- 1Centre for Biodiversity and Environmental Research, Department of Genetics, Evolution and Environment, University College London, London, United Kingdom
- 2Termite Research Group, Department of Life Sciences, The Natural History Museum, London, United Kingdom
Phenotypic plasticity provides organisms with the potential to adapt to their environment and can drive evolutionary innovations. Developmental plasticity is environmentally induced variation in phenotypes during development that arise from a shared genomic background. Social insects are useful models for studying the mechanisms of developmental plasticity, due to the phenotypic diversity they display in the form of castes. However, the literature has been biased toward the study of developmental plasticity in the holometabolous social insects (i.e., bees, wasps, and ants); the hemimetabolous social insects (i.e., the termites) have received less attention. Here, we review the phenotypic complexity and diversity of termites as models for studying developmental plasticity. We argue that the current terminology used to define plastic phenotypes in social insects does not capture the diversity and complexity of these hemimetabolous social insects. We suggest that terminology used to describe levels of cellular potency could be helpful in describing the many levels of phenotypic plasticity in termites. Accordingly, we propose a conceptual framework for categorizing the changes in potential of individuals to express alternative phenotypes through the developmental life stages of termites. We compile from the literature an exemplar dataset on the phenotypic potencies expressed within and between species across the phylogeny of the termites and use this to illustrate how the potencies of different life stages of different species can be described using this framework. We highlight how this conceptual framework can help exploit the rich phenotypic diversity of termites to address fundamental questions about the evolution and mechanisms of developmental plasticity. This conceptual contribution is likely to have wider relevance to the study of other hemimetabolous insects, such as aphids and gall-forming thrips, and may even prove useful for some holometabolous social insects which have high caste polyphenism.
Introduction
Phenotypic plasticity describes how different phenotypes or forms can arise from a single genome, and can manifest as diversity in behavior, physiology or morphology. The ability to express such phenotypic diversity can be considered an individual's “potency.” Phenotypic plasticity can produce novelty, facilitating adaptation and evolution, with effects on biodiversity, from populations to ecological communities (Whitman and Agrawal, 2009). Understanding the proximate (mechanisms by which plasticity arises) and ultimate (effects on fitness and function) causes underpinning phenotypic plasticity is therefore of paramount importance in ecology and evolution (Whitman and Agrawal, 2009). Insects are popular study subjects for phenotypic plasticity, where it has contributed to their ecological success due to their ability to change morphology during development, in response to environmental cues. Such developmental plasticity produces morphologically distinct phenotypes within a species, often called polyphenisms (Moczek, 2010; Lo et al., 2018). Social insects have proven a useful study group where their societies exhibit different phenotypes in the form of reproductive “queen” and non-reproductive “worker” castes; caste polyphenisms are well studied in some groups (e.g., Hymenoptera), they are less well understood in others, specifically the termites. This is surprising as termites exhibit enormous diversity in phenotypic and levels of potency. In this paper we discuss how the many “phenomes” exhibited by termite castes provide us with both complexities and opportunities to further our understanding of the nature of plasticity and developmental potential and propose a conceptual framework that could facilitate future research in this area.
Developmental plasticity can be described in quantitative terms by the number of phenotypes that can be produced from a single genome—its “potency.” Examples of this include the distinct queen and worker phenotypes in ant and social bee and wasp colonies; these hymenopteran species undergo complete metamorphosis, where there is a distinct transformation from a single pre-adult form (the larva) to an adult form (See Box 1): only the pre-adult form can express developmental plasticity and alter its ultimate caste fate, meaning that no external morphological change (i.e., change occurring through a molt) is possible once they are adults. As a result, potency of developmental plasticity in social Hymenoptera is largely binary: individuals are either “totipotent,” i.e., they can become any of the caste options available to that species, or they are “committed,” i.e., their caste fate is sealed, usually irreversibly, and they have lost plasticity (Box 1) (Crespi and Yanega, 1995; Boomsma and Gawne, 2018). There is of course variation within this dichotomy; e.g., simple social species, like Polistes paper wasps, lack any form of commitment and plasticity is retained into adulthood (Jandt et al., 2014); caste is determined genetically in all species for males such that even an egg is committed to a specific caste, and this is also the case in females for a rare number of species (Cahan et al., 2002; Julian et al., 2002; Volny and Gordon, 2002; Cahan and Keller, 2003; Schwander et al., 2010). But largely, in the social Hymenoptera, an individual has the potential to become any caste until some point in development, after which it is developmentally committed to a specific caste. It is the relative simplicity in caste potency that has made Hymenoptera castes popular for studies of phenotypic plasticity, and as a consequence the concept of insect caste polyphenisms is largely considered a dichotomy in terms of potency.
Box 1. Glossary.
Adult: The final stage after the last ecdysis. Therefore, any committed termite workers are adults, and anything not committed is as a juvenile.
Alate: Winged reproductives that undergo nuptial flights before shedding their wings and forming new colonies.
Apterous: wingless
Apterous neotenics: (Ergatoids) Neotenic reproductive that develops from a worker in higher termites or from false workers in lower termites (See Neotenic reproductive).
Brachypterous neotenics: (Nymphoids) Neotenic reproductive that develop from nymphal instars in both lower and higher termites (see Neotenic reproductive). They have small, non-functional, wings.
Brachypterous: reduced wings
False workers: individuals that have not diverged from the winged line and can therefore still become Nymphs, but that help the colony to a greater or lesser extent
Foraging termites: (Formally referred to as separate-piece or multiple piece nesters) Species which live in well-defined nest where workers, at some point in the colony-cycle, will leave the nest to forage. This means the colony longevity is not limited to the availability of food. All foraging termites have true workers which can be thought to have reduced potential. These are found in the Mastotermitidae, Hodotermitidae, most Rhinotermititdae, and all Termitidae.
Hemimetabolous: (Sensu lato) species which undergo incomplete metamorphosis due to no pupal stage. These species progress through a series of morphologically distinct stages separated by a molt, gradually acquiring adult characteristics. Under this definition, any species with hemimetabolous (Sensu stricto) and paurometabolous development are included.
Holometabolous: species which undergo complete metamorphosis. This involves four stages: egg, larva, pupa, and adult.
Larvae: instars which do not have externally visible wing buds and are dependent on others for survival and do not take part in work within the colony.
Molt, Progressive: The gradual development from egg via several instars into an adult. This type of molt involves an increase in body size and morphological development. This is the default developmental program in all hemimetabolous and holometabolous insects.
Molt, Regressive: This type of molt involves a decrease in body size and/or regression of morphological development, generally with a reduction in wing bud size in nymphal instars.
Molt, Stationary: an intermittent molt which involves no increase in body size and morphological development. This has been exhibited in several insect species and is associated with periods of food shortage, when a larva or nymph is not capable of passing a critical mass threshold in an instar. In some termites it might be associated with replacing worn mandibles.
Neotenic reproductive: reproductives with either no wings or small non-functional wings, that develop within a colony from any instar after L3. Gonads grow and they develop some imaginal characters while maintaining an otherwise larval appearance; some characters, like wing pads, may regress but usually lack compound eyes and have a less sclerotized cuticle.
Nymph: Instars with externally visible wing buds.
Presoldier: A single transitional instar during development from previous phenotype to soldier.
Pseudergates: Nymphs which have regressively molted back into a worker-like form without wing buds.
Soldier: Sterile altruistic caste which are generally morphologically and behaviorally specialized for defense.
Termite, Higher: Made up of only termite species within the family Termitidae, which only have non-flagellate gut symbionts.
Termite, Lower: All termites other than the Termitidae. They have both flagellates and non-flagellate gut symbionts.
True workers: Individuals that have diverged and are part of a separate wingless line.
Winged line: (nymphal/pterous) developmental pathway where winged phenotypes develop.
Wingless line: (apterous) developmental pathway where wingless phenotypes develop.
Wood-dwelling termites: (Formally one-piece nesters) species where a colony will live in a single piece of wood which serves as both food and nest source. Only the winged sexuals leave the nest and when their only food source is exploited the colony will die. Species within this life type are thought to have highly flexible development and false workers. These are found within the Termposidae, Kalotermitidae, and some species within the Rhinotermitidae.
Worker: individuals which are now independent and able to help the colony but still do not have externally visible wing buds.
A number of social insect taxa which cannot conform to this dichotomy are gall-forming thrips (Crespi, 1992), aphids (Stern and Foster, 1996), and termites which are the focus of our study. The hemimetabolous life history of species like the termites means that they undergo incomplete metamorphosis, changing their form gradually over multiple pre-adult molts before finally producing a developmentally inflexible adult (Box 1). Each molt provides an opportunity to change their level of developmental plasticity. In other words, unlike most of the social Hymenoptera, there is not a single switch point in termite development during with an individual's potency tips from completely plastic (totipotent) to not plastic (committed). As a result, they exhibit more complex levels of potency both within and between species, across their phylogeny (Toubiana and Khila, 2016; Lo et al., 2018). This difficulty in applying the mainstream binary terminology makes it challenging to study the mechanisms and evolutionary processes underpinning phenotypic plasticity in this group (Corona et al., 2016; Lo et al., 2018). However, if we are able to create a framework which allows us to utilize this complexity, we will be able to gain a deeper understanding of these processes. This is important as our current understanding is largely based on mechanisms and evolution derived from holometabolous insects; it is little known whether the same processes underpin the more complex plasticity found in other taxa. The same can be said of the theorized conditions surrounding major evolutionary transitions to superorganismality, namely the need for every colony member to be committed to a single morphologically distinct adult caste during early development, which is largely Hymenoptera-focused and therefore does not fully take into account hemimetabolous modes of development (Szathmáry and Maynard-Smith, 1995; Boomsma and Gawne, 2018). It is only when the complexity arising from hemimetabolous systems is incorporated into such theories that we can truly understand the processes underpinning the evolution of higher levels of individuality as superorganisms. A key stumbling block in achieving this, however, is the ability to translate descriptors of phenotypic complexity across social taxa.
