- 1Programa de Pós-Graduação em Ecologia Aplicada, Departamento de Ecologia e Conservação, Universidade Federal de Lavras, Lavras, Brazil
- 2Programa de Pós-Graduação em Ecologia, Conservação e Manejo da Vida Silvestre (ECMVS), Universidade Federal de Minas Gerais, Belo Horizonte, Brazil
- 3Programa de Pós-Graduação em Biodiversidade e Uso dos Recursos Naturais (BURN), Universidade Estadual de Montes Claros, Montes Claros, Brazil
- 4Department of Biological Sciences, Columbian College of Arts and Sciences, The George Washington University, Washington, DC, United States
- 5Departamento de Genética, Ecologia e Evolução, Instituto de Ciências Biológicas, Universidade Federal de Minas Gerais, Belo Horizonte, Brazil
Ecological communities vary considerably in space and time and understanding such changes has fundamental relevance for ecology and conservation sciences. Mountains provide an excellent scenario for studies addressing spatial and temporal variation, as they vary in conditions and resources in a small geographic region. Here, we aimed to understand the patterns of variation in ant metacommunity composition across time and along an elevational gradient in a tropical mountain, focusing both on the taxonomic and functional facets of diversity. We used a β-diversity metric and broke it into nestedness and turnover to estimate short-term temporal changes in ant metacommunity composition. We tested the following hypotheses: (i) taxonomic and functional temporal β-diversity increase along the elevational gradient and (ii) turnover is the main component driving taxonomic temporal β-diversity and nestedness for functional temporal β-diversity. Rejecting our first hypothesis, we found that both taxonomic and functional temporal β-diversity did not increase with elevation. Yet, the values were always high, indicating that both species and functional traits are highly variable over time. In accordance with our second hypothesis, we found that turnover was the main component of taxonomic β-diversity. Yet adding complexity to our hypothesis, the contribution of nestedness to functional β-diversity decreased with increasing elevation. These results suggest that at low elevations, the turnover in species composition may then cause changes in trait composition because of the loss of some traits, yet preserving the most common functions (nested functional communities), while at high elevations functional capabilities may change over time (turnover of traits). In the context of global warming, where tropical mountain insects are expected to change their distributional range upwards, it is extremely important to consider the importance of the turnover on the temporal variation in functional traits and functions of ant metacommunity at higher elevations.
Introduction
Understanding how communities are structured in space and time has fundamental relevance in ecology and conservation science (Gaston, 2000; Sutherland et al., 2013). There is abundant evidence in ecological studies that the spatio-temporal patterns we see in nature cannot be disentangled (Schiesari et al., 2019), as individuals and species can move both through space and time, forming metapopulations (Levins, 1969) and metacommunities (Leibold et al., 2004). Knowledge of complex spatial metacommunity dynamics have been advanced by using diversity partitioning analyses (e.g., Bishop et al., 2015; Heino et al., 2015), that partition diversity into local (α), and regional (γ) components, as well as the change among local communities (β) (Crist et al., 2003). However, most studies of this kind are based on one or a few samples in time, creating a gap in our knowledge of the importance of temporal variation of individuals and species in the structuring of metapopulations and metacommunities (Datry et al., 2016; Ruhí et al., 2017). In a changing world (Lewis and Maslin, 2015), there is an urgent need to understand the spatial and temporal distribution of diversity and the underlying mechanisms of these patterns, so we can predict and mitigate the effects of global change on biodiversity, ecosystem functions, and associated services (e.g., Legendre and Condit, 2019).
Since the classic work of von Humboldt and Bonpland (1805), numerous studies have investigated species distributions and the underlying mechanisms of these patterns along spatial and environmental gradients (e.g., Peters et al., 2016). Mountains are central to these studies, because they have significant environmental gradients in a relatively small geographical area (Körner, 2007). The small spatial scale means that all regional species can potentially access the whole gradient, minimizing the effects of the kinds of dispersal limitations seen over larger geographical areas (Longino and Colwell, 2011). Yet, species distribution patterns vary along mountains, with most taxa showing a decline in diversity with increasing elevation and associated changes in species composition (Fernandes et al., 2016; Perillo et al., 2017; Mota et al., 2018; Li et al., 2019). In this context, β-diversity metrics are useful for understanding how species composition changes across habitats or elevations and also attempt to reveal the assembly mechanisms that drive these differences (Bishop et al., 2015; Castro et al., 2019). Differences in habitat and resource use among species determine the spatial structure and maintenance of the β-diversity in mountains, where species turnover among elevations is the dominant component driving taxonomic spatial β-diversity of plants (Mota et al., 2018), birds (Li et al., 2019), termites (Nunes et al., 2017), dung beetles (Nunes et al., 2016), ants (Castro et al., 2020), and benthic invertebrates (Castro et al., 2019).
The abrupt spatial and temporal environmental changes in mountains provide a good experimental setting to study spatio-temporal dynamics of metacommunities. However, most studies on patterns of species and community distributions in mountains focus on the spatial rather than on the temporal dimension (e.g., Fernandes et al., 2016; Lasmar et al., 2020). Yet, the temporal variation in climatic conditions can be just as strong as the spatial variation, regulating plant resource availability and patterns of animal foraging in seasonal tropical systems (e.g., Basset et al., 2015; Costa et al., 2018; Novais et al., 2019). While the temporal variation in climatic conditions that is driven by seasonal variation could be similar to the variation found at different elevations in mountains (Rocha et al., 2016), it is less clear which drivers shape temporal β-diversity of communities on mountains. We have evidence that although spatial taxonomic diversity at the regional scale (γ-diversity) is mainly caused by differences in species compositions of local communities (β-diversity component), the functional regional diversity (γ-diversity) is mainly driven by patterns of local diversity (α-diversity component; species composition changes along the elevational gradient, but functions do not; Nunes et al., 2016; Castro et al., 2020). In contrast, there is a lack of information on how temporal variation structures communities that are subjected to different climatic conditions at different elevations, both taxonomically and functionally. In other words, we need to explicitly address how the temporal taxonomic and functional β-diversity within metacommunities vary in space, i.e., across the elevational gradient.
In this study, we explored the spatio-temporal dynamics of metacommunities in tropical mountains by investigating how communities respond to temporal variation in environmental conditions on an elevational gradient in south-eastern Brazil. We collected taxonomic and functional information on ant metacommunity quarterly over 3 years, totalling 12 temporal samplings at each of seven different elevations. We used ants (Hymenoptera: Formicidae) as a focal taxon, because they respond rapidly to changes in environmental conditions, both spatially (Castro et al., 2020) and temporally (Bishop et al., 2014) and perform important ecological functions such as nutrient cycling and seed dispersal (Farji-Brener and Werenkraut, 2017; Magalhães et al., 2018). We investigated how temporal taxonomic and functional β-diversity of ants vary along the elevation gradient and how turnover and nestedness contribute to overall temporal β-diversity. We tested the following hypotheses: (i) the temporal taxonomic and functional β-diversity increase with increasing elevation along the gradient (Figure 1A); and (ii) turnover is the main component driving taxonomic temporal β-diversity and nestedness for temporal functional β-diversity (Figure 1B). The first hypothesis predicts an increase in temporal β-diversity with increasing elevation because temporal variation in the environment is more pronounced at high elevations than at low elevations. Harsh climatic conditions, climatic instability, and lower resource availability (productivity) at higher elevations are therefore likely to favor higher taxonomic and functional variation of the metacommunity over time [e.g., Costa et al. (2018) for temporal variation in ant species activity among seasons]. With decreasing habitat heterogeneity and resource availability (e.g., during the dry season), we expect to find communities of ants with longer legs, since these communities would be composed mainly by generalist and predator species that usually walk longer distances to forage (Lenoir et al., 2009; Bishop et al., 2016; Fichaux et al., 2019). Accordingly, the communities would be composed mainly by species with longer mandibles, usually found in omnivore generalist and predator species (Gibb and Cunningham, 2013; Bishop et al., 2015). In addition, in harsher environments or seasons, we would expect higher polymorphism in ant colonies, because this would help colonies to deal with temperature and humidity variation through labor division (Lenoir et al., 2009). The second hypothesis predicts a higher contribution of turnover than nestedness to temporal taxonomic β-diversity, following the spatial pattern found for mountain insects (Nunes et al., 2017; Perillo et al., 2017; da Silva et al., 2018). Moreover, it also predicts a lower contribution of turnover compared to nestedness for temporal functional β-diversity, with communities showing a functional redundancy over time, as demonstrated spatially for insects in mountains (Bishop et al., 2015; Castro et al., 2020).
