- 1Department of Vertebrate Ecology and Zoology, Faculty of Biology, University of Gdansk, Gdansk, Poland
- 2Laboratory of Ornithology, Cornell University, Ithaca, NY, United States
- 3Escuela de Biología, Universidad de Costa Rica, San José, Costa Rica
- 4Institute of Oceanology, Polish Academy of Sciences, Sopot, Poland
An increasing number of studies report coordinated chick provisioning by avian parents. Although the pattern of parental coordination varies across species, broad occurrence of this coordination suggests that it has an adaptive value: it may increase individual fitness via higher offspring survival, faster offspring growth rate and/or higher body reserves of the parents. However, to what extent the pattern of coordinated provisioning in a species represents a flexible response to current foraging conditions remains an open question. Here, we examined coordination of chick provisioning in the Little Auk (Alle alle), a planktivorous seabird species that breeds in the Arctic. Harsh environmental conditions impose bi-parental care on this species, and high variability within and across breeding seasons promotes flexibility in parental involvement to secure breeding success. During the chick rearing period, parents exhibit a dual-foraging strategy (i.e., alternating long foraging trips, serving to maintain the adults' body reserves, with several short trips aimed to provision the chick). We examined coordination of parental provisioning across five breeding seasons varying in terms of environmental conditions and found that the parents indeed coordinate their provisioning, avoiding performing long trips simultaneously and thus enabling a more even distribution of feeding through time. We also examined chick body condition in relation to the level of parental coordination to test the potential adaptive value of coordination, but we found no significant relationship between these two parameters. We found high variability in the level of the coordination between pairs, and this variability was similar across all study seasons, which represented a wide range of experienced environmental conditions. Nevertheless, we found that the energy density of food loads delivered to chicks was associated with the level of parental coordination: when conditions were characterized by the delivery of higher-energy food loads, the level of coordination exhibited by the studied population was higher. These findings suggest that environmental conditions somehow affect parental coordination, but the range of the environmental variation could be still below a critical threshold of extreme conditions that would trigger more pronounced modifications of parental foraging patterns and coordination.
Introduction
Ecological conditions associated with food availability and predatory pressure are among the most important determinants of benefits and costs of parental care in birds and are therefore thought to play an important role in the evolution of avian breeding systems (Silver et al., 1985; Martin, 1987; Arnold and Duvall, 2002; Fontaine and Martin, 2006; but see Olson et al., 2008; Remeš et al., 2015). At the evolutionary scale, environments characterized by mild and/or predictable conditions are associated with the system of uniparental care (8% of avian species) while environments with harsher or unpredictable conditions seem to require the involvement of both parents, and sometimes even help from other individuals, in order to raise the offspring successfully (81 and 9% of species, respectively representing bi-parental and cooperative breeding systems; see Cockburn, 2006). Ecological constraints or hazards faced by parents may also operate at a narrower scale, for instance shaping the extent of each parent's engagement and the manner in which they perform their care.
A growing number of studies highlight the importance of subtle partner inter-play in the form of coordinated parental performance (Hinde, 2006; Johnstone and Hinde, 2006; Elliott et al., 2010; Raihani et al., 2010; Massoni et al., 2012; van Rooij and Griffith, 2013; Johnstone et al., 2014; Mariette and Griffith, 2015; Bebbington and Hatchwell, 2016; Tyson et al., 2017; Wojczulanis-Jakubas et al., 2018). Patterns of parental coordination may vary across groups, species and even breeding stages (e.g., alternated vs. intermittent incubation, alternated vs. overlapped feeding patterns, etc.), but overall, coordination of efforts by both breeding partners may substantially increase their reproductive success (e.g., Davis, 1988; Raihani et al., 2010; Mariette and Griffith, 2015). This seems to be particularly important in extreme ecological conditions. A good example is the Kentish Plover, Charadrius alexandrines, which breeds in a hot desert where coordinated incubation between parents is essential for egg survival and also helps the parents to cope with their own heat stress (AlRashidi et al., 2010). However, coordination per se is relatively rarely examined, and studies examining the issue in the context of environmental constraints are even more scarce.
Life-history traits of pelagic polar seabirds make them a particularly interesting ecological group in terms of parental care on the background of environmental conditions. Their harsh and highly variable environment poses a great challenge during the breeding period when, in addition to self-maintenance, the parents need to satiate the needs of their offspring. Many species are known to exhibit flexible strategies to buffer environmental variability until conditions reach a critical threshold beyond which they are unable to buffer suboptimal conditions without visible changes in their survival and/or breeding success. As such, seabirds are often used as binary bio-indicators of environmental conditions (Piatt et al., 2007). In addition, foraging on distant marine resources, which are often patchily distributed (Schreiber and Burger, 2002), forces seabird parents to spend prolonged periods of time away from the nest (for hours or even days, e.g., Congdon et al., 2005; Welcker et al., 2009). Low ambient temperature imposes additional constraints for the parents, as embryos or young can be exposed to risks of death from hypothermia if left unattended for too long. All of these factors promote parental cooperation in seabirds and indeed, all the pelagic seabirds exhibit an obligatory bi-parental care system (Schreiber and Burger, 2002). Importantly, seabirds have been found to coordinate their food provisioning in a way that may potentially increase their breeding success (Congdon et al., 2005; Tyson et al., 2017; Wojczulanis-Jakubas et al., 2018). Nevertheless, substantial variation in the level of coordinated provisioning has been observed in these seabirds, and it raises the interesting question of the extent to which this coordination is a plastic response of parents to foraging conditions. If the coordination is a flexible trait, it should vary with regard to the current foraging context, with two possible scenarios. First, unfavorable foraging conditions could hamper the coordination as each parent faces the challenge of self-maintenance in a way that causes coordination to fail. Alternatively, unfavorable conditions could enhance the coordination if the coordination only has an adaptive value under such challenging circumstances (e.g., regularly provisioning the offspring may compensate for low food quality; Jones, 2002). The question about the relationship between the coordination and environmental conditions is particularly valid in the context of ongoing global warming, when dramatic changes in distribution of ocean currents impose additional constraints on entire marine ecosystems, including seabirds (e.g., Wassmann et al., 2011; Frederiksen et al., 2013).
Here, we examine foraging patterns and food provisioning schemes of breeding partners in the Little Auk (or Dovekie, Alle alle) in two breeding colonies across five breeding seasons. The Little Auk is a small pelagic seabird, breeding exclusively in the High Arctic zone. It is long-lived, with long-term pair bonds and long and extensive bi-parental care of a single egg/chick annually (Stempniewicz, 2001). Parents equally share their incubation duty for 4 weeks (Wojczulanis-Jakubas et al., 2009) and both brood and feed the chick at a similar rate for 3–4 weeks (Harding et al., 2004). Importantly, the Little Auk exhibits a dual-foraging strategy during the chick rearing period, regularly alternating a few short trips in a row (up to 8 h each, serving solely to provision the offspring) with a long foraging trip (> 8 h up to 28 h, primarily serving adult self-maintenance, even though some food is also brought to the chick; see Welcker et al., 2009, 2012; Wojczulanis-Jakubas et al., 2010; Jakubas et al., 2012). This pattern seems to be universal as no evidence of birds performing only one type of trip was found in five colonies located across the whole breeding range (Welcker et al., 2009). Thus, with both parents performing this bimodal foraging strategy, a mismatch between partners can have consequences for breeding success, as long trips by adults represent extended periods of waiting for food by the chick. In the worst-case scenario, when both parents make their long trips simultaneously, the chick may face a periodic risk of starvation. Even if an extended wait for food is not lethal, it may lead to energy allocation switching from growth to thermoregulation, resulting in prolonged growth (Ricklefs, 1990; Schreiber and Burger, 2002). Combined with life-history traits demonstrating the importance of both parents' role in successful breeding, the dual-foraging strategy makes the Little Auk a good model species for investigating coordinated efforts of breeding partners.
A recent study revealed that Little Auks indeed coordinate chick provisioning, avoiding simultaneous performance of long trips (Wojczulanis-Jakubas et al., 2018). A potential benefit of the coordination has also been demonstrated, as parents provisioning the chicks in a coordinated manner reduced the variation in the duration of periods when the chick is waiting for food (i.e., an even distribution of feedings through time). This study, however, was performed in a single breeding colony located at a relatively long distance from optimal foraging grounds, and thus the role of specific environmental conditions in shaping the coordinated provisioning remains unknown. It is known that the foraging patterns of the Little Auk depend on oceanographic conditions, with unfavorable conditions being associated with extension of the overall duration of foraging trips (Welcker et al., 2009; Jakubas et al., 2013; Hovinen et al., 2014; Kidawa et al., 2015). Therefore, it is possible that coordination performance may be different in another ecological context. Wojczulanis-Jakubas et al. (2018) also examined the effect of coordination on chick body condition but found no significant relationship. Why the coordination was not related to chick body condition, despite apparently favorable pattern of food delivery (i.e., reduced variation in duration of inter-feeding intervals), and whether coordination is associated with given environmental conditions, remains unclear.