Here, we provide a new conceptual framework for classifying phenotypic diversity in termites. We explain the challenges that termites bring to the current terminology used to describe developmental plasticity. We apply terminology from cell biology literature to better categorize the complexities of termite phenotypes and discuss how these many stages of potency makes them important for understanding developmental plasticity. We apply these categories to examples from the termite literature to increase the value of these hemimetabolous (eusocial) insects for testing fundamental questions on the evolution and mechanisms of developmental plasticity. In doing so, we also generate a comprehensive, albeit not exhaustive, dataset on the levels of potency found in 73 species of termites from the literature, which allows us to highlight promising groups for future study, using this new framework (See Supplementary Materials 1, 2). Finally, we explore the future research that can be generated from our study which promises to advance our understanding on the proximate and ultimate mechanisms of caste determination in termites, and on the fundamental nature of phenotypic plasticity found across all taxa. We suggest that our framework may also provide a useful conceptual framework for understanding diverse polyphenisms in other taxa such as aphids and gall-forming thrips (Crespi, 1992; Stern and Foster, 1996). Indeed, any rare examples within the holometabolous social insects which don't conform to the dichotomous categorization will also benefit from this new more fine-grained framework.
The Challenges of Classifying Developmental Plasticity in Termites
Originating at least 150 million years ago (Thorne et al., 2000; Bucek et al., 2019; Evangelista et al., 2019), termites are social cockroaches (order Blattodea), sister to the sub-social cockroach genus, Cryptocercus (Lo et al., 2000; Inward et al., 2007; Bourguignon et al., 2015; Bucek et al., 2019; Evangelista et al., 2019). A traditional classification of termites is based on symbionts: “lower termites” have bacteria and flagellates in their guts (Figure 1C). This is true of all termite families except the Termitidae (“higher termites”), which have no flagellates but other gut symbionts, mostly bacteria (Figure 1C). Termites are key cellulose decomposers in hotter ecosystems (Takamura, 2001), can feed on many substrates (grass, wood, leaf litter, or soil) (Eggleton, 2011) and have important roles in enhancing ecosystem resistance to drought (Ashton et al., 2019). The value of their ecosystem services (Jouquet et al., 2011) and the disservices they can cause to buildings and crops (Su and Scheffrahn, 2000; Rouland-Lefèvre, 2011), makes them important species to study (Govorushko, 2019). As a result, there is a considerable body of literature on the natural history of termites, providing a foundation of information on phenotypic potency and plasticity. In this section we review the different facets of termite life-history that provide the means to classify phenotypes based on their level of plasticity, illustrating the difficulties of classifying potency from current literature and highlighting the need for new terminology to explain variation in potency.
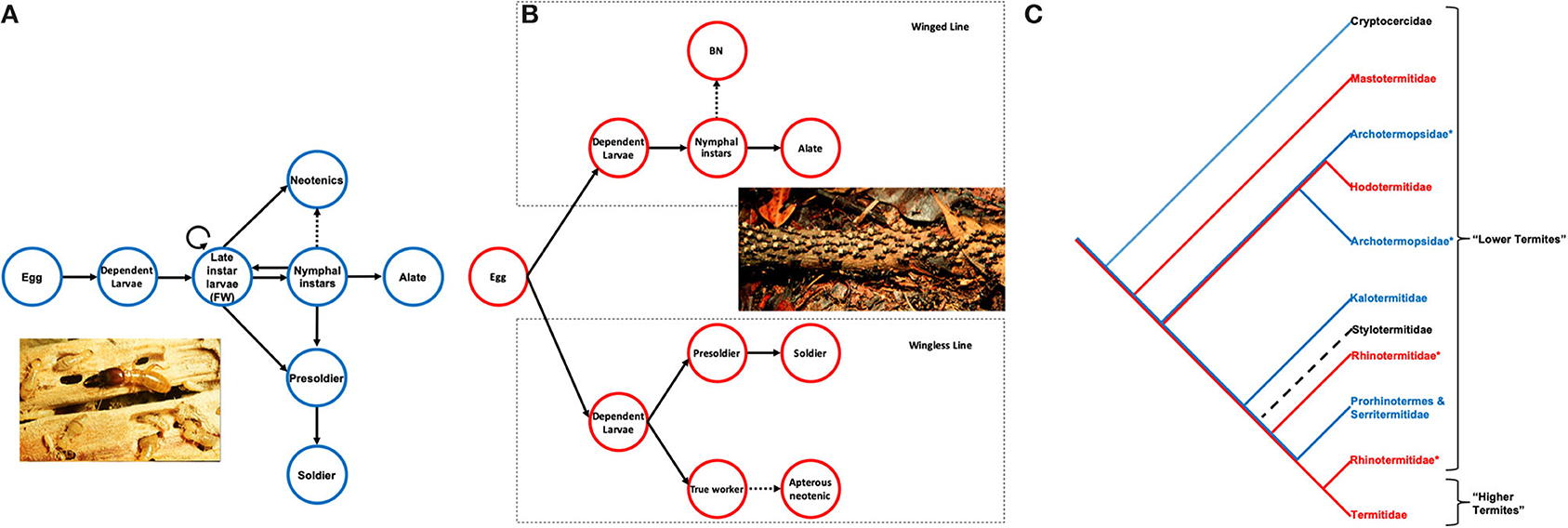
Figure 1. Simplified developmental pathway of (A) wood nesting termites and (B) foraging termites. Solid forward arrows, progressive molts; solid backward arrows, regressive molts; dashed arrows, potential progressive molts in some species; circular arrow, stationary molt; BN, Brachypterous neotenics. Modified after (Korb and Hartfelder, 2008). (C) Phylogenetic relationships of termite families (cladogram) using the sister taxon Cryptoceridae as outgroup, highlighting the phylogenetic uncertainty of life types and ambiguity surrounding which came first in evolutionary terms. Blue, Wood-dwelling termite species; Red, Foraging termite species; Black dashed, not enough is known about this family to definitively categorize but they are likely to be wood-dwelling species, *signifies paraphyletic families. Modified after (Korb, 2019), (See Box 1 for definitions). [photos by Bill Sands, NHM London collection: (A) Zootermopsis (B) Hospitalitermes].
Developmental Stage and Phenotypic Potency
All species of termites exhibit a range of phenotypic potency which is shared across species and largely based on their stage in development (Miura et al., 2000; Bourguignon et al., 2012b; Haifig and Costa-Leonardo, 2016). Phenotypic states exhibit plasticity along a gradient. First, high plasticity, where an individual has the potential to become (almost) any other phenotype, under environmental cues; e.g., first and second instar larvae (Box 1). Second, lower levels of plasticity, where an individual may have limited ability to change into a small number of phenotypes; e.g., nymphs and later instar larvae. Finally, no plasticity, where an individual is committed to a phenotypic state and cannot change, irrespective of the environmental cue; e.g., primary reproductives, neotenic reproductives and soldiers (Box 1). However, there are important exceptions. Both workers and nymphs (Box 1) show variation in their plasticity both within and between species (Noirot, 1955; Roisin, 2000; Lainé and Wright, 2003; Bourguignon et al., 2012b). Moreover, both egg and larval potency can vary across species and often between sexes (Roisin and Lenz, 1999).
In some species, phenotype is determined by sex and this can influence phenotypic potency. This consequently makes categorizing potency based on developmental stage purely more difficult. For instance, high sexual size dimorphism has led to the loss of soldier production in one sex in a number of species as well as ancestral loss in one sex in the higher termites which have led to the majority presence of single sex soldiers (Bourguignon et al., 2012a). In Coptotermes lacteus, only females can become soldiers, and so male egg and larval instars cannot become soldiers (Roisin and Lenz, 1999). Therefore, a sex within a species may have a lower level of phenotypic potency at all developmental stages than the complementary sex. However, more experimental evidence is required to determine whether the constraints on potency of a single sex is genetically or epigenetically determined, and if epigenetically determined, at which developmental stage soldier production is inhibited. Consequently, defining potency of termite phenotypes based on their developmental stage should not be used as the only criterion.
Influence of Life-History on Phenotypic Potency
Two main life-history types are found within termites, classified by the relationship between foraging and nest site (Abe, 1987; Korb, 2019); these life-history types are associated with fundamental differences in developmental plasticity. Wood-dwelling (Box 1) species nest and feed in the same piece of dead wood (Korb, 2019) (Figure 1A, Box 1). Species within this life type are found throughout lower termite families (Korb and Hartfelder, 2008; Bourguignon et al., 2015) (Figure 1C). Wood-dwelling termites have high levels of developmental plasticity, with progressive, regressive and stationary molts (Box 1) allowing reversible morphological change into any phenotype, in response to ecological and social cues (Figure 1A). The immatures (larvae) exhibit highly plastic phenotypes and are a stage from which all other more committed phenotypes, for example soldiers, nymphs, primary reproductive, and neotenic reproductive, develop. Because of this high plasticity, and lack of commitment, working individuals of the wood-dwelling termites are often referred to as “false workers” [See (d), Box 1].
