- 1Department of Ecology, Environment and Evolution, La Trobe University, Melbourne, VIC, Australia
- 2National Research Collections Australia, CSIRO, Canberra, ACT, Australia
Seed dispersal is a vital part of a plant’s life cycle that enables progeny to reach new sites suitable for burial and establishment. Awns are characteristic of many grass species and enable diaspores to self-disperse. Little is known, however, about the role of different awn morphologies in dispersal or burial. The objective of this study was to investigate the influence of awn morphology on diaspore surface dispersal and burial among representative Australian Poaceae. Surface dispersal, burial and microsite selectivity of diaspores were compared across 30 grass species and ten awn morphological types using laboratory and natural simulation experiments. The presence of an awn significantly facilitated surface dispersal and burial of diaspores, with awn length and hygroscopic activity being positively related to both surface dispersal and burial depth. Geniculate (once-bent) and bigeniculate (twice-bent) awns were the most efficient at surface dispersal. Burial efficiency, however, was influenced by microsite conditions; a surface obstruction increased burial for almost all awn types, while falcate (curved)-awned species were effective at burying without such objects. This study indicates that awn morphologies likely play different functional roles in the success of Australian grass progeny.
Introduction
Seed dispersal is a key stage in the life history of plants. The persistence of a non-clonal plant species relies on the ability of its seeds to move away from the parent plant and successfully bury at sites that have favorable conditions for germination and seedling establishment (Harper et al., 1970; Evangelista et al., 2011); this is often the only opportunity for plants to escape resource competition with conspecifics, occupy new habitats, and expand their range (Harper et al., 1970; Howe and Smallwood, 1982; Cain et al., 2000; Nathan, 2006). Indeed, the importance of effective seed dispersal and burial in facilitating the survival and distribution of plant species in the face of changing climates is increasingly recognized (Pearson and Dawson, 2003; Thomas et al., 2004; McConkey et al., 2012).
Diaspores (the dispersal unit of a plant, consisting of the seed and appendages that remain attached to the seed when it leaves the maternal plant) occur in a variety of morphologies which allow differential exploitation of a range of dispersal and burial techniques (Harper et al., 1970; Berg, 1975; Howe, 1980; Howe and Smallwood, 1982; Greene and Johnson, 1989). While the influence of abiotic and biotic vectors on diaspore dispersal are well understood, the ability of diaspores to disperse autochorously (self-disperse) is less well studied. Central to this process are awns, a long and rigid bristle-like seed appendage (Murbach, 1900; Peart, 1979; Stamp, 1984; Peart and Clifford, 1987; Adams and Tainton, 1990; Sindel et al., 1993; Garnier and Dajoz, 2001; Elbaum et al., 2007; Johnson and Baruch, 2014).
Awns are common in species of Geraniaceae (Stamp, 1984; Stamp, 1989; Evangelista et al., 2011) and Poaceae (Peart, 1979; Mallett and Orchard, 2002; Humphreys et al., 2011). The morphology of awns varies considerably across species in these families. For example, 20 awn types have been identified in Australian native grasses that vary in shape, seed attachment and number (Cavanagh et al., 2019). Awns can also either be passive (rigid) or hygroscopically-active. Hygroscopic awns become active when fluctuations in humidity and associated changes in cellular shape and pressure cause the awns to move, often by twisting (Murbach, 1900; Elbaum et al., 2007). This hygroscopic ability enables awns to mechanically move the attached seed across the soil surface and then bury it (Stamp, 1989; Garnier and Dajoz, 2001). Hygroscopic awns have been found to move diaspores across a soil surface up to 6 cm per day (Adams and Tainton, 1990) and have a competitive advantage over passively-awned diaspores in finding a safe-site (sensu Harper et al., 1965; Peart and Clifford, 1987). This dispersal capability is enhanced if the awn is geniculate, that is, it contains a sharp bend (Peart, 1979). Diaspores that do not possess awns are less likely to find suitable safe-sites within their habitat (Peart, 1979; Sindel et al., 1993).
Similarly, awnless diaspores are less likely to bury deeply into soil and require a longer period to bury to the same depths as awned diaspores (Stamp, 1984). Awns may act as levers to facilitate burial because they brace the diaspore as it penetrates the soil (Murbach, 1900; Stamp, 1989; Johnson and Baruch, 2014); geniculate awns have been found to be successful at doing this (Johnson and Baruch, 2014). Longer-awned diaspores have also been found to bury deeper into soil than diaspores with shorter awns of the same species (Garnier and Dajoz, 2001). In some species, however, the presence of an awn may hinder burial (Adams and Tainton, 1990).
Given that awns are a large resource investment for many grass species and potentially convey important plant fitness benefits (Adams and Tainton, 1990), it is surprising that more consideration has not been given to this variation. While some previous studies have examined the effect of awn morphology on diaspore dispersal (e.g., Peart, 1979, 1981), microsite selectivity (e.g., Fowler, 1986) and diaspore burial (e.g., Tothill, 1969; Peart, 1979), most studies on awns have been on the mechanics of hygroscopic awn movement (e.g., Murbach, 1900; Abraham and Elbaum, 2013; Elbaum and Abraham, 2014; Jung et al., 2014; Masrahi and Shaye, 2017), and the comparison between these active awns and rigid, passive awns (e.g., Peart, 1984; Peart and Clifford, 1987). Thus, the influence of awn morphology on dispersal and burial remains largely unknown. A better understanding of this would greatly improve our ability to predict the potential effectiveness of species with different awn morphologies in finding safe-sites and germinating under different conditions. In this study, we aimed to investigate the effect of awn type on the dispersal, microsite selection and burial of diaspores, both in laboratory experiments and in a simulated natural grassland setting. Since hygroscopic awns have been found to mechanically move diaspores (Stamp, 1989; Garnier and Dajoz, 2001) and that geniculate (sharply bent) awns have been found to act as levers (Johnson and Baruch, 2014), we hypothesized that the magnitude of autochorous surface dispersal and burial success would be highest for diaspores containing geniculate and hygroscopic awns, and it would be least for diaspores with straight and/or non-hygroscopic awns.
Materials and Methods
Diaspore Mass and Length Relationships
Diaspores from 28 Australian grass species and two introduced grass species (Cynosurus echinatus and Nassella neesiana) (Table 1), expressing ten different awn types (defined in Cavanagh et al., 2019), were collected from the field, either directly or via commercial seed banks. For each species, we measured the mass of ten air-dried diaspores (in mg) five times. Ten diaspores per species were then placed into a petri dish of water to allow hygroscopic awns to untwist, and then diaspore length, seed length, column length (the stouter lower section of the awn) and awn lengths were measured (mm) using a digital caliper. Species with awns that were hygroscopic were noted. We then determined the relationship between mean awn length and mean diaspore mass across all 30 study species.
Experiment 1: Surface Dispersal on Hardboard
We first quantified the ability of awned diaspores to disperse on a surface by performing a surface movement experiment on Masonite hardboard in the laboratory. Diaspores from each of the 30 study species were used in this experiment to allow for comparison of dispersal across study species and between awned and awnless diaspores. Experiments were conducted between November 2017 and March 2018 during which the laboratory temperature ranged from 23 to 26°C and humidity from 35 to 62%.
Standard 4.8 mm thick Masonite hardboards, measuring 60 cm × 45 cm, were used in the experiment. Masonite has one gently rough side impressed with a fine grid pattern that allows hairs and awns to grip the surface. This side of the board was painted white with one coat of low sheen paint to increase diaspore visibility while maintaining surface roughness. A 12 cm × 11 cm grid was then drawn onto the rough side of each board and each grid intersection was labeled 1–12.
Three intact (Awned) diaspores of four species were placed onto a board at a random grid intersection determined by a random number generator, with their callus pointing “north” in the center of each grid intersection and their awn pointing “south,” with any bend of the awn going out in a “westerly” direction (Figure 1A). This was replicated three times for all 30 species. Each board was sprayed with water 30 times (approximately 20 mL) using a 1 L spray bottle from a distance of 50 cm. The movement of the callus of each diaspore due to this wet cycle (W) was then traced and the location of their final point of movement was marked (W1). The awns were then allowed to dry out over a 24 h period and the location of the callus after this time [the dry cycle (D)] was marked (D1).
