- Program in Science, Technology and Environmental Policy, Woodrow Wilson School of Public and International Affairs, Princeton University, Princeton, NJ, United States
The response of biodiversity to land-use change has been a central focus in applied ecological research for close to half a century. However, despite a vast body of literature, our understanding of how species' traits influence demographic vital rates in anthropogenically-modified habitats is remarkably scant. Such an understanding is crucial because vital rates determine population viability in modified habitats, and underlie emergent occupancy, abundance and community-level patterns. I used capture-recapture analyses to estimate variation in survival of birds in intact and logged tropical montane forest in the eastern Himalayas. In general, variation in body mass and alternative behavioral strategies (e.g., mixed-species flocking vs. solitary behavior) were not associated with survival differences in intact forest. However, year-round residents, and species that did not participate in mixed-species flocks had appreciably lower survival in logged forest compared with intact forest. Solitary foragers, for instance, faced a 30% decline in survival in logged forest compared with intact forest. Non-migratory habit and solitary foraging behavior might make species vulnerable to extinction in logged forest through reduced survival, an especially important process in influencing population viability. Identifying how species' traits modulate their response to land-use change is crucial to predict population responses to forest modification, and to better plan and manage biodiversity-friendly forest use.
Introduction
The alteration of tropical forests is globally pervasive. Two of the most widespread forms of forest conversion are fragmentation, which results in isolated forest patches, and selective logging, which involved the extraction of a subset of forest trees. The impacts of fragmentation and logging on the structure and function of ecological communities has received attention since the dawn of conservation biology (reviews in Gibson et al., 2011; Laurance et al., 2016). Further, there is a broad consensus on traits correlated with vulnerability to forest modification (Burivalova et al., 2015). In general, large, long-lived, higher-trophic species decline with logging, and communities in modified forest tend to be dominated by small, highly fecund species at lower trophic levels (Srinivasan, 2013; Hamer et al., 2015). Despite this extensive body of work, surprisingly little is known about how demographic vital rates (e.g., survival and fecundity) are influenced by the interaction between species' traits and habitat change, even in exceptionally well-studied taxa such as birds (Cossett et al., 2019). Such an understanding is crucial because: (a) vital rates—rather than occupancy or abundance—determine population viability in anthropogenically modified forest, and (b) altered vital rates underpin abundance changes, and therefore community structure and function (Cossett et al., 2019).
In birds, how morphological and behavioral traits influence vital rates is known largely from primary neotropical forest. For instance, body size is positively correlated with survival, but negatively with recruitment (Brawn et al., 1995). A meta-analysis found that species that always foraged in mixed-flocks had higher survival rates than those that did not (Jullien and Clobert, 2000), although several studies from multiple neotropical sites have failed to detect survival differences between flocking and non-flocking species (Brawn et al., 1995; Blake and Loiselle, 2008; Wolfe et al., 2014). There is no compelling evidence that survival rates differ between resident and migratory species (Sandercock and Jaramillo, 2002).
Where demographic vital rates have been compared between intact and modified habitats, such comparisons have been limited to one or few species (Bakermans et al., 2009; Srinivasan et al., 2015; Wolfe et al., 2015), precluding the discovery of generalisable relationships between species' traits and anthropogenically-driven changes in vital rates. For instance, forest fragmentation reduced survival (Doherty and Grubb, 2002) and population growth (Zanette, 2000) in temperate birds, but combined, these studies were able to estimate vital rates for only five species. From the tropics, experimental forest modification did not result in survival differences in two species, but hinted at the survival value of participation in mixed-species flocks (Cruz-Angon et al., 2008). To my knowledge, only Korfanta et al. (2012) compare vital rates for a suite of species in intact and modified (fragmented) forest, concluding that almost all diet guilds are vulnerable to extinction in forest fragments. However, Korfanta et al. (2012) did not test for relationships between vital rates in modified forest and fundamental species' properties such as body mass and migratory behavior, which are crucial determinants of life history. Characterizing such environment-survival relationships—and how these can be modulated by species' traits—is especially important to both understand and mechanistically predict the impacts of land use change on tropical biodiversity (Ruiz-Gutierrez et al., 2012).
I asked how morphological and behavioral species' traits interacted with selective logging—a widespread global threat to tropical biodiversity (Edwards and Laurance, 2013)—to influence the survival of birds in the eastern Himalaya global biodiversity hotspot. Specifically, I tested the following predictions, related to three species traits:
a. Body size. With increasing mass, species should suffer greater survival declines in logged forest, because larger species are more vulnerable to forest modification, and decline in abundance in logged forest (Srinivasan, 2013).
b. Mixed-species flocking. Logging should cause greater survival reductions in species that forage solitarily or in monospecific flocks, compared with species that forage in mixed-species flocks. This is because mixed-species flock participants receive foraging and anti-predator benefits from other species in the group (Sridhar et al., 2009), and should therefore be capable of adapting behaviorally (by altering flock size and composition) to logging-induced resource reduction and/or enhanced predation.
c. Migratory behavior. I remained agnostic about how logging might differentially affect survival for year-round residents vs. elevational migrants. Although migration might allow species to maintain an optimal abiotic environment throghout the year, resident species might benefit through the “local knowledge of food and predator dispersion” on year-round territories (Sandercock and Jaramillo, 2002; Cooper et al., 2015).
I did not test for the effect of diet or vertical stratum because almost all species are understorey insectivores (Table 1), a functional guild particularly vulnerable to forest modification (Powell et al., 2015).
