- 1Division of Biology, Kansas State University, Manhattan, KS, United States
- 2Forest Ecosystems and Society, Oregon State University, Corvallis, OR, United States
The climatic conditions in the North American Great Plains are highly variable, characteristic of an inter-continental climate. Antecedent climate history has impacted the flora of Great Plains grasslands, resulting in high species richness as well as dominance by only a few grass species, such as Andropogon gerardii. While the productivity of A. gerardii is well described, the individual physiological, and morphological characteristics that confer species dominance over wide spatial gradients are not clearly understood. We performed a literature search to assess intra-specific trait variability of A. gerardii from as many locations as possible. Ultimately, only 13 locations in the Great Plains have reported common plant functional traits (PFTs) for this species. To best represent site-specific climate conditions, plant functional trait data (8 PFTs) were collected from literature reporting ambient growing conditions, and excluded experimental manipulations. For most PFTs, we found insufficient data to fully quantify the range of variation across the geographical extent of A. gerardii dominance. This is surprising given that we focused on the most abundant grass in one of the most well-studied regions globally. Furthermore, trait data collected from our literature search showed a high degree of variability, but no strong relationships were observed between mean trait values and climate predictors. Our review of the literature on A. gerardii suggests a role for trait variability as a mechanism enabling the dominance of this species across large regions such as the Great Plains of North America.
Introduction
Biomes are often spatially delineated with sharp boundaries and attributed functional properties based on the primary vegetation represented (Bailey, 1998, 2005). These vegetation types are comprised of species that likely exhibit variation in functional traits that may respond to climatic gradients or change through time. Interspecific variation has been used as a proxy for understanding climate change, because it represents how plant functional types and ecosystem function may be altered (Ryan, 1991; Adler et al., 2006; Taylor et al., 2014). However, the focus on mean-trait values in the literature suggests that the true magnitude of intraspecific trait variation is commonly overlooked, and is rarely incorporated into climate and vegetation process models (Lambert et al., 2011; Johnson et al., 2015; Funk et al., 2017), although intraspecific trait variability appears promising for predicting species change (Lu et al., 2017). This is partly due to a lack of empirical work focusing on patterns of ecotypic and intraspecific trait variation (Violle et al., 2012). Intraspecific trait variation is the occurrence of a genotype expressing various phenotypes in a given environment; it's a combination of genetic (i.e., evolution) and environmental factors reflected in the variation within populations (forming ecotypes) (Valladares et al., 2007, 2014; Turcotte and Levine, 2016; Barbour et al., 2018). Measuring intraspecific trait variation allows for an in-depth understanding of a species' ability to respond and adapt to environmental changes (Molina-Montenegro et al., 2018). In this review, we focus on the average trait expression (reflected in functional traits) change in a single species spanning a large continental gradient. Our goal is to assess the degree to which intraspecific trait variation in dominant species might contribute to the functional responses of grassland ecosystems.
The Central and Great Plains region of the United States experiences a continental climate of temperature extremes and both intra- and inter-annual variability in precipitation (Borchert, 1950; Weaver, 1968). A noteworthy characteristic of the grasslands in this region is the high floristic richness (Collins and Calabrese, 2012), yet dominance by a few C4 grass species that encompass the majority of annual production (Dietrich and Smith, 2016). A combination of site-level and regional landscape heterogeneity contributes to genotypic and phenotypic diversity within dominant species (Olsen et al., 2013). This allows for a mosaic of different genotypes of dominant grass species to exist across the Great Plains region.
Previous research has shown the occurrence of broad genotypic and anatomical differences within species across regional gradients in the United States (Avolio and Smith, 2013; Olsen et al., 2013; McAllister et al., 2015). These differences may arise from climatic events causing changes in populations of dominant species (Hoover et al., 2014a, 2015; Hoffman et al., 2018). Functional trait variability may also play a large role in muting the negative impacts of large stress events, such as drought, across a landscape. Therefore, an innate advantage of increased resistance to stress events exists, and high functional trait variability may decrease the likelihood of species loss on a regional scale (Smith and Knapp, 1999).
The aim of this review is to measure within-region trait variation from a dominant species found in the Great Plains (temperate grassland; Figure 1). Focusing on several functional traits commonly used in the literature (specific leaf area, water potential, photosynthesis, stomatal conductance, above-ground productivity (individual and g/m2), and below-ground biomass (individual and g/m2), we have summarized the variability of functional traits within the species Andropogon gerardii Vitman. A. gerardii is a perennial C4 grass that has significant genotypic and functional trait variability, facilitating a broad distribution throughout the Central and Great Plains. PFTs collected were analyzed to understand if the variability in a given trait varies/relates to gradients in multiple climate factors across this region, including mean annual precipitation (MAP), mean annual temperature (MAT), mean annual minimum temperature (MMinT), and mean annual maximum temperature (MMaxT) from 1980–2015. For example, we would expect biomass to vary across a climate gradient because productivity positively increases in this region with corresponding increases in precipitation and temperature (Nippert et al., 2006; Hufkens et al., 2016). Identifying trait variability within a single species may provide insight for the potential role of adaptive trait variability as a driver of population persistence across broad climatic space.
