- 1Morphology of Earth Surface and Advanced Geohazards in Southeast Asia Research Unit, Department of Geology, Faculty of Science, Chulalongkorn University, Bangkok, Thailand
- 2Department of Geosciences, Biogeology, University of Tübingen, Tübingen, Germany
- 3PALEVOPRIM, UMR CNRS 7262 INEE, Université de Poitiers, Poitiers, France
- 4Department of Archaeology, Faculty of Archaeology, Silpakorn University, Bangkok, Thailand
- 5The Prehistoric Population and Cultural Dynamics in Highland Pang Mapha Project, Princess Maha Chakri Sirindhorn Anthropology Centre, Bangkok, Thailand
- 6Senckenberg Research Centre for Human Evolution and Palaeoenvironment, University of Tübingen, Tübingen, Germany
Three taxa within the subfamily Caprinae (Himalayan goral Naemorhedus goral, Chinese goral Naemorhedus griseus, and Sumatran serow Capricornis sumatraensis) live in the mountainous upland forests of Southeast Asia, where they are considered as vulnerable or near threatened species. Co-occurrences between these two recognized genera have been documented from some Pleistocene fossil sites in Thailand, suggesting more widely overlapping distribution in the past than today. However, diet and habitat preferences of these Pleistocene and present-day coexisting species have rarely been investigated so far. For the past three decades, stable carbon and oxygen isotope analyses become more commonplace in ecological investigations, allowing us to explore the diets and habitats of ancient and extant animals as well as to reconstruct environmental conditions in the past. We reconstructed diets and habitats of these taxa from five fossil sites in Thailand during the past 400,000 years (from the Middle Pleistocene to the Early Holocene) and from some modern wildlife using the isotopic analysis of carbonate in tooth enamel, in order to test species co-occurrence patterns during the Pleistocene and to examine possible changes of their niche breadths over evolutionary time. Our carbon isotope analysis revealed remarkably different ecological patterns between Naemorhedus and Capricornis. The Pleistocene Sumatran serow has been a greater generalist than both the Himalayan and Chinese gorals that fed on pure C4 or mixed C3 and C4 plants restricted to an open landscape habitat and than its extant population that occupies a closed-canopy forest. This suggests that the habitat contraction of the modern wildlife is likely due to the Holocene climate change and the human impacts on Thai ecosystems. In addition to the loss or reduction of grasslands after the latest Pleistocene when rainforests became dominant and besides the human hunting and predation pressure, the high interspecific competition likely contributed to the extirpation of Himalayan gorals in Thailand. Developing a strategic plan for the future biodiversity conservation, a long-term historical isotope approach allowed us to predict the contrasting habitat suitability, a lowland grassland, for these two threatened goral species as testified by their ecological persistence during the Pleistocene.
Introduction
To allow the conservation of endangered mammal species, it is necessary to find a suitable habitat where they could live and prosper. Looking for habitats similar to the ones where they currently live seems a reasonable approach (e.g., Lekagul and McNeely, 1988; Raia et al., 2012; Rovero et al., 2014; Cavada et al., 2019), but many mammal species today are restricted to relic populations occasionally occupying suboptimal niches that do not reflect the habitat preference of the species, and they would possibly have better chances of long-term survival in a different habitat (e.g., Kuemmerle et al., 2012; Bocherens et al., 2015; Lea et al., 2016). Investigating the ecology of past population of mammal species can therefore provide important information about their preferred habitats and diets, and help to define a successful strategy for their long-term conservation (e.g., Bocherens et al., 2015; Jürgensen et al., 2017; Archer et al., 2019).
Southeast Asian caprines are fundamentally represented by four species within two genera, gorals (Naemorhedus goral and Naemorhedus griseus) and serows [Capricornis sumatraensis (possibly including three subspecies: Capricornis sumatraensis thar, Capricornis sumatraensis milneedwardsii, and Capricornis sumatraensis), and Capricornis rubidus] based on the recently genetic evidence (Dou et al., 2016; Bover et al., 2019; Mori et al., 2019). Their taxonomic status is, however, under debate today. All of these Southeast Asian caprine taxa are currently listed in Appendix I (threatened with extinction) of CITES and categorized as near threatened or vulnerable species on the IUCN Red List of Threatened Species (Duckworth and MacKinnon, 2008; Duckworth and Zaw, 2008; Duckworth et al., 2008a, b,c) (Table 1). The Chinese goral N. griseus and Sumatran serow C. sumatraensis are additionally classified as the Class II-protected species in China (Grubb, 2005).
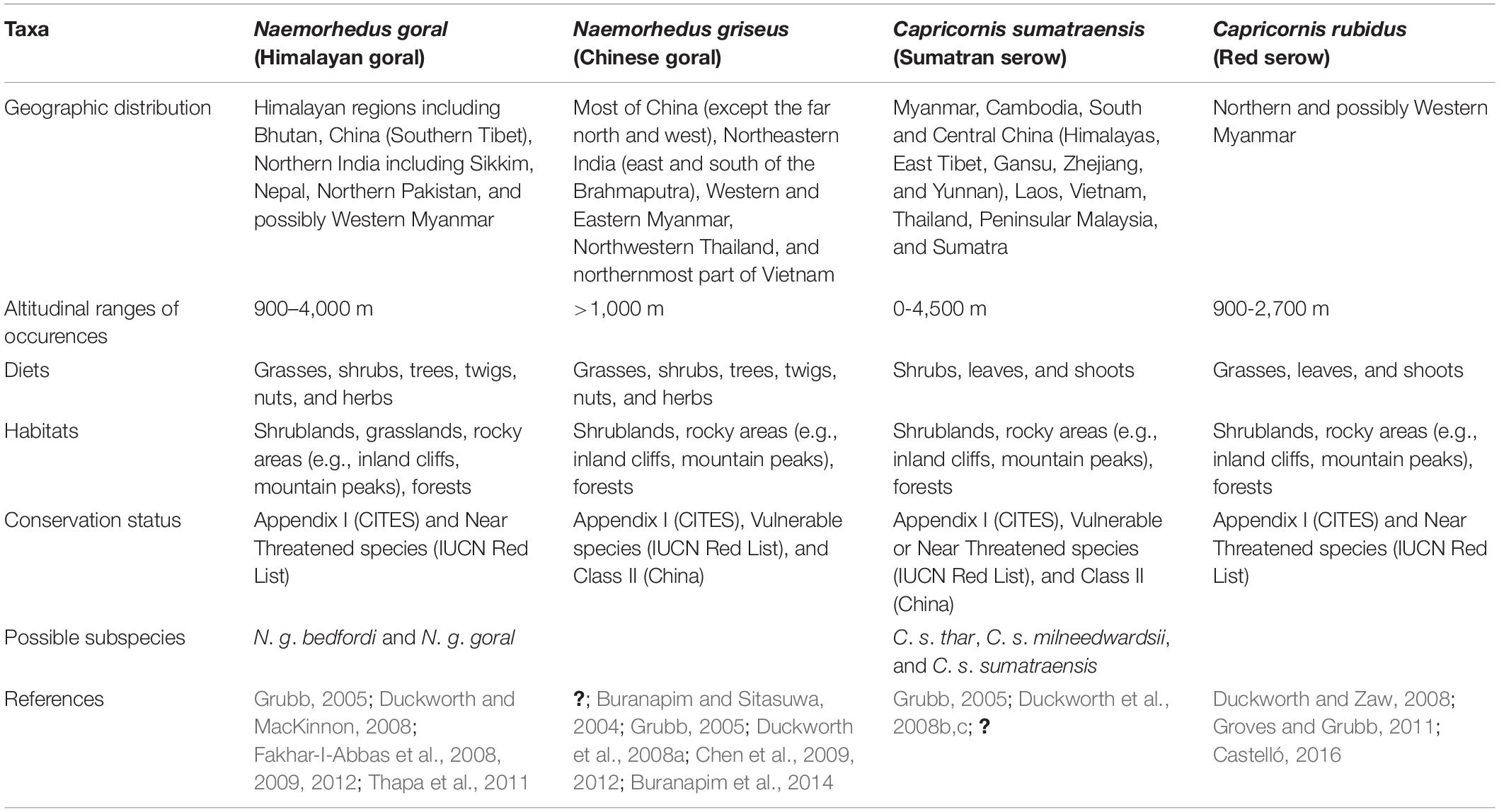
Table 1. Summarized information on geographic distributions, elevation ranges, diets, habitats, and conservation and taxonomic status of extant caprines in Southeast Asia.
These caprines usually inhabit a wide range of habitats from high to low montane forests, along steep slopes and/or near cliffs (Lekagul and McNeely, 1988; Grubb, 2005; Groves and Grubb, 2011) (Table 1). Their current geographic ranges mostly overlap in distributions (except for N. goral), especially C. sumatraensis that coexists widely with N. griseus and possibly C. rubidus in some parts of Myanmar, Thailand, and China (Figure 1). Some previous studies have demonstrated a clear coexistence pattern between extant N. griseus and C. sumatraensis in Western Sichuan, China, where the large area of a nature reserve has an elevation ranging from 1,100 and 4,800 m above sea level and is protected (Chen et al., 2009, 2012). This evidence suggests that these two sympatric caprine species reduced intergeneric competition by occupying different habitats, in terms of canopies, altitudes, and food resources, which may allow their coexistence today. Steep slopes adjacent to the high-altitude area with a high shrub density are preferred by C. sumatraensis, while gentle slopes in the lower altitude area with a lower shrub density are the habitat of N. griseus (Chen et al., 2009, 2012). To better understand occupancy patterns and niche partitioning within these extant caprine communities, investigating their past ecological history that resulted in the basis for those processes is therefore required. In a similar way, an effective future wildlife conservation also needs to take into consideration a substantial difference between the extant and past ecological patterns of the species.
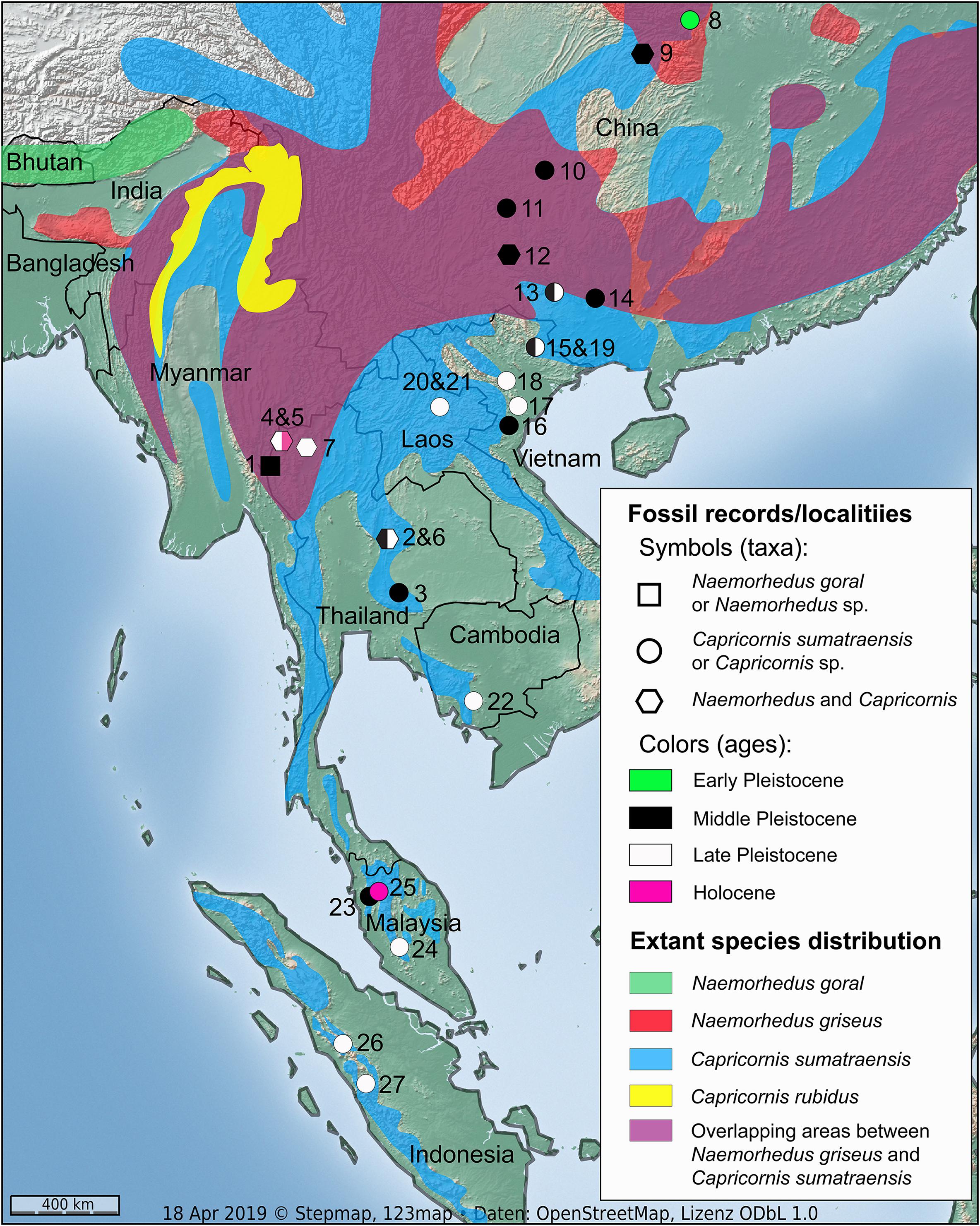
Figure 1. Map of Southeast Asia and South China showing the fossil caprine records from the Early Pleistocene to Holocene sites and the species distribution areas of extant taxa. Numbers of caprine-bearing fossil sites: 1 = Pha Bong (Bocherens et al., 2017), 2 = Tham Wiman Nakin (Tougard, 1998, 2001; Pushkina et al., 2010), 3 = Khok Sung (Suraprasit et al., 2016), 4 = Tham Lod Rockshelter (Wattanapituksakul et al., 2018), 5 = Ban Rai Rockshelter (this study), 6 = Tham Prakai Phet (Filoux et al., 2015), 7 = Cave of the monk (Zeitoun et al., 2010), 8 = Gongwangling (Hu and Qi, 1978; Han and Xu, 1985), 9 = Yenchingkou (Colbert and Hooijer, 1953), 10 = Chihchin (Kahlke, 1961), 11 = Guanyindong (Li and Wen, 1986), 12 = Panxian Dadong (Han and Xu, 1985), 13 = Wuyun (Chen et al., 2002; Wang et al., 2007; Rink et al., 2008), 14 = Wuming (Xue and Zhang, 1991; Rink et al., 2008), 15 = Coc Muoi (Bacon et al., 2018a), 16 = Tham Om (Olsen and Ciochon, 1990), 17 = Lang Trang (Long et al., 1996), 18 = Duoi U’Oi (Bacon et al., 2008a), 19 = Keo Leng (Olsen and Ciochon, 1990), 20 = Tam Hang (Bacon et al., 2011, 2015), 21 = Nam Lot (Bacon et al., 2008b, 2012), 22 = Boh Dambang (Bacon et al., 2018c), 23 = Badak Cave C (Ibrahim et al., 2013), 24 = Batu caves (Ibrahim et al., 2013), 25 = Gua Cha (Groves, 1985), 26 = Lida Ajer (Hooijer, 1958; de Vos, 1983), and 27 = Sibrambang (Hooijer, 1958; de Vos, 1983). For this study, the isotopic samples were collected from five localities (number 1–5) in Thailand.
