- 1Department of Ecology, Evolution, and Environmental Biology, Columbia University, New York, NY, United States
- 2Department of Biology, College of Charleston, Charleston, SC, United States
- 3Institute of Ecology and Evolution, University of Oregon, Eugene, OR, United States
- 4Department of Environmental Science, Policy, and Management, University of California, Berkeley, Berkeley, CA, United States
- 5Department of Earth and Environmental Sciences, Lamont-Doherty Earth Observatory, Columbia University, New York, NY, United States
Green roofs are a way for cities to mitigate environmental stressors, such as heatwaves and droughts. However, these environmental stressors can adversely affect green roof vegetation, causing challenges for plant growth and survival and subsequently reducing the ability of green roof systems to deliver critical ecosystem services, such as heat mitigation and nutrient cycling. Plant-associated microbes may facilitate the resilience and tolerance of green roof vegetation to climate-associated stress. However, despite their crucial role in plant growth and survival in natural ecosystems, there has been little research on plant-associated microbes in green roof systems. Plant choice on green roofs may also determine which microbes established in green roof growing media, and particular plant-microbial combinations may be more resilient to environmental stress. This project sought to characterize soil microbial community composition on green roofs across New York City with different plant palettes and assess how different combinations of green roof plant species and root-associated microbial assemblages responded to isolated and simultaneous heat and drought treatments. We surveyed green roofs planted with either Sedum species or with a mixed-vegetation palette (i.e., wildflowers, grasses, and succulents). We found that mixed-vegetation and Sedum green roofs had distinct soil bacterial and fungal communities (p < 0.0001) with a higher relative abundance of mycorrhizal fungi on mixed-vegetation roofs, and higher pathogen loads on Sedum roofs. Concurrently, we conducted a greenhouse experiment in which plants were grown from seed with live inocula collected from the two different types of vegetation on the green roofs we surveyed. We observed that plant species, soil inoculum, and abiotic stress treatment was correlated with shifts in soil fungal communities. This study demonstrated that soil microbial assemblages on green roofs are linked to the roof vegetation, and that they may facilitate green roof plants' tolerance and resilience to environmental stressors.
Introduction
In urban areas, green roofs are increasingly used to mitigate environmental problems associated with urbanization, such as the urban heat island effect (Takebayashi and Moriyama, 2007). Green roofs are vegetated roof surfaces where the vegetative layer cools roof surfaces through a variety of mechanisms including reflecting solar radiation, absorption, and evapotranspiration (VanWoert et al., 2005). Plant transpiration is one mechanism that provides the cooling benefit of green roofs, where CO2 entry and water loss occur through the leaf stomata, and as a result plants lose about 99% of water through transpiration (Lambers et al., 2008). However, not all plants photosynthesize and transpire at the same rate, so individual plant species may have differential effects on roof cooling. Green roof vegetation is also subject to extreme environmental conditions, including severe drought and elevated temperature (Dunnett and Kingsbury, 2008), and not all plants can tolerate these stressors. Consequently, many green roofs are planted with species of Sedum (Crassulaceae), a genus of drought-tolerant, succulent plants, which can tolerate the extreme fluctuations in roof conditions. Despite the widespread use of Sedum species, different plant types, such as grasses and tall forbs, have also been shown to establish successfully on green roofs and can often outperform Sedum in terms of green roof substrate cooling (Monterusso et al., 2005; Wolf and Lundholm, 2008; Lundholm et al., 2010). Plant choice on green roofs not only affects roof longevity and associated cooling benefits, but is important for the multifunctionality of green roof services including the maintenance of biodiversity in urban ecosystems (Lundholm et al., 2010).
The link between plant community and soil microbial community composition and diversity has been demonstrated in natural ecosystems (van der Heijden et al., 1998; Reynolds et al., 2003; Zak et al., 2003) but no studies have evaluated this relationship in engineered ecosystems. Beneficial fungi, namely mycorrhizal symbionts, decomposers, and endophytes, have been detected in high abundance in green roof substrates (McGuire et al., 2013; John et al., 2014). Plant growth-promoting and endophytic bacteria also enhance plant vigor and survival under harsh environmental (Wang et al., 2016) and biotic conditions (van Loon et al., 1998), and likely play an important role in green roof ecosystems as well (McGuire et al., 2015; Fulthorpe et al., 2018). John et al. (2014) reported arbuscular mycorrhizal (AM) fungal colonization on green roof plant species in the families Asteraceae and Poaceae, providing crucial information on the potential role of AM fungi and their host plants in green roof ecosystems. However, there are no additional surveys or field experiments evaluating the link between green roof plant and fungal assemblages, and there is no information on bacterial communities in green roof substrates. No studies to date have explicitly evaluated differences in soil microbial community composition between mixed-vegetation and single-genus (Sedum) plant communities on green roofs.
While most studies focus the performance of green roof plant communities, underlying mechanisms facilitating plant tolerance and resilience to the environmental extremes relate directly to soil microbial communities in green roof ecosystems. It is well-established that plant-associated bacteria and fungi are integral for plant growth, tolerance to environmental stressors, and nutrient acquisition in non-engineered systems (Rodriguez et al., 2004; Yang et al., 2009). Thus, like plant communities, variation in soil microbial composition and function may affect the benefits and services green roofs provide in urban ecosystems. Microbial composition can vary in response to abiotic factors (Chaudhary et al., 2016; Lenoir et al., 2016) as well as biotic factors, such as plant composition (Smalla et al., 2001; Kowalchuk et al., 2002; Bever et al., 2012) and traits (Lopez-Garcia et al., 2014); it is therefore essential to link this variation in microbial composition to shifts in ecosystem function. To date, there are few studies assessing the role of soil microbiota on plant health and overall function in green roof systems (McGuire et al., 2013, 2015; John et al., 2014, 2017; Molineux et al., 2014; Fulthorpe et al., 2018; Rumble et al., 2018; Xie et al., 2018), and no studies to date have explicitly evaluated differences in soil microbial community composition between mixed-vegetation and single-genus (Sedum) plant communities on green roofs.
This project aimed to evaluate how soil microbial assembly and function vary with plant community composition on green roofs across New York City. We surveyed 32 green roof systems with either Sedum species or with a mixed-vegetation palette (i.e., wildflowers, grasses, and succulents) and characterized bacterial and fungal composition across these two plant palates. Concurrently, we conducted a greenhouse experiment in which plants were grown from seed in autoclaved green roof medium inoculated with live microbiota collected from a subset of our green roof survey sites representing the two different plant palates; once established, the plant-soil communities were subjected to environmental stress treatments (heat and drought). The green house experiment allowed us to directly evaluate the contribution of variations in soil microbial inoculum to plant vitality and ecosystem service delivery, and our survey data allowed us to explore soil microbial assembly patterns across different green roof vegetation communities. We hypothesized that (1) Sedum and mixed-vegetation plant communities would host distinct soil bacterial and fungal communities; (2) green roofs planted with a mixed-vegetation palette would have greater bacterial and fungal diversity due to higher plant diversity; (3) there would be greater relative abundance of AM fungi on mixed-vegetation green roofs; and (4) plants inoculated with the mixed-vegetation green roof soil would have greater survivorship, biomass, and stomatal conductance (gs) in response to environmental stressors. Connecting the variation in plant-associated microbial assemblages with their functional roles in providing plant tolerance and resilience to environmental stressors in green roof ecosystems is crucial to maximizing the benefits obtained by green roofs.
Methods
Soil Microbial Community Survey
To characterize green roof soil microbial assemblages, soil samples were collected from 32 green roof sites across New York City (Figure 1). New York City has a mean annual temperature of 16.4°C and average annual precipitation is ~1,110 mm (Graphical Climatology 2018). All samples were collected between July 10 and 21, 2017. Green roof buildings ranged from 1.5 to 12 stories and vegetated roofs ranged from ~96–6,070.28 m2 in area (Table 1). The average area covered by soil core sampling is 88.73 m2 to account for species-area relationships and the areas surveyed on green roofs ranged from 15 to 100 m2. Two categories, Sedum and mixed-vegetation, were used to broadly characterize the plant communities on each green roof. Sedum green roofs denote rooftops planted exclusively with Sedum species while the mixed-vegetation category captures roofs planted with any or all of the following: grasses, wildflowers, and succulents (Figure 2).
