- 1Biointeractions and Crop Protection Department, Rothamsted Research, Harpenden, United Kingdom
- 2Department of Plant Protection, Faculty of Agriculture, Suez Canal University, Ismailia, Egypt
In response to herbivory, plants synthesize and release variable mixtures of herbivore-induced plant volatiles (HIPVs) as indirect defense traits. Such induction of indirect plant defense can also be “switched on” by certain chemicals known as priming agents. Preceding work showed that the plant HIPV cis-jasmone (CJ) induced the emission of aphid defense-related volatiles affecting their behavioral response. However, little is known about the extent to which CJ-induced volatiles impacts aphid performance. In the current study, we conducted growth assays of potato aphids, Macrosiphum euphorbiae, observing their reproduction, development, and survival on CJ-primed potato plants. Adult M. euphoribae produced fewer neonates on CJ-treated plants compared to untreated plants. The weight and survival of M. euphorbiae reproduced neonates were significantly lower on CJ-treated plants. Additionally, there was a significant reduction in mean relative growth rate (MRGR) of M. euphoribae nymphs that fed on CJ-treated plants. Furthermore, the intrinsic rate of population increase (rm) of M. euphoribae was significantly reduced on CJ-treated plants. Volatile analysis showed that CJ treatment significantly increased the emission of differently assigned volatile groups that have functional or biosynthetic characteristics, i.e., alcohols, benzenoids, homoterpenes, ketones, and sesquiterpenes at all sampling periods. Such enhanced volatile emissions were persistent over 7 days, suggesting a long-lasting effect of CJ defense priming. A negative correlation was found between volatile emission and MRGR of M. euphoribae. Principal component analysis (PCA) of data for the volatiles showed that (Z)-3-hexen-1-ol, α-pinene, (E)-ocimene, (E)-4,8-dimethyl-1,3,7-nonatriene (DMNT), cis-jasmone, indole, and (E,E)-4,8,12-trimethyl-1,3,7,11-tridecatetraene (TMTT) were the main volatiles contributing to the emitted blends, suggesting possible involvement in the reduced performance of M. euphorbiae. Overall, our findings demonstrate that priming potato with CJ significantly results in elevated emission of known biologically active volatiles, which may negatively impact aphid settling and other performance traits on primed plants.
Introduction
Although many crop protection methods are currently utilized, insect pests still reduce the yield and quality of agricultural production worldwide, causing tremendous economic losses and threating global food security (Savary et al., 2019). Spraying chemical pesticides remains the main approach to combatting insect attacks in commercial crops, which entails serious drawbacks such as the evolution of insecticide resistance (Bass et al., 2014) and potential toxicity to beneficial insects such as bees and natural enemies (Desneux et al., 2007). Furthermore, there is an increasing restriction in the registration of conventional pesticides, which globally limits the future availability of active ingredients that are currently used for controlling crop pests (Birch et al., 2011). Thus, more sustainable and ecologically safer alternatives are urgently sought (Bruce, 2010).
During their coevolution with insects, plants have evolved a complex arsenal of defense mechanisms (Wu and Baldwin, 2010; Bruce, 2015). One of these defense traits is the synthesis and release of a diverse mixture of herbivore-induced plant volatiles (HIPVs) in response to insect attack (Pickett and Khan, 2016). These inducible defenses against insect invaders are regulated by a complex network of phytohormonal signaling (Erb et al., 2012), including salicylic acid (SA) and jasmonic acid (JA), which are also involved in HIPVs induction (Dudareva et al., 2013). The emitted HIPVs are perceived by neighboring plants, conspecific and/or heterospecific herbivores, and natural enemies, mediating a diverse array of multitrophic interactions (Turlings and Erb, 2018). Upon perception, whereas herbivores employ HIPVs to avoid infested plants by their conspecifics (De Moraes et al., 2001), neighboring plants make use of HIPVs to potentiate their defense so that they respond faster and more robustly when subsequently challenged by future insect attacks, a phenomenon known as “defense priming” (Engelberth et al., 2004). Such interplay between insects and plants, which is mediated by plant volatiles, has promoted the prospect that manipulating the emission of HIPVs, as a trait of plant natural defense, can be a potential driver for innovation in crop protection against herbivorous insects (Sobhy et al., 2014; Pickett and Khan, 2016; Turlings and Erb, 2018).
More recently, studies have shown that phenotypic manipulation of HIPVs can be achievable by the application of chemical priming agents (Sobhy et al., 2012, 2018). These priming agents represent a new class of agrochemical that does not have a direct toxic impact on the target organisms (Sobhy et al., 2015b), but instead acts on defense signaling pathways and that could be harnessed in ecologically-based pest management programs (Bruce et al., 2017). Nevertheless, whereas chemical agents that prime plant resistance against pathogens are broadly being applied commercially (Gozzo and Faoro, 2013; Walters et al., 2013 and references herein), the development and application of such priming agents against herbivorous insects is still at the experimental stage. This is attributed to the fact that plant genotype, environmental conditions and other stressors substantially affect any subsequent enhancement in plant defense against insects caused by priming agents application (Bruce, 2014).
Aphids are important pests of crops that cause yield losses worldwide (Blackman and Eastop, 2006). In addition to their direct damage (i.e., malformation of the foliage and plant growth reduction) and crop contamination by honeydew and sooty mold, aphids are the most important vectors for non-persistent plant viruses, even for non-host plants (van Emden and Harrington, 2017). It is increasingly evident that the application of chemical priming agents, such as β-aminobutyric acid (BABA) (Hodge et al., 2005, 2006; Zhong et al., 2014) and BTH (acibenzolar-S-methyl) (Cooper et al., 2004; Boughton et al., 2006), has a negative impact upon aphid populations.
Another promising compound that robustly activates plant defense against aphids is cis-jasmone (CJ) (Pickett et al., 2007b), which is a plant metabolite that is synthesized from α-linolenic acid via the biosynthetic pathway of iso-12-oxo-phytodienoic acid (iso-OPDA) (Dabrowska and Boland, 2007). Preceding work showed that exogenous application of CJ resulted in the induction of defense-related volatile organic compounds (VOCs), including (E)-2-hexenal, 6-methyl-5-hepten-2-one (MHO), (Z)-3-hexenyl acetate, myrcene, (E)-ocimene, (E)-4,8-dimethyl-1,3,7-nonatriene (DMNT), methyl benzoate (MeBA), methyl salicylate (MeSA), (E)-(1R,9S)-caryophyllene, (E)-β-farnesene and (E,E)-4,8,12-trimethyl-1,3,7,11-tridecatetraene (TMTT) (Birkett et al., 2000; Moraes et al., 2009; Hegde et al., 2012; Sobhy et al., 2017). Most promising was the finding that CJ can also prime maize plants for enhanced production of defensive VOCs that are antagonist toward cicadellid leafhoppers (Oluwafemi et al., 2013). Nevertheless, at present little is known about the extent to which such enhanced production of defensive VOCs, following plant priming with CJ, affects the performance of aphid on other important crops.
In the current study, to explore potential direct effects of the altered VOC emissions during plant priming on aphid performance, we performed growth assays using potato aphid, Macrosiphum euphorbiae (Thomas), observing their reproduction, development, and survival on potato (Solanum tuberosum L.) plants primed with CJ. Macrosiphum euphorbiae is a deleterious polyphagous aphid feeding on hundreds of plant species across 20 taxonomic families worldwide (van Emden and Harrington, 2017). Nevertheless, as an American native aphid, M. euphorbiae have Solanaceae as the preferred hosts and is known to vector many viruses while colonizing potato plants (Srinivasan and Alvarez, 2011). To correlate aphid performance with the emitted VOC profiles, volatile entrainment was conducted and the collected VOCs under various treatments were analyzed. The outcomes of our study could provide further insights into the potential of using priming agents of plant defense as a new ecologically-benign strategy for deployment in combination with current integrated pest management (IPM) tactics against aphids.
Materials and Methods
Plants and Insects
Potato plants, Solanum tuberosum cv. Désirée, were grown in a glasshouse at 25 ± 2°C under a 16L: 8D h photoperiod. In all experiments, potato plants with 30–45 cm height (nearly 3-week-old) were used. For the insect model, we used the potato aphid (Macrosiphum euphorbiae), which was collected from the field at Rothamsted Research (51.8093° N, 0.3548° W). Afterwards, colonies were maintained from parthenogenetic individuals on the above-mentioned potato variety in a controlled environment room (20 ± 1°C, 60 ± 10% RH, 16L: 8D h photoperiod), which ensured continuous asexual reproduction. Growth assay and volatiles entrainment experiments were carried out using apterae (wingless) adults.
cis-Jasmone Application and Plant Treatments
Plants were treated with an aqueous emulsion of cis-jasmone (CJ; 90%; Avocado, Lancaster, UK) as described in Sobhy et al. (2017) using a hydraulic nozzle (Brown 015-F110) mounted on a variable speed spray track at 1 ms−1. Plants were randomly allocated to one of the following treatments: (a) untreated plants (Control); (b) surfactant formulation (SUR): non-ionic surfactant Ethylan BV (100 μl) in deionized water (100 ml); and (c) CJ formulation (CJ): CJ (25 μl) and Ethylan BV (100 μl) in deionized water (100 ml). Ethylan BV is often used in this formulation to emulsify CJ in water and to lower the interfacial tension between the spray and the plant's epidermis to increase CJ uptake (Hazen, 2000). Given its ability to penetrate minute cavities such as stomata (Wood et al., 1997), it is highly possible that this surfactant can affect stomatal conductance and therefore may cause a change in the volatile emission. To rule out surfactant effects, a SUR treatment was also included in our study as a positive control. Prior to experiments, plants were kept for 24 h in separate glasshouse compartments to minimize unwanted induction of plant defense or “priming” effects by plant–plant volatile interactions between treatments. Plants were thereafter moved, with particular care to avoid any damage, from the glasshouse to laboratory where the experiments took place.
