- 1Environmental & Life Sciences Graduate Program, Trent University, Peterborough, ON, Canada
- 2Ontario Ministry of Natural Resources and Forestry, Peterborough, ON, Canada
Squirrels (Sciuridae) are a diverse group in behavior, morphology, and ecology. This variation is typified by the wide range of vocalizations spanning ground squirrels (Marmotini and Xerini), tree squirrels (Callosciurinae and Sciurini), and flying squirrels (Pteromyini). Squirrels produce calls that range in frequency, modulation, and function, with a complex set of social calls occurring across the family. We review the history of recording methods used in the development of squirrel vocalization repertoires, with emphasis on how the ecology and methodology impact the frequency values reported. The fundamental (F0 – the mean frequency of the fundamental harmonic), dominant (FDom – the frequency of maximum energy or amplitude), minimum (FMin – the minimum frequency of the fundamental harmonic), maximum (FMax – the maximum frequency of the dominant harmonic), and highest harmonic (FHarm – the mean frequency of the highest visible harmonic) frequencies were considered against popular hypotheses that have attempted to explain the evolution of vocal frequency characteristics in terrestrial mammals. These hypotheses include body size, predator avoidance, habitat type, and diel activity pattern. Phylogenetic generalized least squared modeling revealed that body mass and the frequency limits of the methods were the strongest drivers of high-frequency communication. Consistent with popular hypotheses, social squirrels exhibited a broader range of F0, FDom, and FMax than solitary squirrels while habitat openness promoted higher FDom and FHarm. Additionally, nocturnality was significantly associated with higher F0, FDom, and FMax, suggesting that flying squirrels, the only nocturnal squirrels, commonly use high-frequency acoustic signals, a finding that merits further investigation. In conclusion, our review provides a unique insight into the role of behavioral ecology on vocal repertoires and the importance of accurate equipment selection for sampling across a diverse taxon.
Introduction
Mammalian bioacoustics is an expansive field in which biology, physics, and psychology are used to study the taxonomic diversity of signalers and interpret the complexity of vocal communication. Over the last century or so, the bioacoustic community has seen the development, implementation, and specialization of their research technology. Ever-improving recording devices have allowed advancements from phonetic descriptions of non-human animal calls to the first spectrographic visualizations to the development of software allowing quantitative analysis of calls in minute detail (Terhune, 2011). While phonetic descriptions of the calls served an important purpose at one point in time, the recording of calls provided the first opportunity for a detailed comparison of the differences between species and the evolution of vocal production in mammals through spectrographic analyses. The first acoustic spectrograph was developed in 1946 to study human speech, though the authors suggested that this new device could be used to study environmental sounds, musical instruments and orchestral composition, and potentially animal sounds under the right conditions (Koenig et al., 1946).
Spectrographs were, and continue to be, important because they allowed researchers to study the shape and structure of the sounds being produced, but they also allowed the visualization of calls that cannot be heard by the human ear. Humans are limited to a hearing frequency range of about 20 Hz to a maximum of 20 kHz, though 15–18 kHz is the cut-off for the average human (Masterton et al., 1969). Given that it is difficult for even the best human ears to hear sounds near the upper limit (Masterton et al., 1969), some have argued that 15 kHz should be the cut-off for differentiating between the audible range (what can be heard by humans) and the ultrasonic range (calls that exceed the upper limit of human hearing). However, most publications describe 20 kHz, the absolute maximum of human hearing, as the frequency at which calls can be described as ultrasonic.
Ultrasonic vocalizations (USVs), calls that completely lie in the ultrasonic range (>20 kHz herein), were first studied in cetaceans and bats that had been observed navigating and hunting without visual cues. USVs were first recorded with the aid of frequency-altering heterodynes in bats (Griffin and Galambos, 1941) and were first visualized in porpoises (Wood, 1952). Bats and cetaceans largely remained the focus of ultrasonic studies for decades, due in particular to interest in echolocation. The intensive echolocation focus has likely overshadowed ultrasound-production in many other wild species such as shrews (Blarina and Sorex: Gould et al., 1964; Sorex: Tomasi, 1979), singing mice (Baiomys and Scotinomys: Miller and Engstrom, 2007; Scotinomys: Fernández-Vargas et al., 2011), and even several species of squirrel (Urocitellus richardsonii: Wilson and Hare, 2004; Glaucomys: Murrant et al., 2013). Additionally, the widespread availability of lab mice and rats has led to many studies investigating the function and biomechanical processes involved with USV production. The study of USV production in these rodents has been quite active since the early 1970s (Oswalt and Meier, 1975; Geyer and Barfield, 1978; Geyer et al., 1978; Hofer and Shair, 1978, 1980; Corrigan and Flannelly, 1979; Geyer, 1979; Smith, 1979), leading to a wealth of publications, but also, along with bats, a taxonomic bias in publications about ultrasound use. While still crucial in understanding the physiological and cognitive processes associated with USVs, captive rats and mice have been isolated from natural processes for generations and are not known to produce well-studied wild calls such as predator alarm calls (Blanchard et al., 1991; Hahn and Lavooy, 2005; Sirotin et al., 2014; Kalcounis-Rueppell et al., 2018).
Ultrasound can be a useful communication tool because the short sound waves are highly directional and are not readily heard by most predatory birds, reptiles, and amphibians (Knudsen, 1981; Yamazaki et al., 2004). It has been proposed that the main drivers of ultrasound use for all terrestrial vertebrates are body size, predator avoidance, and increasing the signal-to-noise ratio (Arch and Narins, 2008). Several studies support the hypothesis that body size has a strong relationship to the frequency of many vocalizing animals (avian mass: Ryan and Brenowitz, 1985; mammalian mass: Charlton and Reby, 2016; Martin et al., 2016; mass of mole rats: [Cryptomys, Heterocephalus, Spalax], Credner et al., 1997; length of ground squirrels: Spermophilus, Nikol’skii, 2017). The mass-call frequency relationship is influenced directly by the physical mechanisms of vocal production (i.e., lung capacity, larynx size) and through indirect effects like species ecology (e.g., larger animals using different environments than smaller animals; Ryan and Brenowitz, 1985). In contrast, predator-avoidance is more difficult to quantify and this hypothesis seems to be more supported by anecdotal evidence of vocal shifts toward ultrasonic frequencies when animals are in the presence of predatory birds (Kotler, 1984; Tamura and Yong, 1993; Digweed and Rendall, 2009). Finally, the signal-to-noise ratio hypothesis has been supported by only a few examples, such as the Kihansi spray toad (Nectophyrynoides asperginis) which has shifted all vocal production to USVs to reduce interference from nearby roaring, low-frequency waterfalls (Arch et al., 2011). Similarly, the acoustic adaptation hypothesis, a version of the signal-to-noise idea that focuses on sound propagation relative to environmental characteristics (e.g., open versus closed) instead of ambient sound, postulates that higher frequencies will be employed in open habitats where the shorter sound waves are less likely to be attenuated by the environment before reaching the receiver (Ey and Fischer, 2009). In contrast to the acoustic adaptation hypothesis, it has also been shown that some mammals inhabiting forested habitats have improved hearing sensitivities to continue to use high-frequency calls in closed habitats (Charlton et al., 2019).
Our review will focus on literature covering the recording and reporting of the highly vocal family, Sciuridae. With spectrographic reports of squirrel vocalizations beginning in the 1960s, squirrel genera have been well sampled, and many vocal libraries have been developed and updated with improving technologies. We apply popular and novel hypotheses related to vocal characteristics (Table 1) to observe which behavioral and ecological traits drive frequency use in squirrels. We also challenge the currently established repertoires in light of new reports of USVs in flying squirrels and call for future research to employ equipment that is intended for non-human animals, and not subject to the limitations of equipment designed for use with humans.
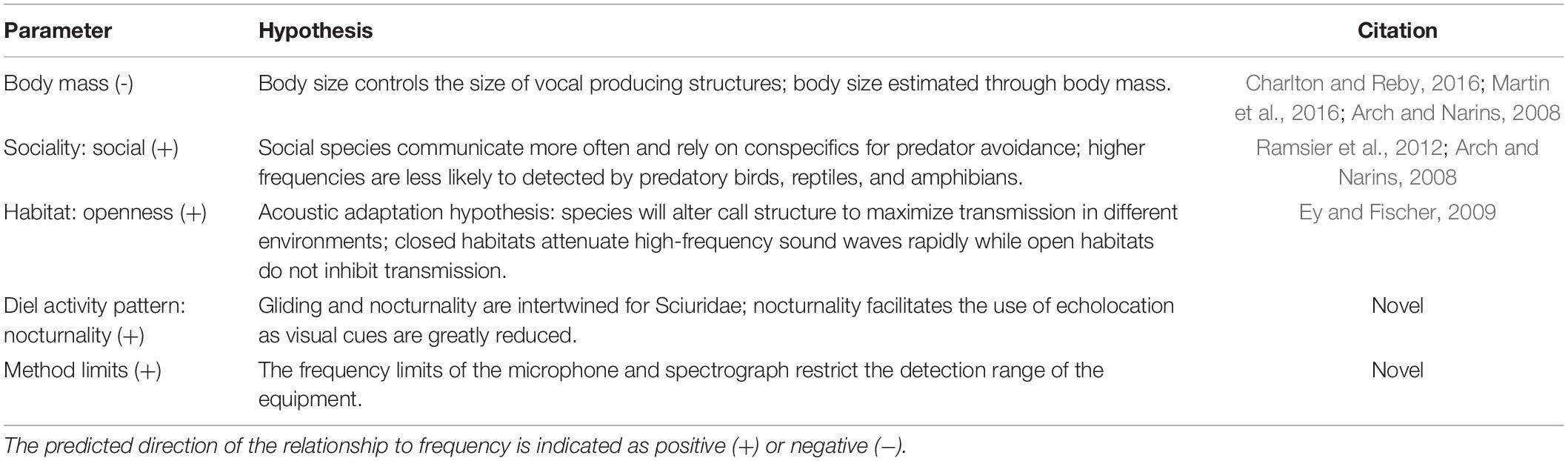
Table 1. Summary of parameters used in the phylogenetic least square (PGLS) analyses and how they relate to popular or novel vocal range hypotheses.
Squirrel Vocalizations – A Short History
The first published spectrographic analyses of squirrels appeared in 1966; the authors used these visualizations to describe a variety of calls of the Uinta ground squirrel (Urocitellus armatus; Balph and Balph, 1966) and the yellow-bellied (Marmota flaviventris) and hoary marmots (M. caligata; Waring, 1966). While the authors published separately, they used the same equipment to record the squirrels: a unidirectional microphone with a relatively narrow frequency bandwidth (Electrovoice-644 Sound Spot, frequency response of ∼0.05–12 kHz; Balph and Balph, 1966; Waring, 1966) attached to a relatively large portable tape recorder. Waring (1966) used a W-610-EV battery-operated tape-recorder (weight ∼7.7 kg; Amplifier Corporation of America, 1959) whereas Balph and Balph (1966) used an 11.8 kg Wollensak T 1700 tape recorder (Revere-Wollsenak Division, 2010). The fundamental frequency (F0 – the vibration frequency of the vocal folds which is determined by the shape of the larynx as well as airflow; Fitch et al., 2002; Charlton and Reby, 2016; Riede et al., 2017) and the dominant frequency (FDom – the frequency with the maximum amplitude or energy; Figure 1) of the calls produced by the ground squirrels and marmots were captured within this narrow frequency range as demonstrated on the spectrographs. However, Waring noted at least one call produced by the yellow-bellied marmot had strong harmonics (resonant frequencies produced at intervals above F0) exceeding 40 kHz, well beyond the dynamic range of the microphone and only detectable with a heterodyne. The dynamic range of the recording microphone is limited by the physical shape of the transducer; this element vibrates when sound is produced, translating the physical sound wave into an electronic signal (Obrist et al., 2010). Similarly, heterodynes (handheld ultrasonic detectors that transpose USVs down to the human hearing range so that researchers can listen to USVs in real-time) can detect a broad range of frequencies with a specialized transducer. However, the transposer (which translates the vibration into an audible sound) is restricted to a narrow bandwidth and the researcher must tune the transducer to listen to different frequencies (Ahlén, 2004).