The aim of the present study was two-fold. Firstly, we verified the results from the previous study (Wojczulanis-Jakubas et al., 2018) by extending the earlier dataset by adding new records from another large breeding colony and subsequent seasons. Furthermore, using a different approach to measure chick body condition, we also re-examined the relationship between parental coordination and chick growth rate. We expected to find a positive correlation, which would show another benefit of coordination and give insights into the adaptive value of coordinated provisioning. Secondly, we analyzed the parental coordination in regard to relevant environmental conditions. If coordination is a flexible trait varying in relation to foraging conditions, we expected to find variation in coordination level somehow associated with differences in environmental conditions.
Methods
Study Area
We carried out the study in two breeding colonies: Hornsund (SW Spitsbergen, 77°00′ N, 15°33′ E) and Magdalenefjorden (NW Spitsbergen, 79°35′ N, 11°05′ E; Figure 1). These two colonies constitute the core of the Little Auk breeding population on Svalbard (ca 590 000 breeding pairs in Hornsund and 18 000 in Magdalenefjorden; Keslinka et al., 2019). Given high gene flow between these two colonies, they could be treated as a single panmictic population (Wojczulanis-Jakubas et al., 2014). However, owing to their different location on the Svalbard archipelago, birds from these two colonies are exposed to different oceanographic conditions. Thus, examining the provisioning schemes in these two locations expands the range of environmental conditions. The sea shelf in the vicinity of Hornsund constitutes the main foraging area of the Little Auks from this colony (Jakubas et al., 2013, 2014; and see Figure 1). This area is typically under the influence of two currents: the coastal Sørkapp Current, which carries cold, less saline Arctic water, and the West Spitsbergen Current (an extension of the Norwegian Atlantic Current), which transports warmer, more saline Atlantic water (Piechura et al., 2001; Cottier et al., 2005). The contribution of the two currents varies among years with greater or smaller contribution from Arctic waters, which in turn creates more or less favorable foraging conditions for the local population of the Little Auk. The nearby sea shelf area in Magdalenefjorden (one of the foraging areas of Little Auks from Magdalenefjorden; Jakubas et al., 2013) is primarily supplied with warm Atlantic waters from the West Spitsbergen Current. The aforementioned area is also under the partial influence of Arctic waters from the Sørkapp Current (Cottier et al., 2005; Piechura and Walczowski, 2009) but the influx of cold waters varies greatly between years, creating in comparison with Hornsund generally less favorable foraging conditions and a greater challenge for the local population of the Little Auk (Jakubas et al., 2013; Kidawa et al., 2015). For these reasons, birds from Magdalenefjorden may also forage in the marginal ice zone despite its distance from the breeding grounds, as it seems to be more profitable foraging grounds than the waters in the close vicinity of the colony (Figure 1).
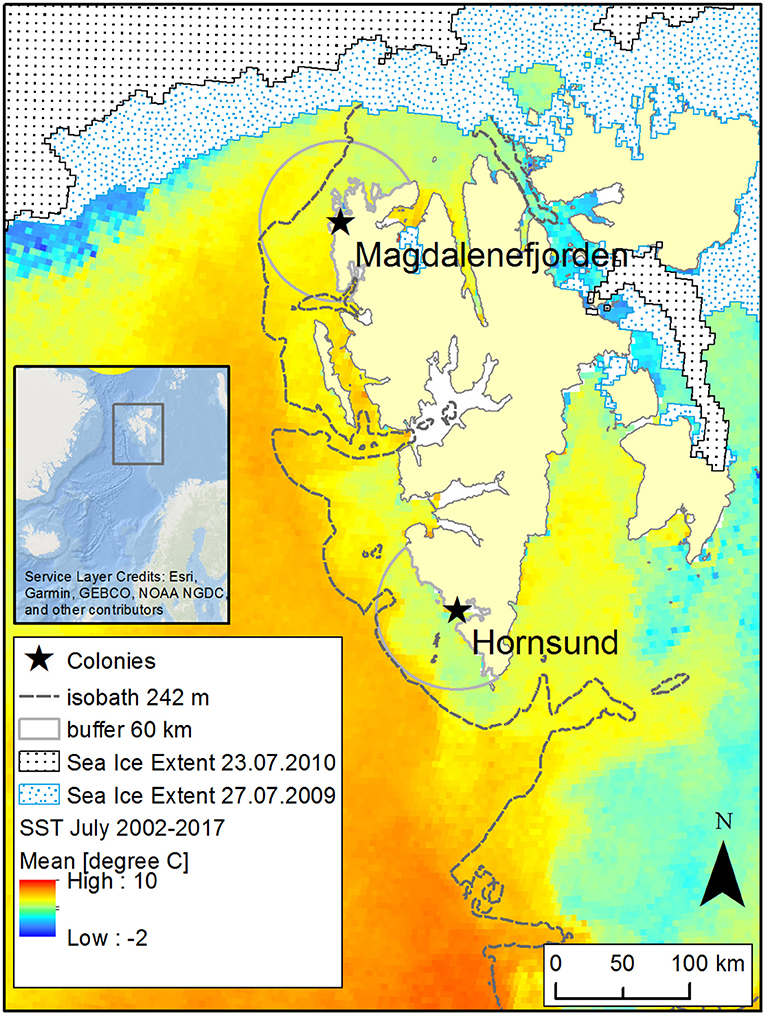
Figure 1. Study area. Mean sea surface temperature (SST) values for July 2002–2017; the 242-m isobath represents the shelf break and boundary of the Arctic zooplankton community (Kwaśniewski et al., 2012), and the 60-km buffers around the studied colonies represent close foraging grounds of Little Auks (Jakubas et al., 2017). Two example sea ice extents are shown for the dates when Little Auks were food sampled in both colonies. Data sources: SST: MODIS Aqua SST data (NASA Goddard Space Flight Center Ocean Biology Laboratory Ocean Biology processimg Group, 2014); sea ice extent: Multisensor Analyzed Sea Ice Extent - Northern Hemisphere (MASIE-NH), Version 1 with 4 215 km grid cell size (National Ice Center (NIC) NSIDC, 2010); bathymetry: a 500 m global relief model of Earth's surface IBCAO ver. 3 (Jakobsson et al., 2012).
Behavioral Observations
We collected data during three breeding seasons in the Little Auk colony at Hornsund (2016 to 2018) and two seasons in the colony at Magdalenefjorden (2009 and 2010). Data from Magdalenefjorden have been already used in Wojczulanis-Jakubas et al. (2018). Here, however, they are restricted to specific chick age, and analyzed along with data from Hornsund. The data from Hornsund are considered in this context for the first time.
To establish bird presence/absence in the colony (and later to obtain duration and time distribution of foraging trips needed to determine the coordination level) we used one of the two following bird monitoring systems: direct observation or video recording, carried out in three and two seasons, respectively (Table 1). The system of monitoring depended on field logistics and had slightly different accuracy. Nevertheless, obtained data were standardized in a way that ensured the two systems were comparable (see details below). To identify individuals, two weeks before the onset of the monitoring we marked both breeding partners from focal nests with a unique code using color combinations of leg-rings and color signs dyed on breast feathers (waterproof markers, Sharpie USA). The breast-signs usually faded away slightly throughout the monitoring period but were still clearly visible at the critical time, allowing quick and reliable individual identification in combination with the permanent colored leg-rings. In both systems we monitored nests of focal birds continuously for 48 h, and we could establish presence and absence of focal parents in the nest and its vicinity during this period with sufficient precision, owing to the nest site “fidelity” of Little Auks when at the colony (personal observations). The 48-h sessions (both observations and video recordings) were divided into 10-min bouts (assigned with presence or absence of focal birds) due to respective methodological constraints of both observation methods and to allow comparison of data originated from the two systems. In both systems, arrival of the parent at the colony with a food load for the chick was evident (indicated by fullness of the gular pouch). Consequently, we considered a sequence of the 10-min periods of absence of a focal bird in the colony, followed by its appearance with a full gular pouch, as a foraging trip.
During the direct observations, pairs of observers (changing every 6–8 h) watched the colony plot with the group of focal nests. The observations were carried out from a blind situated ca 20 m from the colony edge (ensuring minimal disturbance and securing identification of individually marked birds). The observers used binoculars (10 × 35) to confirm the birds' identity, if necessary. It was possible to follow all marked birds because all the focal nests were located relatively close to each other, and marked individuals were never all simultaneously on the plot. The nest areas were observed continuously and presence/absence of parents at a given nest and fullness of their gular pouch were noted every 10 min (owing to uncertainty of exact departure time and securing acceptable accuracy).