Aphid Growth Rate and Performance
The performance of M. euphorbiae on Control, SUR and CJ potato plants was assessed. Previous studies have shown that most of the inducible changes occur after 48 h following CJ treatment (Dewhirst et al., 2012; Sobhy et al., 2017). Therefore, experiments were carried out 48 h after the plant treatment. Six alate M. euphorbiae were placed in one clip cage (5 cm diameter, N = 10), which were attached to the lower surface of potato leaves and left overnight for nymphs viviparity as shown in Figure 1. Subsequently, adults were removed from the clip cages the following morning and neonate nymphs were then collected and weighed together in batches of five in Eppendorf tubes (1.5 mL) on a microbalance (Cahn C33; Scientific and Medical Products Ltd, Manchester, UK), and the average birth weight was calculated. Nymphs were transferred back to fresh potato plants of the same treatment on which they started and then confined to clip cages and their survival and reproduction were monitored over 7 days (Figure 1). The plants were kept in a controlled environment room (25°C, 40% RH, 16L: 8D h photoperiod) with supplementary overhead lighting. After one week, survived aphid individuals were reweighed, and the mean relative growth rate (MRGR) of aphid was calculated (Bruce et al., 2003; Dewhirst et al., 2012), which indicates the growth per unit weight per unit time.
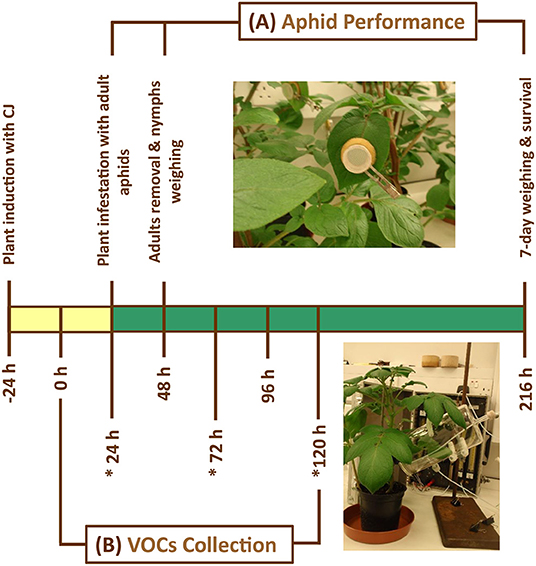
Figure 1. Schematic representation shows the experimental procedure of Macrosiphum euphorbiae performance on potato plants which were allocated randomly to one of the following treatments: (i) Control = untreated plants, (ii) CJ = cis-jasmone-treated plants, and (iii) SUR = surfactant-treated plants. Plants were sprayed and kept separately. (A) Aphid performance assay was carried out 48 after plant treatment. (B) Volatile collection from potato plants was carried out 24 after plant treatment for 5 days in 24 h period. (*) refers to the volatile organic compound (VOC) collection periods that were used in this study. Yellow bar indicates when plants were induced with CJ, whereas green bar indicates when plants were fully primed. The used compound leaves for both experiments were identical in their height and position on the stem of potato plants.
To further study the intrinsic rate of population increase (rm), these obtained nymphs were then allowed to grow on fresh potato plants of the same treatment, which had been treated 48 h earlier. The time taken to reach adulthood and produce their first nymph was recorded, after which the number of neonate nymphs produced per day was noted. To prevent overcrowding, the nymphs were removed soon after recording. The rm value was calculated using the time taken from birth to produce the first nymph (D) and the number of nymphs produced over a period equivalent to time D (FD) starting at the production of the first nymph. We used in the calculations a constant obtained from the mean pre-reproductive times for numerous aphid species (Wyatt and White, 1977), using the following equation:
Data were recorded in the form of number of reproduced nymphs, number of survived nymphs, weights of aphids at birth and after 7 days, the time taken from birth to produce the first nymph and the number of nymphs produced.
Volatile Organic Compound (VOC) Collection
Dynamic headspace collection was carried out following standard procedures as described in Sobhy et al. (2017). For VOC collection, as shown in Figure 1, a compound leaf with 5–7 leaflets from a potato plant was enclosed in a glass vessel (22 cm high × 10 cm internal diameter). It should be noted that we used here identical compound leaves of potato plants to those used earlier in the aphid performance assay in terms of both height and position on potato stem. The bottom of the used glass vessel, which has two ports at the top (one for inlet of air and the other for outlet), was open. To seal such vessels, the bottom was “closed” without pressure around the plant stem using two semicircular aluminum plates with a hole in the center to accommodate the stem, which was wrapped with PTFE tape (Gibbs and Dandy, Luton, UK) at the connection point. To ensure no damage has occurred to plants, plates were clipped to the glass vessel base without constricting the plant. Air, purified by passing through an activated charcoal filter (BDH, 10–14 mesh, 50 g), was pushed into the vessel through the inlet port at 700 ml min−1 (flow rate controlled by needle valve and measured by a flow meter). Air was pulled out at 500 ml min−1 through Porapak Q 50/80 (50 mg, Supelco, Bellefonte, PA, USA) held by two plugs of silanised glass wool in a 5-mm diameter glass tube (Alltech Associates, Carnforth, Lancashire, UK). All connections were made with polytetrafluoroethylene (PTFE) tubing (Alltech Associates) with brass ferrules and fittings (North London Valve, London, UK) and sealed with PTFE tape. Upon each experiment, glassware, metal plates and other equipment were washed with Teepol detergent (Teepol, Kent, UK) in an aqueous solution, acetone and distilled water, and then baked overnight at 180°C. Porapak Q filters were conditioned before use by washing with redistilled diethyl ether (4 ml) and heated to 132°C under a stream of purified nitrogen and kept for 2 h. Diethyl ether was purchased from Sigma Aldrich and was distilled prior to use.
VOC extracts required for GC–MS analyses were collected over 5 days in 24 h periods (n = 3) (Hegde et al., 2012; Sobhy et al., 2017). Although the trapped VOCs in Porapak Q tubes were eluted after each collection period with freshly redistilled diethyl ether (750 μL), we analyzed here only the ones that were collected at 0–24, 48–72, and 96–120 h to be thus consonant with the growth assay. The samples were concentrated under a stream of nitrogen to ~50 μL and stored in small vials (Supelco, Amber Vial, 7 mL with solid cap w/PTFE liner) at −20°C until required for analysis.
Chemical Analysis
VOC extracts were analyzed by high-resolution gas chromatography (GC) using an Agilent 6890 GC equipped with a cool on-column injector, flame ionization detector (FID) and a nonpolar HP-bonded phase fused silica capillary column (50 m × 0.32 mm inner diameter, film thickness 0.5 μm; J & W Scientific). The GC oven temperature was maintained at 30°C for 1 min after sample injection and then raised by 5°C min−1 to 150°C, thereafter by 10°C min−1 to 230°C. The carrier gas was hydrogen. 4 μl of each eluted sample were manually injected into the injector port of the GC instrument.
Coupled gas chromatography–mass spectrometry (GC–MS) analysis of VOCs from treated potato plants was performed using a non-polar column (HP-1, 50 m × 0.32 mm inner diameter, film thickness 0.5 μm), attached to a cool on–column injector, which was directly coupled to a magnetic sector mass spectrometer (Autospec Ultima, Fisons Instruments, Manchester, UK). Ionization was by electron impact at 70 eV, 250°C (source temperature). Helium was the carrier gas. The GC oven temperature was maintained at 30°C for 5 min, and then programmed at 5°C min−1 to 250°C.
Tentative identifications were made by comparison of spectra with mass spectral databases (NIST, 2011). Peak enhancement by co-injection with authentic standards confirmed tentative identifications (Pickett, 1990). Excluding (E)-β-farnesene, (E)-4,8-dimethyl-1,3,7-nonatriene (DMNT), and (E,E)-4,8,12-trimethyl-1,3,7,11-tridecatetraene (TMTT) that were synthesized as described in Sobhy et al. (2017), chemicals (>95% pure) were obtained from Sigma-Aldrich® (Gillingham, Kent, UK) and Botanix Ltd® (Paddock Wood, Kent, UK). An external standard (100 ng/μl non-anal) method was used to quantify the amount of identified chemical components present in the air entrainment samples. The amounts of compounds present in VOC extracts were determined in accordance with the weight of sampled plant material, and the duration of entrainment period. Data were analyzed using HP Chemstation software.
Data Visualization and Statistics
Visualization together with hierarchical clustering of VOCs data was done using the comprehensive online tool suite MetaboAnalyst 4.0 (Chong et al., 2018). Data matrix was first mean-centered, cube-root transformed prior to analysis. Average linkage hierarchical clustering based on Ward clustering algorithm of the Euclidean distance measure for the differentially emitted VOCs detected by ANOVA (Tukey's post-hoc tests, P < 0.05) was used to construct a heatmap. Additionally, principal component analysis (PCA) was used to visualize overall differences among VOC blends emitted from plants subjected to each treatment (i.e., Control, CJ, and SUR) at each collection point (i.e., 24, 72, and 120 h). In this analysis, we used two outputs from the data; a matrix of “scores” which provides the location of each compound along each principal component (PC) and a matrix of “loadings” which indicates the strength of correlation between individual VOCs and each PC.
We used multivariate analysis of variance (two-way MANOVA) to analyze the effects of treatment, sampling time, and their interaction on volatile emission, with the “treatment” and “time” as fixed factors and the volatile compounds as dependent variables (IBM SPSS Statistics version 22.0, Armonk, NY, USA). Given that these VOCs share common precursors and their variation patterns within groups of compounds can be attributed to variation in the quantity of their common precursor (Hare, 2011), VOCs were grouped into alcohols, aldehydes, benzenoids, esters, homoterpene, ketones, monoterpenes, and sesquiterpenes based on their functional or biosynthetic characteristics. Groups containing two compounds (or more) were considered for two-way MANOVA. Subsequently, two-way MANOVA was followed by two-way ANOVA for the different VOC groups on the sum of all compounds under each to determine the effects of treatment, sampling time and their interaction, or by one-way ANOVA to only determine the effects of treatment only, followed by Tukey post-hoc comparisons (P < 0.05). Two-way ANOVA was also performed on individual compounds not included in MANOVA, and on total emitted volatiles (i.e., the sum of all VOCs).