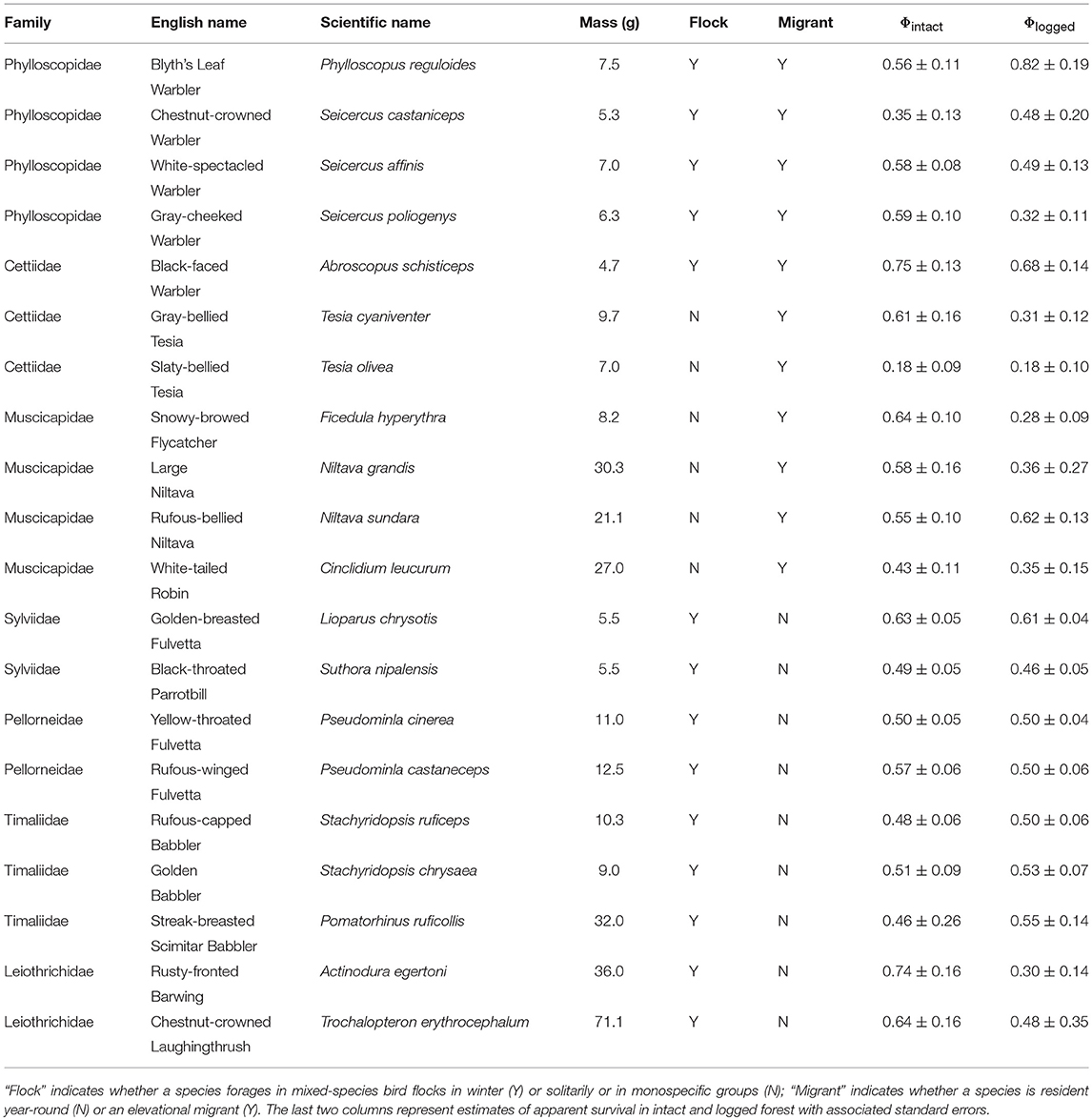
Table 1. List of species analyzed, with relevant life-history traits and estimates of apparent survival in intact and logged forest.
Materials and Methods
I sampled montane broadleaf wet evergreen forest (Champion and Seth, 1968) dominated by Quercus lamellosa and Michelia doltsopa in the canopy, and bamboo (Chimonobambusa sp.) in the understorey. Forest patches were selected for sampling based on interviews with persons involved in logging operations, and variation in tree densities on these plots tallied exactly with semi-quantitative estimates of timber extraction (Srinivasan, 2013). Tree densities in intact forest were roughly twofold that in logged forest. I established six sampling plots, three each in intact and logged forest (varying from 2.5 to 4.0 ha in size) at ~2000 m ASL in Eaglenest Wildlife Sanctuary, West Kameng district, Arunachal Pradesh, India (27.07°N; 92.40°E, Figure 1). Sampling plots varied in size depending on logging history and accessibility for sampling. Three of these plots were intensively logged until 2002 (“logged forest”; tree densities [DBH ≥ 10 cm] ranging from 76 to 109 trees ha−1), while the other three were either never, or very minimally, logged (“intact forest”; tree densities: 168 to 192 trees ha−1; Srinivasan, 2013; Figure 1). In total, across the three plots in each habitat (intact and logged), I sampled 9 ha each in intact and logged forest separately (Figure 1). Arunachal Pradesh has the highest latitudinal extent of tropical forests globally (Proctor and Haridasan, 1998). In each plot, a team operated 24–28 mist nets (12 m length, 4 shelf, 16 mm mesh size; 158 nets in total) from 0500 to 1200 h for three consecutive days in April-May each year, from 2011 to 2017. Nets were set up systematically within a plot, with neighboring nets placed ~40 m apart (Figure 1). April-May is the early breeding season for birds in the eastern Himalayas, when the young of most species (and all species included in my analyses) have not fledged. Therefore, all individuals captured are adults. Birds captured in nets were weighed, ringed with numbered aluminum leg rings, and released.