The second type of life-history is the foraging termites (Abe, 1987), which have well-defined nests, but foraging takes place away from these nests (Korb, 2019) (Figure 1B). Families with this life-history include Mastotermitidae, Hodotermitidae, most Rhinotermitidae, and all Termitidae (Korb and Hartfelder, 2008) (Figure 1C). Foraging termites have lower levels of developmental plasticity than wood-dwelling species. Seasonal cues dictate whether early instar larvae molt into winged or wingless lines (Figure 1B, Box 1). Therefore, there is an irreversible early commitment into either the winged line, where winged sexuals develop from nymphal instars, or the wingless line, which leads to “true” workers and soldiers. Consequently, individuals within the wingless line are unable to become primary winged reproductives (Figure 1B).
However, the seeming dichotomy in developmental potential between wood-dwelling to foraging termites and “false” to “true” workers is an underestimation of the variation in plasticity between species. For instance, there are some species with “true” workers that are not in fact committed to sterility and are able to molt into wingless reproductives under particular conditions, some species with “true” workers which can only become soldiers, and some species with “true” workers which are at a final state. Therefore, they exhibit differing levels of potential and should not be defined purely as “true” workers when discussing phenotypic potency. Instead of a purely “totipotent versus committed” view of plasticity, we envisage a multi-level change in plasticity of certain phenotypes between and within species. We need to find an alternative descriptive framework that accounts for this variation in order to facilitate a better understanding of the complexity in plasticity across the termites.
Employing Cell Biology Terms as Descriptors of Hemimetabolous Potency
There is currently no terminology sufficient to capture the multiple levels of plasticity, and the nuances among different categories of “committed” and “plastic” states within hemimetabolous insects. We suggest, therefore, borrowing terms from cell biology. Comparisons have already been made with the mechanistic basis for aging in termites and germline cells (Elsner et al., 2018), therefore extending this comparison to potency may help us to better comprehend termite plasticity and consequently hemimetabolous insects. Both hemimetabolous insects and stem cells have potential to change into a range of phenotypes across many periods of development, as they are not restricted by a developmental barrier, as with metamorphosis in holometabolous insects. This is exemplified in the polymorphic social aphids, gall-forming thrips, and particularly termites (Crespi, 1992; Stern and Foster, 1996). By having a unifying framework applicable to all hemimetabolous insects, comparisons can be made across all the levels of social complexity; from the highly complex termites, to the subsocial Cryptocercidae and even to solitary hemimetabolous insects like locusts which exhibit high individual plasticity (Nalepa, 2010; Lo et al., 2018). Here, we outline the different stem cell potency terms, specific to mammals, and explore how they can be used to describe termites' and more generally hemimetabolous insect phenotypic diversity.
Stem cells are clone-producing cells, capable of both self-renewal and multilineage differentiation (Till and McCulloch, 1961; Metcalf and Moore, 1971). There is variation in the capacity of different types of stem cells to differentiate into certain mature cell lines (Weissman, 2000), with a terminology used to describe this variation fully (Figure 2). First, Totipotent describes a cell that can turn into any type of cell: within a developing multicellular organism, this is the zygote, and it is the first cell to form after fertilization (Figure 2). Pluripotent is used to describe cells that retain the ability to renew indefinitely and to differentiate into cells of all three germ layers (mesoderm, endoderm, and ectoderm): these are embryonic stem cells produced from the inner cell mass of mammalian blastocysts (Figure 2). Pluripotent stem cells differ from totipotent cells in that they cannot become extraembryonic cells (Evans and Kaufman, 1981; Martin, 1981): they have lost one facet of plasticity.
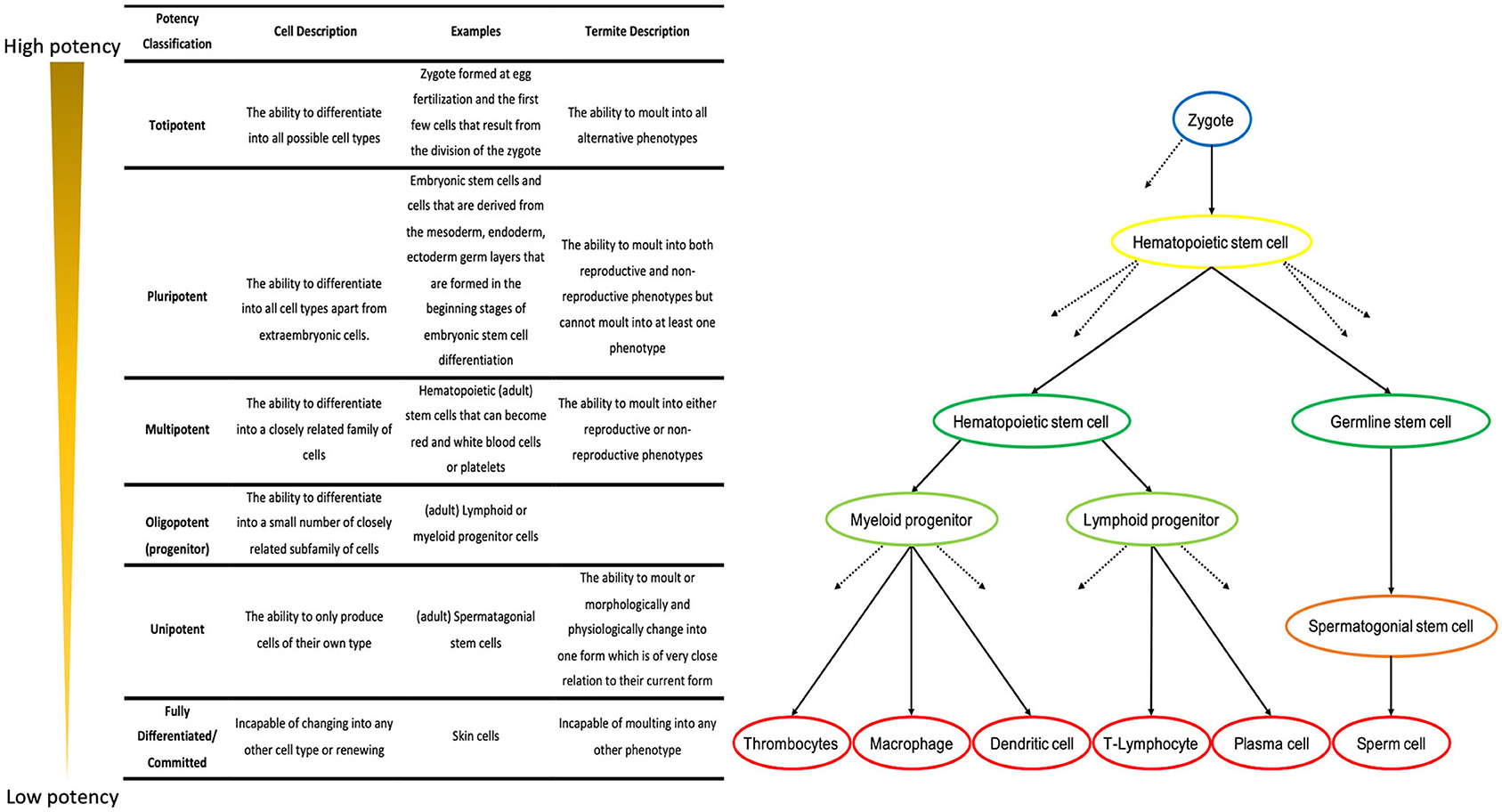
Figure 2. Terminology used to describe levels of potency within cells with examples, adapted from (Kalra and Tomar, 2014), and simplified cellular developmental pathway showing the different levels of developmental plasticity. Everything but the zygote and embryonic stem cells are cells in an adult mammal. Full arrows, pathway from one cell type to another; dashed arrows, presence of other pathways not shown. Blue, Totipotent; Yellow, Pluripotent; Green, multipotent; Light Green, Oligopotent (progenitor); Orange, Unipotent; Red, fully differentiated.
The next level of potency is multipotent cells, which are able to differentiate into multiple organ and tissue-specific lineages (Figure 2). These are organ- and tissue-specific stem cells which are present eight days post-fertilization and throughout adulthood within multicellular organisms; they include haematopoietic stem cells (Weissman, 2000), and bone marrow cells which can self-renew and differentiate into all blood cell lineages (Till and McCulloch, 1961; Becker et al., 1963; Wu et al., 1968). Multipotent cells transition through multiple stages of irreversible maturation, before becoming progenitor cells which cannot renew themselves. This further loss of potency is described as oligopotent (Figure 2) (Weissman et al., 2001). Oligopotent cells are common myeloid progenitors or common lymphocyte progenitors (Akashi et al., 2000). They are committed to a single lineage of a small number of mature cell fates (Figure 2). Of these, only multipotent is needed to describe potency levels within hemimetabolous insects.
A final level of potency in cells is termed unipotent: these are cells that are only able to differentiate into one fully differentiated cell type; this term is limited in use to spermatogonial stem cells, which differentiate into sperm cells (Figure 2) (de Rooij, 2001). The committed state is a fully differentiated cell which is are no longer able to change into any other form; it can be considered a mature cell and it is committed to this final state (Figure 2). For hemimetabolous insects, it may be more appropriate and useful to use “committed” as it better describes their development than the more cell-centric “fully differentiated” used within stem cell research.