For the aphid performance experiments, natural log (ln) transformed data for birth, 7 days and adult weights, the MRGR, D, FD, and rm were compared by one-way ANOVA using SigmaPlot 12.3 (SPSS Inc., Chicago, IL, USA). A natural log (ln) transformation was needed in order to normalize the residuals of the weight data. Prior to analysis, data were examined for a Normal distribution using the Shapiro-Wilk test. Comparisons among means were performed using Tukey post-hoc test (P < 0.05).
Results
Aphid Performance Bioassay
The growth rate parameters of M. euphorbiae were significantly affected by CJ treatment. Females of M. euphorbiae produced significantly less neonate nymphs on treated potato plants compared to control or SUR plants [F(2, 27) = 27.94; P = <0.001; Figure 2A]. In addition, the survival of M. euphorbiae nymphs significantly decreased on CJ-treated than on control plants [F(2, 27) = 40.05; P = <0.001; Figure 2B]. Further, the MRGR of M. euphorbiae nymphs that fed on CJ treated plants was significantly lower compared to those reared on either control or SUR plants [F(2, 27) = 6.203; P = 0.006; Figure 2C]. The mean intrinsic rate of population increase (rm) of M. euphorbiae was significantly reduced on CJ-treated potato compared to control or SUR plants [F(2, 27) = 6.716; P = 0.004; Figure 2D].
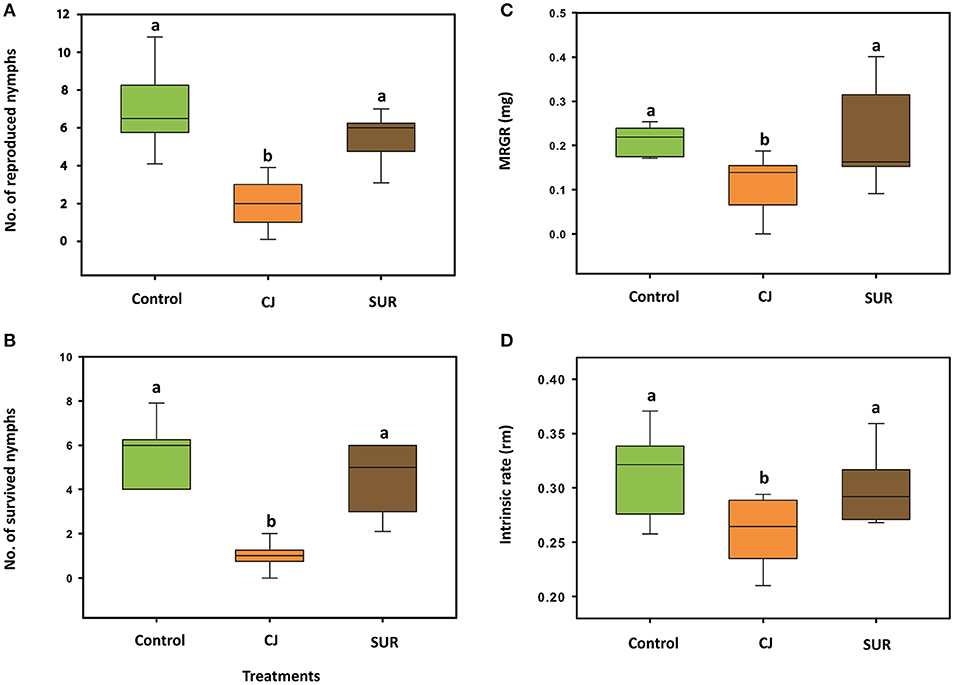
Figure 2. Performance of Macrosiphum euphorbiae on treated potato plants. Control = non-sprayed plants, CJ = cis-jasmone-sprayed plants and SUR = surfactant-sprayed plants. (A) Mean (±SE) no. of reproduced nymphs 24 h after infestation. (B) Mean (±SE) no. of survived nymphs after 7 days. (C) Mean relative growth rate (MRGR) of M. euphorbiae nymphs fed on sprayed potato plants for 7 days. (D) Intrinsic rate of population increase of M. euphorbiae nymphs fed on sprayed potato plants. Different letters indicate statistically significant differences (P < 0.05), based on Tukey's post-hoc test (F-test).
Volatile Emissions
GC-MS Analysis of headspace collections from potato plants revealed the presence of 22 detectable VOCs following eight various classes based on their functional or biosynthetic characteristics (i.e., alcohols, aldehydes, benzenoids, esters, homoterpenes, ketones, monoterpenes, and sesquiterpenes). Overall, two-way MANOVA revealed that the emission of these VOC classes from potato plants was affected significantly by treatments, sampling time, and their interaction (Table 1). In addition, most volatile individuals under the above-mentioned VOC groups were significantly affected by potato treatment with CJ, particularly during 48–72 and 96–120 h after volatile collection was initiated (Table 2). More specifically, the total amount of emitted VOCs was significantly higher from CJ-treated plants compared to the control or SUR plants [0–24 h: F(2, 6) = 165.93, P < 0.001; 48–72 h: F(2, 6) = 154,07, P < 0.001; 96–120 h: F(2, 6) = 232.89, P < 0.001], particularly due to the high emission of several VOCs from CJ-treated plants at the three collection points (i.e., 0–24, 48–72, 96–120 h), including methyl salicylate (MeSA), indole, DMNT, TMTT, CJ, MHO, and (E)-β-farnesene (Table 2). VOCs that were significantly increased but for which identifications remained tentative included (Z)-3-hexen-1-ol, a longifolene isomer, (E)-(1R,9S)-caryophyllene, and a germacrene D isomer (Table 2). Similarly, two-way ANOVA revealed that the emission of all assigned VOC groups, the individual compounds [i.e., (Z)-3-hexen-1-ol and (Z)-3-hexen-1-yl butyrate] and total emitted VOCs were significantly affected by both factors (i.e., treatments and sampling time) and their interactions with one exception for (Z)-3-hexen-1-yl butyrate (Supplementary Table S1).
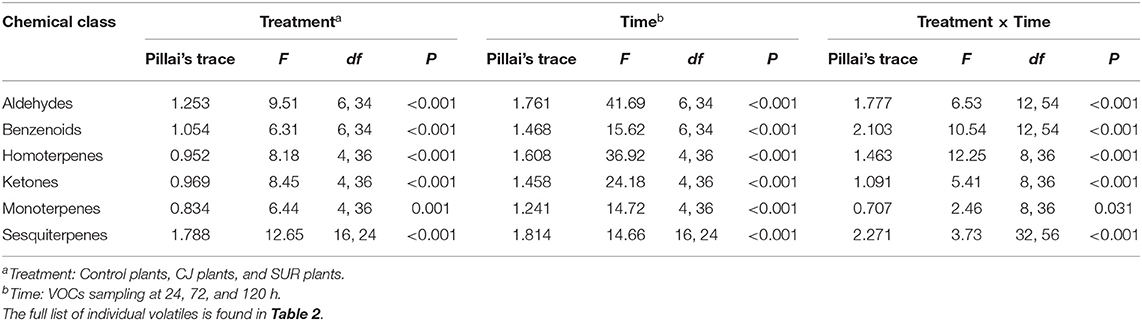
Table 1. Results of Two-way MANOVA for the effects of plant treatment, VOCs sampling time, and their interaction on potato volatile emission.
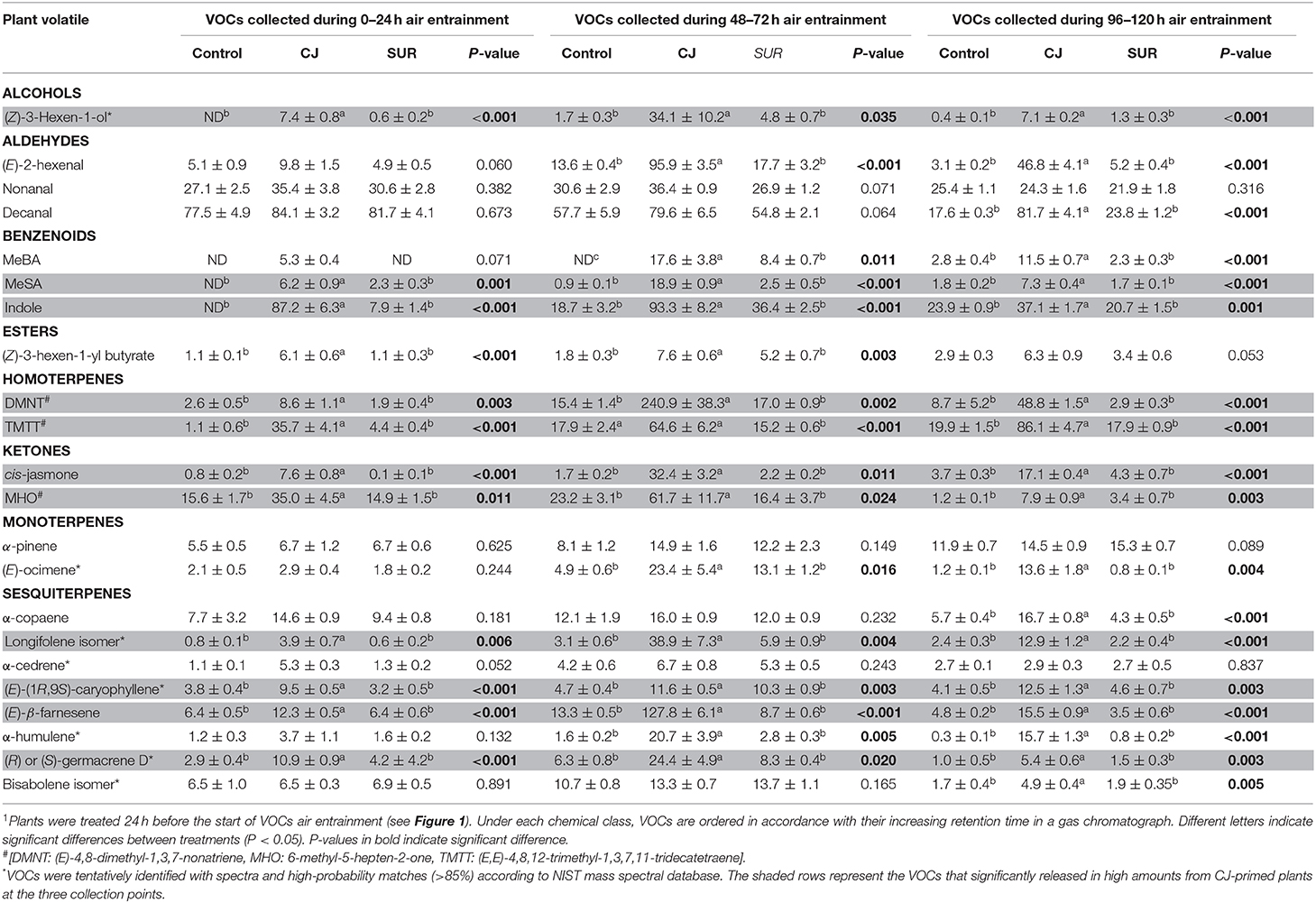
Table 2. Emission (ng ± SE) of volatiles released by cis-jasmone treated (CJ), surfactant-treated (SUR), and non-treated (Intact) potato plants over 24, 72, and 120 h air entrainment1.
