- 1Institute of Psychology, Polish Academy of Sciences, Warsaw, Poland
- 2Department of Biological Sciences, Fordham University, Bronx, NY, United States
Live-trapping of urban rodents and other small mammals poses several challenges for researchers and pest control professionals (PMPs). Most traps are novel to the natural environment and elicit neophobic, or trap-shy, behaviors. Thus, animals captured in traditional traps may either be the least risk-averse, or most desperate. Consequently, individuals of the lowest social ranks, those in poorest health, and the slowest learners are more likely to be captured. This is problematic for research because non-random samples may lead to over-generalization and false conclusions. To address these problems, we developed an inconspicuous, hanging live-trap prototype. In addition to being hard to detect, the trap enables setting several parameters of animal movement and detection before the trap is triggered. The neophobic reaction could then be significantly reduced because animals do not need to enter any trap-like objects—instead they move and feed on a familiar surface. At a time predefined by the user, the triggering mechanism drops a transparent cover over the animal, preventing it from fleeing and enabling either transport to the laboratory, mark-and-release or disposal. Here, we report our initial purpose, design and preliminary results. Animals triggered the trap 34 times during our 1-month preliminary assessment. During this time, 32 individuals were captured (25 Norway rats and 7 house mice) for a 94% catch rate. Video surveillance revealed no obvious signs of non-random sampling as all trapped rats were representative of a broad range of sizes and ages. There were no signs of low social status (e.g., scar markings, parasitism, or poor health). Importantly, we found a low latency following capture, as released animals showed no instances of increased exploratory or cautious behaviors such as rearing or sniffing, near the hidden traps. More monitoring of this design is needed before future conclusions can be drawn. However, these results should encourage a full range of experimental trials from neuroscientists, urban ecologists, pest control professionals (PMPs) and conservationists who seek randomized samples or who work with trap-shy species.
Introduction
Safe, random trapping of live animals is important for research (Calhoun, 1962; Lockard, 1968; Stryjek and Pisula, 2008; Stryjek et al., 2012) and the pest control industry (Macdonald et al., 1999; Mason and Littin, 2003; Littin et al., 2014) alike. However, the use of traditional trap designs has compromised our ability to collect truly random samples of a given species (Mitchell, 1976; Stryjek and Pisula, 2008; Stryjek et al., 2012). For ecologists, trap-and-release studies are essential for accurately estimating the size of a population (Chao, 1989). Conservation biologists trap prior to translocating flocks or herds (Griffith et al., 1989), this is particularly important to do in the case of reintroductions (Abbott, 2000; de Milliano et al., 2016) or when training threatened animals to be “wary” of a novel predator (Blumstein et al., 2002). Psychologists and neuroscientists who use rat models in the laboratory or in the field, may trap wild rats, or even create new strains of outbred laboratory rats to increase variability in their study populations (Stryjek and Pisula, 2008). Epidemiologists and public health officials may trap rodents as part of surveillance programs to determine if, or when, potential pathogens are entering the population (Firth et al., 2014; Frye et al., 2015; Parsons et al., 2015, 2016).
Random live-trapping serves two essential functions. The first rule of warfare is to “know thy enemy” (Macdonald et al., 1999; Singleton et al., 2007). Thus, wildlife researchers and rodentologists must use highly-variable, random samples of a population to fully understand the efficacy of attractants, repellents and learning/appeasement tools on a pest species (Parsons et al., 2017, 2018). Finally, pest management professionals (PMPs) may routinely trap animals as part of their control efforts (Himsworth et al., 2013). Unfortunately trapping rarely removes the healthy, reproductive members of a population. Instead it is biased by sex, age or social status (Firth et al., 2014; Parsons et al., 2015). Pest trapping is particularly likely in food manufacturing facilities where it is often illegal to introduce poisons, or kill-traps (snap traps or glue traps). The latter is an issue because animal carcasses may accumulate along with potential pathogens as well as carry poisons from other areas, whereas glue traps have also fallen out of favor for ethical reasons. In addition, live-traps are often chosen for aesthetic and ethical reasons (many people refuse to kill animals, even if done humanely; Mason and Littin, 2003; Powell and Proulx, 2003). Finally, live-traps are used when protected species become pests locally [e.g., the Beech marten; Martes foina; Rondinini and Boitani, 2002].
