- 1Biology Department, Gonzaga University, Spokane, WA, United States
- 2Museum für Naturkunde, Leibniz Institute for Research on Evolution and Biodiversity, Berlin, Germany
- 3Animal Behavior Lab, Free University Berlin, Berlin, Germany
- 4Smithsonian Tropical Research Institute, Barro Colorado Island, Ancón, Panama
The spatiotemporal distribution of females is a major factor affecting animal social systems. Predation risk and the distribution of feeding resources often determine where females are found, but abiotic factors (e.g., temperature) can also shape the distribution of females and therefore variation in social organization and mating systems. Given the predicted future changes in climatic variation, it is vital to understand how animal mating systems and the sexual selection process may be altered by temperature. In bats, female distribution is tightly linked to roosting ecology and particularly to the microclimatic conditions at the roost. Proboscis bats (Rhynchonycteris naso) form cohesive and stable multi-male-multi-female groups and inhabit exposed day roosts (e.g., tree trunks, vines, buildings). Strong selection to remain inconspicuous to visually oriented predators in the exposed day roosts has been suggested to promote a rather rare male mating strategy termed site-specific dominance where males defend females directly but are successful in doing so only in their own territory. The choice of open-roost structures can result in the bats roosting under direct sunlight, making individuals susceptible to overheating. Here we investigate whether regular relocations of R. naso social groups among male territories are a mechanism of behavioral thermoregulation. Our results suggest that in general R. naso choose the warmest suitable roost sites within a roost, possibly to minimize the energetic costs of thermoregulation. However, on days with high midday temperatures at the primary roost site, bats commonly relocate to alternative, cooler sites within their roosts. These thermoregulatory relocations entail that a social group regularly switches among the territories of several males. Thus, the need for behavioral thermoregulation determines the spatial distribution of females and shapes the mating opportunities of males during the day. This is supported by our result that territorial males defending primary roost sites are reproductively more successful than territorial males of alternate roost sites. In line with other studies, our findings suggest that the increase in ambient temperatures associated with climate change has the potential to affect the intensity of sexual selection in bat species and may have far-reaching behavioral, demographic, and evolutionary consequences for their populations.
Introduction
The spatial and temporal distribution of females is a crucial determinant of male reproductive strategies and the degree of polygamy and, thus, a major factor affecting variation in social systems (Emlen and Oring, 1977; Shuster and Wade, 2003). Potential reproductive rates differ markedly between the sexes. Reproduction of males is typically limited by access to females whereas female fecundity relies heavily on access to resources. Hence, in general males are expected to pursue the distribution of females (Trivers, 1972; Clutton-Brock, 1989; Clutton-Brock and Parker, 1992). Ecological factors such as predation risk as well as the abundance and distribution of feeding resources are most frequently invoked in explaining female distribution and, thus, in determining variation in mammalian social organization (e.g., solitary vs. group living, Lott, 1984; Gehrt and Fritzell, 1998; Maher and Burger, 2011) and mating systems (e.g., monogamous vs. polygynous systems, Emlen and Oring, 1977; Maher and Burger, 2011).
Climatic variation has also been reported as a crucial factor that can shape female distribution in space and time and animal mating patterns, the understanding of which has become critically important given the predicted, continued increase of mean global surface air temperature and that extreme temperature and precipitation events are likely becoming more common (Isaac, 2009; Collins et al., 2013). For example, in the sea lion family (Otariidae) behavioral thermoregulation appears to determine the distribution of females and consequently variation in male mating strategies (Campagna and Le Boeuf, 1988; Francis and Boness, 1991). In another pinniped, the gray seal (Halichoerus grypus), weather conditions have been shown to affect inter-annual changes in the degree of polygamy through their effect on the availability of small pools of water necessary for female behavioral thermoregulation (Twiss et al., 2007). Thus, by altering the potential for polygamy, climatic variation may influence the intensity of sexual selection, an important evolutionary force that has rarely been considered to be affected by climatic variation (e.g., West and Packer, 2002; Twiss et al., 2007).
In bat species that live in year-round male-female associations, the spatiotemporal distribution of females is tightly linked to roosting ecology (McCracken and Wilkinson, 2000; Kunz and Lumsden, 2003; Campbell, 2008; Chaverri and Kunz, 2010; Sagot and Stevens, 2012). Bats rely on shelters that protect them from inclement weather and predators (Entwistle et al., 1997; Sedgeley, 2001). Roosts are important sites promoting complex social interactions, and are sites where bats mate, raise their pups, digest food and hibernate. As a result, roost selection is assumed to have played a pivotal role for the ecology and evolution of bats (Kunz, 1982; Kunz and Lumsden, 2003; Chaverri and Kunz, 2010). Moreover, suitable roosting space is attractive for females, and defensible by males, and might explain the high incidence of roost site based resource-defense polygyny in many tropical bat species (e.g., Storz et al., 2000; Hodgkison et al., 2003; Dechmann et al., 2005; Chaverri and Kunz, 2006). However, the way in which roosts affect social behavior and mating system variation of bats is still poorly understood (Kunz and Lumsden, 2003; Chaverri and Kunz, 2010; Sagot and Stevens, 2012).
The roost microclimate and particularly temperature is likely one of the most important factors affecting roost choice by bats (Bronrier et al., 1999; Kerth et al., 2001; Dechmann et al., 2004; Patriquin et al., 2016). Due to their small size, large lungs, and naked flight membranes, bats must sacrifice a comparably greater share of their daily energy intake to compensate for heat loss and maintain an optimal body temperature of 35–39°C (Neuweiler, 2000). Warm roosts have been shown to be especially important for females due to the positive effects on gestation, pup development and survival (e.g., Mcnab, 1982; Speakman and Racey, 1987; Grinevitch et al., 1995). Accordingly, female bats have been shown to choose warm roosts and/or avoid torpor (i.e., the reduction of metabolic rate and body temperature) during the reproductive period in several species of bats (e.g., Eptesicus fuscus, Hamilton and Barclay, 1994; Plecotus auritus, Entwistle et al., 1997; Myotis bechsteinii, Kerth et al., 2001; Chalinolobus tuberculatus, Sedgeley, 2001; Pipistrellus pygmaeus, Lourenço and Palmeirim, 2004). Other studies have also provided evidence for fitness benefits of torpor to reproductive females. For example, in pregnant and lactating Myotis lucifugus, regular but short bouts of torpor appeared to be an important strategy for balancing the costs of reproduction (Dzal and Brigham, 2013).
Bats living in tropical areas often experience higher ambient temperatures which might constitute an important ecological constraint on roost selection and the distribution of females. Yet, the effects of hot roost microclimates on bat biology have received comparably little attention (e.g., Genoud, 1993; Rodríguez-Durán, 1995; Bronrier et al., 1999). In eastern Australia, several extreme heat events (>42°C) between 1994 and 2002 caused the death of at least 30,000 flying foxes of Pteropus poliocephalus and P. alecto (Welbergen et al., 2008). Bats respond to heat stress through various behaviors: self-anointing with saliva, panting, and wing fanning help to promote evaporative cooling (e.g., in Pteropodids, Bartholomew et al., 1964), and also actively select cooler roost sites (e.g., Lavia frons, Vaughan, 1987).
Sheltered roosts (e.g., caves, crevices, tree cavities) predominate among bats and are advantageous because of their permanency, microclimatic stability, efficient protection from predators, sunlight, and adverse weather (Kunz, 1982). In contrast, while external roosts (e.g., foliage, tree trunks) may not be limited in number, the bats dwelling in such roosts must deal with environmental extremes and their visibility to predators (Kunz, 1982; Kunz and Lumsden, 2003). In our study species, the proboscis bat, Rhynchonycteris naso, stable social groups with up to 50 individuals typically roost on exposed tree boles, branches, vines, and man-made structures (Goodwin and Greenhall, 1961; Bradbury and Emmons, 1974; Bradbury and Vehrencamp, 1976; Simmons and Voss, 1998).
The day roosts of R. naso are well-illuminated and sometimes exposed to direct sunlight, suggesting that strategies to counter high predation pressure and intense heat should have played a crucial role in the evolution of this species' ecology and social system (Günther et al., 2016). To remain inconspicuous to visually oriented predators during the day, R. naso maintain a regular distance of 5–10 cm to one another (i.e., no body contact), assume stereotypical, motionless postures, and possess a cryptic coloration and mottled, dorsal pelage (Dalquest, 1957; Bradbury and Emmons, 1974; Bradbury and Vehrencamp, 1976). Moreover, these bats evolved an unusual way of remaining cryptic even during motion; R. naso synchronize individual behaviors of comfort and self-care with lateral rocking of group members during gusts of wind, probably to mimic vegetation swaying in the wind (Knörnschild et al., 2009). The necessity to remain both alert (i.e., normothermic) and mostly motionless due to predation risk likely limits the thermoregulatory options available to R. naso in day roosts. Neither energy-conserving torpor nor conspicuous behaviors associated with evaporative cooling appear suitable thermoregulatory options, and were also never observed during our year-long observations (unpublished data). This suggests that the choice of roost sites with suitable temperatures is of great importance. Bradbury and Vehrencamp (1976) noted that R. naso used three to six roost sites between which bats moved as a unit at intervals. Similarly, Günther et al. (2016) showed that two social groups within one large roost regularly used alternative roost sites during midday and afternoon, suggesting that relocations among alternative roost sites may be related to an increase of temperature as the day progresses.
