- 1Department of Biological Sciences Graduate Program in Ecology, Evolution, Ecosystems, and Society, Dartmouth College, Hanover, NH, United States
- 2Environmental Studies Program, Dartmouth College, Hanover, NH, United States
Partial migration is a common movement phenomenon in ungulates, wherein part of the population remains resident while another portion of the population transitions to spatially or ecologically distinct seasonal ranges. Although widely documented, the causes of variation in movement strategies and their potential demographic consequences are not well-understood. Here, we used GPS telemetry data and individual-based photographic surveys to describe evidence for the partial migration of giraffe (Giraffa camelopardalis) in the tropical savanna habitat of Murchison Falls National Park, Uganda. Seasonal movements in giraffe have been described but have not been systematically investigated within the framework of partial migration. We characterized movement behaviors of eight female GPS tracked giraffe across one full year using a model-driven approach of net-squared displacement metrics. To further evaluate these space use patterns at the population-level, we used closed robust design multi-state capture recapture models derived from individually based photographic surveys collected seasonally over three years. We also characterized environmental conditions associated with seasonal space use by conducting ground-based vegetation surveys and analyzing remotely sensed phenology data. Our results from both individually based telemetry models and population-level multi-state models suggest intra-population variation in seasonal space use strategies with three dominant movement classes: (1) Residents in deciduous savanna characterized by Acacia sieberiana, Acacia senegal, Harrisonia abyssinica, and Crateva adansonii in the far western end of the park. (2) Residents in the broadleaf savannas characterized by Pseudocedrela kotschyi, Stereospermum kunthianum, Termalia spp., and Combretum spp. in the central sector of the park (3) Male-biased migrants that transitioned seasonally between the acacia savanna in the wet seasons and the broadleaf savanna in the dry seasons. Our results offer insights into how giraffe navigate spatiotemporally dynamic environments at both individual and population levels, providing ecological mechanisms for the emergent population dynamics of these large-bodied topical browsers.
Introduction
Understanding the ecological interactions that influence an organism's movement decisions and the subsequent fitness consequences of movement remains a major theme in ecology. Movement allows organisms to utilize resources that are distributed heterogeneously over space and time (Dingle and Drake, 2007). Landscape level movement patterns vary considerably across species, within species across geographic regions, and even among individuals within a single population (Mueller et al., 2011; Naidoo et al., 2012b; Singh et al., 2012). As such, studying the causes and consequences of variation in movement strategies can cast light on how organisms' life history characteristics influence space use and population dynamics in spatiotemporally varying environments.
Migration is a common movement strategy wherein organisms consistently move to spatially distinct stable ranges to track resource distribution or avoid predation risk in temporally and spatially varying environments (Fryxell and Sinclair, 1988; Dingle and Drake, 2007). Increasingly, however, studies suggest that many populations are only partially migratory (Chapman et al., 2011). In partial migration, some members of the population are resident while others exhibit migratory behaviors (Dingle and Drake, 2007; Chapman et al., 2011). Identified in a diverse suite of taxa (Chapman et al., 2011; Ohms et al., 2019) and observed on a wide range of spatial scales, from several kilometers (Mysterud, 1999; Gaidet and Lecomte, 2013) to hemispheres (Shaffer et al., 2006), partial migration, because of the inherent variation of movement strategies with a population, provides a useful process to evaluate the causes of intraspecific variation in movement behaviors and the fitness consequences of different space-use strategies (Chapman et al., 2011). Although these variations in movement may have a genetic basis in some systems (Berthold and Helbig, 1992; Bensch et al., 2011; Hess et al., 2016) researchers are increasingly identifying scenarios in which these alternative movement strategies are conditional on the state of an individual and may be plastic over the life of an individual (Sutherland, 1998; Found and St. Clair, 2017). Studies examining conditional migration have suggested that these movement behaviors may be contingent upon asymmetries in sex or social dominance, or the ability of individuals to assess resource conditions and respond to social cues (Chapman et al., 2011).
Despite a growing body of research on the ecological mechanisms for the emergence and maintenance of individual variation in movement strategies, there is a lack of studies evaluating this variation at the population level (Ohms et al., 2019). Many studies examining varying movement strategies use tracking devices (GPS, VHF, PIT tags) to monitor the movements of focal individuals and extrapolate these processes to the population level (Struve et al., 2010; Mysterud et al., 2011; Cagnacci et al., 2015). Although useful in characterizing movement behaviors, quantifying seasonal ranges, and identifying the timing of seasonal movements with precision, many of these telemetry/tracking studies are limited in their inference by smaller sample size and relatively short study durations (Hebblewhite and Haydon, 2010). Additionally, few studies account for variation in movement strategies across different age and sex classes because of logistical constraints associated with collaring multiple individuals across these different categories. Because of these limitations, studies connecting varying movement behaviors among different age/sex classes to population level processes over longer time periods are rare and as result, researchers lack the ability to evaluate causes and consequences of movement across multiple scales (Torney et al., 2018). This shortage of empirical inquiry limits the understanding of the associations among varying movement strategies, population dynamics and landscape-level processes and can potentially result in misinformed conservation strategies that do not properly account for the demographic effects of movement processes over larger timescales (Bolger et al., 2008).
In this study we employ multiple complementary approaches to evaluate partial migration at both the individual and population levels across multiple seasons over three years for a large-bodied tropical browser, the giraffe (Giraffa camelopardalis). The unique foraging behaviors and life history characteristics of the giraffe make it a suitable study species for examining variation of movement strategies. Once widely distributed across much of sub-Saharan Africa, giraffe have recently undergone substantial population declines and range restrictions (Muller et al., 2016). Despite this continent scale population decline, the current giraffe distribution encompasses a wide range of habitats and climates, from the hyper-arid Hoanib desert of Namibia to more mesic savannas in Uganda, Tanzania, and Democratic Republic of Congo (van der Jeugd and Prins, 2000; Fennessy, 2009; Flanagan et al., 2016). Giraffe exhibit a wide range of space-use behaviors across these habitat types with larger home ranges reported in more arid environments and smaller home ranges in more mesic savannas (van der Jeugd and Prins, 2000; Fennessy, 2009; Flanagan et al., 2016; Knüsel et al., 2019). Giraffe are large-bodied tropical browsers and forage almost exclusively upon leaves, flowers, and seeds of woody vegetation (Pellew, 1984a). The quality and quantity of forage resources varies considerably in the seasonal tropical savannas that are characteristic of much of their range and as a result, giraffe have been shown to exhibit seasonal variation in diet composition and habitat selection (Field and Ross, 1976; Pellew, 1984a; Bercovitch and Berry, 2018). Unlike many other ungulates, giraffe are aseasonal, asynchronous breeders and consequently do not have defined breeding or birthing seasons that are often characteristic of partially migratory ungulates in temperate systems (Leuthold and Leuthold, 1975), although some studies do report minor increases in calf abundance during dry seasons (Sinclair et al., 2000). Additionally, giraffe are capable of simultaneous gestation and lactation throughout all seasons (Deacon et al., 2015). Giraffe social structure is generally characterized as a fission-fusion system in which loosely associated herds often change membership, with associations potentially influenced by kinship and individual preferences (Carter et al., 2013; Dagg, 2014). As a result of female reproductive asynchrony and fluid social associations, males are thought to adopt a roaming reproductive strategy in which they search for sexually receptive females (Bercovitch et al., 2006). Prior research also suggests sexual variation in resource selection (Pellew, 1984b; Young and Isbell, 1991; Ginnet and Demment, 1997), providing potential mechanisms for sexual variation in space use. Although there is empirical support for seasonal long-distance movements in giraffe (Le Pendu and Ciofolo, 1999) other studies describe giraffe populations as non-migratory (Pellew, 1984a). Despite these foundational studies and the unique opportunities presented by giraffe's natural history, a systematic investigation of intra-population variation of giraffe movement behaviors has not been conducted.
In this study, we analyzed a full year of GPS telemetry data with net squared displacement models to characterize variations in giraffe movement patterns and identify the temporal and spatial extent of landscape-level movements of eight focal female giraffe. We also used over three years of seasonal population surveys and closed robust design multistate mark recapture models to evaluate population level movement behavior at the seasonal scale. Using these models, we tested hypotheses that giraffe adopt space-use strategies to in response to seasonal variation in resource distribution, and that sexual variation in resource use or reproductive tactics influence space use strategies. We evaluated observed movement strategies with regard to measured variation in the woody vegetation community composition to explore the causes and consequences of partial migration of a large-bodied browser in a tropical environment.
Methods
Study Site
We conducted this study in Murchison Falls National Park (MFNP), Uganda. MFNP is located in northwestern Uganda (02°15′ N, 31°48′E), and encompasses an area of 3,840 km2, making it Uganda's largest national park. The park is bisected by the Victoria Nile River, with the southern portion dominated by dense forest and the northern portion characterized by savanna, Borassus palm woodland, and riverine woodland. The average annual rainfall for MFNP ranges from ~1,100–1,500 mm and is bimodally distributed with the short rains occurring from mid-March to June and August to December, and with the long dry season occurring from late-December to mid-March (Fuda et al., 2016). The current natural distribution of giraffe is limited to the northern portion of the park. Northern MFNP is divided into a series of management sectors, which roughly correspond with drainages and habitat type. We restricted our study to the western half of this area, comprised largely of the Delta and Wankwar sectors since small rivers in the central area of the park limit the potential for giraffe movement across this east/west gradient and our own mark-recapture data suggest that the majority of giraffe occur in these two sectors with little interchange with giraffe further east (Figure 1).