Based on fossil records during the Early to Late Pleistocene, both Naemorhedus and Capricornis are considered to be members of the typical Southeast Asian mammal assemblages, also known as the “Ailuropoda-Stegodon fauna complex.” Their fossil remains have been found in large areas of Asia including China (e.g., Colbert and Hooijer, 1953; Kahlke, 1961; Hu and Qi, 1978; Han and Xu, 1985; Rink et al., 2008; Zhu et al., 2015), Vietnam (Long et al., 1996; Bacon et al., 2008a, 2018a), Laos (Bacon et al., 2008b, 2011, 2012), Thailand (Tougard, 1998, 2001; Zeitoun et al., 2010; Filoux et al., 2015; Suraprasit et al., 2016; Wattanapituksakul et al., 2018), Cambodia (Bacon et al., 2018c), Peninsular Malaysia (Ibrahim et al., 2013), and Indonesian islands (Java and Sumatra) (Hooijer, 1958) (Figure 1).
In Thailand, previous studies on several Pleistocene mammal assemblages have documented the occurrence of at least three caprine taxa: N. goral, N. griseus, and C. sumatraensis and have sometimes shown coexistences between these two genera [see Suraprasit et al. (2016): appendices 15–17 for further detailed fauna lists of all caprine-bearing sites in Southeast Asia and China]. For instance, the late Middle Pleistocene of Tham Wiman Nakin (Tougard, 1998, 2001) has yielded both N. goral and C. sumatraensis, whose distribution ranges do not overlap today in the area (Figure 1). The co-occurrence of these three species has also been documented from the late Pleistocene of Tham Lod Rockshelter (Wattanapituksakul et al., 2018) where only two caprine taxa coexist today in the area (Figure 1). This implies that their past biogeographic distributions were latitudinally broader and probably overlapped more widely than today. Moreover, the fossil records have also suggested that the extant Southeast Asian caprines are represented by relict populations, which were more widespread during the Pleistocene but became restricted to the highlands and the high- or even low-altitude mountain ranges during the Holocene interglacial. It is then presumable that these caprines were abundant in tropical lowlands and that some habitat partitioning between these closely related species might have occurred in the past coexistence areas. This fossil evidence emphasizes the need to address the evolutionary issues of niche differentiation and competition between these three caprine taxa through space and time, and to understand better the past ecological factors driving the distribution patterns of their modern populations. Moreover, the understanding of the diet and habitat use of these sympatric species is crucial, not only for improving our knowledge on the refugial hypothesis but also to achieve more effective and efficient conservation plan of the species for the future, as it is currently implemented for other endangered species (e.g., Birks, 2012; Louys, 2012; Archer et al., 2019).
Among several ecological approaches, stable isotope tracking on tooth enamel is recognized as a powerful tool for exploring several aspects of the life history of living and fossil animals. This approach has successfully been used to document shifts or restrictions of ecological niches for mammalian species that survived the final Quaternary extinction wave, such as a Madagascar lemur (Crowley et al., 2012), European bison (Bocherens et al., 2015; Hofman-Kamińska et al., 2019), and saiga antelope (Jürgensen et al., 2017). An analysis of stable carbon isotopes (13C/12C) is an efficient method for investigating the diet and habitat use of animals, whether they foraged mostly C3 plants in a closed canopy, C4 plants in an open-habitat, or mixed C3 and C4 plants in an intermediate area (e.g., DeNiro and Epstein, 1978; MacFadden, 1998; Cerling and Harris, 1999; MacFadden et al., 1999; Cerling et al., 2004; MacFadden and Higgins, 2004; Feranec and MacFadden, 2006; Stacklyn et al., 2017). Measurements of stable oxygen isotope composition (18O/16O) in tooth enamel are also useful to explore the drinking or plant consumption behavior of animals due to isotopic differences in local meteoric water, depending on climatic conditions such as air temperature, humidity, and precipitation amount (e.g., Dansgaard, 1964; Bryant et al., 1996; Fricke and O’Neil, 1996; Kohn, 1996; Higgins and MacFadden, 2004). In some Pleistocene Southeast Asian sites, previous carbon isotope measurements on tooth enamel have revealed variabilities in dietary signatures, either pure C3 or C4 plants or even both types of vegetation, for the species of C. sumatraensis [e.g., data from Tham Wiman Nakin (Pushkina et al., 2010), Khok Sung (Suraprasit et al., 2018), Boh Dambang (Bacon et al., 2018c), and Nam Lot (Bacon et al., 2018b)], leading to the question of whether it was fundamentally a grazer or a browser. Moreover, diet and habitat preferences of both species of Naemorhedus (N. griseus and N. goral) have rarely been investigated so far.
We consequently reconstructed the diets and habitats of three Pleistocene Southeast Asian caprine taxa (N. goral, N. griseus, and C. sumatraensis) using the isotopic analysis of carbonate in tooth enamel in order to (1) identify the dietary niches (grazer versus browser) and habitat use by these sympatric species, to (2) test the species co-occurrence patterns, and to (3) investigate the evolutionary changes in the diets and habitats through a long historical timescale ranging from the Middle Pleistocene to the Anthropocene. As a result, we evaluated the ecological flexibility of these Southeast Asian sympatric caprines and then examined the possible cause of extirpation of N. goral in Thailand. We finally propose ecological criteria to identify optimal habitats for the conservation of the remaining populations of Naemorhedus.
Materials and Methods
Study Areas and Sample Collection
We designed a hierarchical isotope sampling of caprine tooth enamel from five different-aged fossil sites (Table 2), namely, Pha Bong (400 to 200 ka), Khok Sung (217 or 130 ka), Tham Wiman Nakin (>169 ka), Tham Lod Rockshelter (32 to 12 ka), and Ban Rai Rockshelter (10 to 6 ka) (Figure 1), as well as from the modern representatives (see Supplementary Material S1 for more detailed information on geological backgrounds of aforementioned sites and see Supplementary Material S2 for the fauna list). A total of 94 bulk tooth enamel samples were extracted mostly from the second or third molars (fully formed adults) of caprine individuals (Supplementary Material S3). The bulk enamel powder was sampled along the entire length of a tooth in order to obtain average isotopic compositions over the time period of dental growth. The analyzed fossils comprise eight specimens of N. goral from Pha Bong, three specimens of C. sumatraensis from Khok Sung, four specimens of N. goral and nine specimens of C. sumatraensis from Tham Wiman Nakin, eight specimens of N. goral, 19 specimens of N. griseus, and 12 specimens of C. sumatraensis from Tham Lod Rockshelter, and six specimens of C. sumatraensis from Ban Rai Rochshelter. Modern specimens including eight specimens of wild N. goral from the Indian Himalayan region, one specimen of wild N. griseus from Northern Thailand, and 16 specimens of wild C. sumatraensis from Thailand and Sumatra were collected from several natural history museums and institutes including Chulalongkorn University Museum of Natural History (CUMNH, Bangkok), Natural History Museum (THNHM, Pathum Thani), Naturhistorisches Museum Basel (NMB, Basel), and Zoologische Staatssammlung München (ZSM, Munich). In this study, we additionally performed the stable isotope analysis of tooth enamel of other mammals from Tham Lod (12 samples) and Ban Rai (10 samples) rockshelters, used as baseline values for isotopic comparisons, in order to understand the environmental contexts of these sites (Supplementary Material S4).
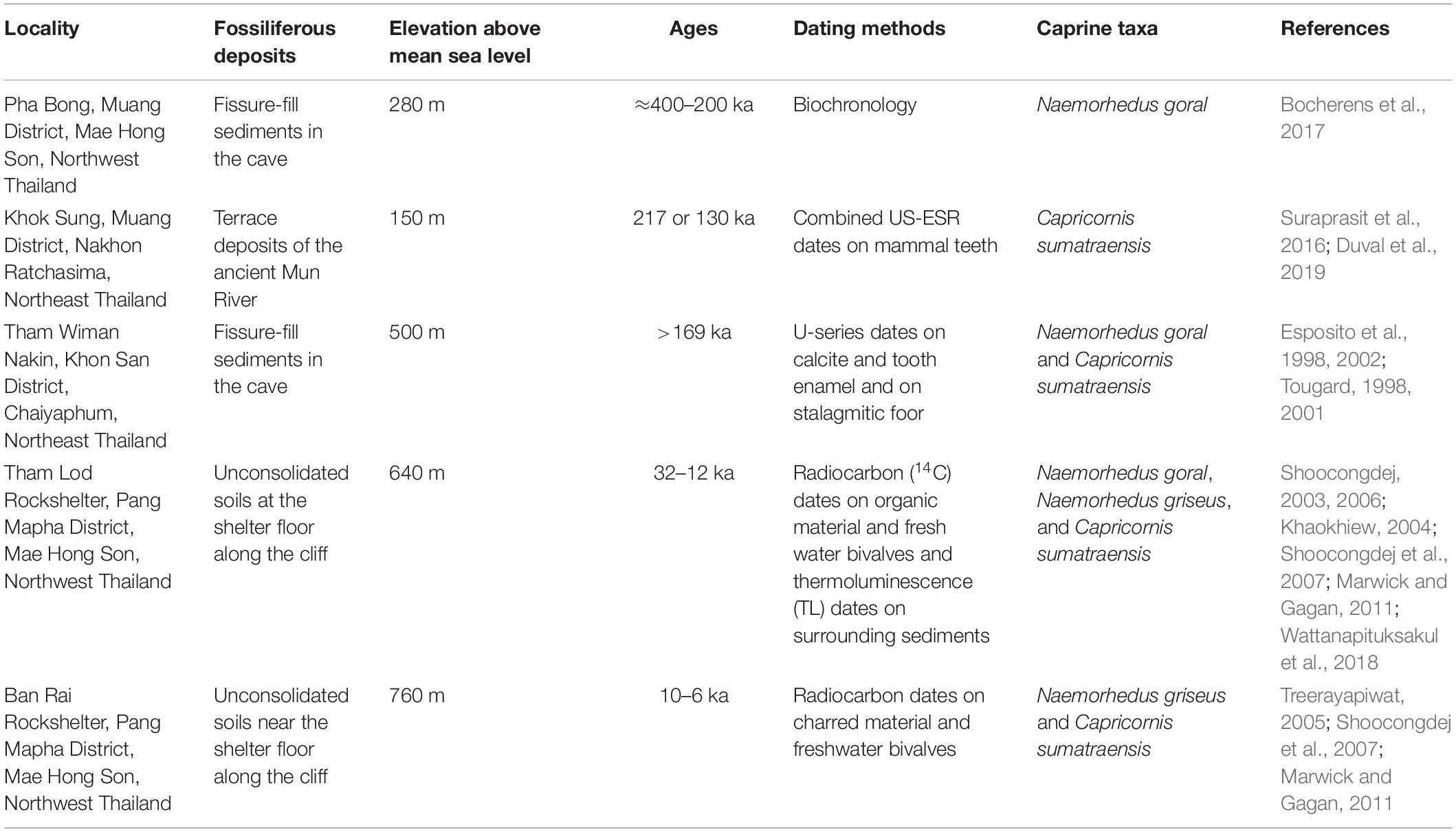
Table 2. Brief information on the locations, geological settings, ages, and presence of caprine taxa of study areas.
Taxonomic Identification
To conduct isotope analysis of tooth enamel, we selected almost complete fossil tooth specimens in order to have access to morphological features that are useful for the taxonomic identification. All analyzed fossil teeth were identified based on their morphological and dimensional comparisons with modern samples. The teeth of N. goral differ from N. griseus in having a less rounded outline on P4, more distinct paracone on upper molars, more distally curved crown on M3 in labial view (straight in N. griseus), U-shaped anterior valley on p3, more prominent labial valley on p4, and more developed conids and entoconulids on lower molars (Wattanapituksakul et al., 2018). The teeth of N. goral are similar in size to those of N. griseus but are clearly smaller than those of C. sumatraensis (Suraprasit et al., 2016; Wattanapituksakul et al., 2018). In addition, Capricornis is distinguished from Naemorhedus by several dental characters: more developed cusps and labial styles on upper premolars and molars (especially the mesostyle and metastylar wing on M3) and more distinct lingual stylids on lower premolars and molars (Wattanapituksakul et al., 2018). In the case of modern samples analyzed here, we followed the taxonomic identification labeled by the field collectors and/or curators.