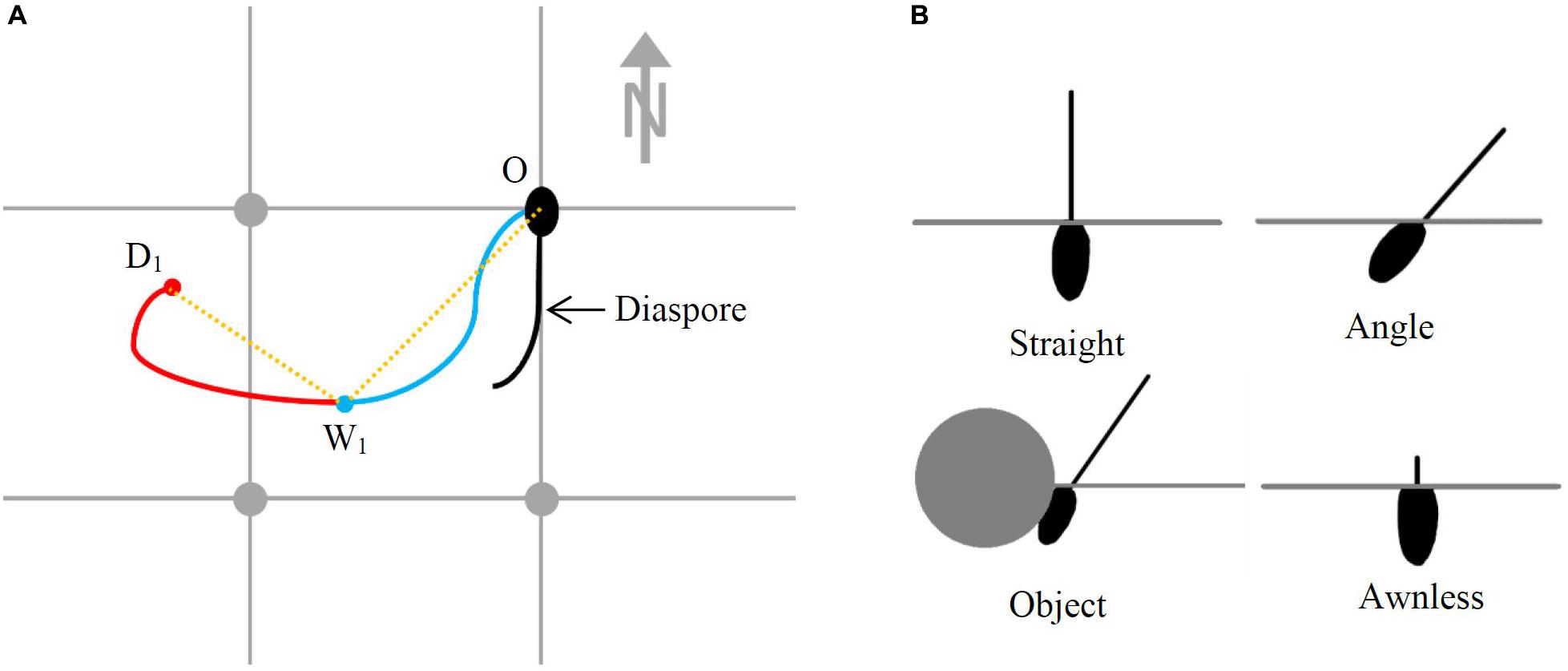
Figure 1. Methods employed to study diaspore (A) surface dispersal and (B) burial. In (A), O indicates the diaspore origin, W1 is the location of the diaspore callus after the first wet cycle, D1 is the location of the diaspore callus after the first dry cycle, the blue line is the movement path of the diaspore callus in the wet cycle, the red line is the movement path of the diaspore callus in the dry cycle and the orange dotted lines are the displacement lengths measured. In (B), the horizontal lines indicate the soil surface.
The displacement of the callus due to both W and D (Figure 1A) was measured (mm) using a digital caliper. Spraying was repeated and W and D displacements measured for five cycles. The total absolute distance moved (TDA) across all five W and D cycles was calculated for each diaspore and the mean TDA was calculated for each species. Awns were removed from a further six diaspores per species by cutting off the awn at its base. The surface dispersal of the Awnless diaspores for all 30 study species was then measured using the same procedure as the Awned diaspores. Diaspores that moved off the board were undisturbed until their current cycle had ceased, after which their dispersal distance was measured and they were placed back on the board in an unobtrusive position. The awn became detached from one diaspore (Chrysopogon fallax) during movement, and this replicate was removed.
The mean TDA for each species in the Awned treatment was compared to the mean TDA in the Awnless treatment. The results indicated that TDA was negligible for diaspores lacking awns, and so we focused only on data collected from awned diaspores for further investigation of species- and awn type-differences in diaspore surface dispersal. Linear mixed-effects model analysis was performed using nlme in R (version 3.1–137) to determine species effects on surface dispersal of diaspores. TDA was the response variable and species (fixed) and board number (random) were the predictor variables; data were transformed [y = ln(x + 1)] to fit the model assumptions. Post hoc comparison of species was performed using least-square means derived from the linear mixed-effects model (using R program lsmeans version 2.30-0).
A linear regression was used to determine the relationship between mean awn length and mean TDA of each species. To compare the diaspore dispersal across awn types, the TDA of all seeds was standardized by dividing by the mean species awn length (TDS). Linear mixed-effects model analysis was then performed using nlme in R with TDS as the response variable, and awn type (fixed) and both species and board number (random) as the predictor variables. Post hoc comparison of awn types was performed using least-square means derived from the linear mixed-effects model using lsmeans in R. Awn types lacking species-level replication (Types 9, 10, 13, and 20) were excluded from the analyses. Data were again transformed [y = ln(x + 1)] to fit the model assumptions.
Experiment 2: Burial Depth in Soil
To quantify the variation in burial ability among species with different awn types, a controlled glasshouse experiment was undertaken using four burial treatments on a homogenous soil between February 19, 2018 and March 12, 2018. In the glasshouse, daily fluctuations in humidity ranged from <20 to 76% and temperatures ranged from 13 to 56°C.
Six plastic trays, each 35 cm × 90 cm, were filled to 5 cm depth with a mixture of four-parts sandy loam topsoil to one-part potting mix; potting mix was added to keep the soil friable. Trays were then well-watered to settle the soil. Four rows, approximately 7 cm apart, were aligned in each tray, and one of each of four treatments (Straight, Angle, Object, and Awnless) was randomly allocated to a row. One diaspore from each of the 30 study species (Table 1) was used per treatment per tray. Species position within a row (treatment) was randomly assigned (however, with positions alternating between longer- and shorter-awned species to reduce the chances of the awns interacting with each other). The species position was replicated in each treatment within a tray.
Diaspores were inserted into moist soil, with the seed just covered and the awns exposed, in the treatments as follows: vertically (Straight), at 45° (Angle), at 45° lodged under a 3 cm diameter piece of roughened dowel (Object) and vertically with the awn removed (Awnless) (Figure 1B). Toothpicks were inserted beside each Awnless diaspore to increase their visibility. Each replicate was misted with 90 sprays of water (approximately 60 mL) every second day, using a 1 L spray bottle. After 21 days, the trays were lightly sprayed with white spray paint, which dried almost immediately on contact, to color the exposed part of the awn. Each seed was then removed from the soil and the depth of burial was measured (mm) from the length of the unpainted part of the awn. Mean absolute burial depth (BDA) was calculated for each species under each treatment.
As diaspores in the Awnless treatment group recorded no burial for all 30 species, this treatment was removed from further analyses. Linear mixed-effects model analysis was performed using nlme in R to determine treatment and species effects on burial of diaspores. BDA was the response variable with treatment (Object vs. Angle vs. Straight; fixed), species (fixed), species × treatment (fixed) and tray (random) as the predictor variables. Some species recorded 0 mm mean BDA in at least one of the Straight, Angle and Object treatments. Therefore, analysis of treatment and species effects was only performed on 15 species that had non-zero means (see Supplementary Table 2). Data were transformed [y = ln(x + 1)] to fit the model assumptions. Post hoc comparison of treatments within species was performed using least-square means derived from the linear mixed-effects model using lsmeans in R.
Linear regression analysis was used to determine the relationship between awn length and BDA of each species in the Object treatment. To compare the burial depth across awn types in the three treatments, the BDA of all seeds was standardized by dividing by the mean species awn length (BDS). Linear mixed-effects model analysis was then performed using nlme in R to determine treatment and awn type effects on burial of diaspores. Of the 15 species that had non-zero means (Supplementary Table 2), only awn types with species-level replication, in this case falcate (Type 2) and bigeniculate (Type 4), were included in the analysis. Data were transformed [y = ln(x + 1)] to fit the model assumptions. BDS was the response variable with treatment (Object vs. Angle vs. Straight; fixed), awn type (falcate vs. bigeniculate; fixed), awn type × treatment (fixed) and both species and tray (random) as the predictor variables. Post hoc comparison of awn types within treatments was performed using least-square means derived from the linear mixed-effects model using lsmeans in R.
Experiment 3: Surface Dispersal, Lodgment and Burial in a Simulated Natural Grassland
The objective of this experiment was to observe and record the dispersal and burial of different awn types in a simulated, natural grassland environment. This experiment was conducted from February 14, 2018 to March 07, 2018.
Six trays (70 cm × 60 cm) were lined with cardboard and filled to a depth of 5 cm with a soil mixture consisting of four-parts loamy grassland soil to one-part sandy loam topsoil. The soil was mixed thoroughly and all stones greater than 2 cm were removed. Ten rocks, 3–5 cm in size, were sporadically placed over the soil, followed by scattering of approximately 2 L of loosely packed grass debris and 1 L of leaf and bark litter less than 10 cm in size, resulting in a thickness of approximately 5 mm. The soil was then soaked with water and allowed to drain.
