- Ecosystems and Environment Research Programme, Faculty of Biological and Environmental Sciences, University of Helsinki, Lahti, Finland
Urban residents and their pets utilize urban greenspaces daily. As urban dog ownership rates increase globally, urban greenspaces are under mounting pressure even as the benefits and services they provide become more important. The urine of dogs is high in nitrogen (N) and may represent a significant portion of the annual urban N load. We examined the spatial distribution and impact of N deposition from dog urine on soils in three urban greenspace typologies in Finland: Parks, Tree Alleys, and Remnant Forests. We analyzed soil from around trees, lampposts and lawn areas near walking paths, and compared these to soils from lawn areas 8 m away from pathways. Soil nitrate, ammonium, total N concentrations, and electrical conductivity were significantly higher and soil pH significantly lower near path-side trees and poles relative to the 8 m lawn plots. Also, stable isotope analysis indicates that the primary source of path-side N are distinct from those of the 8 m lawn plots, supporting our hypothesis that dogs are a significant source of N in urban greenspaces, but that this deposition occurs in a restricted zone associated with walking paths. Additionally, we found that Remnant Forests were the least impacted of the three typologies analyzed. We recommend that landscape planners acknowledge this impact, and design parks to reduce or isolate this source of N from the wider environment.
Introduction
As cities grow (Ritchie and Roser, 2019), so do their impacts on and interactions with urban greenspaces. Urbanization has widely recognized impacts on plant, animal, and microbial communities as well as on local and regional nutrient cycles (McKinney, 2008; Churkina, 2016; Decina et al., 2019). Along with increasing urbanization, dog (Canis familiaris) ownership is also on the rise. While common in western countries such as the United States – where as many as 49% of households own at least one dog (The Insurance Information Institute, 2019) – dog ownership is rapidly becoming more common globally (GfK, 2016).
Many urban residents visit greenspaces daily, and these areas provide residents with many valuable ecosystem services such as stormwater retention and treatment, the sequestration of excess nutrients and metals, and opportunities for recreation and nature-connections for city residents (Bolund and Hunhammar, 1999; McCormack et al., 2010; Irvine et al., 2013; Bertram and Rehdanz, 2015; Setälä et al., 2017). As dog-walking is a common activity in urban greenspaces (Brown and Rhodes, 2006; Goliènik and Ward Thompson, 2010; Iojă et al., 2011), dog feces are a recognized problem in these areas (Mallin et al., 2000; Whitlock et al., 2002; McCormack et al., 2010; Cinquepalmi et al., 2013; Rock et al., 2016). However, the impact of dog urine has received little attention. Recent work by Hobbie et al. (2017) showed that nutrient inputs from pet waste (both feces and urine) contributed up to 28% of total N to urban watersheds, second only to residential lawn fertilizer. Paradeis et al. (2013) examined the distribution and concentrations of soil nutrients and salts within enclosed, off-leash dog parks and found that these variables were distributed along gradients and at hotspots within those parks. While N is a vital nutrient for plant growth, its excess application has negative effects on soil functions and the quality of both ground and surface waters. The urine of dogs is rich in urea, which breaks down to available N in the form of ammonium in the soil through the process of hydrolysis. A recent laboratory study by Lee et al. (2019) showed that even short-term applications of dog urine has significant effects on soil biogeochemistry in urban green infrastructures and negatively impacted the ability of these structures to retain and treat stormwater.
Cities are both sources and sinks for N and other nutrients (Lorenz and Kandeler, 2005; Lorenz and Lal, 2009). The need to better understand the N contribution of dogs (hereafter referred to as dog-deposition N) is becoming more pressing as cities and dog ownership rates grow globally. Based on the average dog-ownership rate for Finland (∼22%), we estimate that dogs living in Helsinki, the capital of Finland, may produce as much as 15 kg N ha–1 annually. This is comparable to atmospheric deposition, which is estimated to be 2.1–25 kg total dissolved inorganic nitrogen ha–1 yr–1 (Manninen, 2018). However, dog-deposition N is unlikely to be homogeneous across the urban area, and likely represents an even more significant impact within a particular area of greenspace (see Paradeis et al., 2013).
In this study, we examined soils from different types of urban greenspace, and from different areas within them to better understand the spatial distribution of dog-deposition N. Due to leash requirements in Finland (Järjestyslaki, 2003), we hypothesized that:
(1) Dog-deposition is not evenly distributed, but objects located near pathways, e.g., trees and utility poles, receive higher inputs than lawn areas adjacent to the same path. Furthermore, dog-deposition effects will be higher close to these objects than further away, due to the preference of dogs to countermark the urine of other dogs (Lisberg and Snowdon, 2011).
(2) The magnitude of dog-deposition along pathways will vary by greenspace type, with Remnant Forests being more heavily impacted than Tree Alleys and Parks the least. We hypothesize that Remnant Forests will show the highest impact due to leash requirements and the presence of understory vegetation and closely spaced trees bounding the paths. This would make excursions away from the pathway more difficult, and so dogs are bound to spend more time on the paths relative to the Tree Alleys and Parks. We expect Tree Alley paths to be more impacted than Park paths due to their linear nature, while the open lawns and widely spaced trees of Parks offer dogs and their owners ample opportunities to deviate from the pathways.