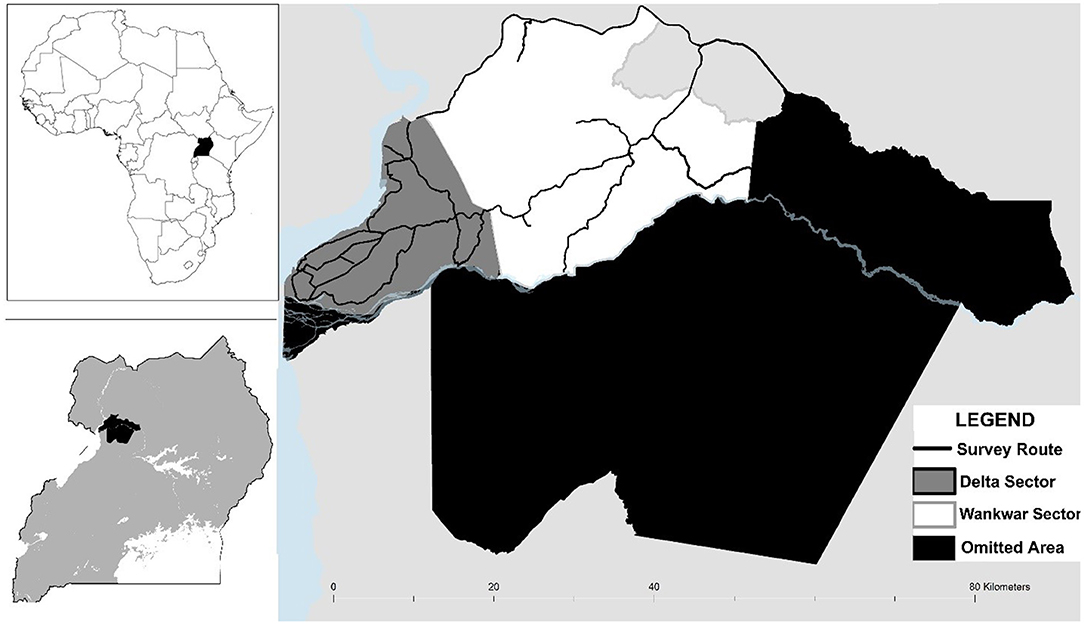
Figure 1. A map of the study site in Murchison Falls National Park, Uganda. Photographic surveys were conducted on the track network in Delta and Wankwar sectors of the northwestern portion of the park.
MFNP currently supports the largest population of giraffe in Uganda with recent surveys estimating a population size of 1,318 adults/subadults (Brown et al., 2019). Over the past 60 years, periods of civil unrest led to large scale defaunation of the park, including a substantial reduction in the giraffe population. However, following the cessation of conflict in Uganda in the mid-1990's, the giraffe population has increased rapidly such that it is now larger than at any point in recorded history. Recent population estimates derived from mark-recapture methods suggest annual population growth rate from 2014 to 2017 of λ = 1.14 (Brown et al., 2019).
Characterizing Spatiotemporal Dynamics of Vegetation
Because spatiotemporal variation of resources is a requisite condition for the emergence of migratory behavior, we first characterized potential bottom-up effects by quantifying the composition of vegetation communities in the study site with a series of woody vegetation surveys using a modified plotless, k-tree sampling method (Kleinn and Vilčko, 2006; Magnussen et al., 2012) across the entire extent of northern MFNP. During concurrent giraffe surveys (see methods below), we conducted vegetation surveys at the location of each giraffe herd, such that vegetation surveys represent plant community composition associated with giraffe positions in the heterogeneous savanna. In each survey, we measured the distance to the nearest ~15 tree (>1 m height) using a laser rangefinder and identified every tree within that radius to species. We then calculated the density of each tree species by dividing the number of trees counted by the area of a circle with radius equal to the distance to the fifteenth tree. We conducted 259 woody vegetation surveys in the Delta and 139 surveys in the Wankwar sector. To validate plant identification, we collected and pressed voucher specimens of each unique species which were then independently identified by botanists at the Makerere University herbarium. To characterize woody vegetation composition in each sector within the park, we combined all the surveys and calculated the proportional species composition in each sector. We compared raw counts of surveyed woody vegetation across the sectors using a Pearson's chi-squared test to evaluate the prediction that the different sectors were comprised of different communities of woody vegetation.
To quantify temporal variation in primary productivity, we used MOD13Q1 MODIS 16-day (250-m) Enhanced Vegetation Index (EVI) data for the period July 2014 to May 2019. EVI is remotely sensed vegetation index that measures greenness and has been shown to be effective for monitoring primary productivity in African savannas (Sjöström et al., 2011) and has been used as an indicator of vegetation quantity and quality for herbivore spatial ecology studies (Naidoo et al., 2012a; Villamuelas et al., 2016). We accessed these data through the NASA Application for Extracting and Exploring Analysis Ready Samples (AppEEARS) platform, extracting all available values for EVI at each of our earlier woody vegetation sampling points. Although the spatial resolution of this product renders it inappropriate for isolating the phenology of woody vegetation—surrounding grassy vegetation likely contributes to the spectral signature at this grain- the overall time series is a useful indicator of the timing of seasonal transitions and potential phenological responses of deciduous woody vegetation to rainfall. We plotted the timeseries of EVI data to evaluate seasonality of productivity across both sectors. If EVI is an effective measure of plant phenology, we expected pronounced declines in EVI values during the dry seasons and subsequent increases in EVI during the wet seasons.
Characterization of Giraffe Movement Behaviors
GPS Telemetry
To track the location of individual giraffe over time, we deployed solar-charged, ossicone-mounted GPS units (Savannah Tracking) on 20 giraffes in MFNP during April 2018 (and an additional GPS unit in August 2018). We deployed the units at the beginning of the wet season, such that all giraffe were expected to be in their wet season ranges when tracking commenced. Giraffe were immobilized from a Landcruiser using a mixture of etorphine and azaperone and tracking units were attached under the supervision of a local government wildlife veterinarian. We selected both male (n = 5) and female (n = 15) focal individuals across both the Delta (n = 12) and Wankwar (n = 8) sectors to monitor movement strategies across sexes and habitat type. Before immobilizing giraffe, we photographed the right side of the candidate focal individuals and compared their spot patterns to a database of previously observed giraffe encounters (see survey methods below; Supplementary Table 1). We then selected individuals to ensure relatively even representation among three possible prior space-use patterns: (1) Individuals that were previously observed only in the Delta (n = 6); (2) Individuals that were previously observed only in Wankwar (n = 6); and (3) Individuals that were previously observed in both the Delta and in Wankwar (n = 8). We programmed all GPS tracking units to record coordinate fixes at hourly intervals and transmit location data to an off-site server twice daily via satellite link. We excluded data from units that lost function before the seasonal transition to the long dry season, resulting in eight functional units deployed on females for subsequent analyses (Table 1).
Analyses: Net Squared Displacement Models
To classify movement behaviors of individual giraffe, we employed a model driven approach based on the net squared displacement calculated from each giraffe's movement trajectory (Bunnefeld et al., 2011). Net squared displacement (NSD) is the squared value of the Euclidean distance between the starting location of a trajectory and every subsequent coordinate fix (Turchin, 1998). To categorize the movement behavior of each giraffe, we fit individual NSD time series data with a set of a-priori non-linear models, each representing the theoretical NSD signature of different movement strategies (residence, migration, mixed migration, dispersal, and nomadism) (Bunnefeld et al., 2011; Singh and Leonardsson, 2014; Spitz et al., 2017). In addition to categorizing movement behaviors, these models have ecologically interpretable parameters, allowing for direct estimates of migration departure date, the rate of movement between seasonal ranges, the distance between seasonal ranges, and the duration of residence on the seasonal ranges. We used Akaike information criterion (AIC) to identify the best fitting model (Burnham and Anderson, 2002; Bunnefeld et al., 2011). Before model fitting, we designed several a priori decision rules to limit the possibility of movement strategy misclassification. To reduce the potential for small scale intra-seasonal movements being misclassified as migration, we only considered migration, mixed migration, and dispersal models in which the estimated parameter value for the squared migration/dispersal distance exceeded 12.25 km. This spatial threshold for migratory behavior was set to exclude most previously reported values for total daily movements for giraffe, such that movements on the scale of reported daily displacement would not be misidentified as migration (McQualter et al., 2015). Additionally, we restricted migrant/partial migrant models to those in which had a minimum time of occupancy in the seasonal range of 21 days, effectively restricting intra-seasonal exploratory behavior being categorized as migration (Spitz et al., 2017). In scenarios where a priori decision criteria disqualified the top model, we used the next supported model according to AIC. We conducted NSD model fitting and model selection with the MigrateR package (Spitz et al., 2017) in R (R Core Team, 2019).
Population-Level Movement
To examine population level patterns of seasonal transitions across all age and sex classes and to evaluate potential differences in survival across geographic sectors over time, we conducted photographic surveys of the entire population and analyzed encounter data with multi-state mark recapture methods.