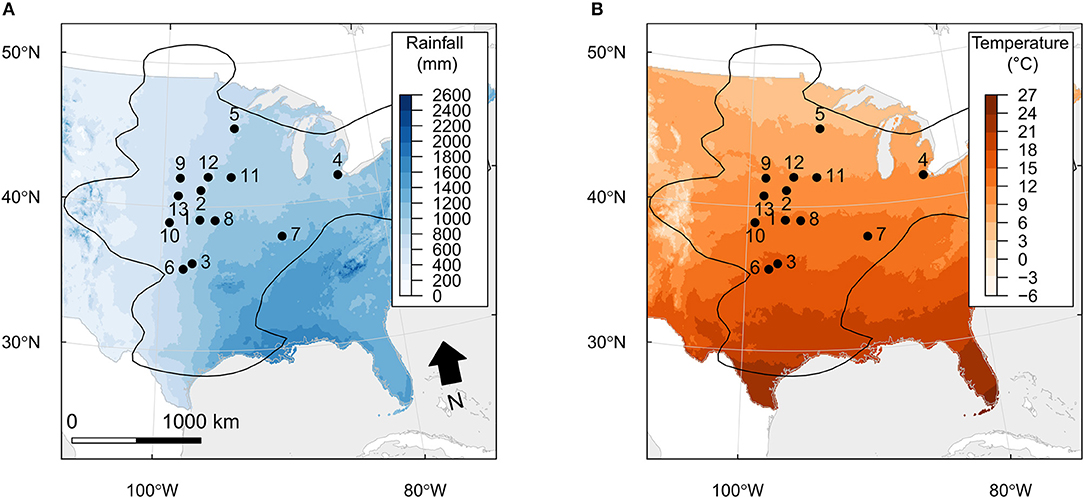
Figure 1. Site locations of gathered A. gerardii functional traits used in this study. The black contour line indicates the 90% percentile of the density of GBIF occurrences. Source ID codes, locations, precipitation (A), and temperature (B) data are located in the Supplementary Material.
Literature Search Criteria For Andropogon Gerardii PFTs
We conducted a literature search to collect specific PFT data from a widely distributed grassland species: Andropogon gerardii, attempting to lend insight into one of many potential reasons for why some species achieve dominance over broad climatic space. A. gerardii was chosen because it is a dominant species in the tallgrass prairie and encompasses roughly 70% of the total aboveground biomass in grasslands throughout the Great and Central Plains region (Rogler, 1944; Weaver, 1968; Smith et al., 2017). We selected 6 important functional traits that reflect major axes of leaf economic variation and properties relevant to ecosystem function. The PFTs included in the literature search were specific leaf area (SLA), water potential (WPall; pre-dawn and midday), photosynthesis (A), stomatal conductance (gs), above-ground biomass (AGB), and below-ground biomass (BGB). ABG and BGB data included biomass on an individual and a per square meter basis. Starting with SLA, we searched Google Scholar and Web of Science (which yielded identical results) with the string [“Andropogon gerardii” AND (“LMA” OR “SLA”) AND “Great Plains”], which produced 60 search results. Only 12 of these studies (from 6 study sites) reported SLA. Similar searches were performed for the less common functional traits. SLA data was also collected from the TRY global database of plant traits (Kattge et al., 2011), which mirrored previous search parameters with greater success. This process resulted in a PFT dataset collected from 36 separate studies, including data from 17 research locations in 7 states from 1984–2017 (Figure 1). Our goal was to include data from across the U.S. Midwestern region and to exclude redundant data that were from the same projects, and only include “control” or “ambient” conditions. Data collection from literature varied by state. Kansas and Illinois contributed 59 data points (each point representing a single datum), which is a substantial amount of the total dataset. In order to determine differences in geographic location, statistical analysis was conducted via ANOVA before and after the data were normalized by natural log. MAP, MAT, MMinT, MMaxT (mean from 1980–2015), and geographic location of each data point was collected from PRISM (PRISM Climate Group, 2014) and used as fixed variables while each PFT was used as response variables. AICc model selection was also used to determine the most impactful climate parameters in the model using the “MuMIn” package (Barton, 2018), according to Grueber et al. (2011). Analyses were conducted in the statistical program R V3.4.3 (R Core Team, 2017). The geographic map (Figure 1) was produced using the “raster” package in program R (Hijmans, 2017).
Plant Functional Traits Reflect Ecophysiological Processes
Plant functional traits (PFTs) are commonly used to identify species' differences in growth, allocation, and competition in relation to environmental effects to reflect plant economics (Grime, 1979; Edwards et al., 2007; Guo et al., 2017; Volaire, 2018). PFTs represent morphological and physiological adaptations that often predict plant responses to biotic (competition, herbivory, etc.), and abiotic factors (MAP, MAT, etc.). PFT's typically include whole-plant traits, tissue specific traits (leaf, stem, and root), and physiological measurements (photosynthesis, stomatal conductance, transpiration, and water potential) (Pérez-Harguindeguy et al., 2013; Carmona et al., 2016). For instance, PFTs have been used to predict how individual A. gerardii and populations of other grassland species will respond to projected drought conditions (Chapin et al., 2000; Nippert et al., 2009; Volder et al., 2010; Liancourt et al., 2015; Maréchaux et al., 2015; Skelton et al., 2015; De La Riva et al., 2016).
