- 1Naturalist Inspired Chemical Ecology (NICE) Lab, National Centre for Biological Sciences, Tata Institute of Fundamental Research, Bangalore, India
- 2SASTRA University, Thanjavur, India
- 3School of Life Sciences, University of Trans-Disciplinary Health Sciences and Technology, Bangalore, India
India is the seventh largest producer of coffee with 395,000 tons of coffee exports that earn 10 billion US dollars annually. Two varieties of coffee are grown in India, Coffea arabica (arabica) and Coffea canephora (robusta). Xylotrechus quadripes, commonly known as Coffee White Stem Borer (CWSB), is a major pest of arabica, causing yearly crop damage of 17–40 million dollars. Management strategies, over 100 years in development, have provided successful, yet inconsistent solutions due to differences in local climate, elevation, natural enemies, grower diligence, and other factors. In addition, increased pesticide use affects both pests as well as their natural enemies, which has severe negative impacts on the biodiverse regions where coffee is grown. As a result, our goal is to develop an ecology-based solution for long term management of CWSB that reduces the use of pesticides and focuses on the importance of natural enemies and native hosts. In situ behavioral experiments were performed to examine the preferences of CWSB for various local species under field conditions. We found that CWSB beetles were attracted to both healthy arabica and robusta plants, and host plant volatiles played a key role in host selection. In addition, the beetles were attracted to the leaves of these coffee plants and also two species of cut stems from common shade trees; Spathodea campanulata (nandi flame) and Grevillea robusta (silver oak). Beetles were not attracted toward cut stems of Tectona grandis (teak) or Coffea arabica. GC-EAD and EAG experiments were then performed to identify host plant volatiles for these species, and these compounds were tested in field conditions to assess their effectiveness against the known chemical attractant pheromone. We found that the CWSB was attracted to our identified host volatile blend as much as the pheromone lure, although trap catches in general were very low. Having an understanding of the behavioral ecology of this pest can form the basis for new methods that use natural attractant and repellent plants to control the pests, reduce the cost of plantation pest management, and avoid the extensive use of insecticides.
Introduction
Coffee is among the most widely grown crops worldwide. India is the seventh largest producer of coffee, growing two varieties, Coffea arabica (arabica) and Coffea canephora (robusta; Dastagiri, 2017). Exports earn India $836 million (US) annually (The Coffee Board of India, 2019). India is known for its shade grown coffee while the rest of the world generally grows coffee as a monocrop. Nearly 250,000 Indian farmers grow coffee across different states, of whom ~98% are small-scale farmers (Lee et al., 2007). The coffee plantation area in India has increased by 56% in the past 25 years (The Coffee Board of India, 2020), converting the diverse forest regions of Western Ghats (Garcia et al., 2010). The planted area of robusta has increased by 810% from 1950 to 2019 (The Coffee Board of India, 2020), replacing arabica farms that experience severe damage from pests. Coffee plantations follow a complex cropping system with different degrees of shade management (Tscharntke et al., 2011) using native and exotic primary shade trees and secondary shade trees (Suresh Kumar et al., 1992). These parameters vary from plantation to plantation depending on the coffee varieties planted and management practices followed (DaMatta, 2004). Several studies have shown that Coffee agroforests could benefit wildlife and provide avenues for conservation strategies as compared to monocultures (Robbins et al., 2015; Karanth et al., 2016; Chang et al., 2018). However, this conversion of the forest to coffee plantations has also created a habitat for Xylotrechus quadripes, a species of longhorn beetle (Cerambicidae) commonly known as Coffee White Stem Borer (CWSB).