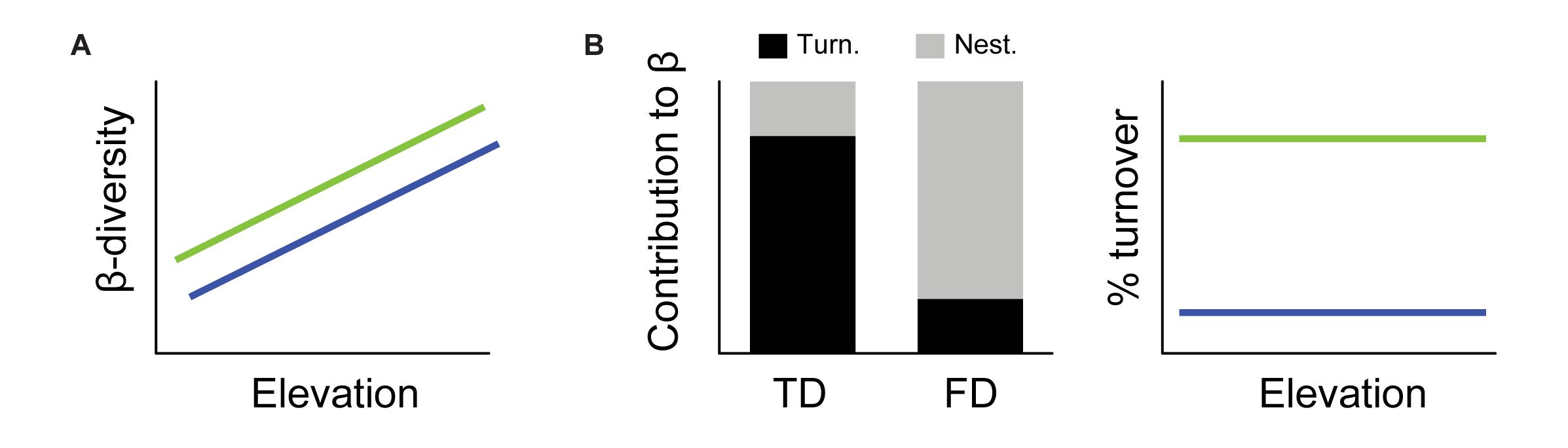
Figure 1. Hypotheses of how temporal β-diversity and its turnover contribution varies in an elevational gradient. (A) Our first hypothesis is that both taxonomic (green line) and functional (blue line) temporal β-diversities increase with elevation, because in more harsh, higher elevation environments, the temporal variation in conditions and resources would be higher. (B) Our second hypothesis is that turnover (black bar) is the most important mechanism generating taxonomic temporal β-diversity (green line) and that nestedness (gray bar) is the most important component of functional temporal β-diversity (blue line) in general and along all the elevational gradient, because the communities would present functional redundancy over time.
Materials and Methods
Study Area
The study was conducted in the southern part of the Espinhaço mountain range (Figure 2), in the permanent plots of the Long Term Ecological Research Project Campos Rupestres (PELD CRSC/CNPq Project) along a gradient of elevation in the Serra do Cipó region, Minas Gerais State, Brazil (19°22′01″S, 43°32′17 ″W) (Silveira et al., 2019). The region has marked wet and dry seasons, and the mean annual precipitation is 1,300–1,500 mm, while the mean annual temperature is 20°C (highland tropical Cwb Köppen climate) (Fernandes et al., 2016). The Serra do Cipó region comprises private areas under environmental protection (APA Morro da Pedreira) and a National Park under full protection (PARNA Serra do Cipó), as well as being part of the Espinhaço Range Biosphere Reserve (Domingues et al., 2011; Fernandes et al., 2018). At the study location, soil and vegetation are very heterogeneous, with the core landscape being a vegetation mosaic dominated by campo rupestre (rupestrian grassland) intermingled by quartzitic outcrops, surrounding forest patches, gallery forests, and mixing with dry forests and cerrado at the lower elevations (Fernandes, 2016; Silveira et al., 2016; Morellato and Silveira, 2018).
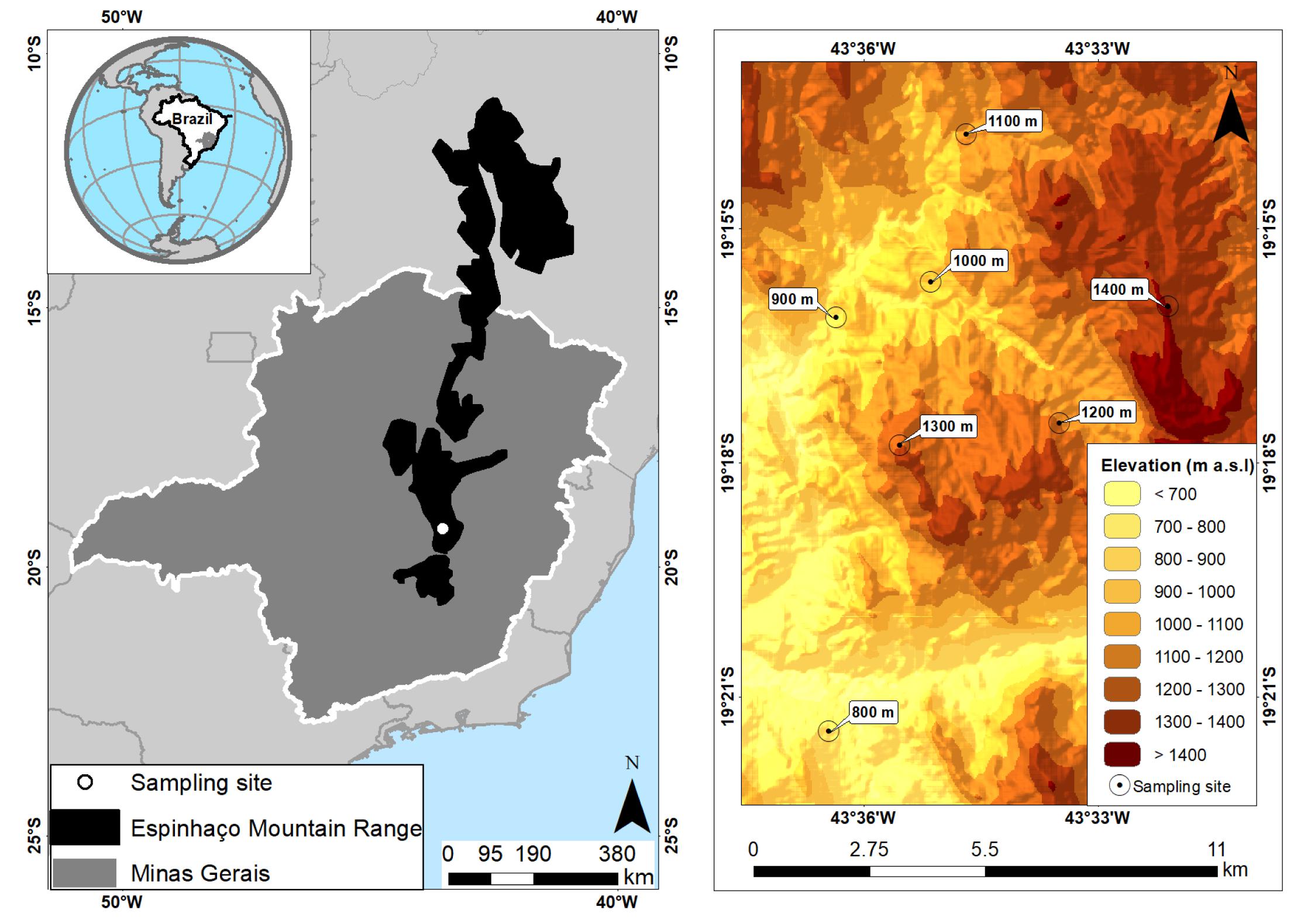
Figure 2. Left panel shows a map with the location of the Espinhaço mountain range in Brazil, with our sampling area in the southern Serra do Cipó region, Minas Gerais. Right panel shows the distribution of the sampling sites along the elevational gradient.
Sampling Design and Explanatory Variables
We distributed our sampling sites every 100 m of elevation on a gradient that ranged from 800 to 1,400 m a.s.l. (Figure 2). At each of these sampling sites, we used three 200 m long transects separated by at least 250 m, totalling 21 transects (three × seven sampling sites). Within each transect, we placed five pitfall traps separated by 50 m (15 traps per sampling site, 105 in total for each temporal sampling) to collect ants foraging on the ground. Traps consisted of a plastic pot with a diameter of 14 cm and a depth of 9 cm, which was filled with 500 ml of a saline-detergent solution. The pots were set at ground level and covered with a plastic plate (20 cm from ground level) to prevent rain from entering the trap. All pitfall traps remained in the field for 48 h per survey (Bestelmeyer et al., 2000). We sampled quarterly (separated by 3 months), totalling 12 samples between April 2011 and January 2014 (January, April, July, and October); such that the samples spanned the beginning and end of both the wet and dry season each year. The transect represents independent sample replicates because the spacing of 50 m between samples is considered enough to avoid interference related to the foraging range of ants belonging to the same colony (Leponce et al., 2004) and we used five times this distance between transects. We pooled data from the five pitfall traps for each transect and each month (see Castro et al., 2020).
To explore the mechanisms behind the patterns we expected with our hypotheses, we accessed information on climatic and vegetation factors. For climatic factors, we used data from meteorological monitoring towers (equipped with the Onset HOBO® U30 data-logger) located at every 100 m of elevation, adjacent to the sampling sites of the PELD CRSC/CNPq Project (Silveira et al., 2019), between January 2012 and January 2014 (January, April, July, and October). We recorded air and soil temperature, humidity, and precipitation, and we calculated the mean and coefficient of variation of these variables for the whole sample period for each sample site (i.e., each elevation). We also used vegetation data from the same project at each elevation [see Mota et al. (2018) for more details] to assess the total plant richness per sample site.
Identification of Species and Definition of Functional Traits of Ants
To identify ants to species and morphospecies we used a comparison method with the Collection of Formicidae from campo rupestre of the Laboratory of Insect Ecology at the Universidade Federal de Minas Gerais, Brazil. In addition, all species were revised by experts of different ant taxa. Classifications were based on Baccaro et al. (2015) and Bolton’s Ants of the World catalog (Bolton, 2020) classifications.
For all ant species collected, we measured key functional response traits related to diet, nesting ecology, foraging capacity, thermoregulation, and habitat association (Leal et al., 2012; Bishop et al., 2016; Paolucci et al., 2016; Tiede et al., 2017; Fichaux et al., 2019). Specifically, we quantified the following seven traits for each species: Weber’s length, femur length, mandible length, predominant color (mesosoma), polymorphism, integument sculpture, and functional groups (six morphological traits and one ecological trait; Table 1).