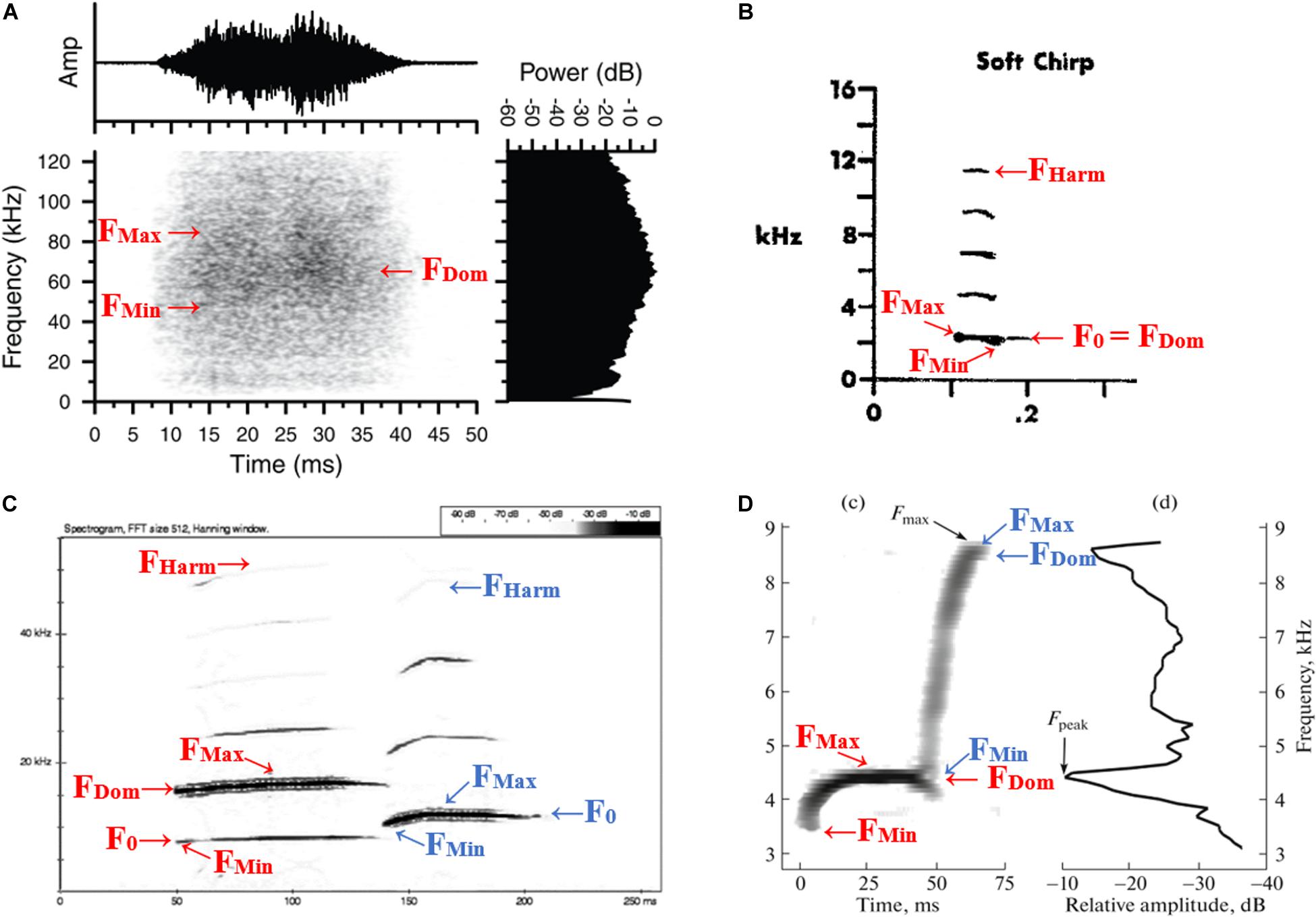
Figure 1. Examples highlighting the variation of how Sciuridae calls are presented as well as how frequency characteristics were measured on different spectrographs and call types. Freqeuncy characteristics measured for all call types: F0 (fundamental frequency: the mean frequency of the primary vibrational frequency of the vocal membrane; when the dominant frequency occurs on the fundamental harmonic, F0 = (FMax + FMin)/2), FDom (dominant frequency: the frequency with the greatest energy, power or amplitude), FMin (minimum frequency: the minimum frequency of the fundamental frequency), FMax [maximum frequency: the maximum frequency of the fundamental frequency (or of harmonic on which FDom is measured)] and FHarm (frequency of highest visible harmonic: mean frequency of the highest complete harmonic visible on the spectrograph). (A) Broadband noise produced by a female southern flying squirrel (Glaucomys volans; Murrant et al., 2013). (B) Alarm call (“shrill chirp”) recorded in a colony of Columbian ground squirrels (Urocitellus columbianus; Betts, 1976). (C) Alarm call (two call elements identified by the initial researchers and therefore recorded separately, red = element 1, blue = element 2) recorded in a population of European ground squirrels in the Ponor Mountains (Spermophilus citellus; Koshev and Pandourski, 2008). (D) Alarm call (also with two distinct elements identified by red and blue) emitted by a female little ground squirrel (Spermophilus pygmaeus; Nikol’skii, 2019). Attribution details for previously published figures available in Supplementary Table 3.
As Waring (1966) demonstrated, harmonics can lead to vocalizations that partially exceed human hearing abilities (i.e., ultrasonic harmonics that exceed 20 kHz). F0 is produced as air exits the lungs (called the glottal flow) and without any interruptions, this tone would only consist of F0 (Titze, 2009). However, if the glottal flow collides with the vocal tract (vocal folds, upper larynx, tongue, mouth, etc.), infinite harmonics will be produced, decreasing in strength as they increase in frequency (Monsen and Engebretson, 1977; Titze, 2009). Harmonics can be strengthened or dampened, depending on the shape of the vocal tract (like the addition of harmonic resonance chambers; Riede et al., 2017) and the presence of strong high-frequency harmonics can be indicative of active manipulation of the vocal tract (Titze, 2009; Fenton et al., 2011). Harmonics can allow animals to extort a larger bandwidth (as in bats; Kingston and Rossiter, 2004; Fenton et al., 2011) or to communicate at a higher frequency (as in anurans and birds; Narins et al., 2004; Arch et al., 2011), though the function of harmonics in most squirrels remains unclear. The yellow-bellied marmot is not the only squirrel for which ultrasonic harmonics have been described. Other genera include Callospermophilus (Eiler and Banack, 2004), Glaucomys (Gilley, 2013; Murrant et al., 2013; Gilley et al., 2019), Sciurus (Zelley, 1971; Lishak, 1982), Spermophilus (Koshev and Pandourski, 2008; Schneiderová, 2008, 2012; Matrosova et al., 2011, 2012, 2016; Schneiderová and Policht, 2012; Schneiderová et al., 2020), Tamias (Blake, 1992), and Urocitellus (Melchior, 1971; Manno et al., 2007). The function of harmonics has been discussed in the context of a few species of ground squirrel (Spermophilus). California ground squirrels (Spermophilus beecheyi) will dampen the strength of higher harmonics to increase the clarity of F0 and improve the ability for receivers to locate the sender (Leger et al., 1980). As well, California ground squirrels habituating to noisy environments have developed stronger harmonics than their counterparts inhabiting quieter environments, improving the signal-to-noise ratio of their calls (Rabin et al., 2003). In contrast, some ground squirrels with larger geographic ranges or with multiple subspecies may alter the strength or number of harmonics to accommodate different habitat or social constraints, resulting in distinct dialects (Eiler and Banack, 2004). So, while all species would have infinite ultrasonic harmonics if recorded under ideal conditions (i.e., facing the recorder directly, maintaining close proximity to reduce attenuation of higher frequencies, remove all background noises, microphone with infinite sensitivity), we consider it of some interest if strong ultrasonic harmonics have only been detected in a subset of free-roaming squirrels.
Since the first spectrographic publications, the calls of over 70 extant squirrel species have been reported. There has been a strong emphasis on alarm calls (reported in 74/89 publications), likely because these are often the highest-amplitude calls, they are produced in response to human observers, and they are most obvious to humans given our own sensory bias (Masterton et al., 1969). Therefore alarm calls are likely the easiest to record. Alarm calls function to notify conspecifics of the presence of a predator and usually prompt a behavioral response from the receivers. Blumstein (1999b) spent over a decade looking at the role of alarm calls in marmots (Marmota spp.), noting that several species can communicate the threat level of a predator by modifying the speed or repetitive pattern of the alarm calls (Blumstein and Armitage, 1997a; Blumstein, 1999a). Additionally, many authors have reported that squirrels will produce different alarm calls (distinct in structure and frequency) to identify unique predator types and to elicit a specific behavioral response in the receivers (Rendall et al., 2009). For example, Richardson’s ground squirrels (Urocitellus richardsonii) produce a short chirp (lasting only 75 ms and rapidly decreasing in frequency from 8 to 3.5 kHz) to warn of aerial predators and evoke an immediate retreat response in conspecifics. In contract, a terrestrial predator is identified by a long whistle (lasting around 400 ms with a constant frequency of 10 kHz) which provokes conspecifics to become for alert stand erect (Davis, 1984). Additionally, these squirrels can add a chuck syllable (only 22.5 ms long and decreasing in frequency from about 6 to 2 kHz) to the end of either the chirp or whistle when predators are nearby, eliciting increased vigilance from receivers after their initial behavioral response (Sloan et al., 2005) and like marmots, Richardson’s ground squirrels will increase the repetition of their calls as the threat level increases (Warkentin et al., 2001). When predator-specific alarm calls are used in a species, the caller most often only distinguishes between terrestrial and aerial predators [Callosciurus (Tamura and Yong, 1993; Tamura, 1995), Cynomys (Ackers and Slobodchikoff, 1999; Loughry et al., 2019), Marmota (Taulman, 1977; Davis, 1991; Blumstein and Arnold, 1995; Blumstein, 1999a), Otospermophilus (Owings and Virginia, 1978; Owings and Leger, 1980), and Urocitellus (Balph and Balph, 1966; Melchior, 1971)], though some Asian tree squirrels have a third call which elicits snake mobbing behavior in conspecifics (Tamura and Yong, 1993).
While alarm calls have been the focus of most studies, squirrels have many distinct call types (Table 2) varying in function from mating (male courtship, pre- and postcopulatory calls, and female mating calls), territoriality, facilitating aggressive (threat, combat) or amicable (maternal, group-cohesion) interactions, and communicating internal states (distress). Several species of flying squirrel have also been observed producing calls during their glides and while exploring their environment (Glaucomys sabrinus and G. volans: Murrant et al., 2013; Petaurista leucogenys: Ando and Kuramochi, 2008), suggesting this nocturnal tribe (Pteromyini; Brandt, 1855) may be using echo-based orientation (Siemers et al., 2009). While this hypothesis has been explored once (Chattin, 1969), the recent discovery of ultrasonic vocalizations in North American flying squirrels (Gilley, 2013; Murrant et al., 2013; Eisinger et al., 2016; Gilley et al., 2019) and the vast improvement in ultrasonic recording since the 1960s warrants a re-examination of the hypothesis.
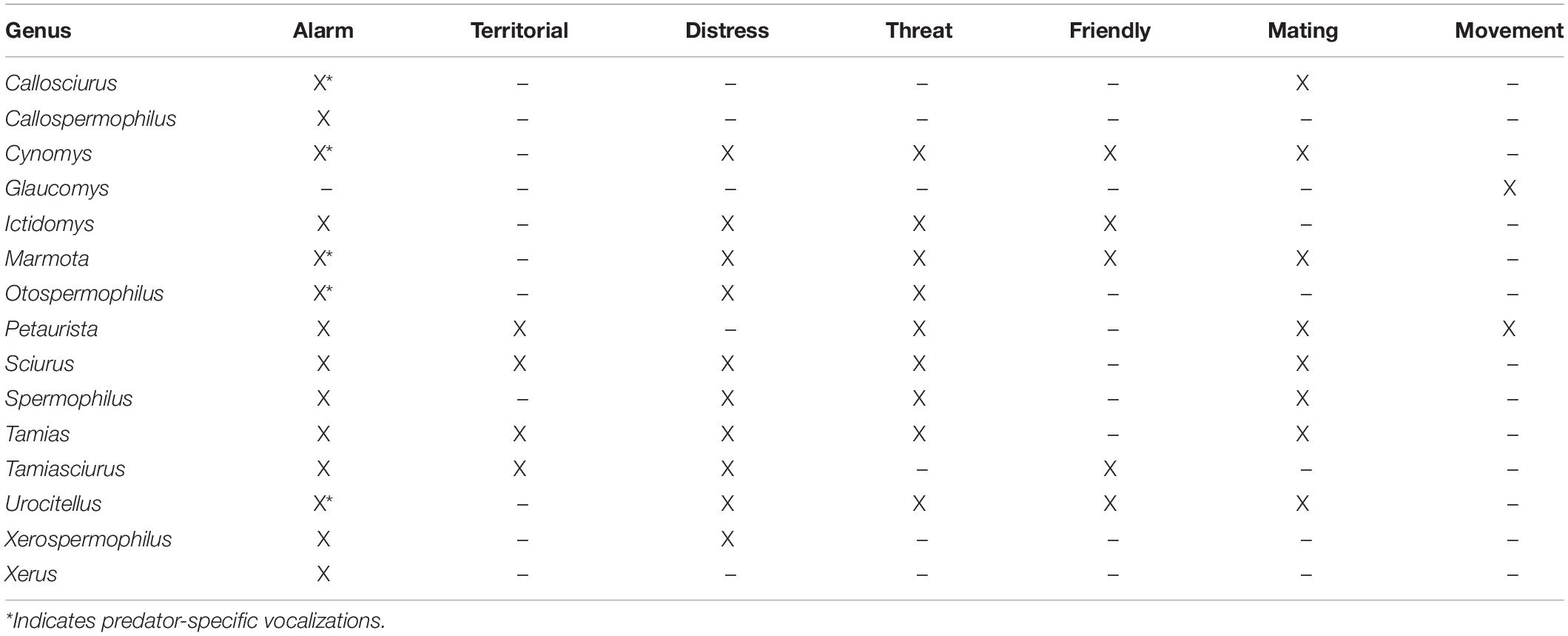
Table 2. Summary of call functions used by all reported vocalizing squirrel genera of Sciuridae (n = 73).