Longhorn beetles (Coleoptera: Cerambycidae) are one of the most widespread insect families with 36,000 identified species across the world (Allison et al., 2004; Wang, 2017). The cerambycidae are also known for severely damaging forest trees as well as agricultural crops (Robert, 2017) even though several cultural and chemical control measures have been taken (Wang, 2017). One reason that farmers are shifting from arabica to robusta is the heavy damage caused by CWSB (Gana, 2016). The first incidence of CWSB attacking coffee plants was reported in early 1838 (Le Pelley, 1968). Among the six major pests of arabica (Venkatesha, 2010), CWSB now causes yearly crop damage of 17.5–40 million dollars (Hall et al., 2006; Venkatesha, 2010). The life cycle of the beetle varies between 142 and 390 days in field conditions depending on the size of the plants (Veeresh, 1995; Seetharama et al., 2005; Venkatesha and Dinesh, 2012). The female beetle lays about 50–103 eggs in deep cracks of the coffee bark (Venkatesha and Dinesh, 2012). Larvae initially feed on the bark and later instars tunnel through the cambium and wood of coffee stems, where they can obstruct plant nutrition and lead to plant death. The larval stage of CWSB averages about 120 days (Seetharama et al., 2005) tunneling deep inside the wood, thus making it hard to kill with pesticides. The beetle has two peak emergences in the year, from April to May and September to December (Veeresh, 1995; Venkatesha and Dinesh, 2012) during which the adult beetles find mates and select host plants for egg laying between the hours of 10:00 and 16:00.
Several management practices have been followed over the past 100 years to control CWSB infestation, including: shade management to repel beetles from shady areas (the beetles prefer open and well-lit areas), insecticides to kill adults and early instars, bark scrubbing and stem wrapping to prevent oviposition, mass trapping with pheromone-baited traps, and uprooting and burning infested plants (CCRI, 2003; Hall et al., 2006; Venkatesha and Dinesh, 2012; Manikandan et al., 2019). Each of these practices have shown moderate to high success rates. However, due to differences in local climate, elevation, grower diligence, lack of continuous monitoring, and the cost and manpower required to practice these methods, the stem borer has become one of the toughest pests to control. As stated, several insecticides have been applied to the coffee stems both to kill and repel CWSB, but most insecticides are not effective because the CWSB lays its eggs deep inside the stem, and non-systemic insecticides do not reach the larvae. Studies have also shown accumulation of chemical residue from systemic and contact pesticides on coffee beans (dosReis et al., 2015). Further, the use of insecticides could have an impact on non-target insects and natural enemies present in the ecosystem, which could exacerbate CWSB infestation. As such, an ecological approach that considers the natural history and host preference behavior of CWSB could lead to integrated pest management that reduces the use of pesticides.
Studies on mating behavior have shown that the beetles mate on different parts of the coffee plant (Seetharama et al., 2004), do not use visual cues for mate selection (Venkatesha et al., 1995), and both females and males attract each other using pheromones (Veeresh, 1995; Venkatesha and Dinesh, 2012). A synthetic version of the CWSB pheromone (Hall et al., 2006) is currently being used, but the pheromone lure does not attract a significant amount of beetles (Rhainds et al., 2001a; Hall et al., 2006). The beetle is endemic to India and a few Asian countries such as Myanmar, Vietnam, Thailand, China, Sri Lanka, Nepal, and Java (Le Pelley, 1968; Venkatesha and Dinesh, 2012). Although the native host is not verified, CWSB has several purported host plants: Cudrania javanensis, Ixora coccinea, Randia dumetorum, Randia spinosa, Wendlandia myriantha, Psilanthus bengalensis, Jasminum dispermum, Olea dioica, Oroxylum indicum, Gardenia sp., Rhus semiciliata, Pterocarpus marsupium, Premna pyramidata, and Trema orientalis (Veeresh, 1995; Santosh et al., 2011; Venkatesha and Dinesh, 2012) but no severe damage by the borer on these plants has been observed (Murphy et al., 2008). CWSB has also been observed on cut wood piles of teak, Tectona grandis (Mathew, 1982) and occasional gathering on other cut wood piles (Veeresh, 1995). Cut arabica stems were placed in plantations but no individuals were attracted (Veeresh, 1995). Severe CWSB infestation has been observed on arabica coffee when compared with robusta (Veeresh, 1995; Venkatesha and Dinesh, 2012), and it was found that CWSB preferred arabica to robusta (Venkatesha and Dinesh, 2012). Laboratory studies have also shown that CWSB was attracted to stems coated with larval frass and coffee sawdust (Rhainds et al., 2001b). Since CWSB has shown such preference toward certain host plants, there is a possibility of finding potential host attractants or non-host repellents from these plants.
