showed some very short torpor bouts, but these were evenly distributed between the groups.
Supplemented males emerged from their hibernacula in spring with higher body mass than unsupplemented ones (Siutz et al., 2018). We found no direct relationship between the number of STBs and body mass change over winter, however, we were unable to measure body mass immediately before the onset of hibernation. The use of shallow torpor combined with food intake during the pre-hibernation period most likely resulted in improved body condition compared to autumnal immergence already prior to hibernation onset. Simultaneously, delaying hibernation probably reduced potential costs associated with torpor as negative effects (e.g., immune suppression, oxidative stress, impaired memory functions) might primarily be related to the low body temperature during deep torpor bouts (Ruf and Geiser, 2015).
With progressing winter and declining ambient temperatures, however, supplemented males hibernated (Siutz et al., 2018) and thus, reduced the number of STBs. During the coldest winter months (January/February; as reflected in minimum body temperatures during deep torpor), expression of deep torpor appeared to be more appropriate because energy savings by shallow torpor might not be sufficient during such periods. This is also supported by previous findings demonstrating that free-ranging hamsters always entered deep torpor during winter (Siutz et al., 2016, 2018), while individuals kept under laboratory or semi-natural conditions frequently showed a combination of shallow and deep torpor or even abandoned deep torpor and exclusively expressed shallow torpor (Wassmer and Wollnik, 1997; Wassmer, 2004). During the post-hibernation period, the extent of shallow torpor expression was similar to the hibernation period and significantly reduced compared to the pre-hibernation period. As hibernating mammals activate their reproductive system and males complete spermatogenesis before vernal emergence (Michener, 1983; Barnes et al., 1986; Barnes, 1996; Millesi et al., 1998), the expression of shallow torpor might constrain these processes.
Among female hamsters, shallow torpor expression did not differ between supplemented and unsupplemented individuals. This is not surprising as they also showed similar timing and duration of hibernation (Siutz et al., 2016). Both supplemented and unsupplemented females remained euthermic inside the hibernacula for several weeks before entering hibernation, although not to the same extent as supplemented males, and hence started to hibernate later than unsupplemented males (Siutz et al., 2016, 2018). One would, therefore, have expected a difference in the use of shallow torpor compared to unsupplemented males, which was only partly supported by our results. Although a marginal trend toward unsupplemented females expressing more STBs than unsupplemented males was observable, the time spent in shallow torpor was similar between the sexes. The reason for this remains unknown as we have no information on the actual quantity and quality of hoarded food in free-ranging hamsters. With our experimental design we aimed at enlarging food stores and ensuring that at least the provided external energy reserves are available (Siutz et al., 2018). The fact, however, that only males intensified shallow torpor in response to food supplements could indicate that mechanisms mediating food intake and/or shallow torpor expression might differ between males and females. Further studies, particularly including measurements of metabolic rate, are required to shed light on these sex-specific patterns of torpor expression.
Finally, the quality of energy reserves has been shown to affect torpor expression. In fat-storing hibernators, high concentrations of polyunsaturated fatty acids (PUFAs), in particular linoleic acid, in diets or white adipose tissue were found to enhance torpor expression (Geiser and Kenagy, 1987; Frank, 1992; Ruf and Arnold, 2008; Giroud et al., 2013; Arnold et al., 2015), while in the food-storing eastern chipmunk (Tamias striatus) high dietary PUFAs resulted in torpor reduction (Munro et al., 2005). It seems, however, unlikely that an increased PUFA content per se accounted for the results of the presented study. Although sunflower seeds are rich in PUFAs, particularly linoleic acid, the fatty acid composition is similar to the natural diet of common hamsters at our study site. Given that beechnuts and especially black walnuts have a high PUFA (and linoleic acid) content (USDA, 2016), it can be assumed that also unsupplemented hamsters had access to a high-PUFA diet during winter. And again, we provided both males and females with sunflower seeds, but only males increased shallow torpor expression.
In conclusion, our results underline the highly flexible use of heterothermy in common hamsters, especially in males. Such flexibility could be particularly adaptive under unpredictable environmental conditions (Canale and Henry, 2010). It is likely that the frequent use of shallow torpor, in combination with hibernation during the coldest periods, constitutes an optimal strategy for males when food stores, particularly of high energetic value, are available as it allows sufficient food intake facilitating body conditions in the burrow while simultaneously saving energy.
Data Availability
The raw data supporting the conclusions of this manuscript will be made available by the authors, without undue reservation, to any qualified researcher.
