- 1Chair of Restoration Ecology, Department Ecology and Ecosystem Management, School of Life Sciences, Technical University of Munich, Freising, Germany
- 2Ecological Novelty Group, Institute of Biology, Department of Biology, Chemistry and Pharmacy, Freie Universitt Berlin, Berlin, Germany
- 3Department of Sustainable Agriculture Sciences, Rothamsted Research, Harpenden, United Kingdom
- 4Norwegian Institute of Bioeconomy Research (NIBIO), Svanvik, Norway
A challenge in many restoration projects, in particular when establishing de novo communities, is the arrival and later dominance of invasive alien plants. This could potentially be avoided by designing invasion-resistant native communities. Several studies suggest achieving this by maximizing trait similarity between natives and potential invaders (“limiting similarity”), but evidence supporting this approach is mixed so far. Others pose that the relative time of arrival by native and invasive species (“priority effects”) could play a stronger role, yet this factor and its interaction with trait similarity is not fully understood in the context of ecological restoration. Thus, we hypothesized that multi-trait similarity would increase suppression of invasive species by native communities, and that the effect would be stronger when natives arrive first. We established two distinct communities of native central European grassland species based on native–invasive trait similarity, and then tested the introduction of invasive Ambrosia artemisiifolia and Solidago gigantea separately when arriving in the native communities at two times, i.e., sown either at the same time as the natives or 2 weeks after. For the traits selected, our data did not provide evidence for a limiting similarity effect, but rather supported priority effects. Both native communities more effectively suppressed invaders that arrived after the natives. In addition, the native community that produced the most biomass suppressed both invasive species more than the most ecologically similar community. This effect of biomass revealed that prioritizing native–invader ecological similarity can fail to account for other community characteristics that affect invasion resistance, such as biomass. Instead, native communities could be designed to enhance priority effects through the inclusion of early and fast developing species. We conclude that native community composition plays a significant role in the establishment success by invasive species, and resource pre-emption seems more significant than trait similarity. In terms of grassland restoration, native species should be selected based on plant traits related to fast emergence and early competitiveness.
Introduction
According to the latest report of the Intergovernmental Science-Policy Platform on Biodiversity and Ecosystem Services, human activities have significantly altered 75% of the global land surface leading to a sharp rise in extinctions (IPBES, 2019). Land-use change is often associated with ecosystem degradation such as soil erosion or the invasion of alien species, which have negative impacts on biodiversity (IPBES, 2019). The report also stressed the importance of ecological restoration of degraded habitats as a promising strategy to mitigate negative effects on biodiversity as well as its associated ecosystem services. However, a common problem during restoration is the presence of invasive alien species, which jeopardize the success of many projects. In particular, during the establishment of target plant communities, often the slow development of natives favors colonization by alien species, especially in scarcely vegetated sites.
Thus, new approaches are needed to suppress biological invasions during the re-introduction of native vegetation. A promising method is to use a trait-based framework to assemble resistant communities with species that outcompete incoming invasive alien plants (Laughlin, 2014; Yannelli et al., 2018). This approach can be useful to prevent the establishment of such species during early phases of ecological restoration, to ensure the success of the project and the effective use of economic resources (Funk et al., 2008). Yet, though often not considered, the selection of suitable plant traits can be challenging given that other factors might act in concert determining competition outcomes during community assembly (Byun et al., 2018).
At the community level, competition for limiting resources, depicted by niche overlap of native and alien species, can be a significant mechanism that modulates the biotic resistance of plant communities (MacDougall et al., 2009). According to this, alien species would only be able to invade communities consisting of ecologically dissimilar species, a concept described by the “limiting similarity hypothesis” (Shea and Chesson, 2002; Funk et al., 2008). Several studies have explored this concept by using a set of plant functional traits for comparing native and invasive species with respect to ecological similarity (Byun et al., 2013; Yannelli et al., 2018; Catford et al., 2019; Fagúndez and Lema, 2019). There is some evidence that trait similarity between native and invasive species can modulate invasion success, particularly in artificial communities, but results have been conflicting so far (Price and Pärtel, 2013; Yannelli et al., 2018).
For instance, when Yannelli et al. (2017b) tested the effect of multi-trait similarity using European grassland species and two invasive plants (Ambrosia artemisifolia and Solidago gigantea), they found that the dominance of an ecologically similar native species could suppress the invasives. In a follow-up study, Yannelli et al. (2018) confirmed that while an ecologically similar community suppressed S. gigantea, fast seedling emergence and canopy development of the native communities overruled the effects of ecological similarity. In other words, temporal differences in traits that confer an advantage in resource exploitation (i.e., niche pre-emption), were more important for invasion suppression than ecological similarity. The question arising is whether functional similarity is a poor predictor of invasive plants suppression, or if temporal dynamics might influence the strength of such effects. Though poorly explored in terms of restoration, timing of arrival can have a significant effect on community composition and invasion suppression (Hess et al., 2019). Namely, temporal aspects, such as the early emergence of certain species in the community could modulate the effects of niche overlap, leading to mixed results found when testing how effective functional similarity is for restoration.
Early colonizers control the establishment of later-arriving species by occupying safe sites for establishment, regulating their persistence by changing the biotic and abiotic conditions of the restored ecosystem (Helsen et al., 2016). For example, the resource uptake by early emerging species can generate so-called “priority effects” leading to fitness inequalities among early- and late-emerging species (Hess et al., 2020). Priority effects could be beneficial for restoration if they arise from early native colonizers that enhance biotic resistance, or could be detrimental if invasive species are able to colonize first and then dominate. That is, early arriving plants, whether native or invasive, can pre-empt the available resources such as space, nutrients and light, thus reducing establishment opportunities for late-arriving individuals. Such effects can ultimately lead to dominance by the first colonizers (Fukami, 2015), and potentially to the failure of restoration projects.
Thus, even if the composition of the restored native community is manipulated to maximize overall ecological similarity with potential invaders, relative timing of arrival mediated by priority effects would have a stark impact on restoration success. Indeed, invasive species often have high early germination rates and fast seedling development, resulting in a temporal advantage over natives (Wainwright et al., 2012; Wainwright and Cleland, 2013). As indicated by previous research, this means that in order to assure resistance to an invasive species during restoration, community design should not only seek to increase the ecological similarity between native and invasive species, but also needs to ensure that priority effects benefit the natives. However, relative effects of interactions between priority effects and ecological similarity during community assembly have not been well explored in previous studies (Hess et al., 2019).
In this contribution we examine the potential roles of ecological similarity and priority effects with respect to the success of invasive species during early restoration. We do so in a controlled glasshouse environment, but from the perspective of applied restoration. We apply the concept of limiting similarity by designing, a priori, native communities that are either more or less similar to the invasive species according to information from plant trait databases. This reflects the information on ecological similarity of native and invasive species likely available to land managers seeking to restore native communities, who may not have extensive resources for on-site characterization of species traits. We test for priority effects by either planting both native and invasive species at the same time, or by planting the invasive species later than the native species. In practice, this scenario could result from land managers timing the planting of native species before the date that invasive species germinate, or from short-term control of invasive species following the planting of native species (Young et al., 2017). We also assess how limiting similarity and priority effects, thus applied, could interact in terms of whether the timing of arrival of invasive species affects their success when arriving in either an ecologically similar or dissimilar native community.