The resulting heatmap showed differential magnitude of VOC emission between treatments with a greater increase from CJ-treated plants (Figure 3).
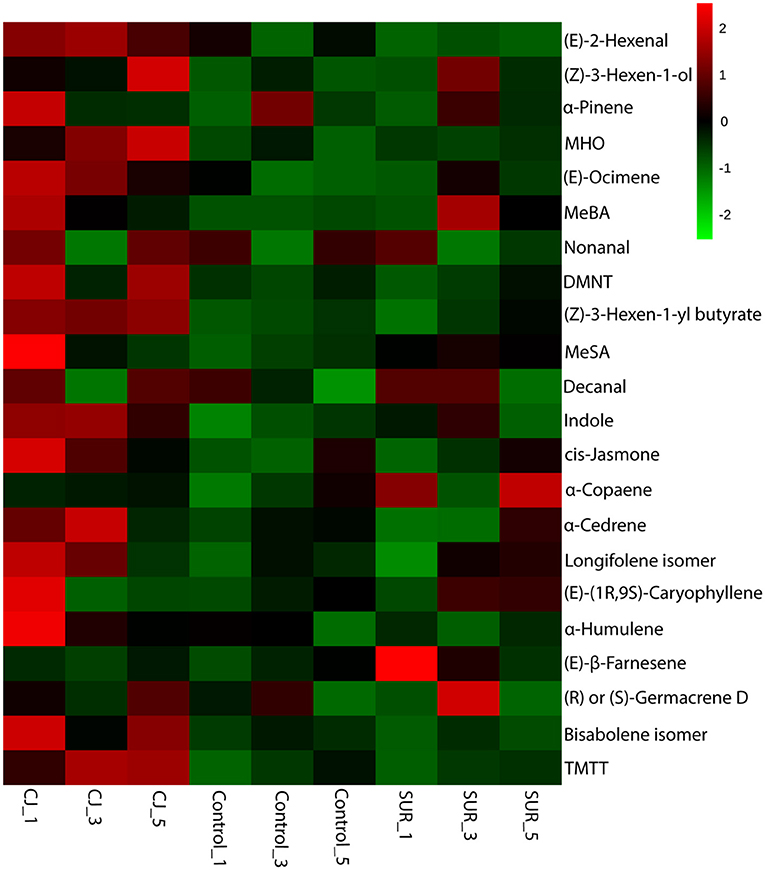
Figure 3. Hierarchical cluster of the volatile profiles emitted by potato plants that were treated with cis-jasmone (CJ) or surfactant (SUR) in comparison to untreated plants (Control). Cube root transformed values of biological replicates (n = 3) are represented as a heatmap according to the scale in which red corresponds to higher VOCs emission whereas green denotes lower VOCs emission. The heatmap was constructed using the average of three biological replicates in each collection point (i.e., 24, 72, and 120 h). The sample/VOC grouping is based on Ward clustering algorithm of the Euclidean distance measure for the differentially emitted VOCs detected by ANOVA (Tukey's post-hoc tests, P < 0.05). Quantitative data are shown in Table 2.
Furthermore, principal component analysis (PCA) of the VOCs showed that the first two principal components accounted for around 47.8, 46.1, and 43.6% of the total variation between treatment groups at 0–24, 48–72, and 96–120 h VOC collection points, respectively (Figure 4). Hence, these two PCs illustrated most of the variation in the data of likely biological relevance. Overall, PCA revealed a clear separation in the obtained VOC profiles, which was mainly driven by cis-jasmone treatment. Specifically, the score plot visualized three neatly separated groups of control plants, SUR plants and CJ-primed plants at 0–24, 48–72, and 96–120 h VOC collection points. The greatest loadings of PC10–24h, in descending order, were for TMTT (0.31), α-pinene (0.29), and DMNT (0.27), whereas the greatest loadings of PC20–24h were for longifolene isomer (0.31), bisabolene isomer (0.28), and cis-jasmone (0.25). In addition, the main loadings of PC148–72h were for α-copaene (0.37), bisabolene isomer (0.30), and MHO (0.23), whereas the main loadings of PC248–72h were for (E)-ocimene (0.42), cis-jasmone (0.39), and indole (0.27). Finally, the major loadings of PC196–120h were for MeBA (0.36), MHO (0.34), and α-humulene (0.31), whereas the major loadings of PC248–72h were for (Z)-3-hexen-1-ol (0.33), DMNT (0.32), and (E)-ocimene (0.31). This suggests that these VOCs are contributing to both PC1 and PC2 at each collection point and may subsequently impact aphid overall performance on potato. Interestingly, as shown in Figure 4C, the vectors of several VOCs were more associated with CJ treatment, indicating enhanced VOC emissions from CJ-plants.
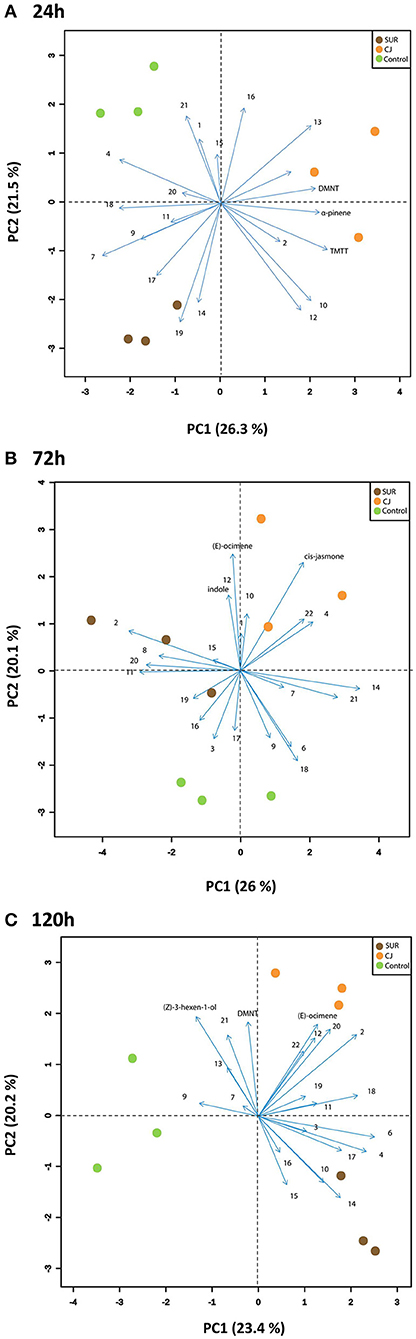
Figure 4. Principal Component Analysis (PCA) of the volatile profiles produced from the different treated potato plants (i.e., Control, non-treated plants; CJ, cis-jasmone-treated plants; SUR, surfactant-treated plants). Score plot visualizes the location of each collected sample on each PC at 24 h (A), 72 h (B), and 120 h (C) with the percentage of explained variation in parentheses, whereas vectors (blue lines) visualize the loadings for each volatile compound. Vector numbers refer to the different volatile compounds measured, including (1) (Z)-3-hexen-1-ol, (2) (E)-2-hexenal, (3) α-pinene, (4) 6-methyl-5-hepten-2-one, (5) (E)-ocimene, (6) methyl benzoate (MeBA), (7) nonanal, (8) (E)-4,8-dimethyl-1,3,7-nonatriene (DMNT), (9) (Z)-3-hexen-1-yl butyrate, (10) methyl salicylate (MeSA), (11) decanal, (12) indole, (13) cis-jasmone (CJ), (14) α-copaene, (15) α-cedrene, (16) longifolene isomer, (17) (E)-(1R,9S)-caryophyllene, (18) α-humulene, (19) (E)-β-farnesene, (20) (R) or (S)-germacrene D, (21) bisabolene isomer, and (22) (E,E)-4,8,12-trimethyl-1,3,7,11-tridecatetraene (TMTT).
Discussion
The prospect of potentiating plant defense against insects by using priming agents that are naturally derived from plants has received recently substantial attention because of its potential for providing more environment-friendly agrochemicals for crop protection (Bruce et al., 2017; Turlings and Erb, 2018). Here, we demonstrate that potato pretreatment with the priming agent CJ reduces aphid reproduction, survival, intrinsic rate of population increase and overall performance. In addition, primed potato plants notably release elevated profiles of defense-related VOCs. Further, a significant negative correlation between elevated VOC profiles and aphid performance was detected when the plants were primed by CJ. This may in turn affect aphid settling on treated plants.
cis-Jasmone Negatively Impacts Aphid Performance
Growth assays revealed that adults of M. euphoribae produced fewer neonates on CJ plants compared to control ones. Similarly, the numbers of grain aphid (Sitobion avenae Fabricius) were reduced in plots of winter wheat treated with CJ (Birkett et al., 2000; Bruce et al., 2003). Using our study model, Brunissen et al. (2010) reported that potato treatment with MeJA, which is JA volatile derivative, reduced the fecundity of M. euphoribae and further prolonged its pre-reproductive period. Likewise, induced tomato plants drastically reduced the reproductive rate of M. euphorbiae (Guerrieri et al., 2004).