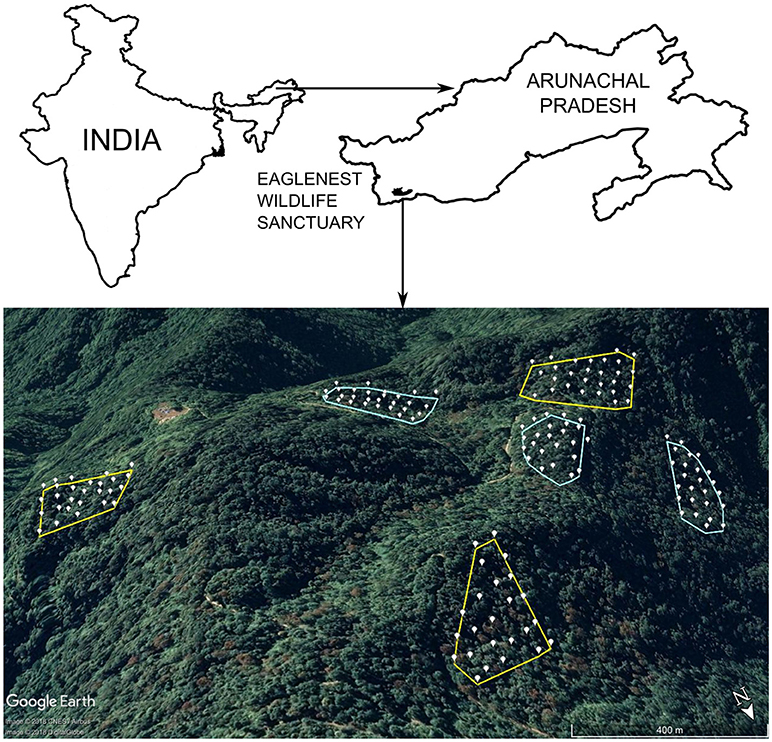
Figure 1. Map of the study area, with sampling plots and net locations shown. Intact forest plots are outlined in green and logged forest plots in red. White points represent the placement of individual mist nets. Inset shows the outline of India, with the black dot indicating the position of Eaglenest Wildlife Sanctuary in northeast India.
For analysis, I selected species represented by at least 20 individuals in intact and logged forest separately. All species included in the analysis breed on our sampling plots. For each individual bird, I created a capture history spanning seven years. Multiple captures within a year (e.g., on subsequent sampling days) were not considered recaptures for analytical purposes. Therefore, in capture histories, all captures within a sampling season (i.e., year) were collapsed into a single occurrence. Because logged forest plots were, on average, closer to each other than intact forest plots, recapture rates in logged forest might be higher than those in intact forest. I therefore calculated plot-level proportion of individuals recaptured for each species (i.e., number of individuals recaptured divided by total number of individuals ringed), and then calculated the mean and standard deviation of capture rate for plot-level information for each species in each habitat (Table S1). For 19 of 20 species analyzed, there were no differences in the proportion of individuals recaptured between intact and logged forest (overlapping standard errors, Figure S1), and no consistent habitat-dependent direction in the mean of recapture rates across species (Figure S1).
I pooled capture histories from the three intact forest plots to represent individuals sampled in intact forest, and did the same with capture histories from the three logged forest plots. I pooled data to enable the robust estimation of apparent survival (Φ) in intact and logged forest for each species, because plot-level data was inadequate to enable survival estimation for each species in each plot. (Separate plots were initially established to span the gradient in logging intensity, ranging from 76 to 192 trees per ha). I used the Cormack-Jolly-Seber (CJS) open-population mark-recapture model to estimate adult survival (Cormack, 1964; Jolly, 1965; Seber, 1965) for each of 20 species in intact and logged forest separately (Table 1), an approach that simultaneously accounts for imperfect detection (p)–situations in which only a proportion of individuals are observed/captured (Seber, 1965). For each species in each habitat (i.e., 40 different sets of capture histories), I first ran tests to ensure that CJS models fitted the capture history data well, using the R2ucare package (Gimenez et al., 2017) in Program R (R Core Team, 2018), and following the decision tree outlined in Figure 1 in Gimenez et al. (2017). For each species, I ran two models each in intact and logged forest separately; one in which recapture probability was constant across all sampling occasions, and another in which recapture probability varied with time. I constrained apparent survival to be invariant across the six annual inter-breeding intervals in both models. For each species, I selected the best model (of the two considered, i.e., time-varying and time-invariant recapture probability) as determined by the small sample size-corrected Akaike's Information Criterion (AICc), and I report survival estimates from the best model (Table 1).
I used ordinary least squares regression models to relate survival estimates in intact and logged forest to the natural log of body size (in grams; averaged from our measurements), a continuous predictor. Species' body masses ranged from 4.5 to 69.0 g (Table 1). I obtained data on other species' traits (mixed-species flocking, migratory and foraging behavior) from published sources (Rasmussen and Anderton, 2012). These traits were both binary categorical predictors. Fourteen species participate in winter mixed-species bird flocks, while six forage either solitarily or in monospecific groups. Ten species hold year-round territories, whereas 10 were elevational migrants, breeding at higher elevations and wintering at lower elevations (Table 1). I compared the survival of species with mutually exclusive strategies for a particular trait (e.g., resident vs. elevational migrant) in intact forest, in logged forest, and between intact and logged forest. For these comparisons, I calculated mean annual survival (and standard errors) across all species sharing a strategy for a given trait, separately for intact and logged forest. To account for uncertainty in the estimation of survival rates, I assessed whether survival means differed from each other using weighted ordinary least-squares ANOVAs (with model weights as the inverse of the standard error of survival estimates).