Rhynchonycteris naso employ a unique male mating strategy that involves elements of both direct female-defense as well as male territoriality (Nagy et al., 2013; Günther et al., 2016). Mixed-sex groups are stable year-round and contain about the same numbers of males and females in the day roost with high fidelity of both sexes to their social groups (Bradbury and Emmons, 1974; Bradbury and Vehrencamp, 1976; Günther et al., 2016). R. naso have two mating seasons per year: one postpartum estrus mating period (April-May) and one seasonal mating period in October–November (Bradbury and Vehrencamp, 1977; Günther et al., 2016). Thus, mating occurs during the hottest periods of the year (Clark, 2013). During the day, social groups often roost cohesively within the territory of one male (i.e., primary roost site) but may relocate together to territories of other males (i.e., alternate roost sites). At their own territory site, males are dominant over all other males in aggressive male-male interactions and in securing copulations. Günther et al. (2016) showed that four to five territorial males performed 82.6% (n = 114) of female-defense actions, 91.8% (n = 456) of copulation attempts rejected by females and 100% (n = 105) of copulations within their own territory. The remainder of observed female-defense actions, copulation attempts, and copulations were attributed to seven to nine of the 7–12 resident non-territorial males (58–75% of resident males were non-territorials, Günther et al., 2016). Thus, within the same social group, territorial males are only dominant over other males of the social group within their own territory; once the group relocates from one roost site (i.e., male territory) to a different site within the roost, another male of the group will successfully defend females and perform copulations (Günther et al., 2016). Hence, if regular relocations between male territories are related to temperature at the roost sites, then thermoregulatory needs and the roosting environment are likely to have shaped the mating strategies of males in R. naso.
This study investigates the pattern of roost site-use and associated temperature regimes available to social groups of the proboscis bat, R. naso, and explores how roost site-use shapes male reproductive opportunities and resulting paternity success. If roost site-use is related to thermoregulation, we hypothesized that primary roost sites will have overall higher temperatures compared to less frequently used, alternate sites. Given the exposed nature of R. naso roosts, we asked whether intense heat during midday may explain the regularly observed relocations among alternative roost sites of our study species. Thus, we predicted that the proportion of bats in alternate sites during midday and or afternoon would increase with higher mean, midday roost site temperature, and daily maximum air temperature to avoid overheating. Finally, for behavioral thermoregulation to shape the reproductive opportunities of males, we expected that territorial males in primary roost sites would be reproductively more successful than males holding alternate territories.
Methods
Field Methods
Data used in this study were collected from 2011 to 2016 (see Supporting Table S1 and Supporting Figure S1 for an overview of temporal overlap of data and their use in statistical analyses). All field data were collected at La Selva Biological Station of the Organization for Tropical Studies (Costa Rica, Province Heredia, 10°25′N, 84°00′W). The 3 day roosts involved in this study (Cabina 5, C; Casa Grande, CG; River Station, RS) are wooden, human-made structures on the station property and bats either roost outside, under the extending roof of inhabited station cabins (C and RS) or on wooden ceiling beams inside of an abandoned house (CG). The study roosts are part of a long-term project on the social system of R. naso and have been studied extensively since 2005 (Nagy et al., 2013; Günther et al., 2016, 2017). We used mist nets (Ecotone® monofilament, Gdynia, Poland) to capture bats when emerging from their roosts at dusk. We banded captured bats with colored plastic bands on their forearms (AC Hughes® Ltd., UK, size XCS) for individual identification. We collected a small tissue sample from the plagiopatagium using a biopsy punch (Stiefel®, 4 mm Ø). In addition, we sexed, weighed, and determined the age (juvenile, subadult, or adult) and reproductive status of all captured bats. See Nagy et al. (2013) and Günther et al. (2016) for more details on field methods.
Census Observations
We determined the composition of social groups during the day (group size, number, and identity of banded males and females, number of unbanded bats) on a daily to at least weekly basis during the following periods: April–December 2013 (C), April–November 2014 (C), October 2015 (C), June–July 2016 (C, CG, RS). Cabina 5 hosted two social groups, Cabina 5 Left (CL), and Cabina 5 Right (CR). River Station and Casa Grande each hosted one social group. Table 1 gives a detailed overview of the social groups' composition. Overall, banded bats showed high fidelity to their roosts and the individual composition of bats per roost did not change throughout a given day. Banded bats in CL and CR were present during 94–100%, in CG 80–86%, and in RS 60–68% of census observations [see Supporting Table S2 for details on stability of social groups during morning (06:00–10:00), midday (10:01–14:00), and afternoon (14:01–18:00)].
Roost Site-Use
We monitored all suitable roost sites for C, RS, and CG (Günther et al., 2017, MN unpublished data). Bats occupied four sites in the River Station (RS1, RS2, RS3, RS4), Casa Grande had two occupied sites (CG1, CG2), Cabina 5 Left had 4 occupied sites (CL1, CL2, CL3, CL4), and Cabina 5 Right had 4 occupied sites (CR1, CR2, CR3, CR4). The locations of these sites are illustrated in the Supporting Figures S2–S4.
We counted the number of bats per roost site 1–3 times per day and separately assessed site-use patterns for morning (06:00–10:00) and midday (10:01–14:00) /afternoon (14:01–18:00). Bat count data are available for the following periods and roosts: April–December 2013 (CL, CR), April–November 2014 (CL, CR), October 2015 (CL, CR), and June–July 2016 (CL, CR, CG, RS). We monitored site-use patterns for an average of 41.8 days per roost and study year (range 13–89 days, Supporting Table S3). As precautions to avoid bias, we randomized the order in which the sites were visited. Researchers, visitors, and La Selva staff maintained, if possible, a minimum distance of 4 m to roost sites to avoid human-induced relocation of the bats.
Temperature Recordings at Occupied Roost Sites
We installed iButton data loggers (DS1920, Maxim Integrated, San Jose, CA, USA) at all occupied roost sites (see Supporting Figures S2–S4 for details on iButton locations) and recorded roost site temperatures during October 2015 (CL, CR, n = 10 days) and June–July 2016 (CL, CR, n = 12 days; CG, n = 16 days; RS, n = 31 days). We installed one iButton per site. Hence, recorded temperature should be considered a close proxy for the temperature regime experienced by bats, but microclimatic effects related to the positioning of the iButtons may exist. We mounted the iButtons at night, when sites were uninhabited by bats, to avoid disturbing our study groups. About the size of a nickel (16.3 × 6.35 mm) and suspended by a plastic holder, we installed iButtons by improvised hangers to complement each roosting surface (i.e., tape, paperclips). In the River Station and Casa Grande roosts, we monitored sites higher than 7 m from the ground by positioning mist net poles (Ecotone® monofilament, Gdynia, Poland) with the logger attached on their ends to within 1 m of the roosting bats at the site. The iButtons recorded temperature every 30 min. Per roost and season, we monitored temperature on average for 15.2 days (range 10–31 days, Supporting Table S4). We used temperatures recorded between 06:00 and 18:00 to calculate daily minimum, daily mean, and daily maximum temperatures for each site (Supporting Table S4). We limited our study to include only days where all occupied sites per roost were monitored concurrently to obtain comparable temperature measurements for all sites of a roost.
Relocations Among Roost Sites and Mean, Midday Roost Temperature
We identified the primary and alternate roost sites of our social groups based on the results of the site-use analysis. The primary roost site per social group was defined as the roost site that was occupied most frequently by the respective social group. We subsequently investigated whether high midday temperatures explained relocations of bats from their primary to alternate roost sites. The highest temperatures were usually reached between the hours of 10:00 and 14:00 (i.e., during midday Supporting Figures S5, S6) and we hypothesized that bats relocate from their primary sites to avoid high midday temperatures. In addition, for days with relocations (i.e., defined as days where ≥50% of social group members roosted in alternate roost sites during midday and/or afternoon) we tested whether bats relocated to cooler alternate roost sites, by asking whether mean midday temperature was lower at the alternate roost sites as compared to the primary roost sites. We used roost site iButton temperature data recorded in October 2015 and June–July 2016 from CR and CL and iButton data recorded in June–July 2016 for RS and CG in this analysis. For the proportion of bats in alternate sites, we used roost site bat counts from days with simultaneous information on roost site temperature.
Relocations Among Roost Sites and Daily Maximum Air Temperature at La Selva
This analysis includes our more extensive site-use data set from CL and CR recorded in 2013 and 2014. Site-use data cover 89 midday or afternoon bat counts from April–December 2013 and 85 midday or afternoon bat counts from April–November 2014. In 2013 and 2014, bats in Cabina 5 primarily roosted in the same sites (i.e., CL1 and CR2; Günther et al., 2016) as shown in Table 2 for the 2015 and 2016 site-use data set. Roost site temperatures were, however, not available for 2013 and 2014. Instead, we used maximum daily air temperature data collected by the automated La Selva meteorological station located ~200 m away from Cabina 5, that can be downloaded from the OTS homepage [http://www.ots.ac.cr/meteoro/data/lsdata/LS%20daily%20T-air%20mean-max-min%20Apr1982-Dec2016%20(Feb%202017).xlsx]. Daily maximum air temperature at La Selva is a good proxy for the temperature regime experienced by R. naso in their roosts (See Supporting Figures S7–S10). We tested whether daily maximum air temperature at La Selva predicts the proportion of bats roosting in alternate sites in CR and CL during midday or afternoon.