The biological link between ecophysiology and environmental factors aids in predicting how species will respond to climatic changes (Nippert et al., 2011; Ocheltree et al., 2012; Hoover et al., 2014b; Griffith et al., 2016); therefore, such traits reflect the ability of a species, like A. gerardii, to respond to changing climatic conditions that are found in the Great Plains (Grime, 2001; McGill et al., 2006; Butterfield and Callaway, 2013; Losapio and Schob, 2017). Andropogon gerardii in the Great Plains exhibits many drought tolerant traits that allow either resistance and/or resilience in response to the abiotic stressors such as increased temperature and precipitation variability (Hoover et al., 2014b; Hoover and Rogers, 2016). Traits that are commonly correlated with drought tolerance include increased water-use efficiency (WUE), decreased leaf area (LA), higher specific root length (SRL), and lower turgor loss point (Eissenstat et al., 2000; Ripley et al., 2007; Hameed et al., 2012; Bartlett et al., 2014). Drought tolerant traits are likely not static in species with populations that span regional gradients, as there is adaptive benefit for greater trait variability in an environment that experiences high climate variability (Chapin, 1980; Avolio and Smith, 2013; Funk et al., 2017). For instance, WUE variability enables populations to maintain relatively high fitness with varying levels of water availability and mean temperatures (Briggs and Knapp, 2001; Nippert et al., 2007). Nippert et al. (2007) showed physiological trait variability for C4 grasses provided an advantage for fast growth under favorable conditions and the ability to withstand (resist) drought during poor (stressful) conditions (Briggs and Knapp, 2001; Nippert et al., 2007). Trait variability may serve as a climate buffering mechanism (Valladares et al., 2007), which may be observable on the individual physiological scale (i.e., WUE), but also in other PFTs at the regional scale.
Trait Variation In Andropogon Gerardii
Plant functional traits (PFTs) are known to differentiate between species, due to their evolutionary history (Violle et al., 2012; Cornwell et al., 2014; Valverde-Barrantes et al., 2017). However, less work has focused on intraspecific trait variability across large geographic scales. To emphasize this point, the dominant Great Plains grass species (Andropogon gerardii) is arguably the most well-studied dominant grass species in the Great Plains and specific leaf area (SLA) is the most widely reported functional trait, yet we only found 12 studies from 6 research locations where PFT data were reported from natural populations without experimental manipulation. In fact, three traits accounted for nearly half the data points in the study (Figure 2A), supporting the clear need for greater reporting of PFT data within common species that span large climate gradients (Figure 2B). Because single PFT data are under-represented in the literature, we aimed to incorporate multiple PFTs for A. gerardii to better understand intraspecific trait variability. More specifically, this amalgamation of PFTs may provide insight into the role of trait variability as a driver of plant species functioning over large regional scales. We assume that for a single species to extend over large geographic regions such as the Great Plains, the species would inevitably maintain a highly plastic phenotype at the population level which may buffer the whole species from variable climate conditions (Figure 2B). Figure 2C theorizes that populations from species with increased trait variability permits survival across a greater range of environmental conditions. For instance, if A. gerardii populations were subjected to drought conditions, varying levels of drought tolerance would be observed due to varying leaf water potential at turgor loss point (Maréchaux et al., 2015). Phenotypic variability has been observed to assuage effects from harsh abiotic pressures, however this may only be realized in the short-term (Becklin et al., 2016).
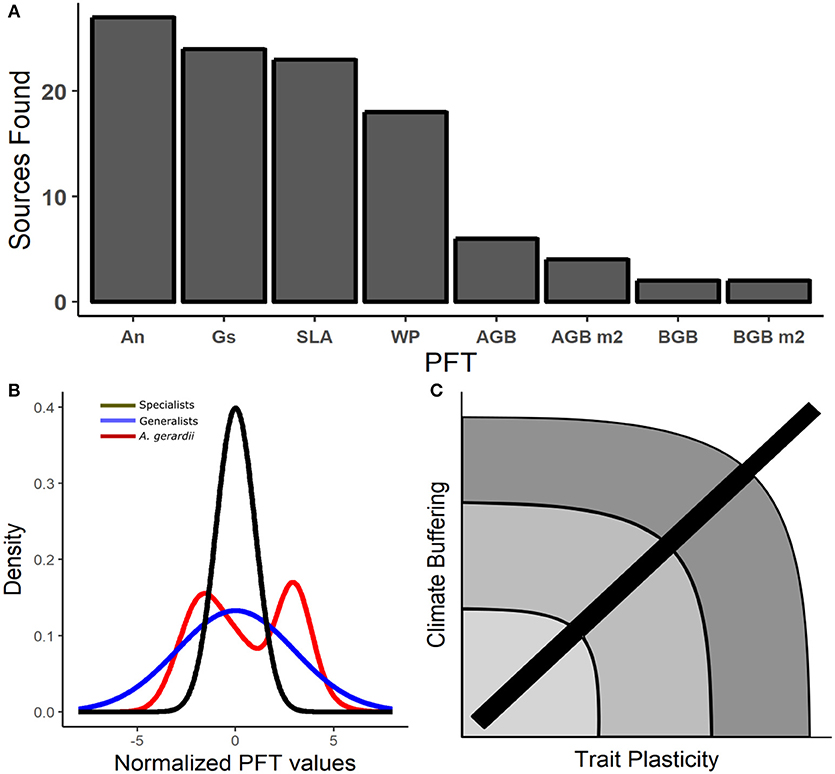
Figure 2. (A) Displays the number of sources found in each PFT, the data were then used to create the density plot in (B). (B) Portrays predictions of normalized PFT data variability within a specialist species exhibiting low trait variability (Black; standard deviation = 1), generalists/high trait variability (Blue, standard deviation = 3), and the normalized data reported from the literature search is represented in red. (C) Illustrates how increasing PFT variability may result in increased buffering (resilience) from environmental variability. Arcs and color describe the theoretical placement of a species on a continuum such that a low trait variability results in low climate buffering (lighter color), and high trait variability facilitates higher climate buffering (darker color). Figures produced using the “ggplot2” package in program R V3.4.3 (R Core Team, 2017).