For automatic video recording, we set a video camera (in total four types, commercial HD models, with 1-s time lapse mode) at each focal nest separately. The cameras recorded the situation in a 3 m radius of the focal nest entrance. Thus, as for the direct observations, we were able to register presence/absence of parents at a given nest and fullness of their gular pouch. Despite the greater time-precision (1 s) of arrival at the nest, this system was less precise concerning arrival at the colony, due to spatial limitations of the camera frame. Presence/absence in the colony was assigned to every 10-min time-window because the birds returning from foraging trips usually enter the nest within the first 10 min after arrival at the colony (average latency = 7 min; unpublished data). Video material was processed using VLC software (VideoLAN, France) and QuickTime player (Apple Inc. USA).
To establish hatching date, nests under monitoring were checked every 2 days for the last week of the incubation, so we were able to adjust the timing of observation and video recording to the chick's age. Although dates of the observations/video recordings varied between the colonies and seasons, focal birds were phenologically all in the same stage of the chick rearing period, i.e., “mid” chick rearing period (7–18 days old chicks; Table 1). Parental coordination may possibly change with age, and homogeneity in chick age among study nests minimizes the variation within this confounding variable.
Determination of Coordination Levels
To establish coordination level within a pair, every 10-min time-window for each individual was assigned to one of four categories: ST – short trip, LT – long trip, CO – presence in the colony, X - unknown. We classified foraging trips as short trip (ST) or long trip (LT) following the method previously used by Welcker et al. (2009), where the best cut-off value to separate the trips is the one that minimizes the sum of variances of both trip types, given their log-normal distribution. We calculated the cut-off value separately for every season and obtained a mean cut-off value of 6.75 h (range: 5.85–7.1 h). At least one 10-min time-window classified as presence at the colony (CO) was always between two foraging trips. As some trips started or ended beyond the fixed 48 h observation/recording period, their duration could not be calculated, and we assigned such trips to the fourth category, unknown whereabouts (X), to avoid losing information when the partner's status was known at the same time.
In total, we obtained data for 81 pair-sessions, balanced between the 5 seasons and with a few repeated pairs across two seasons, and no pair repeated for more than two seasons (Table 1). To establish and test the coordination of provisioning, we followed the procedure applied in Wojczulanis-Jakubas et al. (2018). Thus, for each pair-session we first calculated the frequency of 10-min time-windows in which one pair member was on ST while the other was on LT. Then we tested significance of this frequency using a Monte Carlo randomization approach (i.e., randomization that does not necessarily generate all possible combinations; see Wojczulanis-Jakubas et al., 2018 for detailed information). This way we obtained a single p-value for every pair-observation. To obtain an overall p-value for the given data set, we used the Z-method using the R package metap (Dewey, 2019). Finally, we calculated the coordination index for every pair-observation as the proportional difference between the observed (obs) and expected (exp) proportion of 10-min time-windows in which one pair member was on ST while the other was on LT according to the respective randomization procedure ([obs-exp] x exp−1). The obtained index varied between −1.00 and 1.42, with positive values associated with apparent coordination in the sense we consider in the present study (i.e., avoiding overlap of LTs by the two partners), and values equal to 0 or negative corresponding to an absence of this type of coordination.
Coordination and Inter-feeding Intervals
To verify the relationship found by Wojczulanis-Jakubas et al. (2018) between coordination and the variation in duration of time-intervals between the feedings, we first calculated the coefficient of variation in the duration of inter-feeding intervals (CV = σ duration - μ duration). Then we fitted a linear mixed model with maximum likelihood using the R package lme4 (Bates et al., 2015), in which coordination index (calculated as described above) was the explanatory variable and CV was the response variable. Identity of the pair was also included in the model as a random effect (random intercept). The significance of the explanatory variable was tested with the Anova function using type III Wald Chi-square tests from the R package car (Fox and Weisberg, 2011).
Influence of Environmental Factors on Coordination Levels
To characterize environmental conditions for each season, we considered both biotic and abiotic parameters that are known to be important for foraging Little Auks: (1) total energy density of average food load brought to the chick [in kJ.g−1 dry weight (hereafter dw)]; a proxy of overall efficiency in chick provisioning, being a combination of food availability and parental foraging effort (see Kwaśniewski et al., 2010); (2) the ratio of abundance of the two food items which are considered crucial for the chick diet, i.e., Calanus glacialis being associated with cold Arctic waters (considered as the preferred food item), and its warm-water Atlantic counterpart Calanus finmarchicus; a proxy of efficiency in foraging on preferred food item (Kwaśniewski et al., 2010; Jakubas et al., 2011); (3) the Simpson Diversity Index of the food provided; another proxy of efficiency in foraging on preferred food items (Kwaśniewski et al., 2010); (4) sea surface temperature in the foraging areas (hereafter SST); a proxy of contribution of warm and cold waters, and thus availability of preferred zooplankton items associated with cold waters (Kwaśniewski et al., 2010, 2012).
We established diet parameters based on food samples (on average 41 samples per season; range: 20–65 samples) collected from gular pouches of adults arriving at the colony from a foraging trip during mid chick rearing period (see Wojczulanis et al., 2006; Kwaśniewski et al., 2010 for all the methods related to sample preservation and analysis). We calculated zooplankton dry weight and energy density according to Wojczulanis et al. (2006), Kwaśniewski et al. (2010) and literature therein. To avoid disturbing provisioning schemes of birds under observation/recording, the food samples were collected from different individuals, meaning that diet composition cannot be linked directly to coordination but may serve as a proxy of overall foraging efficiency in a given season. Therefore, we calculated average diet parameters per season, which were used for further analysis. Dates of diet samples collection are provided in Table S1.
We collected SST data for 60 km marine buffers around the studied colonies (after Jakubas et al., 2017) for the period when diet samples were collected from parental birds. We extracted data from the Moderate-resolution Imaging Spectroradiometer (MODIS) Aqua satellite data. We used Level 3 daytime SST data derived from 11 μm thermal IR infrared (IR) bands with a 4 km spatial resolution (see NASA Goddard Space Flight Center Ocean Biology Laboratory Ocean Biology processimg Group, 2014). We mainly used 8-day products from periods corresponding to the dates when we collected diet samples from Little Auks. However, in some cases, due to cloudy conditions, we used monthly composites for July or August (see details in Table S1). Therefore, an average value per season was then calculated and used for further analysis. We extracted all abiotic data from GIS data using ArcGIS software 10.3.1 (Redlands, CA, USA: Environmental Systems Research Institute).
Due to inherent limitations in obtaining biotic and abiotic environmental factors, parameters were averaged per season and were at a very different scale from coordination data (i.e., we had up to five different values for environmental parameters and 81 pair-level calculated coordination indices, thus all pairs from the same season had the same value of each predictor). Thus, we were not able to use those parameters directly in a linear model to explain variation in coordination index as such an approach would lead to artificial data multiplication for predictors. Instead, we chose to use the season as a proxy for environmental conditions. To do so, we needed to first verify whether the five seasons were truly different considering the chosen environmental parameters. For this purpose, we tested each of the environmental parameters separately, using raw values collected for each season, and applied Kruskal-Wallis non-parametric test with season as a grouping variable. As a post-hoc test, we used Mann–Whitney U-tests for all the pairwise comparisons. Then we modeled the previously calculated coordination index against the five seasons investigated, using a linear mixed model fitted with maximum likelihood including the identity of the pair as a random effect. Significance of the explanatory variable was tested using the Anova function. Following this analysis, multiple comparison post-hoc Tukey tests were performed to assess specific differences within the five studied seasons, using the glht function from the R package multcomp (Bretz and Westfall, 2008).
We also investigated the influence of environmental conditions on parental coordination by constructing a regression tree based on recursive partitioning using the R package rpart (Therneau and Atkinson, 2019). Recursive partitioning is a statistical method that examines the relationship between multiple explanatory variables and a single response variable using a recursive binary-partitioning process. It is particularly useful for identifying particular thresholds affecting the degree of response to variation of parameters when this response is expected to be more binary than linear. In this analysis, we used the pair-level coordination index as a response variable, and the seasonal average values of the four environmental parameters presented above were used as explanatory variables. These environmental parameters are thus used as general proxies for foraging conditions in each season. Rpart creates a decision tree classifying members of a given statistical population by splitting it into sub-populations based on the explanatory variables. The process is recursive because each sub-population may in turn be split an indefinite number of times until the subgroups either reach a minimum size or until no improvement can be made. The aim of splitting the data at each step is to establish groups that have a between-variation as large, and a within-variation as small, as possible. The second stage of the procedure consists of using cross-validation to trim back the full tree, based on a number of statistics calculated during the first step. Model outputs produce an “inverted tree,” in which the root at the top contains all observations, which is divided into two branches at the node. These branches can further be split into two subsequent nodes and so on. The nodes provide information about the explanatory variable name used for the split, and the value used for the split is represented on the branches. Each terminal node shows the size of the formed group (n) and the mean of the response variable for this group. Then we tested the significance of the differences between the groups created by each split using Mann–Whitney U-tests. To evaluate to what extent the tree splits along colony lines, we calculated the proportion of cases from the two colonies in each final group.
