- 1School of BioSciences, The University of Melbourne, Melbourne, VIC, Australia
- 2School of Life Sciences, Jinggangshan University, Ji'an, China
- 3Research School of Finance, Actuarial Studies and Statistics, Australian National University, Canberra, ACT, Australia
Task allocation ensures a high level of organization within social insect colonies. Workers reveal their task assignment through cuticular hydrocarbon (CHC) signals. The source and chemical composition of these signals are largely unknown. We ask whether task recognition signals are located on particular body parts of workers of Australian meat ants (Iridomyrmex purpureus). We analyzed the CHC profile on the antennae, legs, and abdomens of workers engaged in different tasks. Discriminant analysis showed that the leg profile is the best indicator of task identification. Behavioral assays confirmed this finding: workers typically reacted differently to non-nestmates engaged in different tasks, but not if the CHCs on the legs of their opponents were removed by a solvent. Lasso and Elastic-Net Regularized Generalized Linear Model (GLMNET) revealed which CHC components show the highest correlation in task and nestmate recognition, suggesting that social insects can simultaneously convey different CHC signals on different body parts, thereby allowing efficient signaling and signal perception.
Introduction
Social insect colonies are self-organized, with social cohesion achieved by individual workers making simple decisions based on their interactions with other workers (Sachs, 2004). A well-developed task discrimination signal system is crucial because it allows workers to determine the tasks of others, assess the needs of the colony (Gordon and Mehdiabadi, 1999; Sachs, 2004) and respond accordingly (Wagner et al., 1998; Gordon and Mehdiabadi, 1999). Understanding how the labor force is distributed in social insect colonies therefore requires some knowledge of the nature and source of these signals (Anderson and McShea, 2001). For example, the mechanism of reproductive tasks signaled by queen mandibular pheromone is well understood because the chemical components and their site of synthesis in mandibular glands are clearly defined, allowing experimental manipulation of behavior using synthetic chemicals (Dietemann et al., 2003; Strauss et al., 2008; Kocher and Grozinger, 2011; Nunes et al., 2014).
Cuticular hydrocarbons (CHCs) are the most abundant chemicals on the surface of ants, and play key roles in several communication contexts, including nestmate recognition (Martin et al., 2008; van Wilgenburg et al., 2010), task recognition (Wagner et al., 1998; Greene and Gordon, 2003; Nascimento et al., 2013), trail marking (Hölldobler et al., 2004), recruitment of foragers (Greene and Gordon, 2003), and queen viability and fertility signals (Cuvillier-Hot et al., 2004; Lommelen et al., 2006; van Oystaeyen et al., 2014). The role of CHCs in communicating tasks is poorly understood. The main challenge to understanding these signals is to identify their chemical components. This requires establishing a bioassay that provides a consistent measure of signal perception; identifying the source of the signal; and synthesizing the putative signaling hydrocarbons. In ants, the level of aggression provides a precise measure of task perception, because workers engaged in different tasks not only exhibit different levels of aggression toward non-nestmates (Sturgis and Gordon, 2013), but also elicit different levels of aggression from non-nestmates (Nascimento et al., 2013). Typically, studies of chemical signals use coupled gas chromatography mass spectrometry (GCMS) to identify the CHC profile obtained from the entire body of the insect: the “whole body” profiles of individuals engaged in different tasks are then compared using principal components and/or discriminant analyses (Heinze et al., 2002; van Wilgenburg et al., 2006; Lenoir et al., 2009; Martin and Drijfhout, 2009b). While these studies reveal broad differences in CHC profiles across different tasks (Wagner et al., 1998; Kaib et al., 2000; Nascimento et al., 2013; Sturgis and Gordon, 2013), and that cocktails of CHCs elicit different behaviors (Wagner et al., 1998; Greene and Gordon, 2003), the identity of the chemicals responsible for signaling different tasks remain unknown. The list of putative chemicals may be reduced substantially if signals indicating task activities, like colony identification signals, are located on specific body parts (see Wang et al., 2016).
Like many ants, task allocation in the Australian meat ant Iridomyrmex purpureus is largely determined by an age polyethism. Newly emerged workers usually tend the brood inside the nest, gradually switching to tasks outside the nest, including maintaining the nest surface (nest maintainers), foraging for food (foragers), and engaging in ritualized display behavior (displayers) with neighboring colonies (van Wilgenburg et al., 2005). Ants can also respond to changes in the ambient environment, allowing individuals to adjust their behavior according to the specific needs of the colony (Gordon and Mehdiabadi, 1999). For example, foragers and nest maintainers may form a line of defense by displaying in large numbers when the colony is under siege from neighbors. The ritualized display behavior is unambiguous, and forms part of a repertoire of increasingly aggressive behaviors directed toward non-nestmates (van Wilgenburg et al., 2005, 2006). Display does not usually escalate into other aggressive behavior, such as biting or fighting and rarely results in injury or death.
Following Wang et al. (2016), who showed that workers of I. pupureus paid more attention to the antennae of non-nestmates and the legs of nest-mate workers, we investigate the role of location specific CHCs in signaling task allocation in I. purpureus. Specifically, we compare the CHC profile across different body parts of workers engaged in different tasks. We use behavioral assays to identify which body part profiles are most informative of task activity, and experimentally washed chemicals from the putative body part to confirm the role of that profile. Finally, we use GLMNET (Friedman et al., 2009) to identify the variation in CHC components that correlate best with the variation in task discrimination and nestmate recognition signals. GLMNET is a fast and reliable method of selecting variables, and resolves the problem where the number of chemical components is larger than the number of samples, a common issue for studies of complex chemical signal mixtures.