However, despite the broadscale need in research and pest control to trap random samples, live-trapping continues to be limited and biased toward age, social status or sex (Firth et al., 2014; Parsons et al., 2015). This is especially surprising given the lack of emphasis made on other field based technologies such as improved camera trapping techniques (Norouzzadeh et al., 2018). To date, several live-trapping methods, of varying levels of efficacy, have been designed and implemented (Corrigan and Moreland, 2001). In the case of rodents, the most popular methods include various kinds of box-style wire mesh or traps made of full metal sheets, such as Sherman, Tomahawk and Havahart traps (Hice and Velazco, 2013). Triggering mechanisms are almost always placed inside and must be stepped on or pulled with some force in order to close the trap. Such traps have either one or two entrances. Anthony et al. (2005) compared large and small Longworth and Sherman traps for efficacy in trapping long tailed shrews (Sorex dispar) and western harvest mice (Reithrodontomys megalotis). While this study focused on the number of animals of each species caught over 3 years, it did not consider whether either trap was more likely to capture a representative sample of the variation in each population. Sampling bias for each trap-type was examined for pit traps, funnel traps and Elliot traps in Western Australia (Thompson and Thompson, 2007). However, these trap designs varied by species-size (herpetofauna and small mammals), and there was no examination of within-species variability. In a rare study in 2002, Jacob et al. (2002) contrasted the performance of two trap-types (Ugglan and Longworth) in field studies of house mice (Mus musculus) in southeastern Australia. Both traps performed similarly, however, Ugglan traps were more likely to attract males. These trials did not relate inter-species variability other than sex bias. In another Australian study Stokes (2013) compared enclosed Elliot traps to open wire cage traps in trapping black rats (Rattus rattus) and the native rat (Rattus fuscipes). Due to the biases of each type toward species, life stage or sex, the author recommended multiple trap types be used together.
Indeed, trap size rather than trap type (Ribeiro-Júnior et al., 2011) or intra-species variability has been the most examined aspect of trapping over the last 30 years. Many of the remaining trap studies have focused on humane traps to minimize wildlife suffering from capture (Olsen et al., 1988).
Multiple catch traps (e.g., Monarch Design) are less commonly used; in this case, animals enter the trap through a funnel entrance which leads to a weighted trapdoor (Vanderduys, 2016). Other live-trapping methods of small mammals are pitfalls, artificial burrows and nest boxes (Sikes et al., 2011). Yet these approaches are decades old and continue to generate biased catches (Zentall and Levine, 1972).
The general problem with most traps is that, as novel objects, they elicit neophobic reactions (Barnett, 1958; Calhoun, 1962; Mitchell, 1976) which may sometimes persist for weeks, with some instances of animals never approaching the object (RS observation). In addition, after several individuals from a given population are trapped, other individuals start avoiding the traps via social facilitation (Zentall and Levine, 1972), which makes those traditional trapping methods suitable for trapping only a sub-sample of less risk averse or slowest learning animals in a given population (Thompson, 1953). Due to the rats' inherent cautious behavior, trap-shyness and ability to develop escape techniques, live-traps are sometimes thought to be impractical and ineffective (Corrigan and Moreland, 2001). Finally, the commonly used triggering mechanisms in traditional designs are susceptible to accumulation of dirt and mechanical defects, which makes the use of classic live-traps even more challenging (RS, MHP personal observations).
Consequently, traditional trapping methods are not only ineffective, they usually do not provide a representative sample of animals(Lockard, 1968; Boice, 1971a). It is well known that individuals of the lowest social ranks, the slowest learners and those in poor health are far more likely to be captured (Lockard, 1968; Boice, 1971a). The latter is of crucial importance for the scientific research because non-random samples could result in false conclusions and over-generalizations (Burnham and Overton, 1978). When only a small number of individuals are caught, such as the most resilient or least shy, it further increases the risk that these individuals are genotypically unique. When captured for laboratory settings, this may cause a phenomenon called the founder effect, which leads to genetic drift toward features rarely present in the original population (Lande, 1976). From a pest management perspective, PMPs who routinely trap rats may be removing the lowest ranking members (e.g., beta through omega) while having no negative impact on the alphas (Lockard, 1968; Boice, 1971a). Due to the time-cost inefficiency and the above-outlined difficulties, and due to a constant need to capture live wild rats for the purposes of research and breeding in the WWCPS colony (Stryjek and Pisula, 2008), we developed a relatively simple and potentially highly effective rodent trapping technique. It is hoped that by following modifications to the size and weight, this design could possibly be used for capturing other species (e.g., feral cats; Parsons et al., 2018) or even such trap-shy species as the beech marten (Rondinini and Boitani, 2002). The device prototype has no commercial equivalent available on the market, and thus a design such as this may be necessary for research and industry alike.
Trap Design
The following description aims to facilitate the construction and modification, as well as the implementation of the presented trapping algorithm to other kinds of live-traps. The main part of the trap prototype is an inconspicuous, suspended transparent plastic cover, which is dropped onto the surface to safely capture and prevent the animal from escaping (Figure 1 and Supplemental Video 1). The main advantage of this approach is that animals are not forced to enter the trap. Instead, they can move on a familiar surface, which significantly decreases neophobic reactions (Stryjek and Modlinska, 2016). The transparent cover (L = 59.5 cm, W = 39.5 cm, H = 16.9 cm; Plast Team Basic 30 L) is suspended 20 cm above the ground. On the inside, it is equipped with a motion detector with regulated range and sensitivity. The transparent cover is fixed to a trigger lock with a chain. The trap is operated by an Arduino computer (Barrett, 2013) placed on a rack with a screen and keypad. The latter makes it possible to set an algorithm for the release operation (i.e., dropping of the transparent cover) based on the readings of the PIR (Passive Infra-Red) sensor. The user can set the number of alert cycles and their duration, and thereby set the conditions for triggering the trap. The algorithm (see Figure 2 and Supplemental Data Sheet 1) enables pre-baiting (Chitty and Kempson, 1949; Babinska and Bock, 1969). i.e., consuming the bait without operating the trap, which enables rats to roam, forage and interact under the transparent cover and gradually become habituated to any novelty such as the scent of the plastic or the electronics, as these factors are not typically associated with any aversive stimuli (Selden et al., 1991). This is crucial, as at the initial stages of contact with the bait, the rats exhibit a high level of caution and are prepared to flee rapidly.