Effect of Coordination on Chick Body Condition
The previous paper on parental coordination in the Little Auk also examined the effect of coordination on chick body condition (Wojczulanis-Jakubas et al., 2018); however, the parameters describing body condition that were used in that study had some limitations, i.e., body mass was measured at several time-points during the second half of the chick rearing period, whereas coordination level was calculated for a restricted period of time. Thus, difference in time scales could blur the effect (see Wojczulanis-Jakubas et al., 2018 for more details). We used a different approach to examine the effect of parental coordination on chick body condition. We evaluated the relative change in chick body mass between the onset (mstart) and end of the observation/recording session (mend). For that purpose, we weighed the focal chicks in these two time-points (dstart, and dend) using an electronic balance (0.1 g accuracy; OHAUS, China) and calculated the daily relative change in chick body mass as [(mend – mstart) x x 100] / [dend - dstart]. The chosen age stage (7–18 days) corresponds to a phase of linear growth of the Little Auk chick (Konarzewski and Taylor, 1989), which should allow detection of changes during 48 h. In this analysis, we considered only data from Hornsund, the only location in which chicks were weighed in a systematic manner before and after observations. We used the daily relative change in chick body mass as a response variable in a linear mixed model fitted with maximum likelihood, with coordination index as an explanatory variable and the identity of the pair as a random effect. The statistical significance of the model was assessed using a type III Wald Chi-square test, as described above.
All analyses were carried out with R version 3.5.1 (R Core Team, 2018) and statistical significance was taken to be P < 0.05.
Results
Coordination Level and Inter-feeding Intervals
We found that the frequency of 10-min time-windows in which one pair member was on ST while the other was on LT was significantly greater than expected by chance according to the combined p-value from our Monte Carlo randomization tests (Z = 2.47, P = 0.007), indicating coordinated provisioning. The mean proportion of 10-min time-windows in which one pair member was on ST while the other was on LT was 22.7% (Interquartile range: 11.5–32.3%). Nevertheless, high variability could be observed between the pairs (Figure 2). We found a significant relationship between the coordination index and the variation of inter-feeding intervals (LMM, χ2 = 14.44, P = 0.0001), with a higher coordination being linked to a more even distribution of feedings through time (Figure 3).
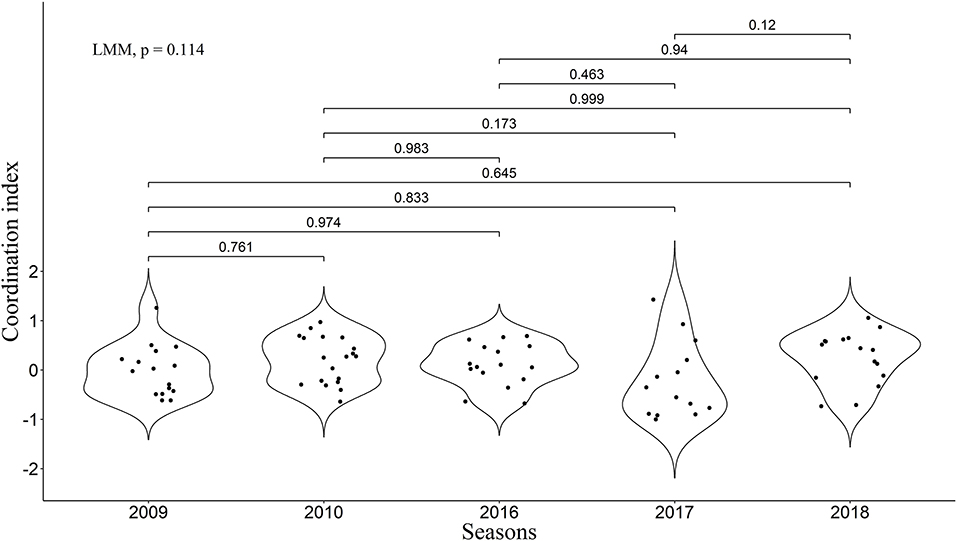
Figure 2. Coordinated index for all five seasons. Violin plots represent the distribution. P-values from inter-season comparisons made with Tukey tests are presented above the lines. Overall differences were statistically tested with linear mixed modeling. Positive values are associated with apparent coordination in the sense we consider in the present study (i.e., avoiding overlap of LTs by the two partners), and values equal to 0 or negative correspond to an absence of this type of coordination.
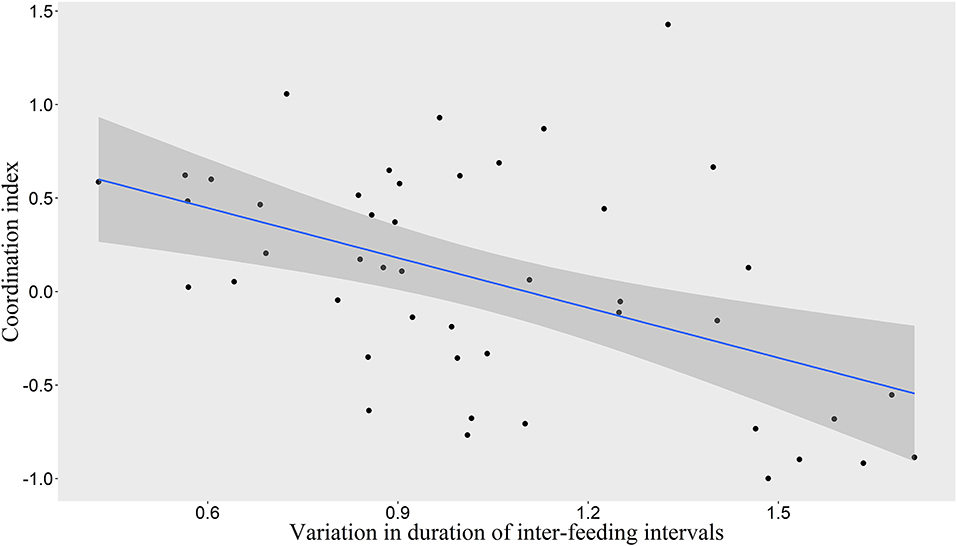
Figure 3. Relationship between coordination index and variation in duration of inter-feeding intervals. Scatterplot with linear regression line (in blue) and 95% Confidence Interval (in shaded gray).
Environmental Conditions and Coordination Level
As assumed, all the five seasons were different in regard to the considered environmental parameters (Kruskal-Wallis tests, Total energy density: P = 0.0008; Ratio between abundance of Calanus glacialis and Calanus finmarchicus: P < 2.2e−16; Simpson's Diversity Index: P = 9.6e−12; SST: P < 2.2e−16; see Figure 4 for detailed U-test post-hoc comparisons concerning Total energy density [highlighted as most important in further recursive partitioning analysis]; and Figure S1 for other parameters). However, no significant effect of the season was found on the coordination index (LMM, χ2 = 7.44, P = 0.11; Tukey test, P > 0.05 for every possible combination), and only trends could be observed on the distribution of coordination index between the seasons (Figure 2).