Materials and Methods
Study Area
Two cities in Finland were included in this study: (1) Helsinki (60°10′15″N 24°56′15″E), population ca. 650 000; and (2) Lahti (60°59′N 025°39′E), population 120 000 (see Setälä et al., 2016 for additional details regarding these localities). Thirty-four sites were selected (Helsinki n = 18, Lahti n = 16), grouped into three typologies: Parks (n = 11): public spaces with maintained pathways, lawns, trees, etc.; Tree alleys (n = 11): linear features consisting of a tree lined path bounded by, e.g., buildings, roads or fencing; and Remnant forests (n = 12): relatively unmanaged areas with a dense tree canopy and networks of maintained and informal pathways. A site location map can be found in the Supplementary Material (Figure S1), and an interactive version of the field site map can be found at https://bit.ly/3lQcrNq.
Sample Collection
We collected soil samples from 22 August to 13 September 2018. Next to a main pathway at each site, we collected composite samples of eight sub-samples from the top 10 cm of soil using a stainless steel push corer (3 cm ø) at: (1) a deciduous tree (Acer, Tilia, Ulmus, Betula or Quercus sp.), (2) a utility or lamppost, and (3) a lawn area. Lawn areas were selected to be >5 m away from any objects (e.g., benches, trash bins, lampposts), and outside of the tree canopy where possible. At trees and poles, one composite sample was taken from within 30 cm around the item and a second one from within an area of 1 m2 centered at 1 m from the edge of the item opposite the pathway. From the lawn, one composite sample was taken from within a 0.5 m2 area immediately adjacent to the path, and the second from within 1 m2 centered at 1 m from the path edge. In Parks we also collected soil samples from lawn areas >8 m away from pathways (n = 30), and from around trees inaccessible to dogs or >8 m from a main pathway (n = 6). Schematics for the typical layout of each typology are given in the Supplementary Material (Figure S2).
Sample Handling, Processing and Analyses
Samples were stored in a freezer at −20°C at the end of each field day to limit the loss of N due to the continued metabolic action of soil bacteria. Prior to analysis, batches of ∼25 samples were removed from the freezer and thawed overnight at +4°C, sieved (2 mm mesh) and homogenized by hand in a 10 L plastic bucket. The sieve and bucket where thoroughly cleaned between each sample using a brush and warm tap water, then dried using paper towels. Disposable nitrile gloves were worn while sieving and homogenizing the soil and were changed between samples. Approximately 0.5 dL of the sieved samples were set aside for soil dry mass determination after drying overnight at 110°C, and organic matter content (%OM) by the standard loss-on-ignition method (Finnish Standard Association, 1990). Soil electrical conductivity (EC) and pH were measured from a 1:2 volume mix of air-dried soil and ultra-pure water, 4 h after mixing.
For the nitrogen analyses, soil samples were extracted following Decina et al. (2018), using a 2M solution of KCl. Laboratory blank samples of filtered 2M KCl were created at least once per day and for each batch of 2M KCl solution. The filtered sample extracts and blanks were stored in 100 mL plastic bottles and frozen at −20°C to await analysis.
Soil extracts were analyzed colometrically for nitrate (NO3–), ammonium (NH4+), and total nitrogen (TN) at the University of Helsinki’s Environmental Laboratory at the Lahti Campus. Briefly, samples were pipetted into 96-well microplates with one standard curve per plate at the beginning with a series of external quality control solutions. Procedures for making and adding reagents to the microplates, as well as their analysis followed Sims et al. (1995) and Doane and Horwáth (2003) for NH4+ and NO3–, and Miranda et al. (2001) for TN. Limit of quantification (LoQ) values for each analysis were established by analyzing multiple blank samples with added reagents. Manufacturer and batch information for the materials, reagents, standards, and equipment used are given in the Supplementary Material.
Soil freezing has been shown to impact the amount of extractable N measured from soils, which may show a marked increase after thawing (see Edwards and Cresser, 1992). While this is a concern, the soils from our study are typically frozen for several months during winter, thus freezing of the soils prior to analyses is unlikely to introduce a bias to our results.
Stable Isotope Analysis
To determine if nitrogen deposited within urban greenspaces originate from similar sources, soil samples from path-side Poles and Trees (0 m) (n = 6) and lawn areas 8 m from the path (n = 4) were analyzed at the Finnish Museum of Natural History’s Laboratory of Chronology in Helsinki to determine their δ15N values. The raw isotope data were normalized with a multi-point calibration using certified isotopic reference materials (USGS-40, USGS-41, IAEA-N1, and IAEA-N2). The mean measured δ15N values for calibration references were −4.32‰ for USGS-40, +46.66‰ for USGS-41, +0.62‰ for IAEA-N1, and +20.13‰ for IAEA-N2. Replicate analyses of quality control reference materials (soil, corn leaf) analyzed alongside the unknowns indicate a 1(σ internal precision of ≤0.10.
Statistical Analyses
All statistical analyses were performed in R (v 3.6.3) (R Core Team, 2020) for each of the response variables: EC, pH, NO3–, NH4+, and TN. Normality of these variables was determined by inspecting histograms and performing Shapiro–Wilks Normality tests. Appropriate power transformations for non-normal variables were determined using the transformTukey function from the rcompanion package (Mangiafico, 2020).
