- 1Department of Zoology and Physiology, University of Wyoming, Laramie, WY, United States
- 2Conservation Department, Ol Pejeta Conservancy, Nanyuki, Kenya
- 3Puget Sound Institute, University of Washington, Tacoma, WA, United States
- 4Agricultural Research Service Rangeland Resource Unit, US Department of Agriculture, Fort Collins, CO, United States
- 5Department of Ecology and Evolutionary Biology, Princeton University, Princeton, NJ, United States
Predator restorations often result in apparent competition, where co-occurring prey populations experience asymmetric predation pressure driven by predator preferences. In many rangeland ecosystems, livestock share the landscape with wildlife, including ungulates and the large carnivores that consume them. We examined whether apparent competition reorganized prey communities following restoration of lions (Panthera leo) to a savanna ecosystem, and whether and how livestock management could alter this indirect interaction between lions and their prey. Three lines of evidence supported the hypothesis that Jackson's hartebeest (Alcelaphus bucelaphus lelwel; an ungulate of conservation concern) are suppressed via lion-mediated apparent competition. First, hartebeest exhibited an Allee effect where they were exposed to lions, but displayed negative density-dependent population growth where they were protected from lions. Second, spatial overlap between plains zebra (Equus burchelli; the primary prey of lions) and hartebeest further exacerbated lion predation on hartebeest. Finally, hartebeest were killed selectively by lions, whereas zebra were killed by lions in proportion to their abundance. We then tested whether glades [nutrient-rich hotspots created by abandoned cattle (Bos indicus) corrals] could be used to manipulate top-down control of hartebeest via their influence on the spatial distribution of zebra. Zebra aggregated at glades, and survival of hartebeest increased with increasing distance from glades, suggesting that corrals may be placed on the landscape away from hartebeest to create spatial refuges from lions. Our findings demonstrate how informed placement of livestock corrals can be used to manipulate the spatial distribution of primary prey (zebra), thereby reducing apparent competition suffered by hartebeest. Our work further provides an example of how integrating apparent competition theory with proactive livestock management can improve conservation efforts in multiple-use landscapes.
Introduction
The restoration of large carnivores can infuse optimism into conservation efforts that, historically, have been characterized by reactive, stopgap measures (Chapron et al., 2014; Wolf and Ripple, 2018). In the aftermath of such predator restoration, however, prey species often exhibit marked changes in numbers and behavior, such that composition and relative abundance of post-restoration prey communities may bear only slight resemblance to that of communities prior to predator extirpation (Lovari et al., 2009; DeCesare et al., 2010). Differences in carnivore-extirpated vs. carnivore-restored communities of prey often are attributed to apparent competition, in which preferences for prey by large carnivores lead to differences in the degree to which prey—some which are themselves conservation concerns—are suppressed via top-down control (Holt, 1977; Holt and Kotler, 1987; see DeCesare et al., 2010 for a review of cases in which apparent competition presents challenges for conservation; Holt and Bonsall, 2017).
Regardless of the mechanism by which they arise, prey preferences often result in secondary (less common) prey incurring greater risk of predation in proximity to primary prey. Secondary prey may decline while primary prey subsidize large carnivores, such that abundances of large carnivores and secondary prey are decoupled, driving the latter to rarity or even local extinction (e.g., Schmidt, 2004; Angulo et al., 2007; Hervieux et al., 2014; Serrouya et al., 2015; Chan et al., 2017). The likelihood that apparent competition generates these predator-mediated Allee effects—the decrease in population growth at small population sizes, (Allee et al., 1949; Courchamp et al., 1999)—is greatest when large carnivores are restored after lengthy periods of extirpation, during which environmental conditions have changed, and prey abundance has increased (but see Berger et al., 2001; Ford and Goheen, 2015; Stier et al., 2016). Against the backdrop of shifted environmental conditions, effects of large carnivores can be stronger than expected, presenting a conservation challenge to species of secondary prey.
The factors that drive prey preferences—and thus predispose secondary prey to high rates of predation—include differential resilience of primary vs. secondary prey to predation, and differential space use between primary and secondary prey (DeCesare et al., 2010; Wittmer et al., 2013). This second feature of predator-prey dynamics can result in “gradients of consumption” (in which risk of predation varies monotonically and directionally; Orrock et al., 2008), creating refuges where encounters between large carnivores and their secondary prey are infrequent. In giving secondary prey a potential foothold for positive population growth, refuges are one of the few ways shown to negate predation-mediated Allee effects in nature, thereby providing a potential tool to ameliorate apparent competition (Sinclair et al., 1998).
African savannas hold promise and conservation importance for understanding how spatial refugia might be used to reduce apparent competition stemming from predator restoration. Almost invariably, communities of savanna ungulates are dominated by a single species that typically constitutes the primary prey for large carnivores, yet achieves sufficiently high abundances to escape top-down control. In contrast, populations of less abundant species (i.e., secondary prey) tend to be suppressed by large carnivores, thus creating strong potential for apparent competition (e.g., Sinclair, 1985; Harrington et al., 1999; Owen-Smith and Mason, 2005; Georgiadis et al., 2007a; Chirima et al., 2012). Ranching occurs alongside wildlife in many African savannas, and landscapes in these human-occupied systems bear the imprint of livestock production in the form of glades: nutrient hotspots that attract wild ungulates and are derived from abandoned corrals or “bomas” (Augustine and McNaughton, 2006; Porensky and Veblen, 2015). Because livelihoods based purely on livestock production are becoming less reliably profitable, a changing mindset—to balance pastoralism with tourism, and potentially wildlife conservation—is gaining traction in many areas (Prins et al., 2000; Odadi et al., 2011; Keesing et al., 2018). Viewing large predators consistently ranks as a top priority among tourists, potentially leading to financial benefits in ecotourism ventures (Lindsey et al., 2007). Restoring large predators along with diverse assemblages of wild ungulates may be key to economic viability in these regions (Cousins et al., 2008; Stein et al., 2010).
The goals of our study were 2-fold. First, we sought to test the hypothesis of apparent competition (Figure 1) in Laikipia County, Kenya. Here, multiple species of wild ungulates—most notably Jackson's hartebeest (Alcelaphus buselaphus lelwel; hereafter simply “hartebeest”)—have experienced recent declines following lion (Panthera leo) restoration in the late 1980s (Georgiadis et al., 2007b; Ng'weno et al., 2017). Restoration of lions has resulted from greater tolerance by ranch managers following decades of control via shooting and poisoning; however, declines in hartebeest populations are increasingly viewed as a particular conservation concern, leading some ranch managers to consider re-implementing lethal control of lions (Georgiadis et al., 2007b J. R. Goheen pers comm with Laikipia ranchers). Indeed, the impact of lion predation is sufficient to shift population growth of hartebeest from positive to negative (Ng'weno et al., 2017). In contrast to hartebeest and other declining species, populations of plains zebra (Equus quagga; hereafter simply “zebra”)—the primary prey for lions in Laikipia—fluctuate in response to rainfall and density (Georgiadis et al., 2007a), but have not decreased with recovering lion numbers (Georgiadis, 2011; O'Brien et al., 2018). Consequently, we hypothesized that zebra populations suppressed those of hartebeest via apparent competition.