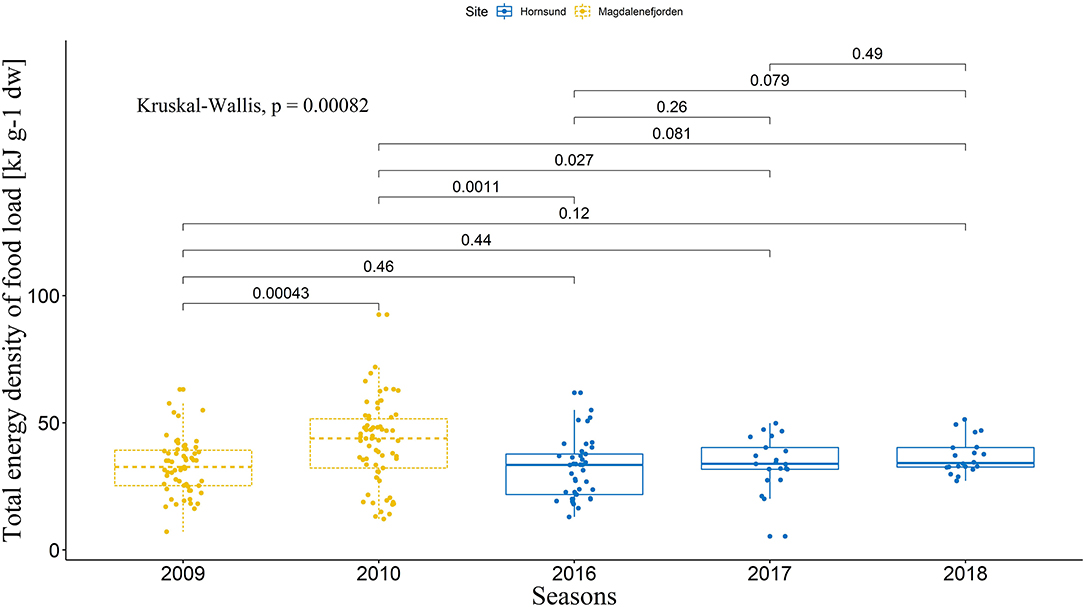
Figure 4. Total energy density of food loads delivered to the chicks during the studied breeding seasons. The boxes depict interquartile range, with median as a bold line inside the box. Whiskers indicate variability outside the upper and lower quartiles. Dots represents the raw data points. Inter-season comparisons were made with a Mann–Whitney U-test, and overall difference was statistically tested with a non-parametric Kruskal–Wallis test. Only Total energy density is presented here as it was highlighted as most important in recursive partitioning analysis (see Figure S1 for other parameters).
Recursive partitioning analysis revealed that, of all the environmental parameters investigated, the mean total energy density of food load in a given season had the highest relative importance in shaping the coordination index. This analysis created a regression tree with two splits based on the total energy density of the food load (Figure 5), resulting in three groups with different foraging conditions regarding this parameter. The first split divided our data set into two significantly different groups (U-test, P = 0.026, and balanced between the two colonies, Figure 5) and identified that when the foraging conditions are characterized by a total energy density of food load ≥ 35 kJ g−1 dw, the coordination index is the highest (mean = 0.22, n = 35), compared to the group characterized by foraging conditions of total energy density of food load <35 kJ g−1 dw (mean coordination index = −0.033, n = 46). A second split was then applied to the latter group and divided it into two sub-groups that were not significantly different (U-test, P = 0.071, Figure 5). When the total energy density of food load is between 34 and 35 kJ g−1 dw, the coordination index is the lowest (mean = −0.22, n = 14), indicating that parents are not coordinated and even have a high chance of performing a LT at the same time. When the total energy density of food load is <34 kJ g−1 dw, the coordination level is close to what is expected by chance (mean = 0.048, n = 32), meaning that parents are not coordinating their provisioning.
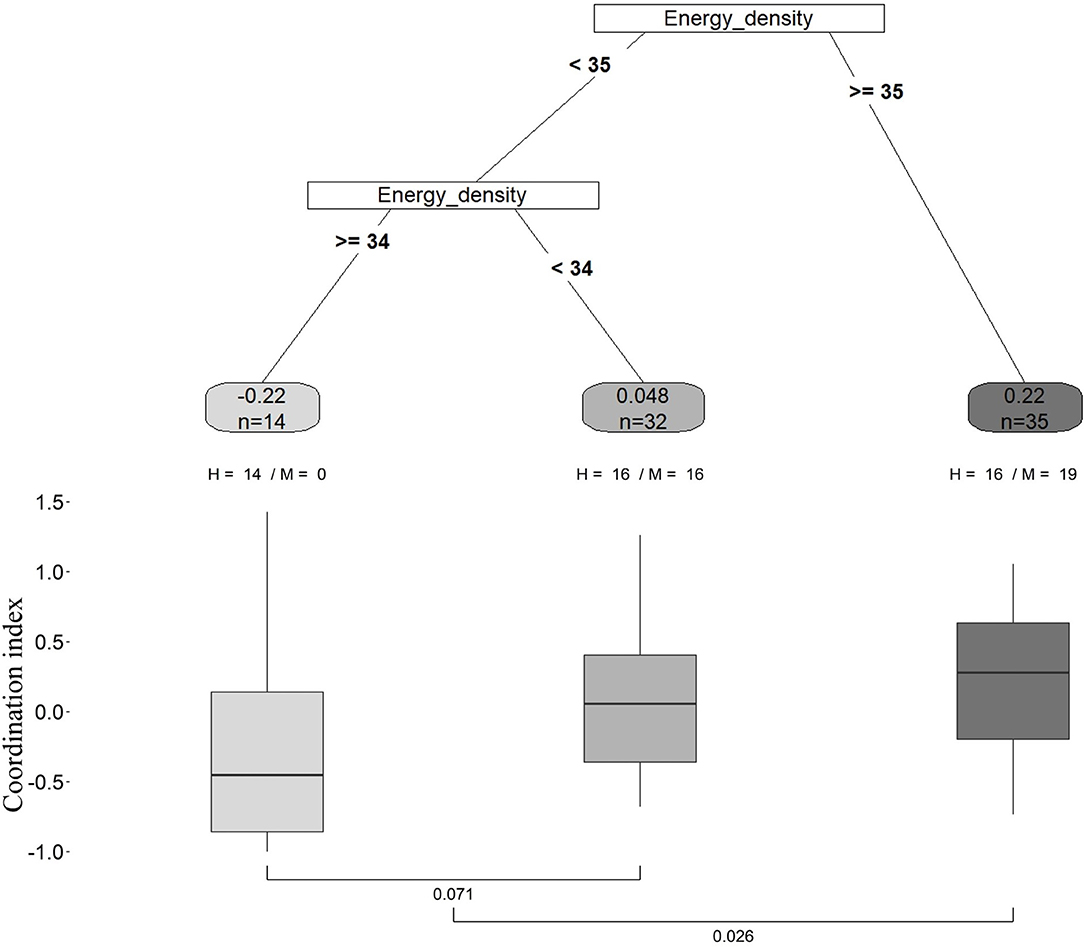
Figure 5. Regression tree obtained with recursive partitioning analysis. The “inverted tree” presents the nodes and branches found by the analysis. The root at the top contains all observations, and is divided into two branches at the node. The group on the left is further split into two subsequent groups. The nodes provide information about the explanatory variable name (in a box) used for the split, and the value used for the split is represented on the branches. Each terminal node (in an oval) is showing the mean of the coordination index and the sample size (n) for the formed group. Proportion of cases from the two colonies in each final group is indicated (H, Hornsund; M, Magdalenefjorden). Boxplots for particular nodes depict the interquartile range of coordination indices of each group, with the median as a bold line and whiskers indicating variability outside the upper and lower quartiles. Inter-group comparisons were made with Mann–Whitney U-tests. Energy_density: Mean total energy density of food load in a given season (in kJ.g−1 dw).
Effect of Coordination on Chick Body Condition
The 48-h period between the onset and end of the observation was characterized by an overall gain in chick body mass. On average, a chick gained 10% of its initial body mass per day during the 48-h period (interquartile range: 6.4–14.2%). However, we found no significant effect of the coordination index on body mass gain (LMM, χ2 = 0.31, P = 0.58).
Discussion
Our results showed that Little Auk parents coordinate chick provisioning, adjusting the timing of ST and LT to those of the partner, thereby reducing the variation in the duration of inter-feeding intervals. Our findings are consistent with the previous study on coordinated provisioning by the Little Auk parents (Wojczulanis-Jakubas et al., 2018). Since the previous study, was performed in a colony located at a relatively long distance from the optimal foraging grounds, it imposed the question of how colony-specific the observed pattern is. Present findings, obtained using a broader spatial and environmental context, showed coordinated provisioning with a similar variability regardless of environmental conditions, suggesting that current foraging conditions have no notable effect on coordination. Nevertheless, we also found that the energy density of food loads delivered to chicks was associated with parental coordination: when conditions were characterized by the delivery of higher-energy food loads, the level of coordination exhibited by the studied population was higher. Thus, the coordination is not entirely independent from environmental conditions. We also examined whether chick body condition is related to the level of parental coordination to test potential adaptive value of the coordination. However, we did not find any significant effect, at least within the observed range of environmental conditions.
Although Little Auks are known to change foraging flight duration in response to environmental conditions (Welcker et al., 2009; Kwaśniewski et al., 2010; Jakubas et al., 2011, 2013; Grémillet et al., 2012; Hovinen et al., 2014; Kidawa et al., 2015), the pattern of dual-foraging strategy (alternated ST and LT) seems to be fixed regardless of the environmental circumstances (Steen et al., 2007; Welcker et al., 2009; Wojczulanis-Jakubas et al., 2010; Jakubas et al., 2016). It has also been suggested that the dual-foraging strategy could be a fixed trait due to its adaptive value. Foraging parents gained weight when returning from LT and lost an equivalent amount of mass during subsequent ST. Thus, the bimodal foraging allows adults to regularly restore their body mass after intensive chick provisioning (Welcker et al., 2012). Therefore, if dual-foraging is a fixed and adaptive trait in the Little Auk, the coordination of foraging trips between partners could be relatively easy to achieve, regardless of the foraging conditions.