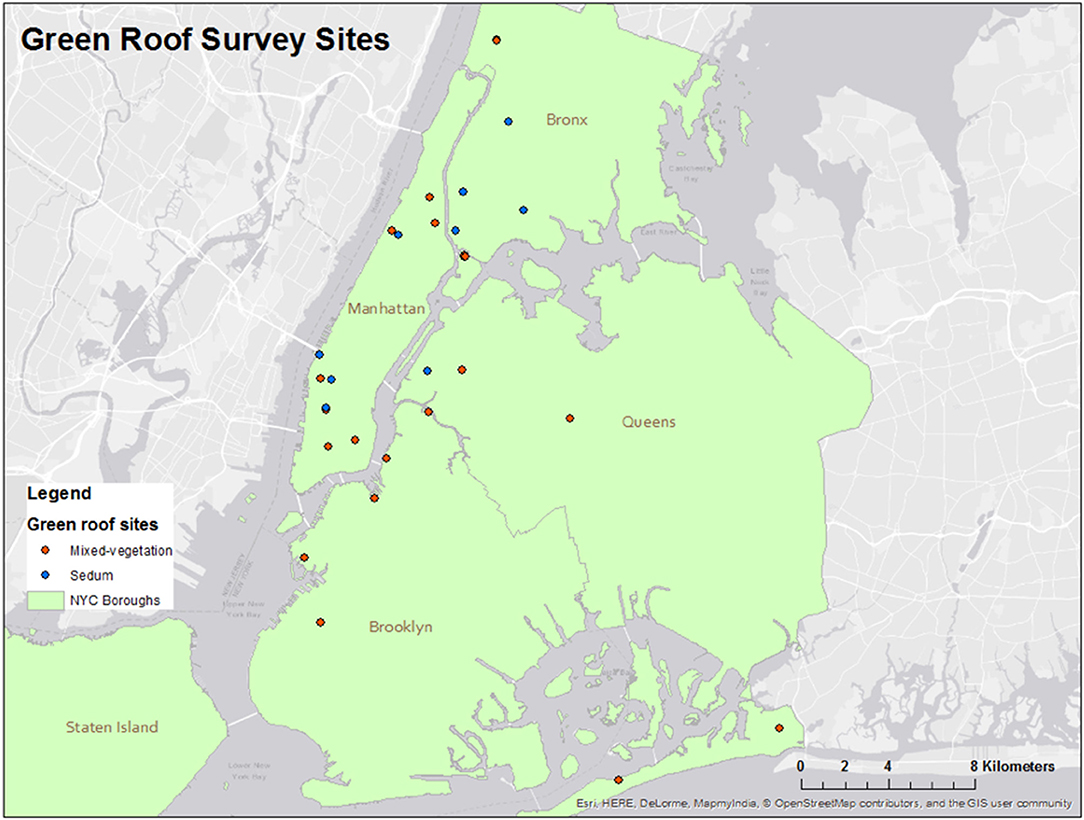
Figure 1. Locations of the green roofs sampled in the study across the Bronx, Brooklyn, Manhattan, and Queens. Blue and red points denote roofs planted with Sedum and mixed-vegetation, respectively.
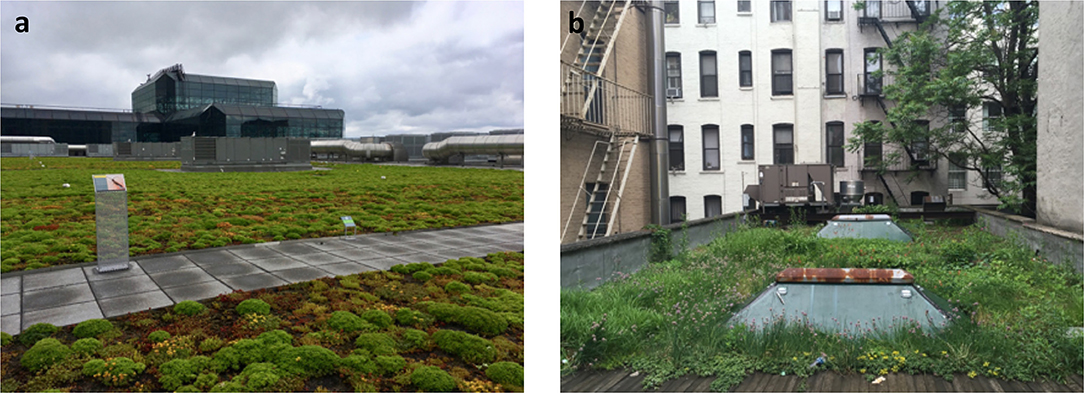
Figure 2. Examples of a Sedum green roof on the Javits Center (a) and a mixed-vegetation green roof, on the Wild Project Theater (b).
To survey soil bacterial and fungal communities on each roof, we collected nine randomized 10-cm deep soil samples using 2.5-cm diameter soil corers. Since host plant species may influence root-associated microbial assemblages (Smalla et al., 2001; Kowalchuk et al., 2002; Singh et al., 2008), we collected soil samples adjacent to species of Sedum from the Sedum green roofs and adjacent to species of Asteraceae on the mixed-vegetation roofs. We chose this method of targeted sampling to account for potential variation in soil microbial community composition due to differences in plant species. Asteraceae plants were selected for targeted sampling due to frequent use of Asteraceae plant species on green roofs (McGuire et al., 2013; Aloisio et al., 2016). Due to variation in green roof design, samples were arranged according to the layout of vegetation. On green roofs with continuous patches of Sedum or Asteraceae plants, soil cores were obtained at three points along three randomly arranged transects. The second method was unique to mixed-vegetation roofs, where all patches of Asteraceae species were numbered and subsequently selected for sampling using a random number generator. We also collected additional roof information from either the green roof database managed by the Green Roof Researchers Alliance, when possible, or contacted green roof managers directly (Table 1).
Greenhouse Experimental Design
To assess the effects of green roof soil microbial communities on plant physiological response to heat and drought, we conducted a replicated, fully factorial experiment in the Arthur Ross Greenhouse at Barnard College, Columbia University (New York, NY, USA). We germinated 120 Panicum virgatum (Poaceae) and 120 Solidago nemoralis (Asteraceae) individuals from seed (Greenbelt Native Plant Center, Staten Island, NY, USA) and propagated 120 Sedum tetractinum (Crassulaceae) plants from cuttings (NYC Department of Parks and Recreation Citywide Nursery, Bronx, NY, USA). Soil microbial communities were established in greenhouse pots via inoculation with field-collected soil from eight conventional green roofs planted with Sedum mats and eight green roofs planted with mixed-vegetation (grasses and wildflowers), and autoclaved green roof media (rooflite Semi-intensive green roof mix) as a control. Hereafter, the soil treatments are referred to as Sedum soil, mixed-vegetation soil, and autoclaved control soil, respectively. Experimental pots were arranged in nine total treatments (three focal plant species × three soil types) (Figure S1).
In the greenhouse, plants underwent four treatments: drought stress, heat stress, heat + drought stress, and a well-watered, non-heated control. Following a 90-day period to allow soil inocula to fully establish in each treatment, drought was induced on 90 of the plants by withholding water for 10 days, simulating summer drought conditions in NYC. Germination heat mats (VIVOSUN) were placed beneath pots receiving heat and heat + drought treatments for 10 days, increasing substrate temperature from an average of 27.2–29.7°C. Pots that were not drought treated received water every 3 days, which was estimated before the stress treatments began by measuring soil moisture and pot weight each day (soil moisture declined from an average 22.3% moisture on the day of watering to ~2% after 3 days post-watering).
We used plant survivorship, biomass, and gs to evaluate individual plant responses to abiotic stressors. Twice per week, plants were recorded as either alive or dead. Final fresh and dry biomass was collected for S. tetractinum by first removing all soil from the plant root system, and separating above-ground and below-ground parts of the plant. Prior to oven-drying the plants, all roots and shoots were weighed to obtain fresh mass. Dry mass was estimated by weighing the roots and shoots after they were oven-dried for 48 h at 60 degrees C.
For each plant-soil-treatment combination, one leaf on four individuals was selected for repeated gs. For all plants, gs, leaf temperature, and pot weight measurements were collected from 10:00 to 13:00 using an SC-1 leaf porometer (Decagon Devices). Measurements were obtained on alternate days for control and drought, and heat and heat and drought treatments, following watering schedules, totaling five samples for each of the four replicates in each plant-soil-treatment combination. All measurements were collected 2 days after watering for the control and heat treatments. Immediately after the treatments were completed, three soil samples from each plant-soil-treatment combination were collected to investigate root-associated fungal and bacterial communities (108 samples total).