Isotopic Preparation and Analysis
All caprine enamel samples were pretreated and analyzed at the Department of Geosciences, University of Tübingen, Germany (see Supplementary Material S5 for more detailed information on pretreatment and isotope protocols). Stable carbon and oxygen isotopic values are expressed as the following standard delta (δ)–notation: X = [(Rsample/Rstandard)-1]∗1000, where X is referred to δ13C and δ18O values and R is equivalent to 13C/12C or 18O/16O, respectively. The recorded delta values follow the international reference standards, “Vienna PeeDee Belemnite” (VPDB) for the carbon and oxygen. Additionally, δ18O values relative to “Vienna Standard Mean Ocean Water” (VSMOW) are given. The carbonate content (CaCO3%) was calculated using the ratio between amount of CO2 released by the reaction, as detected from the peak intensity for mass 44 and the weight of pure carbonate used as a standard, with an analytical error of 0.3%, based on the multiple analysis of reference enamel samples.
Before comparing the stable isotope ratios between fossil and modern faunas, we took into account the changes in the carbon composition due to anthropogenic CO2 emission (13C Suess effect) since the beginning of the industrial revolution in 1850 and ongoing today (Keeling et al., 1979). The atmospheric δ13C values in relation to increased CO2 emissions have decreased approximately from −6.5‰ to −8.0‰ over the past few centuries due to fossil fuel combustion and biomass destruction (e.g., Friedli et al., 1986; Marino and McElroy, 1991). To convert δ13C values to modern plant isotope compositions due to the Suess effect, the δ13C values of modern carbonate bioapatite are therefore adjusted upward by 1.5‰ for comparing with the Pleistocene enamel carbon isotope compositions (Cerling et al., 1997a; Cerling and Harris, 1999; Passey et al., 2005). However, modern samples analyzed herein do not require the Suess effect δ13C correction because these wild mammals died or were collected before or around 1950 (Supplementary Material S3).
Statistical Analysis
We performed statistical analyses of the isotopic data (δ13C and δ18O) to show a significant difference among our analyzed specimens. Based on the Shapiro–Wilk test, our samples do not correspond to a normal distribution with unequal variances. In the case for which a sample of at least five individuals is available, we used non-parametric tests to examine differences in median δ13C and δ18O values among our analyzed caprine samples (Kruskal–Wallis test) and between the species and the locality (Mann–Whitney U-test). Significant differences are statistically attained when p-values are equal to or less than 0.05.
Results and Interpretations
To interpret the δ13C and δ18O results of the caprine bioapatite carbonates, we followed the fundamental principles of stable isotope applications on the ecological study of mammals (e.g., Biasatti et al., 2012, pp. 53, 57; Suraprasit et al., 2018, pp. 26, 27). The carbonate content (CaCO3) of caprine tooth enamel varies from 2.4% to 6.9% (Supplementary Material S3), corresponding to the normal range of modern samples that have not been altered during the diagenetic processes (e.g., Ecker et al., 2013). The results thus do not show any evidence of remarkable diagenetic contamination. The enamel carbonate δ13C values of all analyzed caprines exhibit a median of −3.0‰ and a range between +3.0‰ and −16.6‰, whereas the δ18OVPDB results make on average −5.2‰ for a median and range from +0.1‰ to −11.5‰ (Figure 2 and see Supplementary Material S3 for all of the raw stable isotope data). As examined by the Kruskal–Wallis test, there are statistically significant differences in δ13C and δ18O values among fossil caprine taxa from each site and modern representatives (H = 46.898, p < 0.001 for carbon and H = 19.432, p < 0.001 for oxygen). The Kruskal–Wallis statistical test includes all previous data from Pha Bong analyzed by Bocherens et al. (2017) but excludes data from Khok Sung (less than five samples) (see Supplementary Material S6 for further Mann–Whitney U-tests of each caprine taxon from different localities).
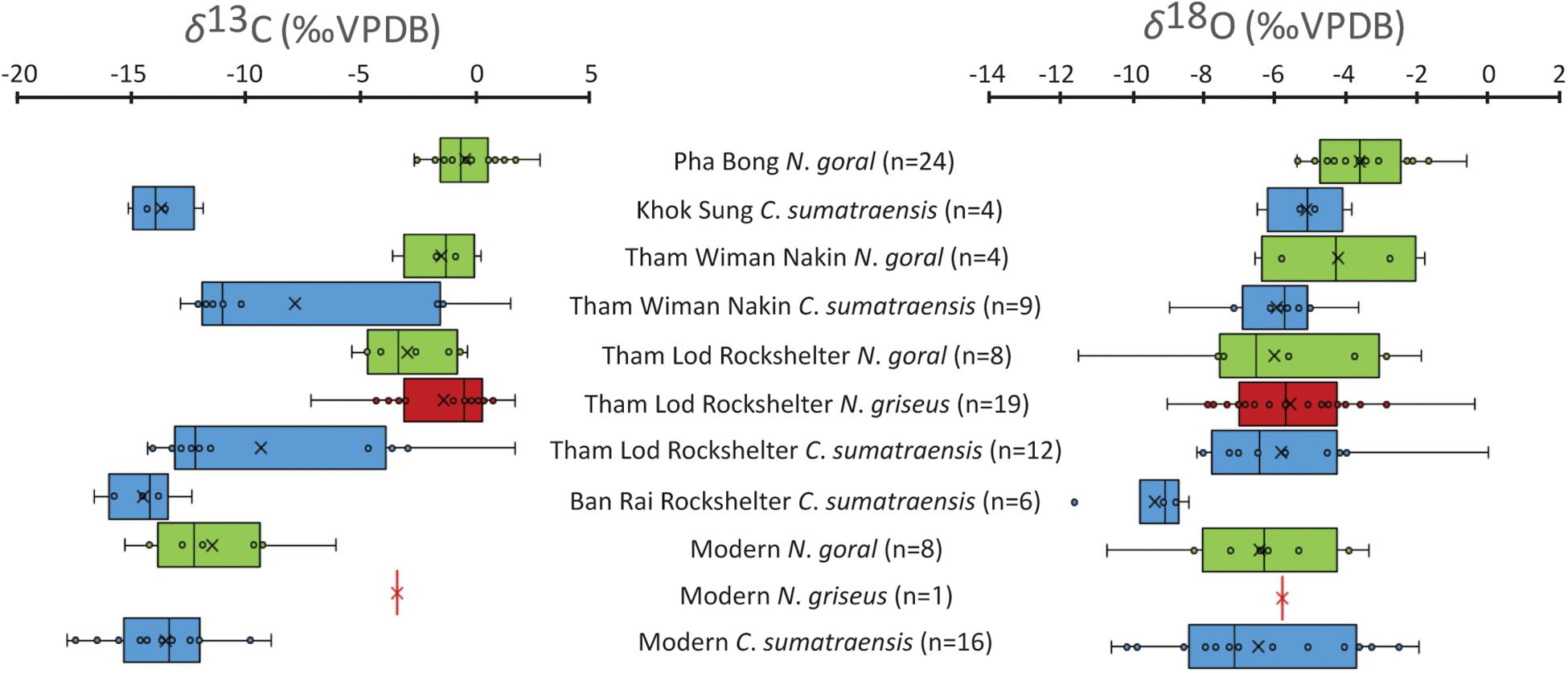
Figure 2. Box plots of enamel δ13C and δ18O values of three caprine species, Naemorhedus goral (green), Naemorhedus griseus (red), and Capricornis sumatraensis (blue) from all analyzed fossil sites and modern representatives. The abbreviation of “n” indicates the number of analyzed samples.
Naemorhedus goral
The δ13C values of Himalayan gorals from Pha Bong (including material previously analyzed by Bocherens et al., 2017) displayed a median of −0.5‰ and ranged from −2.6‰ to +3.0‰ (Figures 2, 3A), indicating the consumption of mostly pure C4 plants with a limited signal of mixed C3 and C4 diets. Compared to the previously isotopic-analyzed samples of Pha Bong Himalayan gorals by Bocherens et al. (2017), our results showed similar tooth enamel δ13C values (Mann–Whitney U: NS; Supplementary Material S6), supporting the occurrence of a single species in this locality. The Himalayan gorals from Tham Wiman Nakin had a similar dietary signature (a median of −1.2‰ and a range between −3.5‰ and +0.4‰) to those of Pha Bong (Figures 2, 3A) but exhibited a shift of the δ13C values toward the C3/C4 mixed signals. A trend of the δ13C shifts into the direction of C3 plants is also observed from the Tham Lod Rockshelter Himalayan gorals, which yielded a median of −3.3‰ and a range between −5.3‰ and −0.1‰ (Figures 2, 3B), indicating their diet selection chiefly on both C3 and C4 plants and only sometimes on pure C4 plants. In contrast, the δ13C values of modern Himalayan gorals displaying a median of −12.2‰ and varying from −15.2‰ to −6.0‰ (Figures 2, 3C) fall into the range of pure C3 and mixed C3/C4 ecosystems, reflecting an adaptation for occupying either more closed habitats or C3-dominated grasslands at the Indian Himalayan Region where the conditions at such an extremely high elevation are unfavorable for C4 plants (Dobremez, 1976; Blasco et al., 1996; Galy et al., 2008; Bhattacharyya et al., 2019).
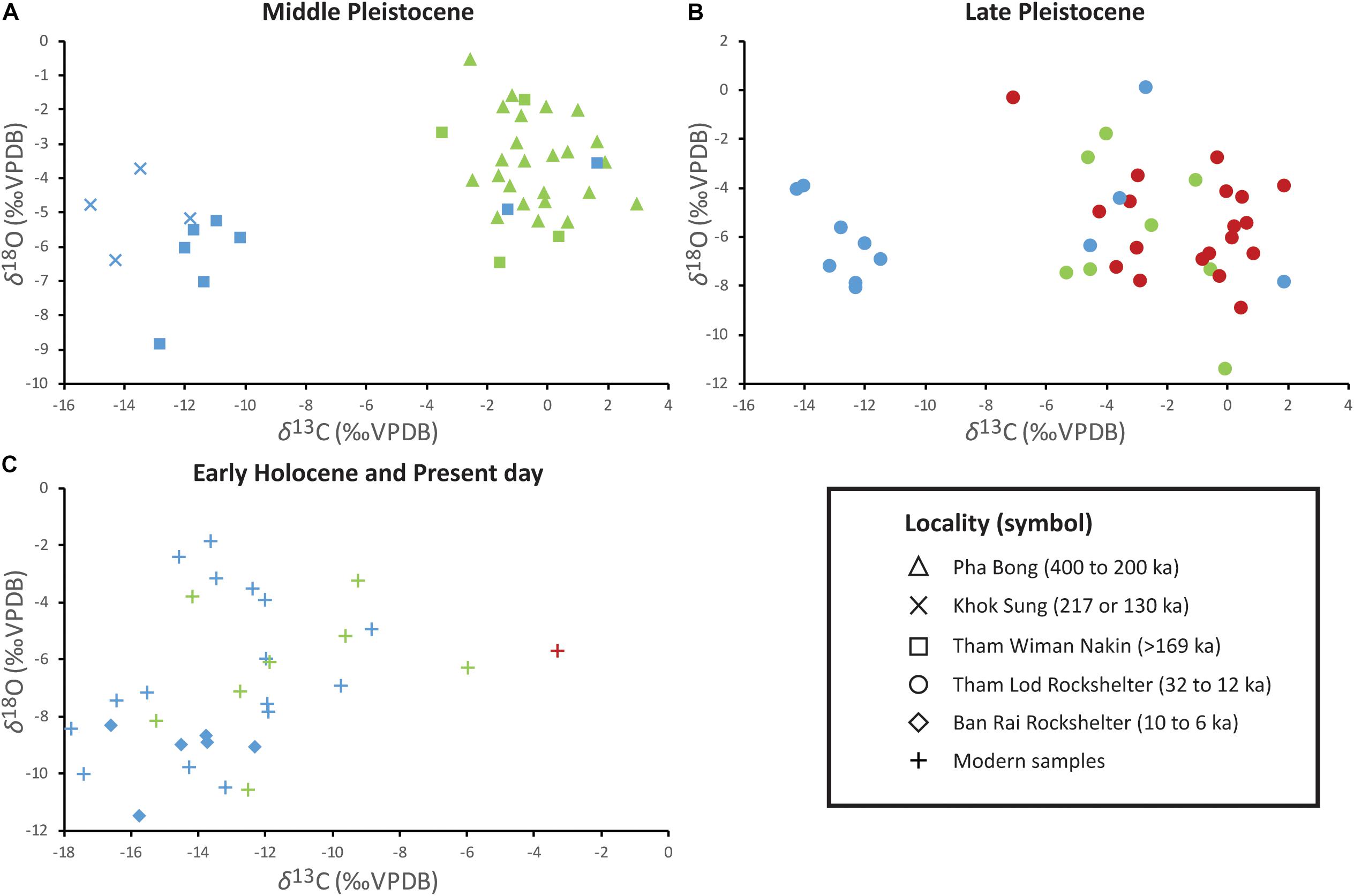
Figure 3. A scatter diagram of enamel δ13C and δ18O values of three caprine species: Naemorhedus goral (green), Naemorhedus griseus (red), and Capricornis sumatraensis (blue), from (A) the Middle Pleistocene of Pha Bong, Khok Sung, and Tham Wiman Nakin, (B) the Late Pleistocene of Tham Lod Rockshelter, and (C) the Early Holocene of Ban Rai Rockshelter as well as the modern representatives. Previous isotope data of N. goral from Pha Bong (16 samples analyzed by Bocherens et al., 2017) and C. sumatraensis from Khok Sung (one sample analyzed by Suraprasit et al., 2018) are plotted together in the diagram.