Cell Potency Terminology Applied to Termites
Here, we illustrate how cell potency terminology can be aligned with levels of developmental plasticity in hemimetabolous insects, particularly termites. To do this, literature data was collated on the plasticity potential of different phenotypes within and between termite species, across developmental stages (See Supplementary Materials 1, 2). The developmental pathways of 73 species were identified using a Web of Science search for each family name and development stage, refining the search by using lower taxonomic units where necessary. Confidence in assigning a particular level of plasticity for a given phenotype depends on the evidence type: observational studies, using morphometric and molting data, are less conclusive than experimental studies, where potency has been experimentally instigated, e.g., by colony orphaning (See Supplementary Material 2 for categorization of the potency of each phenotype in every species and qualitative confidence levels for each assignment). Social and environmental pressures can limit plasticity among termite phenotypes leaving them functionally less plastic; furthermore, the stage in the colony cycle may influence the expression of plasticity (Chouvenc and Su, 2014). Many of our classifications, therefore, will require confirmatory manipulation experiments and more data collection. Despite this, the study shows how aligning potency terminology from the stem cell literature with potency levels in termites provides a useful framework for studying the evolution and mechanisms of plasticity in termites and hemimetabolous insects generally. We now describe each level of potency, explaining their trends across the phylogeny and developmental stages, provide examples of differing levels of plasticity within and between species and then highlight exceptions to these trends that exemplify the need for a framework that transcends the traditional metrics of plasticity categorization (which are discussed in section “The Challenges of Classifying Developmental Plasticity in Termites”).
Totipotent
In termites, individuals can be thought of as totipotent when they are able to molt into all alternative phenotypes: winged or wingless reproductives, workers or soldiers (Table 1, Box 1). Totipotent individuals include all workers (See Figure 3A—blue, Supplementary Materials 1, 2) and dependent larval stages in the Termopsidae, Kalotermitidae, Serritermitidae and some Rhinotermitidae (e.g., Prorhinotermes inopinatus, Termitogeton planus, Psammotermes hybostoma). It also includes nymphal stages when they are able to regressively molt back into workers; totipotent nymphs are restricted to the lower termites (Box 1)—the Termopsidae, Kalotermitidae and a number of Rhinotermitidae.
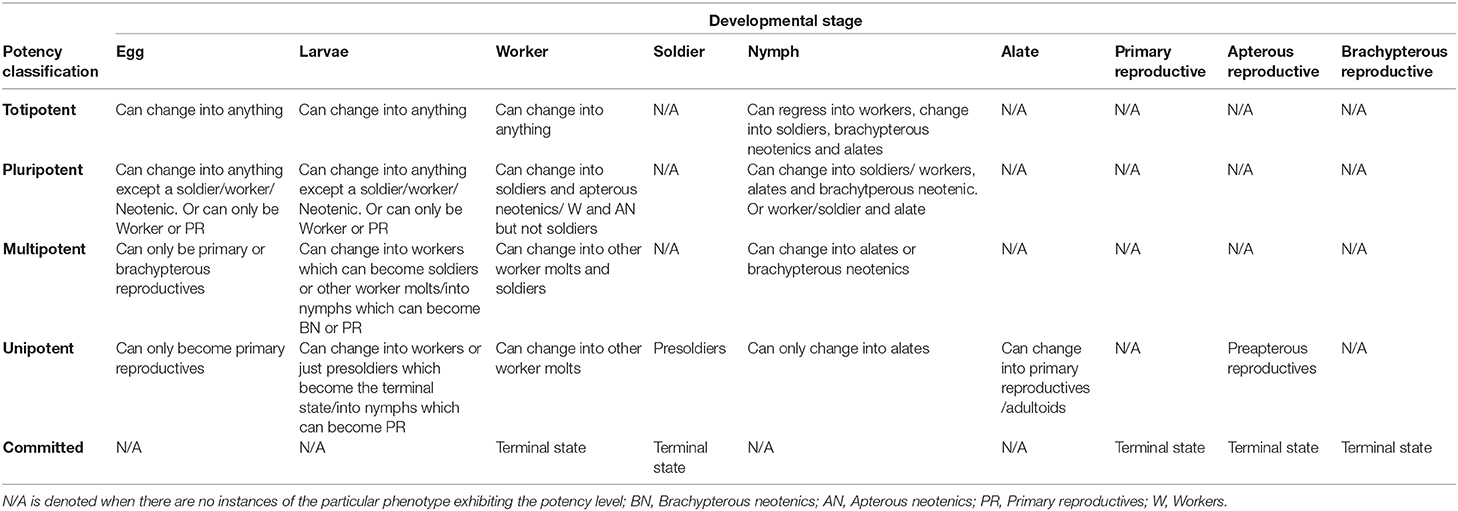
Table 1. Applying cell potency terminology to the different developmental stages of termites; note that a single developmental stage may exhibit different levels of potency, depending on the species; furthermore, a single level of potency can be exhibited by a range of developmental stages, across species.
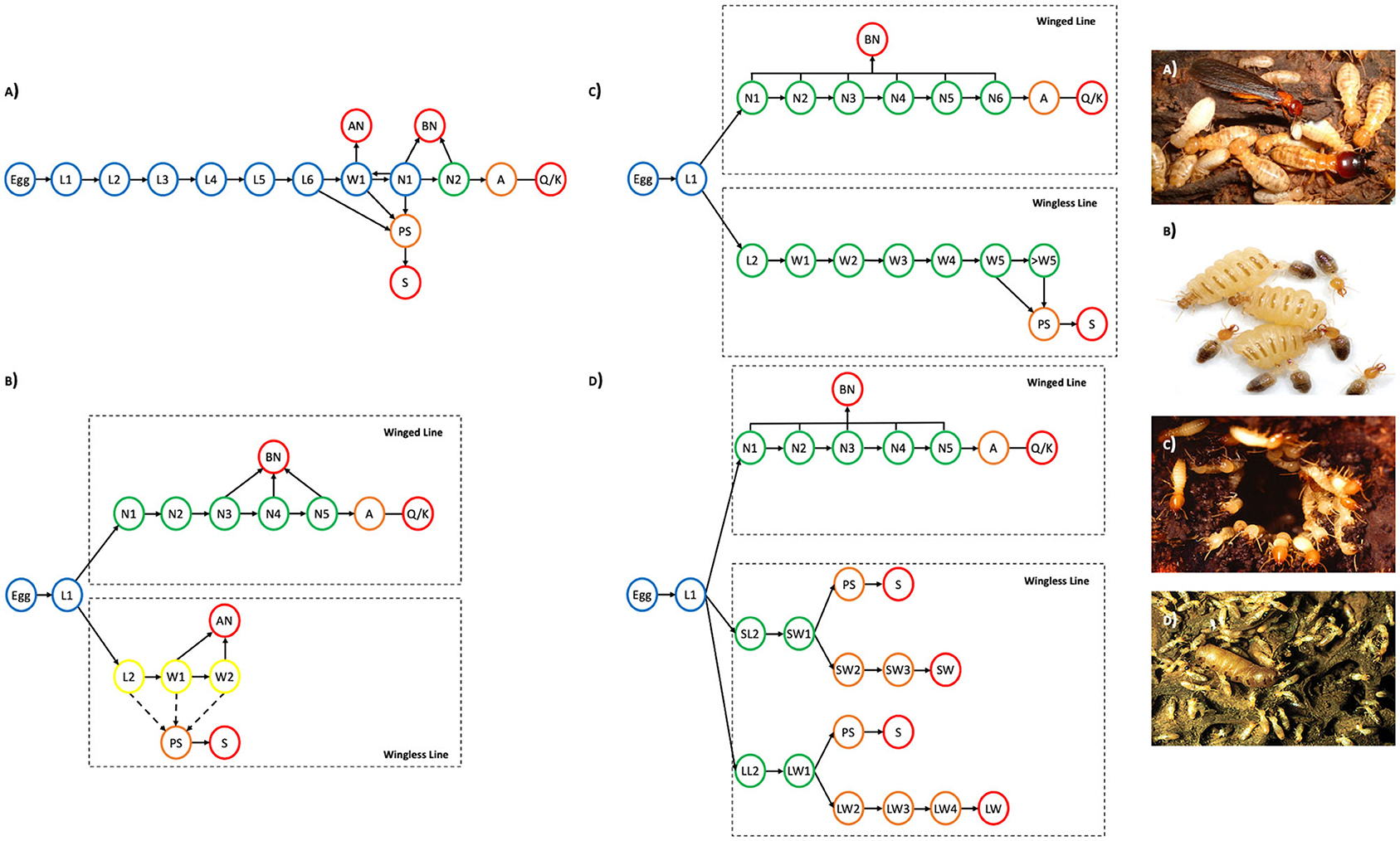
Figure 3. Developmental pathways of (A) the wood-dwelling termite Hodotermopsis sjostedti, Modified after (Miura et al., 2000); (B) Females in the foraging termite Silvestritermes euamignathus (both solid and dashed arrows) and males in the foraging termite Silvestritermes euamignathus (only solid arrows), Modified after (Haifig and Costa-Leonardo, 2016); (C) females in the foraging termite Coptotermes lacteus, Modified after (Roisin and Lenz, 1999); (D) the foraging termite Microcerotermes beesoni, Modified after (Rasib and Akhtar, 2012). Arrows symbolize molts. Forward arrows, progressive molts; Backward arrows, Regressive molts; dashed lines; only one sex can molt into this phenotype. Lines symbolize morphological and physiological change without molt. L1, Larval instar 1; W1, worker instar 1; N1, nymphal instar 1; AN, Apterous Neotenic; BN, Brachypterous Neotenic; A, alate; PS, presoldier; S, soldier; Q/K, queen/king. Blue, Totipotent; Yellow, Pluripotent; Green, Multipotent; Orange, Unipotent; Red, Committed. (photos: A) Hodotermopsis sjostedti- https://polyphenism.wordpress.com/2006/04/01/japanese-damp-wood-termite/hodotermopsis-sjostedti/, (B) Silvestritermes minutus—Robert Hanus, (C) Coptotermes formosanus, (D) Microcerotermes—Bill Sands, NHM London collection.