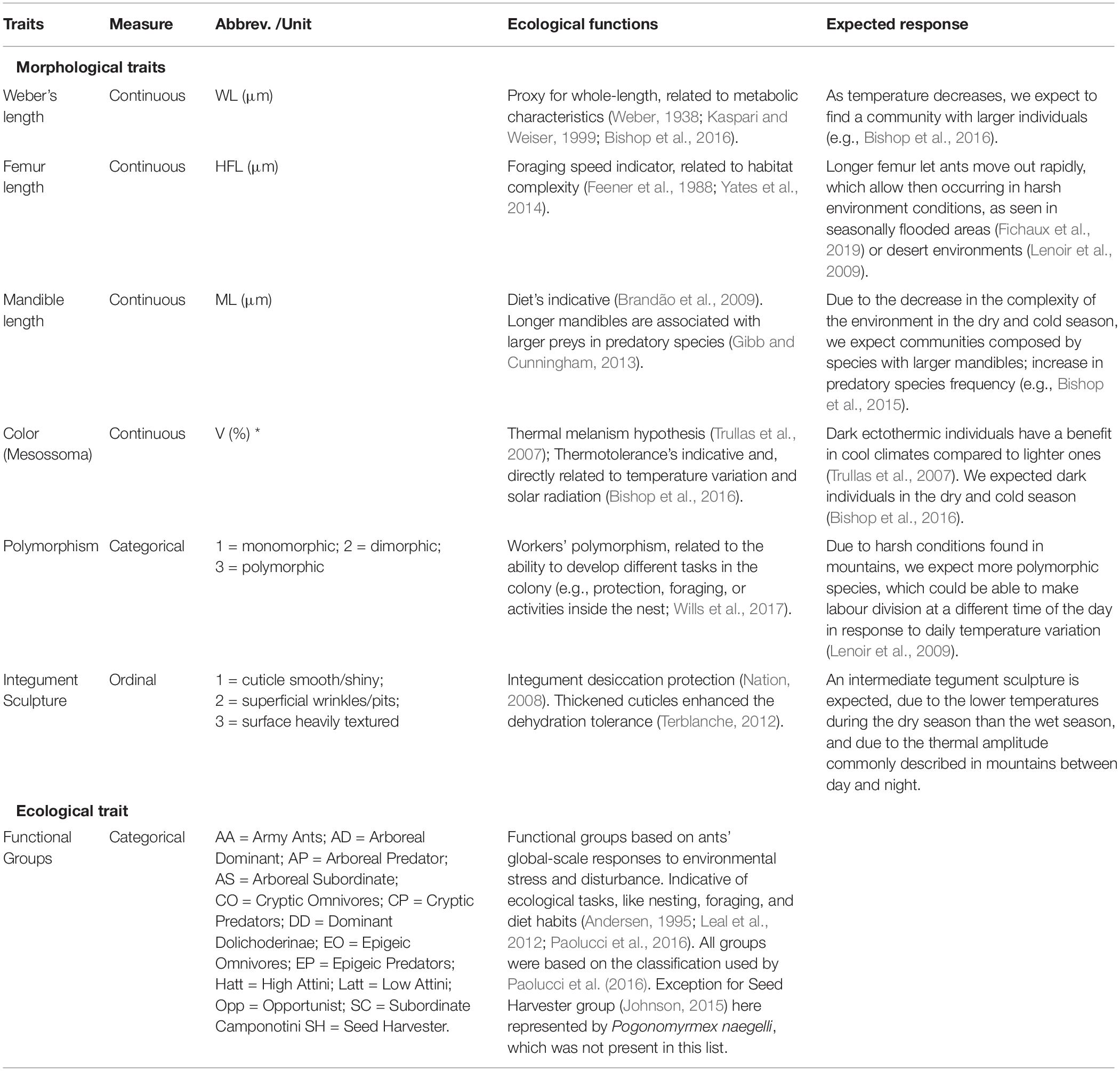
Table 1. List of response functional traits measured (morphological and ecological), their hypothesized ecological functions and the expected response of the traits to environmental change.
Morphological measurements were taken following the guide for identification of functional attributes for ants (The Global Ants trait Database – GLAD; Parr et al., 2017), with exception for the variable “color.” This trait was obtained from the HSV color model using only the variable V (color brightness), as proposed by Bishop et al. (2016). However, we performed the capture of HSV values of the predominant color on the mesosoma of each specimen, in contrast to Bishop et al. (2016), who considered the predominant color between head, mesosoma, and gaster. Since functional traits are not normally distributed (Arnan et al., 2018; Fichaux et al., 2019), all continuous data, except Weber’s length and color brightness, were divided by Weber’s length to correct for individual body size.
Imaging was performed using Microscope Digital Camera LC30 OLYMPUS® mounted on a stereomicroscope SZ61 OLYMPUS®. Measurements were made with a digital capture micrometer (accurate to 0.01 mm) provided in the LC Micro 2.2 OLYMPUS® software. All measured specimens were selected at random from our dataset. When possible, at least six individuals were measured, and whenever it was not possible, we measured all the available individuals. Only minor workers were used, and a total of 2103 images were captured from 701 individuals, with an average of 4.52 individuals per species. Categorical and ordinal morphological traits (i.e., polymorphism and integument sculpture) were attributed using genera/species information available at AntWeb1 and AntWiki website2 (Guénard et al., 2017) and from our own observations.
Calculating Temporal β-Diversity
We calculated the variation of the taxonomic (TD) and functional (FD) composition of the ant community temporally using β-diversity (βSOR) (Baselga, 2010). We used the data collected over the 12 sampling periods to calculate temporal TD and FD β-diversity for each transect (21 in total). We also partitioned TD and FD into the components derived from species turnover (βSIM) and species gain/loss or nestedness (βSNE). In this step, we partitioned β-diversity for the whole gradient and for each transect. In all β-diversity calculations, we used Sørensen dissimilarity index based on a presence/absence species composition matrix. To calculate temporal functional β-diversity, we first generated a distance matrix of species based on their functional traits using the Gower Distance, a useful method for combining categorical and continuous traits (de Bello et al., 2013). We then used this distance matrix to construct a functional space, using Principal Coordinates Analysis (PCoA), which in turn was used to calculate temporal functional β-diversity. We used the “beta.multi” and “functional.beta.multi” functions of the package “betapart” to partition TD and FD β-diversity, respectively (Baselga and Orme, 2012), in the software R (R Core Team, 2019).
Statistical Analyses
We ran Pearson correlation analyses with all the environmental variables against elevation using the “psych” R package (Revelle, 2017). As elevation was correlated with mean air and soil temperature, air humidity, plant richness and the temporal variation of air humidity and soil temperature (see details in section “Results”), we used elevation as a proxy for all these variables in our analyses. To test our first hypothesis, we ran linear mixed-effect models (LMMs), one with TD β-diversity as response variable and other with FD β-diversity (βSOR), and both had elevation as an independent variable and sampling site as a random variable. To test our second hypothesis, we used the turnover contribution (βSIM/βSOR) as the response variable and also ran separate LMMs for TD and FD, with elevation as an independent variable and sampling site as a random variable. We checked for the error distribution and over-dispersion of the data. All statistical analyses were performed in R (R Core Team, 2019).
Results
We recorded a total of 155 ant morphospecies (species hereafter), belonging to eight subfamilies and 49 genera. Myrmicinae was the most representative subfamily with 25 of the identified genera, followed by Ponerinae (eight), Dolichoderinae (five), Dorylinae (four), and Formicinae (three). The subfamilies with the highest species richness were Myrmicinae (85 species), Formicinae (23 species), Ponerinae and Dolichoderinae (12 species each). These four subfamilies accounted for 85% of the species sampled. The genera with most species were Pheidole with 25 species, Camponotus 20 species, and Solenopsis with eight species. Across all samples, we recorded 13 doubletons and 23 singletons (23.2%).
We found that mean air and soil temperatures, plant richness, and coefficient of variation of air humidity were negatively correlated with elevation, whereas mean air humidity, and coefficient of variation of soil temperature were positively correlated with elevation (Figure 3 and Supplementary Table 1). That is, mean air and soil temperature, plant richness and the temporal variation of humidity decrease with increasing elevation, while air humidity and the temporal variation of soil temperature increase with increasing elevation (Figure 3). We also found that precipitation dropped from ∼170 mm to close to 0, while mean air temperature dropped from 21.1 to 17.2°C in the beginning of the wet season to the beginning of the dry season, respectively (Figure 4). Air and soil humidity mirrored this pattern of decrease from the wet to the dry season (Figure 4).