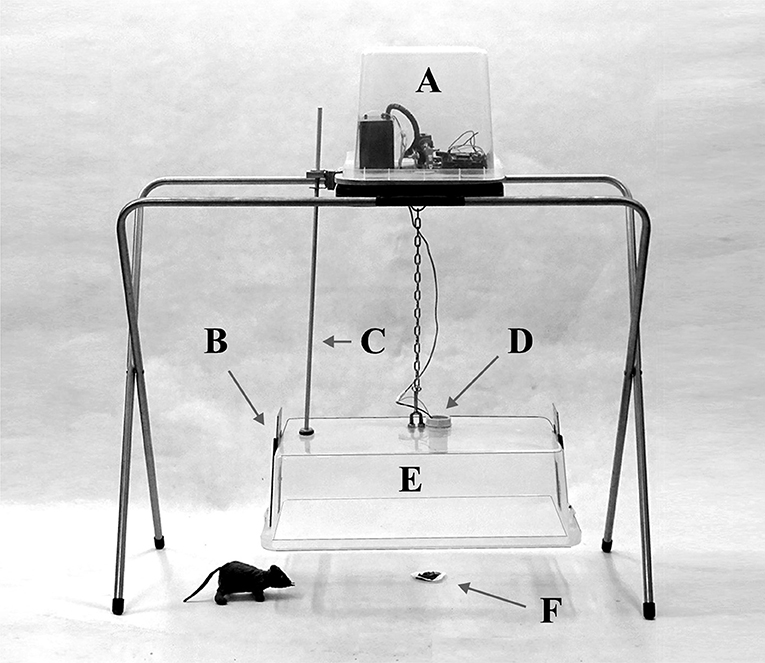
Figure 1. The tested prototype of the trap: (A) Arduino computer and triggering mechanism; (B) upward-sliding entrance gate used for taking the animals out of the trap; (C) bar preventing the transparent cover from moving and rotating; (D) motion detector with regulated range and sensitivity; (E) falling transparent cover made of plastic; (F) bait.
Among rats, much of tactile stimulation and information about the surrounding environment comes from vibrissae and guard hairs (Vincent, 1912). Therefore, the principle design of the proposed trap is composed of a suspended transparent cover hanging out of sight above the surface. Most animals adapted to nocturnal conditions, such as rodents, have reduced vision (Crawford, 1934). Thus, the transparent cover is very difficult to detect. This allows the researcher or PMP to place the device in targeted areas of known activity. Such as on paths frequently chosen by the animals (rats display strong tendencies to continuously use the same pathways—see Calhoun, 1962). Placing bait on a pathway, along a wall, which is a part of familiarized and relatively safe territory increases the chance of the trap being successful. It is necessary, however, for the user to be able to set a condition that the sensor needs to be activated for several seconds to prevent the trap from being triggered by animals passing (without stopping) beneath the transparent cover.
To better conceal the device, it may not only be placed on the prototype rack, but also be suspended from a ceiling or attached to a wall. The triggering algorithm can also be used in a similar manner to that of other classic types of traps (Figure 3) allowing for prebaiting and capturing lightweight (15–20 g) animals such as e.g., mice, shrews and birds that may not be heavy enough to set off a trip pan. A Norway rat (Rattus norvegicus) in comparison may average 300 g (Parsons et al., 2017). As for the bait used, rats should not be able to take it away. This why the bait should be viscous and smeared on the floor (RS, MHP observations). To increase attractiveness of the bait, sex hormones and pheromones may also be applied (Takács et al., 2016). Recent data suggests that such application significantly improves trapping success among rats and mice (Takács et al., 2016; Musso et al., 2017). Another way of facilitating captures is generating species-specific auditory cues, which may increase the attraction for animals (Takács et al., 2016).
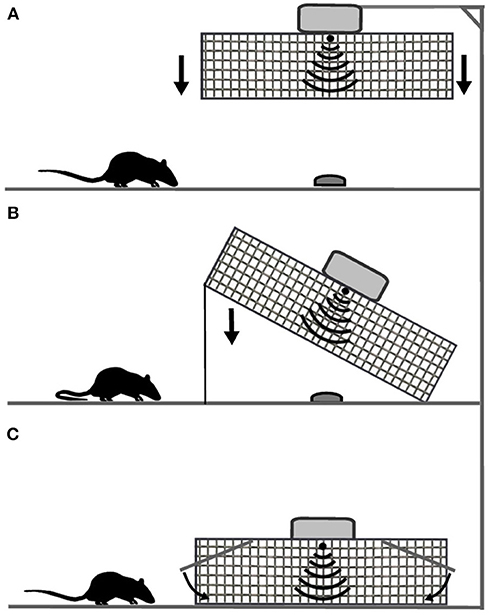
Figure 3. Three ways of implementing the idea: (A) falling transparent cover attached to a rack, ceiling or wall; (B) classic drop trap; (C) classic wire mesh live trap.