Male Status and Reproductive Success
We used genetic paternity data and information on male status (non-territorial, territorial in primary site, territorial in alternate site) published in Günther et al. (2016) to investigate whether territorial males that held primary site territories in Cabina 5 (i.e., CL1 and CR2) were more reproductively successful than males that defended territories in alternate sites (i.e., CL2/3, CR1, and CR3). To investigate whether phenotypic characteristics of males may explain their paternity success, we also tested the effect of forearm size (i.e., as a proxy for body size).
The dataset for this analysis consisted of 5 pup cohorts (2011, 2013–2014) and a total of 52 offspring of the Cabina 5 roost (Table 5 and Günther et al., 2016). In 2013 and 2014, we sampled pup cohorts conceived during both yearly mating seasons (post-partum estrus mating period in April–May and seasonal mating period in October–November). Methodological details on the 10 highly polymorphic microsatellite markers (Nagy et al., 2010) used for parentage analyses with Cervus v. 3.0 (Kalinowski et al., 2007) can be found in Günther et al. (2016). We identified territorial males of the periods when pups were conceived based on night census observations (See Figure 1 and Tables S4–S6 in Günther et al., 2016). We determined territorial males by their high and exclusive nightly fidelity to a specific roost site [Fsite = 0.69–1.00, (Günther et al., 2016)]. Whereas, R. naso roost in cohesive groups during the day, both social groups of Cabina 5 split up among several sites during the night and territorial males roost almost exclusively within their own territory site. For 2013 and 2014, Günther et al. (2016) had determined the territorial males of CL1, CL2/3, CR2, and CR3 but not of CR1. Therefore, we reanalyzed the night census data using the same criteria as in Günther et al. (2016) and were able to determine three males that defended individual territory sites in CR1 during 2013–2014. During three mating periods CR1 had two adjacent male territories (Table 5).
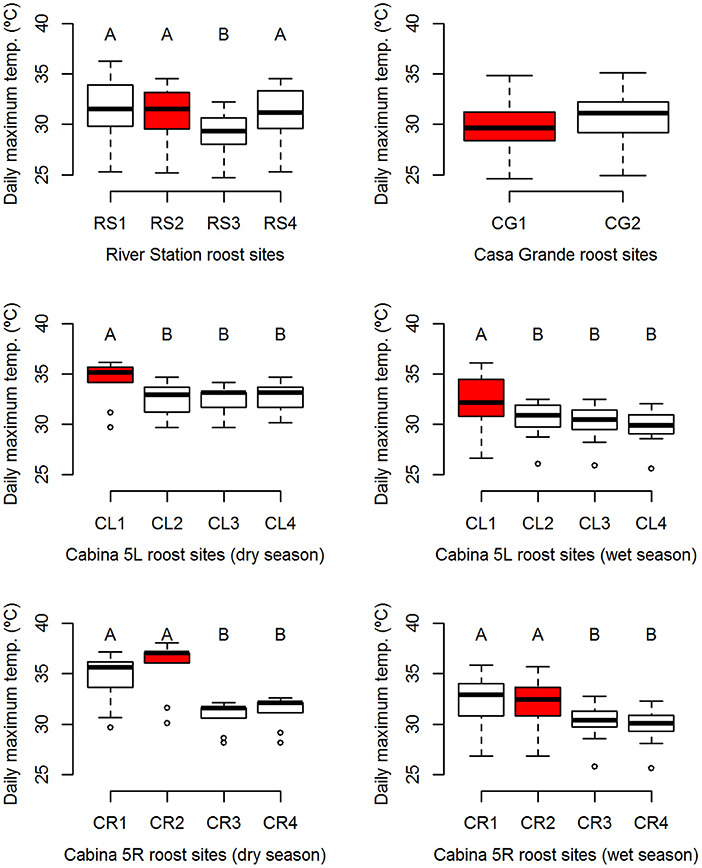
Figure 1. Boxplots of daily maximum temperatures at the studied roost sites per social group. Boxes show median and interquartile range (IQR) and whiskers represent 1.5 * IQR. Significant differences among roost sites in Tukey post-hoc tests are represented by letters above boxplots. Shared letters are statistically similar to one another while different letters represent roost sites with significantly different maximum temperatures. Primary roost sites are highlighted red.
Statistical Analysis
R-version 3.3.1 (R Core Team, 2016), Quantpsy Chi-square test (Preacher, 2001), IBM SPSS Statistics 20, and Microsoft Excel (version 14.5.5 for Mac) were used for data analyses. Means are reported with ± standard deviation (SD).
We used Chi-square tests (Quantspy Chi-square test; Preacher, 2001) to test whether the distribution of bats among primary and alternate roost sites differed between the morning and midday/afternoon for each social group. Number of bats in primary vs. alternate sites was used in statistical tests. Bat count data for this test came from CL and CR (October 2015, mating season) and from CL, CR, CG, and RS (June–July 2016, non-mating season).
We performed one-way ANOVAs followed by Tukey-HSD post-hoc tests to compare differences in daily temperature parameters (daily minimum, maximum, and average temperature) between roost sites for data from CG and RS (June–July 2016). Since we had data from both the mating season (dry season, October 2015) and non-mating season (wet season, June–July 2016) for CR and CL, we used two-way ANOVAs to compare the effect of both roost site and season on daily temperature parameters. Subsequently, we performed Tukey-HSD post-hoc tests for pair-wise comparisons among roost sites. We applied sequential Bonferroni corrections to account for multiple testing (Holm, 1979).
We fitted generalized linear mixed models (GLMMs) with a binomial error structure using the lme4-package (Bates et al., 2015) to test the effect of different explanatory variables on the proportion of bats that relocated to alternate sites during midday and/or afternoon.
For the site-use data set from October 2015 (CL and CR) and June–July 2016 (CL, CR, CG, and RS), for which we have simultaneous iButton measured roost site temperature, we fitted the proportion of bats at alternate sites during midday and/or afternoon as the dependent variable [R-function: “cbind (number of bats in alternate sites/number of bats in primary site)”]. We used mean midday temperature at the primary site (mean temperature between 10:00 and 14:00), social group (CL, CR, CG, RS) and season (mating period, non-mating period) as fixed factors in the model. The October 2015 data originated from the dry season that also corresponds to one of the two yearly mating periods of our study species. The June–July 2016 data came from the wet season which is a non-mating period of our study species (Günther et al., 2016). An observation level random effect was fitted as a random factor in the model to account for overdispersion. We used Tukey Post-hoc tests from the “multcomp” package (Hothorn et al., 2008) to obtain adjusted p-values for multiple comparisons of the social group factor.
For the site-use data set from April–December 2013 and April–November 2014 from CL and CR, for which we possess only simultaneous data on maximum daily air temperature at La Selva, we fitted the proportion of bats at alternate sites during midday and/or afternoon as the dependent variable [R-function: “cbind (number of bats in alternate sites/number of bats in primary sites)”] in a binomial GLMM. We used maximum air temperature at La Selva, social group (CL, CR) and the season (mating or non-mating season) as fixed factors in the model. This data set encompasses both mating seasons of R. naso: the post-partum oestrus mating season from April–May and the seasonal mating season from October–November (Günther et al., 2016). Year and an observation level random effect were fitted as random factors.
To test whether bats relocated to cooler alternate sites, we used a Wilcoxon signed-ranks test for paired samples to compare mean midday temperature at alternate and primary sites on days with relocations. We averaged mean midday temperature measurements at the primary and alternate sites per social group and season to assure independence of paired temperature differences. In this analysis, we used the iButton measured temperature data set from October 2015 (CL and CR) and June–July 2016 (CL, CR, CG, and RS).
We fitted a GLMM with a poisson error distribution to model the effect of male status (non-territorial, territorial in alternate sites, territorial in primary sites) and forearm size (i.e., as a proxy for body size) on paternity success. The number of pups a male fathered per cohort was used as the dependent variable. Male status and forearm size were modeled as fixed factors and male ID and pup cohort were fitted as random factors in the GLMM. We used Tukey Post-hoc tests from the “multcomp” package (Hothorn et al., 2008) to obtain adjusted p-values for multiple comparisons of the male status factor.
All GLMM models were checked for evidence of overdispersion using the “dispersion_glmer” function in the “blmeco” package (Korner-Nievergelt et al., 2015).
Ethical Statement
All field work was approved by Costa Rican authorities (MINAET Ministerio del Ambiente, Energia y Telecomunicaciones and SINAC Sistema Nacional de Areas de Conservación, Permit numbers: 033–2013-SINAC, SINAC-SE-GASP-PI-R-121–2013, R-006–2015-OT-CONAGEBIO, SINAC-SE-CUS-PI-R-088–2016) and followed the current laws of Costa Rica, Germany and the United States of America.