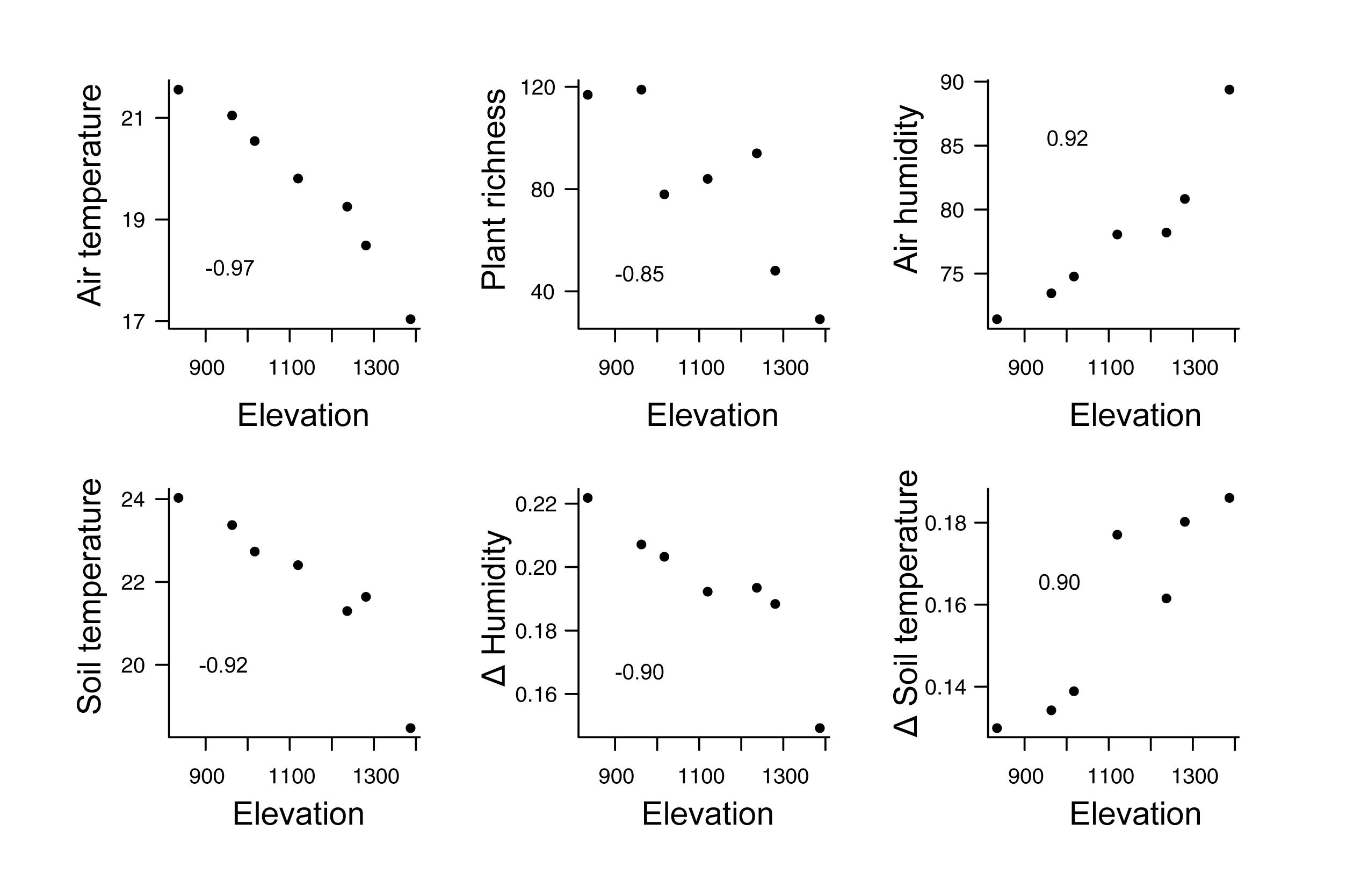
Figure 3. Correlations between elevation and environmental variables. Mean air temperature, soil temperature, plant richness and the variation of air humidity decrease with elevation, while mean air humidity and the variation of soil temperature increase with elevation. All presented Pearson correlations are significant under α = 0.05. Δ is coefficient of variation while the other variables represent mean values. Numbers inside the plots represent the coefficient of correlation. Data of plant richness from Mota et al. (2018). Data of climatic variables sampled using meteorological monitoring towers during 12 periods along an elevational gradient in Serra do Cipó, Minas Gerais State – Brazil.
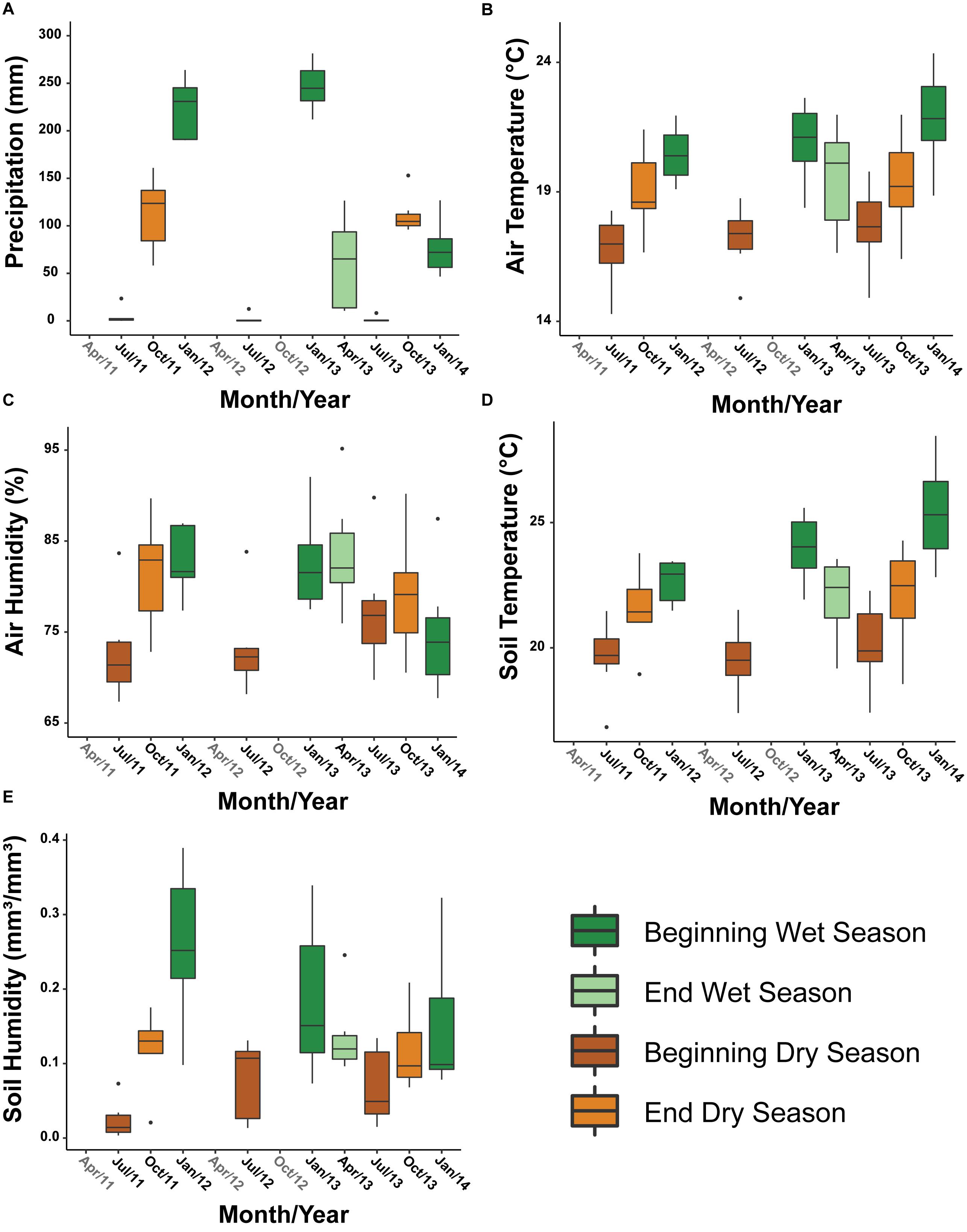
Figure 4. Variation of (A) precipitation, (B) air temperature, (C) air humidity, (D) soil temperature, and (E) soil humidity over 12 periods in the beginning and end of wet and dry seasons. The boxplots represent the variation of the mean values of the seven sampling sites (different elevations) in each month/year. Data sampled using meteorological monitoring towers along an elevational gradient in Serra do Cipó, Minas Gerais State – Brazil.
The main driver of temporal taxonomic β-diversity was the turnover component (βSIM) with 86% of contribution. In contrast, nestedness (βSNE) was the main component to temporal functional β-diversity with 67% of contribution across the entire gradient (Figure 5).
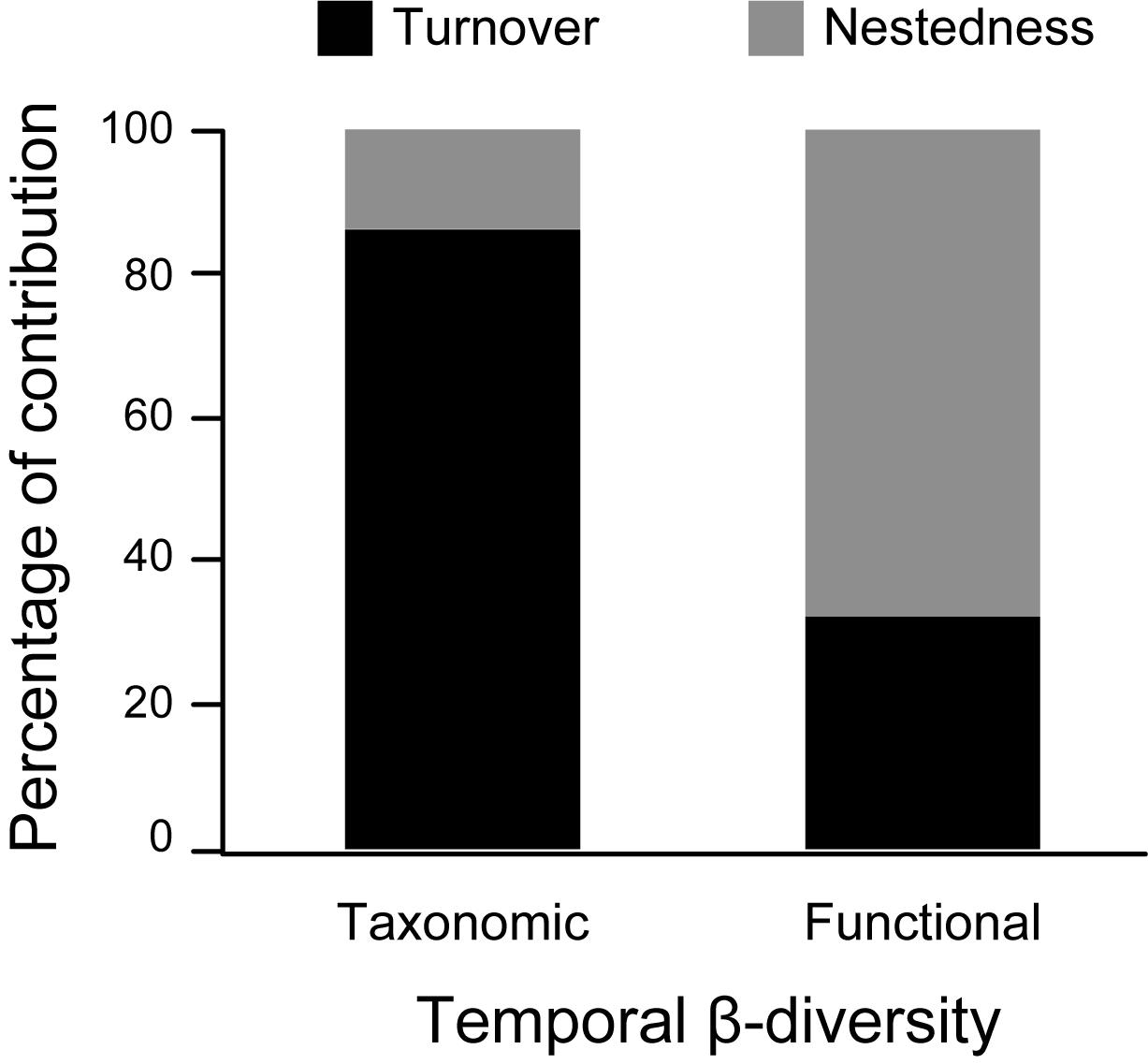
Figure 5. Contribution of turnover and nestedness components to temporal taxonomic and functional β-diversity of ant metacommunity in Serra do Cipó, Minas Gerais State – Brazil. Data from 12 sampling periods between April 2011 and January 2014.