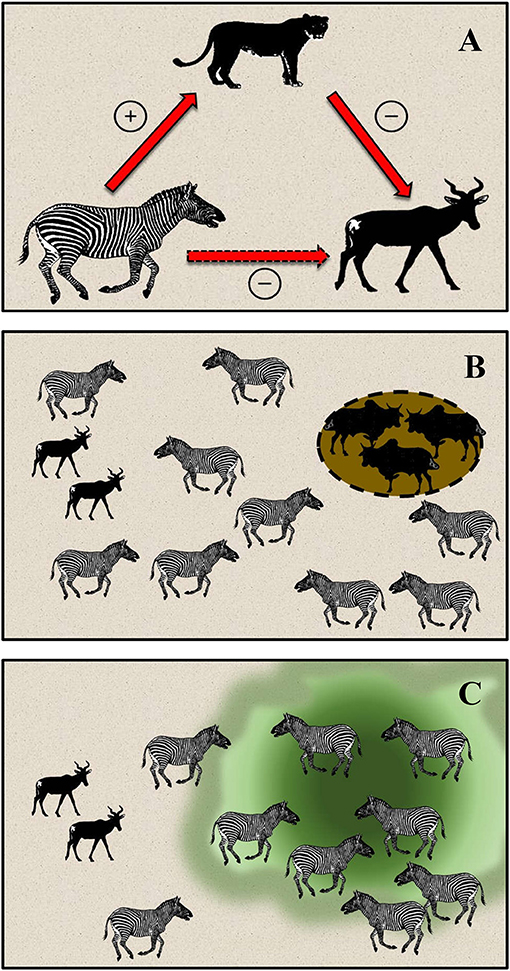
Figure 1. Hypothesized species interactions among lions, plains zebra, Jackson's hartebeest, and cattle in Laikipia, Kenya. (A) The hypothesis of apparent competition. Zebra populations are controlled bottom-up by rainfall, are the primary prey of lions, and support a growing population of lions since the cessation of lethal control in the late-1980s. Although they are less abundant than zebra, hartebeest are selectively killed by lions and are controlled top-down, such that hartebeest incur negative, indirect impacts from zebra via lions. (B) Cattle are corralled overnight in bomas where they deposit dung and urine for a period of ca. 1 month. (C) After abandonment, dung and urine break down over the course of 2–3 years, creating nutrient-rich forage in glades where zebra aggregate, but not hartebeest. This creates spatial separation between zebra and hartebeest, and potentially a refuge from predation if lions target hunting activity toward areas where zebra are abundant.
After assessing the hypothesis of apparent competition, we then tested whether livestock management could be used to manipulate the spatial distribution of predation risk to hartebeest, potentially enhancing coexistence of lions and their prey (and potentially removing a reason to lethally control lions). Under the hypothesis of apparent competition, we expected hartebeest in proximity to zebra to incur higher rates of predation. Because zebra (but not hartebeest) are attracted to glades (Veblen and Young, 2010; Augustine et al., 2011), we sought to quantify whether spatial separation between primary (zebra) and secondary (hartebeest) prey, driven by glade location, moderated apparent competition between zebra and hartebeest. Specifically, we predicted that (1) hartebeest occurring in areas of high zebra density would incur greater risk of predation from lions; and (2) hartebeest survival would increase with increasing distance from glades. In the event that predation risk to hartebeest could be manipulated via spatial separation of hartebeest and zebra, this would provide a potential solution through which hartebeest numbers could be bolstered without lethal control of lions.
Materials and Methods
Study Area
We conducted fieldwork in Laikipia County, Kenya, at Ol Pejeta Conservancy (N0° 00′-S0° 02′; E36° 44′-36° 59′). Ol Pejeta is a 364-km2 savanna managed jointly for wildlife conservation and Boran cattle (Bos indicus). With annual rainfall of 900 mm (Wahungu et al., 2011), Ol Pejeta is characterized by a wooded grassland dominated by the whistling-thorn tree, Acacia drepanolobium. The understory is dominated by the grasses Themeda triandra, Pennisetum straminiem, P. mezianum and Brachiaria lachnatha. Zebra are the most common wild ungulate at Ol Pejeta (individuals/km2 = 11.55 ± 1.22 SEM); other wild ungulates consumed by lions include buffalo (Syncerus cafer), eland (Taurotragus oryx), giraffe (Giraffa camelopardalis), Grant's gazelle (Nanger granti), hartebeest, impala (Aepyceros melampus), oryx (Oryx gazella), Thomson's gazelle (Eudorcas thomsonii), warthog (Phacochoerus africanus), and waterbuck (Kobus ellipsiprymnus). In addition to lions, large (>10 kg) carnivores include black-backed jackal (Canis mesomelas), cheetah (Acinonyx jubatus), leopard (Panthera pardus), and spotted hyena (Crocuta crocuta).
Estimation of Hartebeest Abundance and Population Growth
Between 2009 and 2015, we estimated hartebeest population growth and size within two zones under different management regimes: (1) a 294-km2 area in which cattle production occurred alongside wildlife with the full complement of large carnivores (hereafter “control”); and (2) a 32-km2 lion exclusion zone (hereafter “lion exclusion”), constructed with the intent of boosting numbers of declining ungulates, primarily hartebeest. The exclusion zone was demarcated from the adjacent control zone by a 2.5-m tall solar powered electrified (6,000–7,000-volt) fence with nine strands spaced 0.2 m apart. The fence was fortified with chain-link 1.50 m above and 0.60 m beneath the ground, preventing lion incursion but remaining permeable to other large carnivores (Ng'weno et al., 2017). Stocking rates were maintained at equal densities of 20 cattle /km2 in both zones. From 2009 to 2015, we conducted twice-monthly drive transects for hartebeest in both zones. During each sampling period, we systematically drove 17 (4–13 km) transects (12 in the control zone, 5 in the lion exclusion zone), based on a predetermined random starting point. We conducted surveys during 07:00–11:00 h, driving at a maximum speed of 10–15 km/h with two trained observers. At each hartebeest sighting, we recorded the group size and the distance and compass bearing to the group with binoculars and laser rangefinders following standard distance sampling methods (Buckland et al., 2015).
Hartebeest maintain small groups (Kingdon et al., 2013; x = 16 in this study), characterized by strong dominance hierarchies among females. Groups are relative sedentary and defend small (<5 km2) territories against conspecifics; as a result, we were able to identify and track 179 unique individuals in 11 distinct groups over the latter 4 years of our study (2012–2015; see “Hartebeest survivorship and refugia analysis” below; Ng'weno et al., 2017).
Typically, apparent competition is characterized by one or more species of secondary prey that exhibit an Allee effect (Allee et al., 1949; Courchamp et al., 1999; Stephens et al., 1999). To test for an Allee effect, we calculated the instantaneous rates of increase (r) for each zone (control and lion exclusion) as , where N is the estimate of population size from the ith survey at time t (Sinclair et al., 1998). For hartebeest populations in both zones, we related population size to r, and determined goodness-of-fit of curves from regression analysis (Zar, 2010).