Results
Roost Site Use
All social groups showed a clear preference for a specific roost site within their roosts (Table 2, Supporting Figure 11). Overall, 81% of observed bats roosted at the RS2 site in River Station and 77% of observed bats roosted at the CG1 site in Casa Grande. The Cabina 5R group spent most time roosting at the CR2 site (59 and 89% of observations during the dry and wet season) and the Cabina 5L group primarily occupied the CL1 site (44 and 99% of observations during the dry and wet season). Site-use differed significantly between morning and midday/afternoon in Casa Grande and Cabina 5, but not in River Station. In Casa Grande, Cabina 5R during the dry and wet season, and Cabina 5L during the dry season, bats used the primary site more frequently during the morning than during midday/afternoon. In the wet season, the social group of Cabina 5L was almost always observed at its primary site (Table 2).
Temperature Regime of Roost Sites
In RS daily mean and maximum temperatures differed among roost sites, whereas CG showed no effect of site on any of the daily temperature parameters. CR showed significant differences in daily minimum, mean, and maximum temperature across roost sites, whereas in CL, site had a significant influence on daily maximum temperature only. Mean and maximum temperature showed significant seasonal effects with overall higher temperatures during the dry season (i.e., mating season) as compared to the wet season (i.e., non-mating season) in CL and CR (Table 3).
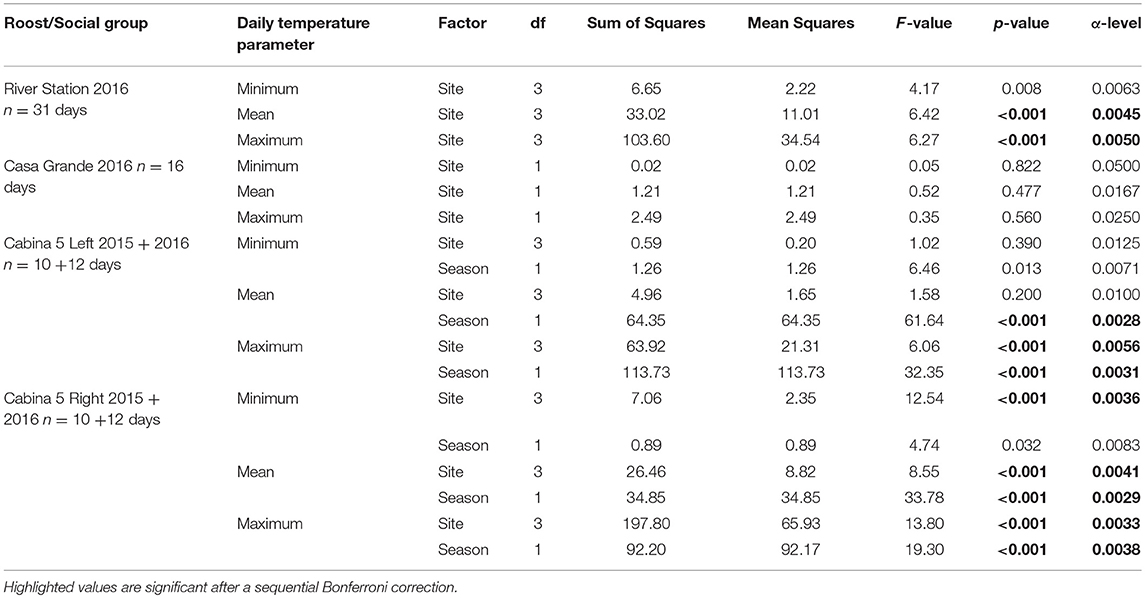
Table 3. Results of one-way ANOVAs (River Station and Casa Grande) and two-way ANOVAs (Cabina 5R/L) comparing differences in daily temperature parameters (site) and, if applicable, season (dry/mating season and wet/non-mating season) between roost sites.
Tukey-HSD post-hoc tests were performed on roost temperature data that showed a significant effect of site on the observed variation in temperature (Table 4). For the River Station, RS3 showed the only difference to other sites with RS3 having lower daily mean (26.6 ± 1.1°C) and daily maximum values (29.2 ± 1.9°C) than RS1 (daily mean: 28.0 ± 1.4°C, daily max: 31.5 ± 2.7°C), RS2 (daily mean: 27.7 ± 1.4°C, daily max: 31.1 ± 2.4°C), and RS4 (daily mean: 27.8 ± 1.4°C, daily max: 31.1 ± 2.4°C). Among the Cabina 5L sites, maximum temperature at CL1 (dry season max: 34.3 ± 2.1°C, wet season max: 32.3 ± 2.6°C) was significantly higher compared to the three other sites available to the social group (CL2: dry season max: 32.5 ± 1.8°C, wet season max: 30.5 ± 1.8, CL3: dry season max: 32.4 ± 1.6°C, wet season max: 30.1 ± 1.8°C, and CL4: dry season max: 32.7 ± 1.5°C, wet season max: 29.8 ± 1.7°C). Finally, for Cabina 5R, daily minimum temperature at CR2 (dry season min: 25.1 ± 0.3°C, wet season min: 24.6 ± 0.4°C) was significantly higher than values recorded at the three other sites, with CR1 (dry season min: 24.4 ± 0.4°C, wet season min: 24.1 ± 0.4°C), CR3 (dry season min: 24.1 ± 0.4°C, wet season min: 24.2 ± 0.5°C), and CR4 (dry season min: 24.1 ± 0.5°C, wet season min: 24.1 ± 0.5°C) showing no difference to each other. For daily mean temperature, CR1 (dry season mean: 28.9 ± 0.8°C, wet season mean: 27.6 ± 1.3°C) and CR2 (dry season mean: 29.6 ± 0.8°C, wet season mean: 27.9 ± 1.2°C) were warmer than CR4 (dry season mean: 28.0 ± 0.8°C, wet season mean: 26.8 ± 1.0°C), and CR2 was also significantly warmer than CR3 (dry season mean: 27.9 ± 0.8°C, wet season mean: 27.1 ± 1.1°C). Lastly, in Cabina 5R, CR1 (dry season max: 34.6 ± 2.5°C, wet season max: 32.3 ± 2.5°C) and CR2 (dry season max: 35.8 ± 2.7°C, wet season max: 32.1 ± 2.4°C) both showed significantly higher daily maximum temperatures than CR3 (dry season max: 30.9 ± 1.4°C, wet season max: 30.3 ± 1.8°C) and CR4 (dry season max: 31.4 ± 1.5°C, wet season max: 29.9 ± 1.8°C) but did not differ from each other. Thus, in Cabina 5 the two primary roost sites of bats (CR2 in Cabina 5R and CL1 in Cabina 5L) had the highest mean and maximum temperatures measured in the roost (Supporting Table S4, Figure 1). Primary roost sites in River Station and Casa Grande were not the warmest of the respective roosts, but daily mean temperatures were similar to temperatures of the primary Cabina 5 sites (daily mean temperatures of 27.0–27.9°C during the wet season, Supporting Table S4).
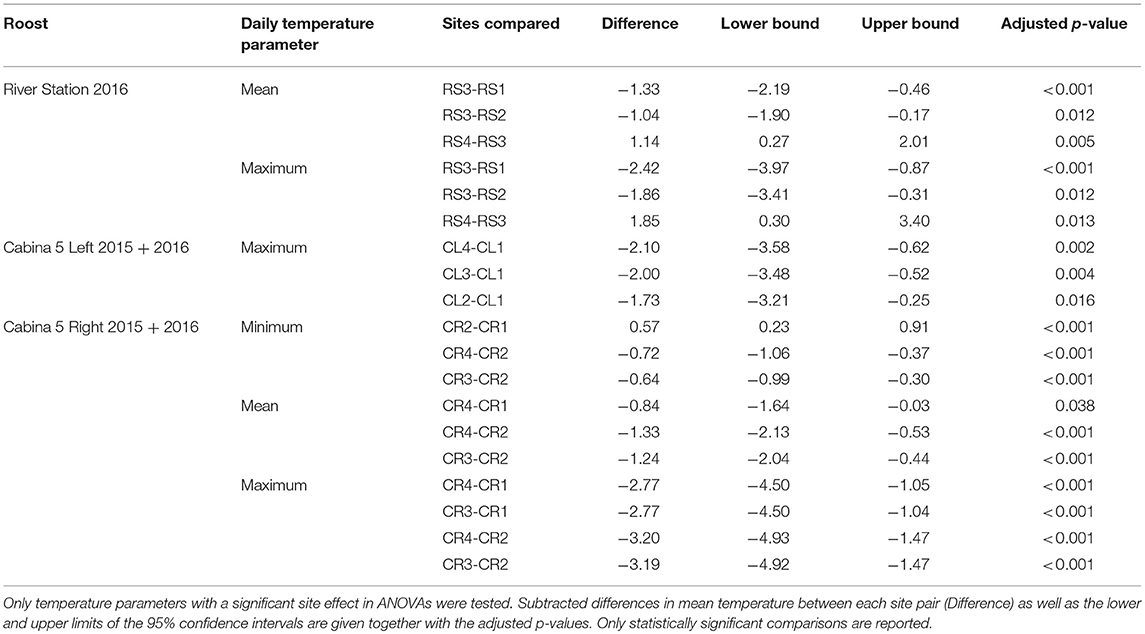
Table 4. Results of post-hoc comparisons using Tukey-HSD test to compare temperature parameters between individual roost sites.