Eggs, and first and second instar larvae in most species of lower termite are totipotent; exceptions are females in Glossotermes occulatus (Bourguignon et al., 2009), Serritermes serrifer (Barbosa and Constantino, 2017) and Hodotermes mossambicus (Roisin, 2000) and males in Coptotermes lacteus (Roisin and Lenz, 1999), which cannot become soldiers and females in Anacanthotermes ahngerianus (Roisin, 2000) which cannot become soldiers or workers. These sex specific developmental trajectories are more prominent in the higher termites. Consequently, most higher termites have either only one sex which have totipotent eggs and early instar larvae or none at all, due to one sex not being able to produce soldiers or neither being able to produce neotenics, respectively. For instance, in Acanthotermes acanthothorax (Noirot, 1955), males are unable to become soldiers and therefore eggs and the first larval instar of males already have reduced developmental potential.
Pluripotent
Pluripotency is a small reduction in developmental potential in comparison with totipotency, therefore they still have very high plasticity. An example is the pluripotent workers (See Figure 3B, Supplementary Materials 1, 2) which have diverged from the winged line and so cannot become winged reproductives. However, they still have the ability to become wingless reproductives and take over a nest when the primary queen or king dies. Therefore, their plasticity is not so different from totipotent workers as they are still able to span both sterile and reproductive phenotypes. Most commonly, therefore, pluripotency is signified by the removal of the ability to become one particular phenotype (Table 1). This would apply also to nymphal instars which cannot become soldiers but can regressively molt to become a worker, therefore are able to switch to a sterile fate (Table 1). A number of species which do not produce neotenics will therefore have pluripotent eggs and L1, such as the egg and L1 stages in Termes baculi (Noirot, 1955). Furthermore, in some species one sex cannot become particular phenotypes (e.g., soldiers); therefore they have pluripotent eggs and L1; e.g., the males of Silvestritermes euamignathus (Haifig and Costa-Leonardo, 2016) (Figure 3B). A less common form of pluripotency is when an individual has lost the ability to express two of the possible phenotypes, but the phenotypes that still can be expressed span both sterility and reproduction. An example of this can be seen in male workers of Silvestritermes euamignathus (Haifig and Costa-Leonardo, 2016) (Figure 3B). Here, they can only become sterile workers or apterous neotenics.
Examples of pluripotent workers are known throughout both lower and higher termites, including Mastotermitidae, Rhinotermitidae and a number of species within the Termitidae. Pluripotent nymphal instars have only been shown within both Reticulitermes lucifugus and Reticulitermes flavipes (Lainé and Wright, 2003). Their nymphal instars cannot molt into soldiers but can become every other phenotype.
Multipotent
Multipotent describes the loss of plasticity with respect to a specific developmental pathway i.e., wingless (non-reproductive) or winged (reproductive) of the foraging termites (Figure 1B). Individuals still show plasticity and can molt into another form within the pathway, but they are committed to either a reproductive or non-reproductive pathway. For example, nymphs of foraging termites are multipotent as they can molt into winged alates, which are committed to dispersing, or brachypterous neotenics, which are committed to non-dispersal; but they are distinct from pluripotent or totipotent nymphs as they cannot regress back into workers or soldiers: they are committed to a reproductive fate (Table 1). Workers, and the larvae that produce these workers, are multipotent when they can only molt into soldiers or other worker forms and are therefore committed to a non-reproductive fate (Table 1).
Multipotent phenotypes are mainly found within the foraging termites. However, there may be more species-specific complexities than initially apparent. For example, Psammotermes hybostoma potentially has both totipotent and multipotent workers, with earlier worker instars potentially becoming apterous neotenics, nymphs or soldiers and the later instars only able to become soldiers (Bourguignon et al., 2012b) (See Figure 3C, Supplementary Materials 1, 2). Further experimental work is required to determine the potency of these and many other species (Table 1).
Female eggs and early instar larvae in the foraging termite, Anacanthotermes ahngerianus, can be considered multipotent because they are only able to become either primary reproductives or brachypterous neotenics (Roisin, 2000). Therefore, females in this species exhibit severely reduced developmental potential compared with males as they are totipotent at the egg and early larval instar stages. When soldier production is sex specific, it is possible that one sex will have multipotent workers and the other sex will have unipotent and committed workers, as seen in multipotent female workers and committed male workers in Macrotermes natalensis (Noirot, 1955).
Unipotent
Individuals are unipotent when they are only able to change into one form that is similar to their current form. For example, presoldiers, alates and pre-apterous reproductives in all species can only become soldiers, primary reproductives and apterous reproductives respectively. They are limited to a phenotype within their own specific lineage and so are unipotent. Unipotent workers are the instars preceding the final committed worker form: they cannot molt to become soldiers (Table 1). For instance, large worker instars L2-4 in Microcerotermes beesoni are unipotent as they are only able to molt into other worker forms (See Figure 3D, Supplementary Materials 1, 2) (Rasib and Akhtar, 2012). Similarly, unipotent nymphs are those which can only molt into alates and therefore are only able to become winged reproductives (Table 1, Figure 3D).
Unipotent workers are mainly found in higher termites, such as Amitermes and Microcerotermes species, and a number of the Nasutitermitinae which have a particular sex which cannot become a soldier. Only Coptotermes lacteus (Roisin and Lenz, 1999), Coptotermes formosanus (Chouvenc and Su, 2014), and Hodotermes mossambicus (Roisin, 2000) in the lower termites have unipotent workers. Unipotent nymphs can be seen in some lower termites, which seemingly lack the ability to produce brachypterous neotenics, but are most prevalent in the higher termites. One instance of unipotent eggs and L1 has been shown in Cornitermes walkeri as females only become primary reproductives (Roisin, 1992) (Table 1).
Committed
Individuals are “committed” when they are incapable of molting into any other form: this includes soldiers, primary reproductives, apterous neotenics, and brachypterous neotenics. Species where workers are the final form and cannot molt into soldiers are also committed (Table 1); these are observed only in a small number of species within the higher termites such as the Macrotermitinae, and in some cases only one sex has committed workers whilst the other sex produces multipotent workers able to become soldiers. The Apicotermitinae are predominantly soldierless species, and all appear to have committed workers. However, they are poorly studied, due to the difficulty in identification without the soldier caste (Bourguignon et al., 2016) (See Figure 3D, Supplementary Materials 1, 2).
Future Directions
Termites show enormous phenotypic diversity due to developmental plasticity. They therefore make excellent study systems for fundamental questions surrounding the nature, mechanisms and evolution of phenotypic plasticity, which are applicable to all taxa (a, c). These questions in turn help shine some light on longstanding questions surrounding termite evolution (b, d). The conceptual framework we propose is made possible through an interdisciplinary translation of terminology from the cellular biology world into the world of termite phenotypic plasticity. We anticipate that the formalization of how to classify individual-level potency in termites will facilitate further research on the proximate and ultimate bases of termite caste evolution, and ultimately the nature of phenotypic plasticity. We unpack a few examples here, to illustrate the utility of our framework and to demonstrate the research potential from identifying the parallels between cellular and termite developmental plasticity.
Are There Conserved Mechanisms That Characterize Changes in Potency of Phenotypes Across Different Organisms?
Our new framework opens up opportunities to examine the mechanistic basis for loss and gains in plasticity at a finer scale and allows for comparisons with other taxa, such as Hymenoptera. A phylogenetically directed comparative analysis across termite species would reveal where and when losses and gains in the different forms of plasticity occurred and afford the opportunity to detect patterns of correlated environmental and ecological traits that may explain their evolution. Much work has been done relating the ecological context of wood-dwelling and foraging termites which leads them to have totipotent and more developmentally restricted workers, respectively (Korb and Thorne, 2017; Korb, 2019). Potentially multiple losses and gains of totipotent workers have occurred throughout the phylogeny (Legendre et al., 2013), providing us with a good study system to elucidate the mechanistic basis for these loss and gains. The classifications in our framework provide the categorical variables required for such comparative analyses to be performed. Moreover, the lowering in potency for one sex, from multipotent to unipotent or committed, due to their inability to become soldiers has been observed in a number of species. These differences have been attributed to high sexual size dimorphism leading to the favoring of the sex which has the appropriate size for defense of the nest (Bourguignon et al., 2012a). It has however been shown that in some species which have low to no sexual size dimorphism, both sex soldiers have evolved again. Therefore, gains in potency through evolutionary time can occur (Bourguignon et al., 2012a). This provides another avenue within termites to explore the fundamental mechanistic basis for losses and gains in plasticity. Furthermore, by comparing these findings to those from Hymenopteran and cellular studies, we may be able to find common molecular processes which have been conserved relating to losses and gains in plasticity.
What Came First, Totipotent or the More Developmentally Committed Workers?
By understanding the proximate mechanisms behind the changes in developmental plasticity which arise because of varying ecological pressures, we will be able to elucidate questions relating specifically to termite evolution. For instance, there has been over 30 years of debate over whether the ancestral state of termites was a linear developmental pathway with totipotent workers or a bifurcated pathway with more developmentally restricted workers, with no conclusive evidence to provide an answer (Noirot, 1985; Watson and Sewell, 1985; Legendre et al., 2013) (Figure 1C). We see from Legendre's (2013) phylogenetic analyses that there have been potentially multiple emergences of more developmentally restricted worker caste formations. Interestingly, there has also potentially been the loss of more developmentally restricted worker castes and the emergence of more plastic totipotent worker species in the phylogeny. It may be that these are examples of rapid evolution under strong ecological pressure, whereby once foraging is required in a species, a more developmentally restricted worker will follow. However, the binary “totipotent or not” categorization has inevitably hindered our ability to elucidate the ancestral developmental state due to its coarse categorization. Instead, by using our new framework we will be more informed on the transitions we see across the phylogeny, be it totipotent to pluripotent or totipotent to multipotent. The new framework will provide greater context to what these transitions between linear and bifurcated pathways entail and therefore break apart the erroneous notions that all the transitions seen on the phylogeny are the same. By bringing new light to the debate we will be better equipped to tackle this fundamental evolutionary question in termite biology.