Prey Selectivity of Lions
From 2012 to 2015, we used distance-sampling methods to conduct quarterly drive transects to estimate the abundance of 11 species of ungulates killed and consumed by lions (hereafter “lion prey”). We systematically drove the 12 transects in the control zone while two observers recorded species, group size, sighting distance, and bearing to all lion prey sighted along transects. To evaluate whether lions killed prey in proportion to their availability, we fit 5 female lions with Global Positioning System (GPS) collars (Vectronic Aerospace GmbH, Berlin, Germany) from March to May 2014. These lions belonged to 5 different prides, which collectively accounted for ca. 80% of lions on Ol Pejeta (Ng'weno et al.; Ng'weno, 2017 in revision). Overlap between home ranges of prides was minimal (1–12%) throughout the course of our study (Ng'weno et al. in revision). All procedures were conducted with a veterinary team under the authority of the Kenya Wildlife Service.
From March 2014 to December 2015, we located lion kills from clustered locations of lions with GPS collars using an algorithm adapted from Knopff et al. (2009). Between August 2014 and December 2015, there were 246 instances in which prey carcasses were found to have been killed by lions (Ng'weno et al.; Ng'weno, 2017 in revision). We then used Jacobs' index (Jacobs, 1974; Hayward and Kerley, 2005) to quantify seasonal selectivity for each of 11 species of lion prey, where r is the proportion of a given species among all kills, and p is its proportional abundance in the total prey population. Jacobs's index is bounded between −1 (highly avoided) and 1 (highly selected). Selectivity indices were calculated for each of the 11 species of lion prey, using carcasses from GPS clusters and estimates of prey abundance collected from August 2014 to December 2015.
Zebra Density and Risk of Predation to Hartebeest
To quantify the impact of primary prey (zebra) density on lion predation of hartebeest, we generated spatially-explicit density surfaces for zebra using resource selection functions (RSF) in a use-availability design (Manly et al., 2002). We constructed a minimum convex polygon (MCP) around all zebra herd locations from the quarterly surveys, and paired these with an equal number of random locations (n = 2,450) to achieve a 1:1 ratio of used to available locations. We then used a Rapideye satellite image (Digital Globe, Longmont, Co, USA) from May 2013 with 5–m spatial resolution to perform an unsupervised classification through isoclustering and maximum likelihood to group pixels with similar spectral reflectance into identify three habitat types: dense bushland (characterized by >50% cover of the tree Euclea divinorium), open bushland (characterized by 10–30% cover of the tree A. drepanolobium), and open grassland (characterized by <5% tree cover) (Birkett, 2002; Goheen and Palmer, 2010; Ng'weno et al., 2017). Following assignment of pixels, we ground-truthed our classification using 50–100 points in each habitat type. We performed all image processing using ERDAS Imagine, version 14 (Hexagonal Geospatial, Madison, Alabama) and ArcGIS version 10.3 (ESRI, Redlands, California).
Using the Euclidian distance and spatial join tools in ArcGIS 10.3, we extracted distances to the nearest water source and the nearest glade for each used and available location in each survey-specific zebra MCP. We related locations of zebra herds to habitat type, distance to nearest water source, and distance to nearest glade in 30 × 30 m pixels. Collinearity between habitat variables (habitat type, distance to water, distance to glade) was minimal (r < 0.50; P > 0.20 for all possible pairwise combinations).
We used logistic regression to estimate RSF coefficients, with selection for or avoidance of a habitat variable indicated by coefficients >1.0 and < 1.0, respectively (Manly et al., 2002). We used the resultant coefficients to generate a zebra RSF, rescaled to create 16 continuous surfaces (one for each of the 16 quarterly surveys conducted during 2012–2015) with 30 × 30 m pixel values ranging between 0 (strongest avoidance) and 1 (strongest selection), divided into 5 bins of equal width following Morris et al. (2016). The first bin corresponded to the lowest probability of zebra selection (0.00–0.20) and the fifth bin corresponded to the highest probability of zebra selection (0.81–1.00). We combined survey-specific zebra densities with survey-specific RSFs to create spatially-explicit density estimates for zebra in each survey, which we validated independently using camera-trap grids (Appendix 1; Ng'weno et al.; Ng'weno, 2017 in revision). See Boyce et al. (2016) for a similar approach in using RSFs to estimate spatial variation in abundance.
Finally, we superimposed locations of hartebeest killed by lions (n = 27) obtained from GPS clusters to the spatially-explicit zebra density surface with which it was most closely associated in time. We used Chi-squared tests to assess differences in the proportion of hartebeest kills occurring within the highest (>9.60 zebra/km2) and lowest (<2.40 zebra/km2) areas of estimated zebra density.
Glade Creation Experiment and Hartebeest Survival
For the period 2012 to 2015, we calculated survival rates of individual hartebeest, with biological years starting 1 October and ending 31 September of each subsequent year (corresponding to birth peaks). Survival of female adults, sub-adults, and calves collectively accounts for >70% of the variation in population growth of hartebeest at Ol Pejeta (Ng'weno et al., 2017). During our twice-monthly sight-resight drive surveys (see “Estimation of hartebeest abundance and population growth” above), we monitored survival of 179 adults, sub-adults, and calves within 11 groups in the control zone. Because they defend small (<5 km2) territories, we were able to use unique marks (i.e., ear nicks, horn size, and shape, scars) to monitor survival through repeated surveys (Ng'weno et al., 2017). Because female hartebeest exhibit high site fidelity (Gosling, 1974), we equated disappearance with death. There were no instances in which an individual classified as “dead” during a particular survey was detected subsequently. Survival rates were calculated using the R package “survival” version 2.41-3 (Therneau, 2017).
Over the course of ~3 years, abandoned boma sites transition into glades and become attractive to zebra because of high biomass of Cynodon and Pennisetum grasses (Veblen and Young, 2010; Porensky, 2011; Veblen, 2012). Glades vary in their sizes and shapes; we therefore selected and restricted our analyses to 37 glades derived from bomas that we established in 2009 and 2010 (Figure 4). Nineteen of these bomas were established along the edges of open plains as part of an experiment to understand how glades give rise to various edge effects (Porensky and Veblen, 2015; Porensky and Young, 2016). Subsequently, 18 additional bomas were established in the middle of open plains to further quantify resource selection of zebra and survival of hartebeest. All bomas were 17.2 ± 0.8 m SEM in diameter, and used by ~200 cattle for 1 month.
To assess the influence of boma-derived glades on survival rates of hartebeest, we constructed 95% isopleths using fixed kernel density estimation to create utilization distributions (UD's), with least squares cross validation and a smoothing factor of 1,000 using R package “adehabitatHR” version 1.8.18 (Seaman and Powell, 1996; Powell, 2000; Calenge, 2006). We then overlaid glades onto hartebeest territories, and calculated the distance from the centroid of each sighting of each hartebeest group to the nearest glade.