We selected grassland species commonly used in restoration projects, and the two invasive plant species Ambrosia artemisiifolia L. and Solidago gigantea Aiton. Ecological similarity between native and invasive species was evaluated by classifying them into groups based on functional traits. In a greenhouse experiment, we composed native communities based on plants from the same functional group as the invasive species or from a different group, and then introduced the invasive species to these similar and dissimilar native communities at different arrival times. We hypothesized that: (i) that native communities with a greater ecological trait similarity to the invasive species will more successfully suppress the invader; (ii) native communities will exert stronger suppression on the invasive species due to resource pre-emption when the natives establish earlier; and (iii) that these two factors positively interact to increase the strength of suppression. By designing functionally similar communities with this method we aim to help managers, who are typically in charge of implementing restoration programs, to be able to plan a priori which natives are the ones likely to suppress invaders.
Materials and Methods
Species, Seed Material and Traits Selection
The two invasive alien plant species Ambrosia artemisiifolia and Solidago gigantea are problematic in many parts of Europe (Kowarik, 2003). Ambrosia artemisiifolia was introduced as seed contaminant and is currently present in most European countries albeit with different abundances (Essl et al., 2015). This annual pioneer occurs at disturbed habitats such as roadsides, waste places, construction sites, agricultural fields, abandoned fields, and urban ruderal habitats. In heavily invaded regions it causes crop-yield losses, and its pollen is highly allergenic (Gerber et al., 2011). Solidago gigantea was initially introduced to Europe as an ornamental, but has naturalized in many countries, developing dense monospecific stands that inhibit native vegetation (Weber and Jakobs, 2005).
Seeds of A. artemisiifolia were collected near river Danube in Vienna, E Austria (48°16′01′′N, 16°22′10′′E), and those of S. gigantea were gathered along river Isar in Freising, S Germany (48°23′57′′N, 11°45′16′′E). The native competitors were commercially produced species for restoration purposes, selected from a pool of 28 species with a frequency ≥10% occurrence in more than 100 surveys of calcareous grasslands in the north of Munich, Germany (Conradi and Kollmann, 2016). The seed material of these species was supplied by Johann Krimmer (Pulling, Germany) based on local provenances. Species nomenclature follows Wißkirchen and Haeupler (1998).
In order to categorize the study species into functional groups we utilized eight traits, namely, canopy height at maturity, shoot morphology (rosette, hemi-rosette, or non-rosette plants), life form (sensu Raunkiaer, 1934), morphology of vegetative organs (rhizome, runner, pleiocorm, and tuft), leaf dry matter, specific leaf area (SLA), seed mass and plant longevity (annual or perennial). These traits are known to be related to different stages such as dispersal, establishment, growth, persistence, as well as the species’ competitive ability (Westoby et al., 2002; Funk et al., 2008; Pérez-Harguindeguy et al., 2013). For example, shoot morphology relates to the plant capacity to ground cover, plant height influences resource capitalization such as light but it also affects plant fecundity, SLA and leaf dry matter have found to affect resource allocation and photosynthetic rate, and seed mass is highly associated to early competitiveness and survival (Pérez-Harguindeguy et al., 2013). Longevity was included as a proxy for temporal niche overlaps. Traits categories are rarely clear-cut, but we considered that including some that might seem redundant, would increase the chances of successfully portraying resource use. Trait information was obtained from the databases BiolFlor and LEDA (Klotz et al., 2002; Kleyer et al., 2008).
Functional Groups and Community Composition
We transformed non-numerical traits to numerical units following Yannelli et al. (2017b). Functional groups were devised by means of cluster analysis, using Gower’s similarity coefficient among species and the Ward linkage method (Pla et al., 2012). The analysis yielded three functional groups according to species trait similarities (FG1, FG2, and FG3), with both invasive species falling into FG2 (Supplementary Figure S1 and Supplementary Table S1). We used a MANOVA test to confirm that the three functional groups were significantly different (F = 13.6, P < 0.0001). All analyses of the functional grouping were performed using Infostat (Di-Rienzo et al., 2013).
Previous results from Yannelli et al. (2017b) showed that FG1 has highest, FG3 intermediate and FG2 lowest suppression success. Though not tested at that point, we found indications that early developing of some natives species might modulate the effect of trait similarity as a predictor of invasive species suppression. Therefore, we decided to only use FG1 and FG2 in order to devise native communities for testing the three hypotheses, given FG1’s previous success and FG2 potentially being the most similar community. In this study though, we randomly selected nine species that were known to germinate well from each functional group to avoid a richness effect due to lack of emergence (Table 1).
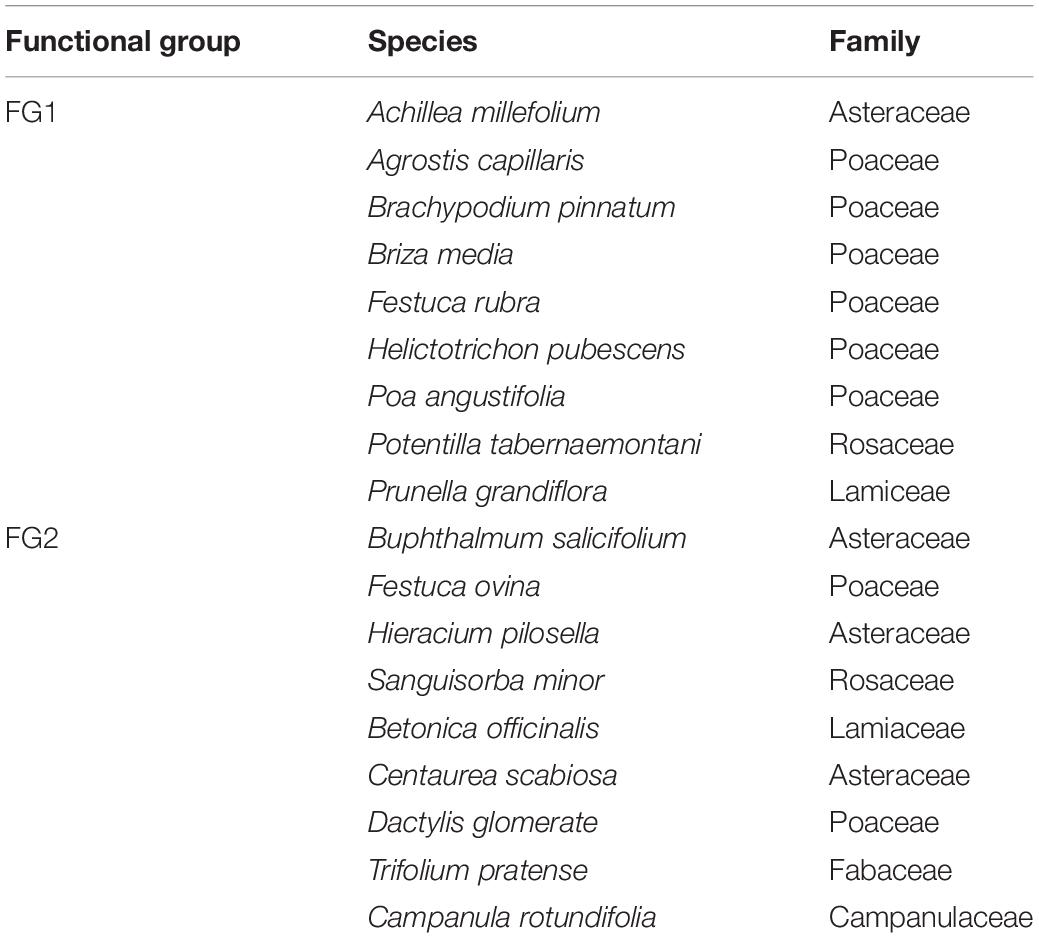
Table 1. Species composition, families, and functional groups included in the two selected experimental communities.