Long-term persistence of abiotic pressures will ultimately cause population reductions, due to climatic conditions moving out of the of historical climate parameters; this was observed in the droughts of the1930's (Romm, 2011; Becklin et al., 2016). Evidence from rain manipulation experiments provides insight into potential responses to climate extremes (Fay et al., 2002; Knapp et al., 2002; Nippert et al., 2009). For instance, Hoover et al. (2015), indicated that C4 grasses subjected to drought conditions in the Colorado Plateau (35% reduction of annual rainfall) were observed to maintain cover for the first year, but decreased cover and increased mortality with prolonged exposure. Climate buffering can also be observed in a similar experimental design (rainfall manipulation) within the tallgrass prairie. A. gerardii did not display the same negative responses as other similar C4 grasses to increasing climate variability, instead a relatively static response was observed (Fay et al., 2003; Avolio and Smith, 2013).
Increased trait variability within a species may provide physiological benefits in regions with high climate variability. A. gerardii lacks highly specific growing conditions and exists across broad geographic gradients in the U.S, including regions with hot and dry climate conditions. We hypothesize that A. gerardii and other generalists that dominate large geographic regions can be represented in Figure 2B as the blue line, whereas species that require more specific growing conditions are hypothetically represented as the black line. The theoretical curves in Figure 1B were created by using a low standard deviation (sd = 1; reflecting ~68% of data explained) and a higher standard deviation (sd = 3; reflecting an increased data distribution) assuming an underlying normal distribution. Specialized species (black line) would not be capable of expanding over large heterogeneous landscapes due to the inability to withstand large fluctuations in temperature or precipitation (Linder et al., 2018), which is represented by a higher/narrower trait density (Figure 2B). Trait variability is documented to lead toward a more stable system due to niche stabilization which affects community composition, the function of the ecosystem, and response to abiotic factors (Turcotte and Levine, 2016).
The PFT data from Andropogon gerardii varied widely between research sites, but without discernable trends due to climate parameters like increasing precipitation and temperature (locations and PFT types are found in the Supplemental Material). For example, A. gerardii from Konza Prairie (KS), exhibited photosynthetic rates that included both the maximum and minimum of observed rates from all states included in the literature search, with many data points falling along the mean. Statistical results (ANOVA) show little to no discernable trend in PFTs when considering climate conditions at the geographic locations, which supports the concept of intraspecific trait variation allowing a single species to occupy such a large geographic range containing large precipitation and temperature differences. Photosynthetic rates did not vary by location, neither did stomatal conductance, SLA, or water potential (P > 0.05). Only two PFTs were observed to statistically vary by research location: above and below-ground biomass (P < 0.05). This result may reflect the small sample size from the literature as only 15 total data points were found for both individual and square meter collection methods. AICc model selection was performed on models containing all combinations of the PRISM climate variables to find the best model (given all variables) using the “model.sel” function, within the MuMIn package (delta <2, Royall's 1/8 rule, and cumulative sum of model weights were used to identify uncertainty and differences in the models). The full model including location, MAP, MAT, MMinT, and MMaxT best explained the SLA results gathered from the literature search (AICc = 974.6; weight = 0.939); which was 23 times more likely to be the best explanation for variation compared to the next model (AICc = 980.9; weight = 0.040) that did not include location as a parameter. Production above/belowground can exhibit a positive relationship (Nippert et al., 2006) with soil moisture and precipitation or relatively no change (Zhou et al., 2009), providing potential for variation across precipitation gradients within the Central and Great Plains. No trends due to climate parameters were visible in the analysis based on geographic location (Figure 1), but we speculate that additional production data collected across this regional gradient may result in a positive relationship with regional precipitation gradients similar to results from precipitation manipulation experiments (Fay et al., 2000, 2003).
The results from our literature review followed the ecological hypotheses presented in Figure 2B. PFTs were normalized (natural log) and combined to view large scale trends in the data. A. gerardii follows very closely to the “generalists” species parabola, meaning that PFTs are variable or plastic across a broad range of environmental conditions (Figure 2C). A. gerardii also expresses a low density of several PFTs, which indicates that traits are not static, but variable. Plastic PFTs allow A. gerardii the ability to respond positively in a given population, which buffers the A. gerardii species as a whole. This interpretation may contradict previously held claims that A. gerardii will experience geographic shifts or experience large population reductions due to climate change (Gray et al., 2014; Smith et al., 2017). Thus, more research is required to understand the role that intraspecific variation plays in the expansion and survival of A. gerardii in the Great Plains.
Future Directions
The literature containing plant functional traits covers many different types of ecosystems and hundreds of species (Pérez-Harguindeguy et al., 2013), which have been emphasized in climate change literature encompassing major biomes across the world (Liancourt et al., 2015). Here, we used PFTs to identify inherent trait variability within a single species, and identify a potential role of adaptive variability as a driver of species persistence across a regional climate gradient. Results from this literature review suggest that the PFTs observed in A. gerardii do not statistically differ (excluding AGB and BGB) between locations measured (Figure 1). Findings from this review underline the importance of adaptive trait variability to permit greater phenotypic plasticity, which provides population buffering for some species that exist across broad climate gradients.