Neither temporal taxonomic nor functional β-diversity were influenced by elevation [TD: χ (1, 19) = 1.02, p = 0.31; FD: χ (1, 19) = 1.86, p = 0.17; Figure 6A]. Turnover (βSIM) was the main driver of temporal taxonomic β-diversity with a consistent pattern along the entire elevation gradient [contribution always higher than 80%; χ (1, 19) = 0.24, p = 0.62; Figure 6B]. In contrast, temporal functional turnover contribution increased with elevation [χ (1, 19) = 5.22, p < 0.05, pseudo-R2 = 0.22], going from less than 20% in lower elevations to more than 50% in higher elevations (Figure 6B).
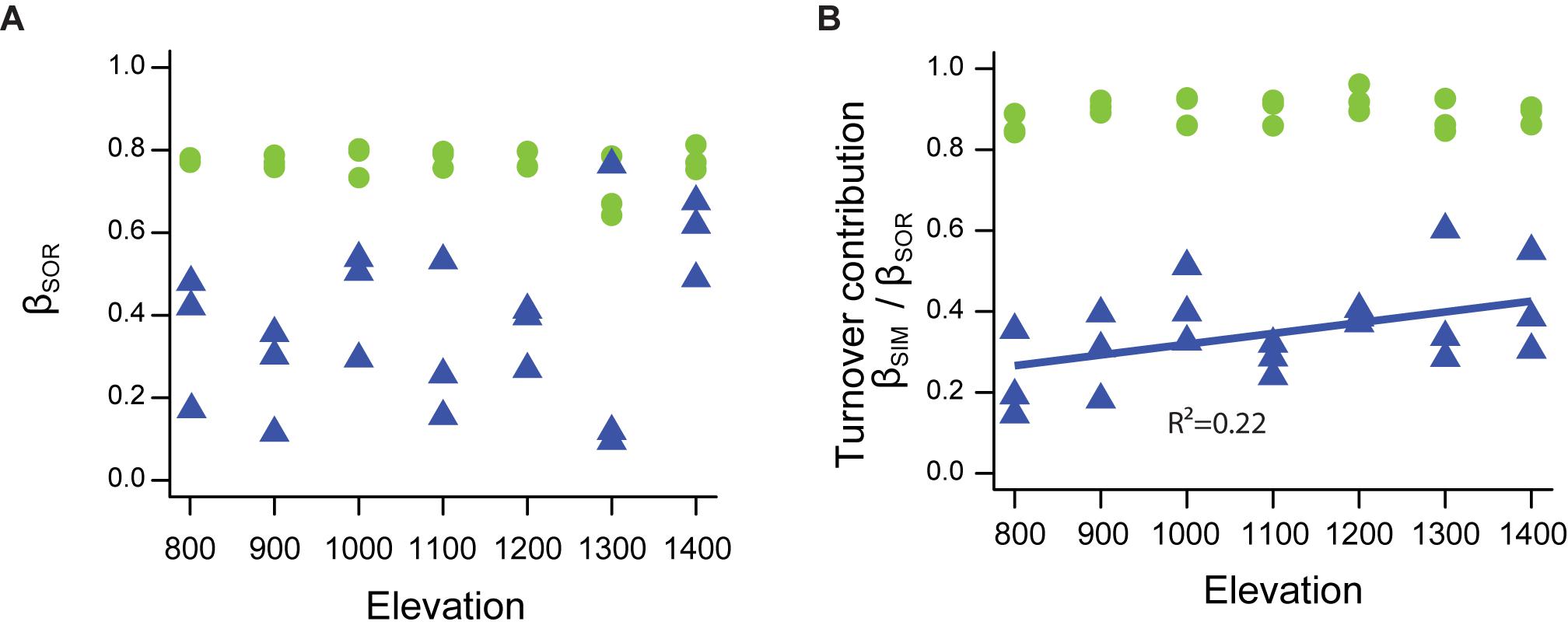
Figure 6. Temporal taxonomic (green dots) and functional (blue triangles) β-diversity of ants along a tropical elevational gradient in Brazil. (A) Values of temporal β-diversity (βSOR) were not correlated with elevation and were always high, both for temporal taxonomic and functional diversity. (B) Temporal turnover contribution (βSIM/βSOR) to β-diversity was always high for taxonomic diversity whereas it increased with elevation for functional diversity. Data from ant communities sampled in 12 periods along an elevation gradient in Serra do Cipó, Minas Gerais State – Brazil. The figure represents four separated models (see section “Materials and Methods”).
Discussion
We found that ant communities had remarkably high temporal taxonomic and functional β-diversity. Contrary to our first hypothesis, values of temporal taxonomic and functional β-diversity are high irrespective of their position along the elevational gradient. Partially in line with our second hypothesis, turnover was the main component of temporal taxonomic β-diversity along the gradient (above 80% of contribution). However, the contribution of nestedness to temporal functional β-diversity decreased significantly with elevation. We propose that ant activity is very sensitive to temporal variation in environmental conditions, and mainly temperature (Costa et al., 2018; Castro et al., 2020). The effect on ant community composition is then the same along the elevational gradient, with both species and functional traits highly variable over time at all elevations. Although ant metacommunities are changing over time, at low elevations the turnover in species composition may cause changes in trait composition because of the loss of some traits, but probably preserving the most common functions (nested functional communities). In contrast, at higher elevations, the turnover in species composition cause changes in the set of functions performed over time (i.e., temporal turnover of traits).
The campo rupestre has two well-defined seasons: a wet season that is also hot (∼170 mm/month and mean temperature of 21.1°C), and a dry season that is cold (close to 0 mm/month and 17.2°C). The changes in temperature and humidity impose changes in plant productivity (Rocha et al., 2016) and animal activity (especially the ectothermic animals; e.g., Costa et al., 2018). Indeed, temporal changes in temperature, humidity and resource availability are known to influence ant foraging activity (Calazans et al., 2020) and consequently the diversity and composition of communities. However, we found high levels of species and trait composition changes along the entire elevation gradient, contrary to our expectations. We propose two non-exclusive hypotheses to explain this result: (i) the sensitivity of ant communities in tropical mountains to changes in environmental conditions (Longino and Colwell, 2011; Castro et al., 2020; Lasmar et al., 2020) are enough to impose drastic changes in species and trait composition; ii. ant communities are regulated by the minimum humidity at low elevations, while the highland ant communities are regulated by the minimum temperature, consistent with our finding that the variation of temperature increases with elevation, while the variation in humidity decreases. Bishop et al. (2017) found that ants are constrained more by the critical thermal minimum temperature (CTmin) than by the maximum in a southern African mountain. Accordingly, Calazans et al. (2020) found, in a study in campo rupestre, that ant activity increased with temperature, but most species were not active under 20°C. Although highland ants would probably have a lower CTmin, the higher variation on temperature at high elevations could filter different species and traits in different seasons.
We found that turnover was the main mechanism generating temporal taxonomic β-diversity, as expected based on the spatial pattern for ants and many other organisms (Perillo et al., 2017; da Silva et al., 2018; Kaltsas et al., 2018; Castro et al., 2020). The spatial variation in conditions and resources provide an environmental filter for species that have pre-adaptations to survive in different places (Heino and Tolonen, 2017; García-Llamas et al., 2019) leading to high rates of turnover of species. In this sense, the same processes would be occurring to generate the temporal pattern but related to ant activity: environmentally filtering ant species to be active in different seasons along all the elevational gradient. In contrast, functional β-diversity is generated by a higher contribution of nestedness in spatial patterns (Nunes et al., 2016, 2017; Castro et al., 2020) especially on elevational gradients, showing high functional redundancy. In our study, at lowlands the temporal pattern mirrors the spatial and nestedness contributes 80% of functional β-diversity (i.e., 20% turnover, Figure 6B). However, the importance of turnover in functional diversity increases with elevation, reaching more than 50% in some highlands. The changes in species composition, caused by a change in the set of active species, lead to creation of subsets of traits among seasons, with a more complete set in hot and dry season and a subset of the most common traits in dry and cold season. However, at higher elevations on the gradient, increasing trait turnover can create a greater variation in trait combinations over space and time. This means that lower elevation ant metacommunities are more functionally resistant to changes in species composition, having greater functional redundancy over time while higher elevation metacommunities are more functionally sensitive to changes in species composition across the seasons.