Toothpicks were inserted into the soil in a 5 × 7 grid (approximately 8 cm apart) in each tray to mark the origin of each diaspore. Diaspores from seven species (Table 1), each with a different awn type, were then placed at the base of each toothpick in a random sample across each tray so that there were five diaspores per species per tray. Diaspores were then left to move in response to the fluctuations in daily humidity. Trays were placed into a glasshouse where humidity ranged from <20 to 76%, while temperatures ranged from 13 to 56°C. The location and orientation of the diaspores was recorded every few days to reduce the chance of losing diaspores in the leaf litter. Every 7 days, a rainfall event was simulated by coarsely spraying each tray with 2 L of water, representing an approximate 4.8 mm rainfall event. At this amount, the surface material was completely wet.
After 3 weeks, the distance moved across the surface from the starting position was measured for each diaspore (mm), along with records of (1) whether the diaspore was lodged or not, (2) what it was lodged under (i.e., microsite type: 3–5 cm rock, <3 cm pebble or soil clod, bark or leaf litter, grass litter, crack or hole, or bare soil) and, (3) the depth of burial (mm). Depth of burial was determined by first clasping tweezers on the diaspore at the location on the awn that the diaspore was buried up to, and then carefully extracting the diaspore in the tweezers and measuring the distance from this point to the diaspore callus.
Mean surface dispersal (TDA) and mean burial depth (BDA) for each species were calculated from the diaspores that were found, and the diaspores that were lodged, respectively. Linear mixed-effects models were used to compare mean TDA and mean BDA between species using nlme in R. In both models, TDA and BDA were response variables, with species (fixed) and tray (random) as the predictor variables; data were transformed [y = ln(x + 1)] to fit the model assumptions. Post hoc comparison of species was performed using least-square means derived from the linear mixed-effects model using lsmeans in R. The percentage of diaspores that were lodged under each microsite type was calculated for each species, and a chi-square analysis was performed with lodgment (lodged vs. not lodged) and species as blocking variables.
Results
Diaspore Mass and Length Relationships
There was a significant relationship between awn length and diaspore mass, with a 1 mg increase in the mass of 10 diaspores corresponding to a 2 mm increase in awn length (length = 0.52[10 × mass] + 17.84, r = 0.76, P < 0.0001).
Mean awn length was found to be longest in the alpine species Austrostipa nivicola (97.0 ± 1.4 mm), followed by Austrostipa scabra (75.8 ± 2.7 mm). Enneapogon nigricans, a widespread inland perennial, had the shortest awns (4.6 ± 0.8 mm). A. nivicola also had the highest mass (148.2 ± 10.8 mg per 10 diaspores), while Chloris truncata diaspores were lightest (2.3 ± 0.1 mg per 10 diaspores). The awn made up the highest percentage of the diaspore length in Austrostipa verticillata and A. scabra (both 92%), while the awn only made up 59% of the spinifex Triodia danthoniodes diaspore. See Supplementary Table 1 for mass and length data for all 30 study species.
Experiment 1: Surface Dispersal on Hardboard
During five wet-dry cycles, the mean total distance moved (TDA) by diaspores on the hardboard ranged from 0 to 500.1 mm across all 30 species. The mean TDA of awned diaspores was 158.0 ± 29.4 mm, while the mean TDA of diaspores with awns removed was 2.7 ± 0.7 mm, with awned diaspores moving significantly further than awnless diaspores for all species (P < 0.04) except C. echinatus (P = 0.31) and Echinopogon ovatus (P = 0.13) (both having straight, non-hygroscopic awns). Awned diaspores moved more than 500 times that of awnless diaspores for four species [N. neesiana (424.5 mm vs. 0.5 mm), Austrostipa mollis (437.7 mm vs. 0.6 mm), Austrostipa rudis (349.6 mm vs. 0.5 mm), and A. nivicola (500.1 mm vs. 0 mm); all with bigeniculate awns (Type 4)], and between ten and 100 times more for a further 11 species. Among diaspores with awns removed, Aristida latifolia moved the greatest distance (19.3 ± 2.8 mm), but diaspores of virtually all other species moved little or not at all, clearly demonstrating the vital role played by awns in diaspore movement (Figure 2).
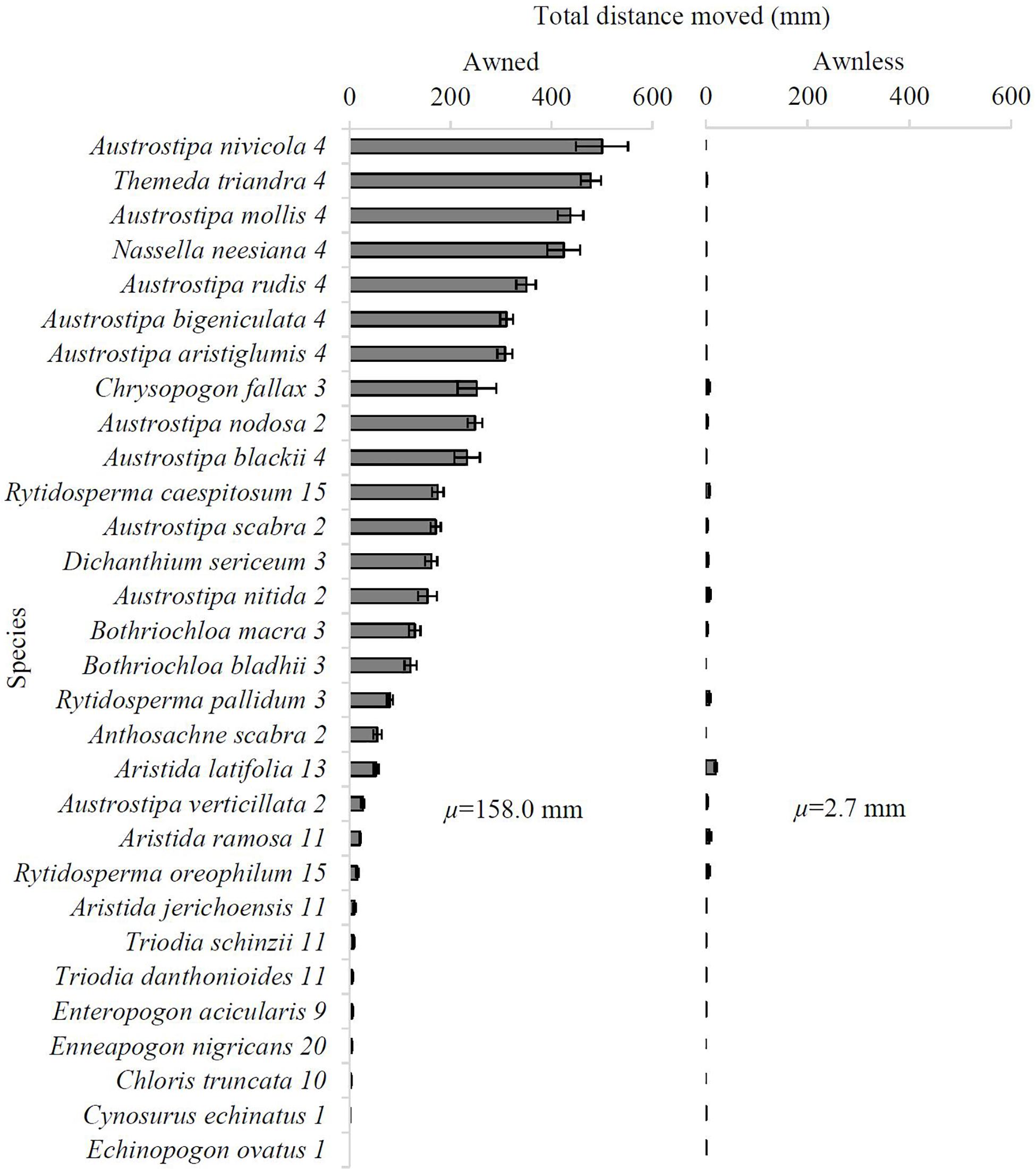
Figure 2. Mean total distance moved (TDA) (in mm) by diaspores across a hardboard in the surface dispersal experiment after five wet-dry cycles for the 30 study species in both the Awned (nine replicates per species) and Awnless (awn removed) (six replicates per species) treatment. Error bars represent one standard error. Species name is followed by awn type (see Table 1). μ = mean TDA (mm) of each treatment.
There was a significant effect of species on the TDA of diaspores (F29,210 = 124.10, P < 0.0001). A. nivicola moved the greatest total distance (500.1 ± 51.5 mm); however, this was not significantly different to the TDA of the next top nine species (Figure 2). The top seven species that moved the greatest distance were all species with bigeniculate (twice-bent) apically-attached, hygroscopic awns (Type 4; Table 1 and Figure 2). E. ovatus and C. echinatus, both with a single, apical straight awn (Type 1), moved the least distance (0 mm and 1.3 ± 0.6 mm, respectively; Figure 2).
Overall, there was a significant positive relationship between awn length and TDA of awned diaspores (r = 0.76, P < 0.0001; Figure 3A) with each 1 mm increase in awn length resulting in a 5.2 mm increase in TDA. The relationship showed that Types 4 (apical, bigeniculate) and 3 (apical, geniculate) moved more than predicted (based on awn length alone), while Types 2 (apical, falcate), 11 (three apical, straight) and 13 (three apical with column, e.g., A. latifolia) moved less (Figure 3A).