The Himalayan gorals from Pha Bong showed more positive δ18O values, compared to those from other localities (Mann–Whitney U: P < 0.05; Supplementary Material S6), ranging from −5.3‰ to +0.5‰ with a median of −3.5‰ (Figures 2, 3A), likely resulting from the ingestion of similar water plant resources mediated by evapotranspiration processes that have 18O enrichment. Some individuals, which exhibited higher δ18O values than about −2.2‰ (the first quartile, Figure 2), suggested the consumption of a significant amount of enriched 18O leaves in an open meadow. Lower δ18O values are observed in some individuals found from Tham Wiman Nakin (a median of −5.2‰ and a range from −6.5‰ to −1.7‰) and Tham Lod Rockshelter (a median of −6.4‰ and a range from −11.4‰ to −1.8‰), as well as from the modern representatives (a median of −6.2‰ and a range from −10.6‰ to −3.3‰) (Figures 2, 3), likely a consequence of the consumption of water depleted in 18O compared to those from Pha Bong. The most variable δ18O values recorded from the Tham Lod Rockshelter Himalayan gorals might be explained by more opportunistic feeding on both 18O enriched and depleted plant water. In general, the wide range of tooth enamel δ18O values observed in the Pleistocene and extant Himalayan gorals might reflect the dietary habit of each individual in response to seasonal differences because the modern population has been suggested to feed in the variable frequency on plant species between the summer and winter (Ashraf et al., 2015, 2016). Otherwise, the wide δ18O ranges in the tooth enamel of both Pleistocene and extant Himalayan gorals were possibly due to their seasonal migration across different altitudes in response to temperature changes and threats from predators and hunters (Perveen and Khan, 2013). Among the Himalayan gorals, some higher δ18O values corresponding to more dominated C4 signals are observed, probably reflecting a consumption of 18O enriched leaf water in open habitats (Figure 3 and Supplementary Material S7).
Naemorhedus griseus
Pleistocene Chinese gorals documented only in Tham Lod Rockshelter yielded a median δ13C values of −0.4‰ with a range between −7.1‰ and +1.9‰ (Figures 2, 3B), wider than those of Himalayan gorals from Pha Bong and Tham Wiman Nakin as well as in the same locality (although non-statistical significance tested by Mann–Whitney U; Supplementary Material S6) (Figure 2). This implies that the Chinese gorals had the ability to forage more exclusively on some C3 plants and/or to occupy more closed habitats than the Himalayan gorals. However, this was possibly due to the sampling bias for which more or less specimens were analyzed among these localities. One modern sample of the wild Chinese goral individual collected from the region of Northern Thailand, displays a δ13C value of −3.3‰ (Figure 3C), suggesting the consumption of mixed C3 and C4 plants, similar to some Pleistocene populations in Tham Lod Rockshelter (Figure 2).
The Tham Rod Rochshelter Chinese gorals had a median δ18O value of −5.6‰, similar to the modern sample (−5.7‰), and exhibited broad δ18O values varying from −8.9‰ to −0.3‰ (Figures 2, 3B), suggesting that they derived their water from highly variable food or local resources, similar to what is observed in the congeneric species (N. goral) in the same locality. Otherwise, it is conceivable that the Chinese gorals might have ingested water from local resources in different altitudes in relation to their seasonal habitat use or migration, as seen in the modern population (Chen et al., 2009, 2012).
Capricornis sumatraensis
The δ13C values of Khok Sung Sumatran serows including three samples in this study and one previously analyzed material from Suraprasit et al. (2018, Table 1) displayed a median of −13.9‰ and a range between −15.1‰ and −11.8‰ (Figures 2, 3A), indicating diet and habitat preferences in a closed C3-dominated environment. The Sumatran serows recovered in Tham Wiman Nakin and Tham Lod Rockshelter showed a median of −11.0‰ and −12.2‰ (Mann–Whitney U: NS; Supplementary Material S6) and exhibited considerably wide δ13C values ranging from −12.8‰ to +1.7‰ and from −14.3‰ to +1.9‰, respectively (Figure 2). The Sumatran serows from both localities inhabited the various types of tropical ecosystems from exclusively closed C3 to open C4 vegetation landscapes. The carbon isotope compositions of tooth enamel of these Pleistocene Sumatran serows clearly support a broader foraging niche than those for Naemorhedus (Mann–Whitney U: P < 0.05; Supplementary Material S6). However, the Early Holocene Ban Rai and modern populations showed more limited diets of either pure C3 or C3/C4 mixed vegetation (Figure 3C) and more restricted habitat use in either closed environments or areas adjacent to forest/woodland landscapes based on the median δ13C values of −14.1‰ with a narrow range between −16.6‰ and 12.3‰, for the former site and on the median δ13C values of −13.3‰ with a narrow range between −17.8‰ and −8.8‰ for the latter (Mann–Whitney U: NS; Supplementary Material S6).
Highly variable δ18O values are observed among most of the Pleistocene and modern Sumatran serows including a median of −5.7‰ and a range between −8.9‰ and −3.6‰ for Tham Wiman Nakin, a median of −6.3‰ and a range between −8.1‰ and +0.1‰ for Tham Lod Rockshelter, and a median of −7.0‰ and a range between −10.5‰ and −1.9‰ for the modern population (Mann–Whitney U: NS; Supplementary Material S6) (Figure 2). Most of the Pleistocene and extant Sumatran serows with the exception of Khok Sung samples thus indicated their independent habits in consuming various leaf water or ingesting meteoric water across variable local resources. The narrower range of the δ18O values, with a median of −5.0‰ and a range between −6.4‰ and −3.7‰ (Figure 2), for Khok Sung serows might be explained by fewer isotopic-analyzed samples from this locality. Compared to other localities, C. sumatraensis from Ban Rai Rockshelter likely displayed more limited and negative δ18O values with a median of −9.0‰ and a range between −11.5‰ and −8.3‰ (Figure 2) (Mann–Whitney U: P < 0.05; Supplementary Material S6), corresponding to its occupation of a closed forest canopy with a more humid condition. Some C4 grazers tended to have higher δ18O values compared to C3 browsers, consistent with their dietary intake and habitat use (Figure 3 and Supplementary Material S7).
Discussion
Evolutionary Niche Differentiation and Adaptation of Naemorhedus and Capricornis Through Time
Analyzing stable carbon isotope composition of fossil caprines from different time periods shows that none of the analyzed Pleistocene N. goral revealed a significant shift in their tooth enamel δ13C values over time prior to the Holocene (Figure 4). N. goral therefore had an ecological persistence on the diet of exclusively C4 plants but sometimes mixed C3/C4 vegetation and on the habitat in open environments through the Middle to latest Pleistocene. In contrast, stable carbon isotope compositions of modern samples of N. goral showed that their δ13C values became lower, indicating a diet with significantly more C3 food items in more closed habitats or C3-dominated grasslands (Figure 4). This isotopic shift corresponds to the extant Indian Himalayan population that is surviving in the extreme condition of highland or mountain climate refugia where the C3-grasslands are dominant (Dobremez, 1976; Blasco et al., 1996; Galy et al., 2008; Bhattacharyya et al., 2019) and where the anthropic effects are presumably weaker.
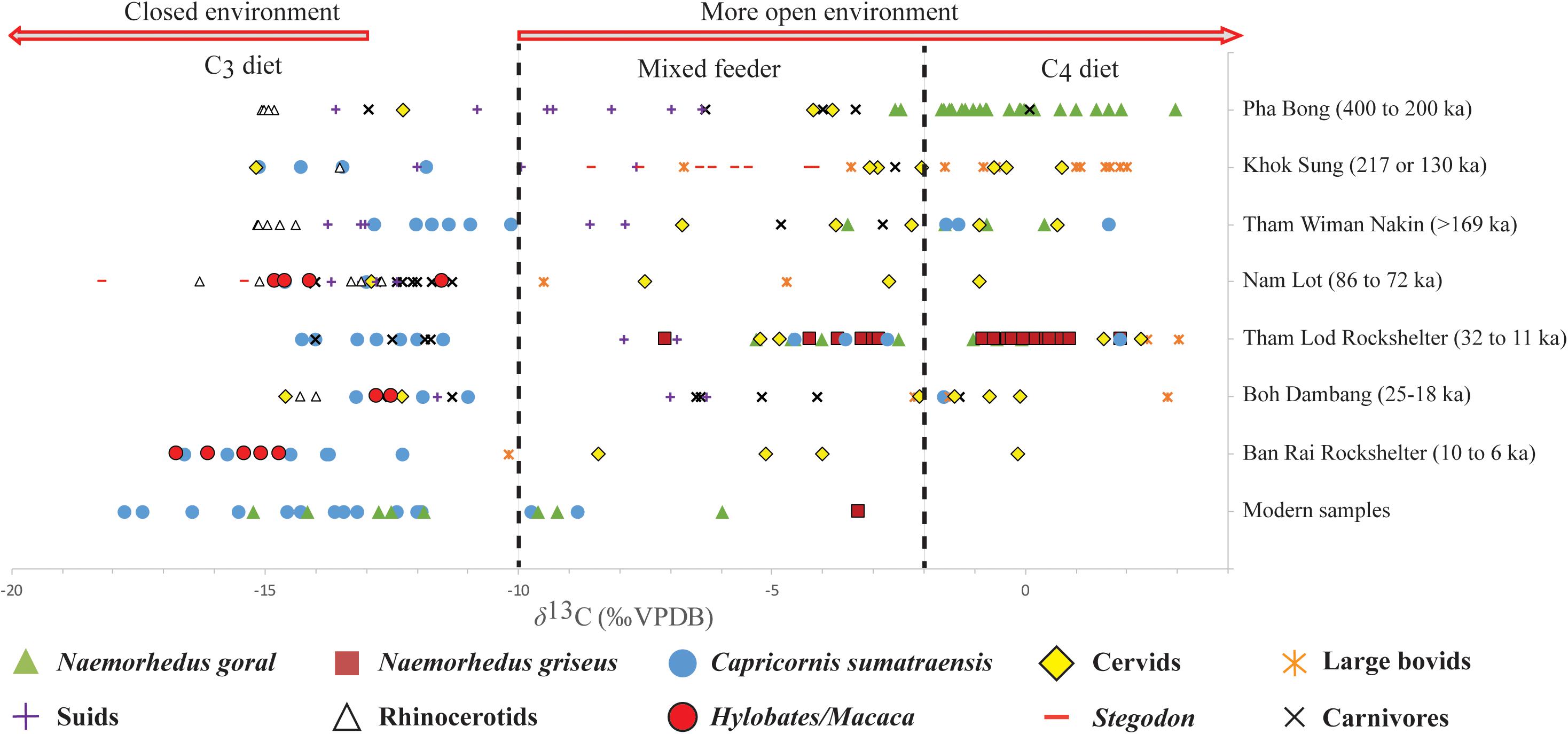
Figure 4. Bulk δ13C values of mammals from several Pleistocene to Holocene caprine-bearing sites in Southeast Asia. In the tropics, the cut-off δ13C values of −10‰ between pure C3 and C3/C4 mixed diets and of +2‰ for pure C4 diet (dashed lines) are applied to herbivorous ungulates according to Cerling et al. (1997a), Cerling and Harris (1999), and Biasatti et al. (2012). The cut-off δ13C value of lower than −13‰ is adopted here for the mammals that lived in closed forests according to Cerling et al. (1997a, 2004), Kohn et al. (2005), and Biasatti et al. (2012). For the isotope comparison between large herbivores and predators, the fractionation factor of δ13C values for carnivores is adjusted by +1.3‰ (Clementz et al., 2009; Domingo et al., 2013). The faunal isotope baseline includes previously analyzed enamel samples from Pha Bong (Bocherens et al., 2017), Khok Sung (Suraprasit et al., 2018), and Tham Wiman Nakin (Pushkina et al., 2010; Bocherens et al., 2017) in Thailand, Nam Lot (Bacon et al., 2018b) in Laos, and Boh Dambang in Cambodia (Bacon et al., 2018c) (see Supplementary Material S4 for raw data).
The isotopic analysis of tooth enamel of N. griseus from the Late Pleistocene of Tham Lod Rockshelter indicated its occupation of resources in the same habitats of N. goral (Mann–Whitney U: NS; Supplementary Material S6). Although only one modern isotope sample of N. griseus has been analyzed for this study due to the inaccessibility and rareness of wild individual specimens, the Chinese goral usually inhabits steep landscapes and plateaus in mountainous areas or sometimes subtropical mixed and evergreen-deciduous forests near cliffs based on the field observation (Duckworth et al., 2008a; Chen et al., 2009, 2012; Groves and Grubb, 2011; Buranapim et al., 2014; Buranapim and Sitasuwa, 2014). This information recorded in the field indicates an ecological adaptation struggling to live in a more closed habitat for the extant population of N. griseus, despite having mixed C3/C4 plants in diet (as seen in the single analyzed sample from this study), different from what has initially been observed in some Pleistocene inhabitants that mainly occupied an open habitat.
Our stable isotope results also indicate that C. sumatraensis has been well-adapted to variable environmental conditions expanding from closed C3 to open C4 landscapes since the Middle Pleistocene and has been a generalist with a greater ecological flexibility in diet and habitat than both Pleistocene and extant Naemorhedus (Figures 2, 4). Although some isotopic data from well-dated localities such as Khok Sung (MIS7 or MIS5) (Suraprasit et al., 2018; Duval et al., 2019; this study) and Nam Lot (MIS5) (Bacon et al., 2018b) as well as from the Early Holocene of Ban Rai Rockshelter have documented the preferred habitat restriction of the Sumatran serows in closed forest environments (Figure 2), this might be explained by the sampling bias for which few specimens are available or analyzed or by the ecological adaptation of C. sumatraensis in response to more homogeneous and closed conditions during interglacial stages. In the heterogeneous environments during the Pleistocene, C. sumatraensis preferably occupied in both closed forest and open grassland landscapes but rarely in an intermediate area between these two canopies. The rareness of C. sumatraensis as being a mixed C3/C4 feeder in the intermediate habitat area was possibly a consequence of its competition avoidance for food resources with other local wildlife such as megaherbivores (e.g., Stegodon) and omnivores (e.g., Sus) (Figure 4). Previous isotope studies have revealed that Stegodon was mainly a C3/C4-mixed feeder and significantly held an intermediate canopy landscape in some coexisting area (e.g., Khok Sung, Suraprasit et al., 2018) (Figure 4). Moreover, the preferred habitat of suids in the intermediate habitat canopy between closed and open ecosystems remained relatively stable over time (e.g., Pha Bong, Khok Sung, Tham Wiman Nakin, Nam Lot, and Boh Dambang) (Pushkina et al., 2010; Bocherens et al., 2017; Bacon et al., 2018b, c; Suraprasit et al., 2018) (Figure 4 and Supplementary Material S4). The limited δ13C range of numerous modern enamel samples suggests that the ecological flexibility of C. sumatraensis has become restricted after the latest Pleistocene (Figures 3C, 4). This rests on the assumption that the present-day wildlife habitat in Thailand is likely constrained by the Holocene climate change and the human impact on the ecosystem restricted its population to more closed canopy habitats such as dense forests in higher altitude areas.