Contextualizing our results within the metacommunity framework, we can propose that ant diversity is explained by a combination of the species sorting and the mass effect models (Leibold et al., 2004; Soininen, 2014). We found a high β-diversity and turnover contribution in our study, showing that environmental conditions and resources are filtering the species comprising the ant metacommunity (i.e., species sorting; Leibold and Chase, 2018). Due to high environmental heterogeneity across the elevational gradient, if we are looking at different sites (e.g., different elevations or different habitats) or different times (e.g., different seasons) we expect to find different ant species compositions. Conversely, generalist ant species may have access to different habitats with different conditions through dispersal and can establish long term populations (e.g., Neves et al., 2020), maintaining the mass effect. Therefore, although environmental filters are strong in spatially and temporally regulating the ant metacommunity (species sorting), some ant species can disperse, overcoming local niche limitations and establish populations in different sites following close-to-optimal conditions (mass effects). In both cases, we observe a greater importance of environmental than dispersal-related factors, a pattern expected when there is high environmental heterogeneity (e.g., He et al., 2020).
Implications for Conservation
Our study builds on growing evidence that montane tropical insects are highly sensitive to local climatic and environmental changes (e.g., Longino and Colwell, 2011; Lasmar et al., 2020). The studied ant metacommunities are highly variable over time along the entire elevational gradient. Montane insect assemblages are thought to be very vulnerable to global warming (Laurance et al., 2011), because with increasing temperature, these organisms are expected to shift their elevational ranges, leading to mountaintop extinctions (Colwell et al., 2008). As there is growing evidence that climate change affects both temperature and precipitation regimes (e.g., Romero et al., 2020) and also the occurrence and intensity of extreme climatic events (e.g., Fischer and Knutti, 2015; Patricola and Wehner, 2018), we may expect that such increase in climatic variation and instability will drastically affect ant metacommunities. With this higher temporal variation in conditions and resources, we might lose the rarer and specialized species (Davies et al., 2004) that cannot survive the new environmental filter, leading to the homogenisation of the metacommunity (Newbold et al., 2019). In plants, generalist species tend to be functionally closer, i.e., have high functional redundancy (Denelle et al., 2020), and in assemblages with homogenized species composition we may observe a loss of functional traits and consequently, ecological functions (Newbold et al., 2019). In addition, species that show temporal shutdown in their activity, are likely to be more active year-round as the climate warms (Costa et al., 2018), potentially increasing competition, although the outcome of competitive interactions will likely be influenced by the prevailing combination of temperature and humidity. Furthermore, as we identified an increasing importance of functional temporal turnover along the elevational gradient, we expect that the higher climatic variation associated with climate change (Fischer and Knutti, 2015) will favor generalist species within communities. This, in turn, will result in the homogenisation of the set of functions performed in different seasons. With mountaintop extinctions, elevational range shifts, and taxonomic and functional homogenisation of assemblages, ecosystem functions provided by ants will be severely jeopardized in tropical mountains under global climatic changes.
Data Availability Statement
The original contributions presented in the study are included in the article/Supplementary Material, further inquiries can be directed to the corresponding author.
Author Contributions
CAN, FN, SP, and RS: study design. GF and FN: project funding. HB and FC: data collection. CAN, FC, and FN: data analyses. CAN, FC, HB, SP, RS, GF, and FN: writing the manuscript. All authors contributed to the article and approved the submitted version.
Funding
This work was supported by the CNPq for funding the Long-Term Ecological Research “PELD Campos Rupestres da Serra do Cipó” (grant number 441515/2016-9) and FAPEMIG (grant number CRA-APQ-00311-15). SP was funded by NSF grant DEB 1442256.
Conflict of Interest
The authors declare that the research was conducted in the absence of any commercial or financial relationships that could be construed as a potential conflict of interest.
Acknowledgments
We thank R. Feitosa for the help with ant identification. We also thank the GSG, the Reserva Vellozia, and Parque Nacional da Serra do Cipó for their logistical support. We thank Nathália Carvalho for the help with constructing the map. RS thanks CNPq (305739/2019-0) and P&D Aneel-Cemig, PROECOS, GT599. SP thanks NSF for support (award DEB 1442256). FN thanks CNPq (306995/2019-0) for funding. CAN, HB, and FC thank CAPES for scholarships (Code 001). This manuscript is a contribution to the Special Issue Metacommunity Spatio-Temporal Dynamics: Conservation and Management Implications.
Supplementary Material
The Supplementary Material for this article can be found online at: https://www.frontiersin.org/articles/10.3389/fevo.2020.571439/full#supplementary-material
Supplementary Figure 1 | Community weighted mean (CWM) values of continuous traits of ant metacommunities during four periods of the year: the beginning and end of wet and dry seasons.
Supplementary Table 1 | Correlations between environmental variables.
Supplementary Table 2 | Functional traits and temporal data used in data analyses.
Footnotes
References
Andersen, A. N. (1995). A Classification of Australian Ant Communities, Based on Functional Groups Which Parallel Plant Life-Forms in Relation to Stress and Disturbance. J. Biogeogr. 22, 15–29. doi: 10.2307/2846070
Arnan, X., Andersen, A. N., Gibb, H., Parr, C. L., Sanders, N. J., Dunn, R. R., et al. (2018). Dominance-diversity relationships in ant communities differ with invasion. Glob. Chang. Biol. 24, 4614–4625. doi: 10.1111/gcb.14331
Baccaro, F. B., Feitosa, R. M., Fernandez, F., Fernandes, I. O., Izzo, T. J., Souza, J. L. P., et al. (2015). Guia para os gêneros de formigas do Brasil. Manaus: INPA. doi: 10.5281/zenodo.32912
Baselga, A. (2010). Partitioning the turnover and nestedness components of beta diversity. Glob. Ecol. Biogeogr. 19, 134–143. doi: 10.1111/j.1466-8238.2009.00490.x
Baselga, A., and Orme, C. D. L. (2012). betapart?: an R package for the study of beta diversity. Methods Ecol. Evol. 3, 808–812. doi: 10.1111/j.2041-210X.2012.00224.x
Basset, Y., Cizek, L., Cuénoud, P., Didham, R. K., Novotny, V., Ødegaard, F., et al. (2015). Arthropod Distribution in a Tropical Rainforest: Tackling a Four Dimensional Puzzle. PLoS One 10:e0144110. doi: 10.1371/journal.pone.0144110
Bestelmeyer, B. T., Agosti, D., Alonso, L. E., Brandão, C. R. F., Brown, W. L. Jr., Delabie, J. H. C., et al. (2000). “Field Techniques for the Study of Ground-Dwelling Ants: An Overview, Description and Evaluation,” in Ants: standard methods for measuring and monitoring biodiversity, eds D. Agosti, J. D. Majer, L. E. Alonso, and T. R. Schultz, (Washington, DC: Smithsonian Institution Press), 122–144.
Bishop, T. R., Robertson, M. P., Gibb, H., van Rensburg, B. J., Braschler, B., Chown, S. L., et al. (2016). Ant assemblages have darker and larger members in cold environments. Glob. Ecol. Biogeogr. 25, 1489–1499. doi: 10.1111/geb.12516
Bishop, T. R., Robertson, M. P., van Rensburg, B. J., and Parr, C. L. (2014). Elevation-diversity patterns through space and time: ant communities of the Maloti-Drakensberg Mountains of southern Africa. J. Biogeogr. 41, 2256–2268. doi: 10.1111/jbi.12368
Bishop, T. R., Robertson, M. P., van Rensburg, B. J., and Parr, C. L. (2015). Contrasting species and functional beta diversity in montane ant assemblages. J. Biogeogr. 42, 1776–1786. doi: 10.1111/jbi.12537
Bishop, T. R., Robertson, M. P., Van Rensburg, B. J., and Parr, C. L. (2017). Coping with the cold: minimum temperatures and thermal tolerances dominate the ecology of mountain ants. Ecol. Entomol. 42, 105–114. doi: 10.1111/een.12364
Bolton, B. (2020). An online catalog of the ants of the world. Available Online at: https://antcat.org (accessed February 6, 2019).
Brandão, C. R. F., Silva, R. R., and Delabie, J. H. C. (2009). “Formigas (Hymenoptera),” in Bioecologia e Nutrição de Insetos. Base para o Manejo Integrado de Pragas, eds A. R. Panizzi, and J. R. P. Parra, (Brasília: EMBRAPA), 323–369.