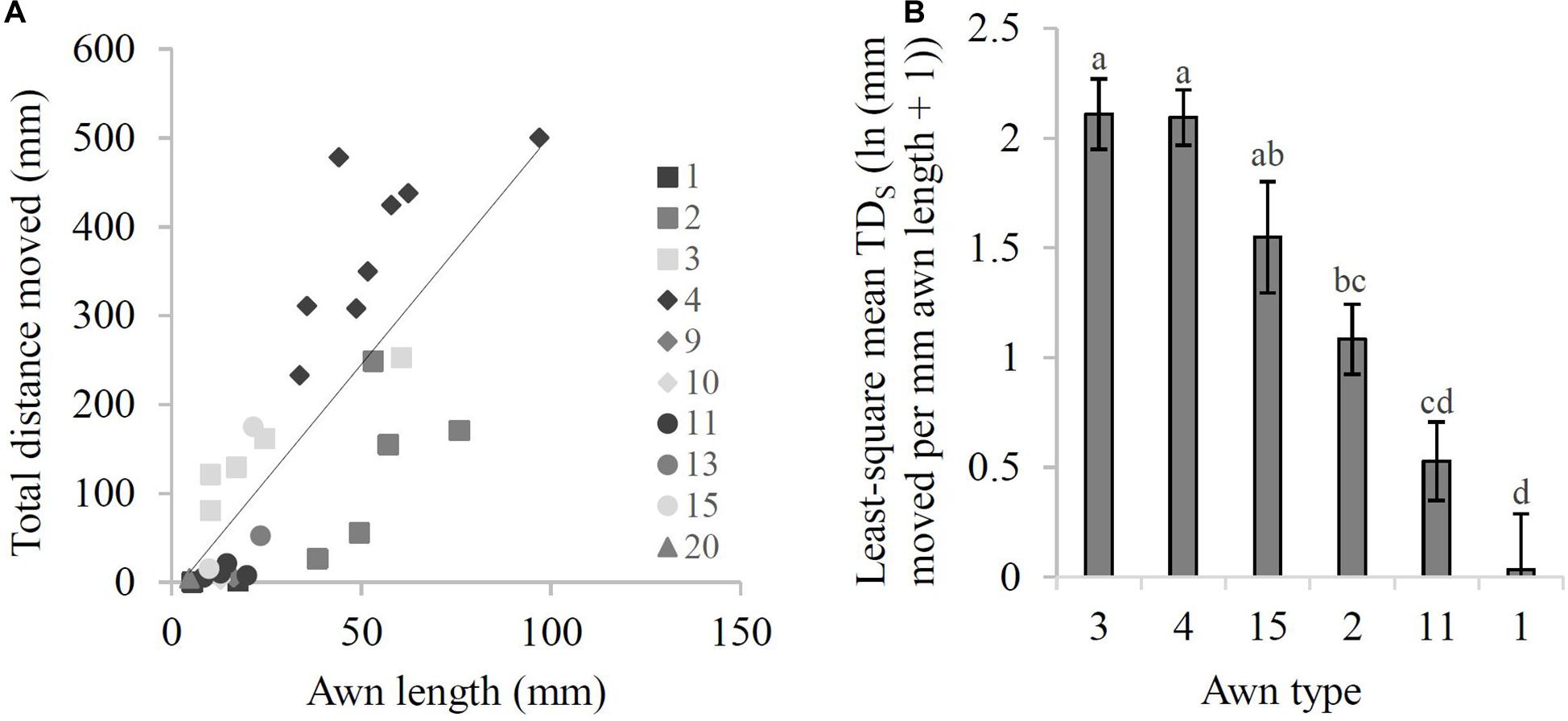
Figure 3. Distance moved by awn types in the hardboard experiment. (A) Linear regression of awn length against total distance moved (TDA) (in mm; after five wet-dry cycles) for all 30 species (y = 5.17x – 13.29, r = 0.76). Legend shows awn type. (B) Least-square mean values for total distance moved (after five wet-dry cycles) standardized by awn length (TDS) [ln (mm moved per mm awn length + 1)]. Error bars represent one standard error. Means sharing the same letter do not differ at the 0.05 level.
The total distance dispersed standardized by awn length (TDS) differed significantly across the six awn types tested (Types 1–4, 11, and 15) (F5,20 = 21.19, P < 0.0001). Types 3 (apical, geniculate) and 4 (apical, bigeniculate) were the most efficient at surface dispersal [least-squared means = 2.1 ± 0.2 ln (mm moved per mm awn length + 1) and 2.1 ± 0.1 ln (mm moved per mm awn length + 1), respectively; Figure 3B]. Examples include Bothriochloa macra and Dichanthium sericeum (Type 3) and Themeda triandra and N. neesiana (Type 4). In contrast, Type 1 (apical, straight) was the least efficient at surface dispersal [least-squared mean = 0.03 ± 0.3 ln (mm moved per mm awn length + 1)], but this was not significantly less than Type 11 [three apical straight awns; least-squared mean = 0.5 ± 0.2 ln (mm moved per mm awn length + 1)] (Figure 3B). Representative species of these weakly moving species were E. ovatus and C. echinatus (Type 1), and the spinifex grasses Triodia schinzii and Triodia danthonioides (Type 11).
Experiment 2: Burial Depth in Soil
The mean burial depth (BDA) of diaspores ranged from 0 to 12.9 mm, increasing across Straight (vertically inserted into soil; 1.1 ± 0.2 mm), Angle (inserted into soil at 45°; 1.6 ± 0.5 mm) and Object (inserted into soil at 45° lodged under a 3 cm diameter piece of roughened dowel; 4.6 ± 0.8 mm) treatment groups (Figure 4). Awnless diaspores never buried (0 mm) in any of the 30 species. In the Object treatment group, Austrostipa bigeniculata (Type 4) achieved the deepest burial (12.9 ± 3.6 mm; Figure 4). In the Angle treatment and the Straight treatment, the deepest burial was achieved by Austrostipa nodosa (11.2 ± 2.2 mm) and Austrostipa nitida (5.0 ± 1.4 mm; Figure 4), both of which have a single falcate awn (Type 2). The top five species to bury the deepest in all treatments had hygroscopic awns and were either from awn types 2 or 4, while E. nigricans (non-hygroscopic) did not bury in any treatment (0 mm; Figure 4).
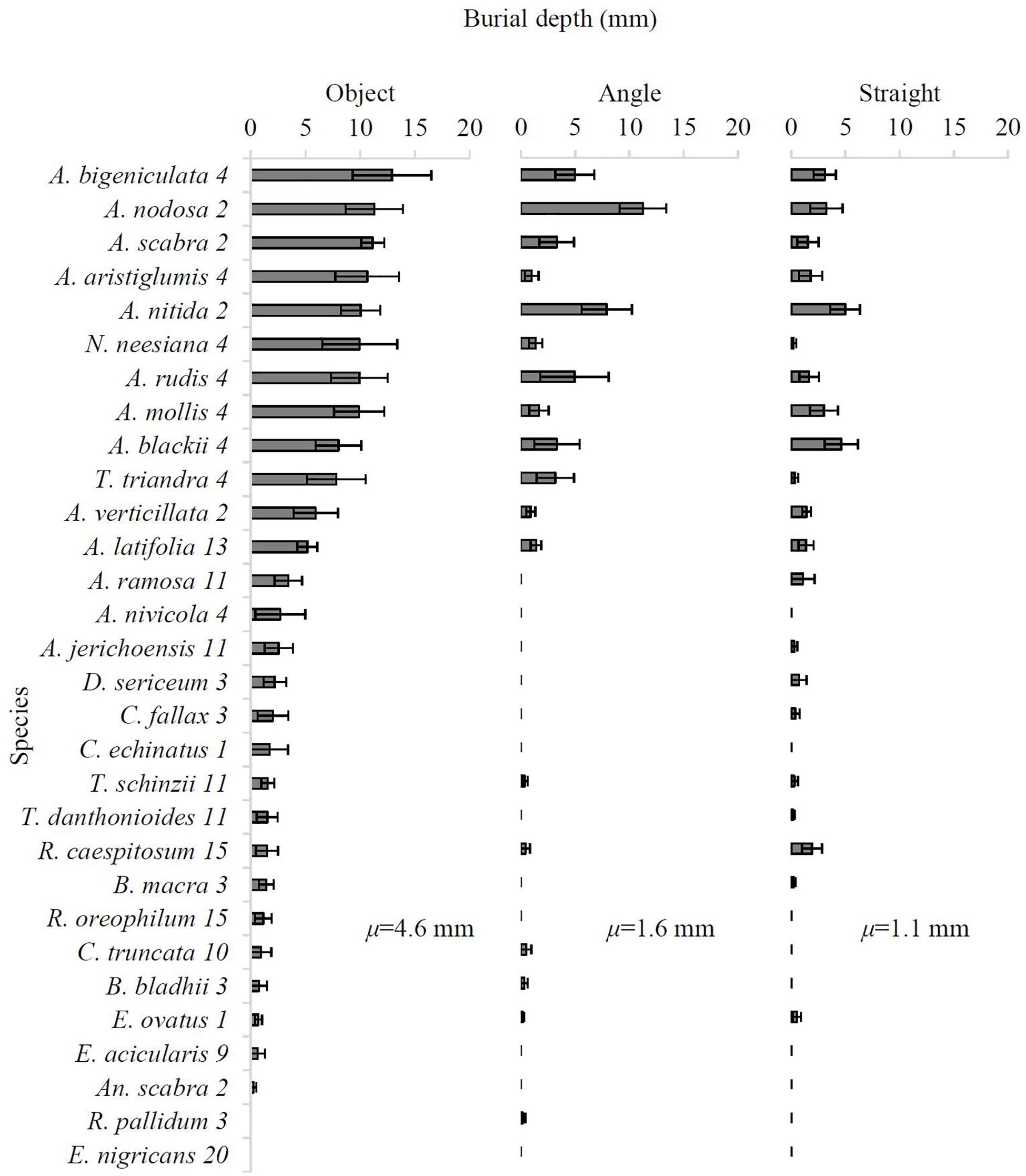
Figure 4. Mean burial depth (BDA) (in mm) of diaspores for all 30 species across the Object, Angle, and Straight treatment classes (six replicates per species per treatment) after 21 days. Error bars represent one standard error. Species name is followed by awn type (see Table 1). μ = mean BDA (mm) of treatment.