The suppressed performance of aphids on CJ-primed plants has been reported on many different crop plants. For instance, cereal aphids were negatively affected by CJ treatment and fewer aphids were observed on CJ plants than on untreated ones (Bayram and Tonğa, 2018). Furthermore, the developmental rate and MRGR, but not intrinsic rate, of the glasshouse potato aphid (Aulacorthum solani Kalt.) were significantly reduced on sweet pepper plants treated with CJ (Dewhirst et al., 2012). Congruously, Bruce et al. (2003) reported a reduced intrinsic rate of population increase and fewer numbers of grain aphid on CJ-treated wheat. This negative influence of CJ application can be also extended to include the foraging activity of aphids (Paprocka et al., 2018). Interestingly, more deleterious insects are negatively influenced by CJ application as such as thrips (Egger and Koschier, 2014; Egger et al., 2016) and stink bugs (da Graça et al., 2016). Further, not only puncturing and/or sucking insects are adversely affected by CJ application, but also chewing lepidopteran pests such as the noctuid, Spodoptera exigua (Hübner), which laid fewer egg masses when reared on CJ-treated tomato plants (Disi et al., 2017). Such broader negative impact on diverse herbivore species raises the question whether CJ may be a common defense-priming signal in nature.
cis-Jasmone Elicits Elevated Volatile Profiles
VOC analysis disclosed a drastic increase in VOC emission from CJ primed plants which clearly illustrated by heatmap analysis. Most promising was our finding that such increased VOC emission was detected at the three collection points (i.e., 0–24, 48–72, and 96–120 h), suggesting long-lasting induction for nearly a week following plant treatment with CJ.
(Z)-3-hexen-1-ol was significantly emitted in higher amounts from CJ plants. (Z)-3-Hexen-1-ol is a key VOC emitted from plants upon aphid herbivory (Webster et al., 2008), as demonstrated by being electrophysiologically and behaviorally active to aphids (Webster et al., 2010) and highly attractive to their natural enemies (Du et al., 1998).
Benzenoid VOCs (i.e., MeSA and indole) were released in considerably higher amounts from CJ-primed plants at all VOC sampling points. Concordant with this, cotton priming with CJ induced the emission of several VOCs, including MeSA (Hegde et al., 2012). For the latter benzenoid, previous work has shown an increased emission of indole from CJ-primed plants (Delaney et al., 2013), which has been shown to be an electrophysiologically active VOC to M. euphorbiae (Sobhy et al., 2017), suggesting its potential role in potato/aphid interactions.
Production of both homoterpenes, DMNT and TMTT, was robustly elicited upon plant treatment with CJ. Similarly, the release of TMTT and/or DMNT was substantially increased following bean (Birkett et al., 2000), soybean (Moraes et al., 2009), maize (Oluwafemi et al., 2013), and potato (Sobhy et al., 2017) treatment with CJ. Boosting the long-lasting induction of CJ treatment, induced release of DMNT and TMTT was observed in CJ-primed cotton over a period of 5 days (Hegde et al., 2012). Interestingly, plant application with other priming agents such as BTH, laminarin (β-1,3 glucan) and JA also increased the emission of both homoterpenes (Smart et al., 2013; Sobhy et al., 2015a, 2018).
CJ treated plants released higher amounts of the ketones MHO and CJ, which have been shown to possess biological activity against aphids (Sobhy et al., 2017). In particular, a higher emission of MHO was reported from various crop plants treated with jasmonate related compounds, such as CJ (Pickett et al., 2007a; Dewhirst et al., 2012; Sobhy et al., 2017) and MeJA (Yu et al., 2018). It should be noted that CJ itself is a stress-related VOC that is emitted in elevated concentrations from plants treated with different priming agents (Sobhy et al., 2015a, 2017).
Of all detected sesquiterpenes, the emission of (E)-β-farnesene and the tentatively identified longifolene isomer, (E)-(1R,9S)-caryophyllene, and (R) or (S)-germacrene D was notably induced upon CJ treatment. Consistent with our results, Oluwafemi et al. (2013) found that primed maize released higher quantities of germacrene D when exposed to CJ. Moreover, it has been also shown that significant emission of germacrene D was released from plants induced by either JA (Obara et al., 2002) or MeJA (Yu et al., 2018). A growing body of evidence suggest that plant priming with CJ elicits the emission of (E)-(1R,9S)-caryophyllene and (E)-β-farnesene (Delaney et al., 2013; Oluwafemi et al., 2013). It should be noted that majority of the above-mentioned VOCs that were induced upon CJ priming are indeed key semiochemicals in plant/aphid interactions.
Associational Resistance of cis-Jasmone Primed Plants
One mechanistic interpretation for the reduced aphid performance observed on CJ-primed plants would be attributed to the higher emission of aphid defense-related HIPVs following CJ application. Aphids may therefore perceive such induced or altered HIPV profiles from CJ-primed plants as signals of a greater risk of competition from conspecifics or as a greater risk of predation from natural enemies (Bruce and Pickett, 2011). Consistent with our findings, the coordinated defense responses expressed in primed plants, when exposed to contact-induced volatiles, reduced aphid settling, making these plants a less suitable host for aphids (Markovic et al., 2019). Furthermore, exposing potato to VOCs from undamaged onion plants altered its volatile profile and this had a deterrent effect against host-seeking M. persicae, which was further confirmed in the field where migration of aphids into potato was significantly reduced by intercropping with onion (Ninkovic et al., 2013). Similarly, lower MRGR of the bird cherry oat aphid (Rhopalosiphum padi L) was reported on barley plants exposed to the volatiles of the weed Chenopodium album (Ninkovic et al., 2009). Remarkably, such reduced aphid performance on primed plants can be also obtained by exposing these aphid-infested plants to a single stress-related volatile. For instance, the fertility of M. euphoribae was significantly reduced when individuals fed on tomato plants exposed to MeSA (Digilio et al., 2012). In addition, exposing Chinese cabbage to β-ocimene negatively influenced the feeding behavior of M. persicae (Kang et al., 2018). Similarly, using M. euphoribae, significant lower numbers of newborn nymphs and lower number of settled individuals, with lower weight, were recorded on tomato plants when primed by β-ocimene (Cascone et al., 2015). More recently, Maurya et al. (2019) reported a reduced fecundity of pea aphid on barrel medic plants upon their exposure to indole.
Multivariate analysis (PCA) of VOC data further indicated which volatiles may be involved in aphid reduced performance. In particular, TMTT, α-pinene, and DMNT had the greatest loadings for PC1, which separated the CJ from the Control and SUR treatments, from the 0–24 h air entrainment. For both the 48–72 and 96–120 h air entrainment periods, PC2 separated the CJ from the Control and SUR treatments. (E)-ocimene, cis-jasmone and indole had the greatest loadings for PC248–72h, whereas (Z)-3-hexen-1-ol, DMNT, and (E)-ocimene had the greatest loadings for PC296–120h and thus were much likely dominating in the emitted VOC blends. This suggests that these volatiles correlate most strongly with aphid reduced performance. Indeed, these VOCs are key semiochemicals in aphid trophic interactions and are directly associated with aphid repellency and/or attraction of aphid natural enemies. Previous work has shown an increased emission of (Z)-3-hexen-1-ol (Sasso et al., 2007; Webster et al., 2008), α-pinene (Sasso et al., 2007), (E)-β-ocimene (Sasso et al., 2007; Staudt et al., 2010), DMNT, and TMTT (Hegde et al., 2011), CJ (Birkett et al., 2000), and indole (Sobhy et al., 2017) in response to aphid herbivory. It should be noted that these aphid-stress signals are significantly repellent to aphids (Bruce et al., 2005; Beale et al., 2006; Hegde et al., 2011). Further, we have shown recently that alate M. euphorbiae showed electrophysiological activity to most of these VOCs that are emitted from CJ treated plants (Sobhy et al., 2017). Alternatively, (Z)-3-hexen-1-ol (Du et al., 1998; Sasso et al., 2009), α-pinene (Corrado et al., 2007), (E)-β-ocimene (Birkett et al., 2000), DMNT and TMTT (Hegde et al., 2011), CJ (Birkett et al., 2000), and indole (Han and Chen, 2002) have shown robust potential in attracting several natural enemies of aphids. This points to possible association of the emission of these VOCs as danger signals and the likely risk of being attacked by their parasitoids and/or predators.
The reduced performance of other herbivores following exposure to HIPVs has been reported previously. For example, exposure to HIPVs decreased the growth rate of Spodoptera littoralis (Boisduval) caterpillars at early larval stages (von Mérey et al., 2013). In addition, leaf beetle, Plagiodera versicolora (Laicharting), larvae showed decreased performance on uninfested host plants when exposed to HIPVs from plants infested by conspecific larvae (Yoneya et al., 2014). Interestingly, such decreased herbivore performance following exposure to HIPVs can be also achieved by treating the plants with priming agents. In this respect, using CJ but with a chewing herbivore, females of S. exigua laid fewer egg masses when subjected to filter papers containing VOCs of CJ-primed plants (Disi et al., 2017). Furthermore, plant treatment with the priming agent prohydrojasmone [propyl (1RS, 2RS)-(3-oxo-2-pentylcyclopentyl) acetate] negatively affected the oviposition of Tetranychus urticae Koch (Uefune et al., 2013), due to several quantitative and qualitative changes in HIPVs in prohydrojasmone-treated plants.
Additional indirect evidence on the potential of bioactive volatiles as priming agents that render exposed plants less appealing to herbivores and possibly more attractive to natural enemies comes from cotton growers in developing countries. More specifically, farmers used to remove the growing buds of cotton plants just before flowering, which is known as cotton topping (Renou et al., 2011). This in turn, in addition to branching and flowers increase, significantly reduced the attack of many lepidopteran pests in cotton. Remarkably, this not only occurred in topped plants, but also was extended to encompass neighboring plants that were not topped, suggesting that the released VOCs from topped plants can protect their neighbor plants from an impending attack by repelling herbivores and/or attracting beneficial insects (Llandres et al., 2019).
