- 1IISER TVM Centre for Research and Education in Ecology and Evolution (ICREEE), School of Biology, Indian Institute of Science Education and Research, Thiruvananthapuram, India
- 2Department of Biology, Ashoka University, Sonepat, India
- 3Centre for Ecological Sciences, Indian Institute of Science, Bengaluru, India
- 4Department of Biology, Lund University, Lund, Sweden
Bees exemplify flights under bright sunlight. A few species across bee families have evolved nocturnality, displaying remarkable adaptations to overcome limitations of their daylight-suited apposition eyes. Phase inversion to nocturnality in a minority of bees that co-exist with diurnal bees provides a unique opportunity to study ecological benefits that mediate total temporal niche shifts. While floral traits and sensory modalities associated with the evolution of classical nocturnal pollination syndromes, e.g. by bats and moths, are well-studied, nocturnality in bees represents a poorly understood, recently invaded, extreme niche. To test the competitive release hypothesis, we examine how nocturnality shapes foraging by comparing pollen loads, nest pollen, and flower visitation of sympatric nocturnal and diurnal carpenter bees. We predicted that nocturnal bees primarily use night-blooming flowers, show little/no resource overlap with diurnal species and competitive release favors night-time pollen collection for provisioning. Contrarily, we found substantial resource overlap between nocturnal and diurnal bees. Flower opening times, floral longevity and plant abundance did not define nocturnal flower use. Smaller pollen loads on nocturnal foragers suggest subsistence on resource leftovers largely from diurnal flowers. Greater pollen types/diversity on nocturnal foragers indicate lower floral constancy compared to diurnal congenerics. Reduced activity during new moon compared to full moon suggests constraints to nocturnal foraging. Invasion and sustenance within the nocturnal niche is characterized by: (i) opportunistic foraging on residual resources as indicated by smaller pollen loads, extensive utilization of day-blooming flowers and substantial overlap with diurnal bees, (ii) generalization at two levels—between and within foraging trips as indicated by lower floral constancy, (iii) reduced foraging on darker nights, indicating visual constraints despite sensitive optics. This together with smaller populations and univoltine breeding in nocturnal compared to multivoltine diurnal counterparts suggest that nocturnality imposes substantial fitness costs. In conclusion, the evolution of nocturnality in bees is accompanied by resource generalization instead of specialization. Reduced floral constancy suggests differences in foraging strategies of nocturnal and diurnal bees which merits further investigation. The relative roles of competition, floral rewards and predators should be examined to fully understand the evolution and maintenance of nocturnality in bees.
Introduction
Partitioning along the time niche axis is an uncommon mechanism for reducing competition or predation avoidance (MacArthur and Levins, 1967; Schoener, 1974; Wiens et al., 1986; Kronfeld-Schor and Dayan, 2003). Temporal niche partitioning usually manifests as within-phase shifts, while complete phase inversions, for example from day to night, are extremely rare with its rarity attributed to evolutionary constraints imposed on extreme modifications in species physiology, anatomy and behavior (Kronfeld-Schor and Dayan, 2003).
Bees are typically day-active and their flights are affected by reduced light levels (Kelber et al., 2006) or the obscuring of solar cues, e.g. during a solar eclipse (Galen et al., 2018). Nocturnality in bees is uncommon, but has evolved repeatedly in several families (Wcislo and Tierney, 2009) and is proposed as a strategy to gain resources from night-blooming flowers (Hopkins et al., 2000; Wcislo et al., 2004; Wcislo and Tierney, 2009), minimize competition or predation (Bohart and Youssef, 1976; Smith et al., 2003; Wcislo et al., 2004) or avoid high temperatures (Gerling et al., 1989; Gottlieb et al., 2005). These hypotheses have not been tested explicitly in detail (but see Wcislo et al., 2004). Bees possess daylight-adapted apposition compound eyes that limit vision under dim-light conditions. That some bees have made the transition to nocturnality is noteworthy, as are investigations into how they function within the nocturnal niche. Some species are facultatively nocturnal (Dyer, 1985; Somanathan et al., 2009b) to exploit a transiently available resource. Best studied amongst dim-light bees are the crepuscular halictid Megalopta genalis in Panama (Greiner et al., 2004a,b; Warrant et al., 2004; Kelber et al., 2006; Warrant, 2008) and the truly nocturnal carpenter bee Xylocopa tranquebarica in India (Somanathan et al., 2008a,b, 2009a, 2019). Recent research on dim-light vision has revealed remarkable anatomical and neurophysiological adaptations in a range of insects, including nocturnal bees (Warrant et al., 2004; Kelber et al., 2006; Somanathan et al., 2008a,b, Warrant and Dacke, 2011; Foster et al., 2017; Narendra and Ramirez-Esquivel, 2017; Stöckl et al., 2017). Dim-light adaptations that contribute to greater sensitivity of apposition eyes include enlarged compound eyes and ocelli, large facet lenses and rhabdoms, slower integration times, wider acceptance angles and lateral branching of the first order neurons (Greiner et al., 2005; Berry et al., 2011; Warrant, 2017). How these dim-light adaptations shape behaviors such as foraging in nocturnal bees is unknown. For example, although color vision even under starlight levels has been reported in the carpenter bee Xylocopa tranquebarica (Somanathan et al., 2008b), the ecological consequences of nocturnality in bees is yet unknown.
The sensory and physiological basis of dim-light flower search in two classical nocturnal pollinators: moths (Heinrich, 1971; Raguso and Pichersky, 1995; Kelber et al., 2002; Raguso and Willis, 2003; Goyret et al., 2008; Okamoto et al., 2008; Kuenzinger et al., 2019; Stöckl and Kelber, 2019) and bats (Heithaus et al., 1975; von Helversen and von Helversen, 1999; Winter et al., 2003; Fleming et al., 2009; Simon et al., 2011) are well understood. Distinct floral traits associated with bat (Baker, 1961; von Helversen and von Helversen, 2003; Fleming et al., 2009) and moth pollination are well studied (Raguso et al., 1996; Svensson et al., 2011), although these pollinators may also opportunistically exploit flowers that do not strictly bear these characteristics (Ollerton et al., 2009; Borges et al., 2016; Borges, 2018). Nocturnal bees co-occur with bats and moths in tropical habitats, but how they find flowers and the floral traits that define their foraging choices are unclear. Are they specialists, generalists or opportunistic foragers? Is foraging limited by moon phase?
We integrate patterns of nocturnal flight activity with floral resource use to comprehensively examine for the first time, what shapes and constrains foraging in nocturnal bees by studying a group of sympatric carpenter bees in India. Xylocopa tranquebarica is truly nocturnal while X. tenuiscapa and X. leucothorax are sympatric diurnal species (Somanathan et al., 2008a, 2019). We evaluate the costs and benefits of nocturnality by examining flight activity across moon phases and resource use and we used population sizes and number of breeding episodes per year (voltinism) as a proxy for fitness across the bee species. We asked the following questions: (A) Does variation in light levels from full moon to new moon impact flight and foraging activity, given that X. tranquebarica is known to be capable of navigation under dim starlight? (B) Does X. tranquebarica largely or exclusively restrict foraging to night-opening flowers and is there significant floral resource partitioning between the nocturnal X. tranquebarica and its diurnal congeneric species X. tenuiscapa and X. leucothorax? (C) Since phase inversion to a nocturnal lifestyle in bees is rare, are there obvious fitness costs associated with nocturnality in X. tranquebarica?
Materials and Methods
This study was carried out during the flowering periods from February–May and November–December 2008 and February–March 2017. This study was conducted in the Bhimashankar Wildlife Sanctuary (19°21’–19°11’N, 73°31’–73°37’E, elevation 900 m), Maharashtra State, in the Western Ghats of India. See Supplementary Section S1a,b for details on the study site and biology of carpenter bees.
Flight Activity
All three species construct nest tunnels inside dead wood of trees (Figure 1). During flowering seasons (2007 and 2008), 12 nests of X. tranquebarica were watched by 2–3 observers using night vision binoculars (US Night Vision, United States) to record departures, returns and flight durations from 1800 to 0600 h during full and new moon phases (± 0–4 nights). Observers positioned themselves at a distance of 1–2 m from nest trees. Tree trunks bearing nests that were within heights of 1 m from the ground were selected for ease of observation in all three bee species making it possible to clearly observe departing and arriving bees. Moreover, the loud buzzing of the bees informed observers about the departure and arrival of bees at the nest site at night. On corresponding dates, such data were also obtained during the daytime at 12 X. tenuiscapa and 10 X. leucothorax nests. For further details see Supplementary Section S1c.