Moving forward, an increased focus from interspecific to intraspecific species trait variation may provide a greater understanding of how future climate variability will impact native plant species that span large regional scales. More specifically, A. gerardii is the quintessential prairie species; yet an extensive examination of the literature showed a relatively small number of sites reporting trait data. We advocate for the development of a grass trait network to examine the effect of climate change on specific dominant species in grasslands worldwide. This network could follow the framework created by Nutrient Network (NutNet; http://www.nutnet.org) and Drought Network (DroughtNet; http://www.drought-net.colostate.edu) to standardize measurements and procedures, and allow for more consistent interpretation of PFTs response to changes in abiotic factors. Increased documentation of spatial climate gradients and species distributions will increase our understanding of the role of trait variability in species resistance and resilience to future changes in the environment.
Author Contributions
SB and DG were both responsible for collecting data for the literature search. SB was responsible for writing the manuscript and collecting the bulk of the data. DG gave insight into the manuscript and utilized the data to create the precipitation map. JN served as SB's advisor, aided in the literature search, and helped shape the manuscript.
Conflict of Interest Statement
The authors declare that the research was conducted in the absence of any commercial or financial relationships that could be construed as a potential conflict of interest.
Acknowledgments
We would like to thank the Konza Prairie LTER program (NSF DEB-1440484) and the NSF Dimensions of Biodiversity program (NSF 002893) for funding this research.
Supplementary Material
The Supplementary Material for this article can be found online at: https://www.frontiersin.org/articles/10.3389/fevo.2018.00217/full#supplementary-material
References
Adler, P. B., HilleRisLambers, J., Kyriakidis, P. C., Guan, Q., and Levine, J. M. (2006). Climate variability has a stabilizing effect on the coexistence of prairie grasses. Proc. Natl. Acad. Sci. U.S.A. 103, 12793–12798. doi: 10.1073/pnas.0600599103
Avolio, M. L., and Smith, M. D. (2013). Intra-specific responses of a dominant C4 grass to altered precipitation patterns. Plant Ecol. 214, 1377–1389. doi: 10.1007/s11258-013-0258-y
Bailey, R. G. (1998). Ecoregions. 2nd ed. London: Springer Berlin Heidelberg. doi: 10.1007/978-1-4612-2200-2
Bailey, R. G. (2005). Identifying ecoregion boundaries. Environ. Manage. 34, s14–s26. doi: 10.1007/s00267-003-0163-6
Barbour, M. A., Erlandson, S., Peay, K., Locke, B., Jules, E. S., and Crutsinger, G. M. (2018). Trait plasticity is more important than genetic variation in determining species richness of associated communities. J. Ecol. 107, 350–360. doi: 10.1111/1365-2745.13014
Bartlett, M. K., Zhang, Y., Kreidler, N., Sun, S., Ardy, R., Cao, K., et al. (2014). Global analysis of plasticity in turgor loss point, a key drought tolerance trait. Ecol. Lett. 17, 1580–1590. doi: 10.1111/ele.12374
Barton Kl. (2018). MuMIn: Multi-Model Inference. R package version 1.40.44. Available online at: https://CRAN.R-project.org/package=MuMIn
Becklin, K. M., Anderson, J. T., Gerhart, L. M., Wadgymar, S. M., Wessinger, C. A., and Ward, J. K. (2016). Examining plant physiological responses to climate change through an evolutionary lens. Plant Physiol. 172, 635–649. doi: 10.1104/pp.16.00793
Borchert, J. R. (1950). The climate of the central north american grassland. Ann. Assoc. Am. Geogr. 40, 1–39. doi: 10.1080/00045605009352020
Briggs, J. M., and Knapp, A. K. (2001). Determinants of C3 Forb growth and production in a C4 dominated grassland. Plant Ecol. 152, 93–100. doi: 10.1023/A:1011400101014
Butterfield, B. J., and Callaway, R. M. (2013). A functional comparative approach to facilitation and its context dependence. Funct. Ecol. 27, 907–917. doi: 10.1111/1365-2435.12019
Carmona, C. P., Bello, F., De Mason, N. W. H., and Leps, J. (2016). Traits without borders : integrating functional diversity across scales. Trends Ecol. Evol. 31, 382–394. doi: 10.1016/j.tree.2016.02.003
Chapin, F. S. (1980). The mineral nutrition of wild plants. Annu. Rev. Ecol. Syst. 11, 233–260. doi: 10.1146/annurev.es.11.110180.001313
Chapin, F. S., Zavaleta, E. S., Eviner, V. T., Naylor, R. L., Vitousek, P. M., Reynolds, H. L., et al. (2000). Consequences of changing biodiversity. Nature 405, 234–242. doi: 10.1038/35012241
Collins, S. L., and Calabrese, L. B. (2012). Effects of fire, grazing and topographic variation on vegetation structure in tallgrass prairie. J. Veg. Sci. 23, 563–575. doi: 10.1111/j.1654-1103.2011.01369.x