Linear mixed-effects model analysis showed that the species × treatment interaction effect was not significant (F28,220 = 1.41, P = 0.09), indicating that each species BDA had a similar response across the different treatments. However, this analysis showed that there was a significant effect of both species (F14,220 = 6.57, P < 0.0001) and treatment (F2,220 = 42.70, P < 0.0001) on BDA. Austrostipa aristiglumis, N. neesiana, A. rudis (all with single bigeniculate awns; Type 4) and Austrostipa scabra (single falcate awn; Type 2) buried significantly deeper in the Object treatment compared to the Angle and Straight treatments (10.6 mm vs. 1.0 mm vs. 1.8 mm, 10.0 mm vs. 1.3 mm vs. 0.2 mm, 9.9 mm vs. 4.9 mm vs. 1.6 mm, and 11.2 mm vs. 3.3 mm vs. 1.6 mm, respectively; P < 0.05; Figure 4). Additionally, a number of shallow-burying species (e.g., Enteropogon acicularis, Rytidosperma oreophilum, and C. echinatus) only buried when adjacent to an object (Figure 4).
The linear regression analysis performed on the Object treatment data showed a significant positive relationship between awn length and BDA (r = 0.61, P < 0.001; Figure 5A), with a 10 mm increase in awn length resulting in a 1 mm increase in BDA. The burial depth standardized by awn length (BDS) did not have a significant awn type × treatment interaction effect (F2,128 = 2.31, P = 0.10) showing that the BDS of bigeniculate (Type 4) and falcate (Type 2) awn types responded similarly across the three treatments. There was a significant main effect of treatment (F2,128 = 42.49, P < 0.0001) with both awn types burying deeper in the Object treatment compared to the Angle and Straight treatments [Type 2: least-squared means = 0.16 ln (mm buried per mm awn length + 1) vs. 0.09 ln (mm buried per mm awn length + 1) vs. 0.05 ln (mm buried per mm awn length + 1), respectively; Type 4: least-squared means = 0.19 ln (mm buried per mm awn length + 1) vs. 0.06 ln (mm buried per mm awn length + 1) vs. 0.05 ln (mm buried per mm awn length + 1), respectively; Figure 5B]. However, there was no significant effect of awn type on BDS (F1,9 = 0.002, P = 0.97). Within treatments, bigeniculate awns were more proficient at burial compared to falcate awns in the Object treatment, while the opposite was true in the Angle treatment (Figure 5B), although a post hoc comparison indicated that there was no significant difference in BDS between the two awn types within any of the treatments (Figure 5B).
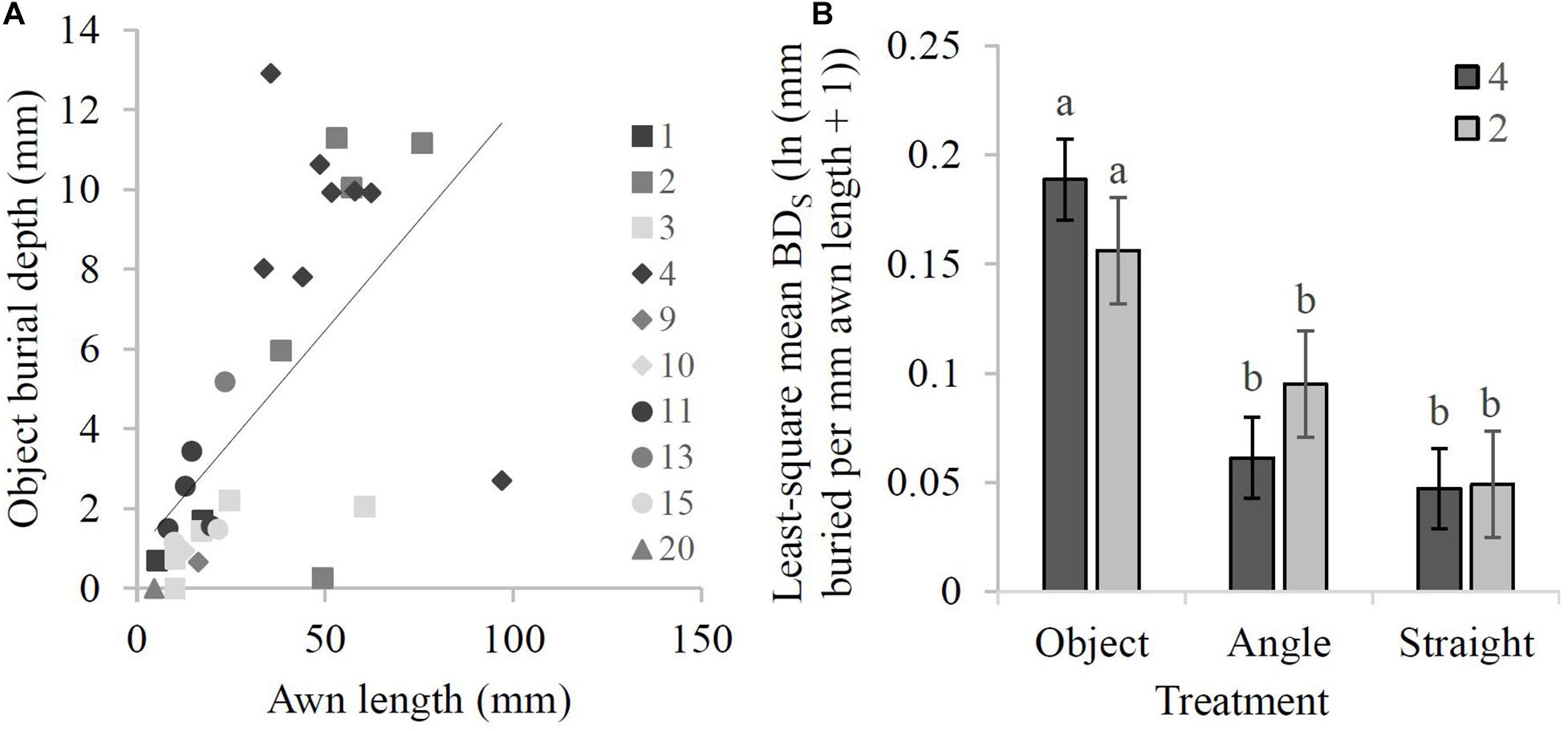
Figure 5. Depth buried by awn types in the burial experiment. (A) Linear regression of awn length against burial depth (BDA) (in mm; after 21 days) for all 30 species in the Object treatment (y = 0.1107x + 0.9274, r = 0.61). Legend shows awn type. (B) Least-square mean values for burial depth (after 21 days) across the three treatments standardized by awn length (BDS) [ln (mm buried per mm awn length + 1)]. Error bars represent one standard error. Means sharing the same letter do not differ at the 0.05 level. Legend shows awn type.
Experiment 3: Surface Dispersal, Lodgment and Burial in a Simulated Natural Grassland
A total of 8% (17/210) of diaspores were not recovered at the end of this experiment and so were excluded from the analysis. Of the 193 diaspores that dispersed, 54% (105/193) lodged. There was a significant effect of species on TDA (F6,181 = 28.38, P < 0.0001) with the hygroscopically-awned T. triandra (Type 4) moving a significantly greater distance across the surface than all other species (76.0 ± 7.1 mm; P < 0.05; Figure 6A). There was also a significant effect of species on BDA (F6,93 = 6.26, P < 0.0001), with T. triandra, B. macra, and A. scabra burying the deepest of the seven species (12.4 mm, 6.4 mm, and 5.9 mm, respectively), and E. acicularis, C. echinatus, and E. nigricans burying the least (<4 mm; Figure 6B).