What Mechanisms Underpin Different Types of Plasticity, and Are These Mechanisms the Same Across Independent Evolutionary Lineages?
As we have shown, termites have high variation in potential developmental trajectories, analogous to the developmental potential in stem cells. Our framework benefits from the extensive cellular literature, which defines this variation in developmental potential. Clearly defined categories of “potency” allow us to select precise termite phenotypes for studying the underlying molecular mechanisms. There is already a body of research on the molecular basis of termite plasticity, predominantly based on the lower termites (Zhou et al., 2006; Korb and Hartfelder, 2008; Korb, 2015), from which a suite of molecular signatures can be identified, highlighting the importance of juvenile hormone and its associated gene pathways (e.g., the hexamerin genes) in caste regulation (Zhou et al., 2006; Korb, 2015). However, research is beginning to look at the higher termites, highlighting the importance of caste-biased gene expression, such as for vitellogenin genes, which have been repeatedly co-opted for diverse functions across different castes in termites (Weil et al., 2007; Sun et al., 2019). The new framework allows the exploration of commonalities within potency level across phenotypes and species of termites and even across other hemimetabolous insects like aphids, gall-forming thrips and locusts (Crespi, 1992; Stern and Foster, 1996; Lo et al., 2018). For instance, the soldier form is shared across the termites and some aphids and gall-forming thrips (Tian and Zhou, 2014). In the parthenogenetically reproductive social aphid, Tuberaphis styraci, soldiers are determined in late first instar female nymphs, meaning before this the female nymphs are pluripotent, able to become either a primary reproductive or soldier (Kutsukake et al., 2004; Shibao et al., 2010). After the late first instar stage, individuals molt into either a soldier which is committed or a second instar nymph which is unipotent, before molting into a primary reproductive which is committed. Males in this species can only become primary reproductives so are unipotent until they molt into adults and are then committed. It will likely also prove useful for comparing across other taxa such as Hymenoptera, due to there being, albeit more restricted, variation between individuals and between species in potency level pre-metamorphosis. For example, it may be that some exceptional hymenopteran species, like Pogonomyrmex barbatus which have genetic caste determination and worker polypmorphism, lose their ability to become reproductives but retain their ability to become one of a number worker phenotypes and therefore could be seen as multipotent at a certain stage of development (Cahan et al., 2002; Julian et al., 2002; Volny and Gordon, 2002; Cahan and Keller, 2003). Also, since every male in Hymenopteran species are genetically determined to be reproductives only, the pre-adult stages can be seen as unipotent transitioning to committed. Moreover, a fundamental further question is whether the molecular signatures that dictate termite potency are the same as those molecular signatures that dictate cell potency. The mechanisms may be conserved across independent evolutionary events or occur via novel mechanisms. To date, there has been very little research drawing comparisons between termite biology and cellular biology (Elsner et al., 2018). However, this new framework opens up a great breadth of potential interdisciplinary research due to identifying the amazing parallels between cellular and termite developmental plasticity. Only by creating this framework can we now go on to study further whether this is more than merely analogy.
What Constitutes a Termite Superorganism?
Termite colonies can be considered to have organism-like characteristics (Elsner et al., 2018). Our study has identified major conceptual parallels in development between cellular and termite colony development, therefore, further work potentially identifying more homologous mechanisms shared between these will support this organism-like quality of a termite colony. This inevitably has connections with superorganismality, leading us to ask what characteristics a termite superorganism would have (Boomsma and Gawne, 2018). The question of commitment is important to any theory of phenotypic plasticity and also for identifying candidates for superorganismality (Boomsma and Gawne, 2018). The mainly binary development within Hymenoptera makes it easier to establish likely candidates for superorganismality. Research has clarified caste potentials and therefore their relative commitment to colony roles. The true and false worker categorization in termites are a useful classification that do show contrasts in social complexity probably due to changes in ecology. However, they do not encapsulate the most important trait of workers which are needed to be classed as superorganismal: that they are sterile and are committed to their worker fate (Boomsma and Gawne, 2018). True workers in some species, although have irreversibly diverged from the winged line, are still able to go on to become apterous neotenic replacement reproductives, and therefore have not completely committed (Noirot, 1955, 1969; Thorne and Noirot, 1982; Roisin and Pasteels, 1987; Vieau, 1991, 1994; Watson and Abbey, 2007; Moura et al., 2011; Haifig and Costa-Leonardo, 2016). Consequently, species with these workers should not be classed as superorganismal as these have not transcended to a higher level of individuality (West et al., 2015). This current categorization used to define whether a termite has reproductively committed workers, and therefore is superorganismal or not, is insufficient to precisely identify potential candidates for superoganismality. Our new framework allows us to more accurately distinguish between workers which are reproductively committed (multipotent, unipotent, and committed) and those which are not (totipotent, pluripotent). Therefore, using the most recent definition of superorganismality (Boomsma and Gawne, 2018), future research surrounding the transition to superorganismality within termites should concentrate on the transition between pluripotent and more developmentally committed workers.
However, it seems that within termites, the prerequisite of reproductively committed worker castes (multipotent, unipotent, and committed workers) for superorganismality may leave out highly socially complex species such as in the Nasutitermitinae. Even the less socially complex species with high plasticity have a committed soldier caste and therefore have high inclusive fitness acting upon them. It may be that Hymenoptera-centric definition of superorganismality will need to be revised to allow the full extent of insect sociality to be acknowledged. Furthermore, clearly hemimetabolous insect development show greater parallels with cellular development within an organism so it may be that when revising this definition, termite sociality should be given greater consideration.
Conclusion
Developmental plasticity drives the vast diversity in form and behavior seen within and between all taxa. Research on the patterns of phenotypic plasticity have investigated both holometabolous and hemimetabolous insects but there is still a bias toward the holometabolous. This bias is clear in the work on social insects, where holometabolous research dominates our understanding of developmental plasticity and social evolutionary processes (Evans and Wheeler, 2001; West-Eberhard, 2003; Toth and Rehan, 2016; Boomsma and Gawne, 2018). The existing dichotomous view of potency, derived from social Hymenoptera, is insufficient to provide a useful framework for termite developmental plasticity. Moreover, the current categories for explaining potency in termites, be it life history or developmental stage, falls short in encapsulating the nuances of developmental plasticity, both within and between termite species. To address this, we have highlighted parallels in developmental potency of hemimetabolous insects with stem cell plasticity and suggested how we might borrow cell potency terminology to categorize potency among the diverse phenotypes exhibited by termite species. In doing so, a comprehensive dataset has been created on the known potencies of phenotypes across the developmental pathways of 73 species of termites which represent the diversity in life histories and evolutionary relationships (See Supplementary Materials 1, 2). We anticipate that in proposing this new terminology and framework, that termites may become more accessible as a resource for advancing our understanding of mechanisms and evolution of developmental plasticity across all taxa. Future work may discover more direct mechanistic parallels relating to plasticity in cellular and termite development systems.
Author Contributions
LR compiled the database and drafted the manuscript. LR, SS, and PE contributed equally in editing the manuscript. All authors contributed to conceiving the manuscript.
Funding
This work was supported by the Natural Environment Research Council through the London NERC Doctoral Training Programme scholarship to LR (Grant No. NE/L002485/1).
Conflict of Interest
The authors declare that the research was conducted in the absence of any commercial or financial relationships that could be construed as a potential conflict of interest.
Acknowledgments
We would like to thank Dr. Thomas Bourguignon for providing vital material otherwise inaccessible and SS's lab group for constructive advice on the manuscript.
Supplementary Material
The Supplementary Material for this article can be found online at: https://www.frontiersin.org/articles/10.3389/fevo.2021.552624/full#supplementary-material
Table S1. Termite potency descriptions.
Table S2. Termite potency categorizations.
Table S3. Bibliography of termite potency descriptions.
References
Abe, T. (1987). “Evolution of life types in termites,” in Evolution and Coadaptation in Biotic Communities, eds S. Kawano, J. H. Connell and T. Hidaka (Tokyo: University of Tokyo Press), 125–148.