Generalized linear mixed models (GLMM) (Bates et al., 2015) were used to test the effects of dog urine on the soil parameters listed above. First, we examined the spatial extent of dog-deposition at 0, 1, and 8 m from the path-side treatments using data from the Park typology only. These models include (i) treatment (a factor with three levels; Lawn, Tree, Pole), (ii) distance (a factor with three levels; 0, 1, and 8 m), their two-way interaction, and percentage organic matter (OM) and soil moisture. We included site, nested within city as a random term in the models. Model selection was performed by removing OM and/or soil moisture when these variables were not statistically significant (p-values > 0.1).
Second, to test if dog-deposition magnitude varies by type of greenspace we again used GLMMs and tested the response variables against (i) typology (a factor with three levels; Tree Alley, Remnant Forests, and Parks, (ii) treatment (a factor with three levels; Lawn, Tree, Pole), (iii) distance (a factor with two levels; 0 and 1 m), the treatment × distance interaction, and percentage OM and soil moisture. The random term was structured as above, and model selection was performed in the same manner.
Results
Soil chemistry varied greatly depending on proximity to path-side trees and poles (Figures 1, 2 and Tables 1, 2). Soil EC, NO3–, NH4+, and TN levels were several times higher, and pH considerably lower within the 30 cm area around path-side trees and poles compared to soils 1 m away (in all three typologies) and 8 m away (in Parks). However, path-side lawn areas were largely indistinguishable from the lawn areas 1 and 8 m away from the path. We also found slight differences between greenspace typologies (Figure 2 and Table 2).
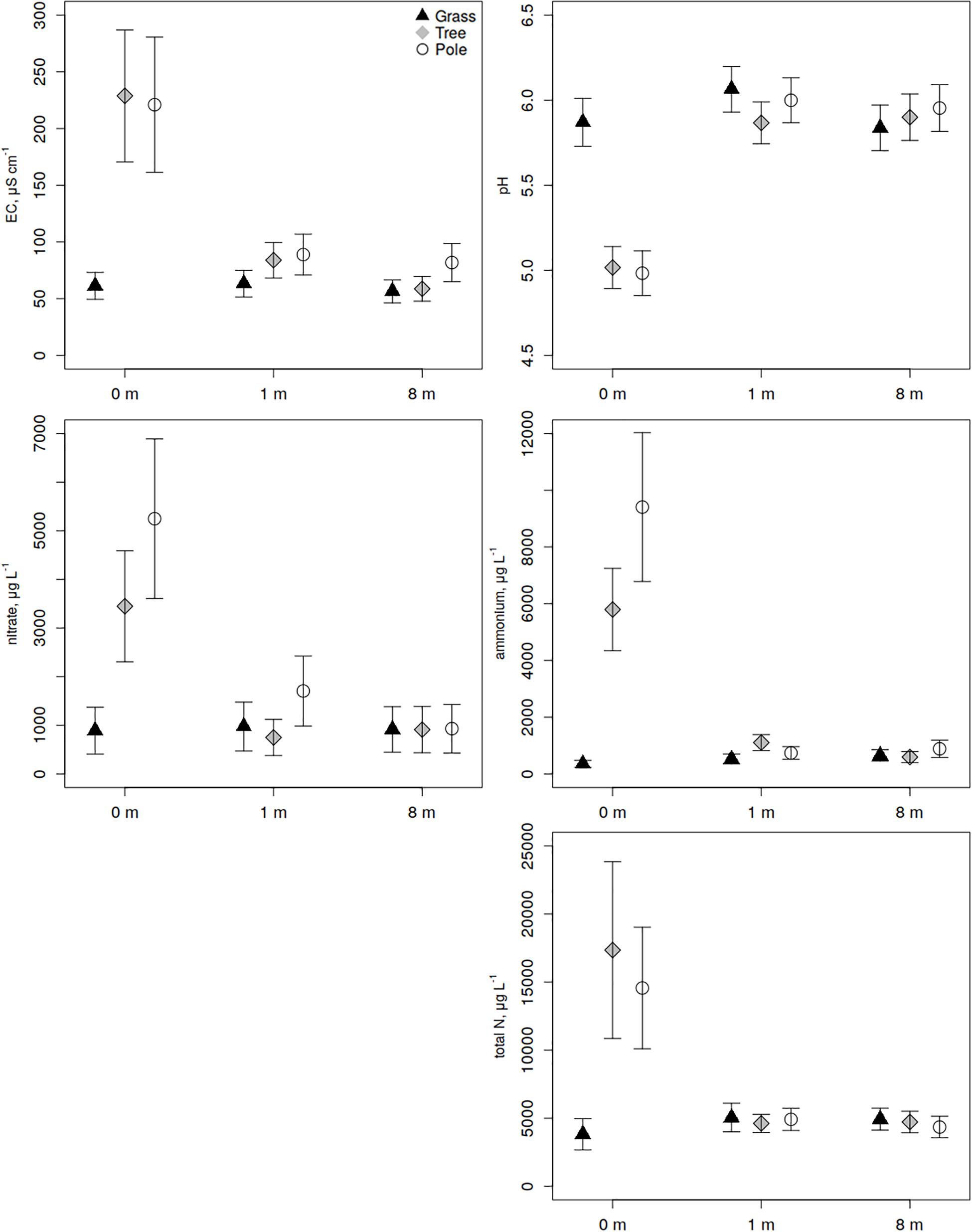
Figure 1. Back-transformed model predicted mean ± SE values for Electrical Conductivity (EC), pH, nitrate, ammonium, and total nitrogen at 0, 1, and 8 m away from path-side grass plots, trees, and poles in Parks only.