Materials and Methods
Collection of Workers
We sourced Australian meat ants (Iridomyrmex purpureus) from colonies located in Serendip Sanctuary, 60 km south-west of Melbourne, Victoria, Australia. All samples were collected between January and March 2015. For chemical analyses and behavior assays, workers of different tasks were collected from 12 distant mature colonies (six colony pairs, colonies of each pair were at least 300 meters apart) while they were performing specific tasks, kept temporarily in clean glass containers, and used within 1 h after collecting to avoid any changes in surface signals. Nest maintainers were collected when they were carrying or moving pebbles on the surface of the nests; foragers were collected from returning workers on foraging trails with food (insect parts) in their mandibles. The displayers were collected where workers were engaged in ritualized display behavior. To avoid collecting ants from the neighboring colonies, each displayer was placed with four workers from the home colony, and only individuals showing no antagonistic behavior were included.
Cuticular Hydrocarbon (CHC) Analysis
The chemical components were identified and confirmed with gas chromatography-mass spectrometry (GC-MS) following Wang et al. (2016). We compared the colony and task CHC profile variation of different body parts by collecting 15 ants (five individuals of each of three tasks) from each of four colonies (n = 60). These individuals were freeze-immobilized within 20 min of collection, and their antennae, heads, legs, and abdomens were dissected with a pair of surgical scissors. Individual body parts were immersed in 200 μL hexane (HPLC grade) for 10 min to extract CHCs, dried under a nitrogen flow and redissolved with 10 μL hexane including tridecane (100 mg/L) as an internal standard. To analyse the abundance of each component in the signals, 1 μL samples were injected into an Agilent 7820A gas-chromatograph with a split/splitless injector and flame ionization detector (GC–FID) and a Zebron ZB-5 capillary column (Phenomenex, USA; 30 m, 0.25 mm in diameter, 0.25 μm film thickness). The oven temperature was raised from 75 to 260°C at 10°C/min, then raised to 325°C at 5°C/min and held for the last 5 min. The injection temperature was 250°C, and detector temperature was 325°C. Helium was used as the carrier gas at a flow rate of 1.0 ml/min. The Agilent ChemStation (E. 02.02) was used to acquire the GC-FID data, and peak area was integrated using the default setting. We minimized the effects of contamination by including only those peaks that exceed 0.1% of the total peak area, and had a peak area larger than 20 μV/s in the analysis. Peak area is usually much larger than 20 μV/s, and anything smaller could not be distinguished reliably from the baseline. As a result, 24 peaks were identified in the samples of antennae and 47 peaks in the leg and abdomen samples. The identification of CHC components follows a previous study on this species (van Wilgenburg et al., 2006), but GC-MS was also used to confirm each component. The GC-MS analysis was carried out on an Agilent 5975C quadrupole mass spectrometer coupled directly with an Agilent 7890A GC and fitted with a Zebron ZB-5MS column. The MS transfer line was set at 280°C, the ion source adjusted to 250°C and the quadrupole operated at 150°C, with the oven program the same as the GC-FID. Helium was used as the carrier gas at a flow rate of 0.8 ml/min.
Task Determination by Body Size
After hexane extraction, the heads of the workers were preserved for measurements. We measured the width of the heads as body length and body weight vary with the different nutrient history of each individual (van Wilgenburg et al., 2005). Workers of I. purpureus can store nectar in their crops, which could affect their body length and weight. The heads of the individuals were placed on an Epson 7520 scanner, and scanned with a reference scale. The width of each head was then measured from the scanned picture with Nano Measurer 1.2. A total of 257 ants of the three tasks from seven colonies were analyzed.
Behavioral Assays
Discrimination of Tasks
To test whether workers of I. purpureus can discriminate the tasks of other workers, we conducted two-on-two aggression assays in containers (7 cm by 10 cm) lined with fluon (Dreier et al., 2007). The assays took place between 10 a.m. and 4 p.m., when the ants are most active. We collected workers from 12 colonies, which were assigned into six colony pairs. For each pair of colonies, foragers from one colony were selected as focal ants because their task and colony origins were easy to identify and they were likely to have encountered different tasks of other nests. The ants were marked individually on the dorsal surface of their thorax with small dots of enamel paint, and allowed to acclimatize in a holding container for at least 5 min. The two focal workers were then placed in the assay container, and two unmarked nest maintainers, foragers, or displayers, were then gently placed into the container. We recorded the behavior of the marked, focal ants toward the stimulus ants, and the behavior of the stimulus ants toward the focal ants for 3 min, using a Panasonic HDC-SD80 video camera. The video was then replayed in slow motion, allowing us to record the number of antennations of the focal individuals toward different body parts of their opponents and the duration of the display behavior. Following Wang et al. (2016), we included antennations toward the antennae and legs only, because we rarely saw antennation toward the thorax, which in any case had been marked with identifying paint. We recorded the behavior of 108 focal workers and 108 stimulus workers, each comprising three trials (six individuals) for each of the three combinations (focal foragers with stimulus nest maintainers, foragers, and displayers), for each of the six colony pairs. We measured the response to non-nestmates because workers of I. purpureus rarely respond aggressively toward nestmates. The behavior was recorded and observed blind to the task and colony source of the opposing ants.