Author Contributions
CS collected field data, carried out the statistical analyses, and wrote the manuscript. VA collected field data, participated in the analyses, and reviewed drafts of the manuscript. EM conceived and designed the study, acquired funding of the study, and reviewed drafts of the manuscript. All authors read and approved the final manuscript.
Funding
This study was funded by the Austrian Science Fund (FWF, Project: 24280-B20).
Conflict of Interest Statement
The authors declare that the research was conducted in the absence of any commercial or financial relationships that could be construed as a potential conflict of interest.
Acknowledgments
We would like to thank M. Valent and A. Niebauer for their help in field work.
Supplementary Material
The Supplementary Material for this article can be found online at: https://www.frontiersin.org/articles/10.3389/fevo.2018.00190/full#supplementary-material
References
Arnold, W., Giroud, S., Valencak, T. G., and Ruf, T. (2015). Ecophysiology of omega fatty acids: a lid for every jar. Physiology 30, 232–240. doi: 10.1152/physiol.00047.2014
Barnes, B. M. (1996). “Relationships between hibernation and reproduction in male ground squirrels,” in Adaptations to the Cold: 10th International Hibernation Symposium, eds F. Geiser, A. J. Hulbert, and S. C. Nicol (Armidale, NSW: University of New England Press), 71–80.
Barnes, B. M., Kretzmann, M., Licht, P., and Zucker, I. (1986). The influence of hibernation on testis growth and spermatogenesis in the golden-mantled ground squirrel, Spermophilus lateralis. Biol. Reprod. 35, 1289–1297. doi: 10.1095/biolreprod35.5.1289
Buck, C. L., and Barnes, B. M. (2000). Effects of ambient temperature on metabolic rate, respiratory quotient, and torpor in an arctic hibernator. Am. J. Physiol. Regul. Integr. Comp. Physiol. 279, R255–R262. doi: 10.1152/ajpregu.2000.279.1.R255
Canale, C. I., and Henry, P.-Y. (2010). Adaptive phenotypic plasticity and resilience of vertebrates to increasing climatic unpredictability. Clim. Res. 43, 135–147. doi: 10.3354/cr00897
Carey, H. V. (1990). Seasonal changes in mucosal structure and function in ground squirrel intestine. Am. J. Physiol. Regul. Integr. Comp. Physiol. 259, R385–R392. doi: 10.1152/ajpregu.1990.259.2.R385
De Rosario-Martinez, H. (2015). phia: Post-Hoc Interaction Analysis. R package version 0.2-1. Available online at: https://CRAN.R-project.org/package=phia
Eibl-Eibesfeldt, I. (1953). Zur ethologie des hamsters (Cricetus cricetus L.). Z. Tierpsychol. 10, 204–254.
Fox, J., and Weisberg, S. (2011). An R Companion to Applied Regression, 2nd Edn. Thousand Oaks, CA: Sage. Available online at: http://socserv.socsci.mcmaster.ca/jfox/Books/Companion
Franceschini, C., Siutz, C., Palme, R., and Millesi, E. (2007). Seasonal changes in cortisol and progesterone secretion in Common hamsters. Gen. Comp. Endocrinol. 152, 14–21. doi: 10.1016/j.ygcen.2007.02.008
Franceschini-Zink, C., and Millesi, E. (2008a). “Population development and life expectancy in Common hamsters,” in The Common Hamster (Cricetus cricetus): Perspectives on an Endangered Species. Biosystematics and Ecology Series, eds E. Millesi, H. Winkler, and R. Hengsberger (Vienna: Austrian Academy of Science Press), 45–59.