Microbial Analyses
To characterize bacterial and fungal communities from the green roof survey and fungal communities from the greenhouse experiment, DNA was extracted from ~0.25 g of each soil sample from the soil survey and greenhouse experiment using a DNeasy PowerSoil Kit (Qiagen). For fungal OTUs, PCR amplification of the internal transcribed spacer region 1 (ITS1F) was carried out using the fungal-specific primer pair, ITS1F and ITS2R, adapted for the Illumina platform (McGuire et al., 2013). For bacterial OTUs, PCR amplicons for the 16S rRNA gene and a corresponding Illumina dataset were generated.
For ITS data, reads were de-multiplexed, quality-filtered, and processed using QIIME (Caporaso et al., 2010). The UNITE fungal reference database was used to define operational taxonomic units (OTUs). High-quality sequences from each sample were rarified to 13,000. OTUs were assembled using the QIIME V1.9.1 1 pipeline.
For 16S data, sequences were de-multiplexed and all sequences with a quality score <20 were excluded from analysis. OTUs were selected using the UCLUST algorithm and taxonomy was assigned using the greengenes reference database (McDonald et al., 2012) with the RDP classifier specialized for 16S rRNA sequences (Wang et al., 2007). For both ITS and 16S data, data were transformed using the “phyloseq” and “dplyr” packages in R (McMurdie and Holmes, 2013; Wickham and François, 2015) to join metadata with the cleaned OTU tables prior to downstream analysis.
Statistical Analyses and Data Visualization
In R, we computed Shannon-Weiner and Simpson diversity indices (Oksanen et al., 2018) to compare bacterial and fungal alpha diversity measures between vegetation communities and tested statistical significance of diversity indices between vegetation communities using PermANOVA in R (Anderson, 2001). We assessed fungal and bacterial community similarity by using Bray-Curtis dissimilarity index to calculate pairwise distances among fungal and bacterial communities due to our method of sampling (maximum area of 100 m2 on a green roof) and its use in similar studies evaluating community composition on green roofs, including microbial communities (McGuire et al., 2013; Parkins and Clark, 2015; Partridge and Clark, 2018). Using non-metric multidimensional scaling (NMDS) plots, we visualized fungal and bacterial community clustering. We tested statistical significance of observed clustering patterns by plant community using PermANOVA in R. A random forest model was used to test if vegetation type could be classified based on bacterial and fungal scaled relative abundance (Liaw and Wiener, 2012; Evans and Murphy, 2018). To explore which taxa were associated with mixed-vegetation compared to Sedum green roof systems, we used the R package indicspecies (De Caceres and Legendre, 2009) to run an indicator taxa analysis.
We assessed fungal and bacterial community similarity on a subset of samples from the NYC Parks Department Five Borough Building, using the same index and analysis that was employed for the city-wide assessment (Bray-Curtis dissimilarity index, PermANOVA).
For the greenhouse experiment, we used generalized linear models (GLMs) to explore if each variable (plant species, soil treatment, and environmental treatment) was predictive of plant response (survivorship, biomass, and gs). GLMs were employed as the data were not normally distributed. We ran a GLM at each sampling time for gs and used the final biomass and survivorship as response variables. Since final survivorship is a binary response variable, we specified the family as binomial in the GLM for plant survivorship.
Using the R package FUNGuild (Nguyen et al., 2015), we categorized all OTUs into the following functional groups: animal endosymbiont, animal pathogen, arbuscular mycorrhizal, ectomycorrhizal, endophyte, epiphyte, ericoid mycorrhizal, fungal parasite, lichen, plant pathogen, saprotroph, and unclassified. All data visualization and figures were created using “ggplot2” (Wickham and François, 2015).
Results
Soil Microbial Diversity on NYC Green Roofs
After filtering the dataset for high-quality sequences, there were 13,332 fungal OTUs among the 266 samples with an average of 494 OTUs per sample. The most abundant phyla were Ascomycota (67.2%), Basidiomycota (19.1%), Glomeromycota (6.4%), and Mortierellomycota (3.5%) in both vegetation systems (Figure 3). Green roofs planted with mixed-vegetation communities had a greater number of fungal OTUs in each sample (540.85 ± 110.28) compared to roofs planted with Sedum species (411.90 ± 75.51). A total of 486,026 bacterial OTUs were classified and samples were rarefied to an even depth of 82,376 OTU per sample. Though there were no significant differences in alpha diversity among indices for bacteria, there was significantly higher observed (df = 1, F = 78.39, p < 0.001) and Shannon (df = 1, F = 7.024, p < 0.01) alpha soil fungal diversity on mixed-vegetation green roof systems.
Green roof soil fungal communities were significantly correlated with both biotic and abiotic factors. Mixed-vegetation and Sedum green roof plant communities had significantly different fungal communities (R = 0.27, p < 0.001; Figure 4A) and bacterial communities (R = 0.03, p < 0.01; Figure 4B). Soil fungal communities were compositionally distinct across plant genera (R = 0.38, p < 0.001), roof age (R = 0.13, p < 0.001), and by green roof system (R = 0.44, p < 0.001).
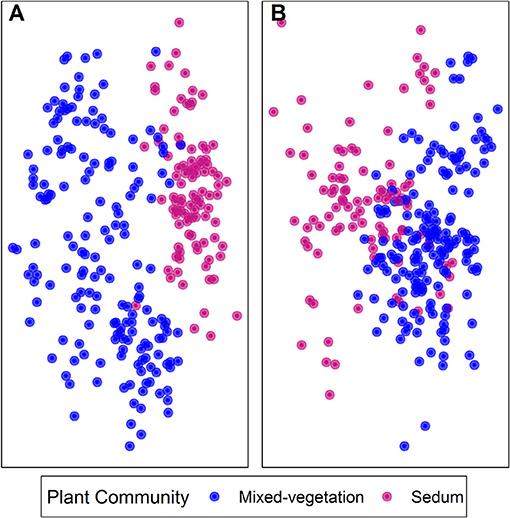
Figure 4. Non-metric multidimensional scaling plot of fungal (A) and bacterial (B) communities on mixed-vegetation and Sedum green roofs. ANOSIM analysis revealed significant clustering of both fungal and bacterial communities across vegetation community type.
To account for our large sample size (n = 266), we focused on green roof systems sampled from the NYC Parks Department Five Borough Building, which is planted with a variety of green roofs containing different plant palates and soil types. This allowed a local test of differences in microbial communities associated with different plant communities, while keeping the location constant. We sampled four green roof systems, which are on the same roof and were built between 2009 and 2011. Among the four green roof systems on the Five Borough Building, there were significant differences in fungal community composition between vegetation type (R = 0.8237, p < 0.001) and across the green roof systems (R = 0.6292, p < 0.001; Figure 5A). There were significant differences in bacterial community composition by vegetation type (R = 0.69; p < 0.001; Figure 5B).
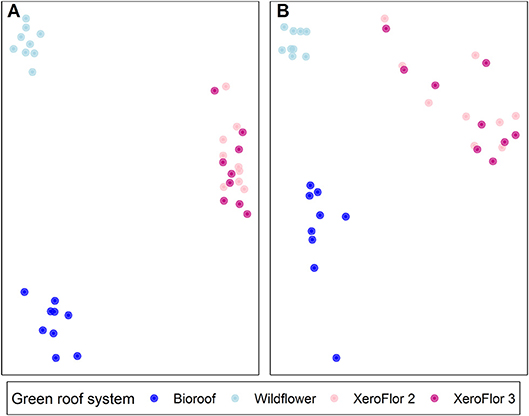
Figure 5. Non-metric multidimensional scaling plot of fungal (A) and bacterial (B) communities on the NYC Parks Five Borough Building. The Bioroof and Wildflower (blue) roof are planted with a mixed-vegetation community and XeroFlor 2 and 3 (pink) are planted with Sedum species.
We ran random forest models to determine if vegetation type (mixed-vegetation or Sedum) could be classified based on bacterial and fungal scaled relative abundance. Both bacterial and fungal communities could classify vegetation type the majority of the time. Running 1,000 permutations, 99% of samples from mixed-vegetation roofs were classified using bacterial communities compared with 97% with fungal communities; and 90.7% of samples from Sedum green roofs were correctly classified with bacterial communities and 94% with fungal communities.