The vast majority of chemical ecology literature on pest host attraction has occurred in temperate, open fields, and not in the unique shade-grown rainforest habitat of Indian coffee plantations. In this study, we aim to assess what cues CWSB uses to locate its hosts under field conditions. This means that we must initiate the understanding of host attraction in this unique habitat. Arabica volatiles from green tissues, branch and stem have been tested in previous studies but very few beetles were trapped (Murphy et al., 2008; Prashant, 2014). Earlier studies have focused on the survival of CWSB on different host plants in laboratory conditions (Shylesha, 1992), but not choice preference assays. No studies to date have looked at CWSB host plant selection behavior and the importance of visual vs. olfactory cues for host selection in field conditions. To this end, we performed a series of field-to-lab experiments to first understand how the beetle reaches the host plants in this habitat, which species and phenological aspects of the plant it prefers, and the potential for chemical ecology to inform ecologically-based pest management strategies in the vulnerable rainforest plantation systems of India.
Methods
Study Area and Plant Species
Experiments were performed in the coffee plantations of the Coffee Research Sub Station (CRSS), Chettali, Kodagu, Karnataka (12°22′50.34"N, 75°50′15.37"E), Central Coffee Research Institute (CCRI), Balehonnur, Chickamagalur, Karnataka (13°22′8.89”N, 75°25′24.92"E), and Belur, Somwarpet, Karnataka (12°33′14.35"N, 75°51′59.14"E). These locations exist in the major coffee growing region of India and also have reported CWSB infestations. CWSB has been observed to infest arabica plants, cut stems, and occasionally robusta (Shylesha and Veeresh, 1995; Veeresh, 1995; Rhainds et al., 2001b). Hence, we selected arabica (S795) and robusta (CxR, peridenia) plants as well as cut stems of arabica, nandi flame (Spathodea campanulata), silver oak (Grevillea robusta), and teak (Tectona grandis). Nandi flame and silver oak are commonly planted shade trees (Muthuramkumar et al., 2006; Ambinakudige and Sathish, 2009; Nath et al., 2011) on which we observed CWSB oviposition during our preliminary observations in the field. Teak was selected as it has been earlier reported to host CWSB (Venkatesha and Seetharama, 1999a). Choice assays were performed in field conditions to understand the CWSB behavior toward host plants in their natural environment followed by laboratory chemical and physiological assays and field trapping studies. The plants and stems used for the different experimental conditions were presented as they are present in their natural condition in coffee plantations in order to represent field conditions as accurately as possible.
Behavior Experiments
CWSB behavioral experiments were performed in coffee plantations by covering two coffee plants of same age (planted between 1982 and 1990) with a 4 × 3 × 4 m (l × b × h) black mesh net such that there was a minimum of 1 m above the plants for the beetle to fly freely (Figure 1A). The behavior experiments were performed during the CWSB peak emergence period: March to May and October to December (Veeresh, 1995). For choice assays between different conditions of the arabica plant (infested, no leaves, or leaf rust), we used one plant for each condition. For the arabica vs. robusta choice assays, we used either one plant of each species covered with a 3.5 × 3.5 × 4 m black mesh net (during November 2015 and May 2016) or two plants of each species covered with a 6 × 6 × 4 m black mesh net (during April 2016). Plants were chosen based on different experimental conditions: healthy (no sign of herbivore damage or disease), infested (evidence of CWSB infestation in the stem), presence of leaf rust infection, or fewer leaves (removed manually immediately prior to experimentation). A minimum of 50 cm distance was kept between condition and control plants as per plantation guidelines. Cut stems of silver oak, nandi flame, and teak used for choice assays were branches ~6 cm in diameter and 1.5 m in length without foliage. Stems were placed upright in the arena at 50 cm distance from the test coffee plant. For cut arabica stems we used the main stem without any foliage, which was ~4 cm in diameter.