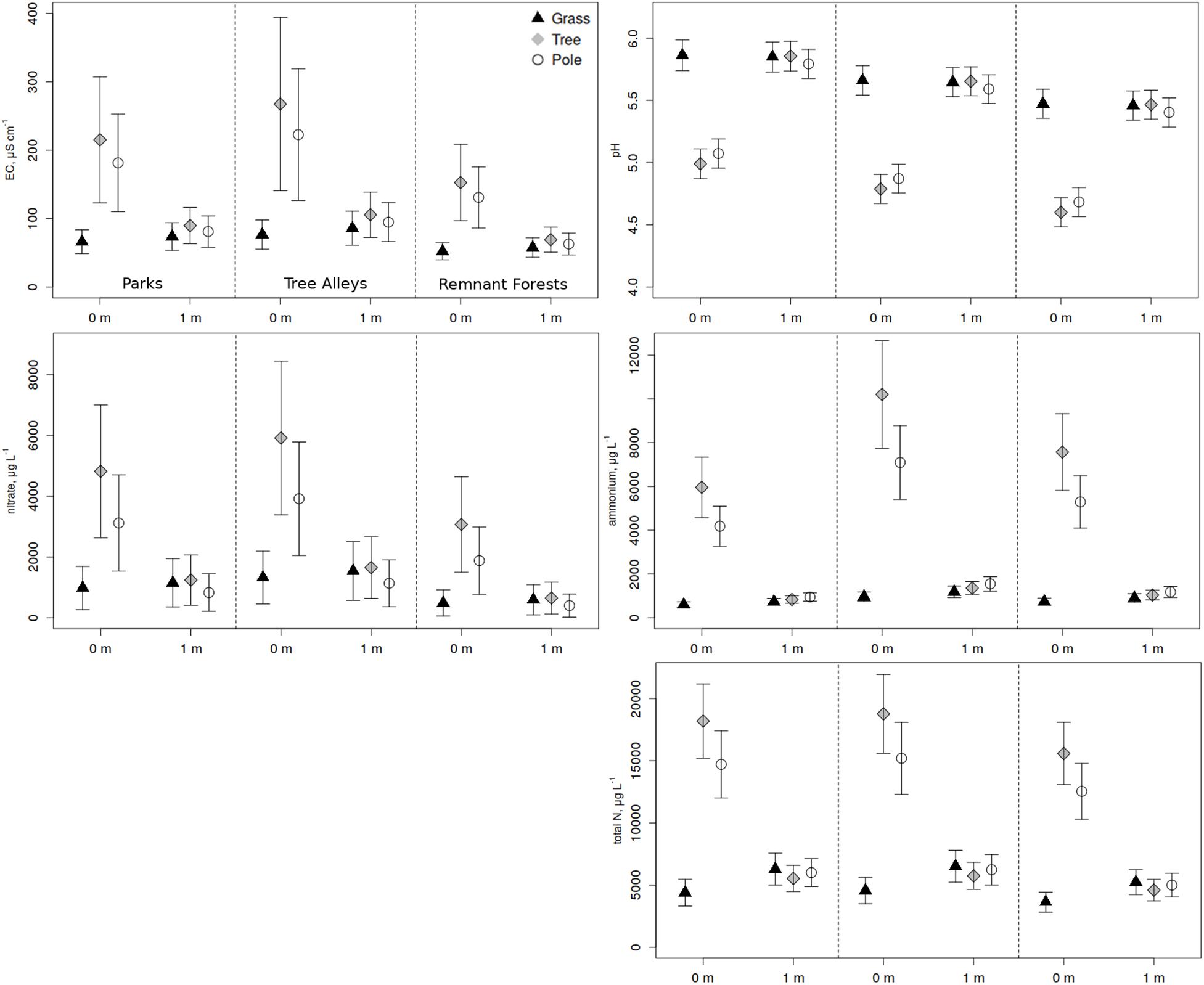
Figure 2. Comparison of back-transformed model predicted mean ± SE values for Electrical Conductivity (EC), pH, nitrate, ammonium, and total nitrogen at 0 and 1 m away from path-side grass plots, trees, and poles in three greenspace typologies: Parks, Tree Alleys and Remnant Forests.