Methods
Study Site
This preliminary study was conducted over 1 month (September, 2015) on a wild living colony of Norway rats (Rattus norvegicus) on a farm consisting of 4 separate, distant buildings separated from one another by ~30 m, situated on the outskirts of Warsaw, Poland (52.13°N, 21.00°E) The farm was inhabited by at least two rat colonies. The size of the tested colony had not been controlled for approximately the last 3 years prior to the experiment—neither by means of poisons, nor mechanically. The second colony was not utilized in order to avoid any possible interference or interruption with other behavioral tests conducted on the property (Modlinska and Stryjek, 2016; Stryjek et al., 2018). Cocoa-peanut butter (Nutella®) was used as bait, and it was smeared on the surface directly underneath the transparent cover. The test was conducted in three separate trapping spots placed in three different rooms on the farm.
Placement
The surface or floor under the trap prototype should be flat and solid to prevent animals from escaping by digging burrows. Another possible way to prevent escapes is to put a metal wire mesh on the ground or to place it underground and cover it with a thin layer of soil. However, if there are concerns that animals try to dig through the wire, then a plexiglass plate covered with soil may be substituted. Importantly, when this device is utilized outdoors in unpredictable or inclement weather, we recommend either using (or installing) a wind break 3 weeks prior to trapping or making changes to the construction of the rack, causing it to be more firmly situated without swinging. Another option might be to construct a composite weighted rack that limits swinging, however, the researchers would have to account for the fact that a weighted rack could then cause harm to animal when it drops. Therefore, for animal ethics concerns, a heavier outer cover might be fixed in place and subsequently release an internal, but lighter, cover. The wind break approach would not have any impact on trap shy animals, whereas the latter choice includes a physical structure and might require prebaiting alongside the rack. The choice would be up to the reader and the local context of presentation. We recommend these traps be remotely monitored, because unlike normal traps, food, water and shelter will be unavailable unless the end-user makes modifications to the design.
To safely and reliably remove captured animals from the trap, we rely on a phenomenon called thigmotaxis (a tendency to hide in small spaces; Treit and Fundytus, 1988; Stryjek and Modlinska, 2013). Captured rodents will almost always try to flee from the trap. We take advantage of this tendency by transferring them to cardboard boxes, from which they can be moved into transport cages (Stryjek and Modlinska, 2013). After placing a cardboard box next to one of the gate entrances at the sides of the transparent cover, the gate may be opened. The animal, seeking a way to flee, will escape into the adjacent box, in which it can then be transported to a different place (Supplemental Video 2).
Except for the instance when the transparent cover is released and dropped, the device operates in absolute silence—neither the PIR sensor, nor the other electronic elements emit sounds (they have been tested with an ultrasound detector—Avisoft-UltraSoundGate 116Hb). The construction of the prototype, as well as a circuit diagram are demonstrated in Figure 4. The cost of parts used in the construction is relatively low (<$75) and would be much smaller if mass produced. Though, this expense, even at its most expensive point, could be justified against the overall benefit of highly-variable animal models in the research laboratory (Stryjek and Pisula, 2008), the potential for reduced risk of disease in the wild (Firth et al., 2014; Frye et al., 2015), and the billion-dollar worldwide industry of rodent control (Parsons et al., 2015).
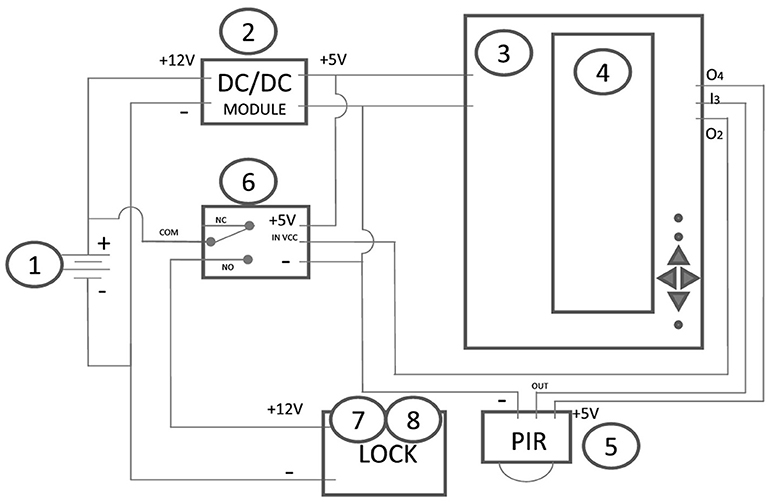
Figure 4. Electric diagram of the trap: (1) battery 12V/7AH; (2) DC LM2596 module; (3) Arduino Leonardo ATmega32u4 R3; (4) LCD keypad shield LCD 1602; (5) PIR HC SR-501; (6) relay; (7) actuator (car central lock actuator); (8) lock (car trunk lock).