I used Pagel's λ to initially assess whether differences in survival between intact and logged forest showed any relationship to evolutionary relatedness using a phylogenetic tree constructed using data from Jetz et al. (2012).
Results
I recorded 5,298 captures of 3,310 individuals of 20 species. Overall, 34.4% of individuals were captured more than once (see Table S1 for species-specific capture rates in intact and logged forest separately). The total effort was 278,712 net-meter-hours. Overall, 93.4% of individuals were recaptured only on the plots on which they were initially captured. In other words, only 6.6% of individuals were captured in more than one sampling plot despite the relative proximity of plots (Figure 1), indicating that populations on each plot were representative of the demographic processes operating on them, and were minimally influenced by the habitats surrounding a plot. Further, only 3.4% of individuals captured initially on one habitat type (intact or logged) were subsequently recaptured in a different habitat type. These individuals were excluded from the analyses. Goodness-of-fit testing indicated that standard CJS models were appropriate for each species in each habitat, and did not require correcting for either overdispersion or the presence of transient individuals (p-value for χ2 goodness-of-fit tests > 0.05 for all species in both habitats).
Across species, the model with time-varying capture probability was a better fit than the model with constant capture probability (based on the small sample size-corrected Akaike's Information Criterion) for only three species, in both intact and logged forest. These species were Niltava sundara (Rufous-bellied Niltava), Pseudominla castaneceps (Rufous-winged Fulvetta) and Pseudominla cinerea (Yellow-throated Fulvetta). For all other (i.e., 17) species, the model with constant capture probability performed better than time-varying capture probability in both intact and logged forest.
Ten species had higher survival in intact than in logged forest, six species had roughly equal survival in both habitats, while for four species, survival increased with logging (Table 1).
Body Mass and Survival
I found no relationship between the natural log of body mass and inherent survival (i.e., survival in intact forest; βintact = 0.02 ± 0.04 SE). and also no relationship between the natural log of body mass and survival differences between intact and logged forest (βlogged = −0.06 ± 0.04; R2 = 0.1; p = 0.22; Figure 2A).
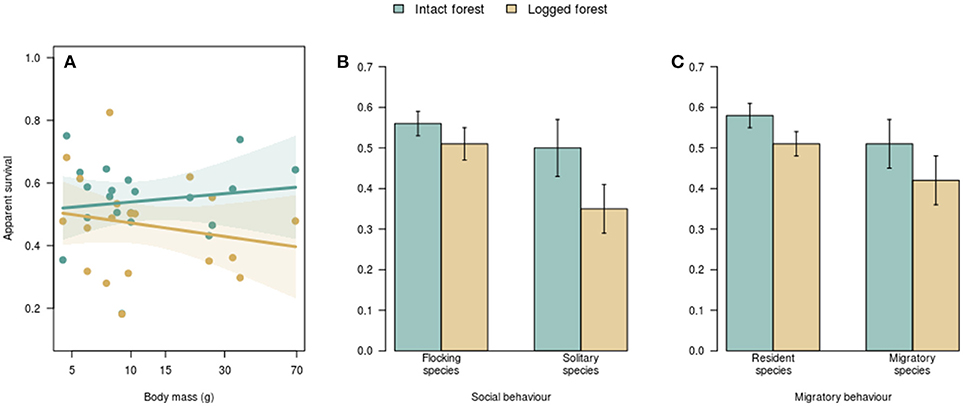
Figure 2. The relationship between species traits and apparent survival in intact vs. logged forest. Body size was not associated with survival rates in either intact or logged forest (A). Each dot represents a species, and the polygons represent the 95% confidence interval around fitted relationships. There was no difference in survival of flocking and non-flocking (B) or resident and migratory (C) species in intact forest. Non-flocking species (B) and residents (C) had lower survival in logged forest. Error bars represent one standard error.
Mixed-Species Flocking and Survival
Flocking behavior was related to the manner in which logging influenced survival (R2 = 0.28; p = 0.01; Figure 2B). In intact forest, the apparent survival of species that foraged in winter mixed-species flocks (0.56 ± 0.03 SE) did not differ from that of non-flocking species (0.50 ± 0.07; Figure 2B). In logged forest, however, the survival of flocking species (0.51 ± 0.04) was higher than that of non-flocking species (0.35 ± 0.06; Figure 2B). While there was no difference in the survival of flocking species between intact (0.56 ± 0.03) and logged forest (0.51 ± 0.04), as expected, non-flocking species had lower survival in logged forest than in intact forest; on average, the survival of non-flocking species in logged forest (0.35) was 30% lower than in intact forest (0.50; Figure 2B).
Elevational Migration and Survival
Migratory behavior was appreciably related to changes in survival with logging (R2 = 0.22; p = 0.03). Residents and elevational migrants had similar survival in intact forest (0.58 ± 0.03 and 0.51 ± 0.05, respectively; Figure 2C) and logged forest (0.51 ± 0.03 and 0.42 ± 0.06; Figure 2C). However, while the survival of elevational migrants did not differ between intact (0.51 ± 0.05) and logged (0.42 ± 0.06) forest, resident species had, on average, 12% lower survival in logged forest (0.51) compared with intact forest (0.58; Figure 2C).