Another interpretation of the reduced performance of M. euphorbiae when fed on CJ-plants is that treating plants with CJ may induce the accumulation of certain anti-herbivore secondary metabolites that renders CJ-plants more inappropriate for aphid survival. In this respect, significantly higher levels of 2,4-dihydroxy-7-methoxy-2H-1,4-benzoxazin-3(4H)-one (DIMBOA), 2-hydroxy-7-methoxy-(2H)-1,4-benzoxazin-3(4H)-one (HBOA), and phenolic acids were detected in aerial parts and roots of wheat, following CJ treatment (Moraes et al., 2008). Similarly, increased levels of the defensive isoflavonoids (i.e., daidzein and genistein) were reported by da Graça et al. (2016) upon CJ treatment, which negatively impacted the weight gain of stink bug (Euschistus heros).
Yet, many questions remain unknown and require more studies to answer, especially concerning the underlying induced changes upon plant priming and how induced responses precisely reduce the herbivore performance. To this end and to obtain further empirical evidence, it is advised that similar experimental work should be performed but using knock-out mutant plants in which the pathways producing specific classes of VOCs are silenced (Matthes et al., 2010; Christensen et al., 2013).
Potential Implications for Durable Aphid Control in Potato
Potato is currently one of the five major crops worldwide that contributes with 2.2% of the global human calorific intake (King and Slavin, 2013). While the increase in consumption requires drastic increase in potato yield (FAO, 2009), insect infestation is still a major constraint reducing potato production worldwide, especially those vector plant viruses and have tremendous indirect damage such as aphids (van Emden and Harrington, 2017). Our results show clear evidence that CJ application decreases aphid growth, reproduction, intrinsic rate and survival. These life history traits are indeed vital factors determining the success of aphid population (Leather and Dixon, 1984). Thus, negatively affecting these traits would likely reduce the overall aphid fitness. Given the current thinking that effective control does not necessarily have to rely on aphid mortality, we believe that priming potato defense against aphids using CJ has the potential to provide new opportunities for sustainable crop protection, especially if being used in the context of IPM, through the induction of VOC emissions (Turlings and Erb, 2018; Brilli et al., 2019). This could minimize the need for applying conventional toxic agrochemicals in potato fields. Bearing in mind that plant genotype, application method and other environmental conditions strongly influence the magnitude of any enhancement in plant defense acquired by these priming agents, more field research is needed before upscaling their application under field conditions.
Data Availability Statement
The datasets generated for this study are available on request to the corresponding author.
Ethics Statement
Experimental manipulation of aphids and plants occurred according to the common and ethical requirements for organism's welfare where both insects and plants, used in this study, were carefully handled during experiments and maintained in the laboratory under appropriate conditions.
Author Contributions
IS, JP, and MB conceived the ideas and designed the experiments. IS performed the experiments and collected the data. IS, JC, JP, and MB analyzed the data. IS and JC contributed to the GC chemical analysis. IS, JP, and MB led the writing of the manuscript. All authors contributed critically to the drafts and gave final approval for publication.
Funding
This work was supported by a Rothamsted International Fellowship (RIF Project No: 0006) to IS. Rothamsted Research receives grant-aided support from the Biotechnology and Biological Sciences Research Council (BBSRC) of the United Kingdom.
Conflict of Interest
The authors declare that the research was conducted in the absence of any commercial or financial relationships that could be construed as a potential conflict of interest.
Acknowledgments
We deeply thank Janet Martin for aphid rearing, Barry Pye for CJ plant treatment, and Lesley Smart for her help in the experimental set-up. IS gratefully acknowledges the permission of Suez Canal University, Ismailia, Egypt for his leave of absence to conduct research in UK.
Supplementary Material
The Supplementary Material for this article can be found online at: https://www.frontiersin.org/articles/10.3389/fevo.2019.00499/full#supplementary-material
References
Bass, C., Puinean, A. M., Zimmer, C. T., Denholm, I., Field, L. M., Foster, S. P., et al. (2014). The evolution of insecticide resistance in the peach potato aphid, Myzus persicae. Insect Biochem. Mol. Biol. 51, 41–51. doi: 10.1016/j.ibmb.2014.05.003
Bayram, A., and Tonğa, A. (2018). cis-Jasmone treatments affect pests and beneficial insects of wheat (Triticum aestivum L.): the influence of doses and plant growth stages. Crop Prot. 105, 70–79. doi: 10.1016/j.cropro.2017.11.011
Beale, M. H., Birkett, M. A., Bruce, T. J. A., Chamberlain, K., Field, L. M., Huttly, A. K., et al. (2006). Aphid alarm pheromone produced by transgenic plants affects aphid and parasitoid behavior. Proc. Natl. Acad. Sci. U.S.A. 103, 10509–105013. doi: 10.1073/pnas.0603998103
Birkett, M. A., Campbell, C. A., Chamberlain, K., Guerrieri, E., Hick, A. J., Martin, J. L., et al. (2000). New roles for cis-jasmone as an insect semiochemical and in plant defense. Proc. Natl. Acad. Sci. U.S.A. 97, 9329–9334. doi: 10.1073/pnas.160241697
Blackman, R. L., and Eastop, V. F. (2006). Aphids on the World's Herbaceous. Chichester: John Wiley & Sons Ltd.
Boughton, A. J., Hoover, K., and Felton, G. W. (2006). Impact of chemical elicitor applications on greenhouse tomato plants and population growth of the green peach aphid, Myzus persicae. Entomol. Exp. Appl. 120, 175–188. doi: 10.1111/j.1570-7458.2006.00443.x
Brilli, F., Loreto, F., and Baccelli, I. (2019). Exploiting plant volatile organic compounds (VOCs) in agriculture to improve sustainable defense strategies and productivity of crops. Front. Plant Sci. 10:264. doi: 10.3389/fpls.2019.00264
Bruce, T. J. (2014). Variation in plant responsiveness to defense elicitors caused by genotype and environment. Front. Plant Sci. 5:349. doi: 10.3389/fpls.2014.00349
Bruce, T. J. (2015). Interplay between insects and plants : dynamic and complex interactions that have coevolved over millions of years but act in milliseconds. J. Exp. Bot. 66, 455–465. doi: 10.1093/jxb/eru391
Bruce, T. J., Birkett, M. A., Blande, J., Hooper, A. M., Martin, J. L., Khambay, B., et al. (2005). Response of economically important aphids to components of Hemizygia petiolata essential oil. Pest Manag. Sci. 61, 1115–1121. doi: 10.1002/ps.1102
Bruce, T. J., and Pickett, J. A. (2011). Perception of plant volatile blends by herbivorous insects-finding the right mix. Phytochemistry 72, 1605–1611. doi: 10.1016/j.phytochem.2011.04.011
Bruce, T. J. A. (2010). Tackling the threat to food security caused by crop pests in the new millennium. Food Secur. 2, 133–141. doi: 10.1007/s12571-010-0061-8
Bruce, T. J. A., Martin, J. L., Pickett, J. A., Pye, B. J., Smart, L. E., and Wadhams, L. J. (2003). cis-jasmone treatment induces resistance in wheat plants against the grain aphid, Sitobion avenae (Fabricius) (Homoptera: Aphididae). Pest Manag. Sci. 59, 1031–1036. doi: 10.1002/ps.730
Bruce, T. J. A., Smart, L. E., Birch, A. N. E., Blok, V. C., MacKenzie, K., Guerrieri, E., et al. (2017). Prospects for plant defence activators and biocontrol in IPM – Concepts and lessons learnt so far. Crop Prot. 97, 128–134. doi: 10.1016/j.cropro.2016.10.003
Brunissen, L., Vincent, C., Le Roux, V., and Giordanengo, P. (2010). Effects of systemic potato response to wounding and jasmonate on the aphid Macrosiphum euphorbiae (Sternorryncha: Aphididae). J. Appl. Entomol. 134, 562–571. doi: 10.1111/j.1439-0418.2009.01493.x
Cascone, P., Iodice, L., Maffei, M. E., Bossi, S., Arimura, G., and Guerrieri, E. (2015). Tobacco overexpressing β-ocimene induces direct and indirect responses against aphids in receiver tomato plants. J. Plant Physiol. 173, 28–32. doi: 10.1016/j.jplph.2014.08.011
Chong, J., Soufan, O., Li, C., Caraus, I., Li, S., Bourque, G., et al. (2018). MetaboAnalyst 4.0: towards more transparent and integrative metabolomics analysis. Nucleic Acids Res. 46, W486–W494. doi: 10.1093/nar/gky310
Christensen, S. A., Nemchenko, A., Borrego, E., Murray, I., Sobhy, I. S., Bosak, L., et al. (2013). The maize lipoxygenase, ZmLOX10, mediates green leaf volatile, jasmonate and herbivore-induced plant volatile production for defense against insect attack. Plant J. 74, 59–73. doi: 10.1111/tpj.12101
Cooper, W. C., Jia, L., and Goggin, F. L. (2004). Acquired and R-gene-mediated resistance against the potato aphid in tomato. J. Chem. Ecol. 30, 2527–2542. doi: 10.1007/s10886-004-7948-9
Corrado, G., Sasso, R., Pasquariello, M., Iodice, L., Carretta, A., Cascone, P., et al. (2007). Systemin regulates both systemic and volatile signaling in tomato plants. J. Chem. Ecol. 33, 669–681. doi: 10.1007/s10886-007-9254-9