Assessment Phase
We did not have any set duration for the initial assessment, our only intentions were to (1). establish a catch rate of the animals in a single colony, and (2). determine whether a random sample of animals from a population were captured. Our measure of success was to catch a range of males, females, juveniles, adults. Most importantly, we wanted to determine if healthy individuals or potential high socially ranking animals (no scars or obvious parasitism) were being captured as frequently as sick animals. Lastly, we were interested in determining the latency for repeated capture of animals or repeated use of target areas by previously captured animals. One important aspect that simplified our assessment was knowing that we did not have to mark animals prior to their participation. Because the trap is non-detectable (the essential aspect of the design), animals are ostensibly unaware that there is any trap present. Thus, following capture, the animal would either continue to use the area, in which case their next possible capture would not have been influenced by the previous capture, or the animal would avoid the area. Either way, repeated captures could not influence our outcomes.
We used 4 IR cameras CMOS EC-832-SCH connected to a DVR recorder (EC-7804T), which enabled 24-h observation. The trap and videos were checked on a daily basis. We focused specifically on testing the triggering mechanism set to release the transparent cover after 5 cycles of sensor activation, each lasting 5 s.
Results
The trap was operated 34 times, and 32 animals were captured (25 Norway rats and 7 house mice) which represents a 94% capture rate of animals that trigger the trap. There were no obvious signs of non-random sampling—all trapped rats appeared to be representative of different sizes and ages. Importantly, there were no signs of reduced social status [e.g., scar markings on tail, rump or neck; see Boice, 1971b] or poor health (porphyrin around eyes, uneven vibrissae and guard hairs, or obvious blood) were observed. A qualitative analysis of the video recordings showed that the transparent cover suspended 20 cm above the ground does not elicit any obvious reaction in animals (there were no observed instances of exploratory behaviors, such as rearing or sniffing). After 2–4 captures, some animals started to exhibit avoidance reactions toward the place of trapping. The animals, however, did not avoid the trap itself—when relocated to a different place within the reach of the same colony, the trap proved effective again. A decreased exploration of a given place was observed also after the trap had been removed to another room, which means that the evoked avoidance reaction was linked to the place, and not to the device itself.
Discussion
We prepared a working prototype to be further developed by other researchers and pest control professionals based on their own context and needs. Our initial results for a single colony of Norway rats in a peri-urban area near Warsaw (Poland) are promising enough to warrant further experimental trials. The range of individuals within a population captured and the low latency suggests the inconspicuous design of the prototype trap helped overcome trap shyness or neophobia. This phenomenon has been identified as the primary barrier to capturing a random sample of animals (Stryjek and Modlinska, 2016) from a population. The efficiency, 32 animals among 34 triggers (94%) was likely higher than among traditional traps, The low efficiency of traditional traps is well known among researchers and PMPs who commonly return to traps that have been triggered, but are empty. We also note that the cost of parts used for construction was relatively low when compared against the benefits of improved lab animal models (Stryjek and Pisula, 2008) and rodent control (Parsons et al., 2015).
The use of a suspended transparent cover meant that it is highly unlikely that the animals would get any sense of a potentially threatening object. This was demonstrated by the low latency of rats approaching the bait with no exploratory behaviors around the trap area. After the trap had been triggered several times, the animals' reaction was to avoid the place where the trap had been placed rather than the trap itself, which when moved elsewhere did not evoke neophobic reaction. Seven non-target animals (Mus musculus) were captured. Though we note that further adjustments could have been made to the algorithm in order to limit species to a given weight range. We believe this design is among the first to provide reasonable control over capture of non-target species.
The efficiency of the trap is increased by using an adjustable time of sensor activation and an adjustable number of alert cycles before the triggering mechanism releases the transparent cover—at the initial stages of contact with a new bait, the animals exhibit caution and are prepared to flee rapidly. Moreover, the condition that the sensor needs to be activated for several seconds prevents the trap from being triggered by animals passing through beneath the transparent cover, which makes it possible to place the trap on frequently chosen paths.
In our opinion, potential improvements could involve reducing the size of the device, which is relatively large in its prototype version, as well as using a metal wire mesh instead of plastic. A transparent cover construction would improve the airflow, prove more difficult to bite through or lift by any animals trying to escape. The type of research desired will determine which changes should be made. For instance, in some rural or field applications, a shaded cover instead of transparent should be considered. This allows animals some form of shelter. Inside a building however, the transparent cover seems ideal, as it allows for the ease of identification of the type of the target animal.
It seems worthwhile to consider suspending the trap from a ceiling or wall. This is because using the rack in the prototype introduced changes into the space in which the animals moved, thereby potentially triggering a neophobic reaction. We believe another improvement would involve software modification to enable the user to set the time in which the trap would be active (e.g., to trap nocturnal animals and avoid trapping diurnal animals). An improved system could also inform its users about all instances when the trap was triggered via text or multimedia messages (Macdonald et al., 1999; Notz et al., 2017), which would allow an identification of the trapped species and shorten the time the animal is kept inside the trap.
The above-described alterations could help test the efficiency of this presented triggering mechanism in trapping other animal species. Its implementation into other kinds of traps may help improve trapping efficiency (both the number of captured rats, and representative samples among a population) while reducing the risk of accidental capture of non-target animals, without any risk of secondary poisoning or comprised animal safety. While improved techniques to camera trapping are regularly being made (Norouzzadeh et al., 2018), there have been few changes to live traps. We hope other researchers and pest control professionals will utilize and adapt the algorithm to their own needs.