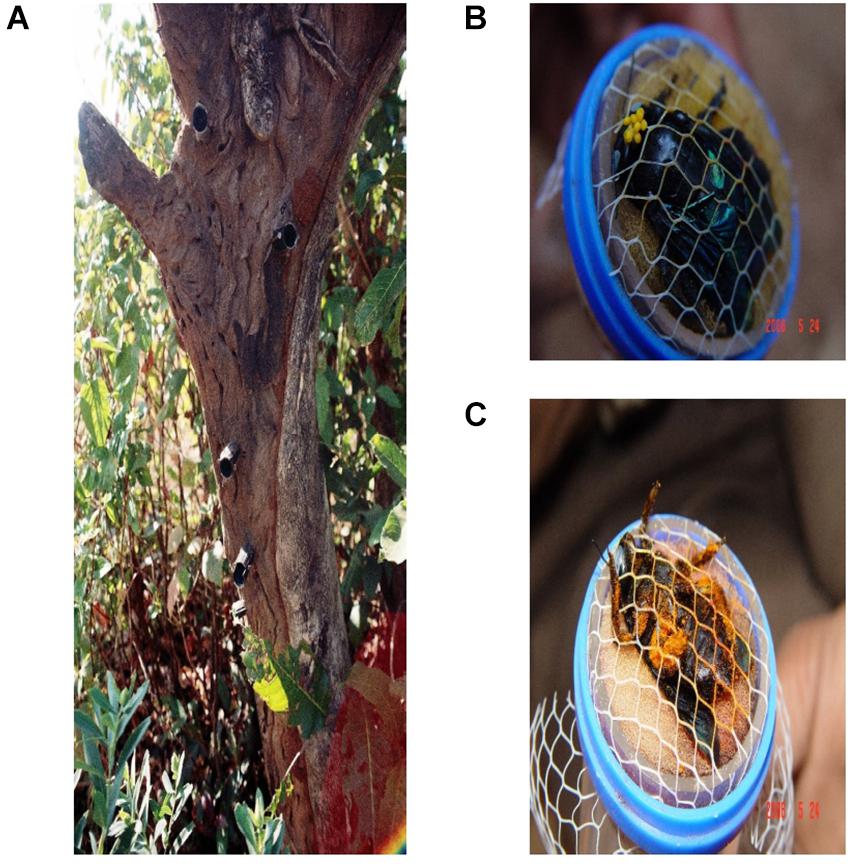
Figure 1. (A) Tree with nests of the nocturnal carpenter bee X. tranquebarica. Pollen traps consisting of opaque plastic tubes lined on the inner surface with sticky tape were placed at nest entrances to strip pollen from returning foragers. (B) The carpenter bee X. tenuiscapa carrying orchid pollinia on its head and (C) tree pollen on its legs.
Pollen Utilization
Bee Pollen Loads
Carpenter bees were captured at nest entrances when they were returning from foraging trips on 12 nights in the nocturnal bee and on 12 corresponding days in the two diurnal bee species. After the bee left the nest to forage, a cotton ball was inserted about an inch inside the nest entrance and a long thin thread which was attached to the cotton ball was allowed to hang freely outside (Figure 1). On arrival, the bee landed on the nest entrance and a plunger was placed over the nest entrance, trapping the bee between the cotton ball and the plunger. By pulling on the thread, the bee was quickly moved into the plunger and covered. At the netted end of the plunger, pollen was extracted from the dorsal and ventral sides and the legs of the bee by thoroughly swabbing with cotton buds dipped into glycerin jelly after which the bees were released (31 individuals per species). Pollen thus harvested were stored in 70% ethanol. By this method we could not completely remove all the pollen but we could harvest as much as possible without injuring the bee. Hence, total pollen counts were not obtained. Instead we estimated pollen numbers and identified pollen types/species on all captured bees. Refer to Supplementary Section S1d for further details of pollen estimation and identification.
Nest Pollen Traps
We used a non-invasive method to assess pollen use by the three bee species by placing traps at nest entrances of the three bee species. Each nest trap consisted of a black plastic tube (3 cm long, 1 cm diameter) attached to the nest entrance of all three species (n = 12–18 nests per bee species) (Figure 1). The inner surface of the trap was lined with sticky tape which stripped small amounts of pollen from returning foragers’ head, thorax abdomen and legs, as they passed through the tube to enter the nest. The traps were replaced at 5-day intervals from February–May and November–December 2007 and stored for later estimation of pollen counts and diversity. The samples thus collected were acetolysed and then stored for identification and counting (for further details see Supplementary Section S1e).
For every plant species i we obtained quantitative measures of pollen use from the nest traps of the three bee species j, which we term as the fractional pollen utilization index (PI), calculated as
Using this method, we compared relative quantitative pollen usage for a given plant species across the three bee species.
Nocturnal Foraging on Night-Blooming H. quadriloculare Flowers
Heterophragma quadriloculare trees (Bignoniaceae) produce white, night-opening flowers that last only one night, and are exclusively pollinated by carpenter bees at night (Somanathan and Borges, 2001). The flowers have touch-sensitive stigmas (TSS) that close rapidly after bee visitation (Somanathan and Borges, 2001; unpublished data). This provided a convenient read-out to score visitation by noting the stigma state (open/closed) in the morning following full-, half-, and new moon nights in 2017. Trees were observed between sunset and sunrise (1800–2100 h, 0000–0300 h, and 0500–0700 h) for 10–30 min per session, resulting in 30–80 observation minutes per tree per night (n = 4–8 trees) in February and March 2017. Nocturnal visits by X. tranquebarica were observed across new moon (n = 5 trees/1 night), half (n = 6–8 trees/4 nights) and full moon phases (n = 8 trees/1 night). Number of flowers visited by X. tranquebarica during each foraging bout (the time an individual spent on the tree from entry to exit) was recorded. The number of flowers visited during a bout was determined by listening to the distinct loud buzzing while in flight which stopped each time a bee entered a flower and resumed when the flower visit was over. These auditory measures have been shown to correspond to visual counts of number of flowers visited at night in H. quadriloculare (Somanathan and Borges, 2001). In the mornings following full and new moon (±5 nights), the TSS states of the stigmas were scored (n = 10 flowers, 4–8 trees).
Community Flower Visitation
Direct observations of flower visits were carried out during flowering months in 2007 and 2008 for 71 plant species (2–3 individuals/species) by 2 or 3 observers from 0600 to 2100 h for one day during peak flowering for each species. Only the identities of flower visitors and their visit timings were recorded. Frequencies could not be obtained as visits were low for most plant species.
Floral Resource Use Networks
Bipartite networks for overall floral use, pollen and nectar use were built (Bipartite package in R (2.9.1), Dormann et al., 2008) from a combination of body pollen loads, nest pollen traps and flower observations of the plant community (n = 71 species) as described above. We constructed three kinds of networks and compared metrics for all three networks.
(i) Overall floral use network: These are qualitative networks of total floral resource use that combine visitor information from direct flower observations, pollen collected and identified from bee bodies and nest traps.
(ii) Pollen network: Pollen resources were identified as those plants whose pollen was collected from bees’ bodies or nest traps. The fractional pollen utilization index (PI; described above) for each bee species was employed to denote interaction strengths for each observed link in a quantitative bipartite network of pollen use.
(iii) Nectar network: Plant species that were visited by a bee species during flower observations (described above), but whose pollen was not recovered from nest traps or from bee bodies were classified as exclusive nectar species. While this method allows us to deduce which plants were visited exclusively for nectar, it was not possible to distinguish plants that were visited for both pollen and nectar.
Indices obtained from the overall visitation network (i above) were compared with 1,000 null models generated with the Patefield algorithm (Patefield, 1981). In these null models, the size of the network along with the total number of interactions (marginal total) was held constant. For the pollen and nectar networks (ii and iii above), the shuffle web function (bipartite package, Dormann et al., 2008), which holds the total number of interactions constant and shuffles the matrix randomly was used. Z-scores were calculated for each index to compare the extent of deviation from randomly generated models. Positive z-values suggest that the mean obtained from null models is lower than the observed values and negative z-values indicate that the mean from null models is higher than the observed index value.
Czekanowski’s index of similarity was used to estimate overlap between bee species in the use of flowers [Vegan package in R (3.1.1), Oksanen et al., 2017].
Influence of Time of Flower Opening and Longevity on Floral Resource Use by Bees
Opening times and longevity of flowers were measured (20–30 flowers from 2 to 3 individuals for 83 plant species). Buds were enclosed in muslin bags and inspected shortly after sunrise between 0600 and 0700 h, in the afternoon (between 1200 and 1300 h) and after sunset (between 1800–2000 h). The morning and evening inspections marked the beginning of the day and the beginning of the night respectively. Accordingly, all plant species were assigned to one of four bins: Diurnal (D) when all flowers opened only during the day time, Largely diurnal (Ld) when 75% of flowers opened during the day and the remaining opened between sunset and sunrise, Nocturnal (N) when all flowers opened at night, and Largely nocturnal (Ln) when 75% of flowers opened at night and the remaining after sunrise. To determine longevity of individual flowers, the bags were opened between 0600–0700 h and 1700–1800 h daily until petals wilted or discolored.
A generalized linear model (GLM) was performed in R (3.1.1) to examine flower utilization (binary response variable obtained by combining body pollen loads, pollen from nest traps and flower visitation observations) in relation to explanatory variables such as bee species, flower opening time, flower longevity, length of flowering season for plant species (which is the number of months a species was observed to flower) and tree species abundance (estimated in transects and classified as low, medium and high; see below for details).
Nest Densities in Diurnal and Nocturnal Carpenter Bees
As a measure of fitness, population sizes were estimated from nest densities in 66 transects (20 × 20 m2) laid randomly in an east–west direction in a 5 km2 area. For each transect we recorded the number of nests of each bee species as well as the abundance of tree species.