It was not until 2004 that a USV (i.e., a call where F0 entirely exceeds 20 kHz) was first reported in a squirrel species: the Richardson’s ground squirrel (Urocitellus richardsonii; Wilson and Hare, 2004). Richardson’s ground squirrels are known to both produce predator-specific calls that differentiate between terrestrial and aerial predators (Davis, 1984) and vary the repetition and call structure to communicate perceived risk (Warkentin et al., 2001; Sloan et al., 2005). USVs were found to be another way for this species to convey the perceived predation risk by varying their calls between weaker, ultrasonic vocalizations and louder, audible vocalizations as the threat imposed by the presumptive predator increases (Wilson and Hare, 2006). When a predator is farther away, it is less likely to detect highly directional USVs and therefore prey individuals can call altruistically while remaining inconspicuous. As the predator becomes more of a threat, the colony benefits from louder, more omnidirectional alarm calls to ensure that the maximum number of receivers are notified. In addition to varying USV use between perceived threats, it was found that there is a significant increase in the frequency of ultrasonic calls when the emerging juveniles reach their highest density, implying that ultrasonic communication may be most beneficial for social cohesion (Wilson and Hare, 2006). It is key to note that the authors did not use a secondary microphone in the audible range (<20 kHz) to confirm that the calls were not biphonic (two unrelated fundamental frequencies are produced at the same time; Matrosova et al., 2012) with one fundamental in the audible range and a second in the ultrasonic range. However, the authors published a follow-up report in which they attempted to quantity biophonic versus ultrasonic calls in the same population by noting ultrasonic call behaviors (thoracic cavity expanding and mouth held in communicative position, sometimes a very faint whistle can be heard by the observer) while recording with an audible microphone and they were able to differentiate between ultrasonic and biphonic calls (termed “mixed calls” by the authors; Wilson and Hare, 2006).
Wilson and Hare’s (2004) publication challenges the squirrel vocal literature because most of the published repertoires have used microphones that are not sensitive to the ultrasonic range (85% of studies reviewed in our report did not use equipment that encompassed ultrasonic frequencies). By constraining the microphones to ranges that maximize human voice recording, the published repertoires have been unintentionally biased by methodology. While the detection of ultrasonic calls can be extremely rare even when the correct equipment is being used (as in some Spermophilus; Matrosova et al., 2012), since 2004, three new Sciuridae species have been observed to produce USVs. USVs have now been reported in northern (Glaucomys sabrinus: Murrant et al., 2013) and southern flying squirrels (G. volans: Murrant et al., 2013; Eisinger et al., 2016) and noted, though rarely, in the speckled ground squirrel (Spermophilus suslicus: Matrosova et al., 2012). Calls where F0 partially extend into the ultrasonic range have been also been noted in both the southern (G. volans: Gilley, 2013; Murrant et al., 2013; Eisinger et al., 2016; Gilley et al., 2019) and northern flying squirrels (G. sabrinus: Gilley, 2013; Gilley et al., 2019) as well as the Carolina flying squirrel (G. sabrinus coloratus: Gilley, 2013).
Despite the underrepresentation of USVs in published squirrel vocal repertoires owing to technological limitations and difficulties associated with recording free-ranging individuals, squirrels are a useful taxonomic group to preliminarily evaluate factors controlling the frequency limits of mammalian vocal signals. Squirrels have been well sampled across the taxa and represent a diverse range of social structure, habitat use, and behavior. We used phylogenetic methods to investigate the following five popular and novel hypotheses relating to different frequency characteristics of the vocalizing members of Sciuridae (Table 1). (1) The role of body size, which has been shown to drive frequencies higher as body size decreases, will be explored (Arch and Narins, 2008; Martin et al., 2016). However, it has already been noted in Sciuridae that body size differences attributed to age class (pup versus adult) do not result in within-species differences in vocal characteristics (Matrosova et al., 2007; Swan and Hare, 2008), therefore this relationship is only expected to be significant when making cross-species comparisons. (2) To investigate the potential role of predator avoidance (prey species will favor acoustic ranges that exceed the detection of common predators; Arch and Narins, 2008), we used sociality as a proxy because species that exhibit social complexity are more likely to exhibit increased vocal complexity (Blumstein and Armitage, 1997b; Blumstein, 2007). Primates that exhibit more complex social systems are sensitive to higher frequencies (Ramsier et al., 2012) and mammals with complex social systems are more likely to produce higher frequency vocalizations associated with submissiveness and appeasement (Charlton and Reby, 2016). Additionally, Wilson and Hare (2006) suggest that squirrel social systems in which kin are more clustered, either spatially or temporally from juvenile emergence, are more likely to favor USV production which is less likely to be detected at a distance by an eavesdropping predator. Therefore, we predicted that more socially complex squirrels will vocalize at higher frequencies. (3) We explored the acoustic-adaptation hypothesis (terrestrial animals will modify call structure depending on their environment; Blumstein, 2007; Ey and Fischer, 2009) by comparing the frequency limits of squirrels in open versus closed habitats, with open habitats expected to promote the use of higher frequencies. (4) The role of diel activity patterns is interesting as the only nocturnal squirrels are the flying squirrels which might be using vocalizations for echolocation as echo-based orientation (or “echonavigation”) is associated with reduced visual cues in other rodents (Ancillotto et al., 2014; Panyutina et al., 2017). Therefore, we predicted that nocturnal species would have higher frequencies than diurnal species. (5) Finally, the relevance of the equipment used will be analyzed by incorporating the method detection limits (defined as the limits of either the microphone or spectrographic analysis, hereinafter “method limits”) of the publication methods into the models defined below. We predicted that method limits, particularly the upper-frequency limit, will constrain the perceived vocal ranges of recorded species.
Reviewing Frequency Characteristics
We developed a database beginning with a list of publications that described the vocalizations of squirrels (summarized in Supplementary Table 1). The minimum requirement for each publication was the description of at least one call with either a spectrographic analysis or numerical data, though the majority of publications described multiple calls per species or described multiple species per publication (493 calls from 72 species represented in 89 publications; summarized in Supplementary Table 1). The databases used to search for these publications were Google Scholar, JSTOR, Web of Science, and Wiley Online Library. We used the keywords acoustics, acoustic repertoire, calls, frequency, Hz, vocalizations, and ultrasound paired with Sciuridae, squirrel, or an exhaustive list of currently valid and invalid genera (the most updated nomenclature was taken from the Integrated Taxonomic Information System1). For each call described in the selected publication, the following characteristics were taken: the fundamental frequency (F0: the mean frequency of the primary vibrational frequency of the vocal membrane; kHz), dominant frequency (FDom: the frequency with the greatest energy, power or amplitude; kHz), minimum frequency (FMin: the minimum frequency of the fundamental frequency; kHz), maximum frequency (FMax: the maximum frequency of the fundamental frequency or of harmonic on which FDom is measured; kHz), and the highest visible harmonic (FHarm: mean frequency of the highest complete harmonic visible on the spectrograph; kHz) (Figure 1).
Once our review of vocalization publications was complete, we searched for the body mass (g), diel activity pattern (diurnal or nocturnal), social complexity, and habitat openness of the dominant habitat (open or closed) of each species from the relevant vocalization papers. If not provided, other resources including Mammalian Species accounts, PanTHERIA (Jones et al., 2009), and the Animal Diversity Web (Myers et al., 2020) were reviewed. Both male and female body masses were initially recorded, but male body size could not be found for Spermophilus taurensis. Male and female body mass were strongly correlated (r = 0.98, p < 0.001), therefore female body mass was chosen to represent squirrel body size. Because we could only assign an adult female body mass to all species, calls that are exclusively produced by males or pups were removed from the dataset before analysis. We pooled all other calls (calls produced by both sexes or females only as well as calls produced by juveniles and adults) as there is little evidence to suggest that juveniles and adults produce acoustically distinct calls across the family (Matrosova et al., 2007, 2011; Volodina et al., 2010; Schneiderová, 2012; but see: Nikol’skii, 2007). While the initial database included a five-tiered social classification ranging from solitary to colonial [based on the social grades of ground squirrels described by Matějů et al. (2016)], social classes were reduced to social or solitary living to reduce model parameters. Species that exhibit dynamic social structures, such as flying squirrels that engage in social nesting to a greater extent during one portion of the year (Garroway et al., 2013), were treated as socially living. Two subspecies (Marmota baibacina centralis and Tamias dorsalis dorsalis) could not be used in the subsequent analyses because ecological data and body mass-specific to each subspecies could not be found; similarly, the species Spermophilus pallidicauda could not be included as body mass for either sex could not be found.
Phylogeny
VertLife, an online resource that allows the user to extract pruned trees from vertebrate supertrees, was used to produce 100 pruned trees from the Mammalian supertree (Figure 2; Upham et al., 2019). Three subspecies had to be incorporated under their parent species, so branch tips were broken in two and subspecies were treated as equivalent to parent species, with branch lengths identical between the parent and subspecies (the addition of a subspecies did not create any polytomies in the tree). Three species are represented by subspecies only: Sciurus aberti kaibensis, Sciurus niger rufiventer, and Callosciurus erythraeus thaiwanensis.