Data Availability Statement
The authors are keen for other researchers to consider or improve upon their design, thus all information is available upon request to RS.
Ethics Statement
This trap meets or exceeds current requirements for ethical use (Powell and Proulx, 2003; Gannon and Sikes, 2007). Under Polish law, trapping pest species does not require permission of the local ethics committee for animal experimentation. None of the captured animals spent more than 10 h inside the trap. Some of the captured rats were set free on the spot (16%). This type of trap should always be remotely monitored because warm bedding, food, and/or water may not be available unless the end-user customizes the application to their needs.
Author Contributions
RS and AK designed the prototype and conducted the study. RS and MP wrote the article.
Funding
This research project was supported by the National Science Centre (Poland)—grant no. UMO-2013/09/B/HS6/03435.
Conflict of Interest Statement
The authors declare that the research was conducted in the absence of any commercial or financial relationships that could be construed as a potential conflict of interest.
The reviewer, AF, declared a past collaboration with one of the authors, MP, to the handling Editor.
Supplementary Material
The Supplementary Material for this article can be found online at: https://www.frontiersin.org/articles/10.3389/fevo.2019.00011/full#supplementary-material
Supplemental Video 1. Video presenting the trap in action.
Supplemental Video 2. Video presenting the technique of taking captured animals out from the trap.
Supplemental Data Sheet 1. Trap algorithm.
References
Abbott, I. (2000). Improving the conservation of threatened and rare mammal species through translocation to islands: case study Western Australia. Biol. Conser. 93, 195–201. doi: 10.1016/S0006-3207(99)00144-5
Anthony, N. M., Ribic, C. A., Bautz, R., and Garland, T. Jr. (2005). Comparative effectiveness of Longworth and Sherman live traps. Wildlife Soc. Bull. 33, 1018–1026. doi: 10.2193/0091-7648(2005)33[1018:CEOLAS]2.0.CO;2
Babinska, J., and Bock, E. (1969). The effect of pre-baiting on captures of rodents. Acta Theriol. 14, 267–270. doi: 10.4098/AT.arch.69-20
Barnett, S. A. (1958). An analysis of social behaviour in wild rats. J. Zool. 130, 107–152. doi: 10.1111/j.1096-3642.1958.tb00565.x
Barrett, S. F. (2013). Arduino microcontroller processing for everyone! Synth. Lect. Dig. Circuits Syst. 8, 1–513. doi: 10.2200/S00522ED1V01Y201307DCS043
Blumstein, D. T., Mari, M., Daniel, J. C., Ardron, J. G., Griffin, A. S., and Evans, C. S. (2002). Olfactory predator recognition: wallabies may have to learn to be wary. Anim. Conser. 5, 87–93. doi: 10.1017/S1367943002002123
Boice, R. (1971a). Laboratorizing the wild rat (Rattus norvegiens). Behav. Res. Methods Instrument. 3, 177–182. doi: 10.3758/BF03208127
Boice, R. (1971b). Excessive water intake in captive Norway rats with scar-markings. Physiol. Behav. 7, 723–725. doi: 10.1016/0031-9384(71)90139-9
Burnham, K. P., and Overton, W. S. (1978). Estimation of the size of a closed population when capture probabilities vary among animals. Biometrika 65, 625–633. doi: 10.1093/biomet/65.3.625
Calhoun, J. (1962). The Ecology and Sociology of the Norway Rat. Bethesda, MD: US Public Health Service Publication,
Chao, A. (1989). Estimating population size for sparse data in capture-recapture experiments. Biometrics 45, 427–438. doi: 10.2307/2531487
Chitty, D., and Kempson, D. (1949). Prebaiting small mammals and a new design of live trap. Ecology 30, 536–542. doi: 10.2307/1932457
Corrigan, R. M., and Moreland, D. (2001). Rodent Control: a Practical Guide for Pest Management Professionals. Cleveland, OH: GIE Media
Crawford, S. C. (1934). The habits and characteristics of nocturnal animals. Q. Rev. Biol. 9, 201–214. doi: 10.1086/394460
de Milliano, J., Di Stefano, J., Courtney, P., Temple-Smith, P., and Coulson, G. (2016). Soft-release versus hard-release for reintroduction of an endangered species: an experimental comparison using eastern barred bandicoots (Perameles gunnii). Wildlife Res. 43, 1–12. doi: 10.1071/WR14257
Firth, C., Bhat, M., Firth, M. A., Williams, S. H., Frye, M. J., Simmonds, P. et al. (2014). Detection of zoonotic pathogens and characterization of novel viruses carried by commensal Rattus norvegicus in New York City. MBio 5:e01933–14. doi: 10.1128/mBio.01933-14
Frye, M. J., Firth, C., Bhat, M., Firth, M., Che, X., Lee, D., Williams, S., and Lipkin, W. (2015). Preliminary survey of ectoparasites and associated pathogens from norway rats in New York City. J. Med. Entomol. 52, 253–259. doi: 10.1093/jme/tjv014
Gannon, W. L., and Sikes, R. S. (2007). Guidelines of the American society of mammalogists for the use of wild mammals in research. J. Mammal. 88, 809–823. doi: 10.1644/06-MAMM-F-185R1.1
Griffith, B., Scott, J. M., Carpenter, J. W., and Reed, C. (1989). Translocation as a species conservation tool: status and strategy. Science 245, 477–480. doi: 10.1126/science.245.4917.477
Hice, C. L., and Velazco, P. M. (2013). Relative effectiveness of several bait and trap types for assessing terrestrial small mammal communities in neotropical rainforest. Mus. Tex Tech Univ. 316, 1–15.