Location of Task Specific Signals
To test whether the CHCs on the legs of I. purpureus convey task specific signals, we removed the signals on their legs using a method similar to that published previously (Wang et al., 2016). Frontal femurs of ants of both treatments were amputated to prevent them from grooming, which might restore the chemical signals on legs and antennae (Lucas et al., 2004; Boroczky et al., 2013). After freeze anesthetization at −20°C for 3 min, either one side or both sides of the mid and hind legs of the experimental workers were carefully immersed in cold hexane in a small glass vial for at least 1 min to remove most CHCs from the legs. Hexane was always carefully kept away from direct contact with the abdomen or thorax of ants, as this could have killed them. Ants with signals from one side removed were used as controls for the manipulation and the impact of the hexane on the mobility of ants. After the operation, ants were allowed to recover in clean containers for at least 15 min before behavior assays, and only individuals that showed no obvious loss of mobility were selected for behavior assays. We placed two marked, focal foragers from one colony of each colony pair (n = 5 pairs of colonies) into the assay container with two manipulated nest maintainers, foragers, or displayers from the other colony, and recorded the behavior of the marked, focal ants for 2 min. Each of the two treatments was replicated four times between five colony pairs and three combinations (n = 240). 92.5% (37 out of 40 individuals) of the remaining hexane treated ants were alive 24 h after the tests, and 95.0% (38 out of 40 individuals) of the untreated ants survived after the tests, indicating that our protocols did not significantly reduce the viability of ants during the test. The antennation behavior and the duration of display behavior were recorded with the observer blind to the colony source of the opposing ants and/or the treatment.
Statistical Analyses
Task Discrimination by Body Size
Differences in body size between colonies or task groups were investigated using least squares ANOVA with colony membership and task groups as main effects. These analyses were conducted using JMP version 9 (SAS Institute).
Chemical Analyses
The relative proportions of each quantifiable peak area to that of the total sample were calculated using the following formula: Proportion of Area = Ai/∑An, where Ai = area of peak i, and ∑An = total peak area, and these transformed peak areas were used for subsequent analyses. We used principal components analysis (PCA) with the transformed peak areas to reduce the number of describing variables for a discriminant function analysis (DA). We extracted the resulting components with eigenvalues which together explained at least 85% of the total variance. We then performed discriminant analysis with the scores produced by the PCA to investigate variation between body-part specific profiles, and whether colonies or tasks could be separated on the basis of the hydrocarbon profiles of different body parts. PCA and DA were conducted using JMP version 9 (SAS Institute). Unless stated otherwise, values are means ± SE.
Behavioral Analyses
Differences in the frequency of antennation and the duration of display behavior in the task discrimination test were analyzed separately for the focal workers and for the stimulus workers, using mixed effects models with task (displayer, forager, or nest maintainer) as a main effect and colony identity as a random effect, with the variance partitioned using restricted maximum likelihood (REML). We used a mixed effects model to investigate how experimental manipulation of signals located on the legs influenced the duration of display behavior, using task (displayer, forager, or nest maintainer), and treatment (three vs. six legs washed) as main effects and colony identity as a random effect, with the variance partitioned using REML. Where appropriate, we used post-hoc t-tests. All of these analyses were conducted using JMP version 13 (SAS Institute).
Signaling Components
Generalized Linear Modeling is an extension of regression models designed to deal with error distributions beyond the normal distribution. Lasso and Elastic-Net Regularized Generalized Linear Model (GLMNET) fits a Generalized Linear Model via penalized maximum likelihood. The regularization path is computed for the lasso or elasticnet penalty at a grid of values for the regularization parameter lambda. GLMNET is efficient in determining the most important elements in a matrix. We conducted this analysis in the R package Glmnet Vignette (Friedman et al., 2009). For task specific signals, we assigned the number 1–3 to nest maintainers, foragers and displayers, respectively, as the response vectors (y). The relative proportions of CHCs on the legs were used as the input matrix (x). After running command cv.glmnet(x, y, family = “multinomial,” type.multinomial = “grouped”), we calculated the coefficient of each component using coef(cvfit, s = “lambda.min”) command. This model generated a group of coefficients for each task. The command coef(cvfit, s = “lambda.1se”) gives the most regularized model such that error is within one standard error of the minimum, which generates another set of components with a smaller number of effective components (Supplementary Table 1). Discriminant analysis of colonies and tasks were conducted using these components. The components with no coefficient are those that have little effect in explaining the task variation. The components with positive coefficients are those that positively correlated to the response, and hence ants with a higher concentration of this component have a high probability of being classified into this task. The components with negative coefficient indicate that ants with a higher content of this component have a lower probability of being classified into this task or colony. The importance of each component is correlated with the absolute value of each coefficient. Similarly, we used colony identity as the response vectors (y), and the relative proportion of CHCs on the antennae as the input matrix (x) to calculate the coefficients for nest-mate recognition signals. The selected components were used to run an additional DA to discriminate colony or task variations using the protocols described above.
Results
Task and Colony Discriminations According to Body-Part Profiles
The chemical profiles of CHCs on the antennae, legs and abdomens differ according to the task of the worker (Figures 1A–C), but the legs provide qualitatively greater task discrimination accuracy (81.4% correct classification) than either the antennae (71.7% correct classification) or abdomens (69.5% correct classification). The chemical profiles of the three body parts also show clear colony discrimination (antennae: 78.3% correct classification, legs: 84.8% correct classification, abdomens: 72.3% correct classification; Supplementary Figures S1A–C).