Individual-Based Photographic Surveys
We conducted photographic surveys of the study area at 4-month intervals between December 2014 and December 2017. We scheduled these surveys to correspond with periods of seasonal transitions (December: end of the long rains, March/April: end of the dry season, and July/August: end of the short rains). In accordance with a robust survey design (Pollock, 1982; Kendall et al., 1995; Pollock et al., 2002), each primary sampling event consisted of two secondary sampling events during which we drove a series of fixed routes comprising the road network over the entire study area. Secondary sampling occasions were separated by a time of <1 week, during which we assumed that the system was closed (no births, deaths, immigration, emigration, or substantial movement). Along these routes, we photographed the right side of each individual and identified every individual giraffe using its unique, unchanging coat pattern in association with WILD-ID, a pattern recognition software program (Foster, 1966; Bolger et al., 2012). We also recorded the spatial coordinates of each observation, the age class and sex of each giraffe, and any visible signs of disease or injury. We estimated the age class of each giraffe (calf: 0–12 months; subadult female: 1–3 years; subadult male: 1–6 years; adult female: >3 years; adult male >6 years) using physical characteristics in association with estimated axial and appendicular body proportions (Strauss et al., 2015). During photographic surveys, we also conducted opportunistic observations of foraging to quantify giraffe diet composition across the different habitat types. While photographing each individual giraffe, if it was foraging, we identified the species of woody vegetation being consumed.
We completed 10 primary events, each comprised of two secondary events, resulting in 20 surveys over 3 years (consisting of 80 total days of field surveys). After filtering the data to exclude individuals observed outside of our defined study area, and individuals with insufficient location data, our photographic database consisted of records for 1,453 unique giraffe over 9,374 individual encounter records.
Analysis: Closed Robust Design Multi-State Capture Recapture Models
From the seasonal robust surveys, we developed encounter histories for every individual giraffe. We assigned a geographic state (Delta or Wankwar sector) for each encounter based on the location of the observation. We then used a closed robust design multi-state (CRDMS) modeling framework to estimate associated parameters: capture probability (p), survival (S), transition probabilities (Ψ) between sectors, and a derived parameter of population size (N) (Lebreton et al., 2009; Chabanne et al., 2017). CRDMS models assume that at there are no sector transitions within each primary sampling event, an assumption that our raw data only infrequently violated (<1% of encounters) (Arnason, 1972, 1973). To correct for this, if we observed an individual in different sector within the same primary sampling event, we assigned both encounters to the sector where it was first encountered during the primary period.
We used multi-model comparisons to test a series of hypotheses relating movement and variation of demographic parameters to sex, location and time of year. To test for the effect of temporal variation of resources on demographic parameters, we developed four different schemes for temporal parameterization of models: constant values, variation by primary session, variation by a three season classification (post short rain, post long rain, and post dry season) and variation by a two season classification (post short rain/post long rain, and post dry season). If there was no temporal variation in movement, survival, or capture probability, we expected best supported models to have constant parameter values across all primary sampling events. If there was a consistent seasonal signature in demographic parameters in which giraffe responded differently during the three seasons, then we expected the three season model to be best supported. Conversely, if there was seasonal variation in demographic parameters but no difference in giraffe response between the short rainy season and the long rainy season, we expected best support for models with the two-season classification. Lastly if demographic parameters varied over time but did not consistently vary in magnitude or direction with our a priori seasonal classification schemes across multiple years, we expected best supported models to have demographic parameters vary across primary sampling events. Similarly, we incorporated sex of the individual as a classification factor to test for sex biased responses of demographic parameters. If sex affected movement or survival, we expected models with sex as a classification to be better supported by the data than models that do not incorporate variation due to differences in sex. Lastly, to test for differences in survival across the sectors, we incorporated location as a potential covariate for the estimate of survival.
We then developed a suite of candidate models in which we allowed most model parameters to vary by state (sector location), sex, and primary session/2-season/3-season. We constrained the capture probability and within session secondary resight probability to better estimate capture probability across primary sampling events. Because we had similar secondary resight rates across all primary sampling events, we also constrained the capture probability so that it remained constant over primary periods. We then ran all possible combinations of session, season, sex, and sector varying parameter estimates and ranked the output models using AIC to identify the model that was best supported by the data (Burnham and Anderson, 2002). We performed these analyses in MARK (White and Burnham, 1999) called from R (R Core Team, 2019) with the RMARK package (Laake, 2013).
Analysis: Assessing Spatial and Sexual Variation of Diet Composition
To test for differences in diet composition across both sex and geographic sectors (Delta/Wankwar), we partitioned opportunistic foraging observations by sex and sector. We then compared the relative proportions of woody plant species in each diet using a series of pairwise Pearson's chi-squared tests.
Results
Spatiotemporal Variation in Resource Distribution
We found a significant difference in the species composition of woody vegetation across the two sectors of the park (χ2 =4583.8, p ≤ 0.01, df = 10). The available woody vegetation in the Delta sector was comprised largely of the deciduous/semi-deciduous acacia species (primarily of A. senegal, A. sieberiana, and A. drepanolobium), the semi-deciduous leafy Crateva adansonii, and the evergreen shrub Harrisonia abyssinica whereas the central Wankwar sector was comprised predominantly of the broad-leaf semi-deciduous Pseudocedrela kotschyi, Stereospermum kunthianum, Piliostigma thonningii, and Terminalia spp. (Figure 2).
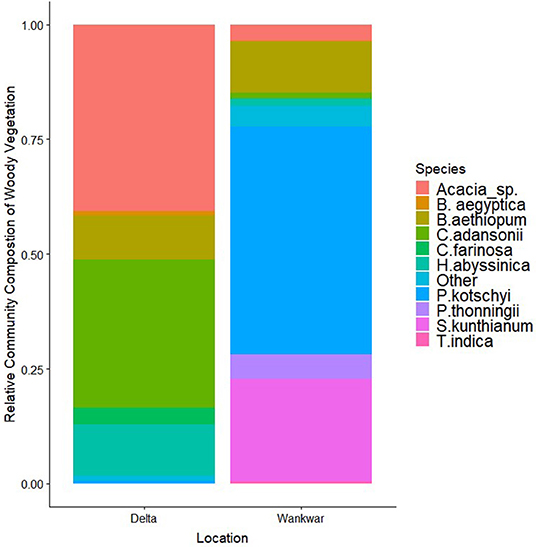
Figure 2. The relative composition of woody vegetation in the two major habitat zones in western Murchison Falls National Park. Bars represent the proportion of each species of the total woody plants (>1 m in height) surveyed in each region. The western delta region of the park is characterized by a deciduous savannah consisting largely of Acacia sp., H. abyssinica and Creteva adansonii, whereas the central Wankwar region of the park was predominantly broadleaf savannah characterized by Psuedocedrela kotschyi, Stereospermum kunthianum, P.thonningi, and Combretum sp.
We found strong seasonal signals in EVI measurements that corresponded with our a priori understanding of seasonal rainfall patterns. During the dry season, which typically commenced mid/late December, EVI values rapidly dropped until the onsets of the rainy season in March/April, after which there was rapid green-up of vegetation. These productivity trends are consistent in timing and magnitude across years and habitat types, demonstrating the strong effects of seasonality on vegetation dynamics in this savanna system (Appendix 2).
Individual-Level Telemetry and NSD Models
The NSD models classified three major space use categories among the eight individually tracked female giraffe: (1) year-round Delta residents (n = 2); (2) year-round Wankwar residents (n = 2); and (3) Individuals that migrated from the Delta to Wankwar seasonally (n = 4) (Table 1).
For seasonal migrants, the transitional period typically occurred rapidly at the onset of the dry season in mid-December/early January (Figure 3). We found individuals' seasonal migration distances varied from 13 to 30.4 km (Table 1), although all migrants moved from the a priori defined boundaries of the Delta to Wankwar during the dry season. Notably, NSD models classify movements independent of underlying environmental covariates, so this finding is independent of a priori definitions of sectors. Individuals categorized as migrants returned to the wet season range in mid/late April. These movement behaviors were mostly consistent with our classification of movement behaviors from previous survey encounters of the tracked individuals (Table 1).