To assess whether glades reduced hartebeest survival in accord with the hypothesis of apparent competition, we fit two Cox proportional hazards (PH) models (Cox, 1972) to a dataset on hartebeest mortality derived from two sources: the 27 kills that we detected from collared lion GPS clusters from May 2014 to December 2015, and 101 additional events in which individuals disappeared (and were equated with mortality events) from sight-resight surveys from January 2012 to May 2014. For every individual killed that was detected from GPS clusters, we noted absences through sight-resight surveys. No individuals disappeared in our sight-resight surveys between May 2014 and December 2015 that we did not independently discover through GPS kill-site clusters. In other words, 100% of the hartebeest in our study were killed by lions. Through the first PH model, we quantified how risk of mortality varied with the (categorical) presence of a glade within home ranges of hartebeest groups. Here, we assessed whether glade presence altered the hazard ratio (probability of death) of individual hartebeest over the course of 3 years. Because individuals belonged to one of 11 groups, and because groups were relatively sedentary over the course of our study, we incorporated frailties (random effects) associated with group identity (Fox et al., 2006; Goheen et al., 2010) using R package “frailtypack” version 2.12-3 (Rondeau et al., 2012). Finding random effects of group membership negligible (see “Results” below), we fit a second PH model in which distance to glade edge was used as a continuous covariate to explain hartebeest mortality (we did not attempt to combine frailties and continuous covariates within a single PH model, because both are challenging to integrate into PH models simultaneously; Lopez de Ullibarri et al., 2012). In calculating distances, we used the midpoint of the pair of sight-resight survey locations where an individual was last sighted with its group, and where it was first noted to have disappeared. All analyses were undertaken in R version 3.4.0 (R Development Core Team, 2017).
Results
Exclusion of lions was sufficient to shift population growth of hartebeest from negative to positive, consistent with a lion-mediated Allee effect (Figure 2). In the lion exclusion zone, population growth of hartebeest displayed a classic signal of negative density-dependence (Figure 2). In contrast, population growth of hartebeest increased with increasing population size in the control zone (Figure 2). Of the 11 species of ungulates killed by lions, zebra were consumed most frequently (40% of kills, n = 98) followed by warthog (15% of kills; n = 37), buffalo (14% of kills; n = 35), impala (14% of kills; n = 34), and hartebeest (13% of kills; n = 27). Lions consumed hartebeest and warthog disproportionately more than expected based on their abundance in both seasons, and zebra were consumed as frequently as expected based on their abundance in both seasons (Figure 3). The remaining 8 species of ungulates consumed by lions were avoided in at least one, and sometimes both, of the two seasons (Figure 3).
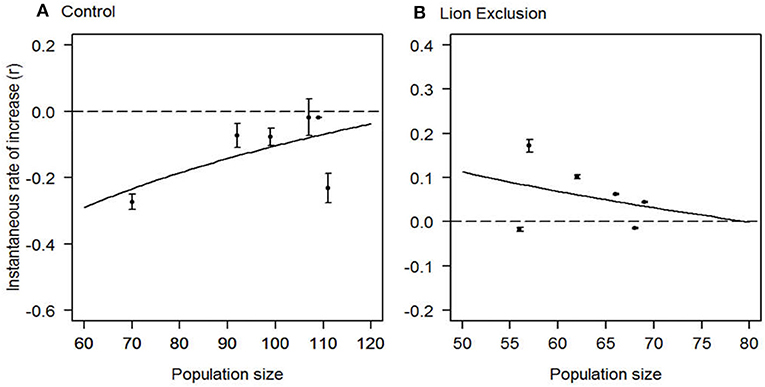
Figure 2. A lion-mediated Allee effect in hartebeest populations. Correlations between annual instantaneous rates of increase (r) for hartebeest populations in (A) control zones exposed to lion predation (r = 0.37 * ln(population size)−1.79); and (B) lion exclusion zones [r = −0.241 * ln(population size) + 1.06] from 2009 to 2015. Error bars are 95% confidence intervals based on sampling variance of population estimates.
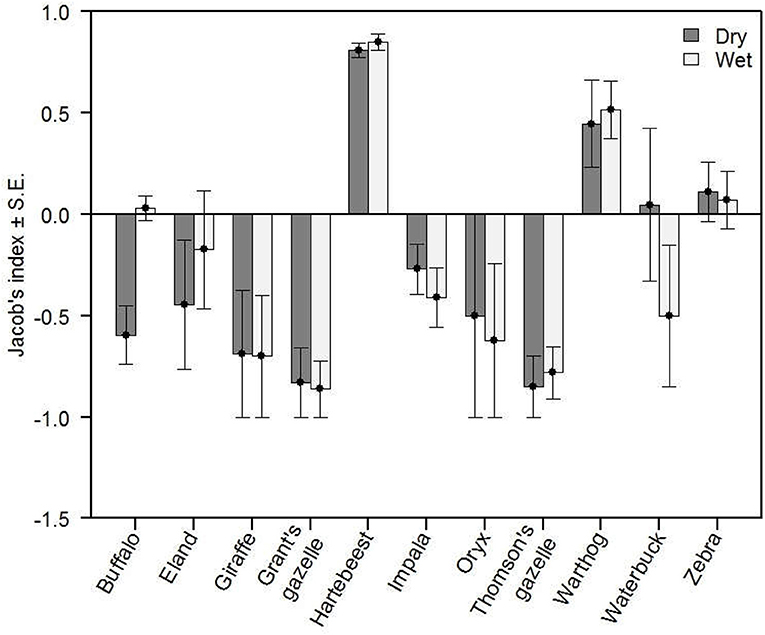
Figure 3. Selectivity indices (Jacobs' index of selection) for 11 species of ungulates by five lion prides during wet and dry seasons. Hartebeest (and warthog, to a lesser extent) are selected regardless of season, while zebra are killed in proportion to their abundance in both seasons. All other species of ungulates are avoided during at least one season. Error bars represent standard errors.
Zebra aggregated in and around glades (distance to glade: β = −2.30 ± 0.41 SEM, Z = −5.61, P < 0.0001), and the best supported RSF for zebra contained terms for distance to glade, distance to dense woodland, and distance to water (Supplemental Table 1). Hartebeest died more frequently than expected in areas of high zebra abundance ( = 42, P < 0.001; Figure 4). Consequently, and after nearly 3 years, survival among hartebeest lacking glades in their territories was over twice as high compared to those individuals whose territories contained glades (β = 2.35 ± 0.19 SEM, hazard ratio = 2.10, P < 0.001; Figure 5). Incorporating frailty terms to account for heterogeneity in survival among hartebeest groups did not significantly reduce PH model deviance ( = 11, P > 0.10). Using distance from the nearest glade's edge, we found evidence for spatial refugia around 600 m: with every 100 m closer to a glade, hartebeest incurred a roughly 15% greater chance of mortality (β = 0.14 ± 0.012 SEM, hazard ratio = 1.15, P < 0.001).