Experimental Design
We performed an additive experiment using a fully randomized design with the treatments “functional group identity of the community” and “time of arrival.” The functional group identity treatment had three levels, namely communities composed with native species from either FG1 or FG2, and a control with no natives (“FG1,” “FG2,” “control”). Specifically, there was a control for each invasive species and timing treatments, consisting of pots in which natives were not sown, and invasives were sown as a monoculture at the different times of arrival. Time of arrival was defined by whether the invasive species was sown at the same time as the natives or 2 weeks later (“same arrival” and “late arrival”). Treatment combinations were tested with the two invasive species separately, and each treatment combination was replicated five times (60 trays in total).
Sowing densities of 3 g m–2 were used for native species as is common practice in restoration projects (Kiehl et al., 2010), and 1 g m–2 for both invasive species, comparable with densities in soil seed banks (Minchin, 1987; Yannelli et al., 2017b). We filled 40 × 30 × 6 cm3 plastic trays with peat-based substrate (EinheitsErde®, Einheitserdewerke Werkverband e.V., Altengronau, Germany; N, 180 g m–3; P, 240 g m–3; K, 240 g m–3; pH 5.8) and scattered the seeds of native species on top of the soil. The invasive species were sown simulating a seed rain at the same time as the natives or after 2 weeks without disturbing the establishing community for the late arrival treatment. We supplied water daily from above until germination and then switched to watering on demand for 1 h from below the trays.
We carried out the experiment in an unheated greenhouse at the Centre of Greenhouses and Laboratories Dürnast, School of Life Sciences Weihenstephan, Technical University of Munich (48°24′N, 11°41′E). The experiment lasted for 8 weeks starting in June 2014, and had an average temperature of 21 ± 6°C.
Measurements and Data Analyses
The experiment was terminated after 8 weeks, when A. artemisiifolia started flowering to decrease work hazards due to its highly allergenic pollen. At this time, we identified and visually estimated the percentage cover of all plant species growing in the trays for each treatment combination. We then harvested the biomass by cutting all plants 1 cm aboveground and separating natives from invasive species. All plant material was oven-dried for 3 days at 65°C and subsequently weighted.
Aboveground biomass data was log (ln) transformed to meet the assumption of a normal distribution. We performed two-way ANOVA to assess differences in invasive biomass among the treatments for each invasive species separately (model: invasive biomass − community identity × time of arrival). Post hoc pairwise comparisons were made using the Bonferroni correction. We calculated the overall native community weighted mean trait distance (CWMTD) difference to both invaders weighted by each species cover and assessed its correlation with native biomass. For this, we used the distances calculated using all traits included in the definition of functional groups, as explained in section “Functional Groups and Community Composition.” As a proxy for niche similarity, given that we did not measure the traits during the experiments (Yannelli et al., 2017b), we calculated phylogenetic distances by constructing a phylogenetic tree using a tree of all angiosperms as a backbone (Zanne et al., 2014), and calculated distances with the R package pez (Supplementary Figure S2) to obtain the community weighted distances based on plant cover (CWMPD). We then checked for correlations among potential explanatory variables to avoid collinearity and found that trait and phylogenetic distance differences were highly correlated (Pearson c = 0.81, P < 0.001). Given that we found a moderate correlation native biomass and CWMTD (Pearson c = 0.65, P < 0.01), we tested which variable was a better predictor of invasive species suppression and selected the native biomass because of its better fit (model: invasive biomass − native biomass × time of arrival).
Results
All native species selected for our communities were perennials and hemicryptophytes. FG1 was overrepresented by species from the family Poaceae (all but two species) and runners, while FG2 included more families. The rest of the traits were evenly represented in both communities. Although FG2 was supposed to be more like both invasive species than FG1, there were still trait differences between this community and the invasive species (Table 2).
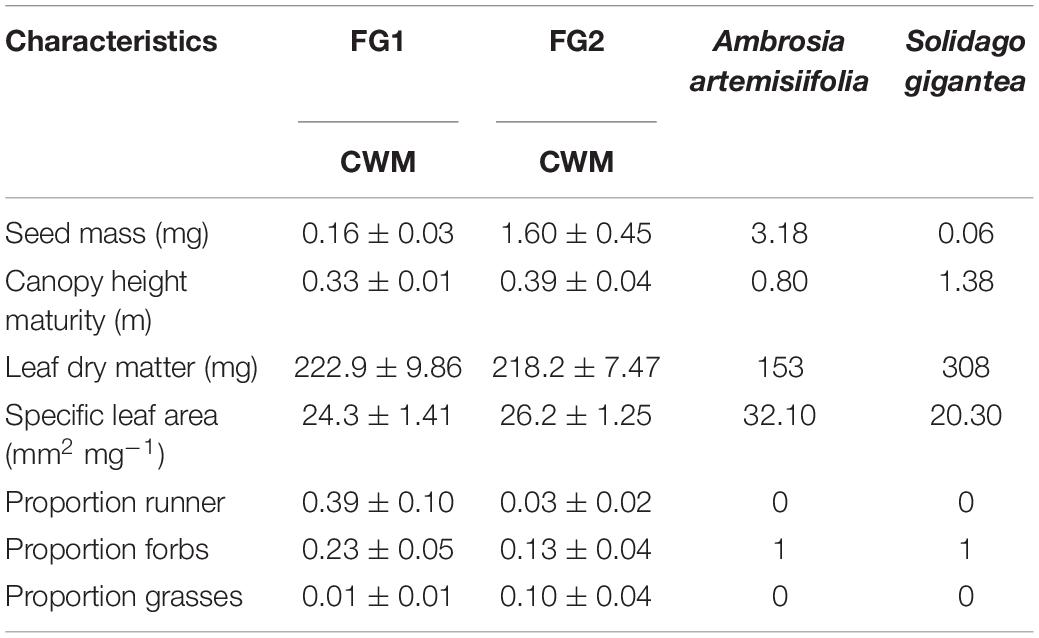
Table 2. Community weighted means (CWM ± SE) of each numerical trait selected calculated for the two communities (FG1, 2) and average values of the same traits for both invasive alien species (Ambrosia artemisiifolia and Solidago gigantea).
We found that functional group identity of the invaded community, time of arrival and their interactions had a significant effect on aboveground biomass of the two invasive species, 8 weeks after sowing. When the invasive A. artemisiifolia arrived late, the lowest biomass was found under competition with the FG1 community [mean ln(mass) + SE = −3.5 + 0.5], followed by FG2 [mean ln(mass) + SE = 1.3 + 0.3] and the control [mean ln(mass) + SE = 1.4 + 0.7; Figure 1 and Supplementary Tables S2, S3; ANOVA: interaction F = 19.4, P < 0.001]. When arriving at the same time, A. artemisiifolia biomass did not differ among the functional group communities [FG1 mean ln(mass) + SE = 4.2 + 0.2, FG2 mean ln(mass) + SE = 4.5 + 0.1, control mean ln(mass) + SE = 4.61 + 0.1]. In S. gigantea, aboveground biomass was lowest at late arrival in the FG1 community [mean ln(mass) + SE = −2.8 + 0.9], followed by FG2 late arrival [mean ln(mass) + SE = −0.2 + 0.5], and with no significant differences among the other treatment combinations [late arrival control mean ln(mass) + SE = 2.1 + 0.2, same arrival FG1 mean ln(mass) + SE = 2.3 + 0.1, same FG2 mean ln(mass) + SE = 3.7 + 0.1, same control mean ln(mass) + SE = 3.6 + 0.2; Figure 1 and Supplementary Tables S2, S3; ANOVA: interaction F = 10.6, P < 0.001].