Pagel's λ indicated that survival differences of between intact and logged forest were not phylogenetically structured (probability of difference from Brownian motion, χ2 test = 0.99).
Discussion
I estimated adult survival rates for 20 eastern Himalayan species in intact and logged forest separately. Based on the small sample size-corrected Akaike's Information Criterion, capture probabilities remained constant across years (in intact and logged forest separately) for 17 of 20 species. For three species, capture probabilities were time-varying in both intact and logged forest. This indicates that land use change did not result in heterogeneity in capture probabilities over time, but that for these three species, capture probabilities might be inherently variable across time. For these species, variation in capture probability might be related to greater flexibility in foraging height compared with other species.
I expected that logging would cause changes in survival in different ways for different species. Indeed, of the 20 species I analyzed, the survival of 10 species was lower in logged forest, did not appear to be influenced by logging for six species, and was associated with increased survival for four species. Variation in how survival is impacted by logging is likely to be driven by the interaction between species' traits and changes to forest structure following logging. Selective logging causes structural changes to forest, including canopy thinning and increased volume of understorey vegetation. In turn, these structural changes can modify resource availability (Zanette, 2000) and predation risk (Hua and Sieving, 2016). Individuals in logged forest would therefore need to adapt to these changes to optimize survival in logged forest. The results of this study show that the degree to which species are capable of surviving such environmental changes hinge on their traits.
For eastern Himalayan tropical montane birds, selective logging did not result in altered survival for species that foraged in winter mixed species flocks or for migratory species. However, non-flocking species and year-round residents showed survival declines in logged forest (Figure 2).
Mixed-Species Flocking and Survival
From a meta-analysis of survival rates of neotropical birds, Jullien and Clobert (2000) concluded that obligate flocking species (i.e., species that always foraged in mixed-species flocks) had higher annual survival than either facultative flocking species or solitary foragers. However, such patterns might be confounded by significant within-species variation in survival over large geographical scales (Wolfe et al., 2014), making across-site species comparisons problematic. Like several prior studies (Brawn et al., 1995; Blake and Loiselle, 2008; Wolfe et al., 2014), I found no evidence for any site-specific survival differences between flocking and non-flocking species in intact forest. The lack of survival differences between flocking and non-flocking species in intact habitat should perhaps not be surprising, given that both types of species might adopt different life-history strategies to maximize survival (Ghalambor and Martin, 2001).
For instance, while flocking species might rely on enhanced resource availability and predator avoidance in flocks (Sridhar et al., 2009) to increase survival probability, non-flocking species might vary reproductive investment between males and females to differentially prioritize sex-specific survival (Santos and Nakagawa, 2012), with consequences for overall species' survival. In this dataset, none of the flocking species are sexually dimorphic; however, males and females of two-thirds of non-flocking species differ in plumage, indicating that flocking and non-flocking species might allocate resources differentially to sex-specific reproduction and survival. Estimating and evaluating sex-specific survival rates for both flocking and non-flocking species will be important to test for such potentially different life-history strategies.
For flocking species, survival was not altered by selective logging (Figure 2B), despite some evidence that flocking species decline in abundance with habitat change (Stouffer et al., 2006; Sigel et al., 2010). Further, the structure and composition of mixed-species bird flocks is sensitive to forest modification; flock richness (number of species) and size (number of individuals) declines with forest fragmentation and degradation (Lee et al., 2005; Cordeiro et al., 2015). Recent evidence also points to the breakdown of interspecific associations in mixed flocks, and therefore, the reduced stability of mixed-species flocks in altered forest (Mokross et al., 2014; Mammides et al., 2015). Predictable changes in flock structure and composition in logged forest, including on the plots I sampled (Borah et al., 2018) are likely a response to altered resource availability and predation risk (Ewers et al., 2015; Hua and Sieving, 2016).
Taken together, unchanged survival but changing flock structure indicate that flocking species possess a degree of behavioral flexibility, allowing them to alter the strength and nature of interspecific associations to maintain survival in the face of modified resources and predation in altered forest. Therefore, participation in mixed flocks might be a trait that reduces, rather than enhances, vulnerability to selective logging, and changes in the structure and composition of mixed flocks in logged forest (Borah et al., 2018) need not necessarily mean that flocking species are demographically particularly vulnerable to logging. With fragmentation, however, isolation might lead to the outright loss of species important for flock cohesion in forest fragments, leading to severely disrupted flock networks (Mokross et al., 2014), and declining survival of species regardless of flocking behavior (Korfanta et al., 2012). Non-flocking species, lacking the ability to forage with other species (and therefore potentially alter interspecific associations to adapt to habitat change) might be behaviorally disadvantaged to deal with increased predation and resource scarcity in logged forest, resulting in depressed survival with habitat change (Figure 2B).
Elevational Migration and Survival
The time-allocation hypothesis proposes that survivorship should increase with time spent on climatically favorable wintering grounds (Greenberg, 1980); this would predict that elevational migrants should have higher survival rates than residents, because year-round residents are likely to face harsher winters. However, the survival of resident and migrant species did not differ in either intact or in logged forest (Figure 2C), contradicting the time-allocation hypothesis. Although elevational migration might serve to maximize year-round constancy in the climatic niche (Srinivasan et al., 2018), migration can also carry energetic and predation costs that offset benefits from enhanced resource availability in milder wintering grounds (Alerstam et al., 2003; Cooper et al., 2015; Rushing et al., 2017). At the same time, residents, despite being subject to food shortages in winter (Ghosh et al., 2011), might benefit from enhanced knowledge of resource and predators distribution on their territories (Sandercock and Jaramillo, 2002). Combined, these trade-offs might result in the lack of survival differences between residents and migrants in both intact and logged forest (Figure 2D).