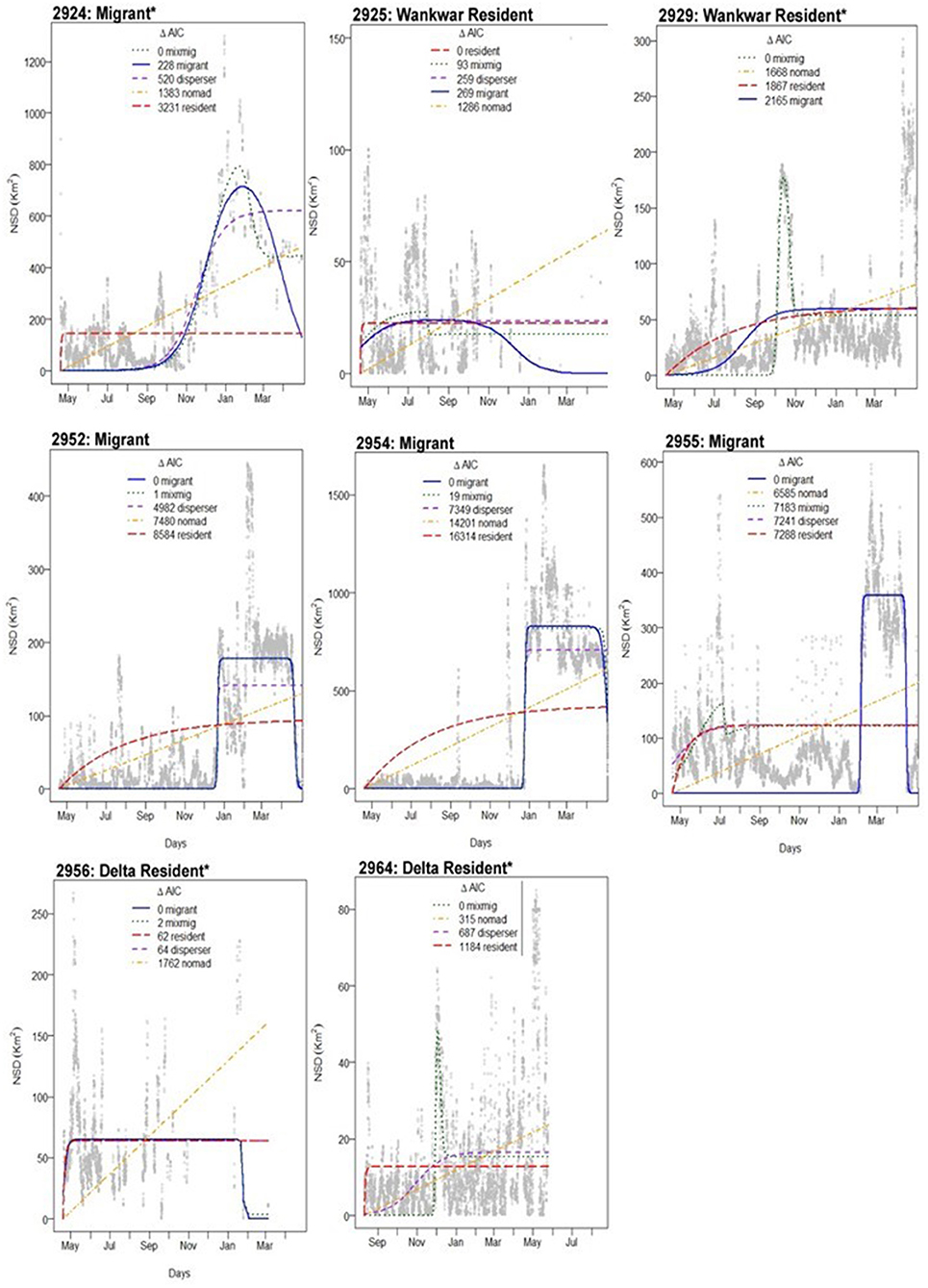
Figure 3. Net squared displacement (NSD) timeseries patterns for individual giraffe over one seasonal transition from wet seasons to dry season. For individuals categorized as migrants, the shift in range coincided with the end of the wet season in late December. Note: Axis scales are different for each timeseries. Individuals in which movement classes are determined by post-hoc decisions criteria are denoted with an asterisk (*).
Seasonal migration was characterized by rapid, directed movement between Wankwar and the Delta. Conversely, both Wankwar residents and the Delta resident exhibited relatively tortuous movement trajectories within their respective sectors throughout the study period (Figure 4).
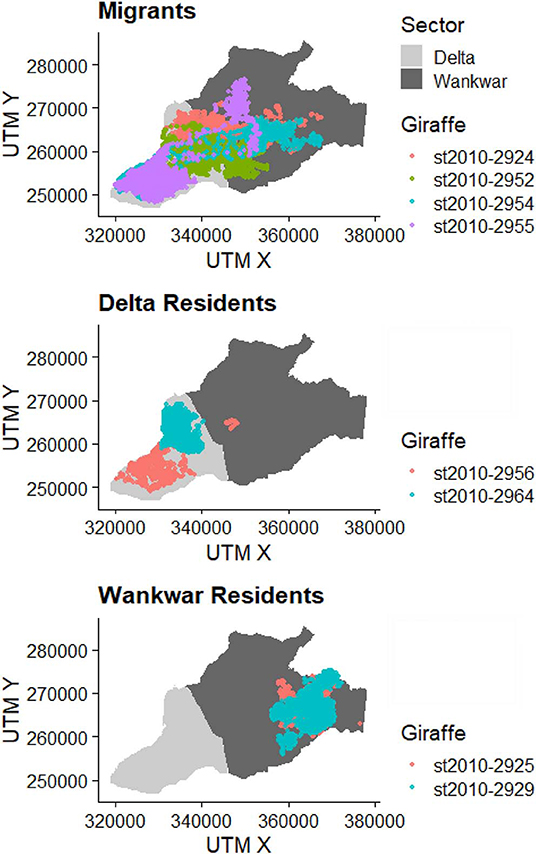
Figure 4. Examples of the movement trajectories of three individual giraffe concurrently exhibiting different seasonal space-use patterns across geographical and ecological space.
Population-Level Surveys and CRDMS Models
Our best fitting CRDMS model based on AIC score was a model where survival (S) varied by sex and time, capture probability (p) varied by sex and sector, and transition probability (Ψ) varied by sector, sex, and the three season temporal categorization scheme (Table 2). Notably, the models with sex varying transition probabilities (Ψ) outperformed models in which sex was not a factor. Males consistently exhibited the highest transition probabilities during both dry and wet season transitions. Capture probability (p) for both sexes varied across sectors with p being higher in the Delta sector (male: 0.485, SE 0.005; female: 0.496, SE 0.006) than in Wankwar sector (male: 0.267, SE 0.006; female: 0.275, SE 0.005). The best fitting model's estimates for transition probability (Ψ) indicated a strong seasonal variation in the direction of transitions. These parameters effectively represented the probability of migration across these distinct sectors. For both males and females, the transition probability from the Delta to Wankwar (Ψ D → W) was consistently highest between December and March (seasonal shift to the dry season) (Figure 5). Transition probabilities from Wankwar to the Delta (Ψ W → D) for both males and females during this same period were consistently low. Similarly, during the seasonal shift from the dry season to the wet season (March to July) and between the wet seasons (July to December), seasonal sector transition probabilities from Wankwar to the Delta (Ψ W → D) were highest.
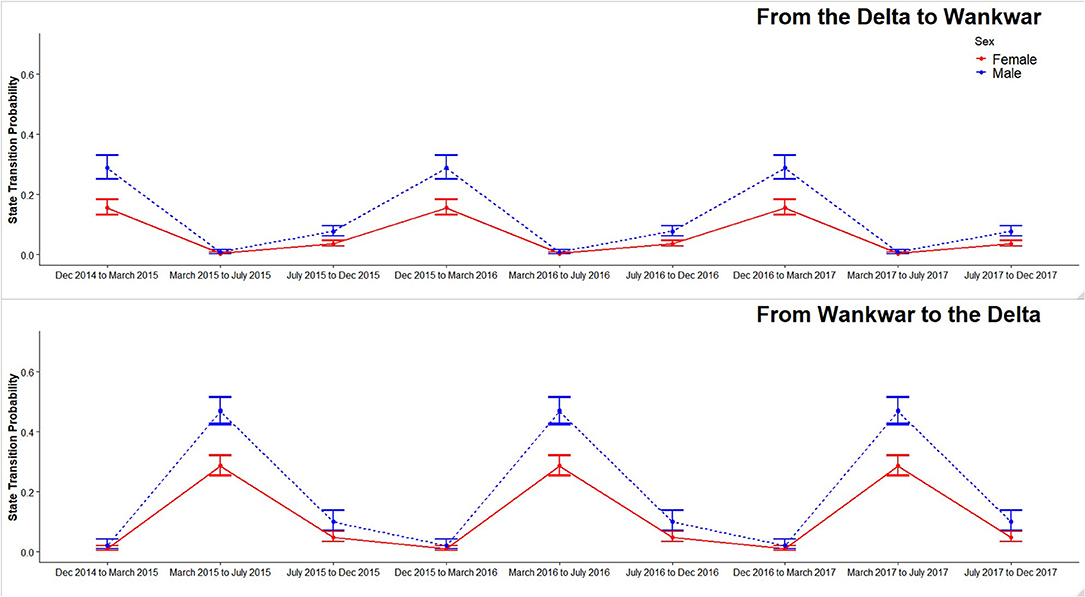
Figure 5. Seasonal estimates of transition probabilities between Wankwar and the Delta for both male and female giraffe. Season and sex varying transition probabilities are drawn from the best supported multi-state mark recapture model with error bars representing 95% CI.
The best supported model yielded apparent survival parameter estimates (S) that varied over sex and primary sampling event. For both male and female, these apparent seasonal survival estimates were consistently high with no apparent seasonal pattern in survival estimates for these adult/subadult giraffe (Figure 6).
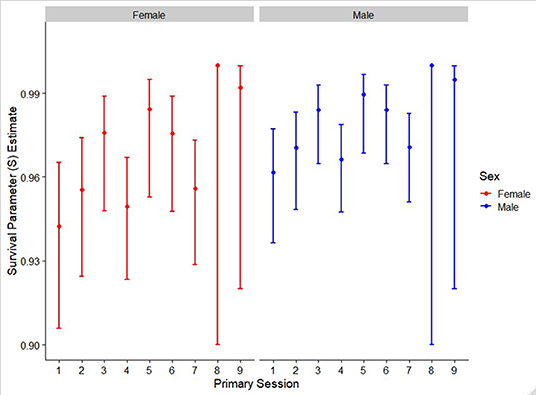
Figure 6. Apparent seasonal survival estimates across sex and time. Parameter estimates are generated from the best support mult-state mark recapture model with error bars representing 95% CI.