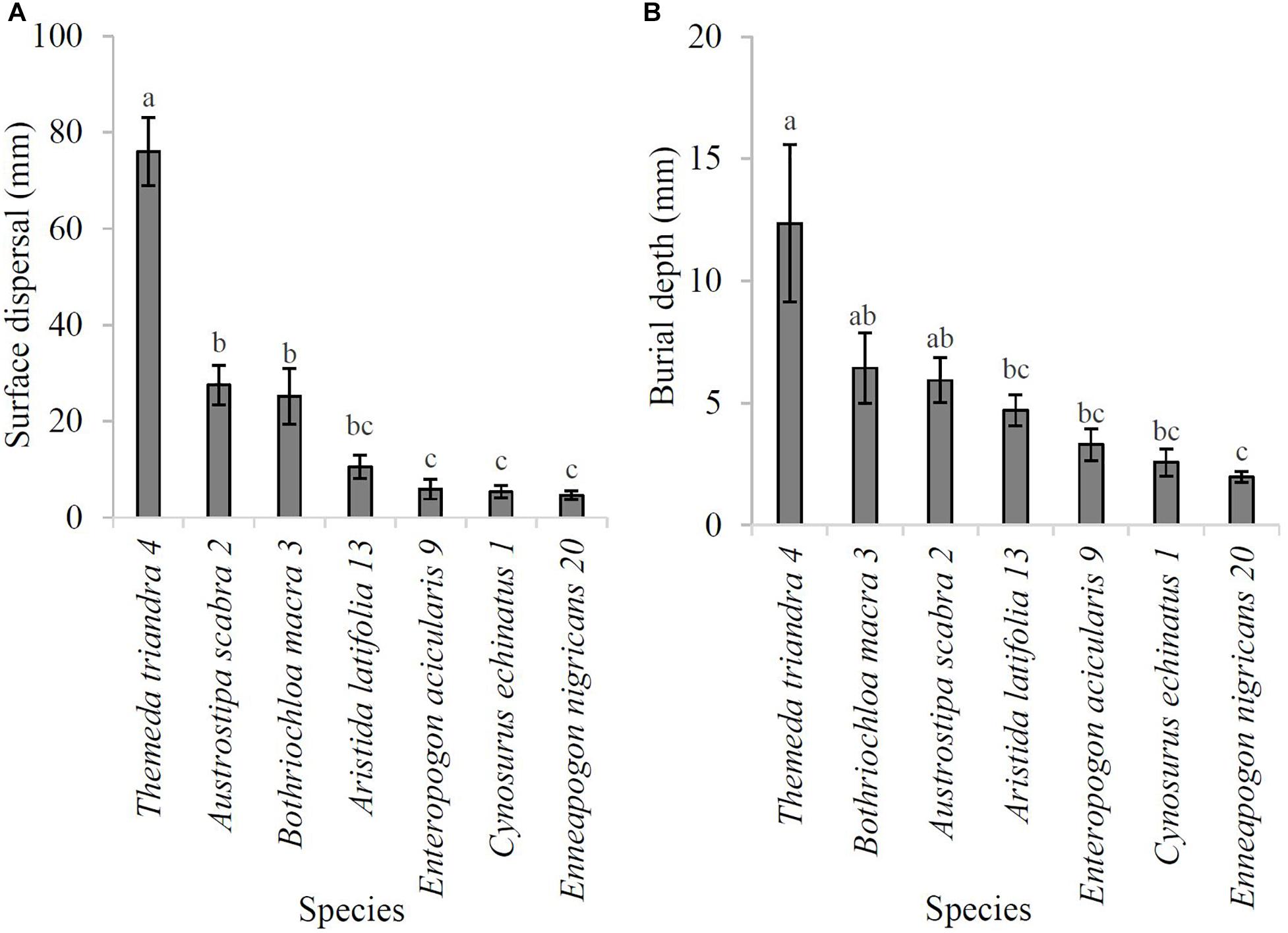
Figure 6. (A) Mean surface dispersal (TDA; in mm) and (B) mean burial depth (BDA; in mm) for the seven study species in the natural experiment after 21 days. Error bars represent one standard error. Species name is followed by awn type (see Table 1). Means sharing the same letter do not differ at the 0.05 level.
There was a significant difference in the percentage of diaspores that became lodged and diaspores that did not across the seven study species (χ26 = 31.05, P < 0.0001). Diaspores of A. latifolia (three apical awns and a column; Type 13) had the highest frequency of lodgment (87%; Figure 7), while the introduced species C. echinatus (single straight awn; Type 1) had the lowest (32%; Figure 7). A. latifolia, A. scabra and E. nigricans diaspores had the highest percent lodgment into bare soil (42%, 33%, and 42%, respectively; Figure 7). B. macra (single geniculate awn; Type 3) lodged the most under soil clods or pebbles (47%; Figure 7). While only 38% of T. triandra diaspores lodged, most of these also lodged under soil clods or pebbles (64%; Figure 7). E. acicularis (two apical straight awns; Type 9) and C. echinatus lodged at a comparatively higher frequency in cracks or holes (36% and 33%, respectively; Figure 7).
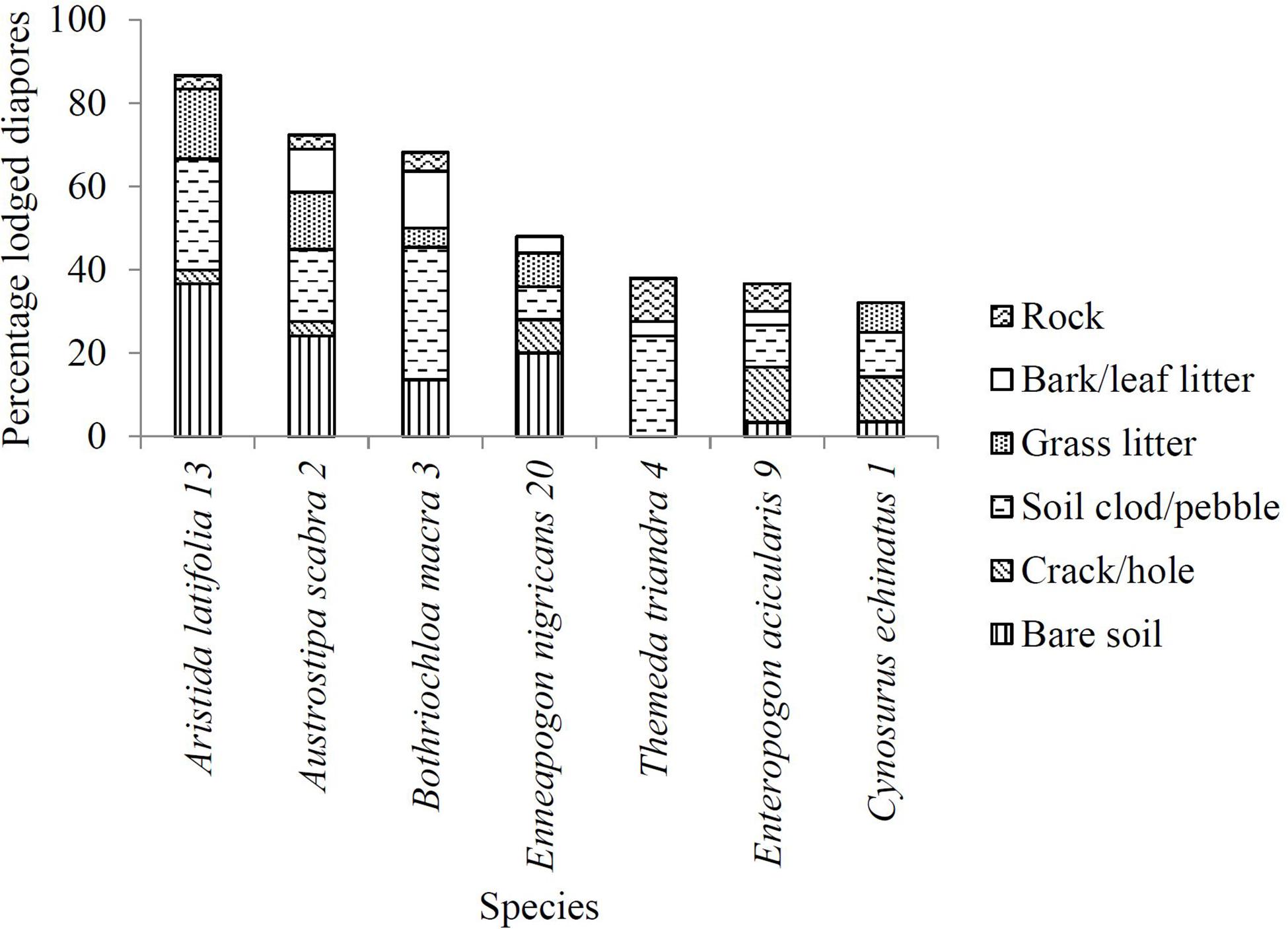
Figure 7. The percentage of diaspores that lodged at each of the different microsites in the natural experiment after 21 days. Species name is followed by awn type (see Table 1).