Calazans, E. G., Costa, F. V. d, Cristiano, M. P., and Cardoso, D. C. (2020). Daily Dynamics of an Ant Community in a Mountaintop Ecosystem. Environ. Entomol. 49, 383–390. doi: 10.1093/ee/nvaa011
Castro, D. M. P., Callisto, M., Solar, R. R. C., Macedo, D. R., and Fernandes, G. W. (2019). Beta diversity of aquatic invertebrates increases along an altitudinal gradient in a Neotropical mountain. Biotropica 51, 399–411. doi: 10.1111/btp.12660
Castro, F. S. d, Da Silva, P. G., Solar, R., Fernandes, G. W., and Neves, F. d. S (2020). Environmental drivers of taxonomic and functional diversity of ant communities in a tropical mountain. Insect Conserv. Divers 13, 393–403. doi: 10.1111/icad.12415
Colwell, R. K., Brehm, G., Cardelús, C. L., Gilman, A. C., and Longino, J. T. (2008). Global warming, elevational range shifts, and lowland biotic attrition in the wet tropics. Science 322, 258–261. doi: 10.1126/science.1162547
Costa, F. V., Blüthgen, N., Viana-Junior, A. B., Guerra, T. J., Di Spirito, L., and Neves, F. S. (2018). Resilience to fire and climate seasonality drive the temporal dynamics of ant-plant interactions in a fire-prone ecosystem. Ecol. Indic. 93, 247–255. doi: 10.1016/j.ecolind.2018.05.001
Crist, T. O., Veech, J. A., Gering, J. C., and Summerville, K. S. (2003). Partitioning species diversity across landscapes and regions: a hierarchical analysis of alpha, beta, and gamma diversity. Am. Nat. 162, 734–743. doi: 10.1086/378901
da Silva, P. G., Lobo, J. M., Hensen, M. C., Vaz-de-Mello, F. Z., and Hernández, M. I. M. (2018). Turnover and nestedness in subtropical dung beetle assemblages along an elevational gradient. Divers. Distrib. 24, 1277–1290. doi: 10.1111/ddi.12763
Datry, T., Bonada, N., and Heino, J. (2016). Towards understanding the organisation of metacommunities in highly dynamic ecological systems. Oikos 125, 149–159. doi: 10.1111/oik.02922
Davies, K. F., Margules, C. R., and Lawrence, J. F. (2004). A synergistic effect puts rare, specialized species at greater risk of extinction. Ecology 85, 265–271.
de Bello, F., Carmona, C. P., Mason, N. W. H., Sebastià, M.-T., and Lepš, J. (2013). Which trait dissimilarity for functional diversity: trait means or trait overlap? J. Veg. Sci. 24, 807–819. doi: 10.1111/jvs.12008
Denelle, P., Violle, C., and Munoz, F. (2020). Generalist plants are more competitive and more functionally similar to each other than specialist plants: insights from network analyses. J. Biogeogr. 47, 1922–1933. doi: 10.1111/jbi.13848
Domingues, S. A., Karez, C. S., Biondini, I. V. F., and Andrade, M. Â (2011). “Instrumentos económicos de gestión ambiental en la Reserva de Biosfera de la Serra do Espinhaço,” in UNESCO Regional Office for Science for Latin America and the Caribbean∗, eds I. Sachs, M. Clüsener-Godt, and C. S. Karez Montevideo.
Farji-Brener, A. G., and Werenkraut, V. (2017). The effects of ant nests on soil fertility and plant performance: a meta-analysis. J. Anim. Ecol. 86, 866–877. doi: 10.1111/1365-2656.12672
Feener, D. H., Lighton, J. R. B., and Bartholomew, G. A. (1988). Curvilinear Allometry, Energetics and Foraging Ecology: A Comparison of Leaf-Cutting Ants and Army Ants. Funct. Ecol. 2, 509–520. doi: 10.2307/2389394
Fernandes, G. W., et al. (2016). Ecology and Conservation of Mountaintop grasslands in Brazil. Cham: Springer International Publishing.
Fernandes, G. W., Almeida, H. A., Nunes, C. A., Xavier, J. H. A., Cobb, N. S., Carneiro, M. A. A., et al. (2016). “Cerrado to Rupestrian Grasslands: Patterns of Species Distribution and the Forces Shaping Them Along an Altitudinal Gradient,” in Ecology and Conservation of Mountaintop grasslands in Brazil, ed. G. W. Fernandes, (Dordrecht: Springer), 345–377. doi: 10.1007/978-3-319-29808-5_15
Fernandes, G. W., Barbosa, N. P. U., Alberton, B., Barbieri, A., Dirzo, R., Goulart, F., et al. (2018). The deadly route to collapse and the uncertain fate of Brazilian rupestrian grasslands. Biodivers. Conserv. 27, 2587–2603. doi: 10.1007/s10531-018-1556-1554
Fichaux, M., Béchade, B., Donald, J., Weyna, A., Delabie, J. H. C., Murienne, J., et al. (2019). Habitats shape taxonomic and functional composition of Neotropical ant assemblages. Oecologia 189, 501–513. doi: 10.1007/s00442-019-04341-z
Fischer, E. M., and Knutti, R. (2015). Anthropogenic contribution to global occurrence of heavy-precipitation and high-temperature extremes. Nat. Clim. Chang. 5, 560–564. doi: 10.1038/nclimate2617
García-Llamas, P., Rangel, T. F., Calvo, L., and Suárez-Seoane, S. (2019). Linking species functional traits of terrestrial vertebrates and environmental filters: A case study in temperate mountain systems. PLoS One 14:1–15. doi: 10.1371/journal.pone.0211760
Gibb, H., and Cunningham, S. A. (2013). Restoration of trophic structure in an assemblage of omnivores, considering a revegetation chronosequence. J. Appl. Ecol. 50, 449–458. doi: 10.1111/1365-2664.12054
Guénard, B., Weiser, M. D., Gómez, K., Narula, N., and Economo, E. P. (2017). The Global Ant Biodiversity Informatics (GABI) database: Synthesizing data on the geographic distribution of ant species (Hymenoptera: Formicidae). Myrmecol. News 24, 83–89.
He, S., Soininen, J., Chen, K., and Wang, B. (2020). Environmental factors override dispersal-related factors in shaping diatom and macroinvertebrate communities within stream networks in China. Front. Ecol. Evol. 8:1–11. doi: 10.3389/fevo.2020.00141
Heino, J., Melo, A. S., Siqueira, T., Soininen, J., Valanko, S., and Bini, L. M. (2015). Metacommunity organisation, spatial extent and dispersal in aquatic systems: patterns, processes and prospects. Freshw. Biol. 60, 845–869. doi: 10.1111/fwb.12533
Heino, J., and Tolonen, K. T. (2017). Ecological drivers of multiple facets of beta diversity in a lentic macroinvertebrate metacommunity. Limnol. Oceanogr. 62, 2431–2444. doi: 10.1002/lno.10577
Johnson, R. A. (2015). A taxonomic revision of South American species of the seed-harvester ant genus Pogonomyrmex (Hymenoptera: Formicidae). Part I. Zootaxa 4029, 1–142. doi: 10.11646/zootaxa.4029.1.1
Kaltsas, D., Dede, K., Giannaka, J., Nasopoulou, T., Kechagioglou, S., Grigoriadou, E., et al. (2018). Taxonomic and functional diversity of butterflies along an altitudinal gradient in two NATURA 2000 sites in Greece. Insect Conserv. Divers. 11, 464–478. doi: 10.1111/icad.12292
Kaspari, M., and Weiser, M. D. (1999). The size–grain hypothesis and interspecific scaling in ants. Funct. Ecol. 13, 530–538. doi: 10.1046/j.1365-2435.1999.00343.x
Körner, C. (2007). The use of “altitude” in ecological research. Trends Ecol. Evol. 22, 569–574. doi: 10.1016/j.tree.2007.09.006
Lasmar, C. J., Ribas, C. R., Louzada, J., Queiroz, A. C. M., Feitosa, R. M., Imata, M. M. G., et al. (2020). Disentangling elevational and vegetational effects on ant diversity patterns. Acta Oecol. 102:103489. doi: 10.1016/j.actao.2019.103489
Laurance, W. F., Carolina Useche, D., Shoo, L. P., Herzog, S. K., Kessler, M., Escobar, F., et al. (2011). Global warming, elevational ranges and the vulnerability of tropical biota. Biol. Conserv. 144, 548–557. doi: 10.1016/j.biocon.2010.10.010
Leal, I. R., Filgueiras, B. K. C., Gomes, J. P., Iannuzzi, L., and Andersen, A. N. (2012). Effects of habitat fragmentation on ant richness and functional composition in Brazilian Atlantic forest. Biodivers. Conserv. 21, 1687–1701. doi: 10.1007/s10531-012-0271-279
Legendre, P., and Condit, R. (2019). Spatial and temporal analysis of beta diversity in the Barro Colorado Island forest dynamics plot, Panama. For. Ecosyst. 6:7. doi: 10.1186/s40663-019-0164-164
Leibold, M. A., and Chase, J. M. (2018). Metacommunity ecology. Princeton: Princeton University Press.
Leibold, M. A., Holyoak, M., Mouquet, N., Amarasekare, P., Chase, J. M., Hoopes, M. F., et al. (2004). The metacommunity concept: a framework for multi-scale community ecology. Ecol. Lett. 7, 601–613. doi: 10.1111/j.1461-0248.2004.00608.x
Lenoir, A., Aron, S., Cerdá, X., and Hefetz, A. (2009). Cataglyphis desert ants: a good model for evolutionary biology in Darwin’s anniversary year - A review. Isr. J. Entomol. 39, 1–32.
Leponce, M., Theunis, L., Delabie, J. H. C., and Roisin, Y. (2004). Scale dependence of diversity measures in a leaf-litter ant assemblage. Ecography 27, 253–267.