Relocations Among Roost Sites and Mean, Midday Roost Temperature (CL, CR 2015; CL, CR, CG, RS 2016)
A GLMM on the proportion of bats roosting in alternate roost sites during midday and/or afternoon showed that the probability of bats to relocate to alternate roost sites increased with increasing mean, midday roost temperature (Estimate = 0.89, SE = 0.29, z = 3.03, p = 0.002, Figure 2A), strongly suggesting that bats relocated to avoid unfavorably high temperatures. Furthermore, a Wilcoxon signed-ranks test indicated that bats relocated to alternate roost sites with lower mean midday temperatures than at primary sites (n = 6, Z = −1.99, exact one-sided p = 0.031). Relocations were more frequent in the mating than in the non-mating season (non-mating season vs. mating season: Estimate = −6.17, SE = 1.83, z = −3.39, p < 0.001), likely because the mating seasons include the hottest months of the year. Social group influenced the proportion of bats in alternate roost sites. Bats in CL were less likely to relocate to alternate sites during midday and/or afternoon than bats in CR (CR vs. CL: Estimate = 4.60, SE = 1.56, z = 2.94, adjusted p = 0.017) and RS (RS vs. CL: Estimate = 5.37, SE = 1.78, z = 3.00, adjusted p = 0.014). Detailed modeling results are provided in the Supporting Information.
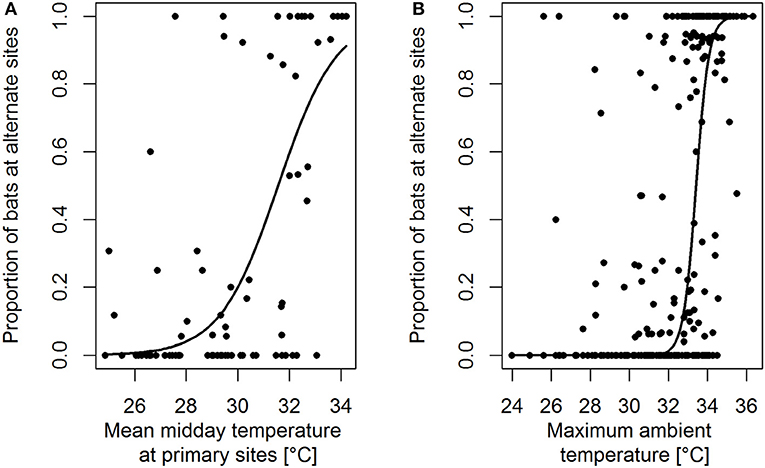
Figure 2. The proportion of bats in alternate roost sites during midday and/or afternoon increases as a function of (A) mean midday roost site temperature at the primary site (iButton and site-use data for CL, CR 2015 and CL, CR, CG, RS 2016) and (B) daily maximum ambient temperature (La Selva meteorological station data and site-use data for CL and CR 2013–2014). The black lines represent the fitted lines from binomial GLMMs.
Relocations Among Roost Sites and Maximum Air Temperature at La Selva (CL, CR 2013–2014)
A GLMM on the proportion of bats roosting in alternate roost sites during midday and/or afternoon showed that the probability of bats to relocate to alternate roost sites increased with increasing daily maximum air temperature (Estimate = 3.07, SE = 0.33, z = 9.27, p < 0.001, Figure 2B). Relocations were more frequent during the mating seasons than during the non-mating seasons (non-mating season vs. mating season: Estimate = −3.18, SE = 1.09, z = −2.91, p = 0.004) and occurred more frequently in CR than in CL (CR vs. CL: Estimate = 4.85, SE = 1.02, z = 4.76, p < 0.001). Detailed modeling results are provided in the Supporting Information.
Male Status and Paternity Success
Non-territorial males (n = 23 males) fathered on average 0.3 ± 0.6 pups per cohort. Territorial males defending alternate roost sites (n = 9 males) fathered 1.0 ± 1.1 pups per cohort and territorial males of primary roost sites (n = 4 males) fathered 2.2 ± 1.4 pups per cohort (Table 5). A GLMM on the number of pups per cohort showed that territorial males that defended primary roost sites had higher paternity success than non-territorial males (primary site territory vs. non-territorial: Estimate = 2.26, SE = 0.39, z = 5.8, adjusted p < 0.001) and territorial males of alternate site territories (primary site territory vs. alternate site territory: Estimate = 1.09, SE = 0.38, z = 2.9, adjusted p = 0.0115). Territorial males of alternate site territories were, however, reproductively more successful than non-territorial males (non-territorial vs. alternate territory: Estimate = −1.17, SE = 0.39, z = −3.0, adjusted p = 0.0072). Forearm size had no significant effect on male paternity success (Estimate = −0.28, SE = 0.18, z = −1.58, p = 0.1132). Detailed modeling results are provided in the Supporting Information.
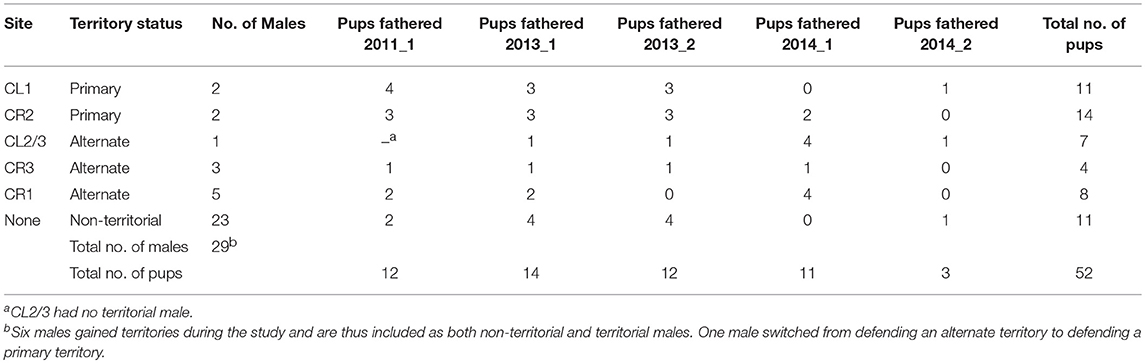
Table 5. Paternity distribution of pups in Cabina 5 Left (CL) and Cabina 5 Right (CR) by cohort year.
Discussion
The results of our study suggest that in addition to the already suspected effect of predation risk on variation in social organization and male mating strategies between day and night (Günther et al., 2016), roost site temperature affects the spatial distribution of social groups within day roosts of R. naso. Intriguingly, temperature related relocations in the day roost entail that several group members or even the entire social group switch from one male territory to another. The various roost sites used by a social group have been shown to correspond to the territories of various males and territorial males are most successful in defending females and copulating in their own territories (Günther et al., 2016). This is well in accordance with our results on paternity success from one roost (Cabina 5), which show that males holding a territory that is primarily occupied by the social group are reproductively more successful than males that hold alternate site territories. Relocations to alternate roost sites (i.e., territories) were more frequent during the two mating seasons in April–May and October–November, likely because these are among the hottest months of the year (Clark, 2013). Thus, the chances of males that defend alternate roost sites to have females roosting in their territory, and accordingly copulation opportunities during the day, are higher during the reproductively relevant mating seasons. Therefore, in R. naso, thermoregulatory needs appear to exert a significant effect on the males' reproductive opportunities during the day and may have been an important factor shaping the evolution of male-mating strategies and the social system of this bat species.
Overall, the most frequently used roost sites consistently showed higher, or at least similar, average temperatures compared to alternative roost sites within the roosts (Supporting Table S4, Table 4). This suggests that in general R. naso prefer and select the warmest available sites of a roost. In River Station and Casa Grande, some alternative roost sites showed slightly higher – though statistically non-significant – average daily mean and maximum temperatures compared to the primary sites. However, the RS1 site in River Station is located in the ceiling area of a stairway (Supporting Figure S3) commonly used by human residents and may, thus, in spite of favorable thermal conditions, be less attractive for the bats. For Casa Grande, the finding that CG1 and CG2 have statistically similar temperatures is also unexpected. This is perhaps due to the fact that the bats change not only location, but also the distance from the corrugated tin roof in their relocation from one site to the other. In CG1, R. naso roost within centimeters of the tin roof (Supporting Figure S12), while in CG2 (Supporting Figure S13), where they roost on a gable strut, they are as much as 2 meters from the tin roof, which is likely to conduct additional heat into the nearby wooden structure in CG1. This could mean that measuring the ambient temperature is far less effective in CG1 as it is in CG2 for reporting the thermal environment experienced by the bats.