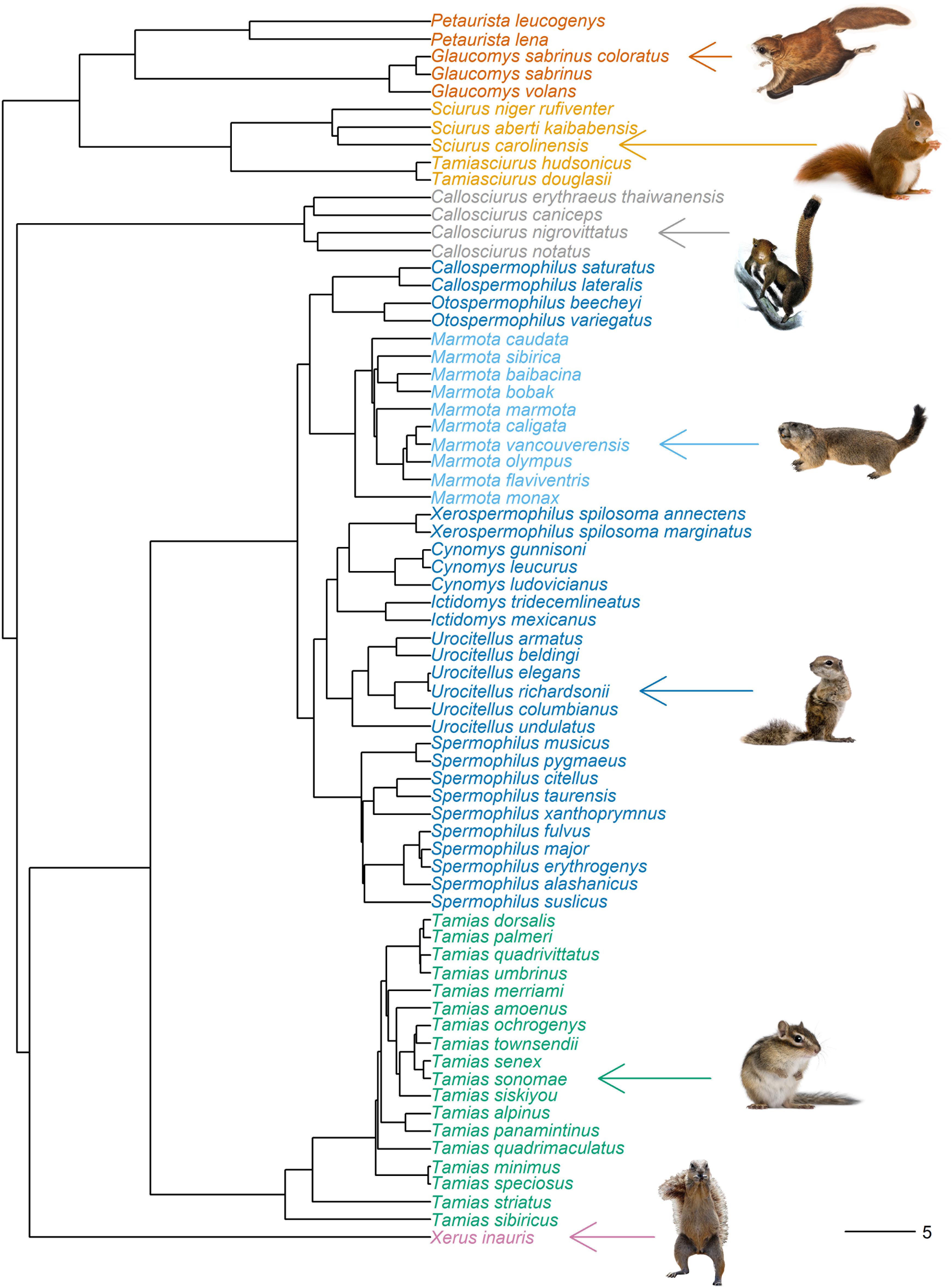
Figure 2. Phylogeny of Sciuridae reduced to all species used in analysis (n = 70). Species present represent the subfamily Callosciurinae (gray: southern Asian tree squirrels), Sciurinae (orange: Pteromyini, flying squirrels; yellow: Sciurini, Holarctic tree squirrels tree squirrels) and Xerinae (pink: tribe Xerini, African ground squirrels; Marmotini, Holarctic ground squirrels). Marmotini is subdivided into the genera Tamias (green: chipmunks), Marmota (light blue: marmots) and the remaining Holarctic ground squirrels (dark blue). Edge lengths to scale; scale bar represents 5 million years from present. Tree downloaded from Vertlife.org. Attribution details for the animal images available in Supplementary Table 3.
Statistical Analysis
Phylogenetic generalized least square (PGLS) modeling was used to account for the variation in acoustic repertoire that may be explained by phylogenetic relatedness. PGLS models produce a lambda parameter, λ, that represents the degree to which the variance of traits is explained by the phylogenetic relationships in the model. The λ parameter varies between 0 and 1, with 0 representing no phylogenetic trace and 1 representing absolute Brownian motion (Freckleton et al., 2002; Martin et al., 2016).
PGLS modeling restricts each species to a single observation (i.e., no subsampling of species permitted). Therefore, the numerous data entries per species had to be reduced. For the fundamental, dominant, maximum, and highest harmonic frequencies, the absolute maximum value for each characteristic reported among all publications was chosen. Likewise, for minimum frequency, the absolute minimum reported frequency was chosen. We use maximum and minimum values rather than the median for a more rigorous test of our hypothesis about method limits.
Body mass and all frequency characteristics were log-transformed to achieve normal distributions. Additive models were built for each frequency type [β0 + body mass (βMass) + diel activity pattern (βDiel) + sociality (βSociality) + habitat openness (βOpen) + method limits (βLim)] using the caper package in R (ver 3.6.2). We reported the test statistics of the regression to evaluate significance and effect size (F-statistic, p-value, and adjusted R2).
Evaluating Correlates of Frequency Characteristics
The effect of body mass and method limits were significant for all five frequency characteristics (F0, FDom, FMin, FMax, and FHarm) whereas habitat openness only had a significant effect for FDom and FHarm (Table 3). The PGLS models fit the data well for most frequency characteristics, with effect sizes (adjusted R2) of 0.64 (p < 0.001), 0.57, (p < 0.001), 0.61 (p < 0.001), and 0.41 (p < 0.001) for F0, FDom, FMax, and FHarm accordingly. The minimum frequency PGLS model did not fit the data well, yielding an effect size of only 0.12 (p < 0.05), however, it was the only model that yielded a significant phylogenetic signal (Pagel’s λ with 95% confidence intervals) of 0.65 [0, 0.88]. In contrast, the phylogenetic signal was estimated to be 0 for F0 (0 [0, 0.96]), FDom (0 [0, 0.32]), FMax (0 [0, 0.95]), and FHarm (0 [0, 0.43]). A summary of model parameters for secondary candidate models (ΔAICc < 2; Mazerolle, 2004) selected through stepwise regression can be found in the Supplementary Material (Supplementary Table 2). Notably, harmonic frequency values could only be found for 43 of the 73 species, with species reported in only one publication being significantly less likely to have reported harmonics (Chi-square = 17.1, p < 0.001) than those appearing in multiple publications.

Table 3. Phylogenetic generalized least square (PGLS) results for the effects of all factors on the frequency characteristics (fundamental, dominant, minimum, maximum, and highest harmonic frequency; kHz) of Sciuridae.
Body Mass
Body mass (Figure 3) had a significant effect on F0 (slope = −0.22 (±SE = 0.05; Table 4), F1,58 = 23.14, p < 0.001), FDom (slope = −0.20 (±0.05), F1,60 = 12.37, p < 0.001), FMin (slope = −0.19 (±0.09), F1,58 = 6.24, p < 0.05), FMax (slope = −0.25 (±0.05), F1,59 = 37.61, p < 0.001), and FHarm (slope = −0.29 (±0.08), F1,37 = 9.18, p < 0.01). These results are consistent with our prediction that increased body mass results in the production of lower frequencies as already shown in mammals (Charlton and Reby, 2016) and birds (Ryan and Brenowitz, 1985). The avian mass-frequency relationship focused on the “emphasized” frequency, similar to FDom reported here (Ryan and Brenowitz, 1985). Our results are somewhat consistent with Martin et al. (2016) who also found that FMin was constrained by mammalian body mass, but the mass-FMin relationship reported was twice as strong as reported here (slope = 0.41). This stronger relationship likely arose because their dataset ranged from microbats (weighing less than 0.1 kg) to whales (larger than 100,000 kg), representing a much larger range of body sizes and therefore showing a stronger effect of body size on frequency types. In comparison, this dataset covered only a range of about 36 g (Tamias alpinus) to 8.0 kg (Marmota sibirica). In contrast to our results, Martin et al. (2016) found that Fmax was not constrained by body size, though the lack of relationship was largely attributed to the interaction between environment and body size. They suggest that because sound waves travel differently in the water, aquatic species are freed from the constraints of body size on the maximum frequency compared to their terrestrial relatives. Like Martin et al. (2016), Ryan and Brenowitz (1985) also found that there was an interaction between environment and body size in avian song frequency, but they compared habitat openness are we did here. Interestingly, adding the interaction between habitat openness and body mass did not change the model outcome (AICc values or variable effects) for most frequency characteristics, but it did yield a significantly better model for FHarm (ΔAICc = −9.78). The effect size of the model increased (adjusted R2 = 0.55, p < 0.001), the effect of habitat openness increased [slope = 3.70 (±0.91), F1,36 = 6.80, p < 0.05] and the interaction between habitat openness and body mass was significant [slope = −0.55 (±0.16), F1,36 = 12.51, p < 0.01]. However, the slope estimate of body mass was reduced [slope = −0.06 (±0.12), F1,36 = 12.04, p < 0.01] and the effect of sociality became non-significant [slope = −0.10 (±0.19), F1,36 = 1.67, p = 0.21]. By observing an interaction plot, we determined that as body size increased in the closed habitat, there was little change in FHarm, whereas there was a large decrease in FHarm with increasing body size in the open habitat.
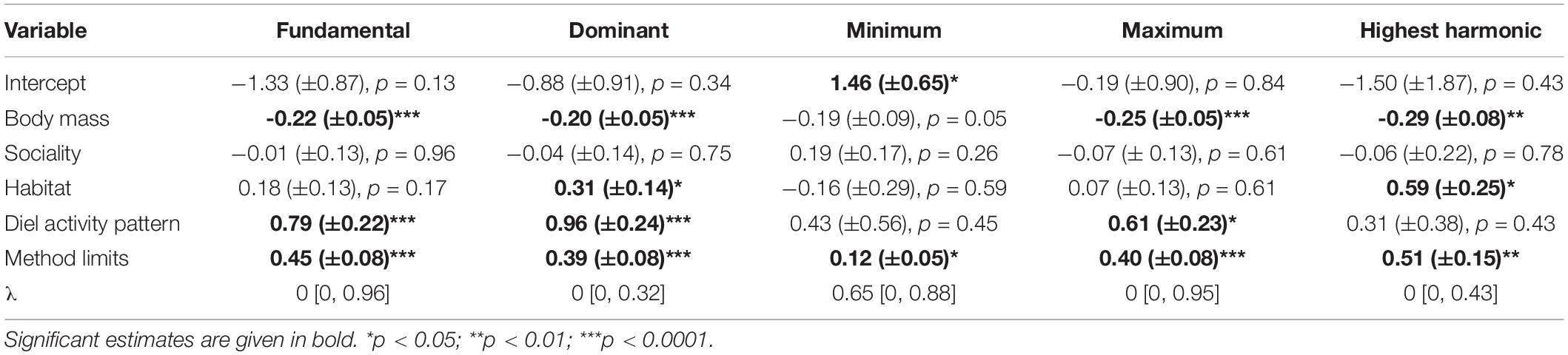
Table 4. Summary of slope (±SE) and phylogenetic signal (λ [95% CI]) estimates for all variables taken from phylogenetic generalized least square (PGLS) models of the frequency characteristics (fundamental, dominant, minimum, maximum, and highest harmonic frequency; kHz) of Sciuridae.
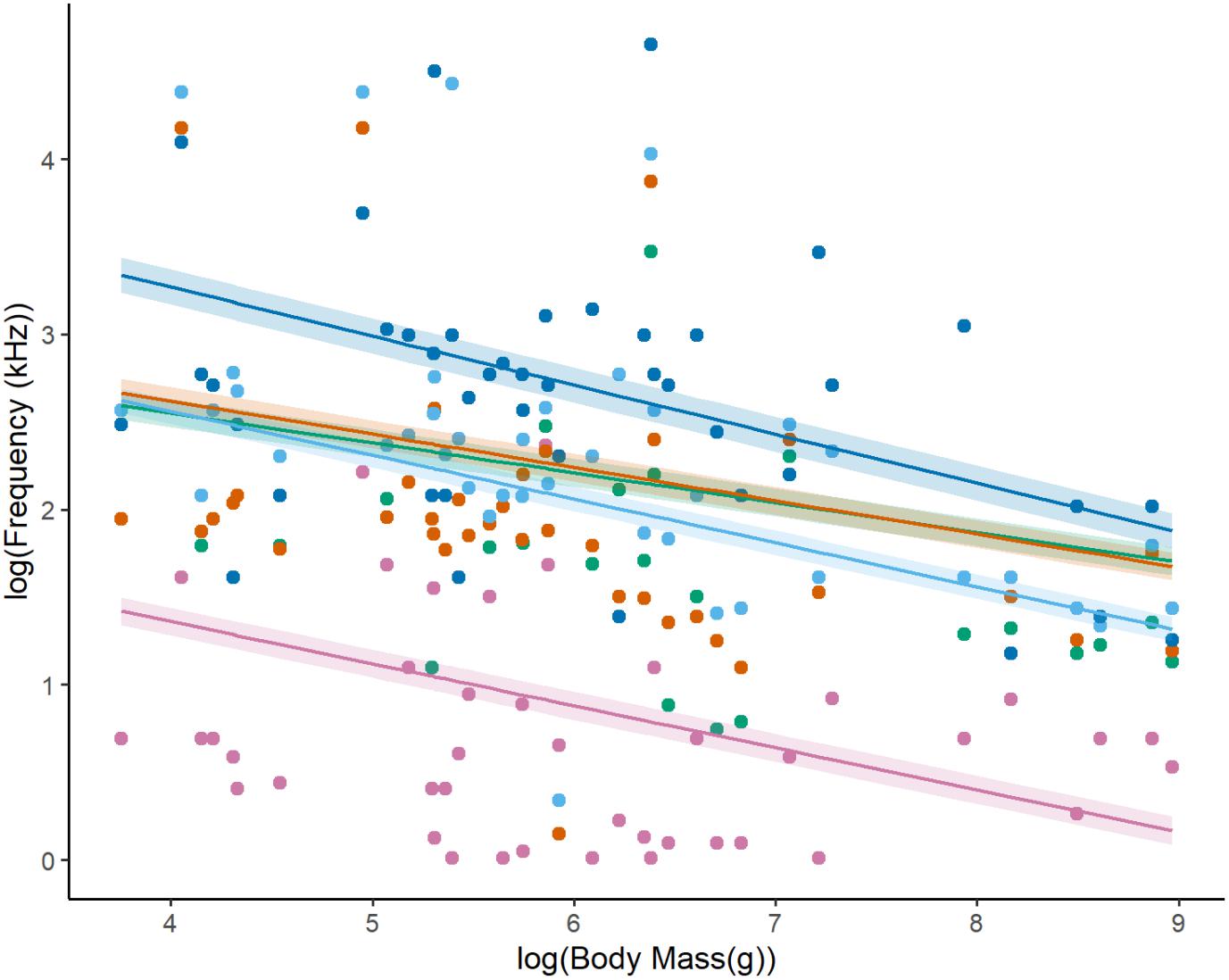
Figure 3. Absolute minimum (pink), fundamental (green), dominant (orange), maximum (light blue), and highest harmonic (dark blue) frequencies of squirrel (Sciuridae, n = 42) vocalizations as a function of body mass (all variables log-transformed for normality; SE of slopes represented as shaded ribbons). Phylogenetic generalized least square (PGLS) models were used to describe the relationships between the variables and are represented by the following linear trend line: minimum [pink, log(Y) = −0.24*log(X) + 2.3], fundamental [green, log(Y) = −0.17*log(X) + 3.2], dominant [orange, log(Y) = −0.19*log(X) + 3.4], maximum [light blue, log(Y) = −0.18*log(X) + 3.6], and highest harmonic [dark blue, log(Y) = −0.28*log(X) + 4.4].