Cornwell, W. K., Westoby, M., Falster, D. S., Fitzjohn, R. G., Meara, B. C. O., Pennell, M. W., et al. (2014). Functional distinctiveness of major plant lineages. J. Ecol. 102, 345–356. doi: 10.1111/1365-2745.12208
De La Riva, E. G., Olmo, M., Poorter, H., Ubera, J. L., and Villar, R. (2016). Leaf mass per area (LMA) and its relationship with leaf structure and anatomy in 34 mediterranean woody species along a water availability gradient. PLoS ONE 11:e0148788. doi: 10.1371/journal.pone.0148788
Dietrich, J. D., and Smith, M. (2016). The effect of timing of growing season drought on flowering of Andropogon gerardii. Oecologia 181, 391–399. doi: 10.1007/s00442-016-3579-4
Edwards, E. J., Still, C. J., and Donoghue, M. J. (2007). The relevance of phylogeny to studies of global change. Trends Ecol. Evol. 22, 243–249. doi: 10.1016/j.tree.2007.02.002
Eissenstat, D., Wells, C., and Yanai, R. (2000). Building roots in a changing environment: implications for root longevity. New Phytol. 147, 33–42. doi: 10.1046/j.1469-8137.2000.00686.x
Fay, P., Carlisle, J. D., Danner, B. T., Lett, M. S., McCarron, J. K., Stewart, C., et al. (2002). Altered rainfall patterns, gas exchange, and growth in grasses and forbs. Int. J. Plant Sci. 163, 549–557. doi: 10.1086/339718
Fay, P. A., Carlisle, J. D., Knapp, A. K., Blair, J. M., and Collins, S. L. (2000). Altering rainfall timing and quantity in a mesic grassland ecosystem: design and performance of rainfall manipulation shelters. Ecosystems 3, 308–319. doi: 10.1007/s100210000028
Fay, P. A., Carlisle, J. D., Knapp, A. K., Blair, J. M., and Collins, S. L. (2003). Productivity responses to altered rainfall patterns in a C4-dominated grassland. Oecologia 137, 245–251. doi: 10.1007/s00442-003-1331-3
Funk, J. L., Larson, J. E., Ames, G. M., Butterfield, B. J., Cavender-bares, J., Firn, J., et al. (2017). Revisiting the Holy Grail : using plant functional traits to understand ecological processes. Biol. Rev. Camb. Philos. Soc. 92, 1156–1173. doi: 10.1111/brv.12275
Gray, M. M., st. Amand, P., Bello, N. M., Galliart, M. B., Knapp, M., Garrett, K. A., et al. (2014). Ecotypes of an ecologically dominant prairie grass (Andropogon gerardii) exhibit genetic divergence across the U. S. Midwest grasslands' environmental gradient. Mol. Ecol. 23, 6011–6028. doi: 10.1111/mec.12993
Griffith, D. M., Quigley, K. M., and Anderson, T. M. (2016). Leaf thickness controls variation in leaf mass per area (LMA) among grazing - adapted grasses in Serengeti. Oecologia 181, 1035–1040. doi: 10.1007/s00442-016-3632-3
Grime, J. P. (2001). Plant Strategies, Vegetation Processes, and Ecosystem Properties. 2nd ed. New York, NY: Wiley.
Grueber, C. E., Nakagawa, S., Laws, R. J., and Jamieson, I. G. (2011). Multimodel inference in ecology and evolution: challenges and solutions. J. Evol. Biol. 24, 699–711. doi: 10.1111/j.1420-9101.2010.02210.x
Guo, C., Ma, L., Yuan, S., and Wang, R. (2017). Morphological, physiological and anatomical traits of plant functional types in temperate grasslands along a large-scale aridity gradient in northeastern China. Sci. Rep. 7:40900. doi: 10.1038/srep40900
Hameed, M., Batool, S., and Naz, N. (2012). Leaf structural modifications for drought tolerance in some differentially adapted ecotypes of blue panic (Panicum antidotale Retz.). Acta Physiol. Plant. 34, 1479–1491. doi: 10.1007/s11738-012-0946-6
Hijmans, R. J. (2017). raster: Geographic Data Analysis and Modeling. R package version 2.6-7. Available online at: https://CRAN.R-project.org/package=raster
Hoffman, A. M., Avolio, M. L., Knapp, A. K., and Smith, M. D. (2018). Codominant grasses differ in gene expression under experimental climate extremes in native tallgrass prairie. PeerJ 6:e4394. doi: 10.7717/peerj.4394
Hoover, D., Duniway, M., and Belnap, J. (2015). Pulse-drought atop press-drought: unexpected plant responses and implications for dryland ecosystems. Oecologia 179, 1211–1221. doi: 10.1007/s00442-015-3414-3
Hoover, D., Knapp, A., and Smith, M. (2014b). Resistance and resilience of a grassland ecosystem to climate extremes. Ecology 95, 2646–2656. doi: 10.1890/13-2186.1
Hoover, D., Knapp, A. K., and Smith, M. D. (2014a). Contrasting sensitivities of two dominant C 4 grasses to heat waves and drought. Plant Ecol. 215, 721–731. doi: 10.1007/s11258-014-0345-8
Hoover, D. L., and Rogers, B. M. (2016). Not all droughts are created equal: the impacts of interannual drought pattern and magnitude on grassland carbon cycling. Glob. Chang. Biol. 22, 1809–1820. doi: 10.1111/gcb.13161
Hufkens, K., Keenan, T. F., Flanagan, L. B., Scott, R. L., Bernacchi, C. J., Joo, E., et al. (2016). Productivity of North American grasslands is increased under future climate scenarios despite rising aridity. Nat. Clim. Chang. 6, 710–714. doi: 10.1038/nclimate2942