Resident species had higher survival in intact than in logged forest, while the survival of elevational migrants did not differ with logging (Figure 2D). This pattern might point to the importance of winter habitat quality in seasonal environments in influencing annual survival. Migratory species breeding in logged forest can potentially search for, and winter in, optimal habitats; on the other hand, species resident in logged forest are likely to face resource shortages and enhanced predation year-round (Cooper et al., 2015; Ewers et al., 2015; Hua and Sieving, 2016). Differences in survival between residents and migrants are consistent with the predictions of the time-allocation hypothesis (Greenberg, 1980)—for resident insectivorous birds, winters in logged forest might be harsher than winters in intact forest, because of higher predation and reduced food resources (Ewers et al., 2015; Hua and Sieving, 2016). Characterizing summer and winter habitat quality for residents and migrants using telemetry-based approaches with concomitant habitat quality data would be essential to test this mechanism.
Conclusions
This study is one of the first to examine how species' traits influence demographic vital rates in anthropogenically modified forest. I identify attributes—, solitary foraging and year-round residency—that predispose eastern Himalayan bird species to vulnerability to selective logging, via reductions in annual survival. Existing long-term demographic data (e.g., Blake and Loiselle, 2001; Korfanta et al., 2012; Wolfe et al., 2014, 2015) should be analyzed to test whether these traits are correlated with reduced survival in fragmented and logged forest across the tropics. Given the importance of annual survival in determining the population persistence in disturbed habitats (Karr, 1990; Korfanta et al., 2012), identifying general relationships between species' traits and anthropogenically altered survival rates is crucial to both predict species responses to forest modification, and to better plan and manage biodiversity-friendly forest use.
Ethics Statement
This study was carried out in accordance with the recommendations of the Arunachal Pradesh Forest Department, Government of India.
Author Contributions
US designed the study, collected the data, conducted the analyses, and wrote the manuscript.
Conflict of Interest Statement
The author declares that the research was conducted in the absence of any commercial or financial relationships that could be construed as a potential conflict of interest.
Acknowledgments
I thank the Arunachal Pradesh Forest Department for permission to conduct this study, and the Shergaon Forest Division for their support of this work. I am especially grateful to I Glow, N Tsering, R Athreya, and RK Pradhan (and his team at Bongpu camp) for logistical support. In the field, S Rai, D Subba, B Tamang, DK Pradhan, M Rai, and V Rai provided exemplary assistance. I thank Z. Burivalova and E. Frank for many useful inputs. Funding for this work came from the High Meadows Foundation, Princeton University, the International Foundation for Science and the National Centre for Biological Sciences. I thank S Quader for discussions and support during the initial part of this work. I thank DS Wilcove for many stimulating discussions, for comments on this manuscript, and for supporting this work. Nandini Velho supported this work in many large and small ways over the past 8 years.
Supplementary Material
The Supplementary Material for this article can be found online at: https://www.frontiersin.org/articles/10.3389/fevo.2019.00017/full#supplementary-material
References
Alerstam, T., Hedenstrom, A., and Akesson, S. (2003). Long-distance migration: evolution and determinants. Oecologia 103, 247–260. doi: 10.1034/j.1600-0706.2003.12559.x
Bakermans, M. H., Vitz, A. C., Rodewald, A. D., and Rengifo, C. G. (2009). Migratory songbird use of the Venezuelan Andes with implications for the conservation of cerulean warbler. Biol. Conserv. 142, 2476–2483. doi: 10.1016/j.biocon.2009.05.018
Blake, J. G., and Loiselle, B. A. (2001). Bird assemblages in secondary and old growth forests, Costa Rica: perspectives from mist nets and points counts. Auk 118, 304–326. doi: 10.1642/0004-8038(2001)118[0304:BAISGA]2.0.CO;2
Blake, J. G., and Loiselle, B. A. (2008). Estimates of apparent survival rates for forest birds in eastern Ecuador. Biotropica 40, 485–493. doi: 10.1111/j.1744-7429.2007.00395.x
Borah, B., Quader, S., and Srinivasan, U. (2018). Responses of interspecific associations in mixed-species bird flocks to selective logging. J. Appl. Ecol. 55, 1637–1646. doi: 10.1111/1365-2664.13097
Brawn, J. D., Karr, J. R., and Nichols, J. D. (1995). Demography of birds in a neotropical forest: effects of allometry, taxonomy and ecology. Ecology 76, 41–51. doi: 10.2307/1940630
Burivalova, Z., Lee, T. M., Giam, X., Sekercioglu, C. H., Wilcove, D. S., and Koh, L. P. (2015). Avian responses to selective logging shaped by species traits and logging practices. Proc. R. Soc. 282:20150164. doi: 10.1098/rspb.2015.0164
Champion, H. G., and Seth, S. K. (1968). A Revised Survey of the Forest Types of India. New Delhi: Manager of Publications, Government of India.