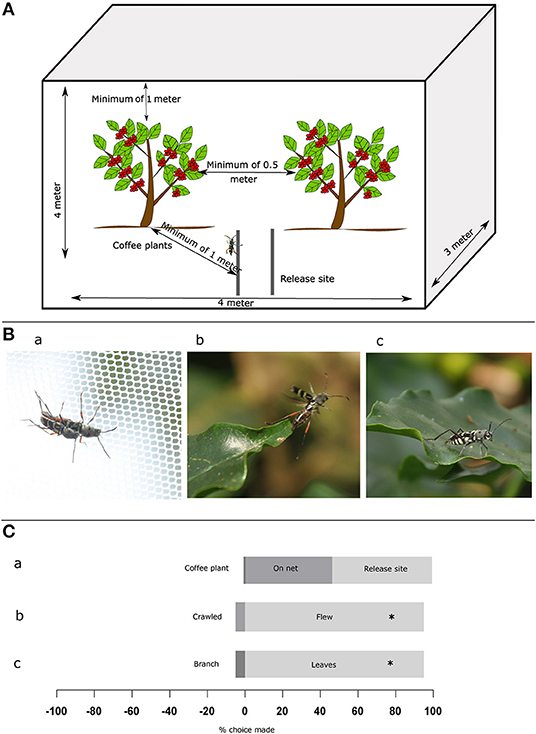
Figure 1. Experimental set-up and behavior of Coffee White Stem Borer (CWSB). (A) Top: Schematic illustration of the experimental setup. The setup is of size 4 × 3 × 4 m (l × b × h) covering two coffee plants and CWSB were released on two PVC pipes 1 m from both plants. (B) Images of CWSB. (a), mating on cage net (b), flying (c), landing on coffee leaves. (C) Mating site preference and behavioral approach of CWSB. (a), Mating location (n = 72) (b), Host plant approach (n = 38) (c), Host plant landing location (n = 37). *p < 0.005, Chi-square test. See Supplementary Table 1 for statistical details.
Beetles used in the choice assays were collected upon emergence once in the morning between 8:00 and 9:00 am and once in the evening between 5:30 and 6:30 pm from infested coffee stems kept in an ambient temperature storage room. The sex of emerged beetles was identified based on morphology as described by Venkatesha and Seetharama (1999b) and beetles were stored separately in cages (size 32 × 32 × 32 cm, model no: 4F3030, BugDorm, Taiwan) with moistened cotton for hydration. Sexually mature adult beetles of both sexes aged 1–6 days were released in batches of 10 (5 on each pipe) at the top of each pipe inside the 4 × 3 × 4 cage from two plastic PVC pipes 1 m in height kept at a distance of 0.5 from each other and 1 m from the test plants (Figure 1A). The beetles were observed from 9:30 am to 5:00 pm according to the observed emergence activity period of this species (Veeresh, 1995). Initial behavior experiments to study how the beetle approached the coffee plants, where it landed and where it mated were conducted for 15 days between 16th Oct and 12th Nov 2015. We recorded observations of 327 CWSB adults (183 males, 144 females) released in batches of 10–39 per day (average of 21–12M, 9F) at the top of each pipe. Subsequent choice assays were conducted between Oct–Dec 2015, Mar–May 2016, and Oct–Dec 2017. The number of beetles released for each choice assay is mentioned in Supplementary Table 1. Once a choice was made, the respective beetles were captured and the sex of the insect was reidentified as described. Beetles that made a choice were removed from further analyses, and beetles that did not move or make any choice during the experiment were collected and stored in a separate cage and used for the experiment the next day. Experiments were conducted for 2–6 days based on the number of beetles that approached the plant in the choice assay (minimum 18) except for the arabica and robusta experiment (12 days) since the experiment was repeated in different locations. The temperature and relative humidity of the plant was recorded every 15 min using a data logger (model no: Dt99, Mextech Technologies India Private Limited, India) and light intensity (lux) was measured using a lux meter (model no: MS 6612, Mastech, China) when each beetle approached the plant (Supplementary Figures 1, 2).