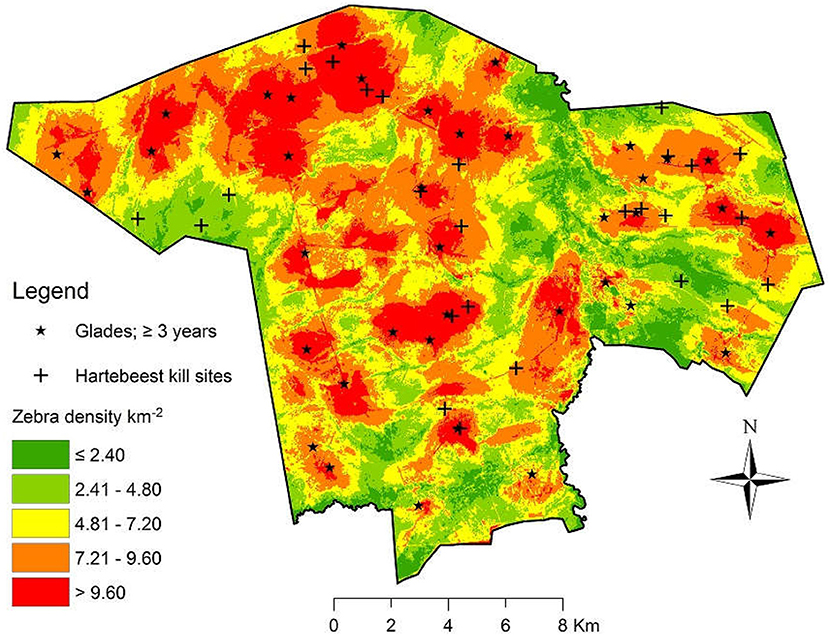
Figure 4. Map with the locations of glades (≥3 years of age, n = 37) and hartebeest kill sites (n = 27) superimposed on a density surface for plains zebra. Estimates of zebra density (individuals/km2) transitions from high (red) to low (green), and is driven largely by glade location.
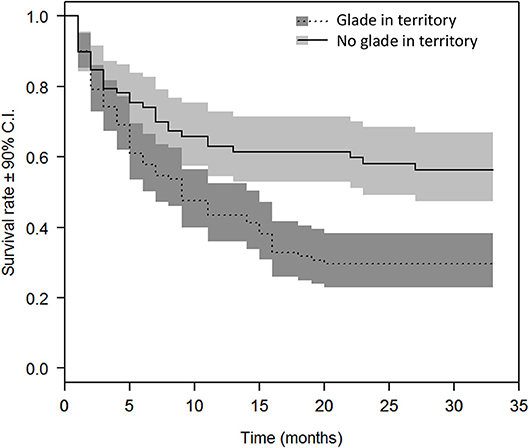
Figure 5. Survival rates and 90% confidence intervals from Cox proportional hazards models for hartebeest with territories containing vs. lacking glades. Survival for hartebeest with territories containing glades is roughly half that of those whose territories lack glades.
Discussion
Multiple lines of evidence suggest that, over the course of 7 years, hartebeest were limited by apparent competition triggered by the restoration of lions on Ol Pejeta Conservancy. Lion exclusion erased Allee effects, and resulted in negative density dependence in hartebeest at populations below around 80 (Figure 2). Lions consumed mostly zebra, and in proportion to their abundance, but selectively killed hartebeest (Figure 3). Zebra aggregated in and near glades, and mortality risk to hartebeest roughly doubled when glades occurred within hartebeest territories (Figure 5). Because ca. 80% of hartebeest mortality at Ol Pejeta is due to predation by lions (Ng'weno et al. in review), we attribute this change in patterns of mortality to a “gradient of consumption” (Orrock et al., 2008) that reduces survival of hartebeest in proximity to glades, and creates spatial refugia from lion predation beyond about 600 m from glades. Importantly, we did not study the mechanism underlying the Allee effect we observed, which reduces our ability to predict future hartebeest population trends at Ol Pejeta, or characterize the generality of our observations to other settings. Across vertebrates, Allee effects have been variously attributed to loss of genetic variability, reduced social facilitation, and difficulty finding mates. In our system, the effect appears linked to lion predation specifically: the higher preference of lions for hartebeest, or a reduction in shared vigilance in hartebeest herds. Lions may search for hartebeest groups, which tend to be in predictable places, and expend disproportionate effort to kill a hartebeest, once a group is detected.
Instances where apparent competition has been implicated in limiting ungulate populations are becoming numerous, including bighorn sheep (Ovis canadensis), mountain caribou (Rangifer tarandus), huemul (Hippocamelus bisulcus), and roan antelope (Hippotragus equinus; Harrington et al., 1999; DeCesare et al., 2010; McLellan et al., 2010; Wittmer et al., 2013; but see O'Brien et al., 2018 for an example contrary to the hypothesis of apparent competition involving Grevy's zebra [E. grevyi] in Laikipia). In our study system, spatial separation between zebra and hartebeest improved survival rates of hartebeest, probably by reducing encounters with lions hunting in areas with high zebra densities (Ng'weno et al.; Ng'weno, 2017 in revision; see also Palmer et al., 2003; Forrester and Steele, 2004). Strategic placement of glades therefore offers a promising approach to creating refuges for hartebeest and perhaps other species of secondary prey. By siting bomas away from hartebeest, the establishment of glades was used to move zebras (and presumably lions) away from hartebeest territories.
The creation of spatial refugia and the reduction of primary prey alongside predators have previously been effective in releasing secondary prey from apparent competition in the past (Sinclair et al., 1998; Wittmer et al., 2013). Only the former is logistically feasible in our study system. Lions are Red-listed as Vulnerable by the IUCN and are at least as high a conservation priority as Jackson's hartebeest. At first glance, elimination of glades through reduction of cattle production might be considered another option for hartebeest conservation, but this also is impractical for at least two reasons. First, local ranchers are unlikely to reduce cattle numbers voluntarily. Second, reducing cattle would likely boost zebra numbers, and potentially lion numbers, increasing top-down control of hartebeest. This is because the diets of cattle and zebra overlap (Kartzinel et al., 2015) and both are likely limited by rainfall (as they are in the wider Laikipia region; Georgiadis et al., 2003, 2007a) and they probably compete in dry times and places (Odadi et al., 2011). Moreover, predation by lions on cattle is rare relative to predation on because zebra (Ogada et al., 2004; O'Brien et al., 2018). Alternative conservation interventions are required for the long-term persistence of lions and their prey not only on Ol Pejeta Conservancy, but more widely in Laikipia County and the whole of sub-Saharan Africa (Bauer et al., 2015).
The extent to which our results generalize and can be extended to other locales depends on several factors: whether the affinity of zebra for glades (a behavioral response) translates to a numerical response (population increase); the length of time over which glades are attractive to zebra, and how the creation and eventual disappearance of glades gives rise to a dynamic landscape of risk to hartebeest (Kohl et al., 2018). In turn, predation for hartebeest varies across the landscape in accord with the density of glades, and the degree to which the attraction of zebra to glades changes with glade density. If increasing density of glades increases zebra and thus lion abundance, predation pressure on hartebeest could also increase. Or, if glades only attract zebra but do not result in population increase, increasing glade density could further disperse zebras across landscapes, negating the ability of glades to aggregate zebra.
We showed that spatial and temporal dynamics of predators and prey on Ol Pejeta Conservancy are consistent with apparent competition theory, and suggested a practical application for how lions and their secondary prey can coexist. Using strategic livestock management to manipulate the spatial distribution of predation pressure, we have provided a possible way to alleviate top-down control on an ungulate of conservation concern (Jackson's hartebeest) without resorting to lethal control of their most important predator (lions). Such solutions for conservation of lions in non-protected areas are needed to complement traditional funding efforts for formally-protected areas (Lindsey et al., 2018).