Results
Moon Phase, Flight Activity, and Durations
Nocturnal flight activity, measured as the number of exits from nests, was higher on full moon than on new moon nights in X. tranquebarica (Figure 2A; Wilcoxon rank sum test with continuity correction, W = 41.5, p = 0.02; n = 76 for full moon and n = 21 for new moon nights). Flight durations were significantly longer during full moon [Figure 2B; Welch t-test for unequal variance, t = 1.91, p = 0.04, n = 7 full moon exits (from 2 nights) and 46 new moon exits (from 10 nights)]. Flight durations varied significantly between the bee species (Figure 2C; Kruskal-Wallis χ2 = 19.015, d.f. = 2, p < 0.001) and was significantly shorter in the nocturnal X. tranquebarica than the diurnal X. tenuiscapa (Figure 2D; post-hoc pairwise Wilcoxon test with Bonferroni correction, X. tranquebarica–X. tenuiscapa: p < 0.0001, X. tenuiscapa–X. leucothorax: p = 0.37, X. tranquebarica–X. leucothorax: p = 0.19). Fifty percentage of flight trips were between 5 and 10 min in the nocturnal X. tranquebarica, 15 and 20 min in X. leucothorax, and 20 and 25 min in X. tenuiscapa.
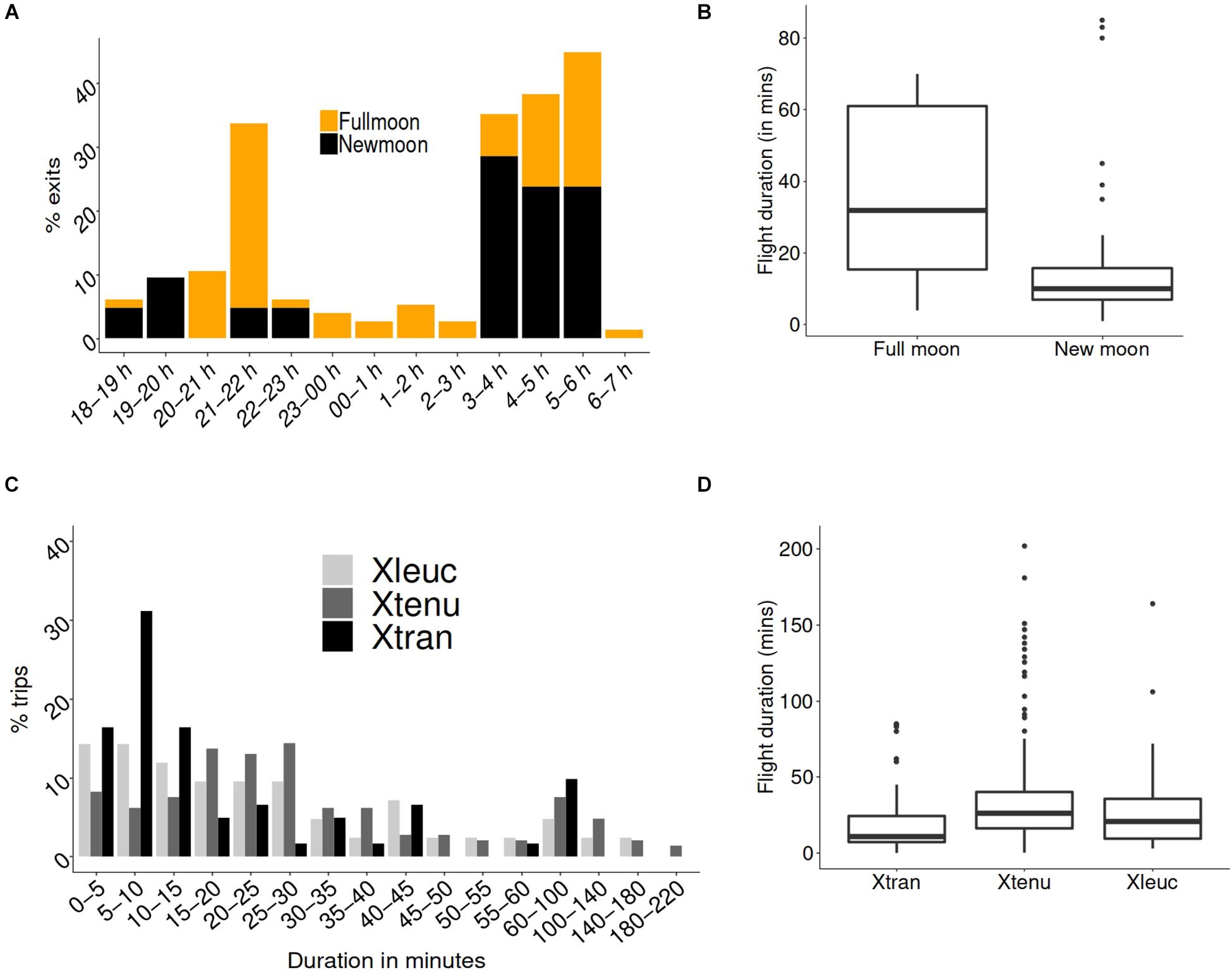
Figure 2. Flight observations at nests of nocturnal (Xtran) and two diurnal (Xtenu, Xleuc) carpenter bee species. (A) Nest observations on full moon nights indicated that X. tranquebarica showed two peaks (between 2100–2200 h and 0300–0600 h) in flight activity, while there was a single broad peak between 0300 and 0600 h on new moon nights. There was no activity between 2300 and 0300 h during new moon, but flight activity persisted during this period during full moon. (B) Box plots indicate that median flight durations in the nocturnal X. tranquebarica were approximately twice as long during full moon compared to new moon nights. (C) Distribution of flight durations in the three carpenter bee species. (D) Boxplots indicate that median flight duration was shorter in the nocturnal X. tranquebarica than in the two diurnal species.
Pollen Load Size and Diversity in Relation to Moon Phase
Pollen loads of individual bees (mean pollen counts) were larger on full moon compared to new moon nights in X. tranquebarica (Figure 3A). The sizes of pollen loads collected on closely corresponding dates (±1–2 days) differed between the three bee species, with the nocturnal bee carrying significantly smaller loads (Figures 3B,C; Kruskal-Wallis χ2 = 72.803, d.f. = 2, p < 0.001). The number of pollen types (plant species) on bodies of individual X. tranquebarica foragers returning to the nest was similar on full moon and new moon nights (Figure 3D; Wilcoxon rank sum test, W = 193.0, p = 0.85). The number of pollen types on individuals differed across bee species (Figure 3E; Kruskal-Wallis χ2 = 53.823, d.f. = 2, p < 0.001); most pollen loads were composed of a single pollen type in the two diurnal bee species, while in the nocturnal bee, pollen loads were predominantly composed of 2–5 species (Figure 3F; Kruskal-Wallis χ2 = 36.90, d.f. = 2, p < 0.001).
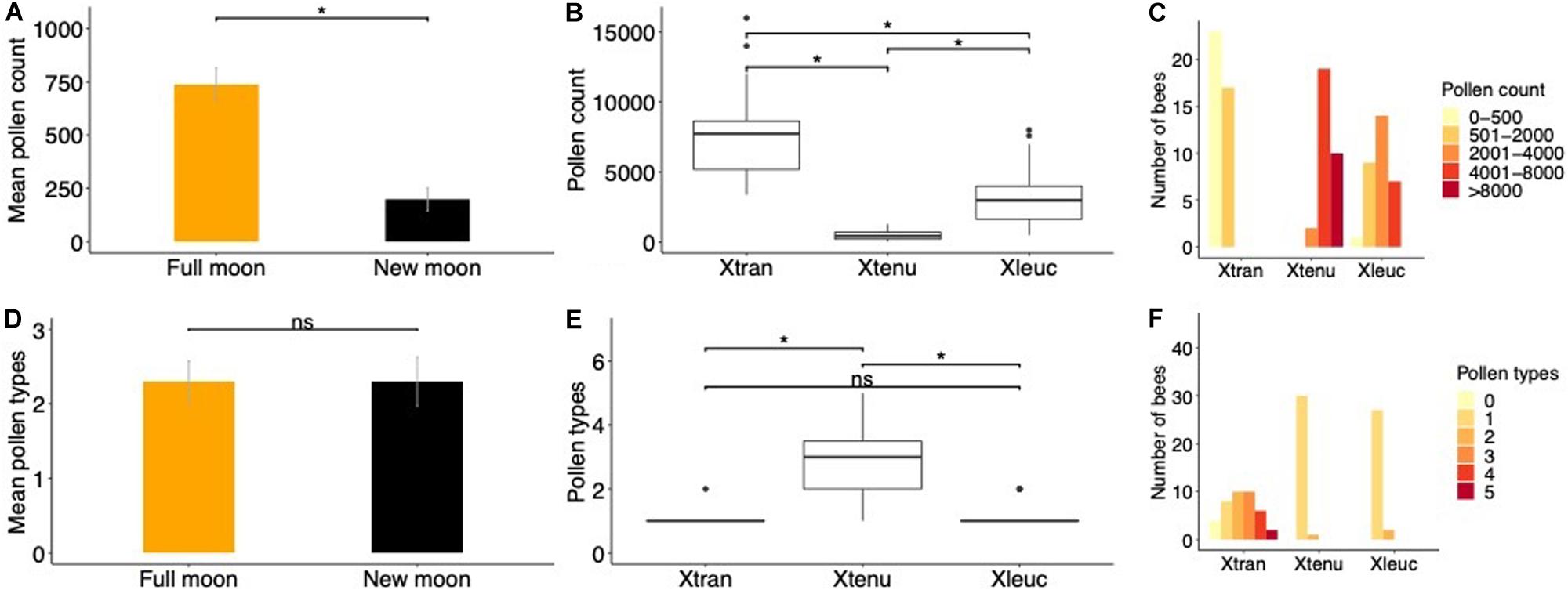
Figure 3. Pollen loads and types collected from bodies of foragers returning to their nests in the nocturnal and diurnal carpenter bee species. (A) Pollen counts (Mean ± SE) from bodies of individuals were significantly larger during full moon compared to new moon nights in the nocturnal X. tranquebarica. (B) Boxplots indicate that median pollen counts were significantly smaller in X. tranquebarica compared to the two diurnal bees, X. tenuiscapa and X. leucothorax. (C) Distribution of pollen counts from the bodies of individual bees in the nocturnal X. tranquebarica and the two diurnal species. (D) Number of pollen types (Mean ± SE) on the bodies of individual bees were similar on full moon and new moon nights in the nocturnal X. tranquebarica. (E) Box plots indicate that median pollen types were higher in X. tranquebarica individuals compared to the diurnal bees. (F) Distribution of pollen types collected from individuals of the three bee species.