As argued in the Introduction, the coordinated provisioning is expected to have an adaptive value. If so, why Little Auk parents coordinate the chick provisioning if it does not influence chick growth rate remains an intriguing question. However, although a positive relationship between coordination and chick body condition has been demonstrated in some species (Mariette and Griffith, 2015), some other studies have also failed to demonstrate a direct effect of parental coordination on nestling growth rate (van Rooij and Griffith, 2013; Bebbington and Hatchwell, 2016). One explanation of this apparent paradox in the Little Auk could be that coordination also aims to improve the parents' condition and thus increases the fitness of the whole family. As body mass of adult Little Auks increases after the long trip and decreases after subsequent short trips (Welcker et al., 2012), coordination between partners could be used to minimize this body mass decrease while assuring regular chick provisioning. This could be a mechanism to secure both present and future breeding success of the two partners. If the participation of both parents is necessary to raise the offspring successfully, and partners are paired for multiple seasons, as is the case in the Little Auk (Stempniewicz, 2001; Kidawa et al., 2012), not only body condition of the current offspring but current and future condition of both partners are expected to be under strong evolutionary selection (Jones, 2002). The lack of association between parental coordination and chick condition could also be a result of methodological constraints, which could operate at two levels. First, we performed the study during the mid-chick rearing period, when Little Auk chicks are already thermally independent and may be quite resistant to a prolonged fasting period (Taylor and Konarzewski, 1989; Konarzewski et al., 1993). Taylor and Konarzewski (1989) found that the estimated fat reserves of 99.7% of chicks are sufficient to support them for longer than the maximum recorded interval between feedings. Another study on parental coordination in seabirds (Tyson et al., 2017) suggests that the propensity for pairs to coordinate declines across the chick rearing period. Thus, at the beginning of the chick rearing period, when chicks' parental care requirements (food and brooding) are higher, the level of coordinated provisioning would probably be higher and could also have a more visible effect on chick body condition. The second methodological issue is that our measure of chick body condition in the form of body mass change may not be an ideal predictor. Although more accurate than that applied in Wojczulanis-Jakubas et al. (2018), it still presents some limitations: some events potentially happening before the measurement (e.g., feeding, defecation, wings training) could have considerably affected chick body mass but were not accounted for in our study. In addition, some studies suggest that increased parental provisioning does not necessarily result in greater chick body mass (Titulaer et al., 2012). A future study could consider examining the effect of parental coordination on other parameters of chick body conditions, e.g., immunological or hematological parameters.
Another intriguing question raised by our study and worth examining in future is the mechanism behind the parental coordination. We have assumed an active foraging coordination of the partners as a response to the feeding needs of growing offspring. However, we cannot exclude the possibility that the observed coordination is a result of selection for different behaviors, diet and/or foraging specializations of the breeding adults. For example, sex-specific provisioning behavior has been observed in another alcid species, the Common Guillemot (Uria lomvia), where males fed on “risk-averse” and females on “risk-prone” prey items. Importantly, availability of the prey types may vary across the day, creating the pattern of males foraging during the night and females foraging during the day (Elliott et al., 2010). Such a sex-specific niche partitioning may lead to a coordinated pattern of provisioning resulting from constraints other than those investigated in our study. However, no sex difference in the diel distribution of feedings has been observed in the Little Auk (unpublished data), suggesting that sex-specific foraging specializations might not play a role in the observed coordination. Nevertheless, other scenarios related to foraging, individual specialization, and assortative/disassortative mating are possible in the Little Auk and could potentially create a misleading coordinated manner of chick provisioning.
Although environmental conditions are considered important in the evolution of avian breeding systems, with numerous examples of direct effects of environment on reproductive success (harsh environment hypothesis; Silver et al., 1985; Martin, 1987; Arnold and Duvall, 2002; Fontaine and Martin, 2006; AlRashidi et al., 2010), the two existing meta-analyses on this topic have not found a significant link between environmental conditions and parental cooperation (Olson et al., 2008; Remeš et al., 2015). Both meta-analyses support the view that the major correlates of parental cooperation are lack of mating opportunities for both sexes and mode of offspring development, rather than the breeding environment. Our results demonstrate a similar level of coordinated provisioning regardless of ecological conditions and also question the importance of environmental parameters in parental cooperative behavior. Nevertheless, we cannot entirely reject the harsh environment hypothesis. First, our findings could suffer from methodological constraints, as we tested for differences in coordination between years and assumed that these differences were driven by differences in the measured environmental parameters. Other environmental parameters not accounted for in our study, or variability in followed pairs could drive the difference, thus we cannot make a causative link between environmental variability and coordination. This approach was chosen due to the unavailability of finer-scale parameters, and measured parameters were carefully chosen from relevant literature, but we cannot exclude the role played by unexpected parameters. Second, it is possible that despite considerable variation in environmental conditions across the studied seasons, the conditions were still within a tolerable range of variation and consequently did not affect parents' provisioning behavior. Third, we found that the level of coordination seemed to be related to the energy value of food, as revealed by our recursive partitioning analysis: seasons characterized by a higher energy density of food were associated with a higher level of coordination. This finding suggests that environmental conditions do affect the coordination of parents but that the relationship may not be straightforward to explain due to the complex nature of the examined parameter, i.e., energy value of the food load. This is because the composition of food load was not obtained from focal pairs but from other birds from the same colony; therefore it is a proxy for energetic value of the food delivered to chicks at the colony scale and is also a combination of environmental conditions and parental effort. Moreover, high energetic value of food may indicate either good foraging conditions on foraging grounds, or high parental efficiency in foraging regardless of conditions on the foraging areas, or a combination of both. Hence, our results could mean that partners better coordinate their foraging trips in good conditions, i.e., high availability of energetic food in close vicinity of the colony. Only in this situation would pair members be able to adjust foraging flights in regard to each other and optimize use of good foraging conditions as much as possible in order to increase the fitness of both parents and chick. Alternatively, however, it could also mean that parents coordinate better in poor conditions i.e., low availability of energy-rich food in close vicinity of the colony. These circumstances would force them to increase energy expenditure allocated to chick provisioning. In consequence, they would need to coordinate their provisioning with each other, as only then could they feed their chick and secure an acceptable body condition for current and future breeding success.
The present study brings insight into the role of one environmental parameter (energy value of the food load) in shaping variability of parental coordination, suggesting that environmental conditions might affect the coordination of Little Auk parents. However, further studies investigating the full extent of the relationship are needed, to fully comprehend the mechanisms behind the parental coordination. They could take advantage of the recent improvements in tracking devices to establish very precise foraging areas and extract finer-scale environmental parameters.
Data Availability
Data provided in Table S2. Contains already calculated coordination indices but allow to repeat the analyses presented in the present paper. A script and relevant data to calculate a coordination index per se have been presented in the previous paper (Wojczulanis-Jakubas et al., 2018). Raw data used in the present paper can be made available without undue reservation, to any qualified researcher.
Ethics Statement
The animal study was reviewed and approved by the Norwegian Animal Research Authority.
Author Contributions
AG, KW-J, DJ, and MA-S: conceptualization. KW-J and KB-S: data curation. AG: formal analysis. KW-J, DK, and KB-S: funding acquisition. KW-J, DJ, DK, and RB: investigation. AG: writing original draft. All co-authors: writing, review and editing.
Funding
This study was supported by Norway through the Norwegian Financial Mechanism (grant number: ALKEKONGE, PNRF-234-AI-1/07), by Poland through National Science Center (no: 2017/25/B/NZ8/01417 to KW-J, and no: 2017/26/D/NZ8/00005 to DK), and received additional support from Polish Ministry of Science and Higher Education co-funded project (3605/SEAPOP/2016/2).
Conflict of Interest Statement
The authors declare that the research was conducted in the absence of any commercial or financial relationships that could be construed as a potential conflict of interest.
Acknowledgments
We thank Mateusz Barcikowski, Lech M. Iliszko, Małgorzata Jakimiak, Magdalena Hadwiczak, and Katarzyna Pinska for their help in the field. We also thank Marion Devogel for her help with video processing and editing of earlier versions of the manuscript. Final version of the manuscript was proof-read by Carissa Ganong, as a native English speaker and specialist in ecology, and we particularly thank her for the improvements she made.
Supplementary Material
The Supplementary Material for this article can be found online at: https://www.frontiersin.org/articles/10.3389/fevo.2019.00349/full#supplementary-material
Table S1. Detailed information on abiotic data used in analyses, and dates of data collection.