Spatial and Sexual Variation of Diet Composition
There were significant differences in diet composition across geographic sector and sex (Figure 7). Females in the Delta had a different diet profile than females in Wankwar (χ2 = 176.82, p ≤ 0.01, df = 13) with the latter group characterized predominantly by broadleaf S. kunthianum, and P. kotschyi and the former group characterized by Acacia sp., C. adansonii, and H. abyssinica. Males exhibited a similar significant difference in diet composition across the two sectors (χ2 = 291.41, p ≤ 0.01, df = 13). We also found a significant difference in diet composition between females and males in the Delta (χ2 = 65.35, p ≤ 0.01, df = 10) with females being observed consuming relatively more Acacia sp. and males consuming proportionally more C. adansonii. We found no difference in diet composition between males and females in Wankwar (χ2 =5.97, p ≤ 0.54, df = 7).
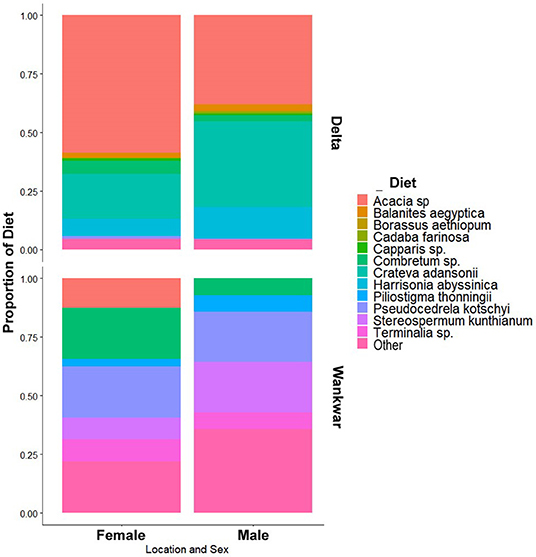
Figure 7. The diet composition across sex classes and geographic location. We found significant differences in all pairwise comparisons except for male and female diets in Wankwar sector.
Discussion
Our study suggests that in the spatiotemporally dynamic savannas of western MFNP, giraffe exhibit intra-population variation in space-use strategies, with some individuals transitioning between wet season ranges dominated by deciduous Acacia sp., Harrisonia abyssinca, and C. adansonii savannas in the Delta, to spatially distinct dry season ranges dominated by semi-deciduous broadleaf P. kotschyi, and S. kunthianum in the Wankwar sector. Given the complementary evidence suggesting variation in seasonal movements in which a portion of the population inhabits geographically and ecologically distinct seasonal ranges, we propose that this population exhibits partial migration. In migratory individuals, NSD models indicate a rapid departure from the wet season ranges at the end of December, characterized by directed movement to the dry season range and a subsequent synchronous return to the wet season range in mid-April at the onset of the short rains. These tracked giraffe exhibited range fidelity within the seasons and typically only exhibited long distance transitions across sectors between seasons. These seasonal transitions were also detected by population-level CRDMS models derived from photographic surveys, which demonstrated consistently higher transition probabilities from the Delta to Wankwar between December and March (dry season), and from Wankwar to Delta in both March to July and July to December (wet seasons). Additionally, these models also suggest sex-biased partial migration, with males having greater seasonal transition probabilities, both to Wankwar in the dry season and to the Delta in the wet seasons. Despite the spatiotemporal variation in habitat quality metrics and the temporal variation in giraffe density across the two sites, we found only a marginal difference in adult/subadult survival over time.
Partial migration is a common movement phenomenon in ungulates, providing unique ecological contexts to explore the causes and consequences of intrapopulation variation in movement strategies (White et al., 2007; Hebblewhite and Merrill, 2009; Mysterud et al., 2011; Singh et al., 2012). Although partial migration has been widely documented in ungulates in temperate systems, which are characterized by strong seasonal variations in resource distribution between summer and winter months, researchers are increasingly identifying evidence for partial migration in tropical ungulate systems, which experience different seasonal resource patterns for both grazing and browsing ungulates (Naidoo et al., 2012a; Gaidet and Lecomte, 2013). Since giraffe are large-bodied browsers in tropical systems, identifying intrapopulation variation in space use strategies of giraffe provides a unique system to evaluate hypotheses on the ecological mechanisms that give rise to partial migration. Although seasonal movements of giraffes have previously been described (Pellew, 1984a; Le Pendu and Ciofolo, 1999; Fennessy et al., 2003) this is the first time that migration between distinct seasonal ranges has been rigorously demonstrated. Our findings also represent the first systematic description of partial migration in giraffe. Furthermore, identifying this pattern of male-biased partial migration in a rapidly growing giraffe population highlights the need to better understand the role of spatiotemporal resource dynamics in driving movement decisions and potentially population dynamics. Studies of temperate ungulates have suggested that not all seasonal variations in space use qualify as migration and that migrants must demonstrate stabilization of seasonal ranges (Gaudry et al., 2015). In our study, however, we noted consistent seasonal ranges for migratory individuals, with relatively few exploratory movements beyond these stable core areas. Furthermore, in migratory or partially migratory populations, migratory individuals move across both geographical space and ecological niche space (Peters et al., 2017). In this population of giraffe, in both collared individuals and population-level surveys, we observed seasonal shifts in space use between phytosociologically distinct sectors. Several hypotheses have been proposed to explain the emergence and maintenance of partial migration (Hebblewhite and Merrill, 2007; Chapman et al., 2011; Mysterud et al., 2011). A prominent hypothesis is the “competitive release hypothesis” wherein individuals move to reduce intraspecific competition in seasonally dynamic environments (Chapman et al., 2011). For this hypothesis to explain partial migration, some individuals in the population must be more vulnerable to competition and thus more likely to migrate to escape it. At high population densities, optimizing resource use by minimizing intraspecific competition may be a dominant driver of movement behaviors (Fryxell and Sinclair, 1988). The MFNP giraffe population is currently at its highest density relative to any point over the past 100 years (Brown et al., 2019), with foraging herds of giraffe exceeding 120 individuals in the Delta during the wet season (M. Brown pers. obs). Given the seasonal dynamics of vegetation in MFNP and the reduction in quantity of forage resources in the dry season, increased resource competition may instigate migratory behaviors. During this period, deciduous trees often lose their leaves and the foliar nutritional and phytochemical properties change, thereby altering the quality and quantity of forage available to browsers (Owen-Smith, 1994). In MFNP, however, plant species vary in their expressed degree of deciduousness with deciduous trees such as Acacia senegal losing a large percentage of their leaves (Omondi et al., 2016), semi-deciduous trees such as C. adansonii and Acacia sieberiana losing only some of their leaves (Shorrocks and Bates, 2015) and evergreen species such as H. abyssinica retaining leaves throughout the dry season. This phenology of leafing, coupled with relatively high browsing pressure in the Delta during the wet seasons, may result in a relative overall decrease in the availability of suitable forage during the dry season. Under these conditions, seasonal partial migration in spatiotemporally heterogenous environments may be viewed as a dynamic realization of ideal free distribution (McPeek and Holt, 1992; Cressman and Krivan, 2006). Lundberg (1987) describes a similar ecological scenario in which the persistence of partial migration in a population results from frequency dependent selection arising from individual decisions conditioned upon resource availability and density of conspecifics. In this way, migration may be perceived as a context dependent tactic in which giraffe respond to shifting cues of relative habitat quality as a function of conspecific density. In this giraffe population, we describe the uncommon scenario of two distinct resident types (Wankwar resident and Delta resident) and a migratory type that seasonally moves between the residential sectors, thereby providing a mechanism for achieving this shifting distribution in a temporally dynamic environment. Under theoretical ideal free distribution, fitness of residents and migrants must be equal over time. Although a more systematic demographic study will be required to assess the relative fitness consequences of various space use strategies in the MFNP giraffe population, the consistently high survival rate across both Delta and Wankwar sector, despite the spatiotemporal changes in giraffe density, lends support to the possibility of dynamic ideal distribution.
For the competitive release hypothesis to explain male-biased seasonal transitions, males must be impacted by competition differently than females or compete for different resources (Dobson, 1982). Among giraffe, this possibility is possible since giraffe exhibit sexually divergent foraging strategies and sexual niche partitioning of forage resources (Young and Isbell, 1991; Ginnet and Demment, 1997; O'Connor et al., 2015). Females typically forage on woody vegetation at lower heights than the larger males (Young and Isbell, 1991; O'Connor et al., 2015). We observed similar trends in the Delta sector of MFNP, with male giraffes consuming proportionally more C. adansonii and female giraffe consuming proportionally more Acacia sp. As a result of the differences in diet composition, the sexes may demonstrate different responses to the availability of the suite of woody vegetation species and the seasonally dynamic competition for forage resources. Furthermore, giraffe exhibit marked sexual size dimorphism with the larger males consequently having different energetic/nutritional requirements. Because of these asymmetries in energetic and nutritional requirements male giraffe may be more affected by the loss of forage quantity due to greater dry season deciduousness in the Delta (Main et al., 1996). Studies examining resource partitioning on the basis of body size in African browsers and grazers suggest that with increased body size, individuals may expand their diets to favor greater quantities of forage species at the expense of consuming forage species of lower nutritional quality (McNaughton and Georgiadis, 1986). In the MFNP ecosystem, this body-size effect may explain the male-biased dry season shift from the diverse, highly nutritious Acacia sp., C. adansonii, and H. abyssinica savannas of the Delta to the lower quality but more abundant forage of the broad-leaf P. kotschyi, S. kunthianum, and Terminalia spp. savanna/woodlands of Wankwar.