Flower Visitation by Nocturnal Bees to H. quadriloculare Flowers in Relation to Moon Phase
The number of flowers visited per foraging bout by X. tranquebarica was higher on full moon compared to the half and new moon phases (Kruskal-Wallis χ2 = 7.45, d.f. = 2, p = 0.02, Supplementary Figure S1A). Post-hoc pairwise comparisons were not possible due to low sample sizes on new moon nights. TSS states were significantly correlated with moon phase, with a higher proportion of stigmas closed following full moon compared to new moon (Kruskal-Wallis χ2 = 9.96, d.f. = 1, p = 0.002; Supplementary Figure S1B).
Community Flower Opening and Longevity
Approximately 70% of the plant community (n = 83 species) had Diurnal (D) or Largely diurnal (Ld) opening, while the remaining had Nocturnal (N) or Largely nocturnal (Ln) opening when binned into these categories (Figure 4A and Supplementary Table S1). Overall flower utilization by combining pollen and flower observation data showed that the nocturnal X. tranquebarica visited both day- (D, Ld) and night-blooming (N, Ln) flowers (Figure 5A), as also indicated by the pollen utilization index (PI; Figure 5C). Proportional flower use between the four categories was similar across bee species (Supplementary Table S2). Over 60% of the plant community produced flowers that lasted ≥2 days (Figure 4B). Flower utilization by bees (overall and PI) and longevity of flowers were unrelated (Figures 5B,D and Supplementary Table S2).
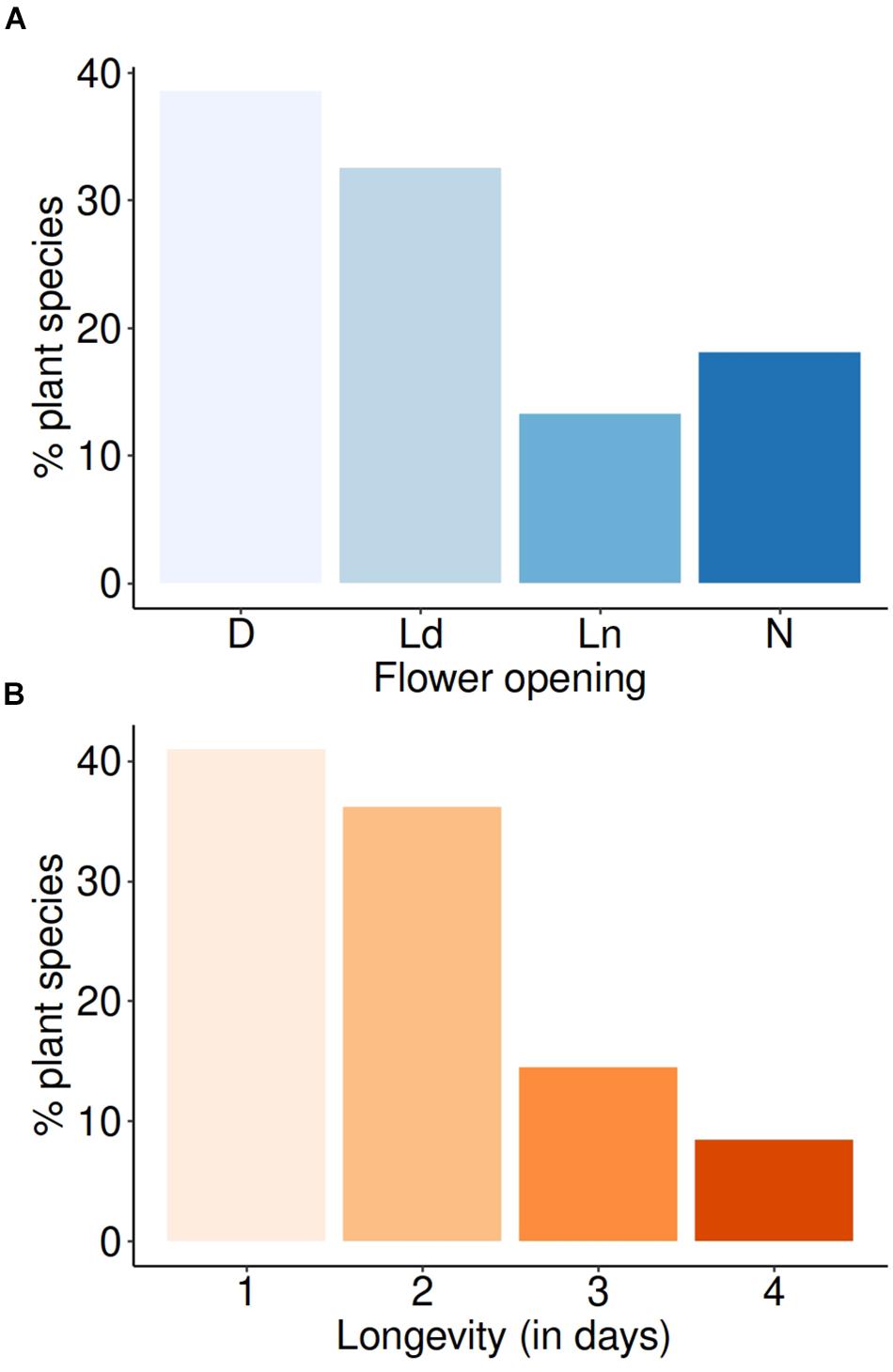
Figure 4. Flower opening times and longevity in the plant community. (A) Distribution of opening times indicated that flowers opened largely during the day in this community. D, diurnal opening; Ld, Largely diurnal opening; Ln, Largely nocturnal opening; N, nocturnal opening. (B) Distribution of flower longevity in this community indicated that more than 60% of plant species produced flowers that lasted 2 or more days.
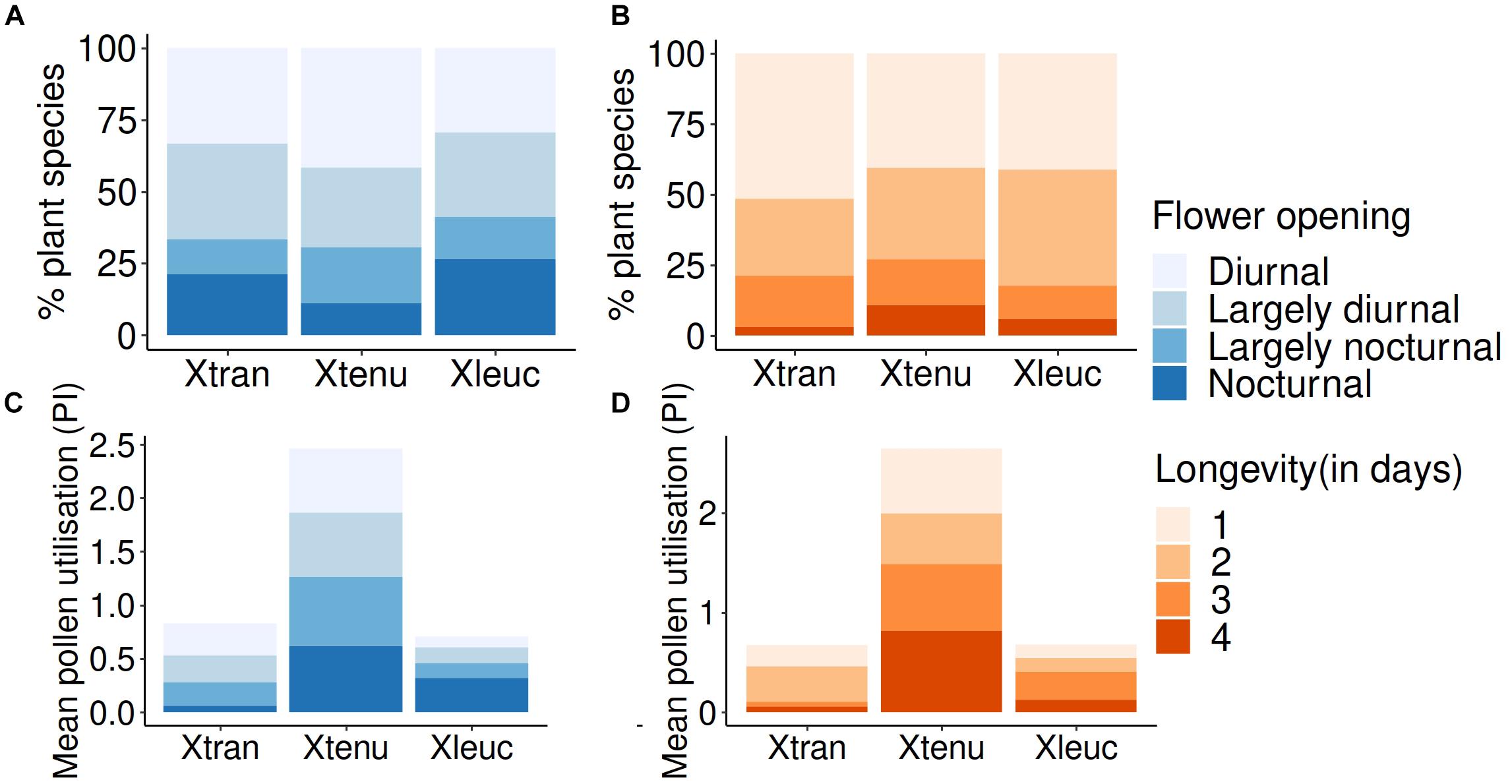
Figure 5. Flower use in carpenter bee species in relation to flower opening times and flower longevity in the plant community. (A) % plant species utilized (pooling body pollen, nest pollen, and flower observation data) suggest that the nocturnal and diurnal bee species used both day-opening and night-opening flowers similarly. (B) % plant species for the similarly pooled data indicated that utilization based on flower longevity was similar for the three bee species. (C) Pollen utilization index (PI) for day-opening and night-opening flower species was similar in the three bee species. (D) PI was also similar for the three bee species irrespective of longevity of flowers. Xtran refers to the nocturnal Xylocopa tranquebarica; Xtenu and Xleuc refer to the diurnal X. tenuiscapa and X. leucothorax.