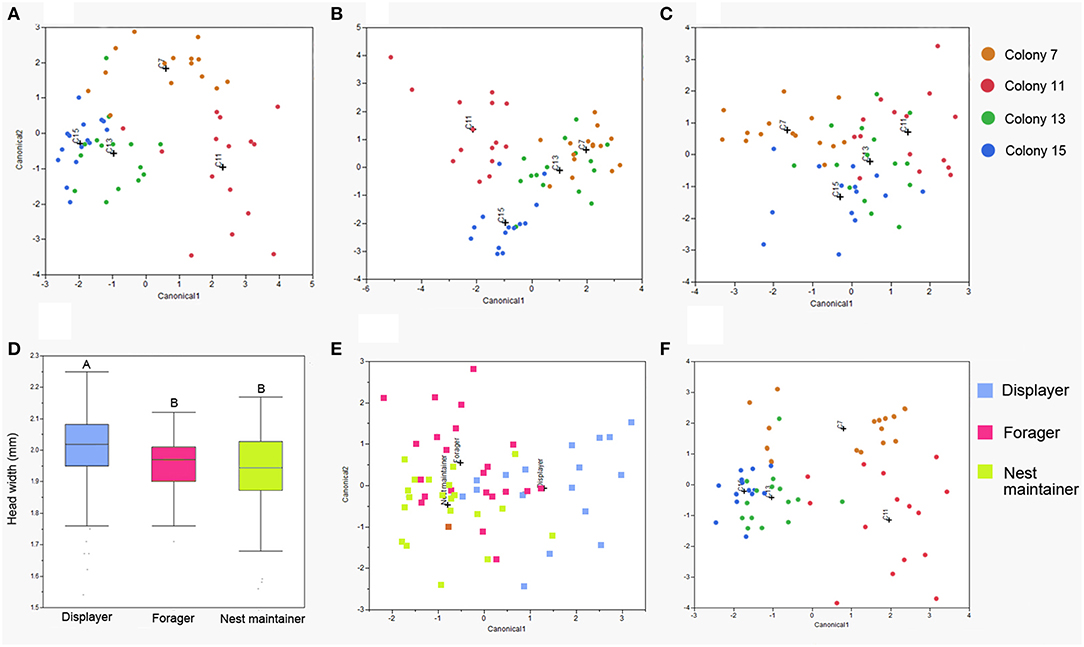
Figure 1. Discriminant analysis of the CHCs on different body parts and body size variation between tasks. Task discriminant analysis plots of (A) antennae, correctly classifies 71.7% individuals, (B) legs, correctly classifies 81.4% individuals, and (C) abdomens, correctly classifies 69.5% individuals. (D) Displaying workers are slightly larger than workers engaged in other tasks: box covers 25–75% quartiles and whiskers complete the range of values; different letters indicate significantly (p < 0.05) different levels. (E) Task and (F) colony discriminant analysis plots using the components selected by GLMNET from legs (correctly classifies 78.0% individuals) and antennae (correctly classifies 86.4% individuals). + marks the centroid of each group.
Task Allocation and Body Size
The head width of workers, used as a measure of body size, differs significantly according to worker task [F(2, 250) = 12.29, p < 0.0001; REML variance component estimate of colony identity: Wald p = 0.1025, 27.2% of the total variance; Figure 1D]. However, body size seems unlikely to be used as a cue for task discrimination because the effect size is very small (displayers head width 1.994 ± 0.012 mm, foragers 1.955 ± 0.010 mm, nest maintainers 1.939 ± 0.011 mm). While task discrimination can be linked with body size (for example, physical castes) it is also associated with chemical signals (Kaib et al., 2000) and sensory structures associated with chemical communication (Wittwer and Elgar, 2018).
Workers Can Discriminate Tasks
Our behavior assays indicate that workers of I. purpureus vary their response to non-nestmates according to the task of their opponent, rather than their own task. The frequency of antennation behavior varied according to the task of the stimulus worker [F(2, 107) = 35.43, p < 0.0001; REML Variance component estimate of colony identity: Wald p = 0.1433, 39.5% of the total variance], with the highest antennation frequency directed toward displayers and foragers than to nest maintainers (Figure 2A). The duration of display behavior of the focal worker varied according to the task of the stimulus worker [F(2, 100) = 14.393, p < 0.0001; REML Variance component estimate of colony identity: Wald p = 0.144, 40.6% of the total variance]. In contrast, the behavior of the stimulus workers did not vary with the task they were undertaking [F(2, 100) = 1.01, p = 0.37; REML Variance component estimate of colony identity: Wald p = 0.155, 33.2% of the total variance; Figure 2B]. Thus, workers of I. purpureus react differently to different tasks of non-nestmate workers, regardless of their own tasks.
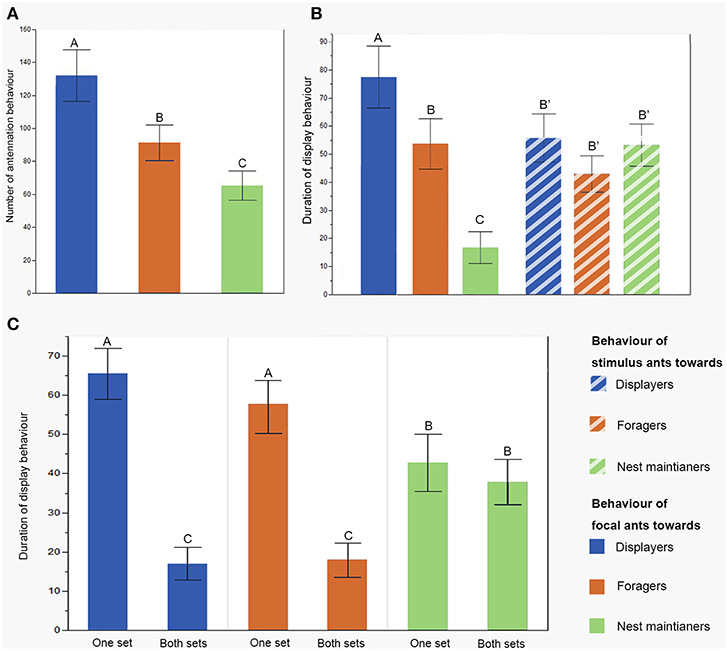
Figure 2. Antennation and aggression behavior of the focal ants toward ants of the three task groups. (A) The variation in antennation frequency of the focal ants toward the three task groups; (B) the duration of display behavior of the focal ants toward the three stimulus task groups, and the stimulus ants toward the focal ants. (C) Focal ants cannot discriminate tasks when the CHCs on the legs were removed in displayers and foragers, as the level of aggression was significantly reduced (tasks: F2, 242 = 0.416, p = 0.6605; leg wash: F1, 242 = 53.56, p < 0.0001; task × leg wash: F2, 242 = 9.506, p = 0.0001; error bars indicate SEM, different letters signify significantly different levels).