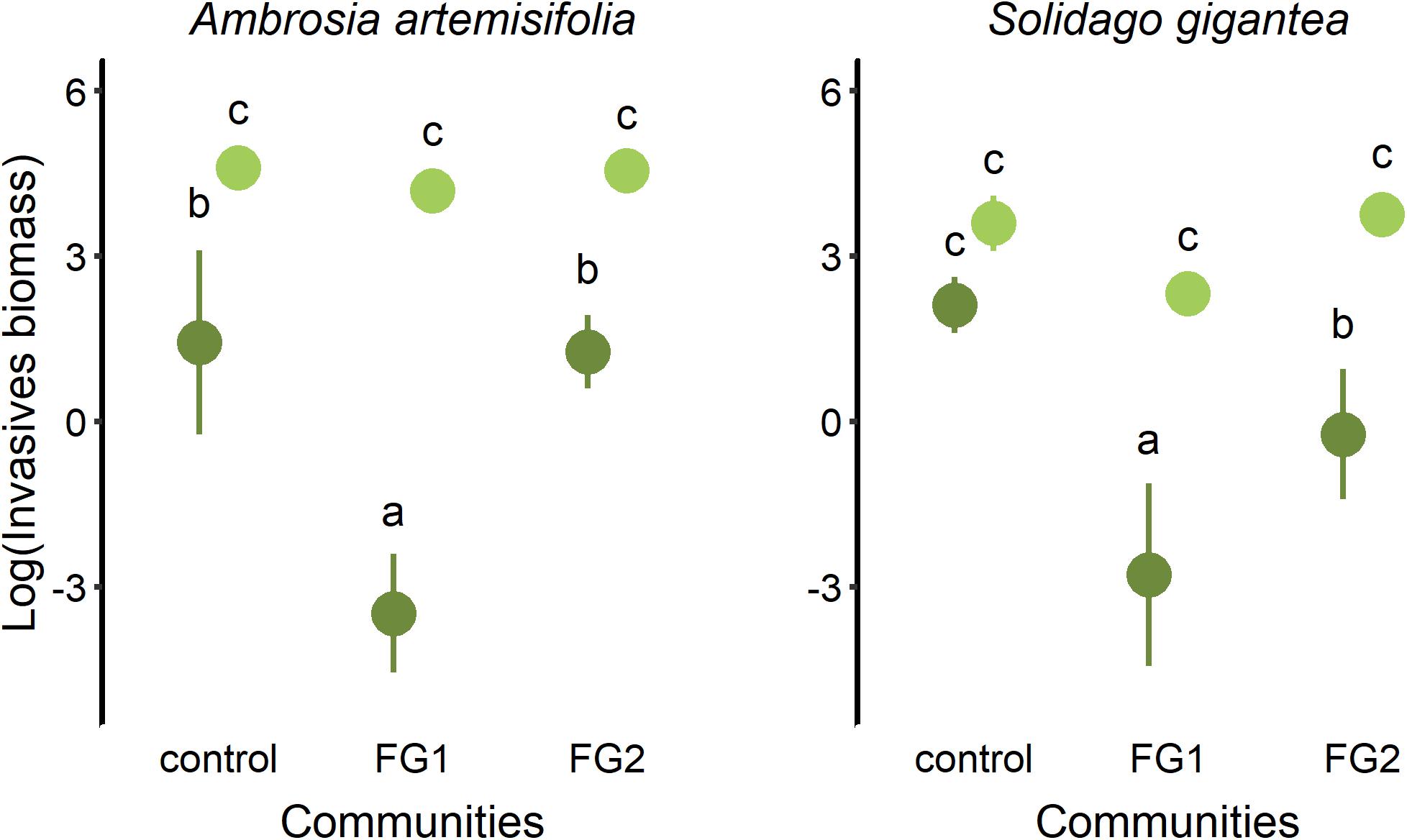
Figure 1. Effect of the community type and time of arrival on biomass of the invasive alien Ambrosia artemisiifolia and Solidago gigantea 8 weeks after sowing (control, monoculture of the invasive plant species; FG1, functional group 1; FG2, functional group 2). Light green symbols indicate simultaneous seeding of native and invasive species, and dark green symbols show results for late arrival of invasive species. ANOVA: interaction FA. artemisiifolia = 19.4, P < 0.001; FS. gigantea = 10.6, P < 0.001; letters indicate significant differences among treatments (P < 0.05).
In terms of CWMTD and CWMPD, FG2 was the community with less average distance to both invaders compared to FG1, confirming our initial calculations for designing the communities (Table 3). When we assessed the effect of the native species biomass and time of arrival on the invasive species, we found that both variables and their interaction significantly affected biomass of A. artemisiifolia, and only time of arrival and the interaction with native biomass for S. gigantea. Specifically, performance of both invasive species decreased as the biomass of the native species increased, when native and invasive were sown at the same time (Figure 2 and Supplementary Table S4; Linear model A. artemisiifolia: adjusted R2 = 0.9, P < 0.0001; Linear model S. gigantea: adjusted R2 = 0.8, P < 0.0001). When the invasive species arrived late, invasive biomass was consistently lower than when arriving at the same time as the natives.
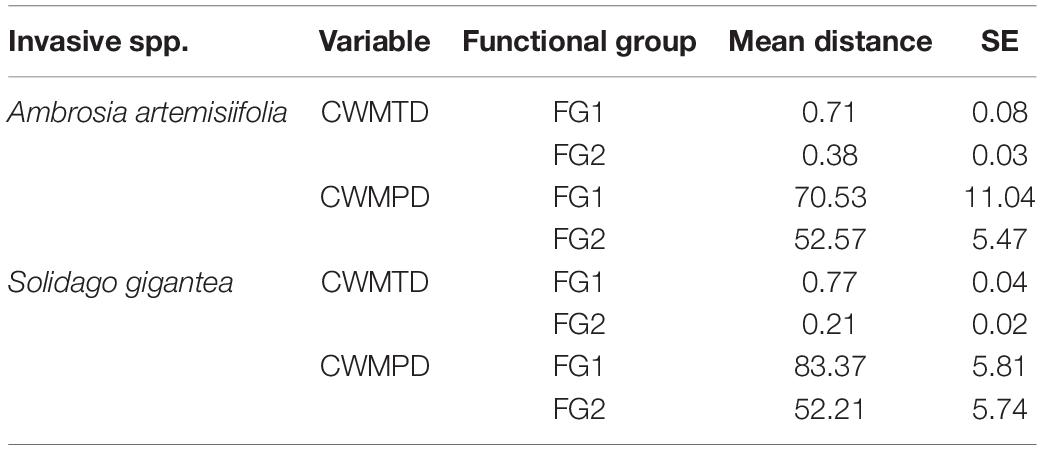
Table 3. Overall native community weighted mean trait (CWMTD ± SE) and phylogenetic (CWMPD ± SE) distances calculated for the two communities (FG1,2) to both invasive alien species (Ambrosia artemisiifolia and Solidago gigantea).