Since forage resource quality and quantity are linked to giraffe space use strategies and population dynamics, it is important to develop a deeper understanding of seasonal dynamics of forage availability. Giraffe seasonal migrations are associated with plant phenology in MFNP, with giraffe rapidly returning to the Delta sector following the onset of the rains and the subsequent green-up during the beginning of the short wet season. This seasonal space use pattern is consistent with the forage maturation hypothesis, which predicts that ungulate movement is influenced by selection for high quality forage resources (Hebblewhite et al., 2008). The quality of forage is typically greatest in newly developed plant tissue because of its high cell soluble content and relative lack of structural carbohydrates (Van Soest, 1982). In this way, as the plant resources respond to the commencement of the wet season with new growth, they are of high nutritional value to giraffe. The forage maturation hypothesis may also partially explain how the Delta sector attracts and sustains such large numbers of giraffe throughout the duration of the wet seasons (Fryxell, 1991; Hebblewhite et al., 2008). Other studies suggest that sustained browsing can keep woody vegetation in a chronic state of regrowth, maintaining high forage quality shoots and young leaves (Du Toit et al., 1990; Fornara and du Toit, 2007). For these “browsing lawns” to persist, however, there must be sufficient resources for plants to maintain regrowth processes (Cromsigt and Kuijper, 2011). Thus, in the wet seasons, the Acacia sp., H. abyssinica, and C. adansonii characteristic giraffe diets in the Delta sector, may be able to sustain intense browsing and still provide high quality forage for giraffe but in the dry season, these plants may lack sufficient resources for regrowth, shifting the distribution of giraffe to favor forage quantity (Fryxell, 1991).
In addition to competition for spatiotemporally varying forage resources, competition for mating opportunities may be a potential factor contributing to male giraffe movement strategies. Interestingly, available literature on ungulate migration describes largely female biased migration (Ohms et al., 2019), however the unique reproductive strategies of giraffe among ungulates may help explain this discrepancy. Since female giraffes are as seasonal, asynchronous breeders, and since they are only sexually receptive for a few days during a biweekly estrous cycling, male giraffe allocate much of their time in all seasons moving among scattered herds of females to assess their sexual receptivity (Pratt and Anderson, 1985; Bercovitch et al., 2006). Male access to sexually receptive females is largely mediated through a dominance hierarchy wherein the largest, oldest bulls may displace subordinate male giraffe to monopolize breeding access to females in estrous (Pratt and Anderson, 1985). Previous studies suggest that competition for mates is a primary driver for subordinate juvenile male dispersal across a wide range of mammalian taxa (Dobson, 1982). Under this premise, younger subordinate adult male giraffe in MFNP may track seasonal shifts in female density to ranges where resident dominant bulls may not be able to monopolize access to the seasonal increases in female abundance. In seasonal environments where resource distribution varies predictably over space and time, these constant temporal shifts in both forage resources and mating resources, coupled with asymmetrical sexual competition among males of different sizes, may lead to male biased partial migration. It is important to note, however, that in MFNP, the adult sex ratio is skewed toward females in Wankwar in all seasons. Thus, males moving in any season to Wankwar would seem to be favored by this mechanism—not just movement there in the dry season.
The combination of individual-level GPS telemetry and population-level CRDMS models employed here can allow for key insights into ecological processes that give rise to changes in these movement strategies and the resulting consequences for population dynamics. For instance, researchers can parameterize CRDMS models with temporarily varying sector-specific population size and transition probability between geographic sectors and thereby test for changes through time in movement behavior. The MFNP giraffe population is growing rapidly (Brown et al., 2019), thus as the population continues to grow, these models provide a technique to evaluate density-dependent effects on transition probabilities and survival parameters across space and time. As the population density in the Delta grows larger, we might expect to see an increasing trend in transition probability to Wankwar during the resource-limited dry season. Individual- based GPS telemetry can provide complementary fine-scaled information on changes in the timing of movement, duration of time spent in each range, and specific resources used in each seasonal range, with population-level surveys providing insights on the resulting demographic impacts. The combination of these two approaches has great potential for increasing our understanding of the ecological drivers of partial migration and better understanding the ecological mechanisms giving rise to intraspecific variation of movement strategies and the effects on population dynamics.
Data Availability Statement
The datasets generated for this study will not be made publicly available. The datasets are part of ongoing research which may generate additional publications.
Ethics Statement
Research protocols were approved under Dartmouth Institutional Animal Care and Use Committee (IACUC) guidelines with all fieldwork conducted under Uganda Wildlife Authority (UWA) approved research permit and Uganda National Council of Science and Technology UNCST permit.
Author Contributions
All authors listed have made a substantial, direct and intellectual contribution to the work, and approved it for publication.
Funding
The majority of funding for fieldwork was provided through a partnership with the Giraffe Conservation Foundation (GCF) a conservation non-governmental organization based out of Windhoek, Namibia. Individual donors that contributed to GCF in support of the Uganda programme are listed in the acknowledgments. GCF supported the project logistically as well, providing access to vehicles and partners in country. Dartmouth College provided graduate fellowship stipends and discretionary research funds through the Department of Biological Sciences. Dartmouth College Library provided additional funds for open access publication fees.
Conflict of Interest
The authors declare that the research was conducted in the absence of any commercial or financial relationships that could be construed as a potential conflict of interest.
Acknowledgments
We extend a special thanks to the Giraffe Conservation Foundation, Julian and Steph Fennessy for their incredible support and partnership. Funding and support for all fieldwork and collaring was provided through the Giraffe Conservation Foundation and partners Cleveland Metroparks, Dallas Zoo, Fort Wayne Childrens Zoo, Hogle Zoo, Maltz Family Foundation, Total Uganda, Wildlife Conservation Alliance and WoodTiger Fund. We would also like to thank the dedicated ranger, wildlife veterinarians, research and monitoring crew and administrative staff of the Ugandan Wildlife Authority for facilitating this research. We also thank Jim Nichols and Jim Hines for their invaluable advice and guidance in developing multi-state MARK models for the analysis of population level surveys. S. Mutebi and the staff at Makerere University Herbarium were instrumental in providing validation for plant identification.
Supplementary Material
The Supplementary Material for this article can be found online at: https://www.frontiersin.org/articles/10.3389/fevo.2019.00524/full#supplementary-material
References
Arnason, A. N. (1972). Parameter estimates from mark-recapture experiments on two populations subject to migration and death. Res. Popul. Ecol. 13, 97–113. doi: 10.1007/BF02521971
Arnason, A. N. (1973). The estimation of eopulation eize, migration rates and survival in a stratified population. Res. Popul. Ecol. 15, 1–8. doi: 10.1007/BF02510705
Bensch, S., Liedvogel, M., and Susanne, A. (2011). The genetics of migration on the move. Trends Ecol. Evol. 26, 561–569. doi: 10.1016/j.tree.2011.07.009
Bercovitch, F. B., Bashaw, M. J, and del Castillo, S.M. (2006). Sociosexual behavior, male mating tactics, and the reproductive cycle of giraffe Giraffa camelopardalis. Horm. Behav. 50, 314–321. doi: 10.1016/j.yhbeh.2006.04.004
Bercovitch, F. B., and Berry, P. S. M. (2018). Social and demographic influences on the feeding ecology of giraffe in the Luangwa Valley, Zambia: 1973–2014. Afr. J. Ecol. 56, 254–261. doi: 10.1111/aje.12443
Berthold, P., and Helbig, A. J. (1992). The genetics of bird migration: stimulus, timing, and direction. Ibis 134, 35–40.
Bolger, D. T., Morrison, T. A., Vance, B., Lee, D. E., and Farid, H. (2012). A computer-assisted system for photographic mark-recapture analysis. Methods Ecol. Evol.3, 813–822. doi: 10.1111/j.2041-210X.2012.00212.x
Bolger, D. T., Newmark, W. D., Morrison, T. A., and Doak, D. F. (2008). The need for integrative approaches to understand and conserve migratory ungulates. Ecol. Lett. 11, 63–77. doi: 10.1111/j.1461-0248.2007.01109.x
Brown, M. B., Bolger, D. T., and Fennessy, J. T. (2019). All the eggs in one basket: a countrywide assessment of current and historical giraffe population distribution in Uganda. Glob. Ecol. Conserv. 19:e00612. doi: 10.1016/j.gecco.2019.e00612
Bunnefeld, N., Börger, L., van Moorter, B., Rolandsen, C. M., Dettki, H., Solberg, E. J., et al. (2011). A model-driven approach to quantify migration patterns: individual, regional and yearly differences. J. Anim. Ecol. 80, 466–476. doi: 10.1111/j.1365-2656.2010.01776.x
Burnham, K. P., and Anderson, D. R. (2002). Model Selection and Multimodel Inference: A Practical Information-Theoretic Approach. 2nd Edn. New York, NY: Springer-Verlag.