Discussion
This study aimed to determine the relationships between awn morphology and diaspore dispersal and burial. Our results clearly demonstrate two key features of these relationships. First, the presence of virtually all awn types dramatically increases both surface dispersal and burial of associated diaspores, with diaspores that have their awns mechanically removed showing virtually no capacity for surface movement or soil burial. Second, while diaspores with longer awns tended to disperse further and bury deeper than short-awned types, both species and broad awn types varied considerably in these abilities. Both lines of evidence support the hypothesis that diaspore characteristics, and particularly awn morphology, play a vital role in the ability of grass species to effectively exploit different ecological niches (Peart, 1979).
We found that awns greatly enhanced diaspore dispersal distance across the surface and was instrumental in facilitating burial into the soil. These results are consistent with those of Peart (1979); Stamp (1984), and Sindel et al. (1993) who also found that diaspores with hygroscopic awns that were then removed were unable to readily find safe-sites (sensu Harper et al., 1965) and did not bury. While there has been ongoing debate about whether the primary purpose of awns is for burial or surface dispersal, and some studies have occasionally shown that awns can hinder seed burial (e.g., Adams and Tainton, 1990), our data indicate that awns, particularly certain forms, play a central role in both processes.
Our data showed that hygroscopic geniculate (once-bent) and bigeniculate (twice-bent) awn types were the most efficient at moving across the surface of both the hardboard and the simulated grassland surfaces. Geniculate awns apparently have a greater capacity for surface dispersal because the “knee-bends” in their awn, which are pivot points, act as levers to facilitate forward movement (Peart, 1979). The greater the distance that a diaspore moves, and hence the area of habitat that it samples, the likelihood it increases its chances of reaching a safe-site and reduces exposure to threats such as desiccation, granivory and fire (Sheldon, 1974; Peart, 1984; Stamp, 1984). The effectiveness of geniculate awns in increasing surface diaspore dispersal may explain why these awn types are the most common and taxonomically diverse among native Australian grasses (Cavanagh et al., 2019). Investigations are required to determine if geniculate-awned species have wider distributions or inhabit a greater diversity of habitat types compared to species with other awn types.
In general, species with longer awns also had a greater mass and were able to move further across the hardboard surface than species with short awns. However, there were some exceptions; falcate (curved)-awned species, such as A. scabra and A. nitida, had awns that were just as long as some of the bigeniculate-awned species but were lighter and did not disperse as far. Thus, the efficiency of falcate awns in dispersing was significantly less than that of geniculate awns. Phylogenetic analyses have shown that these falcate-awned species fall into a distinct Austrostipa subgenus Falcatae (Bustam, 2010; Syme et al., 2012; Winterfeld et al., 2015), and may have evolved separate strategies for dispersal. We also found that awn length was positively correlated with overall burial depth, consistent with Garnier and Dajoz (2001) and Johnson and Baruch (2014). Again, however, there were exceptions; A. nivicola had the longest awns of all study species but buried less than a quarter of the depth of A. bigeniculata, which has awns less than half as long. A. nivicola is found in the alpine region of Australia where soils are rich in organic matter (VicFlora, 2016), so the need for the awn to facilitate burial in these soil types may be less necessary. Similarly, C. fallax had long awns and buried shallower than expected. This species is common on floodplains (PlantNET, 2004) where the soil is also likely to be more fertile and friable (Victorian Resources Online, 2017). These patterns suggest that there may be significant differences in awn function across different soil types, and that awn length itself may not influence dispersal and burial efficiency. This warrants further study.
Overall, we found that the presence of a solid object (here a wooden dowel) on the soil surface generally increased the depth that diaspores were able to bury and, for many species, was essential for burial. Objects provide a contact point for the awns to work against, enabling the awn to act as a lever and direct awn torque downward (Johnson and Baruch, 2014). Meanwhile, some species, especially those with falcate awns, were especially capable of burial when placed at an angle to the soil but in the absence of a surface object (Angle treatment group). As noted previously, falcate awns were poor at surface dispersal, implying that the functional role of the awn in these species is to enhance opportunistic seed burial and minimize the reliance on reaching specific microsites. This idea was supported by observations from the grassland simulation experiment where it was found that A. scabra had the highest number of its diaspores lodge into bare soil. In contrast, the grassland simulation showed that the geniculate- and bigeniculate-awned species, B. macra and T. triandra respectively, had the highest percent of diaspores lodge under soil clods or pebbles. These species were efficient at surface movement and would, therefore, be able to disperse more widely across the soil to find microsites that are more suitable for burial. These results suggest that species with different awn types may have different microsite preferences. Microsite structure was also shown to influence diaspore burial and rate of germination by Harper et al. (1965) and Fowler (1986), with different species shown to prefer particular microsites. Further, it has been found that burying in a position that increases soil moisture contact improves seed germination (Sheldon, 1974) and additional study should be undertaken to determine if awns play a role in germination success.
Although there was some variation in the efficiency of awn types between surface dispersal and burial ability, some awn types were ineffective at both strategies. There was little to no difference in dispersal distance achieved between awned and awnless diaspores of C. echinatus and E. ovatus, which both have a single straight, passive (non-hygroscopic) awn, indicating that these awns are not used for surface dispersal. C. truncata and E. acicularis have two straight, passive awns, and were similarly poor at surface dispersal. In the natural grassland, two of these species (C. echinatus and E. acicularis) also had the highest percentage of diaspores lodge into cracks or holes. These results suggest that burial into the soil is not always directed and can occur by chance. This is supported by Peart (1984) who found that passively awned diaspores rely on stochastic processes to reach safe-sites. While these awn types appear to be functionally redundant for active surface dispersal and burial, they may still serve to orientate diaspores during their fall from the maternal plant and increase their chance of landing on the soil in an optimal position for germination (Harper et al., 1965; Peart, 1981). Diaspores of E. nigricans also had poor surface dispersal and burial but, considering their small mass and the presence of nine short awns, it is likely that this species relies on wind dispersal. Additional study of the role of passive awns, including the potential role of animal dispersal of grass diaspores, would help clarify these questions, particularly for species that do not disperse autochorously.
Finally, in this experiment we used Masonite hardboard as a low-cost alternate for quantifying surface dispersal across multiple species. This approach has many benefits, including easy visibility of small diaspores, precise measurement of diaspore orientation and movement, and the ability to make a record of diaspore movement (by tracing the dispersal path). The near-perfect replication between relative total surface dispersal of seven grass species on both Masonite and in the simulated grassland environment (c.f. Figures 2, 6A) supports the use of hardboard as a potential surrogate for future experiments conducted in natural environments, which are more costly, and difficult to perform and analyze.
Further avenues for the future study of awn morphological variation in Australian grasses include detailed investigation of the relationship between awn type and plant life history (e.g., dispersal may be more important for annual species compared to perennials; Humphreys et al., 2011), and the influence of awn microstructure on awn activity. For example, Masrahi and Shaye (2017) found that microstructure of the hygroscopic awn alters the burial capability of diaspores between species. Further investigation of the linkages between diaspore fitness, awn structure and soil characteristics would also be beneficial, given the often tight association of grass species and soil types across Australia.
We conclude that many awn types significantly facilitate surface dispersal and burial of diaspores, and that different awn types potentially have different functional roles in the diaspore dispersal and burial stage of a plant’s life history. It is likely that such a large diversity of awn morphologies persists in Australian grasses due to this variation in function. The results of our study therefore strongly argue for a significant fitness benefit associated with investment of reproductive resources into awns, particularly geniculate and bigeniculate forms. We hope that further investigation into the ecological role and function of different awns may ultimately allow awn morphology to be used as a plant functional trait (Cornelissen et al., 2003) for predicting species fitness under different environmental conditions and for explaining biogeographic variation of grass species across Australia.
Data Availability Statement
The raw data supporting the conclusions of this article will be made available by the authors, without undue reservation, to any qualified researcher.
Author Contributions
AC, JM, and RG conceived the ideas and design methodology. AC collected and analyzed the data. AC, JM, and RG led the writing of the manuscript. All authors contributed critically to the drafts and gave final approval for publication.
Conflict of Interest
The authors declare that the research was conducted in the absence of any commercial or financial relationships that could be construed as a potential conflict of interest.
Acknowledgments
The Friends of Grasslands, ACT, provided financial support (Grant reference: FSP2017-03). Sarah Mathews instigated the method for quantifying diaspore movement. Warren Paul provided assistance with data analyses. Andre Messina (Royal Botanic Gardens Victoria), Scott Tunbridge (La Trobe Wildlife Sanctuary), Simon Heyes, Zac Walker and Brendan Lepschi provided seed for experiments. Nick Moore provided technical assistance.