da Graça, J. P., Ueda, T. E., Janegitz, T., Vieira, S. S., Salvador, M. C., de Oliveira, M. C. N., et al. (2016). The natural plant stress elicitor cis-jasmone causes cultivar-dependent reduction in growth of the stink bug, Euschistus heros and associated changes in flavonoid concentrations in soybean, Glycine max. Phytochemistry 131, 84–91. doi: 10.1016/j.phytochem.2016.08.013
Dabrowska, P., and Boland, W. (2007). Iso-OPDA: an early precursor of cis-jasmone in plants? Chembiochem 8, 2281–2285. doi: 10.1002/cbic.200700464
De Moraes, C. M., Mescher, M. C., and Tumlinson, J. H. (2001). Caterpillar-induced nocturnal plant volatiles repel conspecific females. Nature 410, 577–580. doi: 10.1038/35069058
Delaney, K. J., Wawrzyniak, M., Lemanczyk, G., Wrzesinska, D., and Piesik, D. (2013). Synthetic cis-jasmone exposure induces wheat and barley volatiles that repel the pest cereal leaf beetle, Oulema melanopus L. J. Chem. Ecol. 39, 620–629. doi: 10.1007/s10886-013-0281-4
Desneux, N., Decourtye, A., and Delpuech, J. M. (2007). The sublethal effects of pesticides on beneficial arthropods. Annu. Rev. Entomol. 52, 81–106. doi: 10.1146/annurev.ento.52.110405.091440
Dewhirst, S. Y., Birkett, M. A., Loza-Reyes, E., Martin, J. L., Pye, B. J., Smart, L. E., et al. (2012). Activation of defence in sweet pepper, Capsicum annum, by cis-jasmone, and its impact on aphid and aphid parasitoid behaviour. Pest Manag. Sci. 68, 1419–1429. doi: 10.1002/ps.3326
Digilio, M. C., Cascone, P., Iodice, L., and Guerrieri, E. (2012). Interactions between tomato volatile organic compounds and aphid behaviour. J. Plant Interact. 7, 322–325. doi: 10.1080/17429145.2012.727104
Disi, J. O., Zebelo, S., Ngumbi, E., and Fadamiro, H. Y. (2017). cis-Jasmone primes defense pathways in tomato via emission of volatile organic compounds and regulation of genes with consequences for Spodoptera exigua oviposition. Arthropod. Plant. Interact. 11, 591–602. doi: 10.1007/s11829-017-9503-y
Du, Y. J., Poppy, G. M., Powell, W., Pickett, J., Wadhams, L. J., and Woodcock, C. M. (1998). Identification of semiochemicals released during aphid feeding that attract parasitoid Aphidius ervi. J. Chem. Ecol. 24, 1355–1368. doi: 10.1023/A:1021278816970
Dudareva, N., Klempien, A., Muhlemann, J. K., Kaplan, I., and Muhlemann, K. (2013). Biosynthesis, function and metabolic engineering of plant volatile organic compounds. New Phytol. 198, 16–32. doi: 10.1111/nph.12145
E Birch, A. N, Begg, G. S, and Squire, G. R. (2011). How agro-ecological research helps to address food security issues under new IPM and pesticide reduction policies for global crop production systems. J. Exp. Bot. 62, 3251–3261. doi: 10.1093/jxb/err064
Egger, B., and Koschier, E. H. (2014). Behavioural responses of Frankliniella occidentalis Pergande larvae to methyl jasmonate and cis-jasmone. J. Pest Sci. 87, 53–59. doi: 10.1007/s10340-013-0532-8
Egger, B., Spangl, B., and Koschier, E. H. (2016). Continuous exposure to the deterrents cis-jasmone and methyl jasmonate does not alter the behavioural responses of Frankliniella occidentalis. Entomol. Exp. Appl. 158, 78–86. doi: 10.1111/eea.12381
Engelberth, J., Alborn, H. T., Schmelz, E. A., and Tumlinson, J. H. (2004). Airborne signals prime plants against insect herbivore attack. Proc. Natl. Acad. Sci. U.S.A. 101, 1781–1785. doi: 10.1073/pnas.0308037100
Erb, M., Meldau, S., and Howe, G. A. (2012). Role of phytohormones in insect-specific plant reactions. Trends Plant Sci. 17, 250–259. doi: 10.1016/j.tplants.2012.01.003
Gozzo, F., and Faoro, F. (2013). Systemic acquired resistance (50 years after discovery): moving from the lab to the field. J. Agric. Food Chem. 61, 12473–12491. doi: 10.1021/jf404156x
Guerrieri, E., Lingua, G., Digilio, C. M., Massa, N., and Berta, G. (2004). Do interactions between plant roots and the rhizosphere affect parasitoid behaviour? Ecol. Entomol. 29, 753–756. doi: 10.1111/j.0307-6946.2004.00644.x
Han, B. Y., and Chen, Z. M. (2002). Composition of the volatiles from intact and mechanically pierced tea aphid – tea shoot complexes and their attraction. J. Agric. Food Chem. 50, 2571–2575. doi: 10.1021/jf010681x
Hare, J. D. (2011). Ecological role of volatiles produced by plants in response to damage by herbivorous insects. Annu. Rev. Entomol. 56, 161–180. doi: 10.1146/annurev-ento-120709-144753
Hazen, J. L. (2000). Adjuvants—terminology, classification, and chemistry 1. Weed Technol. 14, 773–784. doi: 10.1614/0890-037X(2000)014[0773:ATCAC]2.0.CO;2
Hegde, M., Oliveira, J. N., da Costa, J. G., Bleicher, E., Santana, A. E. G., Bruce, T. J. A., et al. (2011). Identification of semiochemicals released by cotton, Gossypium hirsutum, upon infestation by the cotton aphid, Aphis gossypii. J. Chem. Ecol. 37, 741–750. doi: 10.1007/s10886-011-9980-x
Hegde, M., Oliveira, J. N., da Costa, J. G., Loza-Reyes, E., Bleicher, E., Santana, A. E. G., et al. (2012). Aphid antixenosis in cotton is activated by the natural plant defence elicitor cis-jasmone. Phytochemistry 78, 81–88. doi: 10.1016/j.phytochem.2012.03.004
Hodge, S., Pope, T. W., Holaschke, M., and Powell, G. (2006). The effect of β-aminobutyric acid on the growth of herbivorous insects feeding on Brassicaceae. Ann. Appl. Biol. 148, 223–229. doi: 10.1111/j.1744-7348.2006.00061.x
Hodge, S., Thompson, G. A., and Powell, G. (2005). Application of DL-beta-aminobutyric acid (BABA) as a root drench to legumes inhibits the growth and reproduction of the pea aphid Acyrthosiphon pisum (Hemiptera: Aphididae). Bull. Entomol. Res. 95, 449–455. doi: 10.1079/BER2005375
Kang, Z. W., Liu, F. H., Zhang, Z. F., Tian, H. G., and Liu, T. X. (2018). Volatile β-ocimene can regulate developmental performance of peach aphid Myzus persicae through activation of defense responses in Chinese cabbage Brassica pekinensis. Front. Plant Sci. 9:708. doi: 10.3389/fpls.2018.00708
King, J. C., and Slavin, J. L. (2013). White potatoes, human health, and dietary guidance. Adv. Nutr. 4, 393S–401S. doi: 10.3945/an.112.003525
Leather, S. R., and Dixon, A. F. G. (1984). Aphid growth and reproductive rates. Entomol. Exp. Appl. 35, 137–140. doi: 10.1111/j.1570-7458.1984.tb03373.x
Llandres, A. L., Almohamad, R., Brévault, T., Renou, A., Téréta, I., Jean, J., et al. (2019). Plant training for induced defense against insect pests: a promising tool for integrated pest management in cotton. Pest Manag. Sci. doi: 10.1002/ps.5039
Markovic, D., Colzi, I., Taiti, C., Ray, S., Scalone, R., Ali, J. G., et al. (2019). Airborne signals synchronize the defenses of neighboring plants in response to touch. J. Exp. Bot. 70, 691–700. doi: 10.1093/jxb/ery375
Matthes, M. C., Bruce, T. J. A., Ton, J., Verrier, P. J., Pickett, J. A., and Napier, J. A. (2010). The transcriptome of cis-jasmone-induced resistance in Arabidopsis thaliana and its role in indirect defence. Planta 232, 1163–1180. doi: 10.1007/s00425-010-1244-4
Maurya, A. K., Pazouki, L., and Frost, C. J. (2019). Plant seeds are primed by herbivore-induced plant volatiles. bioRxiv. doi: 10.1101/522839
Moraes, M. C. B., Birkett, M. A., Gordon-Weeks, R., Smart, L. E., Martin, J. L., Pye, B. J., et al. (2008). cis-Jasmone induces accumulation of defence compounds in wheat, Triticum aestivum. Phytochemistry 69, 9–17. doi: 10.1016/j.phytochem.2007.06.020
Moraes, M. C. B., Laumann, R. A., Pareja, M., Sereno, F. T. P. S., Michereff, M. F. F., Birkett, M. A., et al. (2009). Attraction of the stink bug egg parasitoid Telenomus podisi to defence signals from soybean activated by treatment with cis-jasmone. Entomol. Exp. Appl. 131, 178–188. doi: 10.1111/j.1570-7458.2009.00836.x
Ninkovic, V., Dahlin, I., Vucetic, A., Petrovic-Obradovic, O., Glinwood, R., and Webster, B. (2013). Volatile exchange between undamaged plants - a new mechanism affecting insect orientation in intercropping. PLoS ONE 8:e69431. doi: 10.1371/journal.pone.0069431
Ninkovic, V., Glinwood, R., and Dahlin, I. (2009). Weed-barley interactions affect plant acceptance by aphids in laboratory and field experiments. Entomol. Exp. Appl. 133, 38–45. doi: 10.1111/j.1570-7458.2009.00900.x
NIST (2011). NIST Mass Spectral Database for NIST/EPA/NIH and Mass Spectral Search Program (version 2.0). National Institute of Standards and Technology, 1–65.
Obara, N., Hasegawa, M., and Kodama, O. (2002). Induced volatiles in elicitor-treated and rice blast fungus-inoculated rice leaves. Biosci. Biotechnol. Biochem. 66, 2549–2559. doi: 10.1271/bbb.66.2549
Oluwafemi, S., Dewhirst, S. Y., Veyrat, N., Powers, S., Bruce, T. J. A., Caulfield, J. C., et al. (2013). Priming of production in maize of volatile organic defence compounds by the natural plant activator cis-jasmone. PLoS ONE 8:e62299. doi: 10.1371/journal.pone.0062299
Paprocka, M., Gliszczynska, A., Dancewicz, K., and Gabryś, B. (2018). Novel hydroxy- and epoxy-cis-jasmone and dihydrojasmone derivatives affect the foraging activity of the peach potato aphid Myzus persicae (Sulzer) (Homoptera: Aphididae). Molecules 23:E2362. doi: 10.3390/molecules23092362
Pickett, J. A. (1990). “Gas chromatography-mass spectrometry in insect pheromone identification: three extreme case histories,” in Chromatography and Isolation of Insect Hormones and Pheromones, eds A. R. McCaffery and I. D. Wilson (New York, NY: Plenum Publishing Corporation), 299–309.