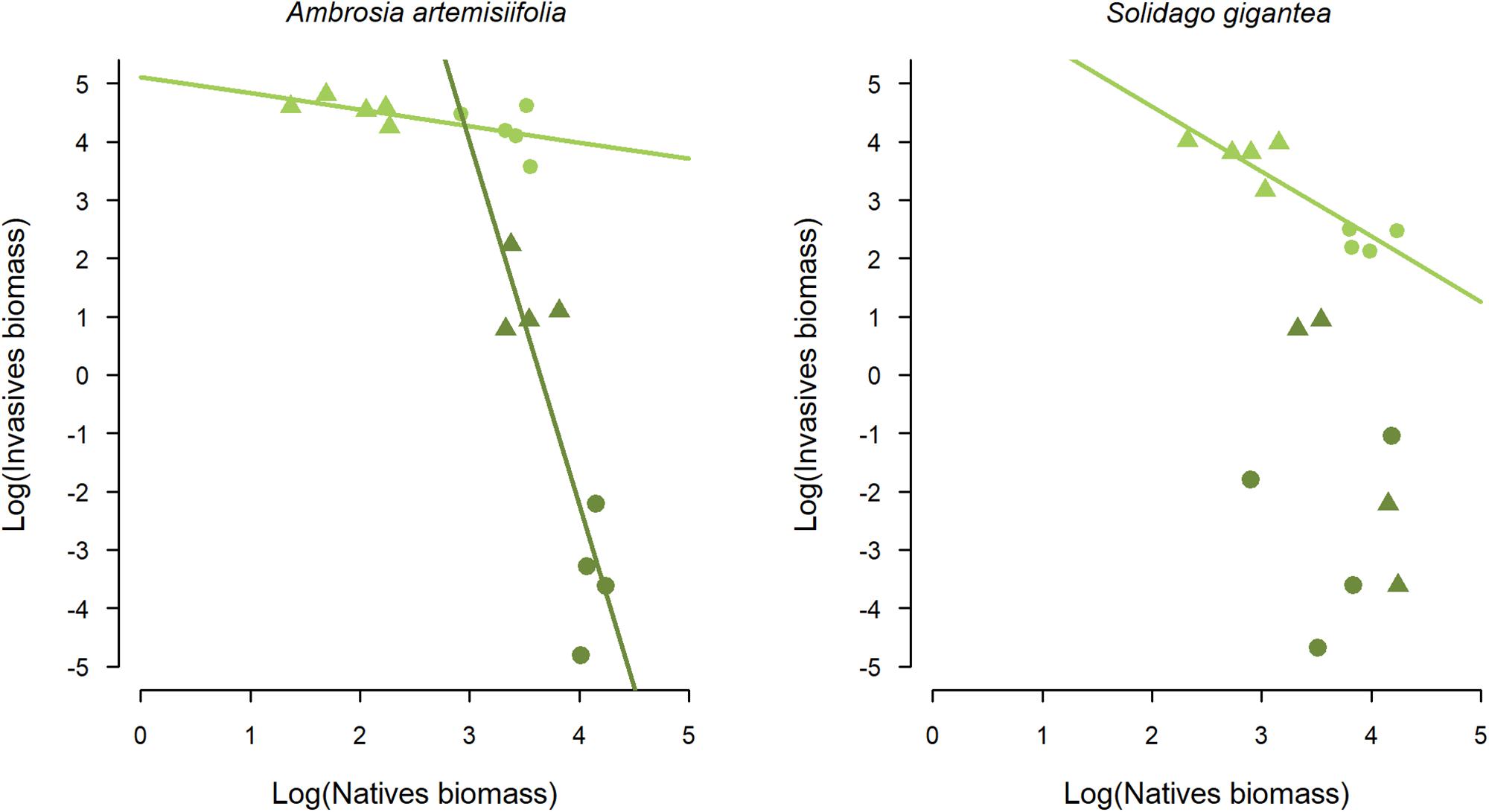
Figure 2. Native species biomass effect on the invasive alien Ambrosia artemisiifolia and Solidago gigantea. Linear model A. artemisiifolia: adjusted R2 = 0.96, P < 0.0001; Linear model S. gigantea: adjusted R2 = 0.91, P < 0.0001. Ln biomass values for the FG1 communities are indicated in dots, with triangles for the FG2 community. Light green symbols indicate simultaneous arrival of native and invasive species, dark green symbols show results for late arrival of the invasive species.
Discussion
This study tested whether using limiting similarity to design communities, priority effects and/or their interactions play a role in the suppression of the invasives A. artemisifolia and S. gigantea during establishment of native grassland communities. With our experimental design and species selection we found evidence for priority effects, with both invasive species disadvantaged (i.e., producing less biomass) by arriving 2 weeks later than the native communities. However, there was no evidence that limiting similarity offered an effective strategy to suppress invasive species, at least in terms of the trait-based design considered in this study. While FG2 was the community considered to be most ecologically similar to both invasive plants, FG1 was more successful in resisting invasion as also observed by Yannelli et al. (2017b). Based on our data, the biomass of the native community was a better predictor of invasive species suppression than CWMTD.
Our results agree with other studies indicating that priority effects can control the establishment of invasive species in plant communities (Dickson et al., 2012; Stuble and Souza, 2016; Delory et al., 2019). For instance, Delory et al. (2019) reported that the invasive species Senecio inaequidens had lower biomass when arriving later into a grassland community. However, contrary to their findings, we found that the strength of the priority effect depends on both the composition of the native community and the identity of the invasive species (Young et al., 2017). Namely, we found that FG1 suppressed both A. artemisifolia and S. gigantea when these species arrived late, while FG2 only exerted a suppression effect on late-arriving S. gigantea, and to a lesser extent than FG1 (Figure 1).
Differences in community effects and invasive species responses may have begun at planting, with differences in resource availability and sowing densities as reflected by seed masses (Table 2). Ambrosia artemisifolia has significantly larger seeds compared to S. gigantea, which has been previously found to result in taller seedlings, allowing them to avoid early competition for light (Yannelli et al., 2017a). However, because sowing quantities for native seed mixtures were calculated as total weights (as it is commonly done in restoration projects), the overall smaller seeds of the species included in FG1 resulted in higher sowing densities compared to FG2. Previous studies have shown a correlation between seed density of seed mixtures and establishment success, later leading to higher biomass production (Yannelli et al., 2017a, 2018; Byun et al., 2020). Indeed, there is evidence that biomass production by the native communities was important in our experiment, with FG1 having more biomass than FG2 in the presence of A. artemisifolia. In contrast, when S. gigantea arrives late, both FG1 and FG2 produced a similar amount of biomass, which may explain why both communities suppressed this invasive species.
Biomass production reflects resource acquisition of plants and can thus be an indicator of niche pre-emption by early arriving individuals. Studies comparing the relative priority effects of native and invasive species generally find that invasives create stronger priority effects, and that these species typically have faster early growth and biomass production than the native species (Delory et al., 2019; Hess et al., 2019). Invasive species, thus, seem to benefit more from early arrival than native species, as these traits allow them to pre-empt more niche space in a shorter time than natives. Likewise, when invasive and native species arrive simultaneously, species that can establish first and quickly produce a canopy to capitalize on the available resources have an advantage compared to slower ones, independently on whether they are native or invasive (Yannelli et al., 2018). In terms of native target communities for restoration, this can be translated in that faster-growing native communities tend be more effective in suppressing invasive species (Byun et al., 2013). Similar findings have been observed in agriculture, with more productive crop mixes capable of greater resource capture and more effective weed suppression (Finney et al., 2016; MacLaren et al., 2019). Therefore, our results suggest that FG1 was more successful than FG2 to suppress both invasive species due to greater niche pre-emption in the 2 weeks before the invasive species were sown.
It has been hypothesized that, for a given amount of biomass, greater niche pre-emption would occur when early- and late-arriving species are ecologically similar (Vannette and Fukami, 2014). Ecologically similar native species would be expected to capture more of the particular resources required by the invasive species due to greater niche overlap, and so would further suppress the invasive species via limiting similarity. We did not find evidence for this based on our definition of functional similarity. In the presence of S. gigantea, biomass production by both native communities was similar, yet the least similar community (FG1) was still more effective at suppressing S. gigantea. This indicates that FG1 may suppress invasive species through other mechanisms in addition to the resource capture, such as allelopathy or modification of the soil biota (Price and Pärtel, 2013). For instance, one of the species dominating FG1, Achillea millefolium L., has been reported to have allelopathic effects (Verma et al., 2017). Nevertheless, with our experimental design we cannot pinpoint the degree that any of these factors would play in this setting.