A GLM with overall flower utilization as a binomial response variable and with stepwise backwards removal of explanatory variables resulted in the best fit, with plant species abundance as the explanatory variable that significantly influenced visitation (Table 1 and Supplementary Figures S2, S3). Fifteen plant species that were highly abundant in the community were visited by the diurnal bee X. leucothorax, while the nocturnal bee visited ten of the highly abundant plant species (Supplementary Figure S2A). In the full model, bee species, flower opening, flower longevity, length of flowering and interaction terms between these variables did not significantly explain floral use (Supplementary Table S3 and Supplementary Figures S3, S4).
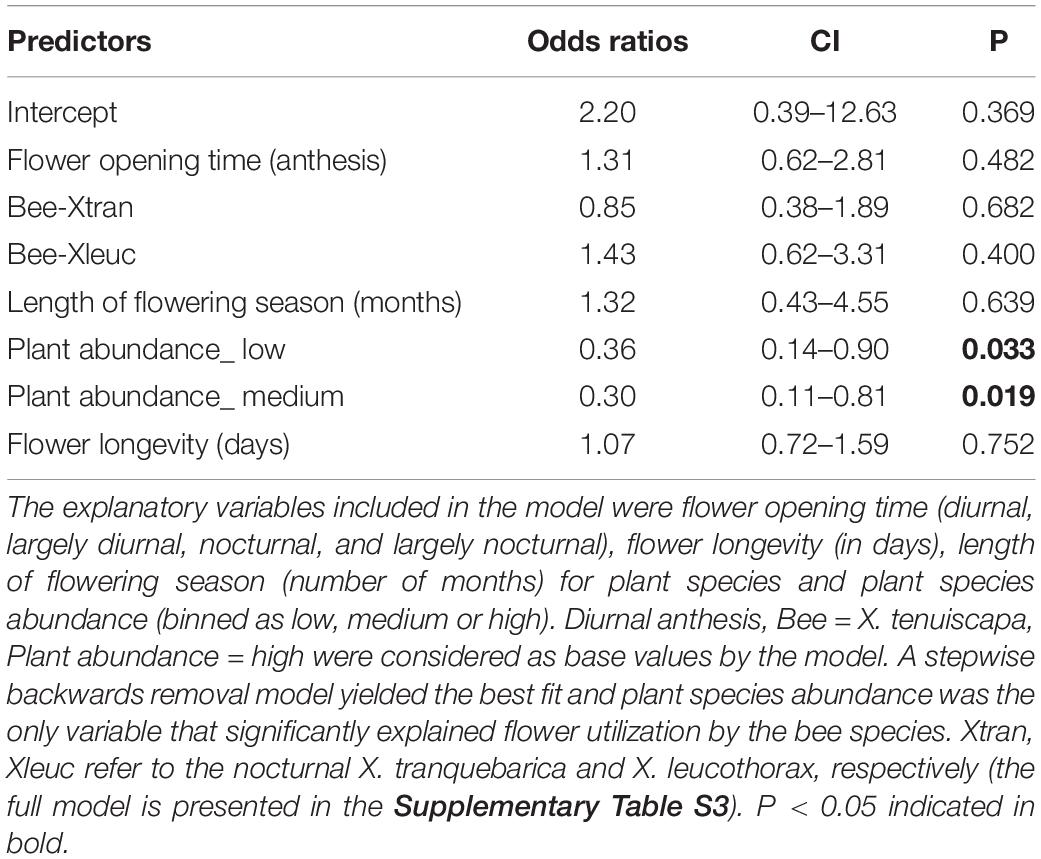
Table 1. Generalized linear model for flower utilization by the three species of carpenter bees using data combined from nest traps, body pollen, and flower observations.
Floral Resource Use Networks
A total of 62 of 71 plant species were visited by at least one bee species (Supplementary Table S1). Bipartite networks, network measures and indices of resource overlap suggest substantial overlap in flower use between the bee species (Figure 6 and Supplementary Tables S4, S5).
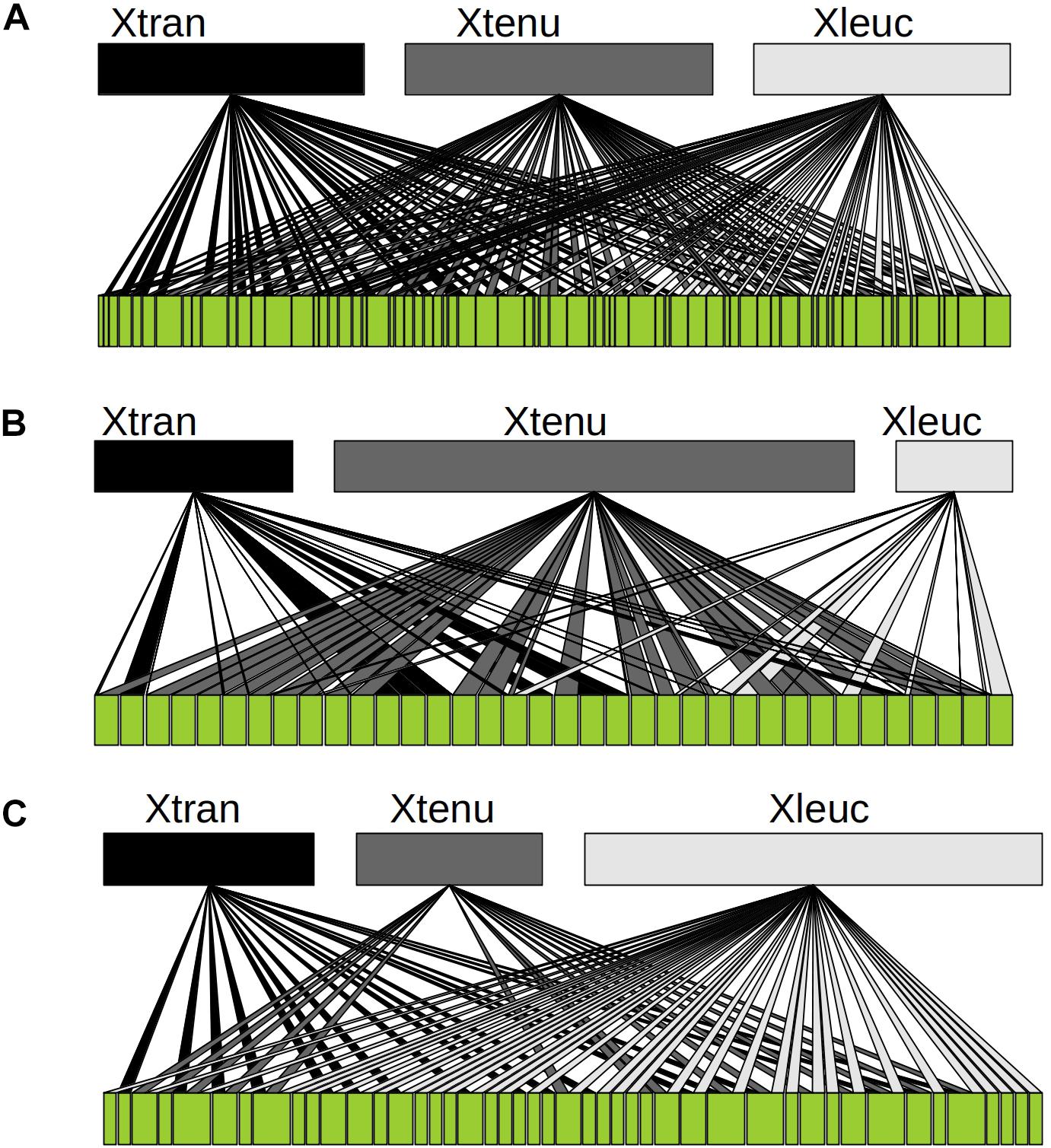
Figure 6. Floral resource use in the three carpenter bee species. (A) Overall bipartite resource use network combining flower visitation observations, body pollen loads and nest pollen for the nocturnal X. tranquebarica (Xtran) and the diurnal X. tenuiscapa (Xtenu) and X. leucothorax (Xleuc). Each line represents an interaction between a bee and a flower species. (B) Quantitative pollen networks constructed using pollen utilization indices (PI) from nest pollen traps of the carpenter bee species. (C) Qualitative nectar utilization network constructed for plants that are potential nectar resources for the three carpenter bee species.