Franceschini-Zink, C., and Millesi, E. (2008b). Reproductive performance in female common hamsters. Zoology 111, 76–83. doi: 10.1016/j.zool.2007.05.001
Frank, C. L. (1992). The influence of dietary fatty acids on hibernation by golden-mantled ground squirrels (Spermophilus lateralis). Physiol. Zool. 65, 906–920. doi: 10.1086/physzool.65.5.30158549
Geiser, F. (1988). Reduction of metabolism during hibernation and daily torpor in mammals and birds: temperature effect or physiological inhibition? J. Comp. Physiol. B Biochem. Syst. Environ. Physiol. 158, 25–37. doi: 10.1007/BF00692726
Geiser, F. (2004). Metabolic rate and body temperature reduction during hibernation and daily torpor. Annu. Rev. Physiol. 66, 239–274. doi: 10.1146/annurev.physiol.66.032102.115105
Geiser, F., and Kenagy, G. J. (1987). Polyunsaturated lipid diet lengthens torpor and reduces body temperature in a hibernator. Am. J. Physiol. Regul. Integr. Comp. Physiol. 252, R897–R901. doi: 10.1152/ajpregu.1987.252.5.R897
Geiser, F., and Ruf, T. (1995). Hibernation versus daily torpor in mammals and birds: physiological variables and classification of torpor patterns. Physiol. Zool. 68, 935–966. doi: 10.1086/physzool.68.6.30163788
Giroud, S., Frare, C., Strijkstra, A., Boerema, A., Arnold, W., and Ruf, T. (2013). Membrane phospholipid fatty acid composition regulates cardiac SERCA activity in a hibernator, the Syrian hamster (Mesocricetus auratus). PLoS ONE 8:e63111. doi: 10.1371/journal.pone.0063111
Heldmaier, G., Ortmann, S., and Elvert, R. (2004). Natural hypometabolism during hibernation and daily torpor in mammals. Respir. Physiol. Neurobiol. 141, 317–329. doi: 10.1016/j.resp.2004.03.014
Heldmaier, G., and Ruf, T. (1992). Body temperature and metabolic rate during natural hypothermia in endotherms. J. Comp. Physiol. B,. Biochem. Syst. Environ. Physiol. 162, 696–706. doi: 10.1007/BF00301619
Hume, I., Beiglböck, C., Ruf, T., Frey-Roos, F., Bruns, U., and Arnold, W. (2002). Seasonal changes in morphology and function of the gastrointestinal tract of free-living alpine marmots (Marmota marmota). J. Comp. Physiol. B,. Biochem. Syst. Environ. Physiol. 172, 197–207. doi: 10.1007/s00360-001-0240-1
Humphries, M. M., Thomas, D. W., and Kramer, D. L. (2001). Torpor and digestion in food-storing hibernators. Physiol. Biochem. Zool. 74, 283–292. doi: 10.1086/319659
Michener, G. R. (1983). Spring emergence schedules and vernal behavior of Richardson's ground squirrels: why do males emerge from hibernation before females? Behav. Ecol. Sociobiol. 14, 29–38. doi: 10.1007/BF00366653
Millesi, E., Huber, S., Dittami, J., Hoffmann, I., and Daan, S. (1998). Parameters of mating effort and success in male European ground squirrels, Spermophilus citellus. Ethology 104, 298–313. doi: 10.1111/j.1439-0310.1998.tb00070.x
Munro, D., Thomas, D. W., and Humphries, M. M. (2005). Torpor patterns of hibernating eastern chipmunks Tamias striatus vary in response to the size and fatty acid composition of food hoards. J. Anim. Ecol. 74, 692–700. doi: 10.1111/j.1365-2656.2005.00968.x
Niethammer, J. (1982). “Cricetus cricetus (Linnaeus, 1758) - Hamster (Feldhamster),” in Handbuch der Säugetiere Europas, Bd. 2/I., eds J. Niethammer and F. Krapp (Wiesbaden: Akademische Verlagsgesellschaft Wiesbaden), 7–28.
Pinheiro, J., Bates, D., DebRoy, S., Sarkar, D., and Team, R. C. (2015). nlme: Linear and Nonlinear Mixed Effects Models. R package version 3.1-122. Available online at: https://CRAN.R-project.org/package=nlme
R Core Team (2015). R: A Language and Environment for Statistical Computing. R Foundation for Statistical Computing, Vienna. Available online at: http://www.R-project.org
Roswag, A., Becker, N. I., Millesi, E., Otto, M. S., Ruoss, S., Sander, M., et al. (2018). Stable isotope analysis as a minimal-invasive method for dietary studies on the highly endangered Common hamster (Cricetus cricetus). Mammalia 82, 600–606. doi: 10.1515/mammalia-2017-0097
Ruf, T., and Arnold, W. (2008). Effects of polyunsaturated fatty acids on hibernation and torpor: a review and hypothesis. Am. J. Physiol. Regul. Integr. Comp. Physiol. 294, R1044–R1052. doi: 10.1152/ajpregu.00688.2007
Ruf, T., and Geiser, F. (2015). Daily torpor and hibernation in birds and mammals. Biol. Rev. 90, 891–926. doi: 10.1111/brv.12137