That FG1 was more effective at suppressing invasive species than FG2 counters our hypothesis that an ecologically more similar community would more effectively suppress the invasive species via limiting similarity. It should be noted, however, that two important issues arose from the a priori trait-based design of the different communities in this study. Firstly, the ecological similarity between the native and invasive species was constrained by the trait space occupied by the native species. The native communities were not highly similar to either invasive species with regard to any particular trait (Table 2), which may have prevented us from detecting an effect of ecological similarity. It has been shown though that some plants may be invasive precisely because they possess traits that native plants do not (Divíšek et al., 2018) and so it may be frequently difficult, if not impossible, to design native communities that are sufficiently similar to invasive species to suppress them. Secondly, the a priori trait-based design of communities in our study led to other differences between FG1 and FG2 apart from their similarity to the invasive species. These included biomass and sowing density, which can also affect invasion suppression, and thus may have confounded or counteracted any effect of ecological similarity (for example, FG1 had a higher biomass than FG2). Thus, our study did not directly test whether ecological similarity reduced invasion, but has led to the perhaps more practical finding that using limiting similarity as a basis to design restoration communities can unintentionally create other vulnerabilities to invasion if, for example, the resulting community produces less biomass.
Furthermore, similarity in resource capture may not be adequately represented by the functional traits selected in our study, or by the use of trait measurements from databases (see discussion in Yannelli et al., 2017a, 2018). It is possible that FG1 was more similar to both invasive species than FG2 in terms of key traits of early growth and resource capture that were not included in the analysis. Fitness inequalities can emerge when certain species within a functional group can make early use of resources, resulting in competitive hierarchies (Hess et al., 2020). In this regard, Yannelli et al. (2017b) showed that suppression could also be explained by competition with the close relative of both invasive species Achillea millefolium. Nevertheless, data from this experiment show that phylogenetic distance differences were highly correlated with trait distance differences, supporting the idea that the traits were not the problem, but rather that other mechanisms might be at play at this stage. One key aspect to consider is that the limiting similarity hypothesis assumes that resources are limited, thus leading to biotic filtering through competition to be the force behind invasive plants suppression. The abiotic conditions of our experiment (e.g., substrate and moisture) may have not portrayed these limiting conditions. If there are still enough available resources, instead of competition-related traits, environmental filters can lead to the success of species with traits associated to an environmental optimum (Funk et al., 2008).
Taken together, our results indicate that the best practice for establishing community resistant to arriving alien invasive species would be to capitalize on priority effects resulting from utilizing early emerging, fast-growing native species at high densities that can quickly develop highly productive communities (Figure 3). It is unclear whether limiting similarity could further increase invasion resistance, but we found it to be an impractical basis for the design of restoration communities. Achieving high native-invader ecological similarity was difficult, and prioritizing ecological similarity at the expense of community biomass is counterproductive. These findings should be considered alongside studies spanning longer terms and located in different environments, as our study provides a snapshot of early growth dynamics between native and invasive species in a controlled environment, which may not predict the long-term outcome of a restoration project.
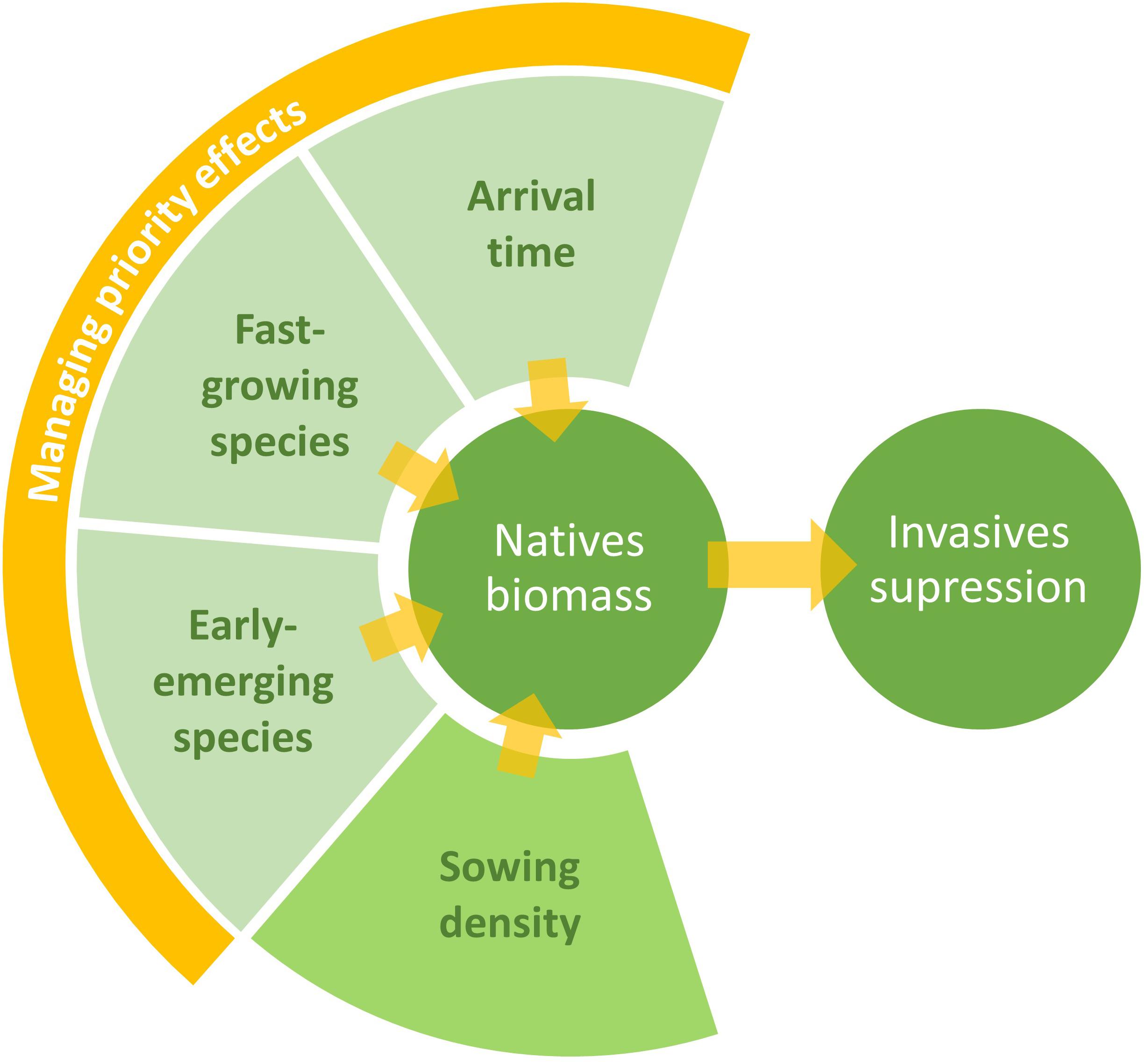
Figure 3. Management recommendations for restoration based on our experiment results. We advise to enhance priority effects when designing seed-mixtures, for instance by selecting early emerging and/or fast-growing native species. Increased native species biomass could also be accomplished by assuring that native species dominate the early community. If possible, the use of high sowing density of natives is also encouraged.