Sociality
Sociality [social (0) or solitary (1)] had a significant effect on F0 [slope = −0.007 (±0.13), F1,58 = 9.54, p < 0.01], FDom [slope = −0.04 (±0.14), F1,60 = 8.60, p < 0.01], and FMax [slope = −0.07 (±0.13), F1,59 = 8.57, p < 0.01]. Notably, sociality had a significant effect on these models while yielding a non-significant slope; investigating the models further, there was no evidence of collinearity between sociality and other variables. However, there was a larger variation in frequencies used by social squirrels compared to solitary squirrels. These results are consistent with Blumstein and Armitage (1997b) who found that social complexity was related to increased use of alarm calls in the repertoire in marmots. In our results, we found that alarm calls are widespread across the taxa, appearing in 64 of the 73 vocalizing Sciuridae species. While social squirrels might not be more likely to have alarm calls in their vocal repertoire than solitary squirrels (Chi-Square = 3.51, p = 0.06), they likely maintain shorter distances between the sender and receiver and increase their production of alarm calls (e.g., Urocitellus beldingi; Sherman, 1985). This reduced distance could facilitate the incorporation of higher frequencies into the repertoire as these frequencies are highly directional but are more likely to be attenuated over longer distances (Smith, 1979). As well, the increased proximity not only results in more altruistic alarm calling (Sherman, 1985) but also the production of more vocalizations to facilitate group cohesion and maintain social hierarchies, particularly between emerging juveniles and established adults. For example, calls in adult female yellow-bellied marmots (Marmota flaviventris) are directly correlated to the emergence of juveniles (Blumstein et al., 1997). Primates, another group of highly communicative and socially variable mammals, also trend toward increased vocal complexity and overall improved hearing sensitivities in more social species and larger groups (Ramsier et al., 2012). To compensate for increased exposure via sound, social animals benefit from communicating in ranges less readily heard by predatory species (Arch and Narins, 2008). In contrast, solitary squirrels would benefit from louder calls if they are warning surrounding conspecifics of potential danger, especially if the species holds relatively large home ranges. However, the maintenance of these territories would likely lead to vocal production that maximizes the effectiveness of territorial displays (which we only found reports of in solitary and socially alternating species), meaning that lower frequencies would be more beneficial to maximize amplitude over longer distances and to increase omnidirectionality. In addition to social structure, mating systems may be complicating the results observed. We grouped monogamous and polygynous species. However, Charlton and Reby (2016) suggests that F0 is a sexually selected trait and is higher in polygynous species and lower in monogamous species. While we excluded calls produced exclusively by males, we kept calls produced by both sexes, therefore not eliminating calls produced by both sexes that are also under sexual selection. We suggest further research into the role of sociality and mating systems in vocal complexity across Sciuridae.
Habitat
The habitat type [open (1) or closed (0)] only had a significant effect on FDom [slope = 0.31 (±0.14), F1,60 = 4.22, p < 0.05] and FHarm [slope = 0.59 (±0.25), F1,37 = 5.65, p < 0.05], with open habitats facilitating higher dominant and higher maximum harmonic frequencies than closed habitats. We expected that the FMax would be most affected by habitat type because higher frequencies are attenuated more rapidly in closed environments (Smith, 1979) resulting in a shift of vocal range (minimum to maximum frequency). Instead, we found that squirrels in open habitats spend their maximum acoustic energy (FDom) on higher frequencies than squirrels in closed habitats, with little effect on range, as FMin and FMax were not significantly influenced by habitat openness (Tables 3, 4). Charlton et al. (2019) have shown that forested animals may not be hindered by their ability to produce or detect high frequencies as many have been shown to co-evolve improved acoustic structures to not lose their access to these frequencies in a closed environment. They suggest that forested animals may boost the amplitude and increase the frequency band of their calls to help counteract the increased attenuation of their environment and improve the ability of receivers to locate the sender (Charlton et al., 2019). This hypothesis would explain why squirrels in closed habitats continue to use high frequencies, despite the limitations. Based on this hypothesis, we would also predict FHarm would not be affected by habitat openness as increased harmonic strength would improve the bandwidth of calls in closed environments and high harmonics would be unhindered in open habitats, making them favorable in both conditions. We found instead that higher harmonics are detected in open habitats, supporting the more traditional acoustic adaptation hypothesis (Blumstein, 2007; Ey and Fischer, 2009). However, we think it is important to note that the detection of the highest harmonic is largely dependent on the recording techniques of the researcher and how the calls have been displayed on spectrographs. It may likely be that high harmonics are easier to record in open environments, whether because the acoustics are better for recording (reduced attenuation of harmonics before reaching the microphone as in bats; Fenton et al., 2011) or the individual squirrel ecology lends to better study subjects (i.e., congregated ground squirrels allow microphones to be placed close to predictable burrow holes compared to free-ranging tree squirrels which have many temporary nests and retreats across a forest canopy). While not explored in this study, environments with greater humidity transmit higher frequencies better than arid environments where calls are attenuated faster (Nikol’skii, 1984). The importance of habitat ariditity was demonstrated in ground squirrels (Spermophilus; Nikol’skii, 2017) when the strength of the relationship between the FDom and body mass was significantly improved when habitat aridity was incorporated as a factor into the models. Overall, our findings suggest that higher dominant frequencies are more easily incorporated and recorded in squirrels in open habitats, but high frequencies are not necessarily unused or completely inhibited by closed habitats.
Diel Activity Pattern
As predicted, nocturnality resulted in significantly higher values for F0 [slope = 0.79 (±0.22), F1,58 = 51.22, p < 0.001], FDom [slope = 0.96 (±0.24), F1,60 = 40.84, p < 0.001], and FMax [slope = 0.61 (±0.23), F1,59 = 33.10, p < 0.001]. Though the frequency traits between diel activity patterns was not significant for FMin [slope = 0.43 (±0.56), F1,58 = 1.63, p = 0.21] and FHarm [slope = 2.96 (±), F1,37 = 2.96, p = 0.09], all frequency characteristics trend toward nocturnal species using higher frequencies than diurnal species (Figure 4). Unfortunately, the lack of behavioral observations associated with the published flying squirrel repertoires, the only nocturnal squirrels, means that interpretation of these results is mostly hypothetical (as nocturnal patterning is only represented by 5 different (sub)species). Both species of Asian giant flying squirrel (Petaurista lena and P. leucogenys) stand out as the only squirrels with movement-associated vocalizations that were recorded while observing free-ranging individuals (Ando and Kuramochi, 2008; Shen, 2013). Additionally, one call associated with the exploration of a novel environment was reported in the southern flying squirrel (Glaucomys volans; Murrant et al., 2013). While there has yet to be any direct evidence of echo-based navigation (Siemers et al., 2009) or echolocation in flying squirrels (Chattin, 1969), we propose that the strong effect of nocturnality on multiple frequency characteristics, correlated to calls in higher frequencies, as well as the recent discovery of USVs in North American flying squirrels (Gilley, 2013; Murrant et al., 2013), warrants a re-examination of the function of USVs in these squirrels with specific echo-based navigation experiments included in the research.
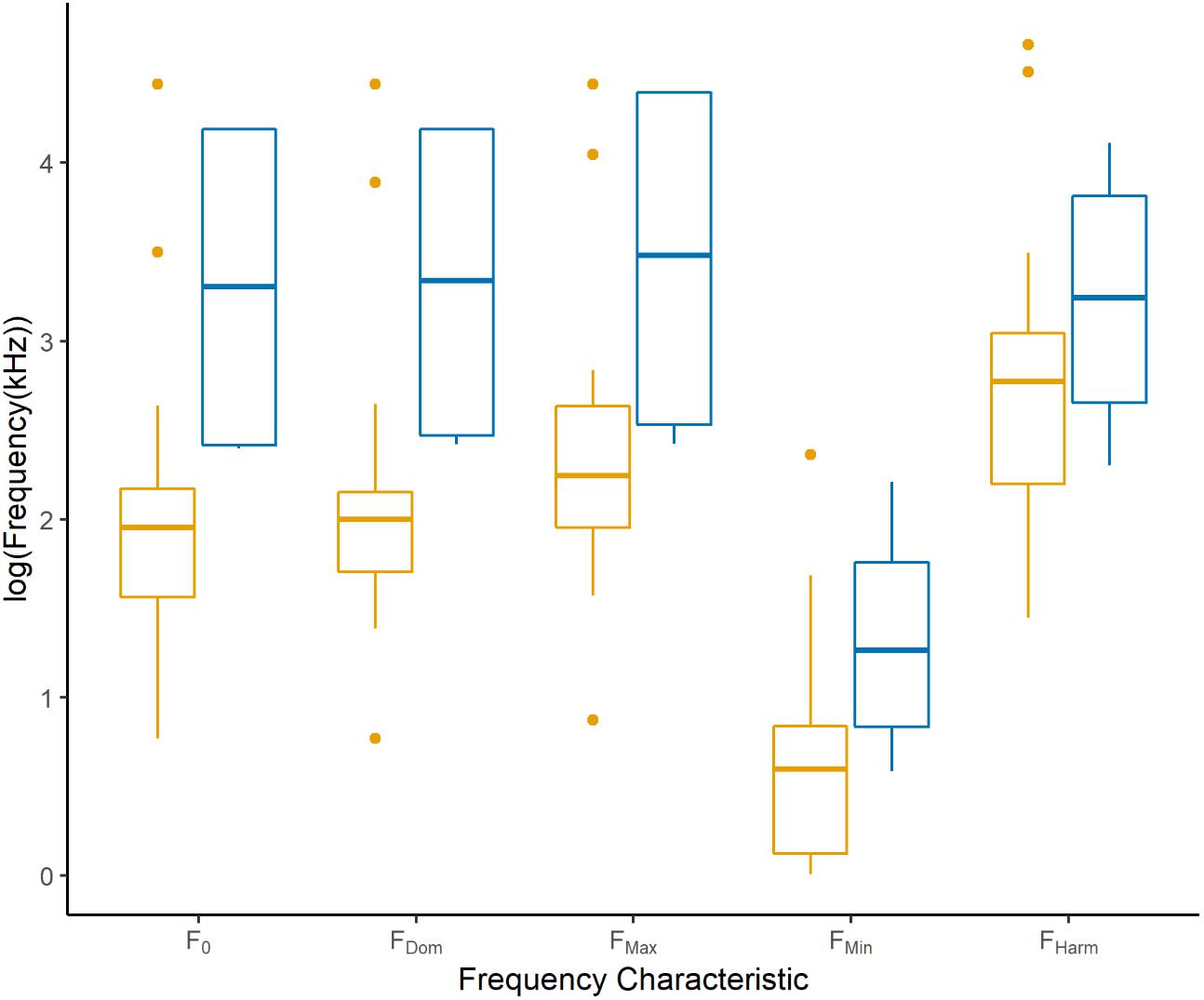
Figure 4. The frequency characteristics of calls produced by diurnal (orange) versus nocturnal (blue) squirrels (species of the family Sciuridae; n = 43). Frequency characteristics presented are the fundmanetal (F0), dominant (FDom), maximum (FMax), minimum (FMin), and maximum harmonic (FHarm) frequencies and all are log-transformed for normality.