Volatile Sampling
Different volatile sampling methods were used for isolating volatiles from coffee plant leaves. Polydimethylsiloxane (PDMS) tubes, push-pull headspace sampling, and solid-phase microextraction (SPME) were used to sample the headspace of the coffee plants. We also employed solvent extraction to increase the concentration of volatiles for identification and testing with electroantennography. These four methods provide a different level of resolution in time and space, so we employed multiple techniques to provide a more comprehensive view of the system and balance costs and benefits of each technique (see Nair et al., 2018 for a more thorough discussion). For volatile sampling using PDMS tubes, volatiles were collected for 4 h by suspending three PDMS tubes above the coffee leaves using a conditioned steel wire. The PDMS tube conditioning and desorption of volatiles has been described earlier by Nordström et al. (2017). The push-pull system used for headspace sampling was modified from a protocol described earlier by Kigathi et al. (2009) and Cha et al. (2017). Briefly, we used a 12 V oil free diaphragm pump [model no: CV 07, Medicare Equipments (India) Pvt. Ltd., India] with a maximum airflow of 7 lpm and vacuum pressure of 0.75 bar powered with a portable 12 V 18 amp DC battery. Clear and cleaned vinyl tubing was used to connect between the inlet (pull) of the pump, the outlet (push), and plastic food storage bags kept in between (Zipouch fresh-n-loc, UFLEX, India) of size 32X42 cm to seal the headspace of coffee leaves on the plant. A 0.1–1 l pm air flow meter (Novatech Instruments, India) was used to control the air flow. Both the Zipouch bag and tubing was cleaned with 70% ethanol, dried by flushing with nitrogen and then kept in the oven at 80°C for 2 h to remove contamination. A hydrocarbon trap was placed before the air entered the bag to avoid hydrocarbon contamination, and two activated charcoal adsorbent tubes (model: Orbo 0424 - 20267-U, Sigma Aldrich, USA) were used near the pull end to collect the volatiles. The airflow at both the ends was held at 0.2 lpm and the sampling collection was performed for 4 h during the CWSB activity period. Once sampled, the filters were sealed and stored in a 4°C refrigerator until extraction. The filter samples were eluted using 1 ml of hexane and then concentrated to 100 μl under nitrogen and 1 μl of the extract was injected in GC for further analysis. Blank runs without the sample present were also performed to note any contamination from the setup.
For solvent extraction, fresh coffee leaves were randomly picked from healthy plants and weighed using a portable pocket weighing scale (model no: MH-series, Manali Digi Commerce Private Limited, India). Leaves were picked between 9 am and 5 pm, cut, and stored at 5 gm per sample in 30 ml borosil glass vials. The solvent (hexane) was added until leaves were completely immersed (~25 ml). The leaves were let to soak in solvent for 24 h and then removed using forceps. The bottle was capped, sealed with parafilm, and stored in a 4°C refrigerator until use. Before concentration, 10 μl of 10−3 v/v of hexyl butyrate dissolved in hexane was added as an internal standard. This chemical was chosen as it has a retention index in the range of the host volatiles found in preliminary trials, but was not observed in the samples themselves. Activated charcoal and sodium sulfate was also added to the extract to remove chlorophyll and water content by visual inspection. This process was repeated until the extract was free of chlorophyll and water. The solvent extract was then filtered using filter paper (model no: 1001110, Whatman, United Kingdom) to remove leaf particles and activated charcoal. Once this process was completed, the solvent extract was concentrated under nitrogen to ~100 μl, and 1 μl of the extract was injected into the GC. For GC-EAD, the solvent extract was made as described above from 40 gm of coffee leaves and 0.1 μl of hexyl butyrate as an internal standard.