Conclusion and Implications for Managements
The approach taken to designing two functionally similar communities in this study aimed to mimic the knowledge available and constraints experienced by land managers implementing restoration programs. It is unlikely that detailed trait information would be available for all species at a given site, so land managers could rely on trait databases to select species. Armed with this trait information, two key community characteristics that land managers could influence are the composition of the native community and its arrival timing relative to invasive species. We found that managing priority effects to advantage native species can increase suppression of invasive species, but that aiming for trait-based ecological similarity can bias other aspects of the community which may reduce invasion resistance, such as total biomass, functional diversity, and phylogenetic diversity. The practical application of limiting similarity is further constrained by difficulties in assessing ecological overlap, and whether it is possible to compose a sufficiently ecologically similar native community within the trait space occupied by a given set of native species (Price and Pärtel, 2013; Divíšek et al., 2018; Yannelli et al., 2018; Hess et al., 2020).
Thus, restoration strategies focused on ensuring priority effects and fast biomass production could be effective. Although priority effects vary between different native and invasive species, and may thus not always be predictable, there are a variety of options for using priority effects in favor of native species in the field (Young et al., 2017; Hess et al., 2019). Plant traits in restoration communities could also be optimized to enhance priority effects, or other significant ecosystem functions (Ostertag et al., 2015), rather than ecological similarity. Based on our results, we suggest that characteristics such as early emergence and fast development can create priority effects, benefiting natives and suppressing invasive species (Figure 3).
Finally, we note that these results should be cautiously extrapolated to other contexts as field conditions, where abiotic conditions may significantly change the outcome of native-invasive plant interactions due to other factors becoming limiting. In our experiment there were no resource limitations (i.e., soil nutrients and moisture) or disturbances. Other mechanisms might be more important under conditions such as stress due to extended drought in roadsides, or the opposite with very high nutrient inputs in former agricultural lands. However, given that grasslands are highly dynamic through time, our recommendation of favoring priority effects is pertinent for this early stage of active restoration.
Data Availability Statement
The datasets generated during this study are available as Supplementary Information.
Author Contributions
FY and JK conceived the experiment. FY carried out the experiment, collected, and analyzed the data. All authors were discussed and interpreted the results. FY and CM drafted the manuscript, which was improved by contributions of all authors. All authors approved the submitted version.
Funding
FY was funded by scholarship financed by the European Commission through the Erasmus Mundus Arcoiris program, and a research grant from Dr.-Ing. Leonhard-Lorenz-Foundation.
Conflict of Interest
The authors declare that the research was conducted in the absence of any commercial or financial relationships that could be construed as a potential conflict of interest.
Acknowledgments
We thank Johann Krimmer and Gerhard Karrer for providing the seed material. We are also grateful to Wolf-Christian Saul for suggestions on the figures; and Katie Wolcott, Holger Paetsch, Robert Hansel, Ivonne Jüttner, and the staff at Greenhouse Laboratory Centre Dürnast, for technical help. We thank the two reviewers CK and PC-D for their input to improve this manuscript. We acknowledge support by the Open Access Publication Initiative of the Freie Universität Berlin.
Supplementary Material
The Supplementary Material for this article can be found online at: https://www.frontiersin.org/articles/10.3389/fevo.2020.00238/full#supplementary-material
References
Byun, C., de Blois, S., and Brisson, J. (2013). Plant functional group identity and diversity determine biotic resistance to invasion by an exotic grass. J. Ecol. 101, 128–139. doi: 10.1111/1365-2745.12016
Byun, C., de Blois, S., and Brisson, J. (2018). Management of invasive plants through ecological resistance. Biol. Invas. 20, 13–27. doi: 10.1007/s10530-017-1529-7
Byun, C., Oh, M., Lee, E. J., and Kang, H. (2020). Seed density is as important as limiting similarity, diversity effect, and propagule pressure in plant restoration to control invasion. Ecol. Eng. 144:105712. doi: 10.1016/j.ecoleng.2019.105712
Catford, J. A., Smith, A. L., Wragg, P. D., Clark, A. T., Kosmala, M., Cavender-Bares, J., et al. (2019). Traits linked with species invasiveness and community invasibility vary with time, stage and indicator of invasion in a long-term grassland experiment. Ecol. Lett. 22, 593–604. doi: 10.1111/ele.13220
Conradi, T., and Kollmann, J. (2016). Species pools and environmental sorting control different aspects of plant diversity and functional trait composition in recovering grasslands. J. Ecol. 104, 1314–1325. doi: 10.1111/1365-2745.12617
Delory, B. M., Weidlich, E. W. A., Kunz, M., Neitzel, J., and Temperton, V. M. (2019). The exotic species Senecio inaequidens pays the price for arriving late in temperate European grassland communities. Oecologia 191, 657–671. doi: 10.1007/s00442-019-04521-x
Dickson, T. L., Hopwood, J. L., and Wilsey, B. J. (2012). Do priority effects benefit invasive plants more than native plants? An experiment with six grassland species. Biol. Invas. 14, 2617–2624. doi: 10.1007/s10530-012-0257-2
Di-Rienzo, J., Casanoves, F., Balzarini, M., Gonzalez, L., Tablada, M., and Robledo, C. (2013). “InfoStat versión 2013,” in Facultad de Ciencias Agropecuarias (Argentina: InfoStat Group, Universidad Nacional de Córdoba).
Divíšek, J., Chytrý, M., Beckage, B., Gotelli, N. J., Lososová, Z., Pyšek, P., et al. (2018). Similarity of introduced plant species to native ones facilitates naturalization, but differences enhance invasion success. Nat. Commun. 9:4631. doi: 10.1038/s41467-018-06995-4
Essl, F., Biró, K., Brandes, D., Broennimann, O., Bullock, J. M., Chapman, D. S., et al. (2015). Biological Flora of the British Isles: Ambrosia artemisiifolia. J. Ecol. 103, 1069–1098. doi: 10.1111/1365-2745.12424
Fagúndez, J., and Lema, M. (2019). A competition experiment of an invasive alien grass and two native species: are functionally similar species better competitors? Biol. Invas. 21, 3619–3631. doi: 10.1007/s10530-019-02073-y
Finney, D. M., White, C. M., and Kaye, J. P. (2016). Biomass production and Carbon/Nitrogen ratio influence ecosystem services from cover crop mixtures. Agron. J. 108, 39–52. doi: 10.2134/agronj15.0182
Fukami, T. (2015). Historical contingency in community assembly: integrating niches, species pools, and priority effects. Ann. Rev. Ecol. Evol., Syst. 46, 1–23. doi: 10.1146/annurev-ecolsys-110411-160340
Funk, J. L., Cleland, E. E., Suding, K. N., and Zavaleta, E. S. (2008). Restoration through reassembly: plant traits and invasion resistance. Trends Ecol. Evol. 23, 695–703. doi: 10.1016/j.tree.2008.07.013
Gerber, E., Schaffner, U., Gassmann, A., Hinz, H. L., Seier, M., and Müller-Schärer, H. (2011). Prospects for biological control of Ambrosia artemisiifolia in Europe: learning from the past. Weed Res. 51, 559–573. doi: 10.1111/j.1365-3180.2011.00879.x
Helsen, K., Hermy, M., and Honnay, O. (2016). A test of priority effect persistence in semi-natural grasslands through the removal of plant functional groups during community assembly. BMC Ecol. 16:22. doi: 10.1186/s12898-016-0077-9
Hess, M. C. M., Buisson, E., Jaunatre, R., and Mesléard, F. (2020). Using limiting similarity to enhance invasion resistance: theoretical and practical concerns. J. Appl. Ecol. 57, 559–565. doi: 10.1111/1365-2664.13552
Hess, M. C. M., Mesléard, F., and Buisson, E. (2019). Priority effects: emerging principles for invasive plant species management. Ecol. Eng. 127, 48–57. doi: 10.1016/j.ecoleng.2018.11.011
IPBES (2019). Global Assessment Report on Biodiversity and Ecosystem Services of the Intergovernmental Science-Policy Platform on Biodiversity and Ecosystem Services. Bonn: IPBES.