Method Limits
As we predicted, the limits of the methods (microphone or analysis ranges) did significantly influence all the frequency characteristics as shown in the PGLS models. As equipment limits increased, so did the F0 [slope = 0.45 (±0.08), F1,58 = 37.91, p < 0.001], FDom [slope = 0.39 (±0.08), F1,60 = 23.78, p < 0.001], FMax [slope = 0.40 (±0.08), F1,59 = 25.16, p < 0.001], and FHarm [slope = 0.51 (±0.15), F1,37 = 11.01, p < 0.01]; similarly, lower method limits were related to a lower FMin, though this relationship was not as strong [slope = 0.12 (±0.05), F1,37 = 4.63, p < 0.05] Additionally, the AICc of all secondary PGLS models were improved by about 10% when method limits were included (note that all secondary candidate models include βLim; Supplementary Table 2). We conclude that the type of equipment being used is crucial for detecting all frequency characteristics and that using equipment with large bandwidths (the entire range of frequencies that could be detected) is important for all squirrel acoustics research as we continue to discover novel USV production in Sciuridae.
Summary
We predicted that body mass, sociality, habitat openness, diel activity pattern, and method limits would influence the fundamental, dominant, minimum, maximum, and highest harmonic frequencies (kHz; Table 1). We found at least some support for all of these hypotheses. We found that across the squirrel family, body mass significantly affected all frequency characteristics, a finding that is mostly consistent with other literature (Arch and Narins, 2008; Charlton and Reby, 2016; Martin et al., 2016). Social squirrels used higher fundamental, dominant, and maximum frequencies which aligns with the predator-avoidance hypothesis (Arch and Narins, 2008). Social squirrels also used a larger range of frequencies which can be partially attributed to increased communication amongst kin (Blumstein and Armitage, 1997b) or sexual selection acting on vocal traits (Charlton and Reby, 2016). As predicted by the acoustic adaptation hypothesis (Blumstein, 2007; Ey and Fischer, 2009), we found that species inhabiting open habitats used higher dominant and harmonic frequencies than those in closed habitats where high frequencies are more rapidly attenuated by the environment (Smith, 1979); however, the maximum frequency was not affected by habitat type which suggests that squirrels in closed habitats may have co-evolved structures to still maintain access to USVs despite the environmental complications (Charlton et al., 2019). Nocturnality was associated with higher frequencies for the fundamental, dominant, and maximum frequencies, suggesting that nocturnal, gliding squirrels are under unique selection pressures for using USVs. An explanation for this general pattern of higher frequencies in nocturnal squirrels remains elusive. Finally, the significant effect of method limits on all frequency characteristics and the presence of method limits in all candidate models (Supplementary Table 2) suggest that method limits are important for framing the ranges detected during recording sessions.
Think Before They Squeak – Conclusion
Squirrels are vocally complex taxa, displaying a wide range of call frequencies and functions. While there has been a representative sampling across the genera, there are large gaps in the geographic sampling of squirrels (Figure 5). The United States alone accounts for over 50% of the published vocal repertoires. In contrast, only a single study has occurred in Africa and no repertoires have been published from Central or South America. In the face of global extinction crises and habitat loss, it is more important than ever to archive the traits of rare and cryptic species so that we can maximize the utility of all available monitoring and conservation tools. Bioacoustic monitoring is an effective tool for cryptic species (Walker, 1964; Gilbert et al., 1994; Tripp and Otter, 2006) and has been shown to improve the monitoring of flying squirrels which are notoriously difficult to detect (Diggins et al., 2016). Considering these analyses, we encourage researches to record squirrel vocalizations in under-sampled locations. We further suggest the use of recording equipment that can resolve frequencies that extend into the ultrasonic range so that repertoires accurately reflect the ranges used by the species being studied. Additionally, we caution that researchers studying the vocalizations of previously reported squirrels should consider the accuracy of previous literature, especially when there is possible evidence of partial USVs or ultrasonic harmonics present. Squirrels that are socially complex or inhabiting open areas are of interest for studies focused on high-frequency vocalizations; we also strongly encourage the examination of the role of USVs in nocturnal, flying squirrels. We caution that while USV research requires specialized technology, this work also requires ideal recording conditions and patience as USVs can be rare and difficult to detect in some Sciuridae species (Matrosova et al., 2012). In conclusion, while rodent bioacoustic studies are typified by controlled environments and lab animals, studying the vocalizations of free-ranging rodents can reveal new information about the natural world, providing informative findings and new insights that apply to real-time conservation efforts.
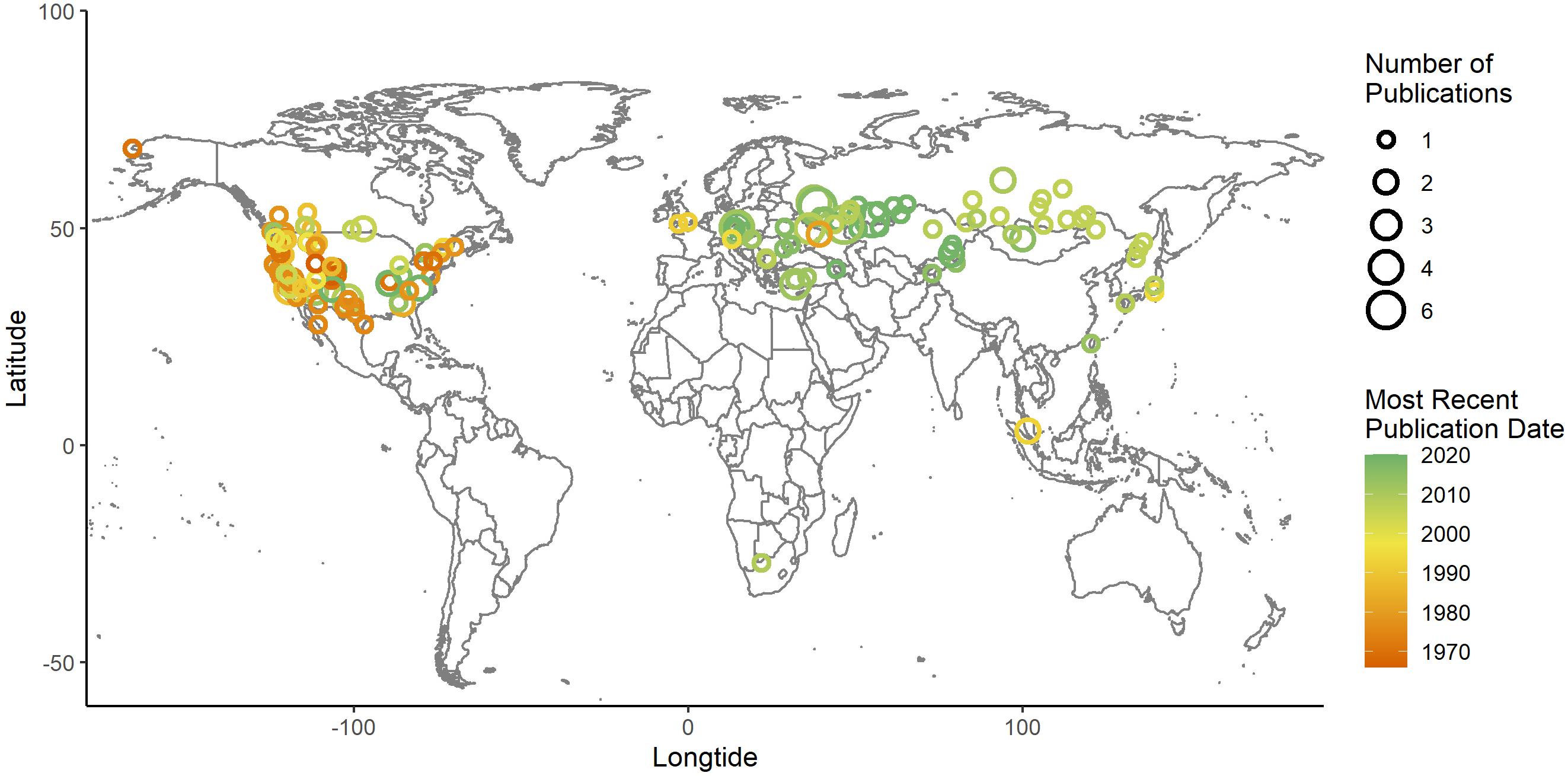
Figure 5. The recording locations of squirrel vocal repertoires published between 1966 and 2020. The year of publication is represented along a color gradient and the number of studies recorded at each location is represented along a size gradient. The epicenter of each circle represents the location; circles are translucent to minimize overlap. The United States alone has hosted 52% of all published studies; Russia, Canada, and the Czech Republic are also notable contributors representing 18, 11, and 9% each. North America, Europe, Asia, and Africa represent 61, 30, 13, and 1% respectively, with no record of South America, India, or the majority of Africa hosting a repertoire study.
Data Availability Statement
The data and R scripts that support the findings of this study are openly available on Dryad at https://doi.org/10.5061/dryad.vt4b8gtpm.
Author Contributions
JB and SN conceptualized the manuscript. SN collected data, conducted analyses, and prepared the manuscript with guidance and critical feedback from JB. All authors contributed to the article and approved the submitted version.
Funding
The authors’ institutions provided support for this publication.
Conflict of Interest
The authors declare that the research was conducted in the absence of any commercial or financial relationships that could be construed as a potential conflict of interest.
Acknowledgments
We thank Trent University for access to online databases, P. O’Brien for assistance in article collection and valuable feedback during conceptualization, and PO’B, K. Solmundson, and R. Dillion for their insightful comments during the final editing stages.
Supplementary Material
The Supplementary Material for this article can be found online at: https://www.frontiersin.org/articles/10.3389/fevo.2020.00193/full#supplementary-material
Footnotes
References
Ackers, S. H., and Slobodchikoff, C. N. (1999). Communication of stimulus size and shape in alarm calls of gunnison’s prairie dogs. Cynomys gunnisoni. Ethology 105, 149–162. doi: 10.1046/j.1439-0310.1999.00381.x
Ahlén, I. (2004). “Heterodyne and time-expansion methods for identification of bats in the field and trough sound analysis,” in Bat Echolocation Research: Tools, Techniques and Analysis, eds R. M. Brigham, E. K. V. Kalko, G. Jones, S. Parsons, and H. J. G. A. Limpens, (Austin, TX: Bat Conservation International), 72–78.
Amplifier Corporation of America, (1959). “Amplifier corporation of America,” in The Audio Tape Recorder Directory, (New York, NY: Audio Devices, INC), 5–6.
Ancillotto, L., Sozio, G., Mortelliti, A., and Russo, D. (2014). Ultrasonic communication in gliridae (Rodentia): the hazel dormouse (Muscardinus avellanarius) as a case study. Bioacoustics 23, 129–141. doi: 10.1080/09524622.2013.838146
Ando, M., and Kuramochi, Y. (2008). Vocal Communication of the japanese giant flying squirrel Petaurista leucogenys. J. Agr. Sci. 53, 176–183.