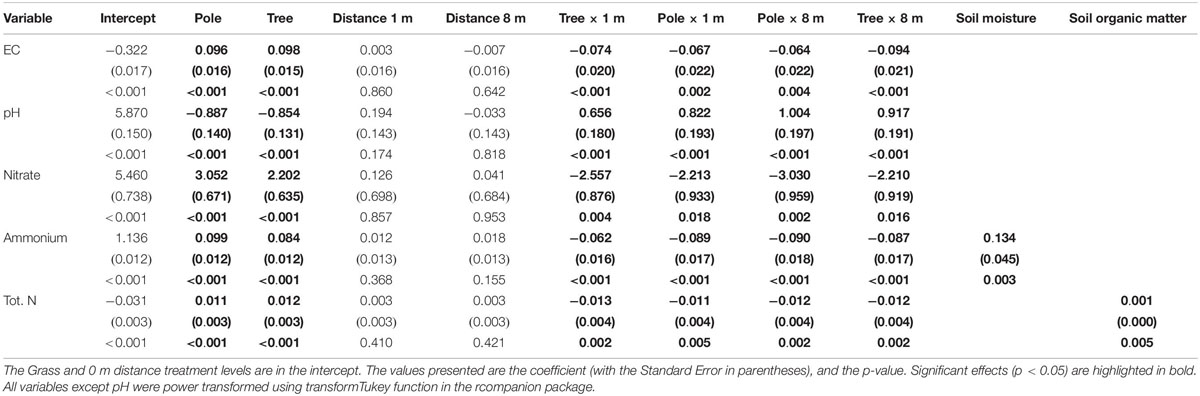
Table 1. GLMM results, testing the effects of treatment (a factor with three levels; grass, pole, tree), distance (a factor with three levels; 0, 1, and 8 m), and their two-way interaction on five variables (pH, EC, nitrate, ammonium, and tot. nitrogen).
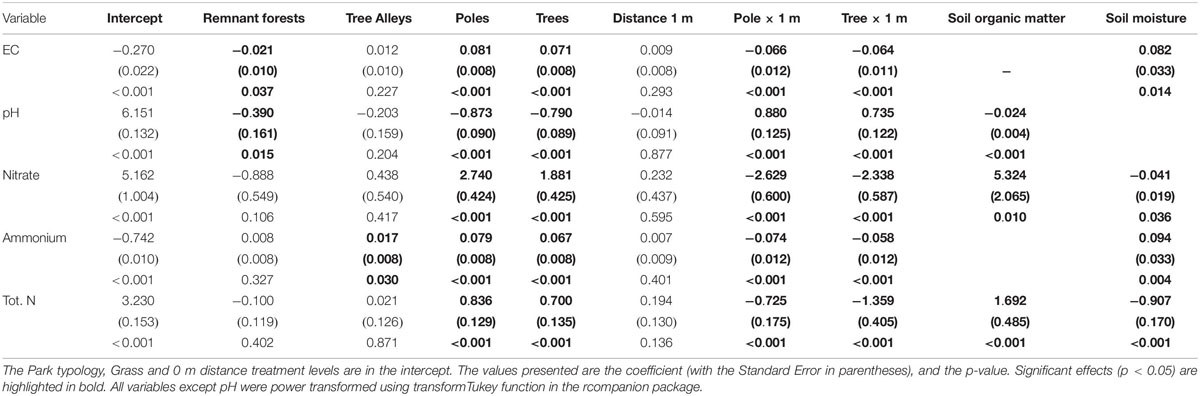
Table 2. GLMM results, testing the effects of typology (a factor with three levels: Parks, Tree Alleys, and Remnant Forests), treatment (a factor with three levels: grass, pole, tree), distance (a factor with two levels: 0 and 1 m), and their two-way interaction on five variables (pH, EC, nitrate, ammonium, and tot. nitrogen).
Stable isotope analysis of a subset of samples showed the soils around path-side trees and poles (n = 6) to have a mean (δ15N value of 8.3, while samples taken from 8 m away (n = 4) had a mean [δ15N value of 3.5 (Welch two sample t test, t = 3.556, p = 0.008) (Supplementary Figure S3)].
Discussion
We have shown that dog-deposition is localized and impacts soil chemistry in urban greenspaces significantly. Supporting the first hypothesis, soil chemical characteristics and δ15N values around path-side trees and poles were significantly different from those located further from the paths and from lawn area soils next to the same pathway. Differences we observed in the δ15N values of soil samples taken from path-side trees and poles at 0 m and 8 m away suggest that the primary N inputs to these areas are derived from different sources. However, contrary to expectations, we found no difference in the measured variables between the path-side (0 m), 1 and 8 m lawn samples, indicating that path-side trees and poles act as focal points for dog-deposition, while lawn areas do not. This is likely a function of gender-specific differences in dogs’ urinating and scent-marking behaviors (countermarking), with male dogs preferring to urinate directly on trees and poles (overmarking) while females generally do not, instead preferring to urinate near, but not at the same locations as other dogs (adjacent-marking) (Pal, 2003; Lisberg and Snowdon, 2011).
Our data do suggest that dog-deposition impacts vary with greenspace typology, but not in the way we expected. Remnant Forests, rather than being the most impacted, were found to be the least affected, while Tree Alleys were found to be the most heavily impacted, followed by Parks. The lower values observed in Remnant Forests could be due to a lower number of dog walkers in these areas, while Tree Alleys may experience higher volumes of traffic and may also be the first area of greenspace that a dog encounters when being taken for a walk. The open design of Parks may allow dogs and their owners more opportunities to deviate from established pathways, thereby spreading their impacts more widely. Another factor that is likely to affect the magnitude of dog-deposition in these areas is residential population density of the surrounding areas. While we did not directly examine this potential correlation, we did select our study sites to be located within the urban core or ≤500 m of multifamily/high-density residential areas.