Z scores for degree and normalized degree suggest that the null models were similar to the observed values (Table 2). Z scores for paired differences index in the overall network (PDI; Table 2), which indicates the level of generalization (0–1: generalist to specialist) suggest that the null models were significantly more specialized than the observed PDI. PDI was lowest in X. tranquebarica indicating greater generalization than the other bee species in the overall network. Czekanowski’s index indicated that overlap between pairs of bee species was similar (Supplementary Table S5).
Nest Abundances
Mean number of nests (±SD) per transect differed between the three species (Kruskal-Wallis χ2 = 35.9, p < 0.001). X. tenuiscapa had significantly higher nest densities per plot (14.34 ± 17.33) than the nocturnal X. tranquebarica (3.28 ± 1.59) or X. leucothorax (2.05 ± 2.47; post-hoc pairwise Wilcoxon test with Bonferroni correction for all three species pairs, p < 0.001).
Discussion
We found that resource use by the nocturnal X. tranquebarica is characterized by opportunistic and generalized feeding on day- as well as night-blooming flowers and by substantial overlap with the diurnal congeneric bees. However, foraging activity in the nocturnal bees is affected at very low light levels during moonless nights. Interestingly, the nocturnal bee showed lower floral constancy (for pollen) relative to diurnal bees, suggesting that the nocturnal lifestyle in this bee is accompanied by a change in foraging behaviors involved in the search and detection of flowers.
Recent studies have shown that transitions to nocturnality in bees, as in the paleotropical carpenter bee X. tranquebarica we studied and the neotropical sweat bee Megalopta genalis, are accompanied by remarkable visual adaptations in their apposition eyes (Greiner et al., 2004a,b, Warrant et al., 2004; Theobald et al., 2006; Somanathan et al., 2008b, 2009a, 2019; Warrant, 2008), including color discrimination under dim light (Somanathan et al., 2008b). Though X. tranquebarica performed flights even on new moon nights, we found that flight activity and durations reduced significantly on new moon nights compared to full moon nights. Moreover, flights of the nocturnal bee were shorter than those of the two diurnal species. A recent study on neotropical nocturnal bees reported that light intensity influenced flower visits more than other factors such as temperature, humidity, wind speed and flower availability (Liporoni et al., 2020). Hence we conclude that despite its remarkable extent of nocturnality, flights in X. tranquebarica are constrained on darker nights.
A comparative analysis of resource use by combining flower visitation, forager pollen loads and nest trap data revealed the following: Firstly, pollen loads of the nocturnal X. tranquebarica are smaller than pollen loads of X. tenuiscapa by a factor of 17 and X. leucothorax by a factor of 7, though their body sizes are similar and X. tranquebarica is covered with dense pubescence (greater pollen carrying potential) compared to the diurnal species. Secondly, the number of pollen species/types in pollen loads on individuals was higher in the nocturnal bee compared to the diurnal species, indicating lower flower constancy in the nocturnal bee compared to the diurnal species. This is particularly interesting because diurnal bees are generally known to show flower constancy during foraging bouts (Grant, 1950; Waser, 1986). Moreover, diurnal bees including carpenter bees are known to trap line during foraging bouts by moving between flowering individuals of a species in a fixed sequence (Saleh and Chittka, 2007; Somanathan et al., 2019). However, during a single foraging trip from the nest, the nocturnal X. tranquebarica collected pollen from more flower species than the diurnal species. Constancy and trap lining behaviors are hypothesized to be adaptive behaviors in pollinators leading to greater foraging efficiency or may be attributed to memory constraints (Chittka et al., 1999). From the plant’s perspective, constancy is proposed to result in pollination efficiency by reducing the probability of heterospecific pollen transfer and negative effect on fitness through stigma clogging (Chittka et al., 1999; Morales and Traveset, 2008) which may contribute to loss of paternal fitness due to erroneous pollen placement (Moreira-Hernández and Muchhala, 2019). Our finding opens up avenues for further enquiry on learning, memory and foraging efficiencies of this and other dim-light bee species. Thirdly, the nocturnal bee utilizes day- or night-opening, and short- or long-lived flowers in a community that comprises largely of day-blooming flowers. Finally, we found substantial dietary overlap between the three species. Thus, we conclude that the truly nocturnal X. tranquebarica is a resource generalist, an opportunistic forager on diverse flowers and subsists on partially depleted resources from day-opening flowers. Although the nocturnal X. tranquebarica collected fewer pollen, we do not know whether they also collected lower nectar quantities compared to the diurnal species. Nevertheless, since developing brood crucially depend on stored pollen, the paucity of pollen may have fitness consequences for X. tranquebarica. Indeed, nest densities of X. tranquebarica are lower than in the diurnal X. tenuiscapa and comparable to X. leucothorax. Also X. tranquebarica is univoltine while the two diurnal species are multivoltine (HS pers. obs.) suggesting fitness costs. To determine voltinism, females returning to nests with pollen and presence of brood in a few nests that were opened up through the year were noted.
It is not known when nocturnality evolved in X. tranquebarica, although the Oriental/Palaearctic subgenus Nyctomellita to which it belongs dates back to 33 mya (Leys et al., 2002). The temporal transition to nocturnality in bees is commonly assumed to be related to an escape from competitors and/or enemies and is presumed to have been facilitated by the availability of flowers bearing bat-pollination syndromes (Roulston, 1997; Wcislo and Tierney, 2009). However, the “rich nocturnal resource niche” hypothesis, proposed to explain evolution of nocturnality in bats (Rydell and Speakman, 1995) does not appear to define resource use in X. tranquebarica.
Given the work on visual adaptations in nocturnal bees (Greiner et al., 2004a,b, Warrant et al., 2004; Kelber et al., 2006), we propose several avenues of research that arise from our findings. (1) At the mechanistic level, how does the nocturnal X. tranquebarica detect flowers? Color is an important sensory modality in diurnal bees, though odor, shape, texture, patterns, temperature, etc. are also attractants (Kevan and Lane, 1985; Chittka and Raine, 2006; Dyer et al., 2006; Schiestl and Johnson, 2013; Balamurali et al., 2015). X. tranquebarica can discriminate color landmarks during nocturnal homing (Somanathan et al., 2008b) and they visit flowers with varied display attributes (color, size, and shape) in our site and in Thailand (Burgett et al., 2005), which suggests involvement of varied sensory cues during foraging. Two recent studies have demonstrated that nocturnal halictid bee species belonging to the genus Megalopta are attracted to odor baits (Carvalho et al., 2012; Knoll and Santos, 2012). Furthermore, the lower flower constancy in this species, at least with respect to pollen collection, merits further investigation into how nocturnal bees detect and memorize features of flowers and their spatial locations. Whether nocturnal carpenter bees are capable of trap lining between specific flower locations as has been suggested for diurnal carpenter bees (Somanathan et al., 2019) is unknown. (2) The contribution of nocturnal bees to pollination services at night is unknown. This study indicates that X. tranquebarica is a flower generalist, as are nocturnal sweat bees Megalopta genalis and M. ecuadoria in the neotropics (Wcislo et al., 2004). The role of nocturnal bees in pollination requires wider investigation. This has been studied so far only for one night-blooming species (Somanathan and Borges, 2001). (3) Does operating in the night environment constrain fitness in nocturnal bees? Smaller population size, smaller pollen loads, the flight constraints on darker nights and the univoltine lifecycles in X. tranquebarica suggest fitness costs compared to diurnal conspecifics. (4) If X. tranquebarica largely subsists on residual diurnal floral resources with possible fitness consequences, then why is nocturnality maintained? The answer could lie within the realms of greater competition and higher risk of predation during the daytime for which we have accumulated evidence (unpublished results). The relative roles of competition, rewards and predators in mediating nocturnality in bees remain to be fully understood. Resource use in nocturnal bees must be examined in other geographical locations to confirm if the flight and foraging constraints we found in this site applies to other habitats.
Data Availability Statement
The datasets generated for this study can be found in the online repositories. The names of the repository/repositories and accession number(s) can be found below: https://doi.org/10.5061/dryad.34tmpg4g0.
Author Contributions
HS, AK, and RB formulated the study. HS, SK, and EJ performed the field work. HS and VG analyzed pollen samples. HS and SK analyzed data. HS wrote the manuscript. All authors contributed to the article and approved the submitted version.
Conflict of Interest
The authors declare that the research was conducted in the absence of any commercial or financial relationships that could be construed as a potential conflict of interest.
Acknowledgments
We thank Kalu Kurade, Ganpat Lohkare, and Subhash Vangere for field assistance and the Maharashtra Forest Department for permissions. We acknowledge funding from the Swedish International Development Agency, Wenner-Gren foundation, and intramural funds from IISER Thiruvananthapuram.
Supplementary Material
The Supplementary Material for this article can be found online at: https://www.frontiersin.org/articles/10.3389/fevo.2020.566964/full#supplementary-material
References
Baker, H. G. (1961). The adaptation of flowering plants to nocturnal and crepuscular pollinators. Q. Rev. Biol. 36, 64–73. doi: 10.1086/403276
Balamurali, G. S., Krishna, S., and Somanathan, H. (2015). Senses and signals: evolution of floral signals, pollinator sensory systems and the structure of plant-pollinator interactions. Curr. Sci. 108, 1852–1861.