Arch, V. S., and Narins, P. M. (2008). ‘Silent’ signals: selective forces acting on ultrasonic communication systems in terrestrial vertebrates. Anim. Behav. 76, 1423–1428. doi: 10.1016/j.anbehav.2008.05.012
Arch, V. S., Richards-Zawaki, C. L., and Feng, A. S. (2011). Acoustic communication in the kihansi spray toad (Nectophrynoides asperginis): insights from a captive population. J. Herpetol. 45, 45–49. doi: 10.1670/10-084.1
Balph, D. M., and Balph, D. F. (1966). Social communication of uinta ground squirrels. J. Mammal. 47, 440–450. doi: 10.2307/1377685
Betts, J. B. (1976). Behaviour in a Population of Columbian Ground Squirrels, Spermophilus columbianus columbianus. Anim. Behav. 24, 652–680. doi: 10.1016/S0003-3472(76)80079-6
Blake, B. H. (1992). Estrous calls in captive asian chipmunks. Tamias sibiricus. J. Mammal. 73, 597–603. doi: 10.2307/1382031
Blanchard, R. J., Blanchard, D. C., Agullana, R., and Weiss, S. M. (1991). Twenty-Two KHz alarm cries to presentation of a predator, by laboratory rats living in visible burrow system. Physiol. Behav. 50, 967–972. doi: 10.1016/0031-9384(91)90423-l
Blumstein, D. T. (1999a). Alarm calling in three species of marmots. Behaviour 136, 731–757. doi: 10.1163/156853999501540
Blumstein, D. T. (1999b). The evolution of functionally referential alarm communication. Evol. Commun. 3, 135–147. doi: 10.1075/eoc.3.2.03blu
Blumstein, D. T. (2007). “The evolution of alarm communication in rodents: structure, function, and the puzzle of apparently altruistic calling,” in Rodent Societies: An Ecological and Evolutionary Perspective, eds J. O. Wolff, and P. W. Sherman, (Chicago, IL: University of Chicago Press), 317–327.
Blumstein, D. T., and Armitage, K. B. (1997a). Alarm calling in yellow-bellied marmots: i. the meaning of situationally variable alarm calls. Anim. Behav. 53, 143–171. doi: 10.1006/anbe.1996.0285
Blumstein, D. T., and Armitage, K. B. (1997b). Does sociality drive the evolution of communicative complexity? A comparative test with ground-dwelling sciurid alarm calls. Am. Nat. 150, 179–200. doi: 10.1086/286062
Blumstein, D. T., and Arnold, W. (1995). Situational specificity in alpine-marmot alarm communication. Ethology 100, 1–13. doi: 10.1111/j.1439-0310.1995.tb00310.x
Blumstein, D. T., Steinmetz, J., Armitage, K. B., and Daniel, J. C. (1997). Alarm calling in yellow-bellied marmots: ii. the importance of direct fitness. Anim. Behav. 53, 173–184. doi: 10.1006/anbe.1996.0286
Brandt, J. F. (1885). Beitrage zur nahern Kenntniss der Säugethiere Russland’s. St. Petersburg: Kaiserlichen Akademie der Wissenschaften.
Charlton, B. D., Owen, M. A., and Swaisgood, R. R. (2019). Coevolution of vocal signal characteristics and hearing sensitivity in forest mammals. Nat. Commun. 10, 1–7.
Charlton, B. D., and Reby, D. (2016). The evolution of acoustic size exaggeration in terrestrial mammals. Nat. Commun. 7:12739. doi: 10.1038/ncomms12739
Chattin, D. (1969). Acoustical Echolocation in Flying Squirrels. Diploma Thesis, Reed College, Portland, OR.
Corrigan, J. G., and Flannelly, K. J. (1979). Ultrasonic vocalizations of defeated male rats. J. Comp. Physiol. Psych. 93, 105–115. doi: 10.1037/h0077565
Credner, S., Burda, H., and Ludescher, F. (1997). Acoustic communication underground: vocalization characteristics in subterranean social mole-rats (Cryptomys sp. Bathyergidae). J. Comp. Physiol. A 180, 245–255. doi: 10.1007/s003590050045
Davis, L. S. (1984). Alarm Calling in Richardson’s ground squirrels (Spermophilus richardsonii). Z. Tierpsychol. 66, 152–164. doi: 10.1111/j.1439-0310.1984.tb01362.x
Davis, R. O. (1991). “Semantical communication in anti-predator alarm calls,” in The Natural History of Eastern California and High Altitude Research, eds C. A. Hall, Jr., V. Doyle-Jones, and B. Widawski, (Oakland, CA: University of California), 275–312.
Diggins, C. A., Gilley, L. M., Kelly, C. A., and Ford, W. M. (2016). Comparison of survey techniques on detection of northern flying squirrels. Wildl. Soc. Bull. 40, 654–662. doi: 10.1002/wsb.715
Digweed, S. M., and Rendall, D. (2009). Predator-associated vocalizations in north american red squirrels (Tamiasciurus hudsonicus): to whom are alarm calls addressed and how do they function? Ethology 115, 1190–1199. doi: 10.1111/j.1439-0310.2009.01709.x
Eiler, K. C., and Banack, S. A. (2004). Variability in the alarm call of golden-mantled ground squirrels (Spermophilus lateralis and S. saturatus). J. Mammal. 85, 43–50. doi: 10.1644/1545-1542(2004)085<0043:vitaco>2.0.co;2
Eisinger, J. W., Scheibe, J. S., and Flaherty, E. A. (2016). Novel Glaucomys volans vocalizations in indiana and evidence of geographic variation in high frequency communication. J. Mammal. 97, 1219–1227. doi: 10.1093/jmammal/gyw076
Ey, E., and Fischer, J. (2009). The “Acoustic Adaptation Hypothesis” - a review of the evidence from birds. Anurans and mammals. Bioacoustics 19, 21–48. doi: 10.1080/09524622.2009.9753613
Fenton, M. B., Skowronski, M. D., McGuire, L. P., and Faure, P. A. (2011). Variation in the use of harmonics in the calls of laryngeally echolocating bats. Acta Chiropterol. 13, 169–178. doi: 10.3161/150811011x578714
Fernández-Vargas, M., Tang-Martinez, Z., and Phelps, S. M. (2011). Singing, allogrooming, and allomarking behaviour during inter- and intra-sexual encounters in the neotropical short-tailed singing mouse (Scotinomys teguina). Behaviour 148, 945–965. doi: 10.1163/000579511x584591
Fitch, W. T., Neubauer, J., and Herzel, H. (2002). Calls out of chaos: the adaptive significance of nonlinear phenomena in mammalian vocal production. Anim. Behav. 63, 407–418. doi: 10.1006/anbe.2001.1912
Freckleton, R. P., Harvey, P. H., and Pagel, M. (2002). Phylogenetic analysis and comparative data: a test and review of evidence. Am. Nat. 160, 712–726. doi: 10.1086/343873
Garroway, C. J., Bowman, J., and Wilson, P. J. (2013). Complex social structure of southern flying squirrels is related to spatial proximity but not kinship. Behav. Ecol. Sociobiol. 67, 113–122. doi: 10.1007/s00265-012-1431-3
Geyer, L. A. (1979). Olfactory and thermal influences on ultrasonic vocalization during development in rodents. Integr. Comp. Biol. 19, 419–431. doi: 10.1093/icb/19.2.419
Geyer, L. A., and Barfield, R. J. (1978). Influence of gonadal hormones and sexual behavior on ultrasonic vocalization in rats: I. Treatment of females. J. Comp. Physiol. Psych. 92, 438–446. doi: 10.1037/h0077480
Geyer, L. A., Barfield, R. J., and McIntosh, T. K. (1978). Influence of gonadal hormones and sexual behavior on ultrasonic vocalization in rats: II. Treatment of males. J. Comp. Physiol. Psych. 92, 447–456. doi: 10.1037/h0077487
Gilbert, G., McGregor, P. K., and Tyler, G. (1994). Vocal individuality as a census tool: practical considerations illustrated by a study of two rare species (Individualidad vocal como herramienta en los censos: consideraciones prácticas ilustradas por un estudio de dos especies raras. J. Field Ornithol. 65, 335–348.
Gilley, L. M. (2013). Discovery and Characterization of High-Frequency Calls in North American Flying Squirrels (Glaucomys sabrinus and G. volans): Implications for Ecology, Behavior, and Conservation. Dissertation, Auburn University, Auburn, AL.
Gilley, L. M., Diggins, C. A., Pearson, S. M., and Best, T. L. (2019). Vocal repertoire of captive northern and southern flying squirrels (Glaucomys sabrinus and G. volans). J. Mammal. 100, 518–530. doi: 10.1093/jmammal/gyz064
Gould, E., Negus, N. C., and Novick, A. (1964). Evidence for echolocaation in shrews. J. Exp. Zool. 156, 19–37.
Griffin, D. R., and Galambos, R. (1941). The sensory basis of obstacle avoidance by flying bats. J. Exp. Zool. 86, 481–506. doi: 10.1002/jez.1400860310
Hahn, M. E., and Lavooy, M. J. (2005). A review of the methods of studies on infant ultrasound production and maternal retrieval in small rodents. Behav. Genet. 35, 31–52. doi: 10.1007/s10519-004-0854-7
Hofer, M. A., and Shair, H. (1978). Ultrasonic vocalization during social interaction and isolation in 2-week-old rats. Dev. Psychobiol. 11, 495–504. doi: 10.1002/dev.420110513
Hofer, M. A., and Shair, H. (1980). Sensory processes in the control of isolation-induced ultrasonic vocalization by 2-week-old rats. J. Comp. Physiol. Psych. 94, 271–279. doi: 10.1037/h0077665
Jones, K. E., Bielby, J., Cardillo, M., Fritz, S. A., O’Dell, J., Orme, C. D. L., et al. (2009). PanTHERIA: a species-level database of life history, ecology, and geography of extant and recently extinct mammals. Ecology 90: 2648. doi: 10.1890/08-1494.1
Kalcounis-Rueppell, M. C., Petric, R., and Marler, C. A. (2018). The bold, silent type: predictors of ultrasonic vocalizations in the genus Peromyscus. Front. Ecol. Evo. 6:198. doi: 10.3389/fevo.2018.00198
Kingston, T., and Rossiter, S. J. (2004). Harmonic-hopping in wallacea’s bats. Nature 429, 654–657. doi: 10.1038/nature02487
Knudsen, E. I. (1981). The hearing of the barn owl. Sci. Am. 245, 112–125. doi: 10.1038/scientificamerican1281-112
Koenig, W., Dunn, H. K., and Lacy, L. Y. (1946). The Sound spectrograph. J. Acoust. Soc. Am. 18, 19–49.
Koshev, Y., and Pandourski, I. (2008). Structure and variability of alarm calls of European ground squirrel Spermophilus citellus L. 1766 (Mammalia: Rodentia) from western bulgaria. Acta Zool. Bulgar. 60, 99–105.
Kotler, B. P. (1984). Risk of predation and the structure of desert rodent communities. Ecology 65, 689–701. doi: 10.2307/1938041
Leger, D. W., Owings, D. H., and Gelfand, D. L. (1980). Single-note vocalizations of california ground squirrels: graded signals and situation-specificity of predator and socially evoked calls. Z. Tierpsychol. 52, 227–246. doi: 10.1111/j.1439-0310.1980.tb00714.x
Lishak, R. S. (1982). Gray squirrel mating calls?: a spectrographic and ontogenic analysis. J. Mammal. 63, 661–663.
Loughry, W. J., Oeser, M., Anderson, C. D., and Hoogland, J. L. (2019). The importance of individual variation in the alarm calls of gunnison’s prairie dogs. Anim. Behav. 150, 59–68. doi: 10.1016/j.anbehav.2019.01.019
Manno, T. G., Nesterova, A. P., Debarbieri, L. M., Kennedy, S. E., Wright, K. S., and Dobson, F. S. (2007). Why do male columbian ground squirrels give a mating call? Anim. Behav. 74, 1319–1327. doi: 10.1016/j.anbehav.2007.02.033
Martin, K., Tucker, M. A., and Rogers, T. L. (2016). Does size matter? Examining the drivers of mammalian vocalizations. Evolution 71, 249–260. doi: 10.1111/evo.13128
Masterton, B., Heffner, H., and Ravizza, R. (1969). The evolution of human hearing. J. Acoust. Soc. Am. 45, 966–985.
Matějů, J., Kratochvíl, L., Pavelková, Z., Řièánková, V. P., Vohralík, V., and Němec, P. (2016). Absolute, not relative brain size correlates with sociality in ground squirrels. P. R. Soc. B 283, 1827. doi: 10.1098/rsp.2015.2725
Matrosova, V. A., Blumstein, D. T., Volodin, I. A., and Volodina, E. V. (2011). The potential to encode sex, age, and individual identity in the alarm calls of three species of marmotinae. Naturwissenschaften 98, 181–192. doi: 10.1007/s00114-010-0757-9
Matrosova, V. A., Rusin, M. Y., Volodina, E. V., Proyavka, S. V., Savinetskaya, L. E., Shekarova, O. N., et al. (2016). Genetic and alarm call diversity across scattered populations of speckled ground squirrels (Spermophilus suslicus). Mamm. Biol. 81, 255–265. doi: 10.1016/j.mambio.2016.01.001
Matrosova, V. A., Schneiderová, I., Volodin, I. A., and Volodina, E. V. (2012). Species-specific and shared features in vocal repertoires of three eurasian ground squirrels (Genus Spermophilus). Acta Theriol. 57, 65–78. doi: 10.1007/s13364-011-0046-9
Matrosova, V. A., Volodin, I. A., Volodina, E. V., and Babitsky, A. F. (2007). Pups crying bass: vocal adaptation for avoidance of age-dependent predation risk in ground squirrels? Behav. Ecol. Sociobiol. 62, 181–191. doi: 10.1007/s00265-007-0452-9
Mazerolle, M. J. (2004). “APPENDIX 1: Making Sense out of Akaike’s Information Criterion (AIC): Its Use and Interpretation in Model Selection and Inference from Ecological Data,” in Mouvements et reproduction des Amphibiens en Tourbières Perturbèes. Dissertation, Université Laval, Quebec City, QB.