Our research indicates that dog-deposition is strongly associated with objects near pathways in urban greenspaces and that it is localized. Significant rapid and long-lasting impacts on soil biogeochemistry have been shown to result from even a single application of urine (see, e.g., Haynes and Williams, 1992; Orwin et al., 2009; Lee et al., 2019). The effects on soil chemical properties observed in our study suggest that the impact of dog urine in urban greenspaces is even greater than the impacts observed in these studies. Furthermore, in addition to being highly localized, the input of N from dogs to urban greenspaces is chronic, and it is likely that multiple dogs will urinate in the same location each day. This sustained input of concentrated N in areas frequented by humans for recreation and leisure represents a uniquely urban phenomenon, one whose closest analog may be pastureland urine patches or waste lagoons in the confined animal feeding operations (CAFOs) of industrial agriculture. In fact, the average concentrations of ammonium we measured from soils located around path-side poles in Parks was 103.9 ± 18.4 mg kg–1 (mean ± SE), which is more than four times the cleanup standard proposed by Volland et al. (2003) for ammonium (25 mg kg–1) in soil underneath CAFOs and is comparable to values found in soils underneath CAFO waste lagoons (DeSutter et al., 2005). By contrast, the ammonium concentrations we measured in Park lawn soils 8 m away from pathways was only 6.7 ± 0.9 mg kg–1 (mean ± SE), which is comparable to the values from urban soils analyzed by Paradeis et al. (2013).
The localized nature of dog-deposition provides urban planners with the opportunity to alleviate this impact by modifying greenspace designs and incorporating structures designed to attract and isolate dog urine from the broader environment. Dog owners could be encouraged, through educational outreach and on-site signage, to direct their dogs toward structures or areas where drains can capture infiltrating urine and stormwater. Such a system would protect ground and surface waters by diverting this nutrient rich flow to sanitary sewers or other treatment systems prior to release. Furthermore, greenspaces can be designed with the likely locations of hotspots already in mind, and so controls can be included in the site plan, rather than retrofitted.
Compared to natural areas, cities are enriched with N, and while environmental quality regulations have led to a decrease in atmospheric N deposition in recent decades (Eshleman et al., 2013), dog ownership rates are increasing. Even now, some countries are seeing a spike in pet adoptions and fostering in response to the COVID-19 crisis, with many pet shelters in the United States being completely emptied during the summer of 2020 (Oppenheim, 2020; Vincent et al., 2020). This spike notwithstanding, if current growth trends in urbanization and dog ownership continue, the localized impacts that we have found will likely increase in severity and possibly in spatial extent, and dog-deposition could become the single largest source of N in urban watersheds.
As cities sprawl and/or density, urban greenspaces are coming under mounting pressure, even while the services they provide are becoming more important to greater numbers of people and their pets (Haaland and van den Bosch, 2015). Dogs have played an important part in human societies for thousands of years and will undoubtedly continue to be valuable partners. However, as our populations continue to grow, so does the need to better understand the role of dogs in urban N deposition and their broader impacts on sustainable urban development and the environment.
Data Availability Statement
The original contributions presented in the study are included in the article/Supplementary Material, further inquiries can be directed to the corresponding author.
Author Contributions
JA conceived, designed, and wrote the manuscript and was responsible for performing the experiment and data analyses. HS provided guidance and support in all aspects of the research and participated in the writing process. DK provided guidance and support in all aspects of the research and writing process, especially regarding experimental design and data analyses. All authors contributed to the article and approved the submitted version.
Funding
This project was made possible through the support of the Onni and Hilja Tuovinen Foundation (grant decision 5/2018), the City of Helsinki (grant no. HEL 2019-001617), the University of Helsinki Funds (grant nos. 1064091, 1065269, and 1116153), MUTKU ry. (grant decision 14062019), and the Päijät-Häme Regional Fund of the Finnish Cultural Foundation (grant no. 70201490).
Conflict of Interest
The authors declare that the research was conducted in the absence of any commercial or financial relationships that could be construed as a potential conflict of interest.
Acknowledgments
We thank Marianne Lehtonen and Jukka Pellinen of the University of Helsinki’s Environmental Laboratory for their material support and help in analyzing our samples, and Changyi Lu for his help in the field. We are also grateful to Juha Raisio from the City of Helsinki Environmental Office, and Markku Saari from the Lahti City Parks Department for granting us permission to work in the cities’ parks.
Supplementary Material
The Supplementary Material for this article can be found online at: https://www.frontiersin.org/articles/10.3389/fevo.2020.615979/full#supplementary-material
References
Bates, D., Mächler, M., Bolker, B., and Walker, S. (2015). Fitting linear mixed-effects models using lme4. J. Stat. Soft 67, 1–48. doi: 10.18637/jss.v067.i01
Bertram, C., and Rehdanz, K. (2015). Preferences for cultural urban ecosystem services: comparing attitudes, perception, and use. Ecosyst. Serv. 12, 187–199. doi: 10.1016/j.ecoser.2014.12.011
Bolund, P., and Hunhammar, S. (1999). Ecosystem services in urban areas. Ecol. Econ. 29, 293–301. doi: 10.1016/S0921-8009(99)00013-0
Brown, S. G., and Rhodes, R. E. (2006). Relationships among dog ownership and leisure-time walking in western canadian adults. Am. J. Prevent. Med. 30, 131–136. doi: 10.1016/j.amepre.2005.10.007
Churkina, G. (2016). The role of urbanization in the global carbon cycle. Front. Ecol. Evol 3:144. doi: 10.3389/fevo.2015.00144
Cinquepalmi, V., Monno, R., Fumarola, L., Ventrella, G., Calia, C., Greco, M. F., et al. (2013). Environmental contamination by dog’s faeces: a public health problem? Int. J. Environ. Res. Public Health 10, 72–84. doi: 10.3390/ijerph10010072
R Core Team (2020). R: A Language and Environment for Statistical Computing. Vienna: R Foundation for Statistical Computing.