Tooth Morphology and Body Size Influences on the Ecological Adaptation of Caprines
As Naemorhedus and Capricornis did coexist in several Thai fossil sites during the Pleistocene, niche partitioning between them might have occurred on other aspects that are not discernible through the stable carbon isotope analysis.
Regarding the dental morphology, these three caprine taxa possess a distinctly selenodont appearance with similar occlusal patterns. In the evolutionary history of mammals, the development of hypsodont teeth allows them to feed on more abrasive food such as grasses and to therefore expand the range of dietary resources (e.g., Mendoza and Palmqvist, 2008; von Koenigswald, 2011). Both Naemorhedus species have the same degree of hypsodonty as an index of 4.03 (Mendoza and Palmqvist, 2008), while C. sumatraensis has comparatively lower (but still high) crowned teeth according to the hypsodonty index of 3.39 (Kaiser et al., 2013). The dental morphologies of these three caprine species are congruent with our stable carbon isotope results, which indicate their life history adaptations to grazing during the Pleistocene.
Additionally, differences in the average body size between Naemorhedus and C. sumatraensis, as exemplified from the tooth dimensions on fossils (e.g., Suraprasit et al., 2016; Wattanapituksakul et al., 2018) and from the observation of recent populations (e.g., Nowak, 1999; Grubb, 2005) may permit them to feed at different elevations. The body size of N. goral and N. griseus is identical and ranges from approximately 22–35 kg for an adult individual (Nowak, 1999; Grubb, 2005). The Sumatran serows, in general, having a larger body size (about 50–140 kg) (Nowak, 1999), are more capable of foraging the higher level of plant bodies, such as leaves of shrubs or small trees, than the Himalayan and Chinese gorals. In an open habitat landscape around Tham Lod Rockshelter, C. sumatraensis might indeed have had more intense competition with other similar-sized grazers such as an Eld’s deer Rucervus eldii (about 95–150 kg; Nowak, 1999) than these two smaller gorals, as suggested by our carbon isotope data (Figure 4 and Supplementary Material S4).
Although the tooth morphology supports a grazing adaptation for both genera, differences in body size between Naemorhedus and C. sumatraensis might have led to niche partitioning and minimized intergeneric competition, which have likely contributed to their prevalent coexistence during the Late Middle Pleistocene (i.e., Tham Wiman Nakin) and during the Last Glacial Maximum (LGM) (i.e., Tham Lod Rockshelter).
Causes of Local Extinction of Himalayan Gorals in Thailand
Today Chinese gorals and Sumatran serows are surviving in the wild despite having been considered as being vulnerable species due to a population decline (Duckworth et al., 2008a, b), while Himalayan gorals went locally extinct from the Thai territory likely at the end of the Pleistocene based on the lack of their fossil remains in the Holocene stratigraphic sequence records, as deduced from the rockshelters of Tham Lod and Ban Rai in Mae Hong Son, Northwestern Thailand. We thus proposed other possible extinction-related causes that are discernible through these two archeological sites.
It is likely that the environments around the Mae Hong Son Province where the three caprine taxa did coexist became more homogeneous and closed after the end of the Pleistocene based on the possible δ13C shift of C. sumatraensis and Bubalus arnee into more exclusively C3-dietary signals (Figure 4), and the high diversity and abundance of primates (Supplementary Material S2) in the Early Holocene of Ban Rai Rockshelter. Although an open landscape might have existed around the region of Ban Rai Rockshelter based on the C4 signals of Sambar deer Rusa unicolor (Figure 4), large bovid remains are very scarce in Ban Rai Rockshelter and cervid subfossils are much less abundant than those found in Tham Lod Rockshelter. Supporting this argument, the sequential δ18O records of fresh water bivalves from both localities have indicated that substantially increased precipitation and decreased climate variability occurred in Northwestern Thailand after 9,800 BP (Marwick and Gagan, 2011). The climatic and environmental changes related to an increasing development of rainforests starting at the Late Pleistocene/Holocene transition likely contributed to the local extinction of grassland-dwelling taxa as Naemorhedus but other ecological factors also need to be considered.
The major role of anthropic activities was characterized by the accumulation of teeth and bones in Tham Lod Rockshelter where the animal remains have been modified (e.g., traces of fire, stone artifacts, and cut marks) by human hunter-gatherers at that time. Carnivores such as Asiatic black bear Ursus thibetanus and tiger Panthera tigris were major predators in the Tham Lod Rockshelter area but likely did not play a significant role in modifying or accumulating the skeletal elements in the assemblage. According to the observed fire modification of bones, it is assumed that prehistoric humans also hunted these carnivores for food and resources (Wattanapituksakul et al., 2018). Wattanapituksakul et al. (2018) demonstrated that prime adult individuals of Naemorhedus and Capricornis were preferentially selected by the hunter-gatherers based on the mortality profile analysis. The highest number of caprine individuals in the accumulation of the Tham Lod Rochshelter assemblages is observed in N. griseus (represented by the minimum number of individuals: MNI = 40), followed by C. sumatraensis (MNI = 15) and N. goral (MNI = 13). This evidence may provide general information on the abundance of caprine populations in this area (N. griseus > C. sumatraensis > N. goral), reflecting that N. goral was likely more scarce than N. griseus in the area and probably started decresing in its population prior the latest Pleistocene. Moreover, the mortality profile analyzed in Tham Lod caprines implies a hunter-gatherer subsistence strategy with selective hunting of easy preys such as these gorals and serows (Wattanapituksakul et al., 2018). As exemplified by the Tham Lod Rockshelter assemblage, we suggest that the impacts of the human hunting on the Himalayan and Chinese goral community were possibly one of the major factors leading to their population decline in that region during the latest Pleistocene.
Concerning the effects of predation pressure on these gorals, the diversity of carnivores in Southeast Asia appears to have been higher in the closed canopy than the open area throughout the Pleistocene based on numerous fossil records and previous carbon isotope data so far (e.g., Pushkina et al., 2010; Bocherens et al., 2017; Bacon et al., 2018b, c) (Figure 4). Our isotope results analyzed on the Tham Lod carnivores indicate their habitat preferences in closed forest ecosystems far away from the ones where the Himalayan and Chinese gorals lived at that time (Figure 4). Accordingly, the predation probably had a direct effect on which both of the goral species were forced to avoid closed habitats occupied by more diverse predators. Other predators that occupied an open habitat have been poorly known from the Late Pleistocene of Southeast Asia since spotted hyaenas possibly went extinct prior to MIS2 (see discussion in Suraprasit et al., 2015, 2019). These gorals consequently maintained their preferred habitats in the different area as an open landscape where predation pressure was lower or minimal.
Regarding the coexistence patterns, other sympatric herbivore species such as large bovids and cervids mainly occupied the open habitat around Tham Lod Rockshelter based on our isotope results (Figure 4). Accordingly, they possibly competed for available food resources with these three caprine taxa. However, this study has demonstrated that the interspecific exploitative competition between sympatric N. goral and N. griseus in Tham Lod Rockshelter was more intense than the intergeneric overlap for Capricornis and Naemorhedus due to the inflexibility of shared food resources and habitats in an open landscape for both goral species. Indeed, N. griseus might have been better adapted to a broader realized niche than N. goral did according to our stable carbon isotope analysis (Figures 2, 3B). The considerable competition for resources between these two closely related species that have a similar dental morphology and body mass and that occupied the same habitat might have led to their reduced population over time and to an extirpation of one species (i.e., N. goral) at the end of the Pleistocene.
Overall, we propose that these four factors: (1) the loss or reduction of grassland habitats after the latest Pleistocene when rainforests became dominant, (2) the negative effects of human hunting, (3) the predation pressure, and (4) the high interspecific competition between both goral species were possibly main causes driving the patterns of local extinction of N. goral in Thailand.
The Ecological Proposal for the Species Conservation of Extant Himalayan and Chinese Gorals
The pace of decline population of extant threatened Himalayan and vulnerable Chinese gorals are accelerating and their distribution ranges are constantly decreasing (Mead, 1989; Duckworth and MacKinnon, 2008) due to the habitat loss caused by several disturbance factors of natural processes (such as forest fire) and human activities (such as deforestation, hunting, and agricultural and commercial use) (Lydekker, 1907; Anwar, 1989; Duckworth and MacKinnon, 2008; Duckworth et al., 2008a; Ashraf et al., 2015; Shakeel et al., 2015). Some studies on the feeding biology of modern Himalayan and Chinese gorals directly targeted at the field have indicated that these two species are both grazers and browsers based on the food availability in their stomach, gizzard, and rumen content (e.g., Anwar and Chapman, 2000; Awasthi et al., 2003; Junaid et al., 2012; Buranapim and Sitasuwa, 2014). However, the most preferred food of both Himalayan and Chinese gorals are grasses, followed by shrubs, trees, twigs, nuts, and herbs (Duckworth and MacKinnon, 2008; Duckworth et al., 2008a; Fakhar-I-Abbas et al., 2008, 2009; Buranapim et al., 2014; Buranapim and Sitasuwa, 2014). Our stable isotope results of tooth enamel of the Himalayan gorals reflected that this refugee species usually consumes the C3 vegetation or grasses nowadays on the Himalayan regions where no or few C4 grasses are able to develop under such an extremely high altitude condition due to an increase of atmospheric CO2 and humidity levels after the LGM (Galy et al., 2008). As is the case for N. griseus, the current geographic distribution clearly shows the habitat restriction of this species in the high-elevation mountain areas (e.g., the regions of Northwest Thailand and South China). Although the natural or anthropogenic grasslands may exist somewhere in these regions, most of which are covered by dense forests such as deciduous dipterocarp and coniferous forests (Duckworth et al., 2008a; Groves and Grubb, 2011; Buranapim et al., 2014) (Table 1). In subtropical regions where Chinese gorals are living and where vegetation types vary along altitudinal gradients, alpine shrubs and grasslands generally occur at the highest altitudinal zonation of mountainous regions (e.g., Sichuan in China), followed by coniferous and mixed evergreen and deciduous forests in the lower-elevation canopy landscapes (Chen et al., 2009, 2012) (Figure 5). In contrast, based on paleobotanical evidence, the plant zonation in Southeast Asia during Pleistocene glacials has been suggested to drop about 1,000 m in elevation lower than today, implying the expansion of grasslands in low-altitude mountain ranges and lowland floodplains (e.g., Kershaw et al., 2001; Morley, 2012) (Figure 5). A long-term ecological stasis in the occupation of both N. goral and N. griseus based on our isotope analysis of tooth enamel through several periods of glacial-interglacial cycles therefore provides a dietary consistency on (C3 or C4) grass components but a contrasting traditional view of their habitat use during the Pleistocene when the anthropic impacts on the ecosystems were lower than today. This study has demonstrated that the populations of both N. goral and N. griseus were C4 grazers in lower altitude grasslands on mountainous areas or lowland floodplain during the Pleistocene glacials (e.g., Pha Bong, Tham Wiman Nakin, and Tham Lod Rockshelter) (Figure 5). It is thus presumable that, during the Holocene, the warming climate and intensely anthropic effects have forced these two goral species to retreat upslope in pursuit of their comfort zone with the food availability (grasses). Particularly, the critical anthropic effects still happen on a regular basis upon the modern wildlife goral community with or without intention and may drive these species to the population decline or extinction risk in the future. Besides minimizing the ecological effects caused by human activities, we propose an alternative conservation-concern plan about the sustainable biodiversity management by supplying an optimal open lowland area with the availability of grass resources to the rest of Himalayan and Chinese goral populations.