Johnson, L. C., Olsen, J. T., Tetreault, H., Delacruz, A., Bryant, J., Morgan, T. J., et al. (2015). Intraspecific variation of a dominant grass and local adaptation in reciprocal garden communities along a US Great Plains' precipitation gradient: implications for grassland restoration with climate change. Evol. Appl. 8, 705–723. doi: 10.1111/eva.12281
Kattge, J., Díaz, S., Lavorel, S., Prentice, I. C., Leadley, P., Bönisch, G., et al. (2011). TRY - a global database of plant traits. Glob. Chang. Biol. 17, 2905–2935. doi: 10.1111/j.1365-2486.2011.02451.x
Knapp, A., Fay, P., Blair, J., Collins, S., Smith, M., Carlisle, J., et al. (2002). Rainfall variability, carbon cycling, and plant species diversity in a mesic grassland. Science 298, 2202–2205. doi: 10.1126/science.1076347
Lambert, A. M., Baer, S. G., Gibson, D. J., and For, S. (2011). Intraspecific variation in ecophysiology of three dominant prairie grasses used in restoration : cultivar versus non-cultivar population sources. Restor. Ecol. 19, 43–52. doi: 10.1111/j.1526-100X.2010.00673.x
Liancourt, P., Boldgiv, B., Song, D. S., and Laura, A. (2015). Leaf-trait plasticity and species vulnerability to climate change in a Mongolian steppe. 3489–3498. doi: 10.1111/gcb.12934
Linder, H. P., Lehmann, C. E. R., Archibald, S., Osborne, C. P., and Richardson, D. M. (2018). Global grass (Poaceae) success underpinned by traits facilitating colonization, persistence and habitat transformation. Biol. Rev. Camb. Philos. Soc. 93, 1125–1144. doi: 10.1111/brv.12388
Losapio, G., and Schob, C. (2017). Resistance of plant – plant networks to biodiversity loss and secondary extinctions following simulated environmental changes. Fucntional Ecol. 31, 1145–1152. doi: 10.1111/1365-2435.12839
Lu, X., Wang, Y. P., Wright, I. J., Reich, P. B., Shi, Z., and Dai, Y. (2017). Incorporation of plant traits in a land surface model helps explain the global biogeographical distribution of major forest functional types. Glob. Ecol. Biogeogr. 26, 304–317. doi: 10.1111/geb.12535
Maréchaux, I., Bartlett, M. K., Sack, L., Baraloto, C., Engel, J., Joetzjer, E., et al. (2015). Drought tolerance as predicted by leaf water potential at turgor loss point varies strongly across species within an Amazonian forest. Funct. Ecol. 29, 1268–1277. doi: 10.1111/1365-2435.12452
McAllister, C., Blaine, R., Kron, P., Bennett, B., Garrett, H., Kidson, J., et al. (2015). Environmental correlates of cytotype distribution in Andropogon Gerardii (Poaceae). Am. J. Bot. 102, 92–102. doi: 10.3732/ajb.1400296
McGill, B. J., Enquist, B. J., Weiher, E., and Westoby, M. (2006). Rebuilding community ecology from functional traits. Trends Ecol. Evol. 21, 178–185. doi: 10.1016/j.tree.2006.02.002
Molina-Montenegro, M. A., Acuña-Rodríguez, I. S., Flores, T. S. M., Hereme, R., Lafon, A., Atala, C., et al. (2018). Is the success of plant invasions the result of rapid adaptive evolution in seed traits? Evidence from a latitudinal rainfall gradient. Front. Plant Sci. 9:208. doi: 10.3389/fpls.2018.00208
Nippert, J. B., Fay, P. A., Carlisle, J. D., Knapp, A. K., and Smith, M. D. (2009). Ecophysiological responses of two dominant grasses to altered temperature and precipitation regimes. Acta Oecol. 35, 400–408. doi: 10.1016/j.actao.2009.01.010
Nippert, J. B., Fay, P. A., and Knapp, A. K. (2007). Photosynthetic traits in C3 and C4 grassland species in mesocosm and field environments. Environ. Exp. Bot. 60, 412–420. doi: 10.1016/j.envexpbot.2006.12.012
Nippert, J. B., Knapp, A. K., and Briggs, J. M. (2006). Intra-annual rainfall variability and grassland productivity: can the past predict the future? Plant Ecol. 184, 65–74. doi: 10.1007/s11258-005-9052-9
Nippert, J. B., Ocheltree, T. W., Skibbe, A. M., Kangas, L. C., Ham, J. M., Arnold, K. B. S., et al. (2011). Linking plant growth responses across topographic gradients in tallgrass prairie. Oecologia 166, 1131–1142. doi: 10.1007/s00442-011-1948-6
Ocheltree, T. W., Nippert, J. B., and Prasad, P. V. V. (2012). Changes in stomatal conductance along grass blades reflect changes in leaf structure. Plant. Cell Environ. 35, 1040–1049. doi: 10.1111/j.1365-3040.2011.02470.x
Olsen, J. T., Caudle, K. L., Johnson, L. C., Baer, S. G., and Maricle, B. R. (2013). Environmental and genetic variation in leaf anatomy among populations of Andropogon gerardii (Poaceae) along a precipitation gradient. Am. J. Bot. 100, 1957–1968. doi: 10.3732/ajb.1200628
Pérez-Harguindeguy, N., Diaz, S., Garnier, E., Lavorel, S., Poorter, H., Jaureguiberry, P., et al. (2013). New Handbook for standardized measurment of plant functional traits worldwide. Aust. J. Bot. 61, 167–234. doi: 10.1071/B.T.12225
PRISM Climate Group (2014). PRISM Climate Group-Oregon State University. Available online at: http://www.prism.oregonstate.edu/ (Accessed June 20, 2018).