Melchior, H. R. (1971). Characteristics of arctic ground squirrel alarm calls. Oecologia 7, 184–190. doi: 10.1007/bf00346360
Miller, J. R., and Engstrom, M. D. (2007). Vocal stereotypy and singing behavior in baiomyine mice. J. Mammal. 88, 1447–1465. doi: 10.1644/06-mamm-a-386r.1
Monsen, R. B., and Engebretson, A. M. (1977). Study of variations in the male and female glottal wave. J.Acoust. Soc. Am. 62, 981–993. doi: 10.1121/1.381593
Murrant, M. N., Bowman, J., Garroway, C. J., Prinzen, B., Mayberry, H., and Faure, P. A. (2013). Ultrasonic vocalizations emitted by flying squirrels. PLoS One 8:e73045. doi: 10.1371/journal.pone.0073045
Myers, P., Espinosa, R., Parr, C. S., Jones, T., Hammond, G. S., and Dewey, T. A. (2020). The Animal Diversity Web (Online). Avaliable at: https://animaldiversity.org (accessed April 30, 2020).
Narins, P. M., Feng, A. S., Lin, W., Schnitzler, H., Denzinger, A., Suthers, R. A., et al. (2004). Old world frog and bird vocalizations contain prominent ultrasonic. J. Acoust. Soc. Am. 115, 10–13.
Nikol’skii, A. A. (1984). Çâóêîâûå Ñèãíàëû Ìëåêîïèòàùèõ â Ýâîëöèîííîì Ïðîöåññå/Sound Signals of Mammals in Evolutionary Process. Moscow: Íàóêà/Nauka.
Nikol’skii, A. A. (2007). A comparative analysis of the alarm call frequency in different age rodent groups (in Russian). Zool. Zh. 86, 499–504.
Nikol’skii, A. A. (2017). Two models of the sound-signal frequency dependence on the animal body size as exemplified by the ground squirrels of eurasia (Mammalia. Rodentia). Dokl. Biol. Sci. 477, 227–231. doi: 10.1134/s0012496617060059
Nikol’skii, A. A. (2019). Sex accent and biphonation in the sound signal of ground squirrels (Mammalia, Rodentia). Dokl. Biol. Sci. 487, 119–123. doi: 10.1134/S0012496619040094
Obrist, M. K., Pavan, G., Sueur, J., Riede, K., Llusia, D., and Márquez, R. (2010). “Chapter 5. bioacoustics approaches in biodiversity inventories,” in Volume 8 - Manual on Field Recording Techniques and Protocols for All Taxa Biodiversity Inventories, eds J. Eymann, J. Degreef, C. Häuser, J. C. Monje, Y. Samyn, and D. VandenSpiegel (Belgium: The Journal Dedicated to Capacity Building in Taxonomy and Collection Management), 68–99.
Oswalt, G. L., and Meier, G. W. (1975). Olfactory, thermal, and tactual influences on infantile ultrasonic vocalization in rats. Dev. Psychobiol. 8, 129–135. doi: 10.1002/dev.420080205
Owings, D. H., and Leger, D. W. (1980). Chatter vocalizations of california ground squirrels: predator- and social-role specificity. Z. Tierpsychol. 54, 163–184. doi: 10.1111/j.1439-0310.1980.tb01070.x
Owings, D. H., and Virginia, R. A. (1978). Alarm calls of California ground squirrels. Z. Tierpsychol. 46, 58–70. doi: 10.1111/j.1439-0310.1978.tb01438.x
Panyutina, A. A., Kuznetsov, A. N., Volodin, I. A., Abramov, A. V., and Soldatova, I. B. (2017). A blind climber: the first evidence of ultrasonic echolocation in arboreal mammals. Integr. Zool. 12, 172–184. doi: 10.1111/1749-4877.12249
Rabin, L. A., Mccowan, B., Hooper, S. L., and Owings, D. H. (2003). Anthropogenic noise and its effect on animal communication: an interface between comparative psychology and conservation biology. Int. J. Comp. Psychol. 16, 172–192.
Ramsier, M. A., Cunningham, A. J., Finneran, J. J., and Dominy, N. J. (2012). Social drive and the evolution of primate hearing. Philos. T. R. Soc. B 367, 1860–1868. doi: 10.1098/rstb.2011.0219
Rendall, D., Owren, M. J., and Ryan, M. J. (2009). what do animal signals mean? Anim. Behav. 78, 233–240. doi: 10.1016/j.anbehav.2009.06.007
Revere-Wollsenak Division (2010). Service Manual: Wollensak T-1500, T- 1500U, T-1515-4, T-1520, T-1700 Recorders. Chicago, IL: Minnesota Mining and Manufacturing Co.
Riede, T., Borgard, H. L., and Pasch, B. (2017). Laryngeal airway reconstruction indicates that rodent ultrasonic vocalizations are produced by an edge-tone mechanism. R. Soc. Open Sci. 4, 170976. doi: 10.1098/rsos.170976
Ryan, M. J., and Brenowitz, E. A. (1985). The role of body size, phylogeny, and ambient noise in the evolution of bird song. Am. Nat. 126, 87–100. doi: 10.1086/284398
Schneiderová, I. (2008). Acoustics Communication and Alarm Calls in Two Species of Ground Squirrels Spermophilus Citellus and Spermophilus taurensis (Sciuridae; Rodentia). Diploma Thesis, Univerzita Karlova, Prague.
Schneiderová, I. (2012). Frequency-modulated second elements of two-element alarm calls do not enhance discrimination of callers in three eurasian ground squirrels. Curr. Zool. 58, 749–757. doi: 10.1093/czoolo/58.5.749
Schneiderová, I., and Policht, R. (2012). Acoustic analysis of the alarm call of the anatolian ground squirrel Spermophilus xanthoprymnus: a description and comparison with alarm calls of the taurus S. taurensis and European S. citellus Ground Squirrels. Naturwissenschaften 99, 55–64. doi: 10.1007/s00114-011-0870-4
Schneiderová, I., Štefanská, L., and Kratochvíl, L. (2020). Geographic variability in the alarm calls of the european ground squirrel. Curr. Zool. 66, 407–415. doi: 10.1093/cz/zoz055
Shen, P. (2013). Acoustic Behavior of White-Faced Flying Squirrel (Petaurista lena) in Guanghau Village, Alishan. Master’s thesis, National Sun Yat-sen University, Kaohsiung.
Sherman, P. W. (1985). Alarm calls of belding’s ground squirrels to aerial predators? nepotism or self- preservation? Behav. Ecol. Sociobiol. 17, 313–323. doi: 10.1007/bf00293209
Siemers, M., Schauermann, G., Turni, H., and von Merten, S. (2009). Why do shrews twitter? communication or simple echo-based orientation. Biol. Lett. 5, 593–596. doi: 10.1098/rsbl.2009.0378
Sirotin, Y. B., Costa, M. E., and Laplagne, D. A. (2014). Rodent ultrasonic vocalizations are bound to active sniffing behavior. Front. Behav. Neurosci. 8:399. doi: 10.3389/fnbeh.2014.003399
Sloan, J. L., Wilson, D. R., and Hare, J. F. (2005). Functional morphology of richardson’s ground squirrel, Spermophilus richardsonii, alarm calls: the meaning of chirps, whistles, and chucks. Anim. Behav. 70, 937–944. doi: 10.1016/j.anbehav.2005.01.013
Smith, W. J. (1979). The study of ultrasonic communication. Am. Zool. 19, 531–538. doi: 10.1093/icb/19.2.531
Swan, D. C., and Hare, J. F. (2008). Signaler and receiver ages do not affect responses to richardson’s ground squirrel alarm calls. J. Mammal. 89, 889–894. doi: 10.1644/07-mamm-a-228.1
Tamura, N. (1995). Postcopulatory mate guarding by vocalization in the formosan squirrel. Behav. Ecol. Sociobiol. 36, 377–386. doi: 10.1007/bf00177333
Tamura, N., and Yong, H. (1993). Vocalizations in response to predators in three species of malaysian callosciurus (Sciuridae). J. Mammal. 74, 703–714. doi: 10.2307/1382292
Taulman, J. F. (1977). Vocalizations of the hoary marmot, Marmota caligata. J. Mammal. 58, 681–683. doi: 10.2307/1380026
Terhune, J. J. (2011). From switches to menus and students to HQPs-the evolving world of marine mammal bioacoustics. Aquat. Mamm. 37, 95–100.
Tomasi, T. E. (1979). Echolocation by the short-tailed shrew Blarina brevicauda. J. Mammal. 60, 751–759. doi: 10.2307/1380190
Tripp, T. M., and Otter, K. A. (2006). Vocal Individuality as a potential long-term monitoring tool for western screech-owls. Megascops kennicottii. Can. J. Zool. 84, 744–753. doi: 10.1139/z06-055
Upham, N. S., Esselstyn, J. A., and Jetz, W. (2019). Inferring the mammal tree: species-level sets of phylogenies for questions in ecology. Evolution, and conservation. PLoS Biol. 17:494. doi: 10.1371/journal.pbio.3000494
Volodina, E. V., Matrosova, V. A., and Volodin, I. A. (2010). An unusual effect of maturation on the alarm call fundamental frequency in two species of ground squirrels. Bioacoustics 20, 87–98. doi: 10.1080/09524622.2011.9753634
Walker, T. J. (1964). cryptic species among sound-producing ensiferan orthoptera (Gryllidae and Tettigoniidae). Q. Rev. Biol. 39, 345–355. doi: 10.1086/404325
Waring, G. H. (1966). Sounds and communications of the yellow-bellied marmot (Marmota flaviventris). Anim. Behav. 14, 177–183. doi: 10.1016/s0003-3472(66)80028-3
Warkentin, K. J., Keeley, A. T. H., and Hare, J. F. (2001). Repetitive calls of juvenile richardson’s ground squirrels (Spermophilus richardsonii) communicate response urgency. Can. J. Zool. 79, 569–573. doi: 10.1139/z01-017
Wilson, D. R., and Hare, J. F. (2004). Ground squirrel uses ultrasonic alarms. Nature 430:523. doi: 10.1038/430523a
Wilson, D. R., and Hare, J. F. (2006). The adaptive utility of richardson’s ground squirrel (Spermophilus richardsonii) short-range ultrasonic alarm signals. Can. J. Zool. 84, 1322–1330. doi: 10.1139/z06-120
Wood, F. C. Jr. (1952). Porpoise Sounds. A Phonograph Record of Underwater Sounds Made by Tursiops Truncatus and Stenella Plagiodon. Marineland, FL: Marineland Research Laboratories.
Yamazaki, Y., Yamada, H., Murofushi, M., Momose, H., and Okanoya, K. (2004). Estimation of hearing range in raptors using unconditioned responses. Ornithol. Sci. 3, 85–92. doi: 10.2326/osj.3.85
Keywords: Sciuridae, squirrels, vocalizations, alarm call, ultrasonic vocalizations, frequency characteristics
Citation: Newar SL and Bowman J (2020) Think Before They Squeak: Vocalizations of the Squirrel Family. Front. Ecol. Evol. 8:193. doi: 10.3389/fevo.2020.00193
Received: 07 February 2020; Accepted: 28 May 2020;
Published: 14 July 2020.
Edited by:
Jane Waterman, University of Manitoba, CanadaReviewed by:
Ilya Volodin, Lomonosov Moscow State University, RussiaJames Frederick Hare, University of Manitoba, Canada
Copyright © 2020 Newar and Bowman. This is an open-access article distributed under the terms of the Creative Commons Attribution License (CC BY). The use, distribution or reproduction in other forums is permitted, provided the original author(s) and the copyright owner(s) are credited and that the original publication in this journal is cited, in accordance with accepted academic practice. No use, distribution or reproduction is permitted which does not comply with these terms.
*Correspondence: Jeff Bowman, SmVmZi5Cb3dtYW5Ab250YXJpby5jYQ==