Cooper, N., Sherry, T. W., and Marra, P. P. (2015). Experimental reduction of winter food decreases body condition and delays migration in a long-distance migratory bird. Ecology 96, 1933–1942. doi: 10.1890/14-1365.1
Cordeiro, N. J., Borghesio, L., Joho, M. P., Monoski, T. J., Mkongewa, V. J., and Dampf, C. J. (2015). Forest fragmentation in an African biodiversity hotspot impacts mixed species bird flocks. Biol. Conserv. 188, 61–71. doi: 10.1016/j.biocon.2014.09.050
Cormack, R. M. (1964). Estimates of survival from the sighting of marked animals. Biometrika 51, 429–438. doi: 10.1093/biomet/51.3-4.429
Cossett, C. C. P., Gilroy, J. J., and Edwards, D. P. (2019). The impacts of tropical forest disturbance on species vital rates. Conserv. Biol. 33, 66–75. doi: 10.1111/cobi.13182
Cruz-Angon, A., Sillett, T. S., and Greenberg, R. (2008). An experimental study of habitat selection by birds in a coffee plantation. Ecology 89, 921–927. doi: 10.1890/07-0164.1
Doherty, P. F., and Grubb, T. C. (2002). Survivorship of permanent-resident birds in a fragmented forest landscape. Ecology 83, 844–857. doi: 10.1890/0012-9658(2002)083[0844:SOPRBI]2.0.CO;2
Edwards, D. P., and Laurance, W. F. (2013). Biodiversity despite selective logging. Science 339, 646–647. doi: 10.1126/science.339.6120.646-b
Ewers, R. M., Boyle, M. J., Gleave, R. A., Plowman, N. S., Benedick, S., Bernard, H., et al. (2015). Logging cuts the functional importance of invertebrates in tropical rainforest. Nat. Commun. 6, 6836-1–6836-7. doi: 10.1038/ncomms7836
Ghalambor, C. K., and Martin, T. E. (2001). Fecundity-survival trade-offs and parental risk-taking in birds. Science 292, 494–497. doi: 10.1126/science.1059379
Ghosh, M., Singh, P., and Mohan, D. (2011). Seasonal variation in foraging ecology of three species of overwintering leaf warblers (genus Phylloscopus) in the Himalayan foothills. J. Ornithol. 152, 869–877. doi: 10.1007/s10336-011-0670-9
Gibson, L., Lee, T. M., Koh, L. P., Brook, B. W., Gardner, T. A., Barlow, J., et al. (2011). Primary forests are irreplaceable for sustaining tropical biodiversity. Nature 478:378. doi: 10.1038/nature10425
Gimenez, O., Lebreton, J.-D., Choquet, R., and Pradel, R. (2017). R2ucare: Goodness-of-Fit Testing for Capture-Recapture Models. R package version 1.0.0. Available online at: https://CRAN.R-project.org/package=R2ucare
Greenberg, R. (1980). “Demographic aspects of long-distance migration,” in Migrant Birds in the Neotropics: Ecology, Behavior, Distribution and Conservation (Washington, DC: Smithsonian Institution Press), 493–504.
Hamer, K. C., Newton, R. J., Edwards, F. A., Benedick, S., Bottrell, S. H., and Edwards, D. P. (2015). Impacts of selective logging in Borneo: the importance of trophic position, body size and foraging height. Biol. Conserv. 188, 82–88. doi: 10.1016/j.biocon.2014.09.026
Hua, F., and Sieving, K. E. (2016). Understorey avifauna exhibits altered mobbing behaviour in tropical forest modified by selective logging. Oecologia 182, 743–754. doi: 10.1007/s00442-016-3695-1
Jetz, W., Thomas, G. H., Joy, J. B., Hartmann, K., and Mooers, A. O. (2012). The global diversity of birds in space and time. Nature 491, 444–448. doi: 10.1038/nature11631
Jolly, G. M. (1965). Explicit estimates from capture-recapture data with both death and immigration-stochastic model. Biometrika 52, 225–247. doi: 10.1093/biomet/52.1-2.225
Jullien, M., and Clobert, J. (2000). The survival value of flocking in neotropical birds: reality or fiction? Ecology 81, 3416–3430. doi: 10.1890/0012-9658(2000)081[3416:TSVOFI]2.0.CO;2
Karr, J. R. (1990). Avian survival rates and the extinction process on Barro Colorado Island. Conserv. Biol. 4, 391–397. doi: 10.1111/j.1523-1739.1990.tb00313.x
Korfanta, N. M., Newmark, W. D., and Kauffman, M. J. (2012). Long-term demographic consequences of habitat fragmentation to a tropical understorey bird community. Ecology 93, 2548–2559. doi: 10.1890/11-1345.1
Laurance, W. F., Camargo, J. L. C., Fearnside, P. M., Lovejoy, T. E., Williamson, G. B., Mesquita, R. C. G., et al. (2016). “An Amazonian forest and its fragments as a laboratory of global change,” in Interactions Between Biosphere, Atmosphere and Human Land Use in the Amazon Basin, eds L. Nagy, B. R. Forsberg, and P. Artaxo, (Berlin; Heidelberg: Springer), 407–440. doi: 10.1007/978-3-662-49902-3_17
Lee, T.-M., Soh, M. C. K., Sodhi, N., Koh, L. P., and Lim, S. L.-H. (2005). Effects of habitat disturbance on mixed species bird flocks in a tropical sub-montane rainforest. Biol. Conserv. 122, 193–204. doi: 10.1016/j.biocon.2004.07.005
Mammides, C., Chen, J., Goodale, U. M., Kotagama, S. W., and Goodale, E. (2015). Does mixed-species flocking influence how birds respond to a gradient of land use intensity? Proc. R. Soc. B. 282:20151118. doi: 10.1098/rspb.2015.1118
Mokross, K., Ryder, T. B., Cortes, M. C., Wolfe, J. D., and Stouffer, P. C. (2014). Decay of avian flock networks along a disturbance gradient in Amazonia. Proc. R. Soc. B. 281:20132599. doi: 10.1098/rspb.2013.2599
Powell, L., Cordeiro, N. J., and Stratford, J. A. (2015). Ecology and conservation of avian insectivores of the rainforest understorey: a pantropical perspective. Biol. Conserv. 188, 1–10. doi: 10.1016/j.biocon.2015.03.025
Proctor, J., and Haridasan, K. (1998). How far north does lowland evergreen tropical rainforest go? Global Ecol. Biogeogr. Lett. 7, 141–146. doi: 10.2307/2997817
R Core Team (2018). R: a Language and Environment for Statistical Computing. Vienna: R Foundation for Statistical Computing.