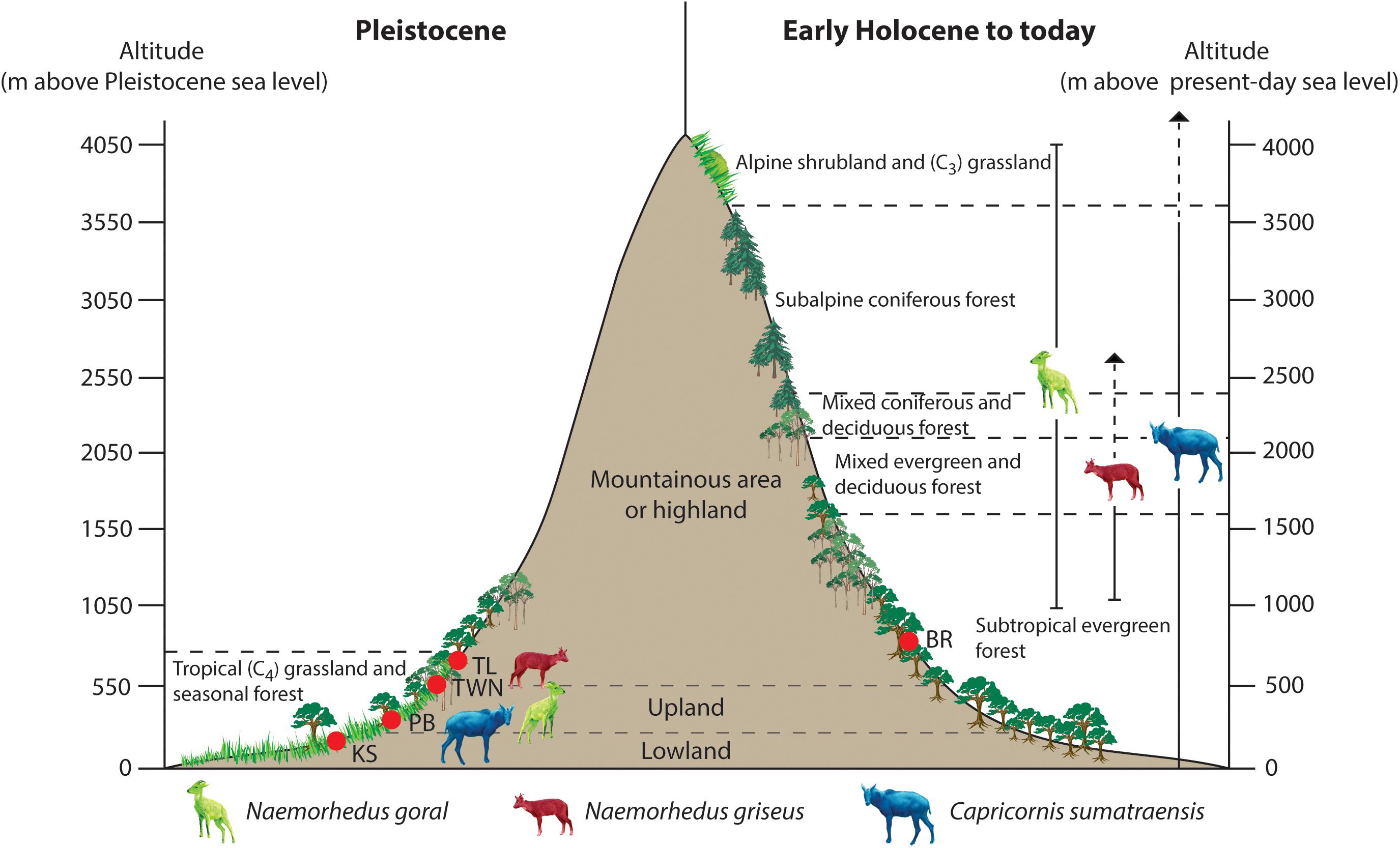
Figure 5. A comparison between Pleistocene and Early Holocene to present-day caprine altitude ranges in relation to major ecosystem distributions in subtropical and tropical regions. For this graphic (as an example), the boundaries of altitudinal vegetation zones are briefly given according to this study for the Pleistocene in Thailand and to Chen et al. (2009, 2012) for the Holocene in Southwest China. The vegetation types and altitudinal zonation may vary along different regions where each caprine taxon is living (i.e., Southeast Asia, Indian Himalayan regions, and Southwest China). Locality abbreviations: PB = Pha Bong, KS = Khok Sung, TWN = Tham Wiman Nakin, TL = Tham Lod Rockshelter, and BR = Ban Rai Rockshelter. The intermediate lowered sea level of 50 m below the present-day level during glacial stages is applied for the Pleistocene context.
Conclusion
Applying a stable isotope approach on tooth enamel of extirpated and extant caprines provides empirical evidence on the diet and habitat widths between Naemorhedus and Capricornis and allows us to better understand their adaptation to modern environments. It is clear that Sumatran serows have been generalist browsers and grazers, unlike Himalayan and Chinese gorals that had a diet specialist (not a specialized C3-plant feeder but C4 grazer prior to the Holocene). In contrast with the traditional view, our study has revealed that both Himalayan and Chinese gorals likely had a preferred open habitat, such as low-elevation grasslands on mountainous areas or lowland floodplain, with a subsistence strategy in more variable climates over long time duration through the Pleistocene. In addition to human activities, the ecological status of these three taxa from what we have observed today is likely a consequence of the habitat contraction in response to the climatic change during the Holocene interglacial. Environmental changes as turning into more closed forest canopies during the Holocene, human impacts on the ecosystems over a long time, effects of predation pressure, and high interspecific competition between these two same-sized gorals advocate the demise of Himalayan gorals in Thailand and likely force the rest of their populations into the refugial stages. As grasses have been major dietary components of both N. goral and N. griseus throughout long-term historical time, this study finally provides an exemplary case of merging paleontological application with conservation biology to guide the future habitat restoration for these two goral species.
Data Availability Statement
All datasets generated for this study are included in the article/Supplementary Material.
Ethics Statement
Ethical review and approval was not required for the animal study because we do not damage the specimens of vertebrates and do not work on the living animals. All the specimens analyzed for this study have been allowed by the curators and/or owners.
Author Contributions
KS, J-JJ, RS, and HB conceived the research project. J-JJ, RS, and YC conducted the field excavations and provided the fossil samples. KS collected the modern samples and was a major investigator for the project. KS and AW identified the fossil material. KS and HB performed and directed the sampling, preparation, and isotopic analysis of the fossil and modern material, and wrote the manuscript. All authors discussed the results and commented on the manuscript.
Funding
For this research, the cost of stable isotope analyses is funded by Georg Forster Research Fellowship (Alexander von Humboldt Foundation). All transportation and living expenses during the sample collection process are supported by MESA RU (Department of Geology, Faculty of Science, Chulalongkorn University) and by Grants for Development of New Faculty Staff (Ratchadaphiseksomphot Endowment Fund, Chulalongkorn University). We would like to express our gratitude for funding supports by the DMR (Bangkok) and the CNRS-TRF (Caenozoic Biodiversity) Program for the fossil expeditions in Pha Bong, Khok Sung, and Tham Wiman Nakin and by the Thailand Science Research and Innovation (TSRI) grant RTA6080001 (The Prehistoric Population and Cultural Dynamics in Highland Pang Mapha Project) for the archaeological excavations in Tham Lod Rockshelter and Ban Rai Rockshelter, all of which make the fossil material available for this study.
Conflict of Interest
The authors declare that the research was conducted in the absence of any commercial or financial relationships that could be construed as a potential conflict of interest.
Acknowledgments
We thank Peter Tung (Senckenberg Centre for Human Evolution and Palaeoenvironment, Tübingen) for his great works in analytical techniques with the mass spectrometer. We are grateful to Noppadon Kitana (CU), Art-Ong Pradatsundarasar (CU), Wachara Sanguansombat (THNHM), Yutthapong Rassamee (THNHM), Loïc Costeur (NMB), Anneke van Heteren (ZSM), and Michael Hiermeier (ZSM) for their curatorial supports and permissions to collect modern isotopic samples at Chulalongkorn University Museum of Natural History (Bangkok, Thailand), Thailand Natural History Museum (Pathum Thani, Thailand), Natural History Museum Basel (Basel, Switzerland), and Zoologische Staatssammlung München (Munich, Germany). We also thank the Department of Mineral Resources (DMR) for allowing us to collect fossil enamel samples from the collections of Pha Bong, Khok Sung, and Tham Wiman Nakin. Finally, we deeply thank two reviewers, MM and SN, for their constructive comments on the manuscript as well as the editor PR for his editorial inputs.
Supplementary Material
The Supplementary Material for this article can be found online at: https://www.frontiersin.org/articles/10.3389/fevo.2020.00067/full#supplementary-material
References
Anwar, M. (1989). Development of A Management Plan for Gray Goral: Lessons from Blackbuck and Cheer Pheasant Reintroduction Attempts. PhD. thesis., University of Utah, Utah (Salt Lake City).
Anwar, M., and Chapman, J. A. (2000). Feeding habits and food of grey goral in the Margalla Hills National Park. Pakistan J. Agric. Res. 16, 28–32.
Archer, M., Bates, H., Hand, S. J., Evans, T., Broome, L., McAllan, B., et al. (2019). The Burramys Project: a conservationist’s reach should exceed history’s grasp, or what is the fossil record for? Philos. Trans. R. Soc. B. 374:20190221. doi: 10.1098/rstb.2019.0221
Ashraf, N., Anwar, M., Hussain, I., Mirza, S. N., Latham, M. C., and Latham, A. D. M. (2015). Habitat use of Himalayan grey goral in relation to livestock grazing in Machiara National Park. Pak. Mammalia 80, 59–70. doi: 10.1515/mammalia-2014-0099
Ashraf, N., Anwar, M., Oli, M. K., Pine, W. E., Sarwar, M., Hussain, I., et al. (2016). Seasonal variation in the diet of the grey goral (Naemorhedus goral) in Machiara National Park (MNP), Azad Jammu and Kashmir, Pakistan. Mammalia 81, 235–244. doi: 10.1515/mammalia-2015-0075
Awasthi, A., Uniyul, S. K., Rawat, G. S., and Sathyakumar, S. (2003). Food plants and feeding habits of Himalayan ungulates. Curr. Sci. 85, 719–723.
Bacon, A.-M., Demeter, F., Duringer, P., Helm, C., Bano, M., Long, V. T., et al. (2008a). The Late Pleistocene Duoi U’Oi cave in northern Vietnam: palaeontology, sedimentology, taphonomy and palaeoenvironments. Quat. Sci. Rev. 27, 1627–1654. doi: 10.1016/j.quascirev.2008.04.017
Bacon, A.-M., Demeter, F., Tougard, C., de Vos, J., Sayavongkhamdy, T., Antoine, P.-O., et al. (2008b). Redécouverte d’une faune pléistocène dans les remplissages karstiques de Tam Hang au Laos: premiers résultats. C. R. Palevol. 7, 277–288. doi: 10.1016/j.crpv.2008.03.009
Bacon, A.-M., Demeter, F., Duringer, P., Patole-Edoumba, E., Sayavongkhamdy, T., Coupey, A.-S., et al. (2012). Les Sites de Tam Hang, Nam Lot et Tam Pà Ling au nord du Laos. Des gisements à vertébrés du Pléistocène aux origines des Hommes modernes. Paris: CNRS.
Bacon, A.-M., Duringer, P., Antoine, P.-O., Demeter, F., Shackelford, L., Sayavongkhamdy, T., et al. (2011). The Middle Pleistocene mammalian fauna from Tam Hang karstic deposit, northern Laos: new data and evolutionary hypothesis. Quat. Int. 245, 315–332. doi: 10.1016/j.quaint.2010.11.024
Bacon, A.-M., Antoine, P.-O., Huong, N. T. M., Westaway, K., Tuan, N. A., Duringer, P., et al. (2018a). A rhinocerotid-dominated megafauna at the MIS6-5 transition: the late Middle Pleistocene Coc Muoi assemblage, Lang Son province, Vietnam. Quat. Sci. Rev. 15, 123–141. doi: 10.1016/j.quascirev.2018.02.017
Bacon, A.-M., Bourgon, N., Dufour, E., Zanolli, C., Duringer, P., Ponche, J.-L., et al. (2018b). Nam lot (MIS 5) and Duoi U’Oi (MIS 4) Southeast Asian sites revisited: zooarchaeological and isotopic evidences. Palaeogeogr. Palaeoclimatol. Palaeoecol. 512, 132–144. doi: 10.1016/j.palaeo.2018.03.034
Bacon, A.-M., Duringer, P., Westaway, K., Joannes-Boyau, R., Zhao, J., Bourgon, N., et al. (2018c). Testing the savannah corridor hypothesis during MIS2: the Boh Dambang hyena site in southern Cambodia. Quat. Int. 464, 417–439. doi: 10.1016/j.quaint.2017.10.047
Bacon, A.-M., Westaway, K., Antoine, P.-O., Duringer, P., Blin, A., Demeter, F., et al. (2015). Late Pleistocene mammalian assemblages of Southeast Asia: new dating, mortality profiles and evolution of the predator–prey relationships in an environmental context. Palaeogeogr. Palaeoclimatol. Palaeoecol. 422, 101–127. doi: 10.1016/j.palaeo.2015.01.011
Bhattacharyya, S., Dawson, D. A., Hipperson, H., and Ishtiaq, F. (2019). A diet rich in C3 plants reveals the sensitivity of an alpine mammal to climate change. Mol. Ecol. 28, 250–265. doi: 10.1111/mec.14842
Biasatti, D., Wang, Y., Gao, F., Xu, Y., and Flynn, L. (2012). Paleoecologies and paleoclimates of Late Cenozoic mammals from Southwest China: evidence from stable carbon and oxygen isotopes. J. Asian Earth Sci. 44, 48–61. doi: 10.1016/j.jseaes.2011.04.013
Birks, H. J. B. (2012). Ecological palaeoecology and conservation biology: controversies, challenges, and compromises. Int. J. Biodivers. Sci. Ecosyst. Serv. Manage. 8, 292–304. doi: 10.1080/21513732.2012.701667
Blasco, F., Bellan, M. F., and Aizpuru, M. (1996). A vegetation map of tropical continental Asia at scale 1.5 million. J. Veg. Sci. 7, 623–634. doi: 10.2307/3236374
Bocherens, H., Hofman-Kamińska, E., Drucker, D. G., Schmölcke, U., and Kowalczyk, R. (2015). European bison as a refugee species? evidence from isotopic data on Early Holocene bison and other large herbivores in Northern Europe. PLoS ONE 10:e0115090. doi: 10.1371/journal.pone.0115090
Bocherens, H., Schrenk, F., Chaimanee, Y., Kullmer, O., Mörike, D., Pushkina, D., et al. (2017). Flexibility of diet and habitat in Pleistocene South Asian mammals: implications for the fate of the giant fossil ape Gigantopithecus. Quat. Int. 434, 148–155. doi: 10.1016/j.quaint.2015.11.059
Bover, P., Llamas, B., Mitchell, K. J., Thomson, V. A., Alcover, J. A., Lalueza-Fox, C., et al. (2019). Unraveling the phylogenetic relationships of the extinct bovid Myotragus balearicus Bate 1909 from the Balearic Islands. Quat. Sci. Rev. 215, 185–195. doi: 10.1016/j.quascirev.2019.05.005
Bryant, J. D., Froelich, P. N., Showers, W. J., and Genna, B. J. (1996). A tale of two quarries: biologic and taphonomic signatures in the oxygen isotope composition of tooth enamel phosphate from modern and miocene equids. PALAIOS 11, 397–408. doi: 10.2307/3515249
Buranapim, N., and Sitasuwa, N. (2014). Behavioral observations of goral (Naemorhedus griseus) in captivity. J. Yala Rajabh. Univ. 9, 73–93.