Task Discrimination Signals Are Located on the Legs
The different responses of focal workers to stimulus workers may reflect differences in the behavior of the stimulus workers, rather than a capacity to perceive different CHC profiles and respond appropriately. We compared the duration of display behaviors by focal ants toward non-nestmate stimulus workers that had been engaged in nest maintenance, foraging or displaying, and whose CHCs had been removed on the legs of either one (three legs) or both (six legs) sides of their body. The variation in the duration of display behavior was explained by a significant task × experimental treatment interaction term [F(2, 226) = 12.420, p < 0.0001; REML Variance component estimate of colony identity: Wald p = 0.1882, 22.9% of the total variance; Figure 2C]. Specifically, the mean duration of displays with forager and displayer stimulus workers was significantly lower when six than three legs had been washed. However, the signal removal treatment had no effect on the mean duration of displays with nest maintainer workers. These results indicate that the variation in the response of the focal ant arises through differences in the chemical signal rather than the behavior of the stimulus workers.
Identification of Active Compounds
We used GLMNET to identify which compounds contributed the most to distinguishing between the CHC profiles on the legs of workers engaged in different tasks. This analysis identified 21 compounds from the CHC profile of the leg samples, including 16 branched, and five linear types that are inferred to be important in explaining task variation. The same analysis identified 17 compounds from the antennal profile that possibly serve as colony identity signals, including 12 branched alkanes, and five linear types (Table 1). The importance of each component is correlated with the absolute value of each coefficient, the components with a positive coefficient indicate that ants with a higher content of this component have a high probability of being classified into this task or colony, and the components with a negative coefficient indicate that ants with a higher content of this component have a lower probability of being classified into this task or colony. Interestingly, task and colony recognition signals have very different active components, with only nine overlapping in both signals. On the other hand, the overlap in the compounds identified for task and colony identity signals indicate that ants from different colonies may use the same set of compounds to convey specific signals.
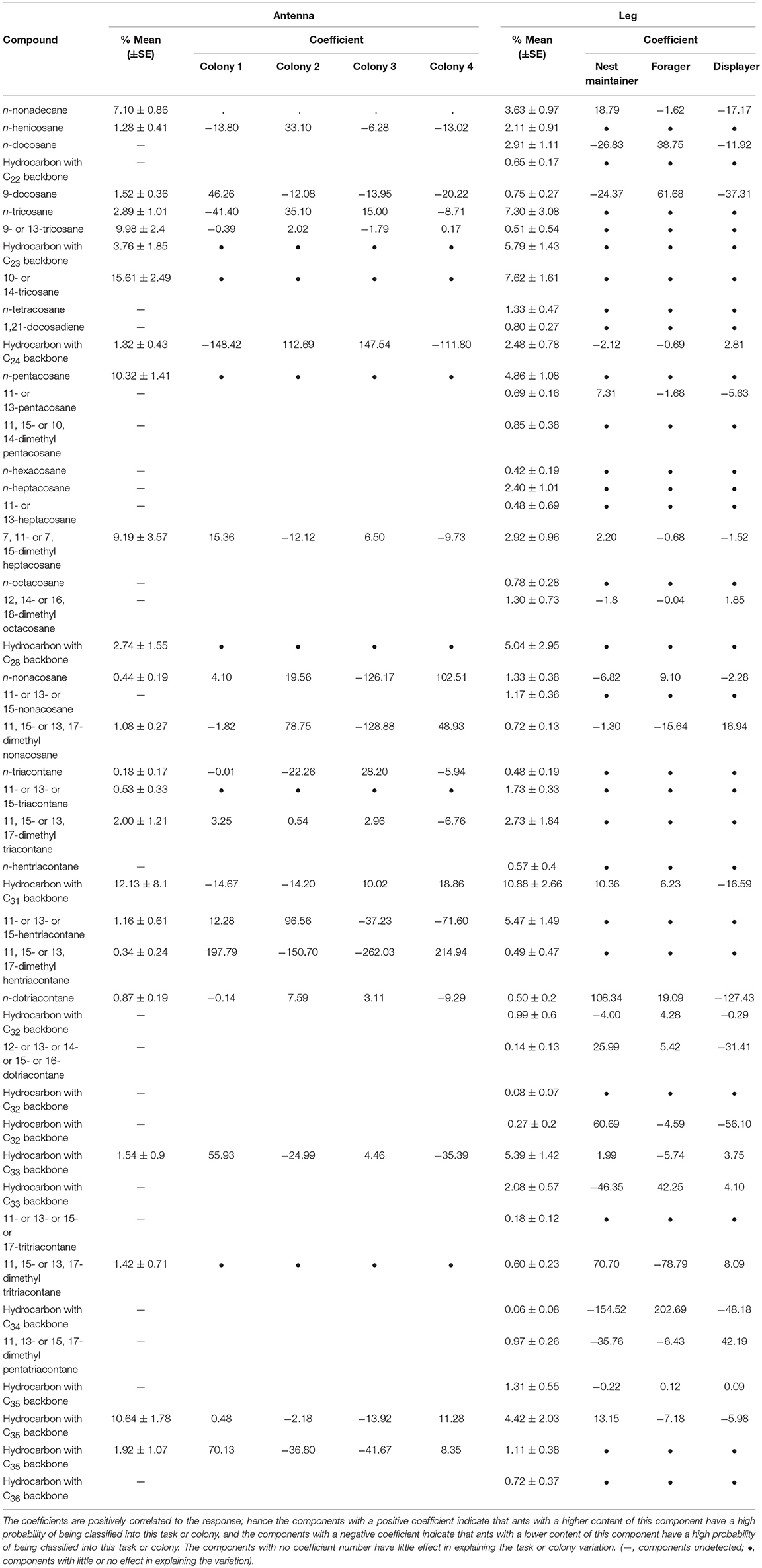
Table 1. GLMNET coefficient of compounds on the antennae and legs of Iridomyrmex purpureus that explain task and nestmate variation.