SPME fibers were used to sample volatiles from wood of teak, nandi flame, silver oak, and arabica, as well as fresh leaves of arabica coffee collected from the University of Agriculture campus Bangalore, India. Volatiles were collected from the wood samples (>6 cm diam. x 10 cm length) 5 days after they were cut from trees at field stations at Chettali, since during our preliminary observations we found that beetles were attracted toward the cut stems of silver oak when the cut wood was 5 days and older and not when it was freshly cut. SPME was performed by placing the samples inside a cleaned and heated glass jar of size 15 × 11 cm (hxd). A 10 μl aliquot of 10−3 v/v hexyl butyrate was added as an internal standard to a circular filter paper disk (0.5 cm diameter; model no: 1001110, Whatman, United Kingdom) and placed inside the glass jar to assess the general efficacy of the extraction technique as well as to compare release rate ratios (see Insect Trapping). The system was sealed with aluminum foil. The SPME fiber used was 50/30 μm of assembly DVB/CAR/PDMS (model no: 5728-u, SUPELCO, USA) and was exposed to the sample for 30 min and then injected into the GC.
For all sampling experiments, control runs were performed without the analytes present to identify potential contaminants. For more details on our general rationale with slightly different protocols and analytes, please see (Nair et al., 2018).
Insect Rearing and Collection
The beetles used for behavior experiments were collected from infested stems stored at Central Coffee Research Sub-Station, Chettali, Kodagu, Karnataka. For EAG and GC-EAD experiments, infested coffee stems were collected from field stations at Chettali, transported to National Center for Biological Sciences (NCBS), Bangalore, and kept inside mesh cages of size (60 × 60 × 90 cm) at ambient temperature. The beetles were collected on emergence and transferred to a smaller mesh cage of size (32 × 32 × 32 cm) (model no: 4F3030, BugDorm, Taiwan) stored in an insect rearing room with a temperature between 25 and 26°C, R.H > 60% and light:dark cycle of 14:10 h. The cage sides were sprayed with water once in the morning at 9:00 and again at 16:00, and cotton soaked in water was placed inside the cage on petri dishes to keep the animals hydrated.
GC-MS
A gas chromatograph from Agilent technologies, GC 7890 B coupled with MSD 5977A Mass spectrometer was used for both GC-MS and GC-EAD experiments. For the GC system, the column used was a DB-5MS (30 m × 0.25 mm id, 0.25 μm film thickness). The front inlet temperature was kept at 275°C. The entire GC run was 23.6 min, where the column oven was kept at 40°C for 1 min, increased to 180°C at a rate of 10°C/min and finally increased to 270°C with a 5 min holding temperature in the second ramp at 25°C/min, with helium as the carrier gas at a flow rate of 1 ml/min. The FID temperature was kept at 300°C, the GC-MS interface at 250°C, the electron impact ionization source at 230°C, and the quadrupole temperature at 150°C, respectively. Ionization was performed in electron impact mode with ionization energy of 70 eV. The response peak compounds were identified using Agilent MassHunter Workstation software B.07.00. Qualitative analysis was assessed using the following four methods, similar to Nordström et al. (2017) and Nair et al. (2018): (1). Assessing the mass spectral peaks themselves, (2). Comparing mass spectral data of the unknown peaks with library spectra [National Institute of Standards and Technology (NIST)], (3). Comparing peak relative retention index (using C8–C40 hydrocarbons alkanes standard, Sigma Aldrich, Bangalore, India), and (4). Comparing to authentic standards.