Himsworth, C. G., Feng, A. Y., Parsons, K., Kerr, T., and Patrick, D. M. (2013). Using experiential knowledge to understand urban rat ecology: a survey of Canadian pest control professionals. Urban Ecosyst. 16, 341–350. doi: 10.1007/s11252-012-0261-4
Jacob, J., Ylönen, H., and Hodkinson, C. (2002). Trapping efficiency of Ugglan traps and Longworth traps for house mice in south-eastern Australia. Wildlife Res. 29, 101–103. doi: 10.1071/WR01048
Lande, R. (1976). Natural selection and random genetic drift in phenotypic evolution. Evolution 30, 314–334. doi: 10.1111/j.1558-5646.1976.tb00911.x
Littin, K., Fisher, P., Beausoleil, N., and Sharp, T. (2014). Welfare aspects of vertebrate pest control and culling: ranking control techniques for humaneness. Rev. Sci. Tech. 33, 281–289. doi: 10.20506/rst.33.1.2281
Lockard, R. B. (1968). The albino rat: a defensible choice or a bad habit? Am. Psychol. 23:734. doi: 10.1037/h0026726
Macdonald, D. W., Mathews, F., and Berdoy, M. (1999). “The behaviour and ecology of Rattus norvegicus: from opportunism to kamikaze tendencies,” in Ecologically Based Rodent Management, ACIAR Monograph, G. Singleton and H. Leirs (Melbourne, VIC: Brown Prior Anderson), 49–80.
Mason, G., and Littin, K. (2003). The humaneness of rodent pest control. Anim. Welfare. 12, 12–37. Available online at: http://hdl.handle.net/10214/4712
Mitchell, D. (1976). Experiments on neophobia in wild and laboratory rats: a reevaluation. J. Comparat. Physiol. Psychol. 90:190. doi: 10.1037/h0077196
Modlinska, K., and Stryjek, R. (2016). Food neophobia in wild rats (Rattus norvegicus) inhabiting a changeable environment—a field study. PLoS ONE 11:e0156741. doi: 10.1371/journal.pone.0156741
Musso, A. E., Gries, R., Zhai, H., Takács, S., and Gries, G. (2017). Effect of male house mouse pheromone components on behavioral responses of mice in laboratory and field experiments. J. Chem. Ecol. 43, 215–224. doi: 10.1007/s10886-017-0819-y
Norouzzadeh, M. S., Nguyen, A., Kosmala, M., Swanson, A., Palmer, M. S., Packer, C. et al. (2018). Automatically identifying, counting, and describing wild animals in camera-trap images with deep learning. Proc. Natl. Acad. Sci. U.S.A. 115, E5716–25. doi: 10.1073/pnas.1719367115
Notz, E., Imholt, C., Reil, D., and Jacob, J. (2017). Testing automated sensor traps for mammal field studies. Wildlife Res. 44, 72–77. doi: 10.1071/WR16192
Olsen, G. H., Linscombe, R. G., Wright, V. L., and Holmes, R. A. (1988). Reducing injuries to terrestrial furbearers by using padded foothold traps. Wildlife Soc. Bull. 16, 303–307.
Parsons, M. H., Apfelbach, R., Banks, P. B., Cameron, E. Z., Dickman, C. R., Frank, A. S. et al. (2018). Biologically meaningful scents: a framework for understanding predator–prey research across disciplines. Biol. Rev. 93, 98–114. doi: 10.1111/brv.12334
Parsons, M. H., Banks, P. B., Deutsch, M. A., Corrigan, R. F., and Munshi-South, J. (2017). Trends in urban rat ecology: a framework to define the prevailing knowledge gaps and incentives for academia, pest management professionals (PMPs) and public health agencies to participate. J. Urban Ecol. 3:jux005. doi: 10.1093/jue/jux005
Parsons, M. H., Banks, P. B., Deutsch, M. A., and Munshi-South, J. (2018). Temporal and space-use changes by rats in response to predation by feral cats in an urban ecosystem. Front. Ecol. Evol. 6:146. doi: 10.3389/fevo.2018.00146
Parsons, M. H., Sarno, R., and Deutsch, M. (2015). Jump-starting urban rat research: conspecific pheromones recruit wild rats into a behavioral and pathogen-monitoring assay. Front. Ecol. Evol. 3:146. doi: 10.3389/fevo.2015.00146
Parsons, M. H., Sarno, R. J., and Deutsch, M. A. (2016). A Detailed Protocol to enable safe-handling, preemptive detection, and systematic surveillance of rat-vectored pathogens in the urban environment. Front. Public Health 4:132. doi: 10.3389/fpubh.2016.00132
Powell, R. A., and Proulx, G. (2003). Trapping and marking terrestrial mammals for research: integrating ethics, performance criteria, techniques, and common sense. Ilar J. 44, 259–276. doi: 10.1093/ilar.44.4.259
Ribeiro-Júnior, M. A., Rossi, R. V., Miranda, C. L., and Ávila-Pires, T. C. (2011). Influence of pitfall trap size and design on herpetofauna and small mammal studies in a Neotropical Forest. Zoologia 28, 80–91. doi: 10.1590/S1984-46702011000100012