Cagnacci, F., Focardi, S., Ghisla, A., van Moorter, B., Merrill, E., Gurarie, E., et al. (2015). How many routes lead to migration? Comparison of methods to assess and characterise migratory movements. J. Animal Ecol. 85, 54–68. doi: 10.1111/1365-2656.12449
Carter, K. D., Seddon, J. M., Frère, C. H., Carter, J. K., and Goldizen, A. W. (2013). Fission–fusion dynamics in wild giraffes may be driven by kinship, spatial overlap and individual social preferences. Anim. Behav. 85, 385–394. doi: 10.1016/j.anbehav.2012.11.011
Chabanne, D. B. H., Pollock, K. H., Finn, H., and Bejder, L. (2017). Applying the multistate capture–recapture robust design to characterize metapopulation structure. Methods Ecol. Evol. 8, 1547–1557. doi: 10.1111/2041-210X.12792
Chapman, B. B., Brönmark, C., Nilsson, J. Å., and Hansson, L. A. (2011). The ecology and evolution of partial migration. Oikos 120, 1764–1775. doi: 10.1111/j.1600-0706.2011.20131.x
Cressman, R., and Křivan, V. (2006). Migration dynamics for the ideal free distribution. Am. Nat. 168, 384–397. doi: 10.1086/506970
Cromsigt, J. P. G. M., and Kuijper, D. P. J. (2011). Revisiting the browsing lawn concept: evolutionary interactions or pruning herbivores? Perspect. Plant Ecol. Evol. Syst. 13, 207–215. doi: 10.1016/j.ppees.2011.04.004
Dagg, A. I. (2014). Giraffe: Biology, Behaviour and Conservation. Cambridge: Cambridge University Press. doi: 10.1017/CBO9781139542302
Deacon, F., Nel, P. J., and Bercovitch, F. B. (2015). Concurrent pregnancy and lactation in wild giraffes (Giraffa camelopardalis). Afr. Zool. 50, 331–334. doi: 10.1080/15627020.2015.1120643
Dobson, F. S. (1982). Competition for mates and predomninant juvenile male dispersal in mammals. Anim. Behav. 30, 1183–1192. doi: 10.1016/S0003-3472(82)80209-1
Du Toit, J. T., Bryant, J., and Frisby, K. (1990). Regrowth and palatability of Acacia shoots following pruning by African savanna browsers. Ecology 71, 149–154 doi: 10.2307/1940255
Fennessy, J. T. (2009). Home range and seasonal movements of Giraffa camelopardalis angolensis in the northern Namib Desert. Afr. J. Ecol. 47, 318–327. doi: 10.1111/j.1365-2028.2008.00963.x
Fennessy, J. T., Leggett, K. E. A., and Schneider, S. (2003). Distribution and status of the desert-dwelling giraffe (Giraffa camelopardalis angolensis) in northeastern Namibia. Afr. Zool. 38, 184–188. doi: 10.1080/15627020.2003.11657208
Field, C. R., and Ross, I. C. (1976). The savanna ecology of kidepo valley national park: II. feeding ecology of elephant and giraffe. Afr. J. Ecol. 14, 1–15. doi: 10.1111/j.1365-2028.1976.tb00148.x
Flanagan, S. E., Brown, M. B., Fennessy, J., and Bolger, D. T. (2016). Use of home range behaviour to assess establishment in translocated giraffes. Afr. J. Ecol. 54, 365–374. doi: 10.1111/aje.12299
Fornara, D. A., and du Toit, J. T. (2007). Browsing lawns? Response of Acacia nigrescens to ungulate browsing in an African savanna. Ecology 88, 200–209. doi: 10.1890/0012-9658(2007)88[200:BLROAN]2.0.CO;2
Foster, J. B. (1966). The giraffe of Nairobi national park: home range, sex ratios, the herd, and food. Afr. J. Ecol. 4, 139–148. doi: 10.1111/j.1365-2028.1966.tb00889.x
Found, R., and St. Clair, C. C. (2017). Ambidextrous ungulates have more flexible behaviour, bolder personalities and migrate less. R. Soc. Open Sci. 4:160958. doi: 10.1098/rsos.160958
Fryxell, J. M. (1991). Forage quality and aggregation by large herbivores. Am. Nat. 138, 478–98. doi: 10.1086/285227
Fryxell, J. M., and Sinclair, A. R. (1988). Causes and consequences of migration by large herbivores. Trends Ecol. Evol. 3, 237–241.
Fuda, R. K., Ryan, S. J., Cohen, J. B., Hartter, J., and Frair, J. L. (2016). Assessing impacts to primary productivity at the park edge in Murchison falls conservation area, Uganda. Ecosphere 7, 1–16. doi: 10.1002/ecs2.1486
Gaidet, N., and Lecomte, P. (2013). Benefits of migration in a partially-migratory tropical ungulate. BMC Ecol. 13:36. doi: 10.1186/1472-6785-13-36
Gaudry, W., Saïd, S., Gaillard, J. M., Chevrier, T., Loison, A., Maillard, D., et al. (2015). Partial migration or just habitat selection? Seasonal movements of roe deer in an alpine population. J. Mammal. 96, 502–510.
Ginnet, T. F., and Demment, M. W. (1997). Sex differences in giraffe foraging behavior at two spatial scales. Oecologia 110, 291–300. doi: 10.1007/s004420050162
Hebblewhite, M., and Haydon, D. T. (2010). Distinguishing technology from biology: a critical review of the use of GPS telemetry data in ecology. Philos. Trans. R. Soc. Lond. B Biol. Sci. 365, 2303–2312. doi: 10.1098/rstb.2010.0087
Hebblewhite, M., Merrill, E., and McDermid, G. (2008). A multi-scale test of the forage maturation hypothesis in a partially migratory ungulate population. Ecol. Monogr. 78, 141–166. doi: 10.1890/06-1708.1
Hebblewhite, M., and Merrill, E. H. (2007). Multiscale wolf predation risk for elk: does migration reduce risk? Oecologia 152, 377–387. doi: 10.1007/s00442-007-0661-y
Hebblewhite, M., and Merrill, E. H. (2009). Trade-offs between predation risk and forage differ between migrant strategies in a migratory ungulate. Ecology 90, 3445–3454. doi: 10.1890/08-2090.1
Hess, J. E., Zendt, J. S., Matala, A. R., and Narum, S. R. (2016). Genetic basis of adult migration timing in anadromous steelhead discovered through multivariate association testing. Proc. R. Soc. B: Biol. Sci. 283:20153064.
Kendall, W. L., Pollock, K. H., and Brownie, C. (1995). A likelihood-based approach to capture-recapture estimation of demographic parameters under the robust design. Biometrics 51, 293–308. doi: 10.2307/2533335
Kleinn, C., and Vilčko, F. (2006). A new empirical approach for estimation in k-tree sampling. Forest. Ecol. Manag. 237, 522–533. doi: 10.1016/j.foreco.2006.09.072
Knüsel, M. A., Lee, D. E., König, B., and Bond, M. L. (2019). Correlates of home range sizes of giraffes, Giraffa camelopardalis. Anim. Behav. 149, 143–151. doi: 10.1016/j.anbehav.2019.01.017
Laake, J. L. (2013). RMark: an R interface for analysis of cap- ture-recapture data with MARK. AFSC Processed Rep 2013-01. Seattle, WA: Alaska Fisheries Science Center, NOAA, National Marine Fisheries Service.
Le Pendu, Y., and Ciofolo, I. (1999). Seasonal movements of giraffes in Niger. J. Trop. Ecol. 15, 341–353. doi: 10.1017/S0266467499000863
Lebreton, J. D., Nichols, J. D., Barker, R. J., Pradel, R., and Spendelow, J. A. (2009). Modeling individual animal histories with multistate capture – recapture models. Ecol. Res. 41. doi: 10.1016/S0065-2504(09)00403-6
Leuthold, W., and Leuthold, B. M. (1975). Temporal patterns of reproduction in ungulates of Tsavo East National Park, Kenya. Afr. J. Ecol. 13, 159–169. doi: 10.1111/j.1365-2028.1975.tb00133.x
Lundberg, P. (1987). Partial bird migration and evolutionarily stable strategies. J. Theor. Biol. 125, 351–360.
Magnussen, S., Fehrman, L., and Platt, W. J. (2012). An adaptive composite density estimator for k-tree sampling. Eur. J. Forest Res. 131, 307–320. doi: 10.1007/s10342-011-0502-8
Main, M. B., Weckerly, F. W., and Bleich, V. C. (1996). Sexual segregation in ungulates: new directions for research. J. Mammal. 77, 449–461. doi: 10.2307/1382821
McNaughton, S. J., and Georgiadis, N. J. (1986). Ecology of African grazing and browsing mammals. Annu. Rev. Ecol. Syst. 17, 39–65. doi: 10.1146/annurev.es.17.110186.000351
McPeek, M. A., and Holt, R. D. (1992). The evolution of dispersal in spatially and temporally varying environments. Am. Nat. 140, 1010–1027. doi: 10.1086/285453
McQualter, K. N., Chase, M. J., Fennessy, J. T., McLeod, S. R., and Legget, K. E. A. (2015). Home ranges, seasonal ranges and daily movements of giraffe (Giraffa camelopardalis giraffe) in northern Botswana. Afr. J. Ecol. 54, 99–102. doi: 10.1111/aje.12232
Mueller, T., Olson, K. A., Dressler, G., Leimgruber, P., Fuller, T. K., Nicolson, C., et al. (2011). How landscape dynamics link individual-to population-level movement patterns : a multispecies comparison of ungulate relocation data. Glob. Ecol. Biogeogr. 20, 683–694. doi: 10.2307/41239406
Muller, Z., Bercovitch, F., Brand, R., Brown, D., Brown, M., Bolger, D., et al. (2016). Giraffa camelopardalis. (Errata Version Published in 2017) The IUCN Red List of Threatened Species 2016. e.T9194A109326950.