Buranapim, N., Sitasuwan, N., Kongprempoon, A., Korkusol, K., Siriaroonrat, B., and Kamolnorranath, S. (2014). Reintroduction and behavioral observations of Chinese gorals (Naemorhedus griseus) in natural conditions. Thai J. Vet. Med. 44, 75–83.
Castelló, J. R. (2016). Bovids of the World: Antelopes, Gazelles, Cattle, Goats, Sheep, and Relatives. Oxford: Princeton University Press.
Cavada, N., Havmøller, R. W., Scharff, N., and Rovero, F. (2019). A landscape-scale assessment of tropical mammals reveals the effects of habitat and anthropogenic disturbance on community occupancy. PLoS ONE 14:e0215682. doi: 10.1371/journal.pone.0215682
Cerling, T. E., and Harris, J. M. (1999). Carbon isotope fractionation between diet and bioapatite in ungulate mammals and implications for ecological and paleoecological studies. Oecologia 120, 347–363. doi: 10.1007/s004420050868
Cerling, T. E., Harris, J. M., and MacFadden, B. J. (1997a). “Carbon isotopes, diets of North American equids, and the evolution of North American C4 grasslands,” in Stable Isotopes and the Integration of Biological, Ecological, and Geochemical Processes, eds H. Griffiths, D. Robinson, and P. van Gardingen (Oxford: Bios Scientific Publishers), 363–379.
Cerling, T. E., Harris, J. M., MacFadden, B. J., Leakey, M. G., Quade, J., Eisenmann, V., et al. (1997b). Global vegetation change through the Miocene/Pliocene boundary. Nature 389, 153–158. doi: 10.1038/38229
Cerling, T. E., Hart, J. A., and Hart, T. B. (2004). Stable isotope ecology in the Ituri Forest. Oecologia 138, 5–12. doi: 10.1007/s00442-003-1375-4
Chen, G. J., Wang, W., Mo, J. Y., Huang, Z. T., Tian, F., and Huang, W. W. (2002). Pleistocene vertebrate fauna from Wuyun Cave of Tiandong County, Guangxi. Vert. PalAs 40, 42–51.
Chen, W., Hu, J. C., and Lu, X. (2009). Habitat use and separation between the Chinese serow (Capricornis milneedwardsi) and the Chinese goral (Naemorhedus griseus) in winter. Mammalia 73, 249–252. doi: 10.1515/MAMM.2009.035
Chen, W., Wu, Q. G., Hu, J. C., Lu, X., and You, Z. Q. (2012). Seasonal habitat use of Chinese goral (Naemorhedus griseus) in a subtropical forest. Russ. J. Ecol. 43, 256–260. doi: 10.1134/S106741361203006X
Clementz, M. T., Fox-Dobbs, K., Wheatley, P. V., Koch, P. L., and Doak, D. F. (2009). Revisiting old bones: coupled carbon isotope analysis of bioapatite and collagen as an ecological and palaeoecological tool. Geol. J. 44, 605–620. doi: 10.1002/gj.1173
Colbert, E. H., and Hooijer, D. H. (1953). Pleistocene mammals from the limestone fissures of Szechwan, China. Bull. Am. Mus. Nat. Hist. 102, 1–134.
Crowley, B. E., Godfrey, L. R., Guilderson, T. P., Zermeńo, P., Koch, P. L., and Dominy, N. J. (2012). Extinction and ecological retreat in a community of primates. Proc. R. Soc. B 279, 3597–3605. doi: 10.1098/rspb.2012.0727
de Vos, J. (1983). The Pongo faunas from Java and Sumatra and their significance for biostratigraphical and paleoecological interpretations. Proc. K. Ned. Akad. Wet. B 86, 417–425.
DeNiro, M. J., and Epstein, S. (1978). Influence of diet on the distribution of carbon isotopes in animals. Geochim. Cosmochim. Acta 42, 495–506. doi: 10.1016/0016-7037(78)90199-0
Dobremez, J. F. (1976). Le Népal: Ecologie et Biogéographie, Editions de la Recherche Scientifique. Paris: CNRS.
Domingo, M. S., Domingo, L., Badgley, C., Sanisidro, O., and Morales, J. (2013). Resource partitioning among top predators in a Miocene food web. Proc. R. Soc. B 280, 20122138. doi: 10.1098/rspb.2012.2138
Dou, H., Zhang, Y., and Feng, L. (2016). Complete mitochondrial genome of the Himalayan serow (Capricornis thar) and its phylogenetic status within the genus Capricornis. Biochem. Syst. Ecol. 65, 115–123. doi: 10.1016/j.bse.2016.02.018
Duckworth, J. W., and MacKinnon, J. (2008). Data from: Naemorhedus Goral. The IUCN Red List of Threatened Species. http://dx.doi.org/10.2305/IUCN.UK.2008.RLTS.T14296A4430073.en.
Duckworth, J. W., Steinmetz, R., and Chaiyarat, R. (2008a). Data from: Naemorhedus griseus. The IUCN Red List of Threatened Species. http://dx.doi.org/10.2305/IUCN.UK.2008.RLTS.T14303A4430834.en.
Duckworth, J. W., Steinmetz, R., and MacKinnon, J. (2008b). Data from: Capricornis sumatraensis. The IUCN Red List of Threatened Species. http://dx.doi.org/10.2305/IUCN.UK.2008.RLTS.T3812A10099434.en.
Duckworth, J. W., Steinmetz, R., and Pattanavibool, A. (2008c). Data from: Capricornis milneedwardsii. The IUCN Red List of Threatened Species. http://dx.doi.org/10.2305/IUCN.UK.2008.RLTS.T3814A10101852.en.
Duckworth, J. W., and Zaw, T. (2008). Data from: Capricornis Rubidus. The IUCN Red List of Threatened Species. http://dx.doi.org/10.2305/IUCN.UK.2008.RLTS.T3815A10102774.en.
Duval, M., Fang, F., Suraprasit, K., Jaeger, J.-J., Benammi, M., Chaimanee, Y., et al. (2019). Direct ESR dating of the Pleistocene vertebrate assemblage from Khok Sung locality, Nakhon Ratchasima Province, Northeast Thailand. Palaeontol. Electron. 22, 1–25. doi: 10.26879/941
Ecker, M., Bocherens, H., Julien, M.-A., Rivals, F., Raynal, J.-P., and Moncel, M.-H. (2013). Middle Pleistocene ecology and Neanderthal subsistence: insights from stable isotope analyses in Payre (Ardèche, southeastern France). J. Hum. Evol. 65, 363–373. doi: 10.1016/j.jhevol.2013.06.013
Esposito, M., Chaimanee, Y., Jaeger, J.-J., and Reyss, J.-L. (1998). Datation des concrétions carbonatées de la Grotte du Serpent (Thaïlande) par la méthode Th/U. C. R. Acad. Sci. Ser. IIA 326, 603–608. doi: 10.1016/S1251-8050(98)80250-4
Esposito, M., Reyss, J.-L., Chaimanee, Y., and Jaeger, J.-J. (2002). U-series dating of fossil teeth and carbonates from Snake Cave, Thailand. J. Archaeol. Sci. 29, 341–349. doi: 10.1006/jasc.2002.0718
Fakhar-I-Abbas, F., Akhtar, T., and Mian, A. (2008). Food and feeding preferences of Himalayan gray goral (Naemorhedus goral bedfordi) in Pakistan and Azad Jammu and Kashmir. Zoo Biol. 27, 371–380. doi: 10.1002/zoo.20202
Fakhar-I-Abbas, F., Akhtar, T., and Mian, A. (2009). Phytosociological analysis within the range of grey goral in Pakistan and Azad Kashmir. Pak. J. Bot. 41, 667–682.
Fakhar-I-Abbas, F., Ruckstuhl, K. E., Mian, A., Akhtar, T., and Rooney, T. P. (2012). Distribution, population size, and structure of Himalayan grey goral Naemorhedus goral bedfordi (Cetartiodactyla: Bovidae) in Pakistan. Mammalia 76, 143–147. doi: 10.1515/mammalia-2011-0038
Feranec, R. S., and MacFadden, B. J. (2006). Isotopic discrimination of resource partitioning among ungulates in C3-dominated communities from the Miocene of Florida and California. Paleobiology 32, 191–205. doi: 10.1666/05006.1
Filoux, A., Wattanapituksakul, A., Lespes, C., and Thongcharoenchaikit, C. (2015). A Pleistocene mammal assemblage containing Ailuropoda and Pongo from Tham Prakai Phet cave, Chaiyaphum Province, Thailand. Geobios 48, 341–349. doi: 10.1016/j.geobios.2015.07.003
Fricke, H. C., and O’Neil, J. R. (1996). Inter- and intra-tooth variation in the oxygen isotope composition of mammalian tooth enamel phosphate: implications for palaeoclimatological and palaeobiological research. Palaeogeogr. Palaeoclimatol. Palaeoecol. 126, 91–99. doi: 10.1016/S0031-0182(96)00072-7
Friedli, H., Lötscher, H., Oeschger, H., Siegenthaler, U., and Stauffer, B. (1986). Ice core record of the 13C/12C ratio of atmospheric CO2 in the past two centuries. Nature 324, 237–238. doi: 10.1038/324237a0
Galy, V., François, L., France-Lanord, C., Faure, P., Kudrass, H., Palhol, F., et al. (2008). C4 plants decline in the Himalayan basin since the Last Glacial Maximum. Quat. Sci. Rev. 27, 1396–1409. doi: 10.1016/j.quascirev.2008.04.005
Groves, C. P. (1985). Plio-Pleistocene mammals in Island Southeast Asia. Modern Quat. Res. SE Asia 9, 43–54.
Grubb, P. (2005). “Order artiodactyla,” in Mammal Species of the World, 3rd Edn, eds D. E. Wilson and D. M. Reeder (Baltimore: The Johns Hopkins University Press), 637–722.
Han, D., and Xu, C. (1985). “Pleistocene mammalian faunas of China,” in Palaeoanthropology and Palaeolithic Archaeology in the People’s Republic of China, eds R. Wu and J. Olsen (Orlando: Academic Press), 267–287.
Higgins, P., and MacFadden, B. J. (2004). “Amount Effect” recorded in oxygen isotopes of Late Glacial horse (Equus) and bison (Bison) teeth from the Sonoran and Chihuahuan deserts, southwestern United States. Palaeogeogr. Palaeoclimatol. Palaeoecol. 206, 337–353. doi: 10.1016/j.palaeo.2004.01.011
Hofman-Kamińska, E., Bocherens, H., Drucker, D. G., Fyfe, R. M., Gumiński, W., Makowiecki, D., et al. (2019). Adapt or die—Response of large herbivores to environmental changes in Europe during the Holocene. Global Change Biol. 25, 2915–2930. doi: 10.1111/gcb.14733
Hooijer, D. A. (1958). Fossil bovidae from the malay archipelago and the Punjab. Zool. Verh. Mus. Leiden 38, 1–112.
Hu, C. K., and Qi, T. (1978). Gongwangling Pleistocene mammalian fauna of Lantian, Shaanxi. Palaeontol. Sin. 155, 1–64.
Ibrahim, Y. K., Tshen, L. T., Westaway, K. E., Cranbrook, E., Humphrey, L., Muhammad, R. F., et al. (2013). First discovery of Pleistocene orangutan (Pongo sp.) fossils in Peninsular Malaysia: biogeographic and paleoenvironmental implications. J. Hum. Evol. 65, 770–797. doi: 10.1016/j.jhevol.2013.09.005
Junaid, M., Ahmad, F., Saxena, R. C., and Bansal, S. K. (2012). Botanical composition determination of Goral Naemorhedus goral (Artiodactyla: Bovidae): goral rescue centre, Pahalgam, Jammu & Kashmir, India. Eur. J. Zool. Res. 1, 99–104.
Jürgensen, J., Drucker, D. G., Stuart, A. J., Schneider, M., Buuveibaatar, B., and Bocherens, H. (2017). Diet and habitat of the saiga antelope during the late Quaternary using stable carbon and nitrogen isotope ratios. Quat. Sci. Rev. 160, 150–161. doi: 10.1016/j.quascirev.2017.01.022
Kahlke, H. D. (1961). On the complex of the Stegodon-Ailuropoda-fauna of southern China and the chronological position of Gigantopithecus blacki von Koenigswald. Vert. PalAs 1, 83–108.
Kaiser, T. M., Müller, D. W. H., Fortelius, M., Schulz, E., Codron, D., and Clauss, M. (2013). Hypsodonty and tooth facet development in relation to diet and habitat in herbivorous ungulates: implications for understanding tooth wear. Mamm. Rev. 43, 34–46. doi: 10.1111/j.1365-2907.2011.00203.x
Keeling, C. D., Mook, W. G., and Tans, P. P. (1979). Recent trends in the 13C/12C ratio of atmospheric carbon dioxide. Nature 277, 121–123. doi: 10.1038/277121a0
Kershaw, A. P., Penny, D., van der Kaars, S., Anshari, G., Thamotherampillai, A. (2001). “Vegetation and climate in lowland Southeast Asia at the Last Glacial Maximum,” in Faunal and Floral Migrations and Evolution in SE Asia–Australasia, eds Metcalfe, I., Jeremy, M. B. S., Morwood, M., and Davidson, I. (Lisse: Balkema), 227–236.