Author Contributions
CN, SB, NG, and JG conceptualized the research problem. CN and JG designed the study and performed the analyses. BG and AK implemented lion capture and assisted with analyses. LP implemented the boma experiment. DR collected and analyzed data on abundance and resource selection of plains zebra. CN, SB, and JG wrote the manuscript, with edits and input from all authors.
Funding
Funding was generously provided by Schlumberger Foundation Faculty for the Future, The World Wildlife Fund, the Biodiversity Institute at the University of Wyoming, Conservation Research and Education Opportunities International, the Haub School of Environment and Natural Resources at the University of Wyoming, the Denver Zoo, and the Earthwatch Institute. National Science Foundation (IBN-9874523, CNS-025214, IOB-9874523 and EAGER 1433428 & 1550881) to DR
Conflict of Interest Statement
The authors declare that the research was conducted in the absence of any commercial or financial relationships that could be construed as a potential conflict of interest.
Acknowledgments
We thank R. Montgomery, M. Hayward, and B. Kissui for their invitation to submit this manuscript. We thank Earthwatch volunteers (2013–2015) and undergraduate students from various universities in Kenya who collected data over the years. We are especially grateful to R. Warungu and others from the Princeton zebra project for collecting zebra data, and to N. Maiyo, D. Kamaru, and C. Bopp for expert field assistance. J. Beck, K. Gerow, and M. Kauffman provided edits on a previous version of this manuscript. The Ecological Monitoring Unit facilitated fieldwork and logistical support at Ol Pejeta Conservancy. Funding was generously provided by Schlumberger Foundation Faculty for the Future, The World Wildlife Fund, the Biodiversity Institute at the University of Wyoming, Conservation Research and Education Opportunities International, the Haub School of Environment and Natural Resources at the University of Wyoming, the Denver Zoo, and Earthwatch.
Supplementary Material
The Supplementary Material for this article can be found online at: https://www.frontiersin.org/articles/10.3389/fevo.2019.00123/full#supplementary-material
References
Allee, W. C., Park, O., Emerson, A. E., Part, T., and Schmidt, K. P. (1949). Principles of Animal Ecology. Philadelphia, PA: Saunders.
Angulo, E., Roemer, G., Berec, L., Gascoigne, J., and Courchamp, F. (2007). Double allee effects and extinction in the Island Fox. Cons. Biol. 21, 1082–1091. doi: 10.1111/j.1523-1739.2007.00721.x
Augustine, D., and McNaughton, S. (2006). Interactive effects of ungulate herbivores, soil fertility, and variable rainfall on ecosystem processes in a semi-arid savanna. Ecosystems 9, 1242–1256. doi: 10.1007/s10021-005-0020-y
Augustine, D. J., Veblen, K. E., Goheen, J. R., Riginos, C., and Young, T. P. (2011). “Pathways for Positive Cattle-Wildlife Interactions in Semi-Arid Rangelands”. in Conserving Wildlife in African Landscapes: Kenya's Ewaso Ecosystem ed. N. J Georgiadis, (Washington, DC: Smithsonian Institution Scholarly Press), 55–71.
Bauer, H., Chapron, G., Nowell, K., Henschel, P., Funston, P., Hunter, L. T. B., et al. (2015). Lion populations are declining rapidly across Africa, except in intensively managed areas. Proc. Nat. Acad. Sci. U.S.A. 112, 14894–14899. doi: 10.1073/pnas.1500664112
Berger, J., Swenson, J. E., and Persson, I. L. (2001). Recolonizing carnivores and naïve prey: conservation lessons from Pleistocene extinctions. Science 291, 1036–1039. doi: 10.1126/science.1056466
Birkett, A. (2002). The impact of giraffe, rhino and elephant on the habitat of a black rhino sanctuary in Kenya. Afr. J. Ecol. 40, 276–282. doi: 10.1046/j.1365-2028.2002.00373.x
Boyce, M. S., Johnson, C. J., Merrill, E. H., Nielsen, S. E., Solberg, E. J., and Van Moorter, B. (2016). Can habitat selection predict abundance? J. Anim. Ecol. 85, 11–20. doi: 10.1111/1365-2656.12359
Buckland, S. T., Rexstad, E., Marques, T. A., and Oedekoven, C. S. (2015). Distance Sampling: Methods and Applications. Heidelberg: Springer.
Calenge, C. (2006). “adehabitat”: R package for the analysis of space and habitat use by animals. Ecol. Model 197, 516–519. doi: 10.1016/j.ecolmodel.2006.03.017
Chan, K., Boutin, S., Hossie, T. J., Krebs, C. J., O'Donoghue, M., and Murray, D. L. (2017). Improving the assessment of predator functional responses by considering alternate prey and predator interactions. Ecology 98, 1787–1796. doi: 10.1002/ecy.1828
Chapron, G. (2014). Recovery of large carnivores in Europe's modern human dominated landscapes. Science 346, 1517–1519. doi: 10.1126/science.1257553
Chirima, G., Owen-Smith, N., Erasmus, B., and Parrini, F. (2012). Distributional niche of relatively rare sable antelope in a South African savanna: habitat versus biotic relationships. Ecography 36, 68–79. doi: 10.1111/j.1600-0587.2012.07333.x
Courchamp, F., Clutton-Brock, T., and Grenfell, B. (1999). Inverse density-dependence and the Allee effect. Trends Ecol. Evol. 14, 405–410. doi: 10.1016/S0169-5347(99)01683-3
Cousins, J. A., Sadler, J. P., and Evans, J. (2008). Exploring the role of private wildlife ranching as a conservation tool in South Africa: stakeholder perspectives. Ecol. Soc. 13:43. doi: 10.5751/ES-02655-130243
Cox, D. R. (1972). Regression models and life-tables. J. Roy. Stat. Soc. Series B 34, 187–220. doi: 10.1111/j.2517-6161.1972.tb00899.x
DeCesare, N., Hebblewhite, M., Robinson, H., and Musiani, M. (2010). Endangered, apparently: the role of apparent competition in endangered species conservation. Anim. Conserv. 13, 353–362. doi: 10.1111/j.1469-1795.2009.00328.x
Ford, A. T., and Goheen, J. R. (2015). Trophic cascades by large carnivores: a case for strong inference and mechanism. Trends Ecol. Evol. 30, 725–735. doi: 10.1016/j.tree.2015.09.012
Forrester, G. E., and Steele, M. A. (2004). Prey refuges, and the spatial scaling of density-dependent prey mortality. Ecology 85, 1332–1342. doi: 10.1890/03-0184
Fox, G. A., Kendall, B. E., Fitzpatrick, J. W., and Woolfenden, G. E. (2006). Consequences of heterogeneity in survival probability in a population of Florida scrub-jays. J. Anim. Ecol. 75, 921–927. doi: 10.1111/j.1365-2656.2006.01110.x
Georgiadis, N. J. (2011). Conserving Wildlife in African Landscapes: Kenya's Ewaso Ecosystem. Smithsonian Contributions to Zoology #632. Washington, DC: Smithsonian Institution Scholarly Press.