Saprotrophs were the most abundant fungal functional group on both mixed-vegetation (55.71%) and Sedum (40.83%) green roof systems (Figure 6). Plant pathogens comprised 14.51% of OTUs on Sedum green roofs, compared to 11.11% on mixed-vegetation roofs. The relative abundance of AM fungi was ~4 times higher on mixed-vegetation green roofs (1.37%) compared to Sedum green roofs (0.32%).
A total of 398 fungal OTUs were significantly associated with either the mixed-vegetation or the Sedum green roof sites. Twenty-nine of the 42 fungal indicator families associated with mixed-vegetation roofs aligned with Ascomycota and nine aligned with Basidiomycota. Two families, Archaeosporaceae and Claroideoglomeraceae, are in the phylum Glomeromycota (AM fungi). All indicator families for Sedum green roofs were within Ascomycota or Basidiomycota.
There were 48 bacterial indicator families associated with mixed-vegetation green roof systems, 22 of which aligned to the phylum Proteobacteria. Indicator taxa included genera recognized as plant growth-promoting bacteria, such as Bacillus (Glick, 2012) and Azospirillum (Saikia et al., 2014). There were 38 bacterial indicator families for Sedum green roofs, including Rhizobiaceae and Bradyrhizobiaceae, both of which contain plant-associated bacterial species.
Spearman's correlation between green roof abiotic and biotic factors revealed a strong association between the substrate depth and vegetation type (r = −1). A redundancy discriminant analysis revealed a much larger proportion of unconstrained variance (0.82) compared to constrained variance (0.18), though vegetation type explained the most variance in soil fungal community composition compared to all other variables analyzed.
Greenhouse Experiment
Plant Survivorship, Biomass, and gs
Sedum tetractinum had the highest mean survivorship (0.9 ± 0.09) compared to P. virgatum (0.79 ± 0.21) and S. nemoralis (0.73 ± 0.21). Survivorship for S. tetractinum was not significantly affected by any environmental stressor or soil treatment. However, P. virgatum and S. nemoralis were significantly affected by all the stress treatments (p < 0.01). Plants subjected to drought had the lowest survivorship compared to the well-watered control, heat and heat and drought treatments for both P. virgatum (0.67 ± 0.25) and S. nemoralis (0.59 ± 0.20). S. nemoralis had slightly lower survivorship under heat (0.71 ± 0.20) and heat + drought (0.70 ± 0.19) compared to P. virgatum (0.78 ± 0.19; 0.79 ± 0.26, respectively). The survivorship of P. virgatum and S. nemoralis were not significantly associated with any soil inoculum treatment.
Compared with the well-watered and heat stress treatments, S. tetractinum plants subjected to drought had the highest above-ground (0.50 ± 0.23 g) and below-ground biomass (0.12 ± 0.07) while those subjected to heat stress had the lowest above-ground (0.41 ± 0.20 g) and below-ground biomass (0.09 ± 0.05 g). While there were no significant correlations between above- or below-ground biomass and environmental treatment, S. tetractinum had greater below-ground biomass when grown in the autoclaved soil (0.12 ± 0.06 g) compared to the mixed-vegetation (0.09 ± 0.03 g) and Sedum (0.10 ± 0.06 g) green roofs soil (Figure 7).
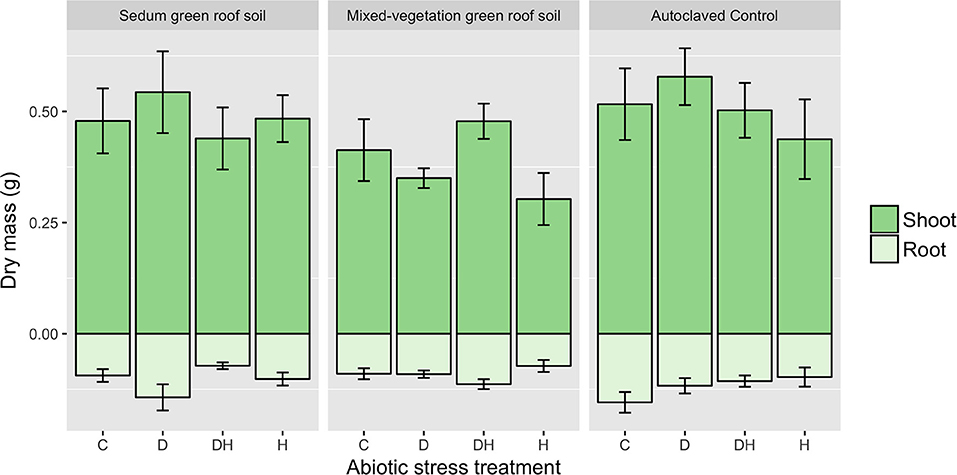
Figure 7. Above and below-ground biomass for Sedum tetractinum by soil and environmental stress treatment (C, control; D, drought; DH, drought and heat; H, heat). Sedum tetractinum had greater average below-ground biomass when grown in autoclaved control soil compared to mixed-vegetation and Sedum soils.
There were no significant overall differences in stomatal conductance among plant species, soil treatments, or abiotic stress treatments. However, consistent trends were found such that Panicum virgatum had the highest mean stomatal conductance (46.71 ± 46.66 mmol m−2s−1) followed by S. nemoralis (45.04 ± 74.24 mmol m−2s−1) and S. tetractinum (29.60 ± 26.33 mmol m−2s−1). Stomatal conductance was higher for P. virgatum (52.12 ± 50.99 mmol mm−2s−1) and S. tetractinum (31.5 ± 33.38 mmol m−2s−1) plants grown in mixed-vegetation green roof soil, compared to Sedum and autoclaved soil.
T2 and T3 represent 5 and 10 days, respectively, of heat and/or drought stress. At T2, S. nemoralis had significantly higher gs (112.21 ± 114.95 mmol mm−2s−1) compared to P. virgatum (63.33 ± 69.63 mmol m−2s−1) and S. tetractinum (34.2 ± 31.29 mmol m−2s−1). During T2, mean S. nemoralis gs was significantly lower when subjected to drought stress (p < 0.01; 31.28 ± 46.11 mmol m−2s−1) and higher under heat stress (p < 0.01; 130.33 ± 93.34 mmol mm−2s−1). At T3, P. virgatum had significantly higher gs (p < 0.0001; 44.62 ± 49.28 mmol m−2s−1) compared with S. nemoralis (23.93 ± 42.86 mmol m−2s−1 and S. tetractinum (30.39 ± 22.74 mmol m−2s−1; Figure 8). Solidago nemoralis plants grown in mixed-vegetation green roof soil had significantly higher gs (p < 0.01; 38.59 ± 54.7 mmol m−2s−1) at T3 compared to those grown in autoclaved (11.08 ± 9.55 mmol m−2s−1) or Sedum green roof soil (24.1 ± 49.93 mmol m−2s−1; Figure 9).
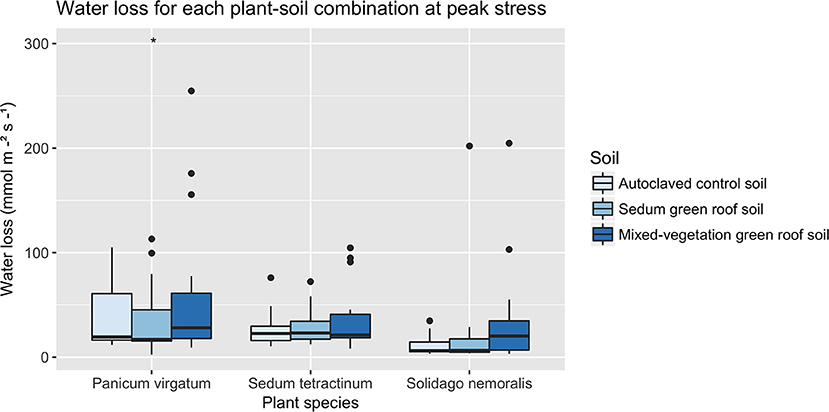
Figure 8. Stomatal conductance for each plant-soil combination after 10 days of heat and drought treatments (T3). Panicum virgatum had significantly higher stomatal conductance compared to Solidago nemoralis and Sedum tetractinum (p < 0.0001). Asterisks denote significance.