Akashi, K., Traver, D., Miyamoto, T., and Weissman, I. L. (2000). A clonogenic common myeloid progenitor that gives rise to all myeloid lineages. Nature 404, 193–197. doi: 10.1038/35004599
Ashton, L. A., Griffiths, H. M., Parr, C. L., Evans, T. A., Didham, R. K., Hasan, F., et al. (2019). Termites mitigate the effects of drought in tropical rainforest. Science 363, 174–177. doi: 10.1126/science.aau9565
Barbosa, J. R. C., and Constantino, R. (2017). Polymorphism in the neotropical termite Serritermes serrifer. Entomol. Exp. Appl. 163, 43–50. doi: 10.1111/eea.12532
Becker, A. J., McCulloch, E. A., and Till, J. E. (1963). Cytological demonstration of the clonal nature of spleen colonies derived from transplanted mouse marrow cells. Nature 197, 452–454. doi: 10.1038/197452a0
Boomsma, J. J., and Gawne, R. (2018). Superorganismality and caste differentiation as points of no return: how the major evolutionary transitions were lost in translation. Biol. Rev. 93, 28–54. doi: 10.1111/brv.12330
Bourguignon, T., Hayashi, Y., and Miura, T. (2012a). Skewed soldier sex ratio in termites: Testing the size-threshold hypothesis. Insectes Soc. 59, 557–563. doi: 10.1007/s00040-012-0252-8
Bourguignon, T., Lo, N., Cameron, S. L., Šobotník, J., Hayashi, Y., Shigenobu, S., et al. (2015). The evolutionary history of termites as inferred from 66 mitochondrial genomes. Mol. Biol. Evol. 32, 406–421. doi: 10.1093/molbev/msu308
Bourguignon, T., Šobotník, J., Dahlsjö, C. A. L., and Roisin, Y. (2016). The soldierless Apicotermitinae: insights into a poorly known and ecologically dominant tropical taxon. Insectes Soc. 63, 39–50. doi: 10.1007/s00040-015-0446-y
Bourguignon, T., Šobotník, J., Hanus, R., and Roisin, Y. (2009). Developmental pathways of Glossotermes oculatus (Isoptera, Serritermitidae): at the cross-roads of worker caste evolution in termites. Evol. Dev. 11, 659–668. doi: 10.1111/j.1525-142X.2009.00373.x
Bourguignon, T., Šobotník, J., Sillam-Dussès, D., Jiroš, P., Hanus, R., Roisin, Y., et al. (2012b). Developmental pathways of psammotermes hybostoma (Isoptera: Rhinotermitidae): old pseudergates make up a new sterile caste. PLoS ONE 7, 9–13. doi: 10.1371/annotation/426a9f1f-f8dc-4f42-a929-adfdc3e117f9
Bucek, A., Šobotník, J., He, S., Shi, M., McMahon, D. P., Holmes, E. C., et al. (2019). Evolution of termite symbiosis informed by transcriptome-based phylogenies. Curr. Biol. 29, 3728–3734.e4. doi: 10.1016/j.cub.2019.08.076
Cahan, S. H., and Keller, L. (2003). Complex hybrid origin of genetic caste determination in harvester ants. Nature 424, 306–309. doi: 10.1038/nature01744
Cahan, S. H., Parker, J. D., Rissing, S. W., Johnson, R. A., Polony, T. S., Weiser, M. D., et al. (2002). Extreme genetic differences between queens and workers in hybridizing Pogonomyrmex harvester ants. Proc. R. Soc. London. Ser. B Biol. Sci. 269, 1871–1877. doi: 10.1098/rspb.2002.2061
Chouvenc, T., and Su, N.-Y. (2014). Colony age-dependent pathway in caste development of Coptotermes formosanus Shiraki. Insectes Soc. 61, 171–182. doi: 10.1007/s00040-014-0343-9
Corona, M., Libbrecht, R., and Wheeler, D. E. (2016). Molecular mechanisms of phenotypic plasticity in social insects. Curr. Opin. Insect. Sci. 13, 55–60. doi: 10.1016/j.cois.2015.12.003
Crespi, B. J. (1992). Behavioural ecology of Australian gall thrips (Insecta, Thysanoptera). J. Nat. Hist. 26, 769–809. doi: 10.1080/00222939200770481
Crespi, B. J., and Yanega, D. (1995). The definition of eusociality. Behav. Ecol. 6, 109–115. doi: 10.1093/beheco/6.1.109
de Rooij, D. G. (2001). Proliferation and differentiation of spermatogonial stem cells. Reproduction 121, 347–354. doi: 10.1530/rep.0.1210347
Eggleton, P. (2011). “An introduction to termites: biology, taxonomy and functional morphology,” in Biology of Termites: A Modern Synthesis, eds D. Bignell, Y. Roisin and N. Lo (Dordrecht: Springer Netherlands), 1–26. doi: 10.1007/978-90-481-3977-4_1
Elsner, D., Meusemann, K., and Korb, J. (2018). Longevity and transposon defense, the case of termite reproductives. Proc. Natl. Acad. Sci. U.S.A. 115, 5504–5509. doi: 10.1073/pnas.1804046115
Evangelista, D. A., Wipfler, B., Béthoux, O., Donath, A., Fujita, M., Kohli, M. K., et al. (2019). An integrative phylogenomic approach illuminates the evolutionary history of cockroaches and termites (Blattodea). Proc. R. Soc. B Biol. Sci. 286:2076. doi: 10.1098/rspb.2018.2076
Evans, J. D., and Wheeler, D. E. (2001). Gene expression and the evolution of insect polyphenisms. BioEssays 23, 62–68. doi: 10.1002/1521-1878(200101)23:1<62::AID-BIES1008>3.0.CO;2-7
Evans, M. J., and Kaufman, M. H. (1981). Establishment in culture of pluripotential cells from mouse embryos. Nature 292, 154–156. doi: 10.1038/292154a0
Govorushko, S. (2019). Economic and ecological importance of termites: a global review. Entomol. Sci. 22, 21–35. doi: 10.1111/ens.12328
Haifig, I., and Costa-Leonardo, A. M. (2016). Caste differentiation pathways in the Neotropical termite Silvestritermes euamignathus (Isoptera: Termitidae). Entomol. Sci. 19, 174–179. doi: 10.1111/ens.12201
Inward, D. J. G., Vogler, A. P., and Eggleton, P. (2007). A comprehensive phylogenetic analysis of termites (Isoptera) illuminates key aspects of their evolutionary biology. Mol. Phylogenet. Evol. 44, 953–967. doi: 10.1016/j.ympev.2007.05.014
Jandt, J. M., Tibbetts, E. A., and Toth, A. L. (2014). Polistes paper wasps: a model genus for the study of social dominance hierarchies. Insectes Soc. 61, 11–27. doi: 10.1007/s00040-013-0328-0
Jouquet, P., Traoré, S., Choosai, C., Hartmann, C., and Bignell, D. (2011). Influence of termites on ecosystem functioning. ecosystem services provided by termites. Eur. J. Soil Biol. 47, 215–222. doi: 10.1016/j.ejsobi.2011.05.005
Julian, G. E., Fewell, J. H., Gadau, J., Johnson, R. A., and Larrabee, D. (2002). Genetic determination of the queen caste in an ant hybrid zone. Proc. Natl. Acad. Sci. U.S.A. 99, 8157–8160. doi: 10.1073/pnas.112222099
Kalra, K., and Tomar, P. C. (2014). Stem cell : basics, classification and applications. Am. J. Phytomedicine Clin. Ther. 2, 919–930.
Korb, J. (2015). “Juvenile hormone. a central regulator of termite caste polyphenism,” in Advances in Insect Physiology, eds A. Zayed and C. F. Kent (Oxford: Academic Press), 131–161. doi: 10.1016/bs.aiip.2014.12.004
Korb, J. (2019). “Social evolution in termites,” in Encyclopedia of Animal Behavior, eds M. D. Breed and J. Moore (London: Elsevier), 609–616. doi: 10.1016/B978-0-12-809633-8.20751-6
Korb, J., and Hartfelder, K. (2008). Life history and development—a framework for understanding developmental plasticity in lower termites. Biol. Rev. 83, 295–313. doi: 10.1111/j.1469-185X.2008.00044.x
Korb, J., and Thorne, B. (2017). Sociality in termites. Comp. Soc. Evol. 124–153. doi: 10.1017/9781107338319.006
Kutsukake, M., Shibao, H., Nikoh, N., Morioka, M., Tamura, T., Hoshino, T., et al. (2004). Venomous protease of aphid soldier for colony defense. Proc. Natl. Acad. Sci. U.S.A. 101, 11338–11343. doi: 10.1073/pnas.0402462101
Lainé, L. V., and Wright, D. J. (2003). The life cycle of Reticulitermes spp. (Isoptera: Rhinotermitidae): what do we know? Bull. Entomol. Res. 93, 267–378. doi: 10.1079/BER2003238
Legendre, F., Whiting, M. F., and Grandcolas, P. (2013). Phylogenetic analyses of termite post-embryonic sequences illuminate caste and developmental pathway evolution. Evol. Dev. 15, 146–157. doi: 10.1111/ede.12023
Lo, N., Simpson, S. J., and Sword, G. A. (2018). Epigenetics and developmental plasticity in orthopteroid insects. Curr. Opin. Insect Sci. 25, 25–34. doi: 10.1016/j.cois.2017.11.003
Lo, N., Tokuda, G., Watanabe, H., Rose, H., Slaytor, M., Maekawa, K., et al. (2000). Evidence from multiple gene sequences indicates that termites evolved from wood-feeding cockroaches. Curr. Biol. 10, 801–804. doi: 10.1016/S0960-9822(00)00561-3
Martin, G. R. (1981). Isolation of a pluripotent cell line from early mouse embryos cultured in medium conditioned by teratocarcinoma stem cells. Proc. Natl. Acad. Sci. U.S.A. 78, 7634–7638. doi: 10.1073/pnas.78.12.7634
Miura, T., Hirono, Y., Machida, M., Kitade, O., and Matsumoto, T. (2000). Caste developmental system of the Japanese damp-wood termite Hodotermopsis japonica (Isoptera: Termopsidae). Ecol. Res. 15, 83–92. doi: 10.1046/j.1440-1703.2000.00320.x
Moczek, A. P. (2010). Phenotypic plasticity and diversity in insects. Philos. Trans. R. Soc. Lond. B. Biol. Sci. 365, 593–603. doi: 10.1098/rstb.2009.0263
Moura, F. M. S., Vasconcellos, A., Silva, N. B., and Bandeira, A. G. (2011). Caste development systems of the Neotropical termite Constrictotermes cyphergaster (Isoptera, Termitidae). Insectes Soc. 58, 169–175. doi: 10.1007/s00040-010-0132-z
Nalepa, C. A. (2010). Altricial development in subsocial cockroach ancestors: foundation for the evolution of phenotypic plasticity in termites. Evol. Dev. 12, 95–105. doi: 10.1111/j.1525-142X.2009.00394.x
Noirot, C. (1955). Recherches sur le polymophisme des termites superieurs (Termitidae). Ann. Sci. Nat. Zool. 17, 399–595.