Georgiadis, N. J., Hack, M., and Turpin, K. (2003). The influence of rainfall on zebra population dynamics: implications for management. J. Appl. Ecol. 40, 125–136. doi: 10.1046/j.1365-2664.2003.00796.x
Georgiadis, N. J., Ihwagi, F., Olwero, J. G. N., and Romañach, S. S. (2007b). Savanna herbivore dynamics in a livestock-dominated landscape: II. ecological, conservation, and management implications of predator restoration. Biol. Conserv. 137, 473–483. doi: 10.1016/j.biocon.2007.03.006
Georgiadis, N. J., Olwero, J. G., Ojwang, G., and Romañach, S. S. (2007a). Savanna herbivore dynamics in a livestock-dominated landscape: I. dependence on land use, rainfall, density, and time. Biol. Conserv. 137, 461–472. doi: 10.1016/j.biocon.2007.03.005
Goheen, J. R., and Palmer, T. M. (2010). Defensive plant-ants stabilize megaherbivore-driven landscape change in an African savanna. Curr. Biol. 20, 1768–1772. doi: 10.1016/j.cub.2010.08.015
Goheen, J. R., Palmer, T. M., Keesing, F., and Young, T. P. (2010). Large herbivores facilitate savanna tree through diverse and indirect pathways. J. Anim. Ecol. 79, 372–382. doi: 10.1111/j.1365-2656.2009.01644.x
Gosling, L. M. (1974). “The social behaviour of Coke's hartebeest, Alcelaphus buselaphus cokei,” in The Behaviour of Ungulates and Its Relation to Management, eds V. Geist and F. Walther (IUCN Publications, New Series), 488–511.
Harrington, R., Owen-Smith, N., Viljoen, P., Biggs, H., Mason, D., and Funston, P. (1999). Establishing the causes of the roan antelope decline in the kruger national park, South Africa. Biol. Conserv. 90, 69–78. doi: 10.1016/S0006-3207(98)00120-7
Hayward, M. W., and Kerley, G. H. (2005). Prey preferences of the lion (Panthera leo). J. Zool. 267, 309–322. doi: 10.1017/S0952836905007508
Hervieux, D., Hebblewhite, M., Stepnisky, D., Bacon, M., and Boutin, S. (2014). Managing wolves (Canis lupus) to recover threatened woodland caribou (Rangifer tarandus caribou) in Alberta. Can. J. Zool. 92, 1029–1037. doi: 10.1139/cjz-2014-0142
Holt, R. D. (1977). Predation, apparent competition, and the structure of prey communities. Theor. Popul. Bio. 12, 197–229. doi: 10.1016/0040-5809(77)90042-9
Holt, R. D., and Bonsall, M. B. (2017). Apparent competition. Annu. Rev. Ecol. Evol. Syst. 48, 447–471. doi: 10.1146/annurev-ecolsys-110316-022628
Holt, R. D., and Kotler, B. P. (1987). Short-term apparent competition. Am. Nat. 130, 412–430. doi: 10.1086/284718
Jacobs, J. (1974). Quantitative measurement of food selection. Oecologia 14, 413–417. doi: 10.1007/BF00384581
Kartzinel, T. R., Chen, P. A., Coverdale, T. C., Erickson, D. L., Kress, J. W., Kuzmina, M. L., et al. (2015). DNA metabarcoding illuminates dietary niche partitioning by African large herbivores. Proc. Nat. Acad. Sci.U.S.A. 112, 8019–8024. doi: 10.1073/pnas.1503283112
Keesing, F, et al. (2018). Consequences of integrating livestock and wildlife in an African savanna. Nat. Sustain. 1, 566–573. doi: 10.1038/s41893-018-0149-2
Kingdon, J., Happold, D., Butynski, T., Hoffmann, M., Happold, M., and Kalina, J. (2013). Mammals of Africa. London: Bloomsbury.
Knopff, K. H., Knopff, A. A., Warren, M. B., and Boyce, M. S. (2009). Evaluating global positioning system telemetry techniques for estimating cougar predation parameters. J. Wildl. Manage. 73, 586–597. doi: 10.2193/2008-294
Kohl, M. T., Stahler, D. R., Metz, M. C., Forester, J. D., Kauffman, M. J., Varley, N., et al. (2018). Diel predator activity drives a dynamic landscape of fear. Ecol. Soc. Am. 88, 638–652. doi: 10.1002/ecm.1313
Lindsey, P. A., et al. (2018). More than $1 billion needed annually to secure Africa's protected areas with lions. Proc. Nat. Acad. Sci. U.S.A. 115, E10788–E10796. doi: 10.1073/pnas.1805048115
Lindsey, P. A., Roulet, P. A., and Romañach, S. S. (2007). Economic and conservation significance of the trophy hunting industry in sub-Saharan Africa. Biol. Conserv. 134, 455–469. doi: 10.1016/j.biocon.2006.09.005
Lopez de Ullibarri, I., Janssen, P., and Cao, R. (2012). Continuous covariate frailty models for censored and truncated clustered data. J. Stat. Plan. Inference 142, 1864–1877. doi: 10.1016/j.jspi.2012.02.044
Lovari, S., Boesi, R., Minder, I., Mucci, N., Randi, E., Dematteis, A., et al. (2009). Restoring a keystone predator may endanger a prey species in a human-altered ecosystem: the return of the snow leopard to sagarmatha national park. Anim. Conserv. 12, 559–570. doi: 10.1111/j.1469-1795.2009.00285.x
Manly, B. F. J., Mcdonald, L. L., Thomas, D. L., McDonald, T. L., and Wallace, W. P. (2002). Resource Selection by Animals. 2nd Edition. Dordrecht: Kluwer Academic Publishers.
McLellan, B. N., Serrouya, R., Wittmer, H. U., and Boutin, S. (2010). Predator-mediated Allee effects in multi-prey systems. Ecology 91, 286–292. doi: 10.1890/09-0286.1
Morris, L. R., Proffitt, K. M., and Blackburn, J. K. (2016). Mapping resource selection functions in wildlife studies: concerns and recommendations. Appl. Geogr. 76, 173–183. doi: 10.1016/j.apgeog.2016.09.025
Ng'weno, C. C. (2017). Predator-Prey Interactions and Apparent Competition Following the Restoration of Lions to a Human-Occupied Savanna. Ph.D. Dissertation, Laramie, WY: University of Wyoming.