Rasmussen, P. C., and Anderton, J. C. (2012). Birds of South Asia: The Ripley Guide. Barcelona: Lynx Edicions.
Ruiz-Gutierrez, V., Doherty, P. F., Santana, E. C., Martinez, S. C., Schondube, J., Munguia, H. V., et al. (2012). Survival of resident neotropical birds: considerations for sampling and analysis based on 20 years of bird-banding efforts in Mexico. Auk 129, 500–509. doi: 10.1525/auk.2012.11171
Rushing, C. S., Hostetler, J. A., Sillett, T. S., Marra, P. P., Rotenberg, J. A., and Ryder, T. B. (2017). Spatial and temporal drivers of avian population dynamics across the annual cycle. Ecology 98, 2837–2850. doi: 10.1002/ecy.1967
Sandercock, B. K., and Jaramillo, A. (2002). Annual survival rates of wintering sparrows: assessing demographic consequences of migration. Auk 119, 149–165. doi: 10.1642/0004-8038(2002)119[0149:ASROWS]2.0.CO;2
Santos, E. S. A., and Nakagawa, S. (2012). The costs of parental care: a meta-analysis of the trade-off between parental effort and survival in birds. J. Evol. Biol. 25, 1911–1917. doi: 10.1111/j.1420-9101.2012.02569.x
Seber, G. A. F. (1965). A note on the multiple recapture census. Biometrika 52, 249–259. doi: 10.1093/biomet/52.1-2.249
Sigel, B. J., Robinson, W. D., and Sherry, T. W. (2010). Comparing bird community responses to forest fragmentation in two lowland central American reserves. Biol. Conserv. 143, 340–350. doi: 10.1016/j.biocon.2009.10.020
Sridhar, H., Beauchamp, G., and Shanker, K. (2009). Why do birds participate in mixed-species flocks? A large-scale synthesis. Anim. Behav. 78, 337–347. doi: 10.1016/j.anbehav.2009.05.008
Srinivasan, U. (2013). A slippery slope: logging alters mass-abundance scaling in ecological communities. J. Appl. Ecol. 50, 920–928. doi: 10.1111/1365-2664.12123
Srinivasan, U., Elsen, P. E., Tingley, M. W., and Wilcove, D. W. (2018). Temperature and competition interact to structure Himalayan bird communities. Proc. R. Soc. B. 284:20172593. doi: 10.1098/rspb.2017.2593
Srinivasan, U., Hines, J. E., and Quader, S. (2015). Demographic superiority with increased logging in tropical understorey insectivorous birds. J. Appl. Ecol. 52, 1374–1380. doi: 10.1111/1365-2664.12475
Stouffer, P. C., Bierregaard, R. O., Strong, C., and Lovejoy, T. E. (2006). Long-term landscape change and bird abundance in Amazonian rainforest fragments. Conserv. Biol. 20, 1212–1223. doi: 10.1111/j.1523-1739.2006.00427.x
Wolfe, J. D., Ralph, C. J., and Elizondo, P. (2015). Changes in the apparent survival of a tropical bird in response to the El Nino Southern Oscillation in mature and young forest in Costa Rica. Oecologia 178, 715–721. doi: 10.1007/s00442-015-3256-z
Wolfe, J. D., Stouffer, P. C., and Seeholzer, G. F. (2014). Variation in tropical bird survival across longitude and guilds: a case study from the Amazon. Oikos 123, 964–970. doi: 10.1111/oik.00849
Keywords: apparent survival, body mass, eastern Himalayas, mark-recapture, mixed-species flocks, understorey birds, vital rates
Citation: Srinivasan U (2019) Morphological and Behavioral Correlates of Long-Term Bird Survival in Selectively Logged Forest. Front. Ecol. Evol. 7:17. doi: 10.3389/fevo.2019.00017
Received: 26 July 2018; Accepted: 21 January 2019;
Published: 08 February 2019.
Edited by:
Laurentiu Rozylowicz, University of Bucharest, RomaniaReviewed by:
Tibor Hartel, BabeÈ-Bolyai University, RomaniaAttila D. Sándor, University of Agricultural Sciences and Veterinary Medicine of Cluj-Napoca, Romania
Jessica Marie Gorzo, University of Minnesota Duluth, United States
Copyright © 2019 Srinivasan. This is an open-access article distributed under the terms of the Creative Commons Attribution License (CC BY). The use, distribution or reproduction in other forums is permitted, provided the original author(s) and the copyright owner(s) are credited and that the original publication in this journal is cited, in accordance with accepted academic practice. No use, distribution or reproduction is permitted which does not comply with these terms.
*Correspondence: Umesh Srinivasan, umesh.srinivasan@gmail.com