Rondinini, C., and Boitani, L. (2002). Habitat use by beech martens in a fragmented landscape. Ecography 25, 257–264. doi: 10.1034/j.1600-0587.2002.250301.x
Selden, N. R., Everitt, B., Jarrard, L., and Robbins, T. (1991). Complementary roles for the amygdala and hippocampus in aversive conditioning to explicit and contextual cues. Neuroscience 42, 335–350. doi: 10.1016/0306-4522(91)90379-3
Sikes, R. S., Gannon, W. L., Care, A., and Mammalogists, U. C.,o.t.A.S.o (2011). Guidelines of the American Society of Mammalogists for the use of wild mammals in research. J. Mammal. 92, 235–253. doi: 10.1644/10-MAMM-F-355.1
Singleton, G. R., Brown, P. R., Jacob, J., and Aplin, K. P. (2007). and Sudarmaji, Unwanted and unintended effects of culling: a case for ecologically-based rodent management. Integrat. zool. 2, 247–259. doi: 10.1111/j.1749-4877.2007.00067.x
Stokes, V. L. (2013). Trappability of introduced and native rodents in different trap types in coastal forests of south-eastern Australia. Aust. Mammal. 35, 49–53. doi: 10.1071/AM12002
Stryjek, R., Mioduszewska, B., Spaltabaka-Gedek, E., and Juszczak, G. R. (2018). Wild Norway rats do not avoid predator scents when collecting food in a familiar habitat: a field study. Sci. Rep. 8:9475. doi: 10.1038/s41598-018-27054-4
Stryjek, R., and Modlinska, K. (2013). A thigmotaxis-based method of recapturing and transporting small mammals in the laboratory. Lab Anim. 42:321. doi: 10.1038/laban.328
Stryjek, R., and Modlinska, K. (2016). Neophobia in wild rats is elicited by using bait stations but not bait trays. Int. J. Pest Manag. 62, 158–164. doi: 10.1080/09670874.2016.1147622
Stryjek, R., Modlinska, K., and Pisula, W. (2012). Species specific behavioural patterns (digging and swimming) and reaction to novel objects in wild type, Wistar, Sprague-Dawley and Brown Norway rats. PLoS ONE 7:e40642. doi: 10.1371/journal.pone.0040642
Stryjek, R., and Pisula, W. (2008). Warsaw wild captive pisula stryjek rats (WWCPS)-establishing a breeding colony of Norway Rat in captivity. Polish Psychol. Bull. 39, 67–70. doi: 10.2478/v10059-008-0011-x
Takács, S., Gries, R., Zhai, H., and Gries, G. (2016). The sex attractant pheromone of male brown rats: identification and field experiment. Angew. Chem. Int. Ed. 55, 6062–6066. doi: 10.1002/anie.201511864
Thompson, G. G., and Thompson, S. A. (2007). Usefulness of funnel traps in catching small reptiles and mammals, with comments on the effectiveness of the alternatives. Wildlife Res. 34, 491–497. doi: 10.1071/WR06081
Thompson, H. V. (1953). Experimental live trapping of rats, with observations on their behaviour. Br. J. Anim. Behav. 1, 96–111. doi: 10.1016/S0950-5601(53)80002-X
Treit, D., and Fundytus, M. (1988). Thigmotaxis as a test for anxiolytic activity in rats. Pharmacol. Biochem. Behav. 31, 959–962. doi: 10.1016/0091-3057(88)90413-3
Vincent, S. B. (1912). The Functions of the Vibrissae in the Behavior of the White Rat. Chicago, IL: University of Chicago.
Keywords: arduino, lab-rat model, neophobia, random-sampling, trapping, trap-shyness, randomization, wild rat
Citation: Stryjek R, Kalinowski A and Parsons MH (2019) Unbiased Sampling for Rodents and Other Small Mammals: How to Overcome Neophobia Through Use of an Electronic-Triggered Live Trap—A Preliminary Test. Front. Ecol. Evol. 7:11. doi: 10.3389/fevo.2019.00011
Received: 21 September 2018; Accepted: 14 January 2019;
Published: 12 February 2019.
Edited by:
Darryl Jones, Griffith University, AustraliaReviewed by:
Yasushi Kiyokawa, The University of Tokyo, JapanAnke Sybille Keiko Frank, Zoological Research Museum Alexander Koenig, Germany
Copyright © 2019 Stryjek, Kalinowski and Parsons. This is an open-access article distributed under the terms of the Creative Commons Attribution License (CC BY). The use, distribution or reproduction in other forums is permitted, provided the original author(s) and the copyright owner(s) are credited and that the original publication in this journal is cited, in accordance with accepted academic practice. No use, distribution or reproduction is permitted which does not comply with these terms.
*Correspondence: Rafal Stryjek, cnN0cnlqZWtAd3AucGw=