Kiehl, K., Kirmer, A., Donath, T. W., Rasran, L., and Hölzel, N. (2010). Species introduction in restoration projects – Evaluation of different techniques for the establishment of semi-natural grasslands in Central and Northwestern Europe. Basic Appl. Ecol. 11, 285–299. doi: 10.1016/j.baae.2009.12.004
Kleyer, M., Bekker, R. M., Knevel, I. C., Bakker, J. P., Thompson, K., Sonnenschein, M., et al. (2008). The LEDA Traitbase: a database of life-history traits of the Northwest European flora. J. Ecol. 96, 1266–1274. doi: 10.1111/j.1365-2745.2008.01430.x
Klotz, S., Kühn, I., and Durka, W. (2002). BIOLFLOR — Eine Datenbank zu biologisch-ökologischen Merkmalen der Gefäßpflanzen in Deutschland. Bonn: Bundesamt für Naturschutz.
Kowarik, I. (2003). Biologische Invasionen. Neophyten und Neozoen in Mitteleuropa. Stuttgart: Ulmer Verlag.
Laughlin, D. C. (2014). Applying trait-based models to achieve functional targets for theory-driven ecological restoration. Ecol. Lett. 17, 771–784. doi: 10.1111/ele.12288
MacDougall, A. S., Gilbert, B., and Levine, J. M. (2009). Plant invasions and the niche. J. Ecol. 97, 609–615. doi: 10.1111/j.1365-2745.2009.01514.x
MacLaren, C., Swanepoel, P., Bennett, J., Wright, J., and Dehnen-Schmutz, K. (2019). Cover crop biomass production is more important than diversity for weed suppression. Crop Sci. 59, 733–748. doi: 10.2135/cropsci2018.05.0329
Minchin, P. (1987). An evaluation of the relative robustness of techniques for ecological ordination. Vegetatio 69, 89–107. doi: 10.1007/bf00038690
Ostertag, R., Warman, L., Cordell, S., and Vitousek, P. M. (2015). Using plant functional traits to restore Hawaiian rainforest. J. Appl. Ecol. 52, 805–809. doi: 10.1111/1365-2664.12413
Pérez-Harguindeguy, N., Díaz, S., Garnier, E., Lavorel, S., Poorter, H., Jaureguiberry, P., et al. (2013). New handbook for standardised measurement of plant functional traits worldwide. Aust. J. Bot. 61, 167–234. doi: 10.1071/BT12225
Pla, L., Casanoves, F., and Rienzo, J. D. (2012). Quantifying Functional Biodiversity. Berlin: Springer.
Price, J. N., and Pärtel, M. (2013). Can limiting similarity increase invasion resistance? A meta-analysis of experimental studies. Oikos 122, 649–656. doi: 10.1111/j.1600-0706.2012.00121.x
Raunkiaer, C. (1934). The Life Forms of Plants and Statistical Plant Geography. Oxford: Oxford University Press.
Shea, K., and Chesson, P. (2002). Community ecology theory as a framework for biological invasions. Trends Ecol. Evol. 17, 170–176. doi: 10.1016/S0169-5347(02)02495-3
Stuble, K. L., and Souza, L. (2016). Priority effects: natives, but not exotics, pay to arrive late. J. Ecol. 104, 987–993. doi: 10.1111/1365-2745.12583
Vannette, R. L., and Fukami, T. (2014). Historical contingency in species interactions: towards niche-based predictions. Ecol. Lett. 17, 115–124. doi: 10.1111/ele.12204
Verma, R. S., Joshi, N., Padalia, R. C., Goswami, P., Singh, V. R., Chauhan, A., et al. (2017). Chemical composition and allelopathic, antibacterial, antifungal and in vitro acetylcholinesterase inhibitory activities of yarrow (Achillea millefolium L.) native to India. Indus. Crops Products 104, 144–155. doi: 10.1016/j.indcrop.2017.04.046
Wainwright, C. E., and Cleland, E. E. (2013). Exotic species display greater germination plasticity and higher germination rates than native species across multiple cues. Biol. Invas. 15, 2253–2264. doi: 10.1007/s10530-013-0449-4
Wainwright, C. E., Wolkovich, E. M., and Cleland, E. E. (2012). Seasonal priority effects: implications for invasion and restoration in a semi-arid system. J. Appl. Ecol. 49, 234–241. doi: 10.1111/j.1365-2664.2011.02088.x
Weber, E., and Jakobs, G. (2005). Biological flora of central Europe: Solidago gigantea Aiton. Flora 200, 109–118. doi: 10.1016/j.flora.2004.09.001
Westoby, M., Falster, D. S., Moles, A. T., Vesk, P. A., and Wright, I. J. (2002). Plant ecological strategies: some leading dimensions of variation between species. Ann. Rev. Ecol. Syst. 33, 125–159. doi: 10.1146/annurev.ecolsys.33.010802.150452
Wißkirchen, R., and Haeupler, H. (1998). Standardliste der Farn- und Blütenpflanzen Deutschlands. Stuttgart: Ulmer Verlag.
Yannelli, F. A., Hughes, P., and Kollmann, J. (2017a). Preventing plant invasions at early stages of revegetation: the role of limiting similarity in seed size and seed density. Ecol. Eng. 100, 286–290. doi: 10.1016/j.ecoleng.2016.12.001
Yannelli, F. A., Koch, C., Jeschke, J. M., and Kollmann, J. (2017b). Limiting similarity and Darwin’s naturalization hypothesis: understanding the drivers of biotic resistance against invasive plant species. Oecologia 183, 775–784. doi: 10.1007/s00442-016-3798-8
Yannelli, F. A., Karrer, G., Hall, R., Kollmann, J., and Heger, T. (2018). Seed density is more effective than multi-trait limiting similarity in controlling grassland resistance against plant invasions in mesocosms. Appl. Veg. Sci. 21, 411–418. doi: 10.1111/avsc.12373
Young, T. P., Stuble, K. L., Balachowski, J. A., and Werner, C. M. (2017). Using priority effects to manipulate competitive relationships in restoration. Restor. Ecol. 25, 114–123. doi: 10.1111/rec.12384
Keywords: biotic resistance, limiting similarity, priority effects, restoration ecology, revegetation
Citation: Yannelli FA, MacLaren C and Kollmann J (2020) Moving Away From Limiting Similarity During Restoration: Timing of Arrival and Native Biomass Are Better Proxies of Invasion Suppression in Grassland Communities. Front. Ecol. Evol. 8:238. doi: 10.3389/fevo.2020.00238
Received: 21 April 2020; Accepted: 01 July 2020;
Published: 17 July 2020.
Edited by:
Ana Sofia Vaz, University of Granada, SpainReviewed by:
Christoph Kueffer, ETH Zürich, SwitzerlandPilar Castro-Diez, University of Alcalá, Spain
Copyright © 2020 Yannelli, MacLaren and Kollmann. This is an open-access article distributed under the terms of the Creative Commons Attribution License (CC BY). The use, distribution or reproduction in other forums is permitted, provided the original author(s) and the copyright owner(s) are credited and that the original publication in this journal is cited, in accordance with accepted academic practice. No use, distribution or reproduction is permitted which does not comply with these terms.
*Correspondence: Florencia A. Yannelli, florencia.yannelli@tum.de; florenciayannelli@gmail.com