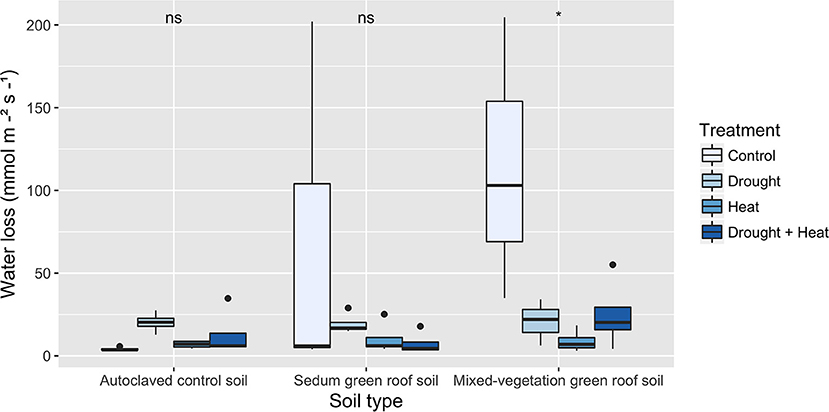
Figure 9. Stomatal conductance for each soil-treatment combination (C, control; D, drought; DH, drought and heat; H, heat) after 10 days of heat and drought treatments (T3) for Solidago nemoralis. When grown in mixed-vegetation soil, Solidago nemoralis plants had significantly higher stomatal conductance compared to those grown in autoclaved control or Sedum green roof soil (p < 0.01). Asterisks denote significance.
Soil Fungal Community Composition
After filtering the dataset for high-quality sequences, the OTU table was rarefied to 3,972 OTU per sample. Soil fungal community composition differed significantly with plant species (R = 0.57, p < 0.001), soil inoculum (R = 0.25, p < 0.001), and abiotic stress treatment (R = 0.20, p < 0.01). A multiplicative model showed significant clustering of soil fungal community composition by soil inoculum and plant species (R = 0.22, p < 0.001) as well as with soil inoculum and abiotic stress treatment (R = 0.26, p < 0.05). However, no significant interaction was observed when combining plant species and abiotic stress treatment or with all three variables combined.
Discussion
Soil Microbial Community Composition
The distinct soil microbial assemblages on Sedum compared to mixed-vegetation green roofs support our first hypothesis that green roof soil microbial community composition shifts with host plant species as observed in natural ecosystems (van der Heijden et al., 1998; Reynolds et al., 2003; Zak et al., 2003). Potential ecological mechanisms supporting these findings include microbial host-specificity (Santos-Gonzalez et al., 2007), host plant root structural differences (el Zahar Haichar et al., 2008), and functional group complementarity (Newsham et al., 1995). We found that within a single roof (the NYC Parks Five Borough Building roof), microbial community composition varied with plant composition and that 99% of the time, vegetation communities could be classified based on bacterial and fungal, strongly suggesting that soil microbial assemblages are not solely driven by differences in roof location and roof planting history, but also by the plant community assemblages themselves.
There is ample material supporting microbial community composition as drivers of plant community structure (Reynolds et al., 2003; van der Heijden et al., 2006; Bever et al., 2010) through direct interactions between plant roots and microbial functional groups, such as pathogens and mutualists, and from microbial nutrient cycling. As constructed ecosystems, green roofs are planted with a specific palette, enabling a directional perspective on the effect of plant communities on soil microbial composition. Previous work has highlighted the role of microbial composition on successional plant community establishment. Unmanaged green roofs are ideal sites to explore the role of microbial communities in plant community development on green roofs.
We chose to target species in Asteraceae on the mixed-vegetation roofs, thus our results may reflect differences in root-associated microbial community composition among Sedum and Asteraceae plant species. Studies assessing root-associated microbial communities with more intensive sampling are crucial to further assess associations between plant and microbial assemblages on green roofs.
Our field survey did not control for green roof substrate type and initial inoculum, which is crucial to obtain a clearer picture of the relationship between plant and soil microbial community assemblages. Molineux et al. (2014) manipulated soil microbial communities using compost tea and AM fungi in two different substrate types, revealing the key role of substrate type and depth on green roof microbial ecology (measured by biomass). Nonetheless, the fact that we observed a difference in microbial communities associated with the different plant communities, irrespective of substrate type and initial inoculum, suggests that the effect of the plant community is a robust driver of microbial assembly in these systems.
McGuire et al. (2013) found distinct soil fungal communities between replicate green roofs and nearby city parks. Many bacteria and fungi are wind dispersed and AM fungi spores are more effectively transported by animals capable of reaching rooftops (Camargo-Ricalde, 2002), thus dispersal limitations could have been driving these differences. Initial inoculum in the starting green roof substrate or on plant roots growing in nursery soil may exhibit priority effects, which could impact the ability of other microbial taxa to establish after planting. Rumble et al. (2018) reported high levels of independent mycorrhizal colonization of Sedum species (S. album, S. spurium, S. reflexum) in a field experiment (92%), while John et al. (2014) reported no mycorrhizal colonization of Sedum acre, but colonization of all other green roof species, especially on Solidago bicolor (Asteraceae) and Danthonia spicata (Poaceae).
The most abundant microbial taxa we identified in green roof soils are also widespread in other temperate ecosystems. For example, species of Mortierella, the most abundant fungal genus in mixed-vegetation green roof soils, and has been confirmed in a variety of habitats including natural compost and forest soils (Wagner et al., 2013). On the Sedum roofs, the most abundant fungal genus on green roofs planted with Sedum was Corynespora, specifically the species Corynespora cassiicola, which is a plant pathogen. This fungal species is widespread in its geographic distribution and was recently described to cause “stem spot” an economically-important succulent plant in Europe (Madriz-Ordeñana et al., 2017). The pathogens identified in our survey may manifest as disease-causing agents for the green roof plants. Proteobacteria are also found in a variety of habitats and comprise a wide array of functions, ranging from pathogens to nitrogen fixing bacteria.
We found that AM fungi were abundant in all green roof samples, suggesting that plant-fungal mutualisms are sustained on green roof ecosystems, which corroborates with previous studies investigating microbial communities on green roofs (McGuire et al., 2013; John et al., 2014; Molineux et al., 2014; Rumble et al., 2018). Mixed-vegetation roofs had a greater abundance of AM fungi than the Sedum roofs, which had lower plant and microbial diversity. Mycorrhizal fungi colonize the root systems of 95% of plant families (Smith and Read, 1994), often benefiting the host by enhancing drought tolerance, increasing aboveground biomass, and promoting plant survivorship through enhanced nutrient uptake and resistance to pathogen attack (Augé, 2001). Due to environmental characteristics of green roofs, such as shallow growing media and drought and heat stress during the summer, grasses and forbs on green roofs likely highly depend on AM fungal symbionts for growth and survival. AM fungi can also mitigate the harmful effects of soil pathogens through reducing pathogen growth (Borowicz, 2001), eliciting plant defense mechanisms and competing with other rhizosphere biota associating with host plants (Azcón-Aguilar and Barea, 1997). Further research elucidating AM fungal community assembly and function in green roof systems is necessary to better understand the roles of these mutualists in stressful environments. We detected additional fungal functional groups, including ericoid mycorrhizal fungi and ectomycorrhizal fungi. A number of ectomycorrhizal host plants are temperate forest tree species common in New York City's canopy (Pregitzer et al., 2019), but these are not planted on green roofs; however, fungal spores in association with these tree species likely dispersed through the air and landed in the green roof substrate where we sampled.
The fact that AM fungi were most abundant in mixed-vegetation soil under drought conditions (Figure 10), compared to all other soil inoculum-treatment pairings in the experiment, suggests that AM fungi may play a role in maintaining plant cooling benefits under dry conditions on green roofs. Numerous field and greenhouse experiments have shown the effects of mycorrhizal fungi on stomatal conductance, the rate at which CO2 enters and water exits the leaf, with higher stomatal conductance in drought vs. well-watered conditions (Augé, 2001; Augé et al., 2015). While adverse abiotic conditions reduce AM fungal diversity compared to non-disturbed soil, some AM fungi exhibit opportunistic life history strategies including investing energy into spore production (Declerck et al., 2001) and, in the case of Rhizophagus irregularis, rapidly colonizing plant roots after disturbance (Sýkorová et al., 2007). In the green roof survey samples, the three most abundant OTUs in Glomeromycota aligned to Rhizophagus irregularis, which occurs in high relative abundance with Asteraceae plants (Wehner et al., 2014), and have previously been observed in green roof fungal communities in New York City (McGuire et al., 2013). In this study, we collected and sequenced bulk soil communities from the rhizosphere of target plant species; this sampling strategy likely only detects AM fungal taxa extraradical mycelia and spores. Since plant biomass and survivorship during drought conditions has been tightly correlated with mycorrhizal colonization (Augé, 2001), quantifying percent root colonization and characterizing AM fungal taxa present in plant roots rather than just bulk soil is necessary to further elucidate the assembly patterns and functional roles of AM fungi in green roof systems.