Berry, R. P., Wcislo, W. T., and Warrant, E. J. (2011). Ocellar adaptations for dim light vision in a nocturnal bee. J. Exp. Biol. 214, 1283–1293. doi: 10.1242/jeb.050427
Bohart, G. E., and Youssef, N. N. (1976). The biology and behavior of Evylaeus galpinsiae Cockerell (Hymenoptera: Halictidae). Wasmann J. Biol. 34, 185–234.
Borges, R. M. (2018). Dark matters: Challenges of nocturnal communication between plants and animals in delivery of pollination services. Yale J. Biol. Med. 91, 33–42.
Borges, R. M., Somanathan, H., and Kelber, A. (2016). Patterns and processes in nocturnal and crepuscular pollination services. Q. Rev. Biol. 91, 389–418. doi: 10.1086/689481
Burgett, M., Sukumalanand, P., and Vorwohl, G. (2005). Pollen species resources for Xylocopa (Nyctomelitta) tranquebarica (F.), a night-flying carpenter bee (Hymenoptera: Apidae) of Southeast Asia. Sci. Asia 31, 65–68.
Carvalho, A. T., Maia, A. C. D., Ojima, P. Y., dos Santos, A. A., and Schlindwein, C. (2012). Nocturnal bees are attracted by widespread floral scents. J. Chem. Biol. 38, 315–318. doi: 10.1007/s10886-012-0084-z
Chittka, L., and Raine, N. E. (2006). Recognition of flowers by pollinators. Curr. Op. Plant Biol. 9, 428–435. doi: 10.1016/j.pbi.2006.05.002
Chittka, L., Thomson, J. D., and Waser, N. M. (1999). Flower constancy, insect psychology, and plant evolution. Naturwissenschaften 86, 361–377. doi: 10.1007/s001140050636
Dormann, C. F., Gruber, B., and Fründ, J. (2008). Introducing the bipartite package: analysing ecological networks. R News. 8, 8–11.
Dyer, A. G., Whitney, H. M., Arnold, S. E., Glover, B. J., and Chittka, L. (2006). Behavioural ecology: bees associate warmth with floral colour. Nature 442:525. doi: 10.1038/442525a
Dyer, F. C. (1985). Nocturnal orientation by the Asian honey bee. Apis dorsata. Anim. Behav. 33, 769–774. doi: 10.1016/s0003-3472(85)80009-9
Fleming, T. H., Geiselman, C., and Kress, W. J. (2009). The evolution of bat pollination: a phylogenetic perspective. Ann. Bot. 104, 1017–1043. doi: 10.1093/aob/mcp197
Foster, J. J., el Jundi, B., Smolka, J., Khaldy, L., Nilsson, D. E., Byrne, M. J., et al. (2017). Stellar performance: mechanisms underlying Milky Way orientation in dung beetles. Phil. Trans. Roy Soc. B 372:20160079. doi: 10.1098/rstb.2016.0079
Galen, C., Miller, Z., Lynn, A., Axe, M., Holden, S., Storks, L., et al. (2018). Pollination on the dark side: Acoustic monitoring reveals impacts of a total solar eclipse on flight behavior and activity schedule of foraging bees. Ann. Entomol. Soc. Am. 112, 20–26. doi: 10.1093/aesa/say035
Gerling, D. H. H., Velthuis, H. H. W., and Hefetz, A. (1989). Bionomics of the large carpenter bees of the genus Xylocopa. Annu. Rev. Entomol. 34, 163–190. doi: 10.1146/annurev.en.34.010189.001115
Gottlieb, D., Keasar, T., Shmida, A., and Motro, U. (2005). Possible foraging benefits of bimodal daily activity in Proxylocopa olivieri (Lepeletier) (Hymenoptera: Anthophoridae). Environ. Entomol. 34, 417–424. doi: 10.1603/0046-225x-34.2.417
Goyret, J., Markwell, P. M., and Raguso, R. A. (2008). Context and scale-dependent effects of floral CO2 on nectar foraging by Manduca sexta. Proc. Natl. Acad. Sci. U.S.A. 105, 4565–4570. doi: 10.1073/pnas.0708629105
Greiner, B., Ribi, W. A., and Warrant, E. J. (2005). A neural network to improve dim-light vision? Dendritic fields of first-order interneurons in the nocturnal bee Megalopta genalis. Cell Tiss. Res. 322, 313–320. doi: 10.1007/s00441-005-0034-y
Greiner, B., Ribi, W. A., and Warrant, E. J. (2004a). Retinal and optical adaptations for nocturnal vision in the halictid bee Megalopta genalis. Cell Tiss. Res. 316, 377–390. doi: 10.1007/s00441-004-0883-9
Greiner, B., Ribi, W. A., Wcislo, W. T., and Warrant, E. J. (2004b). Neural organisation in the first optic ganglion of the nocturnal bee Megalopta genalis. Cell. Tiss. Res. 318, 429–437. doi: 10.1007/s00441-004-0945-z
Heinrich, B. (1971). Temperature regulation of the sphinx moth, Manduca sexta. I. Flight energetics and body temperature during free and tethered flight. J. Exp. Biol. 54, 141–152.
Heithaus, E. R., Fleming, T. H., and Opler, P. A. (1975). Foraging patterns and resource utilization in seven species of bats in a seasonal tropical forest. Ecology 56, 841–854. doi: 10.2307/1936295
Hopkins, M. J. G., Hopkins, H. F., and Sothers, C. A. (2000). Nocturnal pollination of Parkia velutina by Megalopta bees in Amazonia and its possible significance in the evolution of chiropterophily. J. Trop. Ecol. 16, 733–746. doi: 10.1017/s0266467400001681
Kelber, A., Balkenius, A., and Warrant, E. J. (2002). Scotopic colour vision in nocturnal hawkmoths. Nature 419, 922–925. doi: 10.1038/nature01065
Kelber, A., Warrant, E. J., Pfaff, M., Wallén, R., Theobald, J. C., Wcislo, W. T., et al. (2006). Light intensity limits foraging activity in nocturnal and crepuscular bees. Behav. Ecol. 17, 63–72. doi: 10.1093/beheco/arj001
Kevan, P. G., and Lane, M. A. (1985). Flower petal microtexture is a tactile cue for bees. Proc. Natl. Acad. Sci. U.S.A. 82, 4750–4752. doi: 10.1073/pnas.82.14.4750
Knoll, F. R. N., and Santos, L. M. (2012). Orchid bee baits attracting bees of the genus Megalopta (Hymenoptera, Halictidae) in Bauru region, Sao Paulo, Brazil: abundance, seasonality and the importance of odours for dim-light bees. Rev. Bras. Entomol. 56, 481–488. doi: 10.1590/s0085-56262012000400013
Kronfeld-Schor, N., and Dayan, T. (2003). Partitioning of time as an ecological resource. Annu. Rev. Ecol. Evol. Syst. 34, 153–181. doi: 10.1146/annurev.ecolsys.34.011802.132435
Kuenzinger, W., Kelber, A., Weesner, J., Travis, J., Raguso, R. A., and Goyret, J. (2019). Innate colour preferences of a hawkmoth depend on visual context. Biol. Lett. 15:20180886. doi: 10.1098/rsbl.2018.0886
Leys, R., Cooper, S. J., and Schwarz, M. P. (2002). Molecular phylogeny and historical biogeography of the large carpenter bees, genus Xylocopa (Hymenoptera: Apidae). Biol. J. Linn. Soc. 77, 249–266. doi: 10.1046/j.1095-8312.2002.00108.x
Liporoni, R., Cordeiro, G. D., Prado, P. I., Schlindwein, C., Warrant, E. J., and Santos, I. A. (2020). Light intensity regulates flower visitation in Neotropical nocturnal bees. Sci. Rep. 10:15333. doi: 10.1038/s41598-020-72047-x
MacArthur, R., and Levins, R. (1967). The limiting similarity, convergence, and divergence of coexisting species. Am. Nat. 101, 377–385. doi: 10.1086/282505
Morales, C. L., and Traveset, A. (2008). Interspecific pollen transfer: magnitude, prevalence and consequences for plant fitness. Crit. Rev. Plant Sci. 27, 221–238. doi: 10.1080/07352680802205631
Moreira-Hernández, J. I., and Muchhala, N. (2019). Importance of pollinator-mediated interspecific pollen transfer for angiosperm evolution. Annu. Rev. Ecol. Evol. Syst. 50, 191–217. doi: 10.1146/annurev-ecolsys-110218-024804
Narendra, A., and Ramirez-Esquivel, F. (2017). Subtle changes in the landmark panorama disrupt visual navigation in a nocturnal bull ant. Phil. Trans. Roy Soc. B 372:20160068. doi: 10.1098/rstb.2016.0068
Okamoto, T., Kawakita, A., and Kato, M. (2008). Floral adaptations to nocturnal moth pollination in Diplomorpha (Thymelaeaceae). Plant Spec. Biol. 23, 192–201. doi: 10.1111/j.1442-1984.2008.00222.x
Oksanen, J., Blanchet, F. G., Kindt, R., Legendre, P., O’hara, R. B., Simpson, G. L., et al. (2017). Vegan: community ecology package. R package version 3.1.1. https://cran.r-project.Org/web/packages/vegan.html.