Confining the DA analysis to only the components identified by GLMNET yielded broadly similar discrimination success: 86.4% of the individuals were classified into the right tasks using selected leg CHCs (Figure 1E), and 78.0% of the individuals were classified into the right colony using selected antennal CHCs (Figure 1F; Supplementary Material).
Discussion
Workers of I. purpureus can perceive task recognition signals of non-nestmate workers, and respond accordingly. Not only are the CHC profiles of the antennae, legs, and abdomens different (Wang et al., 2016), but they also differ according to the workers' task. This ability is lost when the CHCs on the legs are experimentally removed, indicating that these CHCs are vital for task recognition. The chemical components contributing the most to task and colony recognition signals are significantly different. Further analysis using only these particular chemical components shows improved statistical success in task discrimination and similar colony discrimination. This suggests that different signals can be conveyed by a limited number of particular components of the CHCs, greatly narrowing the search to identify the cuticle-based chemicals that represent task and other recognition signals.
The ability to discriminate between non-nestmate workers according to their task allows workers to respond to different levels of threat faced by the colony. Nest maintenance workers of I. purpureus pose little threat to other colonies because this task is undertaken close to the nest entrances. In contrast, foraging workers may threaten food resources, and thus pose a higher threat, while displayers, typically engaged in colony defense or offense, pose the greatest threat. The ability of workers to perceive displaying, non-nestmate workers, and respond appropriately, may be particularly important if display behavior provides a signal of individual or colony-level competitive ability (van Wilgenburg et al., 2005).
Our experiments indicate that the CHCs on the legs are crucial for task recognition in I. purpureus, expanding the evidence that cuticular-based signaling in social insects is likely to be location specific. Consistent with other task-specific, identity signals (e.g., the queen mandibular pheromone, Dietemann et al., 2003), task recognition signals do not appear to be colony specific. However, while the queens' task remains largely unchanged during her life, workers can change their task according to either long-term colony needs, or short-term emergencies (Gordon, 1989). It is unclear whether the task recognition signals change before or after a change in task, which raises the intriguing question of how the workers change the task recognition signals on their legs. Age induced hormone or gene expression changes are associated with activities of exocrine glands in social insects involved in different tasks (Lengyel et al., 2007; Ueno et al., 2015), and these changes could influence the CHCs that serve as task recognition signals (Lengyel et al., 2007). The environment may also influence task recognition signals. For example, the CHC profile on the legs of Lasius niger is similar to that on the surface of the nest (Lenoir et al., 2009): perhaps task recognition signals are at least partly derived from the surface that each insect commonly encounters whilst completing its task.
Studies that attempt to identify the chemical compounds involved in communication about task allocation have been dogged by the number of compounds present on the insect cuticle. Our statistical analyses have proved helpful in identifying which components are most closely associated with particular tasks, and thus help narrow the range of potential compounds that may act as task recognition and nest identity signals. The chemical components identified by GLMNET as possible candidates for signaling compounds include mostly branched hydrocarbons, which is consistent with other lines of evidence suggesting that this class of hydrocarbons are likely to have signaling functions (Martin et al., 2008; Martin and Drijfhout, 2009a; van Wilgenburg et al., 2010, 2012). However, this does not indicate that the chemical components highlighted by GLMNET are the actual signals used by the ants: experimental results using synthesized components are still needed to confirm the results. Interestingly, the vast majority of the chemicals with high coefficient values are clearly differentiated between the two signal functions, despite the overlap of the CHC profile across body parts. This suggests that not all components revealed by whole body samples represent signals (Hölldobler et al., 2004; Martin et al., 2008), an arrangement that likely minimizes any signal ambiguity because it allows ants to perceive particular signals from targeted sources, rather than having to distinguish signals from a complex pool of CHCs, as implied in other insects (Chin et al., 2014). Further, these data highlight the importance of recognizing that candidate CHC signals may be location-specific, and are thus obscured by conventional methods of analyzing the CHCs extracted from the entire insect cuticle.
Ethics Statement
No animal ethics approval was required for this study, and permission was granted to conduct behavioral assays at Serendip Sanctuary.