EAD/EAG
GC-EAD experiments were performed to initially identify the host volatiles detected by the insect olfactory system and then EAG experiments were performed to assess the sensitivity of the beetles toward the identified volatile compounds. For GC-EAD, a Gerstel odor detection port ODP3 (Olfactory Detection Port; Gerstel, Germany) was connected to the column using a Capillary Flow Technology 3-way splitter with makeup gas (Agilent G3183-60500, Agilent Technologies, USA). The splitter was kept in the ratio of 1:1:5 for GC-MS: FID: EAD. The ODP transfer line was kept at 250°C and the ODP exit port at 150°C. 0.5 lpm humidified airflow was added at the ODP head and another 0.5 lpm was added at the exit nozzle. The GC-EAD files were analyzed using GC/EAD32 version 4.3 by Syntech (Germany) for insect responses and retention times of responses.
Healthy and actively moving insects, 3–7 days old, were used for EAD and EAG experiments. Live whole beetles were placed inside 1 ml pipette tips and sealed at the back using dental wax to reduce movement. A small hole was made in the pipette tip right above the thorax to ground the insect preparation using a minutian pin. A silver wire of diameter 1 mm and glass micropipette of diameter 1.5 × 1.1 mm (outer x inner diameter; model no: B150-110-10, Sutter Instrument, USA) filled with hemolymph ringer solution (see Olsson and Hansson, 2013) was used as a recording electrode inserted over the antennal tip. Control, blank, and chemical stimuli were used to test antennal sensitivity. For EAG, the stimuli were presented using a custom built delivery system at 0.5 s pulse at 0.5 lpm as described in (Getahun et al., 2012; Tait et al., 2016). The EAG data acquisition was performed using EAG2000 (Ockenfels Syntech, DE) and a Syntech intelligent data acquisition controller (IDAC-4). The chemicals used for EAG studies were dissolved in mineral oil (Sigma Aldrich, India) in serial dilutions ranging from 10−1 to 10−6 v/v. Stimuli were prepared and delivered in Pasteur pipettes as in Getahun et al. (2012) and Ghaninia et al. (2014).
Chemicals