Khaokhiew, C. (2004). Geoarchaeology of Tham Lod Rockshelter, Changwat Mae Hong Son, Northern Thailand. MSc. Thesis., Chulalongkorn University, Thailand (Bangkok).
Kohn, M. J. (1996). Predicting animal δ18O: Accounting for diet and physiological adaptation. Geochim. Cosmochim. Acta 60, 4811–4829. doi: 10.1016/S0016-7037(96)00240-2
Kohn, M. J., McKay, M. P., and Knight, J. L. (2005). Dining in the Pleistocene—Who’s on the menu? Geology 33, 649–652. doi: 10.1130/G21476AR.1
Kuemmerle, T., Hickler, T., Olofsson, J., Schurgers, G., and Radeloff, V. C. (2012). Refugee species: which historic baseline should inform conservation planning? Divers. Distrib. 18, 1258–1261. doi: 10.1111/ddi.12013
Lea, J. M., Kerley, G. I., Hrabar, H., Barry, T. J., and Shultz, S. (2016). Recognition and management of ecological refugees: a case study of the Cape mountain zebra. Biol. Conserv. 203, 207–215. doi: 10.1016/j.biocon.2016.09.017
Lekagul, B., and McNeely, J. A. (1988). Mammals of Thailand: Association for the Conservation of Wildlife. Bangkok: Kurusapa Ladprao Press.
Li, Y., and Wen, B. (1986). Guanyindong: A Lower Paleolithic Site at Qianxi County, Guizhou Province. Beijing: Cultural Relics Publishing House.
Long, V. T., de Vos, J., and Ciochon, R. S. (1996). The fossil mammal fauna of the Lang Trang caves, Vietnam, compared with Southeast Asian fossil and recent mammal faunas: the geographical implications. Bull. Indo-Pacific Prehist. Assoc. 14, 101–109.
Louys, J. (2012). “The future of mammals in Southeast Asia: Conservation insights from the fossil record,” in Paleontology in Ecology and Conservation, Springer Earth System Sciences, ed. J. Louys (Berlin: Springer), 227–238. doi: 10.1007/978-3-642-25038-5_11
Lovari, S. (1997). “Thailand,” in Wild Sheep and Goats and Their Relatives: Status Survey and Conservation Action Plan for Caprinae, eds D. M. Shackleton (Cambridge: Information Press), 287–291.
MacFadden, B. J. (1998). Tale of two Rhinos: isotopic ecology, paleodiet, and niche differentiation of Aphelops and Teloceras from the Florida Neogene. Paleobiology 24, 274–286.
MacFadden, B. J., Cerling, T. E., Harris, J. M., and Prado, J. (1999). Ancient latitudinal gradients of C3/C4 grasses interpreted from stable isotopes of New World Pleistocene horse (Equus) teeth. Global Ecol. Biogeogr. 8, 137–149. doi: 10.1046/j.1466-822X.1999.00127.x
MacFadden, B. J., and Higgins, P. (2004). Ancient ecology of 15-million-year-old browsing mammals within C3 plant communities from Panama. Oecologia 140, 169–182. doi: 10.1007/s00442-004-1571-x
Marino, B. D., and McElroy, M. B. (1991). Isotopic composition of atmospheric CO2 inferred from carbon in C4 plant cellulose. Nature 349, 127–131. doi: 10.1038/349127a0
Marwick, B., and Gagan, M. K. (2011). Late Pleistocene monsoon variability in northwest Thailand: an oxygen isotope sequence from the bivalve Margaritanopsis laosensis excavated in Mae Hong Son province. Quat. Sci. Rev. 30, 3088–3098. doi: 10.1016/j.quascirev.2011.07.007
Mendoza, M., and Palmqvist, P. (2008). Hypsodonty in ungulates: an adaptation for grass consumption or for foraging in open habitat. J. Zool. 274, 134–142. doi: 10.1111/j.1469-7998.2007.00365.x
Mori, E., Nerva, L., and Lovari, S. (2019). Reclassification of the serows and gorals: the end of a neverending story? Mamm. Rev. 49, 256–262. doi: 10.1111/mam.12154
Morley, R. J. (2012). “A review of the Cenozoic palaeoclimate history of Southeast Asia,” in Biotic Evolution and Environmental Change in Southeast Asia, eds D. Gower, K. G. Johnson, B. R. Rosen, J. Richardson, L. Rüber, and S. T. Williams (Cambridge: Cambridge University Press), 79–114. doi: 10.1017/cbo9780511735882.006
Nowak, R. M. (1999). Walker’s Mammals of the World, 6th Edn, Vol. 2. London: The John Hopkins University Press.
Olsen, J. W., and Ciochon, R. L. (1990). A review of evidence for postulated Middle Pleistocene occupations in Viet Nam. J. Hum. Evol. 19, 761–788. doi: 10.1016/0047-2484(90)90020-C
Passey, B. H., Robinson, T. F., Ayliffe, L. K., Cerling, T. E., Sponheimer, M., Dearing, M. D., et al. (2005). Carbon isotope fractionation between diet, breath CO2, and bioapatite in different mammals. J. Archaeol. Sci. 32, 1459–1470. doi: 10.1016/j.jas.2005.03.015
Perveen, F., and Khan, A. (2013). Migratory status of the goral, Naemorhedus goral (Hardwicke) (Artiodactyla: Bovidae) in Kohistan, Pakistan. Int. J. Biodivers. Conserv. 5, 671–677.
Pushkina, D., Bocherens, H., Chaimanee, Y., and Jaeger, J.-J. (2010). Stable carbon isotope reconstructions of diet and paleoenvironment from the late Middle Pleistocene Snake Cave in Northeastern Thailand. Naturwissenschaften 97, 299–309. doi: 10.1007/s00114-009-0642-6
Raia, P., Passaro, F., Fulgione, D., and Carotenuto, F. (2012). Habitat tracking, stasis and survival in Neogene large mammals. Biol. Lett. 8, 64–66. doi: 10.1098/rsbl.2011.0613
Rink, W. J., Wei, W., Bekken, D., and Jones, H. L. (2008). Geochronology of Ailuropoda–Stegodon fauna and Gigantopithecus in Guangxi province, southern China. Quat. Res. 69, 377–387. doi: 10.1016/j.yqres.2008.02.008
Rovero, F., Martin, E., Rosa, M., Ahumada, J. A., and Spitale, D. (2014). Estimating species richness and modelling habitat preferences of tropical forest mammals from camera trap data. PLoS ONE 9:e103300. doi: 10.1371/journal.pone.0103300
Shakeel, U., Minhas, R. A., Awan, M. S., Bibi, S. S., and Iftikhar, N. (2015). Conservation status of himalayan grey goral (Naemorhedus goral bedfordi Hardwicke, 1825) in Machiara National Park, Azad Jammu and Kashmir, Pakistan. Pak. J. Zool. 47, 1297–1304.
Shoocongdej, R. (2003). “Paleoenvironment during Late Pleistocene to Late Holocene on highland in Pang Mapha distinct, Mae Hong Son province,” in Proceedings of the Conference on People, Culture, and Paleoenvironment in Highland Pang Mapha, Mae Hong Son Province, ed. R. Shoocongdej (Bangkok: Silpakorn University), 292–317.
Shoocongdej, R. (2006). “Late Pleistocene activities at the Tham Lod Rockshelter in highland Pang Mapha, Mae Hong Son province, northwestern Thailand,” in Uncovering Southeast Asia’s Past: Selected Papers from the 10th International Conference of the European Association of Southeast Asian Archaeologists, eds E. A. Bacus, I C. Glover, and V. Pigott (Singapore: NUS press), 22–37.
Shoocongdej, R., Phumijumnong, N., Chintakanon, K., Pureepatpong, N., Hoontrakul, U., and Treerayapiwat, C. (2007). Final report of Highland Archaeology Project in Pang Mapha District, Mae Hong Son Province Phase 2, Vol. 2. Bangkok: Thailand Research Fund (TRF).
Stacklyn, S., Wang, Y., Jin, C., Wang, Y., Sun, F., Zhang, C., et al. (2017). Carbon and oxygen isotopic evidence for diets, environments and niche differentiation of early Pleistocene pandas and associated mammals in South China. Palaeogeogr. Palaeoclimatol. Palaeoecol. 468, 351–361. doi: 10.1016/j.palaeo.2016.12.015
Suraprasit, K., Bocherens, H., Chaimanee, Y., Panha, S., and Jaeger, J.-J. (2018). Late Middle Pleistocene ecology and climate in Northeastern Thailand inferred from the stable isotope analysis of Khok Sung herbivore tooth enamel and the land mammal cenogram. Quat. Sci. Rev. 193, 24–42. doi: 10.1016/j.quascirev.2018.06.004
Suraprasit, K., Jaeger, J.-J., Chaimanee, Y., Benammi, M., Chavasseau, O., Yamee, C., et al. (2015). A complete skull of Crocuta crocuta ultima indicates a late Middle Pleistocene age for the Khok Sung (northeastern Thailand) vertebrate fauna. Quat. Int. 374, 34–45. doi: 10.1016/j.quaint.2014.12.062
Suraprasit, K., Jaeger, J.-J., Chaimanee, Y., Chavasseau, O., Yamee, C., Tian, P., et al. (2016). The Middle Pleistocene vertebrate fauna from Khok Sung (Nakhon Ratchasima, Thailand): biochronological and paleobiogeographical implications. ZooKeys 613, 1–157. doi: 10.3897/zookeys.613.8309
Suraprasit, K., Jongautchariyakul, S., Yamee, C., Pothichaiya, C., and Bocherens, H. (2019). New fossil and isotope evidence for the Pleistocene zoogeographic transition and hypothesized savanna corridor in peninsular Thailand. Quat. Sci. Rev. 221, 105861. doi: 10.1016/j.quascirev.2019.105861
Thapa, A., Thapa, S., and Poudel, B. S. (2011). Habitat and distribution of goral (Naemorhedus goral) in Mahabharat goral wildlife conservation area, Nawalparasi, Western Nepal. Nepal J. For. 14, 1–12.
Thuc, P. D., Hieu, D. N., Thap, H. V., Van, V. H., and Khu, N. X. (2012). Notes on food of Capricornis milneedwardsii in the Cat Ba Archipelago, Hai Phong, Vietnam. TAP CHí SINH HQC 34, 432–440.
Tougard, C. (1998). Les faunes de Grands mammifères du Pléistocène Moyen Terminal de Thaïlande dans leur cadre Phylogénétique, paléoécologique et Biochronologique. PhD. Thesis., University of Montpellier II, Montpellier.
Tougard, C. (2001). Biogeography and migration routes of large mammal faunas in South–East Asia during the Late Middle Pleistocene: focus on the fossil and extant faunas from Thailand. Palaeogeogr. Palaeoclimatol. Palaeoecol. 168, 337–358. doi: 10.1016/S0031-0182(00)00243-1
Treerayapiwat, C. (2005). Patterns of habitation and burial activity in the Ban Rai Rock Shelter, Northwestern Thailand. Asian Perspect. 44, 231–245. doi: 10.1353/asi.2005.0001
von Koenigswald, W. (2011). Diversity of hypsodont teeth in mammalian dentitions–construction and classification. Palaeontogr. Abteil. A 294, 63–94. doi: 10.1127/pala/294/2011/63
Wang, W., Potts, R., Baoyin, Y., Huang, W., Cheng, H., Edwards, R. L., et al. (2007). Sequence of mammalian fossils, including hominoid teeth, from the Bubing Basin caves, South China. J. Hum. Evol. 52, 370–379. doi: 10.1016/j.jhevol.2006.10.003
Wattanapituksakul, A., Filoux, A., Amphansri, A., and Tumpeesuwan, S. (2018). Late Pleistocene Caprinae assemblages of Tham Lod Rockshelter (Mae Hong Son Province, Northwest Thailand). Quat. Int. 493, 212–226. doi: 10.1016/j.quaint.2018.06.003
Xue, X., and Zhang, Y. (1991). “Quaternary mammalian fossils and fossil human beings,” in The Quaternary of China, eds Z. H. Zhang and S. X. Shao (Beijing: China Ocean Press), 307–374.
Zeitoun, V., Lenoble, A., Laudet, F., Thompson, J., Rink, W. J., Mallye, J.-B., et al. (2010). The Cave of the Monk (Ban Fa Suai, Chiang Dao wildlife sanctuary, northern Thailand). Quat. Int. 220, 160–173. doi: 10.1016/j.quaint.2009.11.022
Keywords: serow, goral, refugee, bulk carbon isotope, paleoecology, Quaternary, Southeast Asia
Citation: Suraprasit K, Jaeger J-J, Shoocongdej R, Chaimanee Y, Wattanapituksakul A and Bocherens H (2020) Long-Term Isotope Evidence on the Diet and Habitat Breadth of Pleistocene to Holocene Caprines in Thailand: Implications for the Extirpation and Conservation of Himalayan Gorals. Front. Ecol. Evol. 8:67. doi: 10.3389/fevo.2020.00067
Received: 03 January 2020; Accepted: 02 March 2020;
Published: 21 April 2020.
Edited by:
Pasquale Raia, University of Naples Federico II, ItalyReviewed by:
Marina Melchionna, University of Naples Federico II, ItalySherry Nelson, The University of New Mexico, United States
Copyright © 2020 Suraprasit, Jaeger, Shoocongdej, Chaimanee, Wattanapituksakul and Bocherens. This is an open-access article distributed under the terms of the Creative Commons Attribution License (CC BY). The use, distribution or reproduction in other forums is permitted, provided the original author(s) and the copyright owner(s) are credited and that the original publication in this journal is cited, in accordance with accepted academic practice. No use, distribution or reproduction is permitted which does not comply with these terms.
*Correspondence: Kantapon Suraprasit, c3VyYXByYXNpdEBnbWFpbC5jb20=