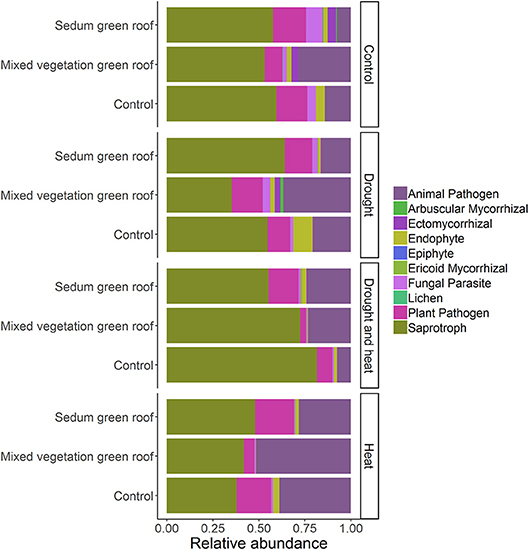
Figure 10. The relative abundance of fungal functional groups detected from greenhouse experiment substrates.
The shifts in relative abundances of plant-growth promoting bacteria on mixed-vegetation compared to Sedum green roofs suggests the importance of integrating knowledge of soil bacteria into green roof strategies. Plant growth-promoting and endophytic bacteria promote plant vigor and survival under harsh environmental (Wang et al., 2016) and biotic conditions (van Loon et al., 1998), and thus likely play an important role in green roof ecosystems (McGuire et al., 2015; Fulthorpe et al., 2018). Xie et al. (2018) inoculated a variety of plant species with Bacillus amyloliquefaciens, a plant-growth promoting bacteria that associates with plant roots and confers benefits, such as pest resistance and nutrient absorption to the host. In addition to facilitating plant shoot growth, B. amyloliquefaciens demonstrated promotion of host colonization by AM fungus R. irregularis (Xie et al., 2018). The co-occurrence of plant growth-promoting bacteria and mutualist fungi associated with mixed-vegetation roofs suggest the role of root-associated biotic interactions in facilitating plant growth, survival, and subsequent ecosystem function on green roofs.
Plant Survivorship, Growth, and Physiological Response to Abiotic Stress
Obtaining direct measurements on plant gs and biomass, and tracking survivorship of plants grown with different soil microbial inocula in controlled conditions enabled a more focused understanding of the soil microbial control on plant vitality and overall ecosystem services in green roof systems. The high survivorship of S. tetractinum aligns with results from previous green roof experiments, which have shown the resilience of Sedum species to abiotic stress on green roofs (Monterusso et al., 2005; Lundholm et al., 2010; Nagase and Dunnett, 2010). These results also demonstrate the negative impact of drought relative to heat on the survivorship of grasses and forbs.
The unique response of S. tetractinum compared with P. virgatum and S. nemoralis to abiotic stress treatment and soil inocula suggest potential functional complementarity between succulents, grasses, and forbs as they relate to cooling capacity. When subjected to drought, S. tetractinum had the greatest above and below-ground biomass, as Sedum species may be more adversely affected by overwatering than by under-watering (Sterphenson, 1994). We found that S. tetractinum plants had significantly greater root biomass when grown in autoclaved soil, which contrasts to previous work, in which plant-associated beneficial microbes increased shoot and root biomasss of Sedum alfredii (Ma et al., 2016). However, John et al. (2014) found no colonization of Sedum acre on green roofs. We speculate that S. tetractinum may have greater root biomass in autoclaved soil since it may have less percent root colonization by mycorrhizal fungi and as a result, needs to grow its root network to access nutrients in the soil.
Differences among plant species and stomatal conductance (gs), which were especially pronounced during peak stress, highlighted the effects of plant life form and on potential green roof cooling benefits. Previous studies reported that tall forbs and grasses, including Solidago bicolor, experienced high water loss, compared to succulents (John et al., 2014) and that grass had the highest water loss across all watering treatments while forbs had equivalent water loss to grasses in well-watered and intermediate drought conditions (Wolf and Lundholm, 2008). Planting green roofs with grass and forb species may contribute to greater cooling benefits due to the relatively high transpiration rates in grasses and forbs compared to succulents, with the caveat that forbs lose cooling capacity under severe drought.
The elevated gs of P. virgatum at peak heat and drought conditions indicate its potential usefulness in maintaining cooling benefits under abiotic stress conditions. Conversely, S. nemoralis had relatively low gs at peak stress, but had elevated gs when grown in mixed-vegetation soil during the heat and drought treatments. Solidago nemoralis had extremely low gs under all treatments when grown in autoclaved soil. We speculate that AM fungi present in the field-collected inoculum enabled S. nemoralis to retain elevated gs in a variety of conditions including well-watered and moderate heat stress.
Conclusions
This study demonstrated that soil microbial assemblages on green roofs are directly linked to the roof plant species composition, and that root-associated microbial communities confer tolerance to abiotic stressors in green roof ecosystems. Patterns of microbial functional group composition across vegetation community, specifically the presence of important AM fungal taxa and plant growth-promoting bacteria, and high relative abundance of plant and animal pathogens on Sedum green roofs, indicated that green roof soil management needs to consider biotic elements more explicitly. High survivorship of S. tetractinum and elevated gs of P. virgatum indicated the utility of maintaining a diverse plant palette in order to optimize cooling benefits associated with green roofs. Further, the crucial role of root-associated microbial taxa, specifically AM fungi and plant-growth promoting bacteria, in mitigating drought and heat stress on S. nemoralis hosts demonstrated the need for correspondingly diverse microbial assemblages to promote green roof plant health and ecosystem function.
Our results provide strong evidence that various components of green roof design, namely soil physicochemical properties and vegetation, can structure green roof microbial communities and the relative abundance of specific functional groups, which needs to be considered more intentionally in green infrastructure planning. These results are foundational for future studies assessing relationships between plant communities and soil microbial communities, and the extent to which they improve ecosystem service delivery on green roofs.
Author Contributions
JH, KM, MR, MP, and KG conceived the ideas and designed methodology. JH, KS, and AS collected field data, set up the greenhouse experiment, and collected data. JH, KS, AS, and TH processed samples. TH, DD, and AG conducted bioinformatics work. JH, KM, MP, and KG interpreted results, and JH, KM, DD, TH, and KS wrote the manuscript. All authors contributed to drafts and gave final approval for publication.
Funding
Funds were provided by the National Science Foundation DEB Coastal SEES 1325185 to KM and MP; by the Jacob K. Javits Convention Center to MR and KM; and by the Earth Institute, the Graduate School of Arts and Sciences, and the Department of Ecology, Evolution, and Environmental Biology at Columbia University and the Botanical Society of America to JH.
Conflict of Interest Statement
The authors declare that the research was conducted in the absence of any commercial or financial relationships that could be construed as a potential conflict of interest.
Acknowledgments
We thank the New York City Department of Parks and Recreation for granting permits for this research. Thank you to Maya Tiwari and Minul Asgar for field, lab, and greenhouse assistance. We thank Nicholas Gershberg and staff at the Arthur Ross Greenhouse at Barnard College for experiment assistance including set-up and insight; and the New York City Department of Parks and Recreation Greenbelt Native Plant Center and Citywide Nursery for providing plant material.
We thank all organizations and individuals that provided green roof access and project support: Rebecca Marshall (Javits Center), Cecilia de Corral and Gwen Schantz (Brooklyn Grange), Max Lerner (NYC Parks), Vicki Santos (PS 40), Suzanne Mcgillicuddy (Fashion Institute of Technology), Guillermo Fernandez, Kinsley Jabouin (Saint Simon Stock School), Karen Argenti, Michelle Forcella (Silvercup Studios), Marni Majorelle (Alive Structures), Niki Jackson (NYC Audubon), Mike Dimarino (Linda Tool) Dustin Partridge (NYC Audubon), Howie Waldman and John Baglio (Ethical Culture Fieldson School), Chris Brunner (New York Green Roofs), The Point Community Development Corporation, Chris Goode, Wild Project Theater, and NYC Parks Rec Center staff.