Decina, S. M., Hutyra, L. R., and Templer, P. H. (2019). Hotspots of nitrogen deposition in the world’s urban areas: a global data synthesis. Front. Ecol. Environ. 18:2143. doi: 10.1002/fee.2143
Decina, S. M., Templer, P. H., and Hutyra, L. R. (2018). Atmospheric inputs of nitrogen. carbon, and phosphorus across an urban area: unaccounted fluxes and canopy influences. Earths Fut. 6, 134–148. doi: 10.1002/2017EF000653
DeSutter, T. M., Pierzynski, G. M., and Ham, J. M. (2005). Movement of lagoon-liquor constituents below four animal-waste lagoons. J. Environ. Qual. 34, 1234–1242. doi: 10.2134/jeq2004.0296
Doane, T. A., and Horwáth, W. R. (2003). Spectrophotometric determination of nitrate with a single reagent. Anal. Lett. 36, 2713–2722. doi: 10.1081/AL-120024647
Edwards, A. C., and Cresser, M. S. (1992). Freezing and Its Effect on Chemical and Biological Properties of Soil. In Advances in Soil Science. New York, NY: Springer, 59–79.
Eshleman, K. N., Sabo, R. D., and Kline, K. M. (2013). Surface water quality is improving due to declining atmospheric N deposition. Environ. Sci. Technol. 47, 12193–12200. doi: 10.1021/es4028748
Finnish Standard Association (1990). SFS 3008, Determination of Total Residue and Total Fixed Residue in Water, Sludge and Sediment. Finland: Finnish Standard Association.
Goličnik, B., and Ward Thompson, C. (2010). Emerging relationships between design and use of urban park spaces. Landsc. Urban Plan. 94, 38–53. doi: 10.1016/j.landurbplan.2009.07.016
Haaland, C., and van den Bosch, C. K. (2015). Challenges and strategies for urban green-space planning in cities undergoing densification: a review. Urban For. Urban Green. 14, 760–771. doi: 10.1016/j.ufug.2015.07.009
Haynes, R. J., and Williams, P. H. (1992). Changes in soil solution composition and pH in urine-affected areas of pasture. J. Soil Sci. 43, 323–334. doi: 10.1111/j.1365-2389.1992.tb00140.x
Hobbie, S. E., Finlay, J. C., Janke, B. D., Nidzgorski, D. A., Millet, D. B., and Baker, L. A. (2017). Contrasting nitrogen and phosphorus budgets in urban watersheds and implications for managing urban water pollution. Proc. Natl. Acad. Sci. U.S.A. 114, 4177–4182. doi: 10.1073/pnas.1618536114
Iojă, C. I., Rozylowicz, L., Pãtroescu, M., Niţã, M. R., and Vânau, G. O. (2011). Dog walkers’ vs. other park visitors’ perceptions: The importance of planning sustainable urban parks in Bucharest. Romania. Landscape and Urban Planning 103, 74–82. doi: 10.1016/j.landurbplan.2011.06.002
Irvine, K., Warber, S., Devine-Wright, P., and Gaston, K. (2013). Understanding urban green space as a health resource: a qualitative comparison of visit motivation and derived effects among park users in sheffield. UK. IJERPH 10, 417–442. doi: 10.3390/ijerph10010417
Järjestyslaki (2003). Järjestyslaki. Available online at: https://finlex.fi/fi/laki/ajantasa/2003/20030612 (accessed August 25, 2020).
Lee, J. M., Tan, J., Gill, A. S., and McGuire, K. L. (2019). Evaluating the effects of canine urine on urban soil microbial communities. Urban Ecosyst. 22, 721–732. doi: 10.1007/s11252-019-00842-0
Lisberg, A. E., and Snowdon, C. T. (2011). Effects of sex, social status and gonadectomy on countermarking by domestic dogs, Canis familiaris. Anim. Behav. 81, 757–764. doi: 10.1016/j.anbehav.2011.01.006
Lorenz, K., and Kandeler, E. (2005). Biochemical characterization of urban soil profiles from Stuttgart, Germany. Soil Biol. Biochem. 37, 1373–1385. doi: 10.1016/j.soilbio.2004.12.009
Lorenz, K., and Lal, R. (2009). Biogeochemical C and N cycles in urban soils. Environ. Int. 35, 1–8. doi: 10.1016/j.envint.2008.05.006
Mallin, M. A., Williams, K. E., Esham, E. C., and Lowe, R. P. (2000). Effect of human development on bacteriological water quality in coastal watersheds. Ecol. Appl. 10, 1047–1056. doi: 10.1890/1051-0761(2000)010[1047:eohdob]2.0.co;2
Mangiafico, S. (2020). rcompanion: Functions to Support Extension Education Program Evaluation. Available online at: https://CRAN.R-project.org/package=rcompanion (accessed April 29, 2020).