Ollerton, J., Alarcón, R., Waser, N. M., Price, M. V., Watts, S., and Cranmer, et al. (2009). A global test of the pollination syndrome hypothesis. Ann. Bot. 103, 1471–1480. doi: 10.1093/aob/mcp031
Patefield, W. M. (1981). Algorithm AS 159: an efficient method of generating R × C tables with given row and column totals. Appl. Stat. 30, 91–97. doi: 10.2307/2346669
Raguso, R. A., Light, D. M., and Pickersky, E. (1996). Electroantennogram responses of Hyles lineata (Sphingidae: Lepidoptera) to volatile compounds from Clarkia breweri (Onagraceae) and other moth-pollinated flowers. J. Chem. Ecol. 22, 1735–1766. doi: 10.1007/bf02028502
Raguso, R. A., and Pichersky, E. (1995). Floral volatiles from Clarkia breweri and C. concinna (Onagraceae): Recent evolution of floral scent and moth pollination. Plant Syst. Evol. 194, 55–67. doi: 10.1007/bf00983216
Raguso, R. A., and Willis, M. A. (2003). “Hawkmoth pollination in Arizona’s Sonoran Desert: behavioral responses to floral traits,” in Butterflies: Evolution and Ecology Taking Flight, eds C. L. Boggs, W. B. Watt, and P. R. Ehrlich (Chicago: University of Chicago Press), 43–65.
Roulston, T. H. (1997). Hourly capture of two species of Megalopta (Hymenoptera: Apoidea; Halictidae) at black lights in Panama with notes on nocturnal foraging by bees. J. Kansas Entomol. Soc. 70, 189–196.
Rydell, J., and Speakman, J. R. (1995). Evolution of nocturnality in bats: potential competitors and predators during their early history. Biol. J. Linn. Soc. 54, 183–191. doi: 10.1111/j.1095-8312.1995.tb01031.x
Saleh, N., and Chittka, L. (2007). Traplining in bumblebees (Bombus impatiens): a foraging strategy’s ontogeny and the importance of spatial reference memory in short-range foraging. Oecologia 151, 719–730. doi: 10.1007/s00442-006-0607-9
Schiestl, F. P., and Johnson, S. D. (2013). Pollinator-mediated evolution of floral signals. Trends Ecol. Evol. 28, 307–315. doi: 10.1016/j.tree.2013.01.019
Schoener, T. W. (1974). Resource partitioning in ecological communities. Science 185, 27–39. doi: 10.1126/science.185.4145.27
Simon, R., Holderied, M. W., Koch, C. U., and von Helversen, O. (2011). Floral acoustics: conspicuous echoes of a dish-shaped leaf attract bat pollinators. Science 333, 631–633. doi: 10.1126/science.1204210
Smith, A. R., Wcislo, W. T., and O’Donnell, S. (2003). Assured fitness returns favor sociality in a mass provisioning sweat bee, Megalopta genalis (Hymenoptera: Halictidae). Behav. Ecol. Sociobiol. 54, 14–21. doi: 10.1007/s00265-003-0589-0
Somanathan, H., and Borges, R. M. (2001). Nocturnal pollination by the carpenter bee Xylocopa tenuiscapa (Apidae) and the effect of floral display on fruit set of Heterophragma quadriloculare (Bignoniaceae) in India. Biotropica 33, 78–89. doi: 10.1111/j.1744-7429.2001.tb00159.x
Somanathan, H., Borges, R. M., Warrant, E. J., and Kelber, A. (2008a). Nocturnal bees learn landmark colours in starlight. Curr. Biol. 18, R996–R997.
Somanathan, H., Borges, R. M., Warrant, E. J., and Kelber, A. (2008b). Visual ecology of Indian carpenter bees I: Light intensities and flight activity. J. Comp. Physiol. A 194, 97–107. doi: 10.1007/s00359-007-0291-1
Somanathan, H., Kelber, A., Borges, R. M., Wallén, R., and Warrant, E. J. (2009a). Visual ecology of Indian carpenter bees II: adaptations of eyes and ocelli to nocturnal and diurnal lifestyles. J. Comp. Physiol. A 195, 571–583. doi: 10.1007/s00359-009-0432-9
Somanathan, H., Warrant, E. J., Borges, R. M., Wallén, R., and Kelber, A. (2009b). Resolution and sensitivity of the eyes of the Asian honeybees Apis florea, Apis cerana and Apis dorsata. J. Exp. Biol. 212, 2448–2453. doi: 10.1242/jeb.031484
Somanathan, H., Saryan, P., and Balamurali, G. S. (2019). Foraging strategies and physiological adaptations in large carpenter bees. J. Comp. Physiol. 29(10), 3496–3502 doi: 10.1007/s00359-019-01323-7
Stöckl, A. L., and Kelber, A. (2019). Fuelling on the wing: sensory ecology of hawkmoth foraging. J. Comp. Physiol. A 205, 399–413.
Stöckl, A. L., Kihlström, K., Chandler, S., and Sponberg, S. (2017). Comparative system identification of flower tracking performance in three hawkmoth species reveals adaptations for dim light vision. Phil. Trans. Roy Soc. B 372:20160078. doi: 10.1098/rstb.2016.0078
Svensson, G. P., Pellmyr, O., and Raguso, R. A. (2011). Pollinator attraction to volatiles from virgin and pollinated host flowers in a yucca/moth obligate mutualism. Oikos 120, 1577–1583. doi: 10.1111/j.1600-0706.2011.19258.x
Theobald, J. C., Greiner, B., Wcislo, W. T., and Warrant, E. J. (2006). Visual summation in night-flying sweat bees: a theoretical study. Vis. Res. 46, 2298–2309. doi: 10.1016/j.visres.2006.01.002
von Helversen, D., and von Helversen, O. (1999). Acoustic guide in a bat-pollinated flower. Nature 398, 759–760. doi: 10.1038/19648
von Helversen, D., and von Helversen, O. (2003). Object recognition by echolocation: a nectar-feeding bat exploiting the flowers of a rain forest vine. J. Comp. Physiol. A 189, 327–336. doi: 10.1007/s00359-003-0405-3
Warrant, E., and Dacke, M. (2011). Vision and visual navigation in nocturnal insects. Annu. Rev. Entomol. 56, 239–254. doi: 10.1146/annurev-ento-120709-144852
Warrant, E. J. (2008). Seeing in the dark: vision and visual behaviour in nocturnal bees and wasps. J. Exp. Biol. 211, 1737–1746. doi: 10.1242/jeb.015396
Warrant, E. J. (2017). The remarkable visual capacities of nocturnal insects: vision at the limits with small eyes and tiny brains. Phil. Trans. Roy Soc. B 372:20160063. doi: 10.1098/rstb.2016.0063
Warrant, E. J., Kelber, A., Gislén, A., Greiner, B., Ribi, W., and Wcislo, W. T. (2004). Nocturnal vision and landmark orientation in a tropical halictid bee. Curr. Biol. 14, 1309–1318. doi: 10.1016/j.cub.2004.07.057
Waser, N. M. (1986). Flower constancy: definition, cause, and measurement. Am. Nat. 127, 593–603. doi: 10.1086/284507
Wcislo, W. T., Arneson, L., Roesch, K., Gonzalez, V., Smith, A., and Fernández, H. (2004). The evolution of nocturnal behaviour in sweat bees, Megalopta genalis and ecuadoria (Hymenoptera: Halictidae): an escape from competitors and enemies?. Biol. J. Linn. Soc. 83, 377–387. doi: 10.1111/j.1095-8312.2004.00399.x
Wcislo, W. T., and Tierney, S. M. (2009). Behavioural environments and niche construction: the evolution of dim-light foraging in bees. Biol. Rev. 84, 19–37. doi: 10.1111/j.1469-185x.2008.00059.x
Wiens, J. A., Rotenberry, J. T., and Van Horne, B. (1986). A lesson in the limitations of field experiments: shrub steppe birds and habitat alteration. Ecology 67, 365–376. doi: 10.2307/1938579
Keywords: carpenter bees, floral rewards, floral constancy, nocturnality, nocturnal niche, nocturnal pollination networks, pollination syndromes, specialization
Citation: Somanathan H, Krishna S, Jos EM, Gowda V, Kelber A and Borges RM (2020) Nocturnal Bees Feed on Diurnal Leftovers and Pay the Price of Day – Night Lifestyle Transition. Front. Ecol. Evol. 8:566964. doi: 10.3389/fevo.2020.566964
Received: 29 May 2020; Accepted: 13 August 2020;
Published: 03 November 2020.
Edited by:
Renoult P. Julien, UMR 5175 Centre d’Ecologie Fonctionnelle et Evolutive (CEFE), FranceCopyright © 2020 Somanathan, Krishna, Jos, Gowda, Kelber and Borges. This is an open-access article distributed under the terms of the Creative Commons Attribution License (CC BY). The use, distribution or reproduction in other forums is permitted, provided the original author(s) and the copyright owner(s) are credited and that the original publication in this journal is cited, in accordance with accepted academic practice. No use, distribution or reproduction is permitted which does not comply with these terms.
*Correspondence: Hema Somanathan, aHNvbWFuYXRoYW5AaWlzZXJ0dm0uYWMuaW4=
†Present address: Elsa M. Jos, Department of Biology, Utah State University, Logan, UT, United States; Vishwas Gowda, Department of Neuroscience, University of Arizona, Tucson, AZ, United States