In contrast to other tropical insectivorous bats, Neotropical emballonurids have been demonstrated to exhibit relatively high basal metabolic rates, which is possibly driven by foraging on predictable aerial insects (Saccopteryx bilineata, S. leptura, and Peropteryx macrotis; McNab, 1969; Genoud and Bonaccorso, 1986; Genoud et al., 1990). The two emballonurids, whose thermoregulatory behavior has been studied most thoroughly, remain alert and normothermic during daytime rest (S. bilineata, P. macrotis; Genoud and Bonaccorso, 1986; Genoud et al., 1990). The temperature in S. bilineata roosts averaged 26.1–26.5°C during the day, necessitating the expenditure of additional energy on top of an already high basal metabolic rate to maintain body temperature throughout the day (i.e., 35.5°C, Genoud and Bonaccorso, 1986). These high costs of thermoregulation are, according to Genoud and Bonaccorso (1986), balanced by the fitness benefits of maintaining normothermy that enable S. bilineata to avoid predators in their comparatively open and accessible roosts. Due to the high predation risk in the extremely exposed day roosts, R. naso are constantly alert and, most probably, normothermic during the day. During more than 10 years of behavioral observations of R. naso in their day roosts, bats never appeared torpid (unpublished observations); however, studies measuring body temperature and/or heart rate in R. naso are lacking. Furthermore, thermoregulatory “cuddling” does not exist in R. naso, which never roost with body contact to one another (Bradbury and Emmons, 1974; Bradbury and Vehrencamp, 1976). Hence, it appears plausible that the choice of the warmest suitable roost sites within a roost should allow R. naso to minimize energetic costs of thermoregulation and is likely a fitness relevant behavior. High temperatures are especially beneficial for female bats during the energetically demanding periods of pregnancy and lactation (e.g., Mcnab, 1982; Speakman and Racey, 1987; Grinevitch et al., 1995). Moreover, evidence for behavioral thermoregulation to save energy, whereby bats select optimal temperatures by changing locations within roosts, also comes from other temperate and tropical bat species such as E. fuscus (Williams and Brittingham, 1997), Mops condylurus (Bronrier et al., 1999), Myotis evotis (Chruszcz and Barclay, 2002), and P. pygmaeus (Lourenço and Palmeirim, 2004).
Our results indicate that the choice of warm roost sites can result in unfavorably high midday roost temperatures on hot days. Higher temperatures were clearly avoided by the bats, as suggested by the fact that virtually all R. naso relocated when mean midday temperature at the primary roost sites reached ~34°C or when ambient temperature at La Selva exceeded 35°C (Figures 2A,B). In addition, our results provide evidence that alternate roost sites occupied by relocated group members were cooler than primary roost sites. An alternative explanation for relocations during midday and/or afternoon may be avoidance of visually oriented predators, because changes in light intensity may increase visibility of R. naso. However, whereas the primary roost sites of three social groups were on the eastern side of their roost buildings (CL, CR, RS), potentially rendering the bats more visible during midday and/or afternoon, the primary roost site of CG bats was on the western side of the building and bats relocated to the eastern side. Also, R. naso in CG and RS roost sites are not visible from above to aerial predators because their roost sites are either inside the house or face inward. Thus, the observed relocations in R. naso are more probable to be a strategy of behavioral thermoregulation. Bats usually do not have access to water in their day roosts and would face a serious risk of dehydration through profuse evaporative cooling. Moreover, irrespective of thermoregulatory matters, there is reason to assume strong selection against conspicuous behaviors to promote evaporative cooling that would compromise the visual crypsis of R. naso in their day roosts (e.g., wing fanning or panting).
Due to the strong dependence on roosts and the major role temperature plays in roost choice of bats (Kunz, 1982), it may well be that roost site temperature is an important factor affecting the mating systems of other bat species as well. Particularly, in the frequently resource-defense based mating systems of many tropical bat species, where males defend roosting space rather than feeding resources (McCracken and Wilkinson, 2000), temperature may play an important role in determining which roost sites are indeed defensible resources for males. One notable example is the white-throated round-eared bat (Lophostoma silviculum), where males build their own shelters by excavating active arboreal termite nests. Temperature in active excavated termite nests was about 2.1–2.8°C warmer than in dead termite nests and tree holes, permitting the bats to save about 5% of their daily energy expenditure when roosting in active termite nests (Dechmann et al., 2004). Correspondingly, many caves have cavities in their ceilings that serve as heat traps and are used as roost sites (Kunz, 1982). Males of Jamaican fruit-eating bats (Artibeus jamaicensis) and greater spear-nosed bats (Phyllostomus hastatus) defend solution cavities against intrusion from other males or defend female-groups that roost in ceiling cavities directly (McCracken and Bradbury, 1981; Kunz et al., 1983).
The thermal regime of day roost sites may not be the only factor affecting the mating opportunities of males in R. naso. Male body size did not predict male reproductive success in R. naso, suggesting that larger males are not more successful in acquiring primary territory sites and/or that body size may not be a phenotypic trait selected by females. At present, the mechanism of territory acquisition and maintenance is unknown in R. naso, but we suspect that the numerous agonistic male-male interactions preceding the mating seasons, which are also accompanied by frequent and complex male vocalizations might be involved (Günther et al., 2017, LG, MK, MN unpublished data). In the closely related greater sac-winged bat S. bilineata, female choice plays a prominent role (Voigt et al., 2008). Studies involving S. bilineata have shown that olfactory cues are probably mediating MHC-dependent (i.e., major histocompatibility complex) female mate-choice and that male territorial song signals male quality (Behr et al., 2006; Santos et al., 2016). Likewise, olfactory cues and/or male vocalizations may convey a male's quality in R. naso and females may choose male-mating partners based on these or other characteristics. Opportunities for female choice seem, however, limited during the day due to strong predation pressure and the associated need for social groups to remain clumped and cryptic in their roosts. Females have only been observed to individually leave their primary roost site to visit and copulate with other territorial males during the night (Günther et al., 2016). Hence, male mating opportunities are possibly also mediated by female choice in addition to the defense of thermally favorable roost sites.
In the context of the anticipated future changes in climatic variation, knowledge on how weather conditions affect the evolutionary process of sexual selection and animal mating systems is vital, but our understanding of their interconnection is limited (West and Packer, 2002; Twiss et al., 2007; Isaac, 2009). Higher ambient temperature has for example been demonstrated to increase encounter rate and the degree of polygyny and polyandry in sand lizards (Lacerta agilis, Olsson et al., 2011) and the proportion of extra-pair litters increased with earlier onset of spring in alpine marmots (Marmota marmota, Bichet et al., 2016). Sexually selected traits may also be affected by temperature, as has been shown for the lion's mane (West and Packer, 2002). A recent study also found an effect of inter-annual variation in precipitation on the spatial distribution of females and the degree of polygyny in feral horses (Equus ferus caballus, Manning and McLoughlin, 2017). The degree to which sexual selection may depend on climatic variation in R. naso could be studied by testing whether male-reproductive skew varies with weather conditions. In hotter years relocations among male territories should be more frequent, potentially lowering reproductive skew. Likewise, reproductive skew and the level of aggression among males could be compared between R. naso roosts that are not subject to direct sun and where bats probably rarely relocate (e.g., below bridges) and roosts where relocations among male territories are frequent.
In conclusion, our results support those of a previous study (Günther et al., 2016) that the selective regime of an exceptionally exposed roost habitat was a prime factor shaping the mating strategies of male R. naso. In addition to the already suspected role of predation risk in promoting intraspecific variation in male mating strategies between day and night (Günther et al., 2016), the current results strongly suggest that behavioral thermoregulation decisively affects the distribution of females and male mating opportunities during the day. Males are probably limited in their options to exclude other males from their territory during the day, as overt aggression is likely to compromise the bats' crypsis. This results in a mating system that is mainly based on direct female defense during the day. However, the strategy of direct-female defense is superimposed on a territorial mating strategy and thermoregulatory requirements may have been important in promoting and/or maintaining territoriality in our study species during day. Our findings raise important questions of how climate change will influence the strength of sexual selection in day roosts of R. naso, but also in other bat species that use rather exposed roosts (Kunz, 1982; Kunz and Lumsden, 2003) and pose the possibility that climate change, by modulating the sexual selection process, can have profound consequences for the behavior, demography, and evolutionary dynamics of tropical bat populations.
Author Contributions
LW and MN designed the study. LW, LG, MK, and MN performed fieldwork. LW, LG, FM, MK, and MN analyzed data. LW and MN wrote the manuscript with input from LG, FM, and MK.
Conflict of Interest Statement
The authors declare that the research was conducted in the absence of any commercial or financial relationships that could be construed as a potential conflict of interest.
Acknowledgments
We thank the Costa Rican authorities, especially Javier Guevara and Lourdes Vargas for support and research permits and the Organization for Tropical Studies for technical assistance and logistics. This work was funded by the National Science Foundation Research Experiences for Undergraduates (NSF-REU) program (LW), by the Deutsche Forschungsgemeinschaft (DFG, MA1737/4, FM), and by grants from the German Academic Exchange Service (DAAD) and the Elsa-Neumann-Fellowship to LG. Many thanks to Dr. Marc-Oliver Rödel for providing iButtons, to Erica Carcelen and Don Jones for field work assistance, Leticia Classen Rodriguez for statistical advice, and to Dr. Carissa Ganong and Dr. Joey Haydock for feedback on the manuscript. Finally, we are most grateful to Dr. Geoffrey While, Dr. Teague O'Mara, and Dr. Cory Toth for the most helpful comments and suggestions on this manuscript.