Supplementary Material
The Supplementary Material for this article can be found online at: https://www.frontiersin.org/articles/10.3389/fevo.2020.581967/full#supplementary-material
References
Abraham, Y., and Elbaum, R. (2013). Hygroscopic movements in Geraniaceae: the structural variations that are responsible for coiling and bending. New Phytol. 199, 584–594. doi: 10.1111/nph.12254
Adams, K. M., and Tainton, N. M. (1990). The function of the hygroscopic awn of Themeda triandra. J. Grassland Soc. S. Afr. 7, 271–273. doi: 10.1080/02566702.1990.9648248
Berg, R. Y. (1975). Myrmecochorous plants in Australia and their dispersal by ants. Aust. J. Bot. 23, 475–508. doi: 10.1071/bt9750475
Bustam, B. M. (2010). Systematic studies of Australian stipoid grasses (Austrostipa) based on micro-morphological and molecular characteristics. Biodiversitas 11, 9–14. doi: 10.13057/biodiv/d110103
Cain, M. L., Milligan, B. G., and Strand, A. E. (2000). Long distance seed dispersal in plant populations. Am. J. Bot. 87, 1217–1227. doi: 10.2307/2656714
Cavanagh, A. M., Godfree, R. C., and Morgan, J. W. (2019). An awn typology for Australian native grasses (Poaceae). Aust. J. Bot. 67, 309–334. doi: 10.1071/bt18216
Cornelissen, J. H. C., Lavorel, S., Garnier, E., Diaz, S., Buchmann, N., Gurvich, D. E., et al. (2003). A handbook of protocols for standardised and easy measurement of plant functional traits worldwide. Aust. J. Bot. 51, 335–380. doi: 10.1071/bt02124
Elbaum, R., and Abraham, Y. (2014). Insights into the microstructure of hygroscopic movement in plant seed dispersal. Plant Sci. 223, 124–133. doi: 10.1016/j.plantsci.2014.03.014
Elbaum, R., Zaltzman, L., Burgert, I., and Fratzl, P. (2007). The role of wheat awns in the seed dispersal unit. Science 316, 884–886. doi: 10.1126/science.1140097
Evangelista, D., Hotton, S., and Dumais, J. (2011). The mechanics of explosive dispersal and self-burial in the seeds of the filaree, Erodium cicutarium (Geraniaceae). J. Exp. Biol. 214, 521–529. doi: 10.1242/jeb.050567
Fowler, N. L. (1986). Microsite requirements for germination and establishment of three grass species. Am. Midland Nat. 115, 131–145. doi: 10.2307/2425843
Garnier, L. K. M., and Dajoz, I. (2001). Evolutionary significance of awn length variation in a clonal grass of fire-prone savannas. Ecology 82, 1720–1733. doi: 10.1890/0012-9658(2001)082[1720:esoalv]2.0.co;2
Greene, D. F., and Johnson, E. A. (1989). A model of wind dispersal of winged or plumed seeds. Ecology 70, 339–347. doi: 10.2307/1937538
Harper, J. L., Lovell, P. H., and Moore, K. G. (1970). The shapes and sizes of seeds. Ann. Rev. Ecol. Systemat. 1, 327–356. doi: 10.1146/annurev.es.01.110170.001551
Harper, J. L., Williams, J. T., and Sagar, G. R. (1965). The behaviour of seeds in soil: I. The heterogeneity of soil surfaces and its role in determining the establishment of plants from seed. J. Ecol. 53, 273–286. doi: 10.2307/2257975
Howe, H. F. (1980). Monkey dispersal and waste of a neotropical fruit. Ecology 61, 944–959. doi: 10.2307/1936763
Howe, H. F., and Smallwood, J. (1982). Ecology of seed dispersal. Ann. Rev. Ecol. Syst. 13, 201–228.
Humphreys, A. M., Antonelli, A., Pirie, M. D., and Linder, H. P. (2011). Ecology and evolution of the diaspore “burial syndrome”. Evolution 65, 1163–1180. doi: 10.1111/j.1558-5646.2010.01184.x
Johnson, E. E., and Baruch, Z. (2014). Awn length variation and its effect on dispersal unit burial of Trachypogon spicatus (Poaceae). Rev. Biol. Trop. 62, 321–326. doi: 10.15517/rbt.v62i1.8146
Jung, W., Kim, W., and Kim, H. (2014). Self-burial mechanisms of hygroscopically responsive awns. Integr. Compar. Biol. 54, 1034–1042. doi: 10.1093/icb/icu026
Mallett, K., and Orchard, A. E. (2002). Flora of Australia. Vol. 43: Poaceae 1 Introduction and Atlas. Melbourne: ABRS/CSIRO.
Masrahi, Y. S., and Shaye, N. A. A. (2017). Microstructure of hygroscopic awns in three Poaceae species. Int. Res. J. Plant Sci. 8, 1–8.
McConkey, K. R., Prasad, S., Corlett, R. T., Campos-Arceiz, A., Brodie, F., Rogers, H., et al. (2012). Seed dispersal in changing landscapes. Biol. Conserv. 146, 1–13. doi: 10.1016/j.biocon.2011.09.018
Murbach, L. (1900). Note on the mechanics of the seed-burying awns of Stipa avenacea. Bot. Gazette 30, 113–117. doi: 10.1086/328020
Nathan, R. (2006). Long-distance dispersal of plants. Science 313, 786–788. doi: 10.1126/science.1124975
Pearson, R. G., and Dawson, T. P. (2003). Predicting the impact of climate change on the distribution of species: are bioclimate envelope models useful? Glob. Ecol. Biogeogr. 12, 361–371. doi: 10.1046/j.1466-822x.2003.00042.x
Peart, M. H. (1979). Experiments on the biological significance of the morphology of seed-dispersal units in grasses. J. Ecol. 67, 843–863. doi: 10.2307/2259218
Peart, M. H. (1981). Further experiments on the biological significance of the morphology of seed-dispersal units in grasses. J. Ecol. 69, 425–436. doi: 10.2307/2259677
Peart, M. H. (1984). The effects of morphology, orientation and position of grass diaspores on seedling survival. J. Ecol. 72, 437–453. doi: 10.2307/2260057
Peart, M. H., and Clifford, H. T. (1987). The influence of diaspore morphology and soil-surface properties on the distribution of grasses. J. Ecol. 75, 569–576. doi: 10.2307/2260435
PlantNET (2004). New South Wales Flora Online. Sydney: The NSW Plant Information Network System, Royal Botanic Gardens and Domain Trust. Available online at: http://plantnet.rbgsyd.nsw.gov.au (accessed March 01, 2018).
Sheldon, J. C. (1974). The behaviour of seeds in soil: III. The influence of seed morphology and the behaviour of seedlings on the establishment of plants from surface-lying seeds. J. Ecol. 62, 47–66. doi: 10.2307/2258879
Sindel, B. M., Davidson, S. J., Kilby, M. J., and Groves, R. H. (1993). Germination and establishment of Themeda triandra (Kangaroo Grass) as affected by soil and seed characteristics. Aust. J. Bot. 41, 105–117. doi: 10.1071/bt9930105
Stamp, N. E. (1984). Self-burial behaviour of Erodium cicutarium seeds. J. Ecol. 72, 611–620. doi: 10.2307/2260070
Stamp, N. E. (1989). Efficacy of explosive vs. hyrgroscopic seed dispersal by an annual grassland species. Am. J. Bot. 76, 555–561. doi: 10.1002/j.1537-2197.1989.tb11347.x
Syme, A. E., Murphy, D. J., Holmes, G. D., Gardner, S., Fowler, R., and Cantrill, D. J. (2012). An expanded phylogenetic analysis of Austrostipa (Poaceae: Stipeae) to test infrageneric relationships. Aust. Syst. Bot. 25, 1–10. doi: 10.1071/sb10049
Thomas, C. D., Cameron, A., Green, R. E., Bakkenes, M., Beaumont, L. J., Collingham, Y. C., et al. (2004). Extinction risk from climate change. Nature 427, 145–148.
Tothill, J. C. (1969). Soil temperatures and seed burial in relation to the performance of Heteropogon contortus and Themeda australis in burnt native woodland pastures in eastern Queensland. Aust. J. Bot. 17, 269–275. doi: 10.1071/bt9690269
VicFlora (2016). Flora of Victoria. Melbourne: Royal Botanic Gardens Victoria. Available online at: http://vicflora.rbg.vic.gov.au (accessed March 1, 2018).
Victorian Resources Online (2017). Soil landform units: Floodplain. Melbourne: Agriculture Victoria.
Keywords: awns, awn length, burial, diaspore, dispersal, Poaceae
Citation: Cavanagh AM, Morgan JW and Godfree RC (2020) Awn Morphology Influences Dispersal, Microsite Selection and Burial of Australian Native Grass Diaspores. Front. Ecol. Evol. 8:581967. doi: 10.3389/fevo.2020.581967
Received: 13 July 2020; Accepted: 15 October 2020;
Published: 11 November 2020.
Edited by:
Hang Sun, Kunming Institute of Botany (CAS), ChinaReviewed by:
Bo Song, Kunming Institute of Botany (CAS), ChinaZhimin Liu, Institute of Applied Ecology (CAS), China
Copyright © 2020 Cavanagh, Morgan and Godfree. This is an open-access article distributed under the terms of the Creative Commons Attribution License (CC BY). The use, distribution or reproduction in other forums is permitted, provided the original author(s) and the copyright owner(s) are credited and that the original publication in this journal is cited, in accordance with accepted academic practice. No use, distribution or reproduction is permitted which does not comply with these terms.
*Correspondence: Annette M. Cavanagh, YS5tLmNhdmFuYWdoQG91dGxvb2suY29tLmF1