Author Contributions
QW and ME: conceived and designed the study. QW: performed behavioral assays. QW, JG, and IW: conducted the chemical analysis and interpretation. LC, QW, and ME: conducted the statistical analyses. QW and ME: drafted the manuscript. QW, JG, IW, LC, and ME: edited and revised the manuscript.
Funding
This study was support by the Australian Research Council (DP120100162 to ME, LP150100798 to IW), and a University of Melbourne Postgraduate Scholarship and a Holsworth Wildlife Research Endowment (to QW).
Conflict of Interest Statement
The authors declare that the research was conducted in the absence of any commercial or financial relationships that could be construed as a potential conflict of interest.
Acknowledgments
We thank Matt Wills of Parks Victoria for his enthusiastic support at Serendip Sanctuary, and Y. Wang for her help with the field experiments.
Supplementary Material
The Supplementary Material for this article can be found online at: https://www.frontiersin.org/articles/10.3389/fevo.2019.00227/full#supplementary-material
References
Anderson, C., and McShea, D. W. (2001). Individual versus social complexity, with particular reference to ant colonies. Biol. Rev. 76, 211–237. doi: 10.1017/S1464793101005656
Boroczky, K., Wada-Katsumata, A., Batchelor, D., Zhukovskaya, M., and Schal, C. (2013). Insects groom their antennae to enhance olfactory acuity. Proc. Natl. Acad. Sci. U.S.A. 110, 3615–3620. doi: 10.1073/pnas.1212466110
Chin, J. S. R., Ellis, S. R., Pham, H. T., Blanksby, S. J., Mori, K., Koh, Q. L., et al. (2014). Sex-specific triacylglycerides are widely conserved in Drosophila and mediate mating behavior. Elife 3:e01751. doi: 10.7554/eLife.01751
Cuvillier-Hot, V., Lenoir, A., Crewe, R., Malosse, C., and Peeters, C. (2004). Fertility signalling and reproductive skew in queenless ants. Anim. Behav. 68, 1209–1219. doi: 10.1016/j.anbehav.2003.11.026
Dietemann, V., Peeters, C., Liebig, J., Thivet, V., and Hölldobler, B. (2003). Cuticular hydrocarbons mediate discrimination of reproductives and nonreproductives in the ant Myrmecia gulosa. Proc. Natl. Acad. Sci. U.S.A. 100, 10341–10346. doi: 10.1073/pnas.1834281100
Dreier, S., van Zweden, J. S., and D'Ettorre, P. (2007). Long-term memory of individual identity in ant queens. Biol. Lett. 3, 459–462. doi: 10.1098/rsbl.2007.0224
Friedman, J., Hastie, T., and Tibshirani, R. (2009). Glmnet: Lasso and Elastic-net Regularized Generalized Linear Models. R package version 1.
Gordon, D. M. (1989). Dynamics of task switching in harvester ants. Anim. Behav. 38, 194–204. doi: 10.1016/S0003-3472(89)80082-X
Gordon, D. M., and Mehdiabadi, N. J. (1999). Encounter rate and task allocation in harvester ants. Behav. Ecol. Sociobiol. 45, 370–377. doi: 10.1007/s002650050573
Greene, M. J., and Gordon, D. M. (2003). Social insects - cuticular hydrocarbons inform task decisions. Nature 423, 32–32. doi: 10.1038/423032a
Heinze, J., Stengl, B., and Sledge, M. F. (2002). Worker rank, reproductive status and cuticular hydrocarbon signature in the ant, Pachycondyla cf. inversa. Behav. Ecol. Sociobiol. 52, 59–65. doi: 10.1007/s00265-002-0491-1
Hölldobler, B., Morgan, E. D., Oldham, N. J., Liebig, J., and Liu, Y. (2004). Dufour gland secretion in the harvester ant genus Pogonomyrmex. Chemoecology 14, 101–106. doi: 10.1007/s00049-003-0267-8
Kaib, M., Eisermann, B., Schoeters, E., Billen, J., Franke, S., and Francke, W. (2000). Task-related variation of postpharyngeal and cuticular hydrocarbon compositions in the ant Myrmicaria eumenoides. J. Comp. Physiol. A 186, 939–948. doi: 10.1007/s003590000146
Kocher, S. D., and Grozinger, C. M. (2011). Cooperation, conflict, and the evolution of queen pheromones. J. Chem. Ecol. 37, 1263–1275. doi: 10.1007/s10886-011-0036-z
Lengyel, F., Westerlund, S. A., and Kaib, M. (2007). Juvenile hormone III influences task-specific cuticular hydrocarbon profile changes in the ant Myrmicaria eumenoides. J. Chem. Ecol. 33, 167–181. doi: 10.1007/s10886-006-9185-x
Lenoir, A., Depickere, S., Devers, S., Christides, J. P., and Detrain, C. (2009). Hydrocarbons in the ant Lasius niger: from the cuticle to the nest and home range marking. J. Chem. Ecol. 35, 913–921. doi: 10.1007/s10886-009-9669-6
Lommelen, E., Johnson, C. A., Drijfhout, F. P., Billen, J., Wenseleers, T., and Gobin, B. (2006). Cuticular hydrocarbons provide reliable cues of fertility in the ant Gnamptogenys striatula. J. Chem. Ecol. 32, 2023–2034. doi: 10.1007/s10886-006-9126-8
Lucas, C., Pho, D. B., Fresneau, D., and Jallon, J. M. (2004). Hydrocarbon circulation and colonial signature in Pachycondyla villosa. J. Insect Physiol. 50, 595–607. doi: 10.1016/j.jinsphys.2004.04.006