Ng'weno, C. C., Ford, A. T., Kibungei, A., and Goheen, J. R. (In press). Interspecific prey neighborhoods shape risk of predation in a savanna ecosystem. Ecology doi: 10.1002/ecy.2698
Ngweno, C. C., Maiyo, N. J., Ali, A. H., Kibungei, A. K., and Goheen, J. R. (2017). Lions influence the decline and habitat shift of hartebeest in a semiarid savanna. J. Mammal. 98, 1078–1087. doi: 10.1093/jmammal/gyx040
O'Brien, T. G., Kinnaird, M. F., Ekwanga, S., Wilmers, C., Williams, T., Oriol-Cotterill, A., et al. (2018). Resolving a conservation dilemma: vulnerable lions eating endangered zebras. PLoS ONE 13:e0201983. doi: 10.1371/journal.pone.0201983
Odadi, W. O., Karachi, M. K., Abdulrazak, S. A., and Young, T. P. (2011). African wild ungulates compete with or facilitate cattle depending on season. Science 333, 1753–1755. doi: 10.1126/science.1208468
Ogada, M. O., Woodroffe, R., Oguge, N., and Frank, L. G. (2004). Limiting depredation by African carnivores: the role of livestock husbandry. Cons. Biol. 17, 1521–1530. doi: 10.1111/j.1523-1739.2003.00061.x
Orrock, J. L., Witter, M. S., and Reichman, O. J. (2008). Apparent competition with an exotic plant reduces native plant establishment. Ecology 89, 1168–1174. doi: 10.1890/07-0223.1
Owen-Smith, N., and Mason, D. (2005). Comparative changes in adult vs. juvenile survival affecting population trends of African ungulates. J. Anim. Ecol. 74, 762–773. doi: 10.1111/j.1365-2656.2005.00973.x
Palmer, S. C. F., Hester, A. J., Elston, D. A., Gordon, I. J., and Hartley, S. E. (2003). The perils of having tasty neighbors: grazing impacts of large herbivores at vegetation boundaries. Ecology 84, 2877–2890. doi: 10.1890/02-0245
Porensky, L. M. (2011). When edges meet: interacting edge effects in an African savanna. J. Ecol. 99, 923–934. doi: 10.1111/j.1365-2745.2011.01824.x
Porensky, L. M., and Veblen, K. E. (2015). Generation of ecosystem hotspots using short-term cattle corrals in an African savanna. Rangeland Ecol. Manag. 68, 131–141. doi: 10.1016/j.rama.2015.01.002
Porensky, L. M., and Young, T. P. (2016). Development of edge effects around experimental ecosystem hotsports is affected by edge density and matrix type. Landsc. Ecol. 31, 1663–1680. doi: 10.1007/s10980-016-0344-3
Powell, R. A. (2000). “Animal home ranges and TERRITORIEs and home range estimators,” in Research Techniques in Animal Ecology: Controversies and Consequences. eds L. Boitani and T. K Fuller, (New York, NY: Columbia University Press), 65–110.
Prins, H. H. T., Grootenhuis, J. G., and Dolan, T. T. (2000). Wildlife Conservation by Sustainable Use (Dordrecht, Netherlands). Springer.
R Development Core Team (2017). R: A Language and Environment for Statistical Computing. Vienna: R Foundation for Statistical Computing. Available online at: https://cran.r-project.org/web/packages/survival/survival.pdf
Rondeau, V., Mazroui, Y., and Gonzalez, J. (2012). Frailtypack: R package for the analysis of correlated survival data with frailty models using penalized likelihood estimation or parametric estimation. J. Stat Soft. 47, 1–28. doi: 10.18637/jss.v047.i04
Schmidt, K. A. (2004). Incidental predation, enemy-free space and the coexistence of incidental prey. Oikos 106, 335–343. doi: 10.1111/j.0030-1299.2004.13093.x
Seaman, D. E., and Powell, R. A. (1996). Accuracy of kernel estimators for animal home range analysis. Ecology 77, 2075–2085. doi: 10.2307/2265701
Serrouya, R., Wittmann, M. J., McLellan, B. N., Wittmer, H. U., and Boutin, S. (2015). Using predator-prey theory to predict outcomes of broadscale experiments to reduce apparent competition. Am. Nat. 185, 665–679. doi: 10.1086/680510
Sinclair, A. R. E. (1985). Does interspecific competition or predation shape the African ungulate community? J. Anim. Ecol. 54, 899–918. doi: 10.2307/4386
Sinclair, A. R. E., Pech, R., Dickman, C., Hik, D., Mahon, P., and Newsome, A. (1998). Predicting effects of predation on conservation of endangered prey. Cons. Bio. 12, 564–575. doi: 10.1046/j.1523-1739.1998.97030.x
Stein, A. B., Fuller, T. K., Damery, D., Siever, P. R., and Marker, L. L. (2010). Farm management practices and financial analysis of farmer tolerance of leopards in the Waterberg region, North-central Namibia. Anim. Conserv. 13, 419–427. doi: 10.1111/j.1469-1795.2010.00364.x
Stephens, P. A., Sutherland, W. J., and Freckleton, R. P. (1999). What is the allee effect? Oikos 87, 185–190. doi: 10.2307/3547011
Stier, A. C., Samhouri, J. F., Novak, M., Marshall, K. N., Ward, E. J., and Holt, R. D. (2016). Ecosystem context and historical contingency in apex predator recoveries. Sci. Adv. 2:e1501769. doi: 10.1126/sciadv.1501769
Therneau, T. (2017). Survival: R Package for Survival Analysis Version 2.41-3. Available online at: http://www.CRAN.R-project.org/package=survival (accessed April 30, 2017)
Veblen, K. E. (2012). Savanna glade hotspots: plant community development and synergy with large herbivores. J. Arid Environ. 78, 119–127. doi: 10.1016/j.jaridenv.2011.10.016
Veblen, K. E., and Young, T. P. (2010). Contrasting effects of cattle and wildlife on the vegetation development of a savanna landscape mosaic. J. Ecol. 98, 993–1001. doi: 10.1111/j.1365-2745.2010.01705.x
Wahungu, G. M., Mureu, L. K., Kimuyu, D. M., Birkett, A., Macharia, P. G., and Burton, J. (2011). Survival, recruitment and dynamics of Acacia drepanolobium Sjostedt seedlings at Ol pejeta conservancy, Kenya, between 1999 and 2009. Afr. J. Ecol. 49, 227–233. doi: 10.1111/j.1365-2028.2010.01254.x
Wittmer, H. U., Serrouya, R., Elbroch, M., and Marshall, A. (2013). Conservation strategies for species affected by apparent competition. Cons. Bio. 27, 254–260. doi: 10.1111/cobi.12005
Keywords: African savanna, Allee effect, glade, human-occupied landscape, refuge, refuge-mediated apparent competition, wildlife–livestock interactions, hartebeest
Citation: Ng'weno CC, Buskirk SW, Georgiadis NJ, Gituku BC, Kibungei AK, Porensky LM, Rubenstein DI and Goheen JR (2019) Apparent Competition, Lion Predation, and Managed Livestock Grazing: Can Conservation Value Be Enhanced? Front. Ecol. Evol. 7:123. doi: 10.3389/fevo.2019.00123
Received: 30 October 2018; Accepted: 27 March 2019;
Published: 12 April 2019.
Edited by:
Matt W. Hayward, Faculty of Science, University of Newcastle, AustraliaReviewed by:
Inger Suzanne Prange, Appalachian Wildlife Research Institute, United StatesAntónio Onofre Soares, Universidade dos Açores, Portugal
Copyright © 2019 Ng'weno, Buskirk, Georgiadis, Gituku, Kibungei, Porensky, Rubenstein and Goheen. This is an open-access article distributed under the terms of the Creative Commons Attribution License (CC BY). The use, distribution or reproduction in other forums is permitted, provided the original author(s) and the copyright owner(s) are credited and that the original publication in this journal is cited, in accordance with accepted academic practice. No use, distribution or reproduction is permitted which does not comply with these terms.
*Correspondence: Caroline C. Ng'weno, Y25nd2Vub0BnbWFpbC5jb20=
Jacob R. Goheen, amdvaGVlbkB1d3lvLmVkdQ==