Manninen, S. (2018). Deriving nitrogen critical levels and loads based on the responses of acidophytic lichen communities on boreal urban Pinus sylvestris trunks. Sci. Total Environ. 61, 751–762. doi: 10.1016/j.scitotenv.2017.09.150
McCormack, G. R., Rock, M., Toohey, A. M., and Hignell, D. (2010). Characteristics of urban parks associated with park use and physical activity: a review of qualitative research. Health Place 16, 712–726. doi: 10.1016/j.healthplace.2010.03.003
McKinney, M. L. (2008). Effects of urbanization on species richness: a review of plants and animals. Urban Ecosyst. 11, 161–176. doi: 10.1007/s11252-007-0045-4
Miranda, K. M., Espey, M. G., and Wink, D. A. (2001). A Rapid. Simple Spectrophotometric Method for Simultaneous Detection of Nitrate and Nitrite. Nitric Oxide 5, 62–71. doi: 10.1006/niox.2000.0319
Oppenheim, B. (2020). Pet Ownership Increases During Pandemic. The DCA Page. Available online at: https://thedcapage.blog/2020/08/10/pet-ownership-increases-during-pandemic/ (accessed October 5, 2020).
Orwin, K. H., Bertram, J. E., Clough, T. J., Condron, L. M., Sherlock, R. R., and O’Callaghanc, M. (2009). Short-term consequences of spatial heterogeneity in soil nitrogen concentrations caused by urine patches of different sizes. Appl. Soil Ecol. 42, 271–278. doi: 10.1016/j.apsoil.2009.05.002
Pal, S. K. (2003). Urine marking by free-ranging dogs (Canis familiaris) in relation to sex, season, place and posture. Appl. Anim. Behav. Sci. 80, 45–59. doi: 10.1016/S0168-1591(02)00178-8
Paradeis, B., Lovas, S., Aipperspach, A., Kazmierczak, A., Boche, M., He, Y., et al. (2013). Dog-park soils: concentration and distribution of urine-borne constituents. Urban Ecosyst. 16, 351–365. doi: 10.1007/s11252-012-0264-1
Ritchie, H., and Roser, M. (2019). Urbanization. Available online at: https://ourworldindata.org/urbanization (accessed November 19, 2019).
Rock, M. J., Graham, T. M., Massolo, A., and McCormack, G. R. (2016). Dog-walking, dog-fouling and leashing policies in urban parks: Insights from a natural experiment designed as a longitudinal multiple-case study. Landsc. Urban Plan. 153, 40–50. doi: 10.1016/j.landurbplan.2016.04.018
Setälä, H., Francini, G., Allen, J. A., Jumpponen, A., Hui, N., and Kotze, D. J. (2017). Urban parks provide ecosystem services by retaining metals and nutrients in soils. Environ. Pollut. 231, 451–461. doi: 10.1016/j.envpol.2017.08.010
Setälä, H. M., Francini, G., Allen, J. A., Hui, N., Jumpponen, A., and Kotze, D. J. (2016). Vegetation type and age drive changes in soil properties, nitrogen, and carbon sequestration in urban parks under cold climate. Front. Ecol. Evol. 4:93. doi: 10.3389/fevo.2016.00093
Sims, G. K., Ellsworth, T. R., and Mulvaney, R. L. (1995). Microscale determination of inorganic nitrogen in water and soil extracts. Commun. Soil Sci. Plant Anal. 26, 303–316. doi: 10.1080/00103629509369298
The Insurance Information Institute (2019). Facts + Statistics: Pet statistics | III. Available online at: https://www.iii.org/fact-statistic/facts-statistics-pet-statistics (accessed November 21, 2019).
Vincent, A., Mamzer, H., Ng, Z., and Farkas, K. J. (2020). People and their pets in the times of the covid-19 pandemic. Soc. Res. 4, 111–128. doi: 10.14746/sr.2020.4.3.06
Volland, C., Zupancic, J., and Chappelle, J. (2003). Cost of remediation of nitrogen-contaminated soils under CAFO impoundments. J. Hazardous Substance Res. 4, 1–18. doi: 10.4148/1090-7025.1028
Keywords: urban, parks, greenspace, dogs (Canis familiaris), nitrogen, soil, urine, city
Citation: Allen JA, Setälä H and Kotze DJ (2020) Dog Urine Has Acute Impacts on Soil Chemistry in Urban Greenspaces. Front. Ecol. Evol. 8:615979. doi: 10.3389/fevo.2020.615979
Received: 10 October 2020; Accepted: 30 November 2020;
Published: 22 December 2020.
Edited by:
Jason Antony Byrne, University of Tasmania, AustraliaReviewed by:
Tom DeSutter, North Dakota State University, United StatesKrista L. McGuire, University of Oregon, United States
Copyright © 2020 Allen, Setälä and Kotze. This is an open-access article distributed under the terms of the Creative Commons Attribution License (CC BY). The use, distribution or reproduction in other forums is permitted, provided the original author(s) and the copyright owner(s) are credited and that the original publication in this journal is cited, in accordance with accepted academic practice. No use, distribution or reproduction is permitted which does not comply with these terms.
*Correspondence: John A. Allen, am9obi5hbGxlbkBoZWxzaW5raS5maQ==