R Core Team (2017). R: A Language and Environment for Statistical Computing. Vienna: R Foundation for Statistical Computing. Available online at: https://www.R-project.org/
Ripley, B. S., Gilbert, M. E., Ibrahim, D. G., and Osborne, C. P. (2007). Drought constraints on C4 photosynthesis: stomatal and metabolic limitations in C3 and C4 subspecies of Alloteropsis semialata. J. Exp. Bot. 58, 1351–1363. doi: 10.1093/jxb/erl302
Rogler, G. A. (1944). Relative payabilities of grasses under cultivation on the northern Great Plains. J. Am. Soc. Agron. 35, 547–559. doi: 10.2134/agronj1943.00021962003500070001x
Ryan, M. G. (1991). Effects of climate change on plant respiration. Ecol. Appl. 1, 157–167. doi: 10.2307/1941808
Skelton, R. P., West, A. G., and Dawson, T. E. (2015). Predicting plant vulnerability to drought in biodiverse regions using functional traits. Proc. Natl. Acad. Sci. U.S.A. 112, 5744–5749. doi: 10.1073/pnas.1503376112
Smith, A. B., Alsdurf, J., Knapp, M., Baer, S. G., and Johnson, L. C. (2017). Phenotypic distribution models corroborate species distribution models: a shift in the role and prevalence of a dominant prairie grass in response to climate change. Glob. Chang. Biol. 23, 4365–4375. doi: 10.1111/gcb.13666
Smith, M. D., and Knapp, A. K. (1999). Exotic plant species in a C4 dominated grassland : invasibility, disturbance, and community structure. Oecologia 120, 605–612. doi: 10.1007/s004420050896
Taylor, S. H., Ripley, B. S., Martin, T., De-Wet, L. A., Woodward, F. I., and Osborne, C. P. (2014). Physiological advantages of C4 grasses in the field: a comparative experiment demonstrating the importance of drought. Glob. Chang. Biol. 20, 1992–2003. doi: 10.1111/gcb.12498
Turcotte, M. M., and Levine, J. M. (2016). Phenotypic plasticity and species coexistence. Trends Ecol. Evol. 31, 803–813. doi: 10.1016/j.tree.2016.07.013
Valladares, F., Gianoli, E., and Gómez, J. M. (2007). Ecological limits to plant phenotypic plasticity. New Phytol. 176, 749–763. doi: 10.1111/j.1469-8137.2007.02275.x
Valladares, F., Matesanz, S., Guilhaumon, F., Araújo, M. B., Balaguer, L., Benito-Garzón, M., et al. (2014). The effects of phenotypic plasticity and local adaptation on forecasts of species range shifts under climate change. Ecol. Lett. 17, 1351–1364. doi: 10.1111/ele.12348
Valverde-Barrantes, O. J., Freschet, G. T., Roumet, C., and Blackwood, C. B. (2017). A worldview of root traits: The influence of ancestry, growth form, climate and mycorrhizal association on the functional trait variation of fine-root tissues in seed plants. New Phytol. doi: 10.1111/nph.14571
Violle, C., Enquist, B. J., McGill, B. J., Jiang, L., Albert, C. H., Hulshof, C., et al. (2012). The return of the variance: intraspecific variability in community ecology. Trends Ecol. Evol. 27, 244–252. doi: 10.1016/j.tree.2011.11.014
Volaire, F. (2018). A unified framework of plant adaptive strategies to drought: crossing scales and disciplines. Glob. Chang. Biol. 24, 2929–2938. doi: 10.1111/gcb.14062
Volder, A., Tjoelker, M. G., and Briske, D. D. (2010). Contrasting physiological responsiveness of establishing trees and a C4 grass to rainfall events, intensified summer drought, and warming in oak savanna. Glob. Chang. Biol. 16, 3349–3362. doi: 10.1111/j.1365-2486.2009.02152.x
Keywords: plant functional traits, grasslands, variability, ecophysiology, intraspecific trait variability
Citation: Bachle S, Griffith DM and Nippert JB (2018) Intraspecific Trait Variability in Andropogon gerardii, a Dominant Grass Species in the US Great Plains. Front. Ecol. Evol. 6:217. doi: 10.3389/fevo.2018.00217
Received: 30 April 2018; Accepted: 03 December 2018;
Published: 14 December 2018.
Edited by:
Oana Moldovan, Emil Racovita Institute of Speleology, RomaniaReviewed by:
Cristian Atala, Pontificia Universidad Católica de Valparaíso, ChileChristine McAllister, Principia College, United States
Copyright © 2018 Bachle, Griffith and Nippert. This is an open-access article distributed under the terms of the Creative Commons Attribution License (CC BY). The use, distribution or reproduction in other forums is permitted, provided the original author(s) and the copyright owner(s) are credited and that the original publication in this journal is cited, in accordance with accepted academic practice. No use, distribution or reproduction is permitted which does not comply with these terms.
*Correspondence: Seton Bachle, c2JhY2hsZUBrc3UuZWR1