Pickett, J. A., Birkett, M. A., Bruce, T. J. A., Chamberlain, K., Gordon-weeks, R., Matthes, M. C., et al. (2007a). cis-jasmone as an allelopathic agent through plant defence induction. J. Allelopath 19, 109–118.
Pickett, J. A., Birkett, M. A., Bruce, T. J. A., Chamberlain, K., Gordon-Weeks, R., Matthes, M. C., et al. (2007b). Developments in aspects of ecological phytochemistry: the role of cis-jasmone in inducible defence systems in plants. Phytochemistry 68, 2937–2945. doi: 10.1016/j.phytochem.2007.09.025
Pickett, J. A., and Khan, Z. R. (2016). Plant volatile-mediated signalling and its application in agriculture : successes and challenges. New Phytol. 212, 856–870. doi: 10.1111/nph.14274
Renou, A., Téréta, I., and Togola, M. (2011). Manual topping decreases bollworm infestations in cotton cultivation in Mali. Crop Prot. 30, 1370–1375. doi: 10.1016/j.cropro.2011.05.020
Sasso, R., Iodice, L., Cristina Digilio, M., Carretta, A., Ariati, L., and Guerrieri, E. (2007). Host-locating response by the aphid parasitoid Aphidius ervi to tomato plant volatiles. J. Plant Interact. 2, 175–183. doi: 10.1080/17429140701591951
Sasso, R., Iodice, L., Woodcock, C. M., Pickett, J. A., and Guerrieri, E. (2009). Electrophysiological and behavioural responses of Aphidius ervi (Hymenoptera: Braconidae) to tomato plant volatiles. Chemoecology 19, 195–201. doi: 10.1007/s00049-009-0023-9
Savary, S., Willocquet, L., Pethybridge, S. J., Esker, P., McRoberts, N., and Nelson, A. (2019). The global burden of pathogens and pests on major food crops. Nat. Ecol. Evol. 3, 430–439. doi: 10.1038/s41559-018-0793-y
Smart, L. E., Martin, J. L., Limpalaër, M., Bruce, T. J. A., and Pickett, J. A. (2013). Responses of herbivore and predatory mites to tomato plants exposed to jasmonic acid seed treatment. J. Chem. Ecol. 39, 1297–1300. doi: 10.1007/s10886-013-0345-5
Sobhy, I. S., Bruce, T. J. A., and Turlings, T. C. J. (2018). Priming of cowpea volatile emissions with defense inducers enhances the plant's attractiveness to parasitoids when attacked by caterpillars. Pest Manag. Sci. 74, 966–977. doi: 10.1002/ps.4796
Sobhy, I. S., Erb, M., Lou, Y., and Turlings, T. C. J. (2014). The prospect of applying chemical elicitors and plant strengtheners to enhance the biological control of crop pests. Philos. Trans. R. Soc. Lond. B Biol. Sci. 369:20120283. doi: 10.1098/rstb.2012.0283
Sobhy, I. S., Erb, M., Sarhan, A. A., El-Husseini, M. M., Mandour, N. S., and Turlings, T. C. J. (2012). Less is more: treatment with BTH and laminarin reduces herbivore-induced volatile emissions in maize but increases parasitoid attraction. J. Chem. Ecol. 38, 348–360. doi: 10.1007/s10886-012-0098-6
Sobhy, I. S., Erb, M., and Turlings, T. C. J. (2015a). Plant strengtheners enhance parasitoid attraction to herbivore-damaged cotton via qualitative and quantitative changes in induced volatiles. Pest Manag. Sci. 71, 686–693. doi: 10.1002/ps.3821
Sobhy, I. S., Mandour, N. S., and Sarhan, A. A. (2015b). Tomato treatment with chemical inducers reduces the performance of Spodoptera littoralis (Lepidoptera: Noctuidae). Appl. Entomol. Zool. 50, 175–182. doi: 10.1007/s13355-014-0319-2
Sobhy, I. S., Woodcock, C. M., Powers, S. J., Caulfield, J. C., Pickett, J. A., and Birkett, M. A. (2017). cis-Jasmone elicits aphid-induced stress signalling in potatoes. J. Chem. Ecol. 43, 39–42. doi: 10.1007/s10886-016-0805-9
Srinivasan, R., and Alvarez, J. M. (2011). Specialized host utilization of Macrosiphum euphorbiae on a nonnative weed host, Solanum sarrachoides, and competition with Myzus persicae. Environ. Entomol. 40, 350–356. doi: 10.1603/EN10183
Staudt, M., Jackson, B., El-Aouni, H., Buatois, B., Lacroze, J. P., Poëssel, J. L., et al. (2010). Volatile organic compound emissions induced by the aphid Myzus persicae differ among resistant and susceptible peach cultivars and a wild relative. Tree Physiol. 30, 1320–1334. doi: 10.1093/treephys/tpq072
Turlings, T. C. J., and Erb, M. (2018). Tritrophic interactions mediated by herbivore-induced plant volatiles: mechanisms, ecological relevance, and application potential. Annu. Rev. Entomol. 63, 433–452. doi: 10.1146/annurev-ento-020117-043507
Uefune, M., Ozawa, R., and Takabayashi, J. (2013). Prohydrojasmon treatment of lima bean plants reduces the performance of two-spotted spider mites and induces volatiles. J. Plant Interact. 9, 69–73. doi: 10.1080/17429145.2012.763146
van Emden, H. F., and Harrington, R., (2017). Aphids as Crop Pests, 2nd Edn. (Oxfordshire: CAB International). doi: 10.1079/9781780647098.0000
von Mérey, G. E., Veyrat, N., Alessandro, M. D., and Turlings, T. C. J. (2013). Herbivore-induced maize leaf volatiles affect attraction and feeding behavior of Spodoptera littoralis caterpillars. Front. Plant Sci. 4:209. doi: 10.3389/fpls.2013.00209
Walters, D. R., Ratsep, J., and Havis, N. D. (2013). Controlling crop diseases using induced resistance : challenges for the future. J. Exp. Bot. 64, 1263–1280. doi: 10.1093/jxb/ert026
Webster, B., Bruce, T., Dufour, S., Birkemeyer, C., Birkett, M., Hardie, J., et al. (2008). Identification of volatile compounds used in host location by the black bean aphid, Aphis fabae. J. Chem. Ecol. 34, 1153–1161. doi: 10.1007/s10886-008-9510-7
Webster, B., Gezan, S., Bruce, T., Hardie, J., and Pickett, J. (2010). Between plant and diurnal variation in quantities and ratios of volatile compounds emitted by Vicia faba plants. Phytochemistry 71, 81–89. doi: 10.1016/j.phytochem.2009.09.029
Wood, B. W., Tedders, W. L., and Taylor, J. (1997). Control of pecan aphids with an organosilicone surfactant. HortScience 32, 1074–1076. doi: 10.21273/HORTSCI.32.6.1074
Wu, J., and Baldwin, I. T. (2010). New insights into plant responses to the attack from insect herbivores. Annu. Rev. Genet. 44, 1–24. doi: 10.1146/annurev-genet-102209-163500
Wyatt, I. J., and White, P. F. (1977). Simple estimation of intrinsic rates from aphids and tertanyxhid mites. J. Appl. Ecol. 14, 757–766. doi: 10.2307/2402807
Yoneya, K., Kugimiya, S., and Takabayashi, J. (2014). Leaf beetle larvae, Plagiodera versicolora (Coleoptera: Chrysomelidae), show decreased performance on uninfested host plants exposed to airborne factors from plants infested by conspecific larvae. Appl. Entomol. Zool. 49, 249–253. doi: 10.1007/s13355-013-0243-x
Yu, H., Zhang, Y., Li, Y., Lu, Z., and Li, X. (2018). Herbivore- and MeJA-induced volatile emissions from the redroot pigweed Amaranthus retroflexus Linnaeus: their roles in attracting Microplitis mediator (Haliday) parasitoids. Arthropod Plant Interact. 12, 575–589. doi: 10.1007/s11829-018-9606-0
Keywords: defense priming, cis-jasmone, VOCs, aphids, growth, survival, intrinsic rate
Citation: Sobhy IS, Caulfield JC, Pickett JA and Birkett MA (2020) Sensing the Danger Signals: cis-Jasmone Reduces Aphid Performance on Potato and Modulates the Magnitude of Released Volatiles. Front. Ecol. Evol. 7:499. doi: 10.3389/fevo.2019.00499
Received: 01 September 2019; Accepted: 05 December 2019;
Published: 08 January 2020.
Edited by:
Maria L. Pappas, Democritus University of Thrace, GreeceReviewed by:
Alexander Weinhold, German Center for Integrative Biodiversity Research, GermanyPaula Baptista, Polytechnic Institute of Bragança, Portugal
Copyright © 2020 Sobhy, Caulfield, Pickett and Birkett. This is an open-access article distributed under the terms of the Creative Commons Attribution License (CC BY). The use, distribution or reproduction in other forums is permitted, provided the original author(s) and the copyright owner(s) are credited and that the original publication in this journal is cited, in accordance with accepted academic practice. No use, distribution or reproduction is permitted which does not comply with these terms.
*Correspondence: Islam S. Sobhy, aS5zb2JoeUBrZWVsZS5hYy51aw==; Michael A. Birkett, bWlrZS5iaXJrZXR0QHJvdGhhbXN0ZWQuYWMudWs=
†Present address: Islam S. Sobhy, School of Life Sciences, Huxley Building, Keele University, Keele, United Kingdom
John A. Pickett, School of Chemistry, Cardiff University, Cardiff, United Kingdom
‡ORCID: Islam S. Sobhy orcid.org/0000-0003-4984-1823