Mysterud, A. (1999). Seasonal migration pattern and home range of roe deer (Capreolus capreolus) in an altitudinal gradient in southern Norway. J. Zool. Lond. 247, 479–486. doi: 10.1111/j.1469-7998.1999.tb01011.x
Mysterud, A., Loe, L. E., Zimmermann, B., Bischof, R., and Meisingset, E. (2011). Partial migration in expanding red deer populations at northern latitudes - a role for density dependence? Oikos 120, 1817–1825. doi: 10.1111/j.1600-0706.2011.19439.x
Naidoo, R., Du Preez, P., Stuart-Hill, G., Chris Weaver, L., Jago, M., and Wegmann, M. (2012a). Factors affecting intraspecific variation in home range size of a large African herbivore. Landsc. Ecol. 27, 1523–1534. doi: 10.1007/s10980-012-9807-3
Naidoo, R., Du Preez, P., Stuart-Hill, G., Jago, M., and Wegmann, M. (2012b). Home on the range: factors explaining partial migration of African buffalo in a tropical environment. PLoS ONE 7:e36527. doi: 10.1371/journal.pone.0036527
O'Connor, D. A., Butt, B., and Foufopoulos, J. B. (2015). Foraging ecologies of giraffe (Giraffa camelopardalis reticulata) and camels (Camelus dromedarius) in northern Kenya: effects of habitat structure and possibilities for competition? Afr. J. Ecol. 50, 183–193. doi: 10.1111/aje.12204
Ohms, H. A., Gitelman, A. I., Jordan, C. E., and Lytle, D. A. (2019). Quantifying partial migration with sex-ratio balancing. Can. J. Zool. 97, 352–361. doi: 10.1139/cjz-2018-0014
Omondi, S. F., Odee, D. W., Ongamo, G. O., Kanya, J. I., and Khasa, D. P. (2016). Synchrony in leafing, flowering, and fruiting phenology of Senegalia senegal within Lake Baringo Woodland, Kenya: Implication for conservation and tree improvement. Int. J. Forestry Res. 2016:6904834. doi: 10.1155/2016/6904834
Owen-Smith, N. (1994). Foraging responses of kudus to seasonal changes in food resources: elasticity in constraints. Ecology 75, 1050–1062.
Pellew, R. A. (1984a). The feeding ecology of a selective browser, the Giraffe (Giraffa camelopardalis tippelskirchi). J. Zool. Lond. 202, 57–81. doi: 10.1111/j.1469-7998.1984.tb04288.x
Pellew, R. A. (1984b). Food consumption and energy budgets of the giraffe. J. Appl. Ecol. 21, 141–159. doi: 10.2307/2403043
Peters, W., Hebblewhite, M., Mysterud, A., Spitz, D., Focardi, S., Urbano, F., et al. (2017). Migration in geographic and ecological space by a large herbivore. Ecol. Monogr. 87, 297–320. doi: 10.1002/ecm.1250
Pollock, K. H. (1982). A capture-recapture design robust to unequal probability of capture. J. Wildl. Manage. 46, 752–757. doi: 10.2307/3808568
Pollock, K. H., Nichols, J. D., Simons, T. R., Farnsworth, G. L., Bailey, L. L., and Sauer, J. R. (2002). Large scale wildlife monitoring studies: statistical methods for design and analysis. Environmetrics 13, 105–119. doi: 10.1002/env.514
Pratt, D. M., and Anderson, V. H. (1985). Giraffe social structure. J. Nat. Hist. 19, 771–781. doi: 10.1080/00222938500770471
R Core Team (2019). R: A Language and Environment for Statistical Computing. Vienna: R Foundation for Statistical Computing. Available online at: https://www.R-project.org/
Shaffer, S. A., Tremblay, Y., Weimerskirch, H., Scott, D., Thompson, D. R., Sagar, P. M., et al. (2006). Migratory shearwaters integrate oceanic resources across the Pacific Ocean in an endless summer. Proc. Natl. Acad. Sci. U.S.A. 103, 12799–12802. doi: 10.1073/pnas.0603715103
Shorrocks, B., and Bates, W. (2015). The Biology of African Savannahs, 2nd Edn. Oxford: Oxford University Press.
Sinclair, A. R. E., Mduma, S. A. R., and Arcese, P. (2000). What determines phenology and synchrony of ungulate breeding in Serengeti? Ecology 81, 2100–2111. doi: 10.1890/0012-9658(2000)081[2100:WDPASO]2.0.CO;2
Singh, N. J., Börger, L., Dettki, H., Bunnefeld, N., and Ericsson, G. (2012). From migration to nomadism: movement variability in a northern ungulate across its latitudinal range. Ecol. Appl. 22, 2007–2020. doi: 10.1890/12-0245.1
Singh, N. J., and Leonardsson, K. (2014). Partial migration and transient coexistence of migrants and residents in animal populations. PLoS ONE 9:e94750. doi: 10.1371/journal.pone.0094750
Sjöström, M., Ardö, J., Arneth, A., Boulain, N., Cappelaere, B., Eklundh, L., et al. (2011). Exploring the potential of MODIS EVI for modeling gross primary production across African ecosystems. Remote Sens. Environ. 115, 1081–1089. doi: 10.1016/j.rse.2010.12.013
Spitz, D. B., Hebblewhite, M., and Stephenson, T. R. (2017). ‘MigrateR': extending model-driven methods for classifying and quantifying animal movement behavior. Ecography 40, 788–799. doi: 10.1111/ecog.02587
Strauss, M. K. L., Kilewo, M., Rentsch, D., and Packer, C. (2015). Food supply and poaching limit giraffe abundance in the Serengeti. Popul. Ecol. 57, 505–516. doi: 10.1007/s10144-015-0499-9
Struve, J., Lorenzen, K., Blanchard, J., Börger, L., Bunnefeld, N., Edwards, C., et al. (2010). Lost in space? Searching for directions in the spatial modelling of individuals, populations and species ranges. Biol. Lett. 6, 575–578. doi: 10.1098/rsbl.2010.0338
Sutherland, W. J. (1998). Evidence for flexibility and constraint inmigration systems. J. Avian Biol. 29, 441–446. doi: 10.2307/3677163
Torney, C. J., Hopcraft, G. C., Morrison, T. A., Couzin, I. A., and Levin, S. A. (2018). From single steps to mass migration: the problem of scale in the movement ecology of the Serengeti wildebeest. Philos. Trans. R. Soc. B. 373:20170012. doi: 10.1098/rstb.2017.0012
Turchin, P. (1998). Quantitative Analysis of Movement: Measuring and Modeling Population Redistribution in Animals and Plants. Sunderland, MA: Sinauer Associates, Inc.
van der Jeugd, H. P., and Prins, H. H. T. (2000). Movements and group structure of giraffe (Giraffa camelopardalis) in Lake Manyara National Park, Tanzania. J. Zool. 251, 15–21. doi: 10.1111/j.1469-7998.2000.tb00588.x
Van Soest, P. J. (1982). Nutritional Ecology of the Ruminant. 2nd Edn. Corvallis, OR: Comstock Publishing Associates; Cornell University Press.
Villamuelas, M., Fernández, N., Albanell, E., Gálvez-Cerón, A., Bartolomé, J., Mentaberre, G., et al. (2016). The enhanced vegetation index (EVI) as a proxy for diet quality and composition in a mountain ungulate. Ecol. Indic. 61, 658–666. doi: 10.1016/j.ecolind.2015.10.017
White, G. C., and Burnham, K. P. (1999). Program MARK: survival estimations from populations of marked animals. Bird Study 46(Suppl):120–138.
White, P. J., Davis, T. L., Barnowe-Meyer, K. K., Crabtree, R. L., and Garrott, R. A. (2007). Partial migration and philopatry of Yellowstone pronghorn. Biol. Conserv. 135, 502–510. doi: 10.1016/j.biocon.2006.10.041
Keywords: partial migration, giraffe, mark recapture, multi-state model, net squared displacement, GPS telemetry
Citation: Brown MB and Bolger DT (2020) Male-Biased Partial Migration in a Giraffe Population. Front. Ecol. Evol. 7:524. doi: 10.3389/fevo.2019.00524
Received: 02 July 2019; Accepted: 31 December 2019;
Published: 21 April 2020.
Edited by:
Brett K. Sandercock, Norwegian Institute for Nature Research (NINA), NorwayReviewed by:
Derek Benjamin Spitz, University of California, Santa Cruz, United StatesColleen Cassady St. Clair, University of Alberta, Canada
Copyright © 2020 Brown and Bolger. This is an open-access article distributed under the terms of the Creative Commons Attribution License (CC BY). The use, distribution or reproduction in other forums is permitted, provided the original author(s) and the copyright owner(s) are credited and that the original publication in this journal is cited, in accordance with accepted academic practice. No use, distribution or reproduction is permitted which does not comply with these terms.
*Correspondence: Michael B. Brown, bWljaGFlbC5iLmJyb3duLmdyQGRhcnRtb3V0aC5lZHU=