Table S2. Coordination and environmental data.
Figure S1. Environmental conditions during the studied breeding seasons (A: the ratio of Calanus glacialis/Calanus finmarchicus abundance; B: Simpson's Diversity Index; C: Sea Surface Temperature).
References
AlRashidi, M., Kosztolányi, A., Küpper, C., Cuthill, I. C., Javed, S., and Székely, T. (2010). The influence of a hot environment on parental cooperation of a ground-nesting shorebird, the Kentish plover Charadrius alexandrinus. Front. Zool. 7, 1–10. doi: 10.1186/1742-9994-7-1
Arnold, S. J., and Duvall, D. (2002). Animal mating systems: a synthesis based on selection theory. Am. Nat. 143, 317–348. doi: 10.1086/285606
Bates, D., Mächler, M., Bolker, B. M., and Walker, S. C. (2015). Fitting linear mixed-effects models using lme4. J. Stat. Softw. 67, 1–48. doi: 10.18637/jss.v067.i01
Bebbington, K., and Hatchwell, B. J. (2016). Coordinated parental provisioning is related to feeding rate and reproductive success in a songbird. Behav. Ecol. 27, 652–659. doi: 10.1093/beheco/arv198
Bretz, F., and Westfall, P. (2008). Simultaneous inference in general parametric models. Biom. J. 50, 346–363. doi: 10.1002/bimj.200810425
Cockburn, A. (2006). Prevalence of different modes of parental care in birds. Proc. R. Soc. B Biol. Sci. 273, 1375–1383. doi: 10.1098/rspb.2005.3458
Congdon, B. C., Krockenberger, A. K., and Smithers, B. V. (2005). Dual-foraging and co-ordinated provisioning in a tropical Procellariiform, the wedge-tailed shearwater. Mar. Ecol. Prog. Ser. 301, 293–301. doi: 10.3354/meps301293
Cottier, F., Tverberg, V., Inall, M., Svendsen, H., Nilsen, F., and Griffiths, C. (2005). Water mass modification in an Arctic fjord through cross-shelf exchange: the seasonal hydrography of Kongsfjorden, Svalbard. J. Geophys. Res. 110:C12. doi: 10.1029/2004JC002757
Davis, L. S. (1988). Coordination of incubation routines and mate choice in Adelie penguins (Pygoscelis adeliae). Auk. 105, 428–432.
Elliott, K. H., Gaston, A. J., and Crump, D. (2010). Sex-specific behavior by a monomorphic seabird represents risk partitioning. Behav. Ecol. 21, 1024–1032. doi: 10.1093/beheco/arq076
Fontaine, J. J., and Martin, T. E. (2006). Parent birds assess nest predation risk and adjust their reproductive strategies. Ecol. Lett. 9, 428–434. doi: 10.1111/j.1461-0248.2006.00892.x
Fox, J., and Weisberg, S. (2011). An R Companion to Applied Regression, 2nd Edn. Thousand Oaks, CA: Sage. Available online at: http://socserv.socsci.mcmaster.ca/jfox/Books/Companion
Frederiksen, M., Anker-Nilssen, T., Beaugrand, G., and Wanless, S. (2013). Climate, copepods and seabirds in the boreal Northeast Atlantic - current state and future outlook. Glob. Chang. Biol. 19, 364–372. doi: 10.1111/gcb.12072
Grémillet, D., Welcker, J., Karnovsky, N., Walkusz, W., Hall, M., Fort, J., et al. (2012). Little auks buffer the impact of current Arctic climate change. Mar. Ecol. Prog. Ser. 454, 197–206. doi: 10.3354/meps09590
Harding, A. M. A., Van Pelt, T. I., Lifjeld, J. T., and Mehlum, F. (2004). Sex differences in little auk Alle alle parental care: transition from biparental to paternal-only care. Ibis. 146, 642–651. doi: 10.1111/j.1474-919X.2004.00297.x
Hinde, C. A. (2006). Negotiation over offspring care?-a positive response to partner-provisioning rate in great tits. Behav. Ecol. 17, 6–12. doi: 10.1093/beheco/ari092
Hovinen, J. E. H., Wojczulanis-Jakubas, K., Jakubas, D., Hop, H., Berge, J., Kidawa, D., et al. (2014). Fledging success of Little Auks in the high Arctic: do provisioning rates and the quality of foraging grounds matter? Polar Biol. 37, 665–674. doi: 10.1007/s00300-014-1466-1
Jakobsson, M., Mayer, L., Coakley, B., Dowdeswell, J. A., Forbes, S., Fridman, B., et al. (2012). The international bathymetric chart of the Arctic Ocean (IBCAO) version 3.0. Geophys. Res. Lett. 39:L12609. doi: 10.1029/2012GL052219
Jakubas, D., Głuchowska, M., Wojczulanis-Jakubas, K., Karnovsky, N., Keslinka, L., Kidawa, D., et al. (2011). Foraging effort does not influence body condition and stress level in Little Auks. Mar. Ecol. Prog. Ser. 432, 277–290. doi: 10.3354/meps09082
Jakubas, D., Iliszko, L., Wojczulanis-Jakubas, K., and Stempniewicz, L. (2012). Foraging by Little Auks in the distant marginal sea ice zone during the chick-rearing period. Polar Biol. 35, 73–81. doi: 10.1007/s00300-011-1034-x
Jakubas, D., Iliszko, L. M., Strøm, H., Darecki, M., Jerstad, K., and Stempniewicz, L. (2016). Foraging behavior of a high-Arctic zooplanktivorous alcid, the little auk, at the southern edge of its breeding range. J. Exp. Mar. Biol. Ecol. 475, 89–99. doi: 10.1016/j.jembe.2015.11.010
Jakubas, D., Trudnowska, E., Wojczulanis-Jakubas, K., Iliszko, L., Kidawa, D., Darecki, M., et al. (2013). Foraging closer to the colony leads to faster growth in Little Auks. Mar. Ecol. Prog. Ser. 489, 263–278. doi: 10.3354/meps10414
Jakubas, D., Wojczulanis-Jakubas, K., Iliszko, L., Darecki, M., and Stempniewicz, L. (2014). Foraging strategy of the Little Auk Alle alle throughout breeding season – switch from unimodal to bimodal pattern. J. Avian Biol. 45, 551–556. doi: 10.1111/jav.00303
Jakubas, D., Wojczulanis-Jakubas, K., Iliszko, L. M., Strøm, H., and Stempniewicz, L. (2017). Habitat foraging niche of a High Arctic zooplanktivorous seabird in a changing environment. Sci. Rep. 7:16203. doi: 10.1038/s41598-017-16589-7
Johnstone, R. A., and Hinde, C. (2006). Negotiation over offspring care - how should parents respond to each other's efforts? Behav. Ecol. 17, 818–827. doi: 10.1093/beheco/arl009
Johnstone, R. A., Manica, A., Fayet, A. L., Stoddard, C., Rodriguez-Gironés, M. A., and Hinde, C. A. (2014). Reciprocity and conditional cooperation between great tit parents. Behav. Ecol. 25, 216–222. doi: 10.1093/beheco/art109
Jones, K. M. (2002). Model parents: is full compensation for reduced partner nest attendance compatible with stable biparental care? Behav. Ecol. 13, 838–843. doi: 10.1093/beheco/13.6.838
Keslinka, L. K., Wojczulanis-Jakubas, K., Jakubas, D., and Neubauer, G. (2019). Determinants of the little auk (Alle alle) breeding colony location and size in W and NW coast of Spitsbergen. PLoS ONE 14:e0212668. doi: 10.1371/journal.pone.0212668
Kidawa, D., Jakubas, D., Wojczulanis-Jakubas, K., Iliszko, L., and Stempniewicz, L. (2012). The effects of loggers on the foraging effort and chick-rearing ability of parent Little Auks. Polar Biol. 35, 909–917. doi: 10.1007/s00300-011-1136-5
Kidawa, D., Jakubas, D., Wojczulanis-Jakubas, K., Stempniewicz, L., Trudnowska, E., Boehnke, R., et al. (2015). Parental efforts of an Arctic seabird, the little auk Alle alle under variable foraging conditions. Mar. Biol. Res. 11, 349–360. doi: 10.1080/17451000.2014.940974
Konarzewski, M., Taylor, J., and Gabrielsen, G. (1993). Chick energy requirements and adult energy expenditures of dovekies (Alle alle). Auk. 110, 343–353.