Sheriff, M. J., Williams, C. T., Kenagy, G., Buck, C. L., and Barnes, B. M. (2012). Thermoregulatory changes anticipate hibernation onset by 45 days: data from free-living arctic ground squirrels. J. Comp. Physiol. B Biochem. Syst. Environ. Physiol. 182, 841–847. doi: 10.1007/s00360-012-0661-z
Siutz, C., Franceschini, C., and Millesi, E. (2016). Sex and age differences in hibernation patterns of common hamsters: adult females hibernate for shorter periods than males. J. Comp. Physiol. B Biochem. Syst. Environ. Physiol. 186, 801–811. doi: 10.1007/s00360-016-0995-z
Siutz, C., and Millesi, E. (2017). Torpor patterns in common hamsters with and without access to food stores. J. Comp. Physiol. B,. Biochem. Syst. Environ. Physiol. 187, 881–888. doi: 10.1007/s00360-017-1093-6
Siutz, C., Nemeth, M., Wagner, K.-H., Quint, R., Ruf, T., and Millesi, E. (2017). Effects of food store quality on hibernation performance in common hamsters. PLoS ONE 12:e0185913. doi: 10.1371/journal.pone.0185913
Siutz, C., Pluch, M., Ruf, T., and Millesi, E. (2012). “Sex differences in foraging behaviour, body fat, and hibernation patterns of free-ranging Common hamsters,” in Living in a Seasonal World: Thermoregulatory and Metabolic Adaptations, eds T. Ruf, C. Bieber, W. Arnold, and E. Millesi (Heidelberg: Springer), 155–165. doi: 10.1007/978-3-642-28678-0_14
Siutz, C., Valent, M., Ammann, V., Niebauer, A., and Millesi, E. (2018). Sex-specific effects of food supplementation on hibernation performance and reproductive timing in free-ranging common hamsters. Sci. Rep. 8:13082. doi: 10.1038/s41598-018-31520-4
Strumwasser, F. (1958). Factors in the pattern, timing and predictability of hibernation in the squirrel, Citellus beecheyi. Am. J. Physiol. 196, 8–14. doi: 10.1152/ajplegacy.1958.196.1.8
USDA (2016). US Department of Agriculture, Agricultural Research Service, Nutrient Data Laboratory. USDANational Nutrient Database for Standard Reference, Release 28. Version Current: September 2015, slightly revised May 2016.
Wassmer, T. (1998). Die Zeitliche Organisation des Winterschlafs Beim Europäischen Feldhamster (Cricetus cricetus L.). München: Herbert Utz Verlag.
Wassmer, T. (2004). Body temperature and above-ground patterns during hibernation in European hamsters (Cricetus cricetus L.). J. Zool. 262, 281–288. doi: 10.1017/S0952836903004643
Wassmer, T., and Wollnik, F. (1997). Timing of torpor bouts during hibernation in European hamsters (Cricetus cricetus L.). J. Comp. Physiol. B Biochem. Syst. Environ. Physiol. 167, 270–279. doi: 10.1007/s003600050074
Weitten, M., Oudart, H., and Habold, C. (2016). Maintenance of a fully functional digestive system during hibernation in the European hamster, a food-storing hibernator. Comp. Biochem. Physiol. Part A Mol. Integr. Physiol. 193, 45–51. doi: 10.1016/j.cbpa.2016.01.006
Weitten, M., Robin, J.-P., Oudart, H., Pévet, P., and Habold, C. (2013). Hormonal changes and energy substrate availability during the hibernation cycle of Syrian hamsters. Horm. Behav. 64, 611–617. doi: 10.1016/j.yhbeh.2013.08.015
Wilz, M., and Heldmaier, G. (2000). Comparison of hibernation, estivation and daily torpor in the edible dormouse, Glis glis. J. Comp. Physiol. B Biochem. Syst. Environ. Physiol. 170, 511–521. doi: 10.1007/s003600000129
Keywords: hibernation, shallow torpor, food supplementation, sex differences, common hamster
Citation: Siutz C, Ammann V and Millesi E (2018) Shallow Torpor Expression in Free-Ranging Common Hamsters With and Without Food Supplements. Front. Ecol. Evol. 6:190. doi: 10.3389/fevo.2018.00190
Received: 30 July 2018; Accepted: 29 October 2018;
Published: 16 November 2018.
Edited by:
Jordi Figuerola, Estación Biológica de Doñana (EBD), SpainReviewed by:
Gerhard Koertner, University of New England, AustraliaThomas Wassmer, Siena Heights University, United States
Copyright © 2018 Siutz, Ammann and Millesi. This is an open-access article distributed under the terms of the Creative Commons Attribution License (CC BY). The use, distribution or reproduction in other forums is permitted, provided the original author(s) and the copyright owner(s) are credited and that the original publication in this journal is cited, in accordance with accepted academic practice. No use, distribution or reproduction is permitted which does not comply with these terms.
*Correspondence: Carina Siutz, Y2FyaW5hLnNpdXR6QHVuaXZpZS5hYy5hdA==