Levins, R. (1969). Some Demographic and Genetic Consequences of Environmental Heterogeneity for Biological Control. Bull. Entomol. Soc. Am. 15, 237–240. doi: 10.1093/besa/15.3.237
Lewis, S. L., and Maslin, M. A. (2015). Defining the Anthropocene. Nature 519, 171–180. doi: 10.1038/nature14258
Li, N., Chu, H., Qi, Y., Li, C., Ping, X., Sun, Y., et al. (2019). Alpha and beta diversity of birds along elevational vegetation zones on the southern slope of Altai Mountains: Implication for conservation. Glob. Ecol. Conserv. 19:e00643. doi: 10.1016/j.gecco.2019.e00643
Longino, J. T., and Colwell, R. K. (2011). Density compensation, species composition, and richness of ants on a neotropical elevational gradient. Ecosphere 2:art29. doi: 10.1890/ES10-00200.1
Magalhães, V. B., Espírito Santo, N. B., Salles, L. F. P., Soares, H., and Oliveira, P. S. (2018). Secondary seed dispersal by ants in Neotropical cerrado savanna: species-specific effects on seeds and seedlings of Siparuna guianensis (Siparunaceae). Ecol. Entomol. 43, 665–674. doi: 10.1111/een.12640
Morellato, L. P. C., and Silveira, F. A. O. (2018). Plant life in campo rupestre?: New lessons from an ancient biodiversity hotspot. Flora 238, 1–10. doi: 10.1016/j.flora.2017.12.001
Mota, G. S., Luz, G. R., Mota, N. M., Silva Coutinho, E., das Dores, Magalhães Veloso, M., et al. (2018). Changes in species composition, vegetation structure, and life forms along an altitudinal gradient of rupestrian grasslands in south-eastern Brazil. Flora 238, 32–42. doi: 10.1016/j.flora.2017.03.010
Nation, J. L. (2008). “Integument: Structure and Function,” in Encyclopedia of Entomology, ed. J. L. Capinera, (Netherlands: Springer), 2015–2019. doi: 10.1007/978-1-4020-6359-6_1557
Neves, F. D. S., da Silva, P. G., Solar, R., Nunes, C. A., Beirão, M. D. V., Brant, H., et al. (2020). Habitat generalists drive nestedness in a tropical mountaintop insect metacommunity. Biol. J. Linn. Soc. 2020:blaa059. doi: 10.1093/biolinnean/blaa059
Newbold, T., Adams, G. L., Albaladejo Robles, G., Boakes, E. H., Braga Ferreira, G., Chapman, A. S. A., et al. (2019). Climate and land-use change homogenise terrestrial biodiversity, with consequences for ecosystem functioning and human well-being. Emerg. Top. Life Sci. 3, 207–219. doi: 10.1042/ETLS20180135
Novais, S. M. A., Monteiro, G. F., Macedo-Reis, L. E., Leal, C. R. O., and Neves, F. D. S. (2019). Changes in the insect herbivore fauna after the first rains in a tropical dry forest. Oecol. Aust. 23, 381–387. doi: 10.4257/oeco.2019.2302.16
Nunes, C. A., Braga, R. F., Figueira, J. E. C., Neves, F. D. S., and Fernandes, G. W. (2016). Dung beetles along a tropical altitudinal gradient: environmental filtering on taxonomic and functional diversity. PLoS One 11:e0157442. doi: 10.1371/journal.pone.0157442
Nunes, C. A., Quintino, A. V., Constantino, R., Negreiros, D., Reis Júnior, R., and Fernandes, G. W. (2017). Patterns of taxonomic and functional diversity of termites along a tropical elevational gradient. Biotropica 49, 186–194. doi: 10.1111/btp.12365
Paolucci, L. N., Maia, M. L. B., Solar, R. R. C., Campos, R. I., Schoereder, J. H., and Andersen, A. N. (2016). Fire in the Amazon: impact of experimental fuel addition on responses of ants and their interactions with myrmecochorous seeds. Oecologia 182, 335–346. doi: 10.1007/s00442-016-3638-x
Parr, C. L., Dunn, R. R., Sanders, N. J., Weiser, M. D., Photakis, M., Bishop, T. R., et al. (2017). GlobalAnts?: a new database on the geography of ant traits (Hymenoptera: Formicidae). Insect Conserv. Divers. 10, 5–20. doi: 10.1111/icad.12211
Patricola, C. M., and Wehner, M. F. (2018). Anthropogenic influences on major tropical cyclone events. Nature 563, 339–346. doi: 10.1038/s41586-018-0673-672
Perillo, L. N., Neves, F. D. S., Antonini, Y., and Martins, R. P. (2017). Compositional changes in bee and wasp communities along Neotropical mountain altitudinal gradient. PLoS One 12:e0182054. doi: 10.1371/journal.pone.0182054
Peters, M. K., Hemp, A., Appelhans, T., Behler, C., Classen, A., Detsch, F., et al. (2016). Predictors of elevational biodiversity gradients change from single taxa to the multi-taxa community level. Nat. Commun. 7:13736. doi: 10.1038/ncomms13736
Revelle, W. R. (2017). psych: Procedures for Personality and Psychological Research. R Package Version 1.8.4.
Rocha, N. M. W. B., Carstensen, D. W., Fernandes, G. W., Le Stradic, S., Buisson, E., and Morellato, L. P. C. (2016). “Phenology Patterns Across a Rupestrian Grassland Altitudinal Gradient,” in Ecology and Conservation of Mountaintop grasslands in Brazil, ed. G. W. Fernandes, (Cham: Springer International Publishing), 275–289. doi: 10.1007/978-3-319-29808-5_12
Romero, G. Q., Marino, N. A. C., MacDonald, A. A. M., Ceìreìghino, R., Trzcinski, M. K., Mercado, D. A., et al. (2020). Extreme rainfall events alter the trophic structure in bromeliad tanks across the Neotropics. Nat. Commun. 11:3215. doi: 10.1038/s41467-020-17036-17034
Ruhí, A., Datry, T., and Sabo, J. L. (2017). Interpreting beta-diversity components over time to conserve metacommunities in highly dynamic ecosystems. Conserv. Biol. 31, 1459–1468. doi: 10.1111/cobi.12906
Schiesari, L., Matias, M. G., Prado, P. I., Leibold, M. A., Albert, C. H., Howeth, J. G., et al. (2019). Towards an applied metaecology. Perspect. Ecol. Conserv. 17, 172–181. doi: 10.1016/j.pecon.2019.11.001
Silveira, F. A. O., Barbosa, M., Beiroz, W., Callisto, M., Macedo, D. R., Morellato, L. P. C., et al. (2019). Tropical mountains as natural laboratories to study global changes: A long-term ecological research project in a megadiverse biodiversity hotspot. Perspect. Plant Ecol. Evol. Syst. 38, 64–73. doi: 10.1016/j.ppees.2019.04.001
Silveira, F. A. O., Negreiros, D., Barbosa, N. P. U., Buisson, E., Carmo, F. F., Carstensen, D. W., et al. (2016). Ecology and evolution of plant diversity in the endangered campo rupestre: a neglected conservation priority. Plant Soil 403, 129–152. doi: 10.1007/s11104-015-2637-2638
Soininen, J. (2014). A quantitative analysis of species sorting across organisms and ecosystems. Ecology 95, 3284–3292. doi: 10.1890/13-2228.1
Sutherland, W. J., Freckleton, R. P., Godfray, H. C. J., Beissinger, S. R., Benton, T., Cameron, D. D., et al. (2013). Identification of 100 fundamental ecological questions. J. Ecol. 101, 58–67. doi: 10.1111/1365-2745.12025
Terblanche, J. S. (2012). “Thermal relations,” in The Insects: Structure and Function, eds R. F. Chapman, S. J. Simpson, and A. E. Douglas, (New York: Cambridge University Press), 588–621.
Tiede, Y., Schlautmann, J., Donoso, D. A., Wallis, C. I. B., Bendix, J., Brandl, R., et al. (2017). Ants as indicators of environmental change and ecosystem processes. Ecol. Indic. 83, 527–537. doi: 10.1016/j.ecolind.2017.01.029
Trullas, S. C., van Wyk, J. H., and Spotila, J. R. (2007). Thermal melanism in ectotherms. J. Therm. Biol. 32, 235–245. doi: 10.1016/j.jtherbio.2007.01.013
von Humboldt, A., and Bonpland, A. (1805). Essai sur la géographie des plantes. Paris: Librarie Lebrault Schoell.
Weber, N. A. (1938). The biology of the fungus-growing ants. Part IV. Additional new forms. Part V. The Attini of Bolivia. Biología de las hormigas cultivadoras de hongos. Parte IV. Nuevas formas adicionales. Part V. Las Attini de Bolivia. Rev. Entomol. 7, 154–206.
Wills, B. D., Powell, S., Rivera, M. D., and Suarez, A. V. (2017). Correlates and Consequences of Worker Polymorphism in Ants. Annu. Rev. Entomol. 63, 575–598. doi: 10.1146/annurev-ento-020117-043357
Keywords: campo rupestre, metacommunity, beta-diversity, nestedness, species turnover, rupestrian grassland, traits, environmental instability
Citation: Nunes CA, Castro FS, Brant HSC, Powell S, Solar R, Fernandes GW and Neves FS (2020) High Temporal Beta Diversity in an Ant Metacommunity, With Increasing Temporal Functional Replacement Along the Elevational Gradient. Front. Ecol. Evol. 8:571439. doi: 10.3389/fevo.2020.571439
Received: 10 June 2020; Accepted: 16 October 2020;
Published: 10 November 2020.
Edited by:
Juliano André Bogoni, University of São Paulo, BrazilReviewed by:
Thiago Gonçalves-Souza, Federal Rural University of Pernambuco, BrazilMark Robertson, University of Pretoria, South Africa
Copyright © 2020 Nunes, Castro, Brant, Powell, Solar, Fernandes and Neves. This is an open-access article distributed under the terms of the Creative Commons Attribution License (CC BY). The use, distribution or reproduction in other forums is permitted, provided the original author(s) and the copyright owner(s) are credited and that the original publication in this journal is cited, in accordance with accepted academic practice. No use, distribution or reproduction is permitted which does not comply with these terms.
*Correspondence: Frederico S. Neves, ZnJlZC5uZXZlc0BnbWFpbC5jb20=