Konarzewski, M., and Taylor, J. R. E. (1989). The Influence of Weather conditions on growth of little auk Alle alle chicks. Ornis Scand. 20, 112–116. doi: 10.2307/3676877
Kwaśniewski, S., Gluchowska, M., Jakubas, D., Wojczulanis-Jakubas, K., Walkusz, W., Karnovsky, N., et al. (2010). The impact of different hydrographic conditions and zooplankton communities on provisioning Little Auks along the West coast of Spitsbergen. Prog. Oceanogr. 87, 72–82. doi: 10.1016/j.pocean.2010.06.004
Kwaśniewski, S., Gluchowska, M., Walkusz, W., Karnovsky, N. J., Jakubas, D., Wojczulanis-Jakubas, K., et al. (2012). Interannual changes in zooplankton on the West Spitsbergen Shelf in relation to hydrography and their consequences for the diet of planktivorous seabirds. ICES J. Mar. Sci. 69, 890–901. doi: 10.1093/icesjms/fss076
Mariette, M. M., and Griffith, S. C. (2015). The adaptive significance of provisioning and foraging coordination between breeding partners. Am. Nat. 185, 270–280. doi: 10.1086/679441
Martin, T. E. (1987). Food as a limit on breeding birds: a life-history perspective. Annu. Rev. Ecol. Syst. 18, 453–487. doi: 10.1146/annurev.es.18.110187.002321
Massoni, V., Reboreda, J. C., López, G. C., and Aldatz, M. F. (2012). High coordination and equitable parental effort in the rufous hornero. The Condor. 114, 564–570. doi: 10.1525/cond.2012.110135
NASA Goddard Space Flight Center Ocean Biology Laboratory Ocean Biology processimg Group (2014). Moderate-resolution Imaging Spectroradiometer (MODIS) Terra Sea Surface Temperature Data.
National Ice Center (NIC) and NSIDC (2010). “Multisensor Analyzed Sea Ice Extent – Northern Hemisphere,” in IMS Daily Northern Hemisphere Snow and Ice Analysis at 4 km Resolution Data Set. Developed by F. Fetterer, M. Savoie, S. Helfrich, and P. Clemente-Colón. Boulder, CO. Available online at: https://nsidc.org/data/masie
Olson, V. A., Liker, A., Freckleton, R. P., and Sze, T. (2008). Parental conflict in birds: comparative analyses of offspring development, ecology and mating opportunities. Proc. R. Soc. 275, 301–307. doi: 10.1098/rspb.2007.1395
Piatt, J. F., Harding, A. M. A., Shultz, M., Speckman, S. G., van Pelt, T. I., Drew, G. S., and Kettle, A. B. (2007). Seabirds as indicators of marine food supplies: cairns revisited. Mar. Ecol. Prog. Ser. 352, 221–234. doi: 10.3354/meps07078
Piechura, J., Beszczynska-Möller, A., and Osinski, R. (2001). Volume, heat and salt transport by the west spitsbergen current. Polar Res. 20, 233–240. doi: 10.3402/polar.v20i2.6522
Piechura, J., and Walczowski, W. (2009). Warming of the west spitsbergen current and sea ice north of Svalbard. Oceanologia 51, 147–164. doi: 10.5697/oc.51-2.147
R Core Team (2018). R: A Language and Environment for Statistical Computing. R Foundation for Statistical Computing, Vienna. Available online at: http://www.R-project.org/
Raihani, N. J., Nelson-Flower, M. J., Moyes, K., Browning, L. E., and Ridley, A. R. (2010). Synchronous provisioning increases brood survival in cooperatively breeding pied babblers. J. Anim. Ecol. 79, 44–52. doi: 10.1111/j.1365-2656.2009.01606.x
Remeš, V., Freckleton, R. P., Tökölyi, J., Liker, A., and Székely, T. (2015). The evolution of parental cooperation in birds. Proc. Natl. Acad. Sci. 112, 13603–13608. doi: 10.1073/pnas.1512599112
Ricklefs, R. E. (1990). Seabird life histories and the marine environment: some speculations. Colonial Waterbirds. 13, 1–6. doi: 10.2307/1521414
Silver, R., Andrews, H., Ball, G. F., Andrews, H., and Ball, F. (1985). Parental care in an ecological perspective: a quantitative analysis of avian subfamilies. Am. Zool. 25, 823–840. doi: 10.1093/icb/25.3.823
Steen, H., Vogedes, D., Broms, F., Falk-Petersen, S., and Berge, J. (2007). Little auks (Alle alle) breeding in a High Arctic fjord system: bimodal foraging strategies as a response to poor food quality? Polar Res. 26, 118–125. doi: 10.1111/j.1751-8369.2007.00022.x
Stempniewicz, L. (2001). BWP update. little auk (Alle alle). J. Birds Western Palearctic 3, 175–201.
Taylor, J. R. E., and Konarzewski, M. (1989). On the importance of fat reserves for the little auk (Alle alle) chicks. Oecologia 81, 551–558 doi: 10.1007/BF00378968
Therneau, T. M., and Atkinson, E. J. (2019). An Introduction to Recursive Partitioning Using the Rpart Routines. Rochester: Mayo Foundation.
Titulaer, M., Spoelstra, K., Lange, C. Y., and Visser, M. E. (2012). Activity patterns during food provisioning are affected by artificial light in free living great tits (Parus major). PLoS ONE 7:e37377. doi: 10.1371/journal.pone.0037377
Tyson, C., Kirl, H., Fayet, A., Van Loon, E. E., Shoji, A., Dean, B., et al. (2017). Coordinated provisioning in a dual-foraging pelagic seabird. Anim. Behav. 132, 73–79. doi: 10.1016/j.anbehav.2017.07.022
van Rooij, E. P., and Griffith, S. C. (2013). Synchronised provisioning at the nest: parental coordination over care in a socially monogamous species. PeerJ 1:e232. doi: 10.7717/peerj.232
Wassmann, P., Duarte, C. M., Agustí, S., and Sejr, M. K. (2011). Footprints of climate change in the Arctic marine ecosystem. Glob. Chang. Biol. 17, 1235–1249. doi: 10.1111/j.1365-2486.2010.02311.x
Welcker, J., Beiersdorf, A., Varpe, Ø., and Steen, H. (2012). Mass fluctuations suggest different functions of bimodal foraging trips in a central-place forager. Behav. Ecol. 23, 1372–1378. doi: 10.1093/beheco/ars131
Welcker, J., Harding, A. M. A., Karnovsky, N. J., Steen, H., Strøm, H., and Gabrielsen, G. W. (2009). Flexibility in the bimodal foraging strategy of a high Arctic alcid, the little auk Alle alle. J. Avian Biol. 40, 388–399. doi: 10.1111/j.1600-048X.2008.04620.x
Wojczulanis, K., Jakubas, D., Walkusz, W., and Wennerberg, L. (2006). Differences in food delivered to chicks by males and females of Little Auks (Alle alle) on South Spitsbergen. J. Ornithol. 147, 543–548. doi: 10.1007/s10336-006-0077-1
Wojczulanis-Jakubas, K., Araya-Salas, M., and Jakubas, D. (2018). Seabird parents provision their chick in a coordinated manner. PLoS ONE 13:e0189969. doi: 10.1371/journal.pone.0189969
Wojczulanis-Jakubas, K., Jakubas, D., Karnovsky, N. J., and Walkusz, W. (2010). Foraging strategy of Little Auks under divergent conditions on feeding grounds. Polar Res. 29, 22–29. doi: 10.1111/j.1751-8369.2009.00145.x
Wojczulanis-Jakubas, K., Jakubas, D., and Stempniewicz, L. (2009). Sex-specific parental care by incubating Little Auks (Alle alle). Ornis Fennica. 86, 140–148.
Keywords: coordinated provisioning, environmental effect, little auk (Dovekie), seabird, parental care
Citation: Grissot A, Araya-Salas M, Jakubas D, Kidawa D, Boehnke R, Błachowiak-Samołyk K and Wojczulanis-Jakubas K (2019) Parental Coordination of Chick Provisioning in a Planktivorous Arctic Seabird Under Divergent Conditions on Foraging Grounds. Front. Ecol. Evol. 7:349. doi: 10.3389/fevo.2019.00349
Received: 27 May 2019; Accepted: 02 September 2019;
Published: 18 September 2019.
Edited by:
James Luke Savage, University of Sheffield, United KingdomCopyright © 2019 Grissot, Araya-Salas, Jakubas, Kidawa, Boehnke, Błachowiak-Samołyk and Wojczulanis-Jakubas. This is an open-access article distributed under the terms of the Creative Commons Attribution License (CC BY). The use, distribution or reproduction in other forums is permitted, provided the original author(s) and the copyright owner(s) are credited and that the original publication in this journal is cited, in accordance with accepted academic practice. No use, distribution or reproduction is permitted which does not comply with these terms.
*Correspondence: Antoine Grissot, YW50b2luZS5ncmlzc290QGdtYWlsLmNvbQ==