Noirot, C. (1969). “Formation of castes in the higher termites,” in Biology of Termites, Vol 1, K. Krishna and F. M. Weesner (New York, NY: Academic Press), 311–350. doi: 10.1016/B978-0-12-395529-6.50014-3
Noirot, C. (1985). “The caste system in higher termites,” in Caste Differentiation in Social Insects, eds J. A. L. Watson, B. M. Okot-Kotber and C. Noirot (Oxford: Elsevier), 75–86. doi: 10.1016/B978-0-08-030783-1.50011-2
Rasib, K. Z., and Akhtar, M. S. (2012). Caste Developmental Pathways in Populations of Microcerotermes championi (Isoptera: Termitidae, Microcerotermitinae). Int. J. Agric. Biol. 14, 161–168.
Roisin, Y. (1992). Development of non-reproductive castes in the neotropical termite genera Cornitermes, Embiratermes and Rhynchotermes (Isoptera, Nasutitermitinae). Insectes Soc. 39, 313–324. doi: 10.1007/BF01323951
Roisin, Y. (2000). “Diversity and evolution of caste patterns,” in Termites: Evolution, Sociality, Symbioses, Ecology, eds. T. Abe, D. E. Bignell and M. Higashi (Dordrecht: Springer Netherlands), 95–119. doi: 10.1007/978-94-017-3223-9_5
Roisin, Y., Lenz, M. (1999). Caste developmental pathways in colonies of Coptotermes lacteus (Froggatt) headed by primary reproductives (Isoptera, Rhinotermitidae). Insectes Soc. 46, 273–280. doi: 10.1007/s000400050146
Roisin, Y., Pasteels, J. M. (1987). Caste developmental potentialities in the termite Nasutitermes novarumhebridarum. Entomol. Exp. Appl. 44, 277–287. doi: 10.1111/j.1570-7458.1987.tb00556.x
Rouland-Lefèvre, C. (2011). “Termites as pests of agricultur,” in Biology of Termites: A Modern Synthesis, eds D. E. Bignell, Y. Roisin and N. Lo (Dordrecht: Springer Netherlands), 499–517. doi: 10.1007/978-90-481-3977-4_18
Schwander, T., Lo, N., Beekman, M., Oldroyd, B. P., and Keller, L. (2010). Nature versus nurture in social insect caste differentiation. Trends Ecol. Evol. 25, 275–282. doi: 10.1016/j.tree.2009.12.001
Shibao, H., Kutsukake, M., Matsuyama, S., Fukatsu, T., and Shimada, M. (2010). Mechanisms regulating caste differentiation in an aphid social system. Commun. Integr. Biol. 3, 1–5. doi: 10.4161/cib.3.1.9694
Stern, D. L., and Foster, W. A. (1996). The evolution of soldiers in Aphids. Biol. Rev. 71, 27–79. doi: 10.1111/j.1469-185X.1996.tb00741.x
Su, N.-Y., and Scheffrahn, R. H. (2000). “Termites as pests of buildings.” in Termites: Evolution, Sociality, Symbioses, Ecology, Y. Abe, D. E. Bignell and T. Higashi (Dordrecht: Springer Netherlands), 437–453. doi: 10.1007/978-94-017-3223-9_20
Sun, P., Li, G., Jian, J., Liu, L., Chen, J., Yu, S., et al. (2019). Transcriptomic and functional analyses of phenotypic plasticity in a higher termite, Macrotermes barneyi light. Front. Genet. 10:964. doi: 10.3389/fgene.2019.00964
Szathmáry, E., and Maynard-Smith, J. (1995). The Major Transitions in Evolution. Oxford: WH Freeman Spektrum.
Takamura, K. (2001). Effects of termite exclusion on decay of heavy and light hardwood in a tropical rain forest of Peninsular Malaysia. J. Trop. Ecol. 17, 541–548. doi: 10.1017/S0266467401001407
Thorne, B. L., Grimaldi, D. A., and Krishna, K. (2000). “Early fossil history of the termites,” in Termites: Evolution, Sociality, Symbioses, Ecology, eds Y. Abe, D. E. Bignell and T. Higashi (Dordrecht: Springer Netherlands), 77–93. doi: 10.1007/978-94-017-3223-9_4
Thorne, B. L., and Noirot, C. (1982). Ergatoid reproductives in Nasutitermes corniger (Motschulsky) (Isoptera: Termitidae). Int. J. Insect Morphol. Embryol. 11, 213–226. doi: 10.1016/S0020-7322(82)80006-8
Tian, L., and Zhou, X. (2014). The soldiers in societies: defense, regulation, and evolution. Int. J. Biol. Sci 10, 296–308. doi: 10.7150/ijbs.6847
Till, J. E., and McCulloch, E. A. (1961). A direct measurement of the radiation sensitivity of normal mouse bone marrow cells. Radiat. Res. 14:213. doi: 10.2307/3570892
Toth, A. L., and Rehan, S. M. (2016). Molecular evolution of insect sociality: an eco-evo-devo perspective. Annu. Rev. Entomol. 62, 419–442. doi: 10.1146/annurev-ento-031616-035601
Toubiana, W., and Khila, A. (2016). The benefits of expanding studies of trait exaggeration to hemimetabolous insects and beyond morphology. Curr. Opin. Genet. Dev. 39, 14–20. doi: 10.1016/j.gde.2016.05.015
Vieau, F. (1991). Le termite de Saintonge: Un danger pour l ‘Ouest de la France. Penn. Bed. 140,19–32.
Vieau, F. (1994). Les stades nymphaux 7 et 8 chez Reticulitermes santonensis Feytaud (Isoptera, Rhinotermitidae). Actes Colloq. Insect. Soc. 9, 61–66.
Volny, V. P., and Gordon, D. M. (2002). Genetic basis for queen-worker dimorphism in a social insect. Proc. Natl. Acad. Sci. U.S.A. 99, 6108–6111. doi: 10.1073/pnas.092066699
Watson, J., and Abbey, H. (2007). The development of reproductives in Nasutitermes exitiosus (Hill) (Isoptera: Termitidae). Austr. J. Entomol. 16, 161–164. doi: 10.1111/j.1440-6055.1977.tb00080.x
Watson, J. A. L., and Sewell, J. J. (1985). “Caste development in Mastotermes and Kalotermes: which is primitive?” in Caste Differentiation in Social Insects eds J. A. L. Watson, B. M. Okot-Kotber and C. Noirot (Oxford: Elsevier), 27–40. doi: 10.1016/B978-0-08-030783-1.50008-2
Weil, T., Rehli, M., and Korb, J. (2007). Molecular basis for the reproductive division of labour in a lower termite. BMC Genomics 8:198. doi: 10.1186/1471-2164-8-198
Weissman, I. L. (2000). Stem cells: units of development, units of regeneration, and units in evolution. Cell 100, 157–168. doi: 10.1016/S0092-8674(00)81692-X
Weissman, I. L., Anderson, D. J., and Gage, F. (2001). Stem and progenitor cells: origins, phenotypes, lineage commitments, and transdifferentiations. Annu. Rev. Cell Dev. Biol. 17, 387–403. doi: 10.1146/annurev.cellbio.17.1.387
West, S. A., Fisher, R. M., Gardner, A., and Kiers, E. T. (2015). Major evolutionary transitions in individuality. Proc. Natl. Acad. Sci. U.S.A. 112, 10112–10119. doi: 10.1073/pnas.1421402112
West-Eberhard, M. J. (2003). Developmental Plasticity and Evolution. New York, NY: Oxford University Press. doi: 10.1093/oso/9780195122343.001.0001
Whitman, D. W., and Agrawal, A. A. (2009). “What is phenotypic plasticity and why is it important?” in Phenotypic Plasticity of Insects: Mechanisms and Consequences, ed D. W. Whitman (Boca Raton, FL: CRC Press), 1–63. doi: 10.1201/b10201-2
Wu, A. M., Till, J. E., Siminovitch, L., and McCulloch, E. A. (1968). Cytological evidence for a relationship between normal hemotopoietic colony-forming cells and cells of the lymphoid system. J. Exp. Med. 127, 455–464. doi: 10.1084/jem.127.3.455
Keywords: social insects, Blattodea, caste differentiation, hemimetabolism, cellular biology, stem cells, Isoptera (termites)
Citation: Revely L, Sumner S and Eggleton P (2021) The Plasticity and Developmental Potential of Termites. Front. Ecol. Evol. 9:552624. doi: 10.3389/fevo.2021.552624
Received: 16 April 2020; Accepted: 22 January 2021;
Published: 18 February 2021.
Edited by:
Heikki Helanterä, University of Oulu, FinlandReviewed by:
Graham J. Thompson, Western University (Canada), CanadaKaren Meusemann, University of Freiburg, Germany
Copyright © 2021 Revely, Sumner and Eggleton. This is an open-access article distributed under the terms of the Creative Commons Attribution License (CC BY). The use, distribution or reproduction in other forums is permitted, provided the original author(s) and the copyright owner(s) are credited and that the original publication in this journal is cited, in accordance with accepted academic practice. No use, distribution or reproduction is permitted which does not comply with these terms.
*Correspondence: Lewis Revely, bGV3aXMucmV2ZWx5QGdtYWlsLmNvbQ==; Seirian Sumner, cy5zdW1uZXJAdWNsLmFjLnVr