Supplementary Material
The Supplementary Material for this article can be found online at: https://www.frontiersin.org/articles/10.3389/fevo.2018.00199/full#supplementary-material
References
Bartholomew, G. A., Leitner, P., and Nelson, J. E. (1964). Body temperature, oxygen consumption, and heart rate in three species of Australian flying foxes. Physiol. Zool. 37, 179–198. doi: 10.1086/physzool.37.2.30152330
Bates, D., Mächler, M., Bolker, B., and Walker, S. (2015). Fitting linear mixed effects models using Lme4. J. Stat. Softw. 16, 1–48. doi: 10.18637/jss.v067.i01
Behr, O., Von Helversen, O., Heckel, G., Nagy, M., Voigt, C. C., and Mayer, F. (2006). Territorial songs indicate male quality in the sac-winged bat Saccopteryx bilineata (Chiroptera, Emballonuridae). Behav. Ecol. 17, 810–817. doi: 10.1093/beheco/arl013
Bichet, C., Allainé, D., Sauzet, S., and Cohas, A. (2016). Faithful or not: direct and indirect effects of climate on extra-pair paternities in a population of Alpine marmots. Proc. R. Soc. B Biol. Sci. 283:20162586. doi: 10.1098/rspb.2016.2240
Bradbury, J. W., and Emmons, L. H. (1974). Social organization of some Trinidad bats. Ethology 36, 137–183.
Bradbury, J. W., and Vehrencamp, S. L. (1976). Social-organization and foraging in emballonurid bats 1. field studies. Behav. Ecol. Sociobiol. 1, 337–381. doi: 10.1007/BF00299399
Bradbury, J. W., and Vehrencamp, S. L. (1977). Social-organization and foraging in emballonurid bats 4. parental investment patterns. Behav. Ecol. Sociobiol. 2, 19–29. doi: 10.1007/BF00299285
Bronrier, G. N., Maloney, S. K., and Buffenstein, R. (1999). Survival tactics within thermally-challenging roosts: heat tolerance and cold sensitivity in the Angolan free-tailed bat, Mops condylurus. South African J. Zool. 34, 1–10. doi: 10.1080/02541858.1999.11448481
Campagna, C., and Le Boeuf, B. J. (1988). Thermoregulatory behavior of southern sea lions and its effect on mating strategies. Behaviour 107, 72–90. doi: 10.1163/156853988X00205
Campbell, P. (2008). The Relationship between roosting ecology and degree of polygyny in harem-forming bats: perspectives from Cynopterus. J. Mammal. 89, 1351–1360. doi: 10.1644/08-MAMM-S-059.1
Chaverri, G., and Kunz, T. H. (2006). Roosting ecology of the tent-roosting bat Artibeus watsoni (Chiroptera: Phyllostomidae) in southwestern costa rica. Biotropica 38, 77–84. doi: 10.1111/j.1744-7429.2006.00107.x
Chaverri, G., and Kunz, T. H. (2010). Ecological determinants of social systems: perspectives on the functional role of roosting ecology in the social behavior of tent-roosting bats. Adv. Study Behav. 42, 275–318. doi: 10.1016/S0065-3454(10)42009-4
Chruszcz, B. J., and Barclay, R. M. R. (2002). Thermoregulatory ecology of a solitary bat, Myotis evotis, roosting in rock crevices. Funct. Ecol. 16, 18–26. doi: 10.1046/j.0269-8463.2001.00602.x
Clutton-Brock, T. H. (1989). Mammalian mating systems. Proc. Royal Soc. Seri. B Biol. Sci. 236, 339–372. doi: 10.1098/rspb.1989.0027
Clutton-Brock, T. H., and Parker, G. A. (1992). Potential reproductive rates and the operation of sexual selection. Q. Rev. Biol. 67, 437–456. doi: 10.1086/417793
Collins, M., Knutti, R., Arblaster, J., Dufresne, J. L., Fichefet, T., Friedlingstein, P., et al. (2013). “Long-term climate change: projections, commitments and irreversibility,” in Climate Change 2013: The Physical Science Basis: Working Group I to the Fifth Assessment Report of the Intergovernmental Panel on Climate Change, eds T. F. Stocker, D. Qin, G. K. Plattner, M. Tignor, S. K. Allen, J. Boschung, A. Nauels, Y. Xia, V. Box, and P.M. Midgley (Cambridge, UK; New York, NY: Cambridge University Press), 1029–1136.
Dalquest, W. (1957). Observations on the sharp-nosed bat, Rhynchiscus naso (Maximilian). Tex. J. Sci. 9, 219–226.
Dechmann, D. K. N., Kalko, E. K. V., and Kerth, G. (2004). Ecology of an exceptional roost: energetic benefits could explain why the bat Lophostoma silvicolum roosts in active termite nests. Evol. Ecol. Res. 6, 1037–1050. doi: 10.5167/uzh-584
Dechmann, D. K. N., Kalko, E. K. V., Konig, B., and Kerth, G. (2005). Mating system of a Neotropical roost-making bat: the white-throated, round-eared bat, Lophostoma silvicolum (Chiroptera: Phyllostomidae). Behav. Ecol. Sociobiol. 58, 316–325. doi: 10.1007/s00265-005-0913-y
Dzal, Y. A., and Brigham, R. M. (2013). The tradeoff between torpor use and reproduction in little brown bats (Myotis lucifugus). J. Comp. Physiol. B. Biochem. Syst. Environ. Physiol. 183, 279–288. doi: 10.1007/s00360-012-0705-4
Emlen, S. T., and Oring, L. W. (1977). Ecology, sexual selection, and evolution of mating systems. Science 197, 215–223. doi: 10.1126/science.327542
Entwistle, A. C., Racey, P. A., and Speakman, J. R. (1997). Roost selection by the brown long-eared bat Plecotus auritus. J. Appl. Ecol. 34, 399–408. doi: 10.2307/2404885
Francis, J. M., and Boness, D. J. (1991). The effect of thermoregulatory behavior on the mating system of the Juan Fernandez Fur-Seal, Arctocephalus philippii. Behaviour 119, 104–126. doi: 10.1163/156853991X00391
Gehrt, S. D., and Fritzell, E. K. (1998). Resource distribution, female home range dispersion and male spatial interactions: group structure in a solitary carnivore. Anim. Behav. 55, 1211–1227. doi: 10.1006/anbe.1997.0657
Genoud, M. (1993). Temperature regulation in subtropical tree bats. Comparative biochemistry and physiology. Compar. Physiol. 104, 321–331.
Genoud, M., and Bonaccorso, F. J. (1986). Temperature regulation, rate of metabolism, and roost temperature in the greater white-lined bat Saccopteryx bilineata (Emballonuridae). Physiol. Zool. 59, 49–54. doi: 10.1086/physzool.59.1.30156089
Genoud, M., Bonaccorso, F. J., and Arends, A. (1990). Rate of Metabolism and temperature regulation in two small tropical insectivorous bats (Peropteryx macrotis and Natalus tumidirostris). Compar. Biochem. Physiol. A Physiol. 97, 229–234. doi: 10.1016/0300-9629(90)90177-T
Goodwin, G. G., and Greenhall, A. M. (1961). A review of the bats of trinidad and tobago: descriptions, rabies infection, and ecology. Bull. Am. Mus. Nat. Hist. 122, 187–302.
Grinevitch, L., Holroyd, S. L., and Barclay, R. M. R. (1995). Sex-differences in the use of daily torpor and foraging time by big brown bats (Eptesicus fuscus) during the reproductive season. J. Zool. 235, 301–309. doi: 10.1111/j.1469-7998.1995.tb05146.x
Günther, L., Knörnschild, M., Nagy, M., and Mayer, F. (2017). The evolution of a rare mammalian trait - benefits and costs of male philopatry in proboscis bats. Sci. Rep. 7:15632. doi: 10.1038/s41598-017-15990-6
Günther, L., Lopez, M. D., Knörnschild, M., Reid, K., Nagy, M., and Mayer, F. (2016). From resource to female defence: the impact of roosting ecology on a bat's mating strategy. R. Soc. Open Sci. 3:160503. doi: 10.1098/rsos.160503
Hamilton, I. M., and Barclay, R. M. R. (1994). Patterns of daily torpor and day-roost selection by male and female big brown bats (Eptesicus fuscus). Can. J. Zool. Revue Can. De Zool. 72, 744–749. doi: 10.1139/z94-100
Hodgkison, R., Balding, S. T., Akbar, Z., and Kunz, T. H. (2003). Roosting ecology and social organization of the spotted-winged fruit bat, Balionycteris maculata (Chiroptera: Pteropodidae), in a Malaysian lowland dipterocarp forest. J. Trop. Ecol. 19, 667–676. doi: 10.1017/S0266467403006060
Holm, S. (1979). A simple sequentially rejective multiple test procedure. Scand. J. Stat. 6, 65–70. doi: 10.2307/4615733
Hothorn, T., Bretz, F., and Westfall, P. (2008). Simultaneous inference in general parametric models. Biometr. J. 50, 346–363. doi: 10.1002/bimj.200810425
Isaac, J. L. (2009). Effects of climate change on life history: implications for extinction risk in mammals. Endanger. Species Res. 7, 115–123. doi: 10.3354/esr00093
Kalinowski, S. T., Taper, M. L., and Marshall, T. C. (2007). Revising how the computer program CERVUS accommodates genotyping error increases success in paternity assignment. Mol. Ecol. 16, 1099–1106. doi: 10.1111/j.1365-294X.2007.03089.x
Kerth, G., Weissmann, K., and König, B. (2001). Day roost selection in female Bechstein's bats (Myotis bechsteinii): a field experiment to determine the influence of roost temperature. Oecologia 126, 1–9. doi: 10.1007/s004420000489
Knörnschild, M., Harview, C., Moseley, R., and Von Helversen, O. (2009). Remaining cryptic during motion–behavioral synchrony in the proboscis bat (Rhynchonycteris naso). Acta Chiropterol. 11, 208–211. doi: 10.3161/150811009X465839
Korner-Nievergelt, F., Roth, T., Felten, S. V., Guelat, J., Almasi, B., and Korner-Nievergelt, P. (2015). Blmeco: Data Files and Functions Accompanying the Book “Bayesian Data Analysis in Ecology Using R. Amsterdam: Academic Press; BUGS and Stan.