Martin, S. J., and Drijfhout, F. P. (2009a). Nestmate and task cues are influenced and encoded differently within ant cuticular hydrocarbon profiles. J. Chem. Ecol. 35, 368–374. doi: 10.1007/s10886-009-9612-x
Martin, S. J., and Drijfhout, F. P. (2009b). How reliable is the analysis of complex cuticular hydrocarbon profiles by multivariate statistical methods? J. Chem. Ecol. 35, 375–382. doi: 10.1007/s10886-009-9610-z
Martin, S. J., Vitikainen, E., Helantera, H., and Drijfhout, F. P. (2008). Chemical basis of nest-mate discrimination in the ant Formica exsecta. Proc. Roy. Soc. B 275, 1271–1278. doi: 10.1098/rspb.2007.1708
Nascimento, F. S., Tannure-Nascimento, I. C., Dantas, J. O., Turatti, I. C., and Lopes, N. P. (2013). Task-related variation of cuticular hydrocarbon profiles affect nestmate recognition in the giant ant Dinoponera quadriceps. J. Insect Behav. 26, 212–222. doi: 10.1007/s10905-012-9353-5
Nunes, T. M., Mateus, S., Favaris, A. P., Amaral, M. F. Z. J., von Zuben, L. G., Clososki, G. C., et al. (2014). Queen signals in a stingless bee: suppression of worker ovary activation and spatial distribution of active compounds. Sci. Rep. 4:7449. doi: 10.1038/srep07449
Sachs, T. (2004). Self-organization of tree form: a model for complex social systems. J. Theor. Biol. 230, 197–202. doi: 10.1016/j.jtbi.2004.05.006
Strauss, K., Scharpenberg, H., Crewe, R. M., Glahn, F., Foth, H., and Moritz, R. F. A. (2008). The role of the queen mandibular gland pheromone in honeybees (Apis mellifera): honest signal or suppressive agent? Behav. Ecol. Sociobiol. 62, 1523–1531. doi: 10.1007/s00265-008-0581-9
Sturgis, S. J., and Gordon, D. M. (2013). Aggression is task dependent in the red harvester ant (Pogonomyrmex barbatus). Behav. Ecol. 24, 532–539. doi: 10.1093/beheco/ars194
Ueno, T., Takeuchi, H., Kawasaki, K., and Kubo, T. (2015). Changes in the gene expression profiles of the hypopharyngeal gland of worker honeybees in association with worker behavior and hormonal factors. PLoS ONE 10:20. doi: 10.1371/journal.pone.0130206
van Oystaeyen, A., Oliveira, R. C., Holman, L., van Zweden, J. S., Romero, C., Oi, C. A., et al. (2014). Conserved class of queen pheromones stops social insect workers from reproducing. Science 343, 287–290. doi: 10.1126/science.1244899
van Wilgenburg, E., Felden, A., Choe, D. H., Sulc, R., Luo, J., Shea, K. J., et al. (2012). Learning and discrimination of cuticular hydrocarbons in a social insect. Biol. Lett. 8, 17–20. doi: 10.1098/rsbl.2011.0643
van Wilgenburg, E., Ryan, D., Morrison, P., Marriott, P. J., and Elgar, M. A. (2006). Nest- and colony-mate recognition in polydomous colonies of meat ants (Iridomyrmex purpureus). Naturwissenschaften 93, 309–314. doi: 10.1007/s00114-006-0109-y
van Wilgenburg, E., Sulc, R., Shea, K. J., and Tsutsui, N. D. (2010). Deciphering the chemical basis of nestmate recognition. J. Chem. Ecol. 36, 751–758. doi: 10.1007/s10886-010-9812-4
van Wilgenburg, E., van Lieshout, E., and Elgar, M. A. (2005). Conflict resolution strategies in meat ants (Iridomyrmex purpureus): ritualised displays versus lethal fighting. Behaviour 142, 701–716. doi: 10.1163/1568539054729150
Wagner, D., Brown, M. J. F., Broun, P., Cuevas, W., Moses, L. E., Chao, D. L., et al. (1998). Task-related differences in the cuticular hydrocarbon composition of harvester ants, Pogonomyrmex barbatus. J. Chem. Ecol. 24, 2021–2037. doi: 10.1023/A:1020781508889
Wang, Q., Goodger, J. Q. D., Woodrow, I. E., and Elgar, M. A. (2016). Location-specific cuticular hydrocarbon signals in a social insect. Proc. R. Soc. B 283, 20160310. doi: 10.1098/rspb.2016.0310
Keywords: social insects, task recognition, cuticular hydrocarbons, body parts, GLMNET
Citation: Wang Q, Goodger JQD, Woodrow IE, Chang L and Elgar MA (2019) Task-Specific Recognition Signals Are Located on the Legs in a Social Insect. Front. Ecol. Evol. 7:227. doi: 10.3389/fevo.2019.00227
Received: 04 December 2018; Accepted: 31 May 2019;
Published: 02 July 2019.
Edited by:
Heikki Helanterä, University of Oulu, FinlandReviewed by:
Brian R. Johnson, University of California, Davis, United StatesDavid Richard Nash, University of Copenhagen, Denmark
Copyright © 2019 Wang, Goodger, Woodrow, Chang and Elgar. This is an open-access article distributed under the terms of the Creative Commons Attribution License (CC BY). The use, distribution or reproduction in other forums is permitted, provided the original author(s) and the copyright owner(s) are credited and that the original publication in this journal is cited, in accordance with accepted academic practice. No use, distribution or reproduction is permitted which does not comply with these terms.
*Correspondence: Qike Wang, d2FuZ3Fpa2UxMjNAZ21haWwuY29t