Supplementary Material
The Supplementary Material for this article can be found online at: https://www.frontiersin.org/articles/10.3389/fevo.2019.00198/full#supplementary-material
Figure S1. The experimental design, detailing plant species, soil inocula, and abiotic stress treatments.
References
Aloisio, J. M., Palmer, M. I., Giampieri, M. A., Tuininga, A. R., and Lewis, J. D. (2016). Spatially dependent biotic and abiotic factors drive survivorship and physical structure of green roof vegetation. Ecol. Appl. 27, 297–308. doi: 10.1002/eap.1444
Anderson, M. J. (2001). A new method for non-parametric multivariate analysis of variance. Austral Ecol. 26, 32–46. doi: 10.1046/j.1442-9993.2001.01070.x
Augé, R. M. (2001). Water relations, drought and vesicular-arbuscular mycorrhizal symbiosis. Mycorrhiza 11, 3–42. doi: 10.1007/s005720100097
Augé, R. M., Toler, H. D., and Saxton, A. M. (2015). Arbuscular mycorrhizal symbiosis alters stomatal conductance of host plants more under drought than under amply watered conditions: a meta-analysis. Mycorrhiza 25, 13–24. doi: 10.1007/s00572-014-0585-4
Azcón-Aguilar, C., and Barea, J. M. (1997). Arbuscular mycorrhizas and biological control of soil-borne plant pathogens–an overview of the mechanisms involved. Mycorrhiza 6, 457–464. doi: 10.1007/s005720050147
Bever, J. D., Dickie, I. A., Facelli, E., Facelli, J. M., Klironomos, J., Moora, M., et al. (2010). Rooting theories of plant community ecology in microbial interactions. Trends Ecol. Evol. 25, 468–478. doi: 10.1016/j.tree.2010.05.004
Bever, J. D., Platt, T. G., and Morton, E. R. (2012). Microbial population and community dynamics on plant roots and their feedbacks on plant communities. Annu. Rev. Microbiol. 66, 265–283. doi: 10.1146/annurev-micro-092611-150107
Borowicz, V. A. (2001). Do arbuscular mycorrhizal fungi alter plant-pathogen relations? Ecology 82, 3057–3068. doi: 10.1890/0012-9658(2001)082[3057:DAMFAP]2.0.CO;2
Camargo-Ricalde, S. (2002). Dispersal, distribution and establishment of arbuscular mycorrhizal fungi: a review. Bot Sci. 71, 33–34. doi: 10.17129/botsci.1661
Caporaso, J. G., Kuczynski, J., Stombaugh, J., Bittinger, K., Bushman, F. D., Costello, E. K., et al. (2010). QIIME allows analysis of high-throughput community sequencing data. Nat. Methods 7, 335–336. doi: 10.1038/nmeth.f.303
Chaudhary, V. B., Rua, M. A., Antoninka, A., Bever, J. D., Cannon, J., Craig, A., et al. (2016). MycoDB, a global database of plant response to mycorrhizal fungi. Sci. Data 3:160028. doi: 10.1038/sdata.2016.28
De Caceres, M., and Legendre, P. (2009). Associations between species and groups of sites: indices and statistical inference. Ecology 90, 3566–3574. doi: 10.1890/08-1823.1
Declerck, S., D'or, D., Cranenbrouck, S., and Boulengé, L. E. (2001). Modelling the sporulation dynamics of arbuscular mycorrhizal fungi in monoxenic culture. Mycorrhiza 11, 225–230. doi: 10.1007/s005720100124
Dunnett, N., and Kingsbury, N. (2008). Planting Green Roofs and Living Walls. Portland, OR: Timber Press.
el Zahar Haichar, F., Marol, C., Berge, O., Rangel-Castro, J. I., Prosser, J. I., Balesdent, J., et al. (2008). Plant host habitat and root exudates shape soil bacterial community structure. ISME J. 2, 1221–1230. doi: 10.1038/ismej.2008.80
Fulthorpe, R., MacIvor, J. S., Jia, P., and Yasui, S. E. (2018). The green roof microbiome: improving plant survival for ecosystem service delivery. Front. Ecol. Evol. 6:5. doi: 10.3389/fevo.2018.00005
Glick, B. R. (2012). Plant growth-promoting bacteria: mechanisms and applications. Scientifica 2012:963401. doi: 10.6064/2012/963401
John, J., Kernaghan, G., and Lundholm, J. (2017). The potential for mycorrhizae to improve green roof function. Urban Ecosyst. 20, 113–127. doi: 10.1007/s11252-016-0573-x
John, J., Lundholm, J., and Kernaghan, G. (2014). Colonization of green roof plants by mycorrhizal and root endophytic fungi. Ecol. Eng. 71, 651–659. doi: 10.1016/j.ecoleng.2014.08.012
Kowalchuk, G. A., Buma, D. S., de Boer, W., Klinkhamer, P. G. L., and van Veen, J. A. (2002). Effects of above-ground plant species composition and diversity on the diversity of soil-borne microorganisms. Anton. Leeuw. Int. J G. 81, 509–520. doi: 10.1023/A:1020565523615
Lambers, H., Chapin III, F. S., and Pons, T. L. (2008). Plant Physiological Ecology, 2nd Edn. New York, NY: Springer-Verlag.
Lenoir, I., Fontaine, J., and Sahraoui, A. L. H. (2016). Arbuscular mycorrhizal fungal responses to abiotic stresses: a review. Phytochemistry 123, 4–15. doi: 10.1016/j.phytochem.2016.01.002
Lopez-Garcia, A., Azcon-Aguilar, C., and Barea, J. M. (2014). The interactions between plant life form and fungal traits of arbuscular mycorrhizal fungi determine the symbiotic community. Oecologia 176, 1075–1086. doi: 10.1007/s00442-014-3091-7
Lundholm, J., MacIvor, J. S., MacDougall, Z., and Ranalli, M. (2010). Plant species and functional group combinations affect green roof ecosystem functions. PLoS ONE 5:e9677. doi: 10.1371/journal.pone.0009677
Ma, Y., Rajkumar, M., Zhang, C., and Freitas, H. (2016). Beneficial role of bacterial endophytes in heavy metal phytoremediation. J. Environ. Manage. 174, 14–25. doi: 10.1016/j.jenvman.2016.02.047
Madriz-Ordeñana, K., Jørgensen, H. J. L., Nielsen, K. L., and Thordal-Christensen, H. (2017). First report of Kalanchoe leaf and stem spot caused by Corynespora cassiicola in Denmark. Plant Dis. 101, 505–505. doi: 10.1094/PDIS-08-16-1173-PDN
McDonald, D., Price, M. N., Goodrich, J., Nawrocki, E. P., DeSantis, T. Z., Probst, A., et al. (2012). An improved Greengenes taxonomy with explicit ranks for ecological and evolutionary analyses of bacteria and archaea. ISME J. 6, 610–618. doi: 10.1038/ismej.2011.139
McGuire, K. L., Payne, S. G., Orazi, G., and Palmer, M. I. (2015). “Bacteria and fungi in green roof ecosystems,” in Green Roof Ecosystems, ed R. K. Sutton (New York, NY: Springer International Publishing), 175–191.
McGuire, K. L., Payne, S. G., Palmer, M. I., Gillikin, C. M., Keefe, D., Kim, S. J., et al. (2013). Digging the New York City skyline: soil fungal communities in green roofs and city parks. PLoS ONE 8:e58020. doi: 10.1371/journal.pone.0058020
McMurdie, P. J., and Holmes, S. (2013). phyloseq: an R package for reproducible interactive analysis and graphics of microbiome census data. PLoS ONE 8:e61217. doi: 10.1371/journal.pone.0061217
Molineux, C. J., Connop, S. P., and Gange, A. C. (2014). Manipulating soil microbial communities in extensive green roof substrates. Sci. Total Environ. 493, 632–638. doi: 10.1016/j.scitotenv.2014.06.045
Monterusso, M. A., Rowe, D. B., and Rugh, C. L. (2005). Establishment and persistence of Sedum spp. and native taxa for green roof applications. HortScience 40, 391–396.