Kunz, T. H. (1982). “Roosting ecology of bats,” in Ecology of Bats, ed T. H. Kunz (Boston, MA: Springer), 1–55. doi: 10.1007/978-1-4613-3421-7
Kunz, T. H., August, P. V., and Burnett, C. D. (1983). Harem social organization in cave roosting Artibeus jamaicensis (Chiroptera: Phyllostomidae). Biotropica 15, 133–138. doi: 10.2307/2387958
Kunz, T. H., and Lumsden, L. F. (2003). “Ecology of cavity and foliage roosting bats,” in Bat Ecology, eds T. H. Kunz and M. Fenton (Chicago, IL; London: The University of Chicago Press), 3–89.
Lott, D. F. (1984). Intraspecific variation in the social-systems of wild vertebrates. Behaviour 88, 266–325. doi: 10.1163/156853984X00353
Lourenço, S. I., and Palmeirim, J. M. (2004). Influence of temperature in roost selection by Pipistrellus pygmaeus (Chiroptera): relevance for the design of bat boxes. Biol. Conserv. 119, 237–243. doi: 10.1016/j.biocon.2003.11.006
Maher, C. R., and Burger, J. R. (2011). Intraspecific variation in space use, group size, and mating systems of caviomorph rodents. J. Mammal. 92, 54–64. doi: 10.1644/09-MAMM-S-317.1
Manning, J. A., and McLoughlin, P. D. (2017). Climatic conditions cause spatially dynamic polygyny thresholds in a large mammal. J. Anim. Ecol. 86, 296–304. doi: 10.1111/1365-2656.12608
McCracken, G. F., and Bradbury, J. W. (1981). Social-organization and kinship in the polygynous bat Phyllostomus hastatus. Behav. Ecol. Sociobiol. 8, 11–34. doi: 10.1007/BF00302840
McCracken, G. F., and Wilkinson, G. S. (2000). “Bat mating systems,” in Reproductive Biology of Bats, eds E. G. Crichton, and P. H. Krutzsch (San Diego, CA: Academic Press), 321–362. doi: 10.1016/B978-012195670-7/50009-6
McNab, B. K. (1969). Economics of temperature regulation in neotropical bats. Compar. Biochem. Physiol. 31, 227–268. doi: 10.1016/0010-406X(69)91651-X
Mcnab, B. K. (1982). “Evolutionary alternatives in the physiological ecology of bats,” in Ecology of Bats, ed T. Kunz (Boston, MA: Springer), 151–200.
Nagy, M., Günther, L., Knornschild, M., and Mayer, F. (2013). Female-biased dispersal in a bat with a female-defence mating strategy. Mol. Ecol. 22, 1733–1745. doi: 10.1111/mec.12202
Nagy, M., Ustinova, J., and Mayer, F. (2010). Characterization of eight novel microsatellite markers for the neotropical bat Rhynchonycteris naso and cross-species utility. Conserv. Genet. Resour. 2, 41–43. doi: 10.1007/s12686-009-9139-z
Neuweiler, G. (2000). “Heat and water balance,” in The Biology of Bats, ed G. Neuweiler (New York, NY: Oxford University Press), 63–97.
Olsson, M., Wapstra, E., Schwartz, T., Madsen, T., Ujvari, B., and Uller, T. (2011). In hot pursuit: fluctuating mating system and sexual selection in sand lizards. Evolution 65, 574–583. doi: 10.1111/j.1558-5646.2010.01152.x
Patriquin, K. J., Leonard, M. L., Broders, H. G., Ford, W. M., Britzke, E. R., and Silvis, A. (2016). Weather as a proximate explanation for fission-fusion dynamics in female northern long-eared bats. Anim. Behav. 122, 47–57. doi: 10.1016/j.anbehav.2016.09.022
Preacher, K. J. (2001). Calculation for the Chi-Square Test. An Interactive Calculation Tool for Chi-Square Tests of Goodness of Fit and Independence. Ohio State University. Available online at: http://quantrm2.psy.ohio-state.edu/kris/chisq/chisq.htm
Rodríguez-Durán, A. (1995). Metabolic rates and thermal conductance in four species of neotropical bats roosting in hot caves. Compar. Biochem. Physiol. Part A Physiol. 110, 347–355. doi: 10.1016/0300-9629(94)00174-R
Sagot, M., and Stevens, R. D. (2012). The evolution of group stability and roost lifespan: perspectives from tent-roosting bats. Biotropica 44, 90–97. doi: 10.1111/j.1744-7429.2011.00774.x
Santos, P. S., Courtiol, A., Heidel, A. J., Höner, O. P., Heckmann, I., Nagy, M., et al. (2016). MHC-dependent mate choice is linked to a trace-amine-associated receptor gene in a mammal. Sci. Rep. 6:38490. doi: 10.1038/srep38490
Sedgeley, J. A. (2001). Quality of cavity microclimate as a factor influencing selection of maternity roosts by a tree-dwelling bat, Chalinolobus tuberculatus, in New Zealand. J. Appl. Ecol. 38, 425–438. doi: 10.1046/j.1365-2664.2001.00607.x
Shuster, S. M., and Wade, M. J. (2003). Mating Systems and Strategies. Princeton, NJ: Princeton University Press.
Simmons, N. B., and Voss, R. S. (1998). The mammals of Paracou, French Guiana: a neotropical lowland rainforest fauna part−1. bats. Bull. Am. Museum Nat. History 237, 1–219.
Speakman, J., and Racey, P. (1987). “The energetics of pregnancy and lactation in the brown long-eared bat, Plecotus auritus,” in Recent Advances in the Study of Bats, eds M. Fenton, P. Racey, and J. Rayner (Cambridge: Cambridge University Press), 367–393.
Storz, J. F., Bhat, H. R., and Kunz, T. H. (2000). Social structure of a polygynous tent-making bat, Cynopterus sphinx (Megachiroptera). J. Zool. 251, 151–165. doi: 10.1111/j.1469-7998.2000.tb00600.x
Twiss, S. D., Thomas, C., Poland, V., Graves, J. A., and Pomeroy, P. (2007). The impact of climatic variation on the opportunity for sexual selection. Biol. Lett. 3, 12–15. doi: 10.1098/rsbl.2006.0559
Vaughan, T. A. (1987). Behavioral thermoregulation in the African yellow-winged bat. J. Mammal. 68, 376–378. doi: 10.2307/1381476
Voigt, C. C., Behr, O., Caspers, B., Von Helversen, O., Knörnschild, M., Mayer, F., et al. (2008). Songs, scents, and senses: sexual selection in the greater sac-winged bat, Saccopteryx bilineata. J. Mammal. 89, 1401–1410. doi: 10.1644/08-MAMM-S-060.1
Welbergen, J. A., Klose, S. M., Markus, N., and Eby, P. (2008). Climate change and the effects of temperature extremes on Australian flying-foxes. Proc. Royal Soc. B Biol. Sci. 275, 419–425. doi: 10.1098/rspb.2007.1385
West, P. M., and Packer, C. (2002). Sexual selection, temperature, and the lion's mane. Science 297, 1339–1343. doi: 10.1126/science.1073257
Keywords: behavioral thermoregulation, day roost, mating system, resource-defense polygyny, Rhynchonycteris naso, temperature
Citation: Wilde LR, Günther L, Mayer F, Knörnschild M and Nagy M (2018) Thermoregulatory Requirements Shape Mating Opportunities of Male Proboscis Bats. Front. Ecol. Evol. 6:199. doi: 10.3389/fevo.2018.00199
Received: 24 April 2018; Accepted: 09 November 2018;
Published: 27 November 2018.
Edited by:
Geoffrey M. While, University of Tasmania, AustraliaReviewed by:
M. Teague O'Mara, Max-Planck-Institut für Ornithologie, GermanyCory Alexander Toth, Boise State University, United States
Copyright © 2018 Wilde, Günther, Mayer, Knörnschild and Nagy. This is an open-access article distributed under the terms of the Creative Commons Attribution License (CC BY). The use, distribution or reproduction in other forums is permitted, provided the original author(s) and the copyright owner(s) are credited and that the original publication in this journal is cited, in accordance with accepted academic practice. No use, distribution or reproduction is permitted which does not comply with these terms.
*Correspondence: Martina Nagy, bWFydGluYW5hZ3lAZ214Lm5ldA==