Nagase, A., and Dunnett, N. (2010). Drought tolerance in different vegetation types for extensive green roofs: effects of watering and diversity. Landsc. Urban Plan. 97, 318–327. doi: 10.1016/j.landurbplan.2010.07.005
Newsham, K. K., Fitter, A. H., and Watkinson, A. R. (1995). Arbuscular mycorrhiza protect an annual grass from root pathogenic fungi in the field. J. Ecol. 83, 991–1000. doi: 10.2307/2261180
Nguyen, N. H., Song, Z., Bates, S. T., Branco, S., Tedersoo, L., Menke, J., et al. (2015). FUNGuild: an open annotation tool for parsing fungal community datasets by ecological guild. Fungal Ecol. 20, 241–248. doi: 10.1016/j.funeco.2015.06.006
Parkins, K., and Clark, J. (2015). Green roofs provide habitat for urban bats. Glob. Ecol. Conserv. 4, 349–357. doi: 10.1016/j.gecco.2015.07.011
Partridge, D., and Clark, J. (2018). Urban green roofs provide habitat for migrating and breeding birds and their arthropod prey. PLoS ONE 13:e0202298. doi: 10.1371/journal.pone.0202298
Pregitzer, C. C., Charlop-Powers, S., Bibbo, S., Forgione, H. M., Gunther, B., Hallett, R. A., et al. (2019). A city-scale assessment reveals that native forest types and overstoryspecies dominate New York City forests. Ecol. Appl. 29:e01819. doi: 10.1002/eap.1819
Reynolds, H. L., Packer, A., Bever, J. D., and Clay, K. (2003). Grassroots ecology: plant-microbe-soil interactions as drivers of plant community structure and dynamics. Ecology 84, 2281–2291. doi: 10.1890/02-0298
Rodriguez, R. J., Redman, R. S., and Henson, J. M. (2004). The role of fungal symbioses in the adaptation of plants to high stress environments. Mitig. Adapt. Strateg. Glob. Chang. 9, 261–272. doi: 10.1023/B:MITI.0000029922.31110.97
Rumble, H., Finch, P., and Gange, A. C. (2018). Green roof soil organisms: anthropogenic assemblages or natural communities? Appl. Soil Ecol. 57, 197–204. doi: 10.1016/j.apsoil.2018.01.010
Saikia, S. P., Goswami, A., and Mudoi, K. D. (2014). Effect of 2, 4-D treatment and Azospirillum inoculation on growth of Cymbopogon winterianus. Afr. J Microbiol. Res. 8, 955–960. doi: 10.5897/AJMR2013.6130
Santos-Gonzalez, J. C., Finlay, R. D., and Tehler, A. (2007). Seasonal dynamics of arbuscular mycorrhizal fungal communities in roots in a seminatural grassland. Appl. Environ. Microbiol. 73, 5613–5623. doi: 10.1128/AEM.00262-07
Singh, B. K., Nunan, N., Ridgway, K. P., McNicol, J., Young, J. P. W., Daniell, T. J., et al. (2008). Relationship between assemblages of mycorrhizal fungi and bacteria on grass roots. Environ. Microbiol. 10, 534–541. doi: 10.1111/j.1462-2920.2007.01474.x
Smalla, K., Wieland, G., Buchner, A., Zock, A., Parzy, J., Kaiser, S., et al. (2001). Bulk and rhizosphere soil bacterial communities studied by denaturing gradient gel electrophoresis: plant-dependent enrichment and seasonal shifts revealed. Appl. Environ. Microbiol. 67, 4742–4751. doi: 10.1128/AEM.67.10.4742-4751.2001
Sýkorová, Z., Ineichen, K., Wiemken, A., and Redecker, D. (2007). The cultivation bias: different communities of arbuscular mycorrhizal fungi detected in roots from the field, from bait plants transplanted to the field, and from a greenhouse trap experiment. Mycorrhiza 18, 1–14. doi: 10.1007/s00572-007-0147-0
Takebayashi, H., and Moriyama, M. (2007). Surface heat budget on green roof and high reflection roof for mitigation of urban heat island. Build. Environ. 42, 2971–2979. doi: 10.1016/j.buildenv.2006.06.017
van der Heijden, M. G. A., Bakker, R., Verwaal, J., Scheublin, T. R., Rutten, M., Van Logtestijn, R., et al. (2006). Symbiotic bacteria as a determinant of plant community structure and plant productivity in dune grassland. FEMS Microbiol. Ecol. 56, 178–187. doi: 10.1111/j.1574-6941.2006.00086.x
van der Heijden, M. G. A., Klironomos, J. N., Ursic, M., Moutoglis, P., Streitwolf-Engel, R., Boller, T., et al. (1998). Mycorrhizal fungal diversity determines plant biodiversity, ecosystem variability and productivity. Nature 396, 69–72. doi: 10.1038/23932
van Loon, L. C., Bakker, P., and Pieterse, C. M. J. (1998). Systemic resistance induced by rhizosphere bacteria. Annu. Rev. Phytopathol. 36, 453–483. doi: 10.1146/annurev.phyto.36.1.453
VanWoert, N. D., Rowe, D. B., Andresen, J. A., Rugh, C. L., and Xiao, L. (2005). Watering regime and green roof substrate design affect Sedum plant growth. HortScience 40, 659–664.
Wagner, L., Stielow, B., Hoffmann, K., Petkovits, T., Papp, T., Vágvölgyi, C., et al. (2013). A comprehensive molecular phylogeny of the Mortierellales (Mortierellomycotina) based on nuclear ribosomal DNA. Persoonia 30, 77–93. doi: 10.3767/003158513X666268
Wang, B. X., Seiler, J. R., and Mei, C. S. (2016). A microbial endophyte enhanced growth of switchgrass under two drought cycles improving leaf level physiology and leaf development. Envr. Exper. Bot. 122, 100–108. doi: 10.1016/j.envexpbot.2015.09.004
Wang, Q., Garrity, G. M., Tiedje, J. M., and Cole, J. R. (2007). Naive Bayesian classifier for rapid assignment of rRNA sequences into the new bacterial taxonomy. Appl. Environ. Microbiol. 73, 5261–5267. doi: 10.1128/AEM.00062-07
Wehner, J., Powell, J. R., Muller, L. A. H., Caruso, T., Veresoglou, S. D., Hempel, S., et al. (2014). Determinants of root-associated fungal communities within Asteraceae in a semi-arid grassland. J. Ecol. 102, 425–436. doi: 10.1111/1365-2745.12197
Wickham, H., and François, R. (2015). dplyr: A Grammar of Data Manipulation. R package version 0.43.
Wolf, D., and Lundholm, J. T. (2008). Water uptake in green roof microcosms: effects of plant species and water availability. Ecol. Eng. 33, 179–186. doi: 10.1016/j.ecoleng.2008.02.008
Xie, L., Lehvävirta, S., Timonen, S., Kasurinen, J., Niemikapee, J., and Valkonen, J. P. T. (2018). Species-specific synergistic effects of two plant growth-promoting microbes on green roof plant biomass and photosynthetic efficiency. PLoS ONE 13:e0209432. doi: 10.1371/journal.pone.0209432
Yang, J., Kloepper, J. W., and Ryu, C. M. (2009). Rhizosphere bacteria help plants tolerate abiotic stress. Trends Plant Sci. 14, 1–4. doi: 10.1016/j.tplants.2008.10.004
Keywords: urban ecosystem services, green roof, AM fungi, plant-growth promoting bacteria, plant-microbial interactions
Citation: Hoch JMK, Rhodes ME, Shek KL, Dinwiddie D, Hiebert TC, Gill AS, Salazar Estrada AE, Griffin KL, Palmer MI and McGuire KL (2019) Soil Microbial Assemblages Are Linked to Plant Community Composition and Contribute to Ecosystem Services on Urban Green Roofs. Front. Ecol. Evol. 7:198. doi: 10.3389/fevo.2019.00198
Received: 21 February 2019; Accepted: 14 May 2019;
Published: 11 June 2019.
Edited by:
Jeremy Lundholm, Saint Mary's University, CanadaReviewed by:
Alan Gange, Royal Holloway, University of London, United KingdomErin Cameron, Saint Mary's University, Canada
Copyright © 2019 Hoch, Rhodes, Shek, Dinwiddie, Hiebert, Gill, Salazar Estrada, Griffin, Palmer and McGuire. This is an open-access article distributed under the terms of the Creative Commons Attribution License (CC BY). The use, distribution or reproduction in other forums is permitted, provided the original author(s) and the copyright owner(s) are credited and that the original publication in this journal is cited, in accordance with accepted academic practice. No use, distribution or reproduction is permitted which does not comply with these terms.
*Correspondence: Jessica M. K. Hoch, amgzNzU4QGNvbHVtYmlhLmVkdQ==