All compounds used as standards for identification, for EAG experiments, and for making the volatile blend of coffee leaves and cut stems were purchased at highest purity available (purity >90%) from vendors listed in Table 1. (E)-β-ocimene (mixture of isomers), CAS 13877-91-3, was purchased from Sigma-Aldrich, India at >90% purity.
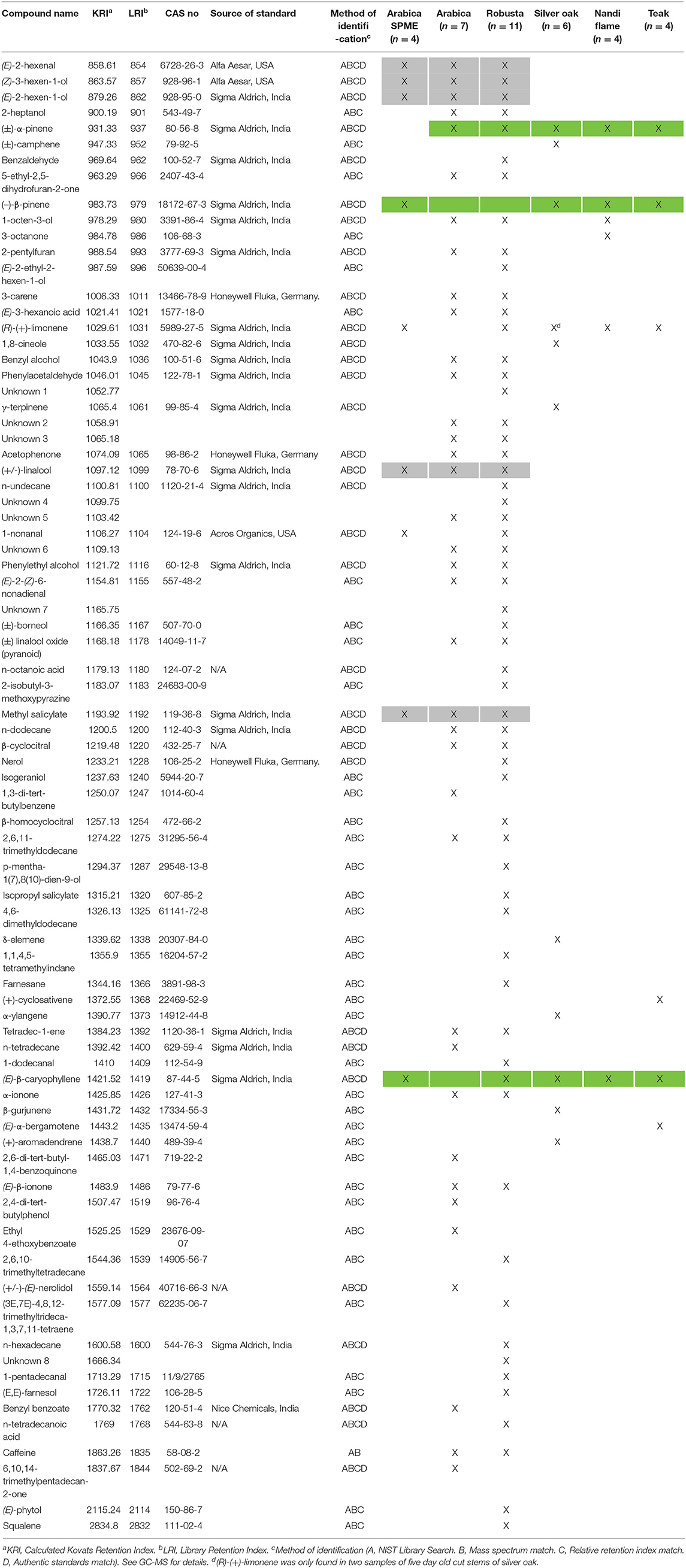
Table 1. List of volatile compounds of arabica coffee (SPME), arabica coffee (Solvent extraction), robusta coffee (Solvent extraction), cut stems of silver oak, nandi flame and teak (SPME).
Insect Trapping
Insect trapping experiments were performed to assess the attraction of CWSB toward the identified host volatile compounds in field settings as compared to the currently used pheromone lure. The host volatile blends used in the traps were created based on the results from GC-EAD, EAG, SPME, and solvent extractions and the ratio was modified empirically to match the average relative area ratios of the identified compounds found in the leaf and cut wood extracts [Supplementary Tables 2–4; see Nordström et al. (2017) for a similar process]. The blend release rate ratio was assessed by adding 1 ml of each blend in mineral oil to 2 cotton swabs of size 4 × 0.5 cm (hxr) in a heavy duty reclosable bag of size 2 × 3 in (Cousin Corporation of America, USA) and placing it in outdoor field conditions. SPME was performed on the blends after approximately every 2 h from 9:00 to 18:00 h to measure the release rate consistency with 30 min exposure time (time calibrated to avoid saturation) by placing the bag inside a glass jar of size (15 × 11 cm) similar to the one used for cut wood volatile sampling. Files were analyzed using Agilent MassHunter Workstation software B.07.02.1938 and the relative area was compared with the relative area of leaf and cut wood extracts so that it matched close to the relative ratios (note, not total abundance) of the plant release rate. One ml of each blend was used.
For field studies, we tested three blends: a coffee leaf blend based on volatiles of coffee leaves (“LB”), wood blend 1 (“WB1”) and wood blend 2 (“WB2”), both based on the volatiles of cut stems of silver oak and nandi flame. The 1 ml aliquot of LB contained 0.18 μl of (E)-2-hexen-1-ol, 0.54 μl of (Z)-3-hexen-1-ol, 0.11 μl of (E)-2-hexenal, and 0.15 μl of (±)-linalool in 1,000 μl (v/v) of mineral oil. WB1 contained 3.4 μl of (±)-α-pinene, 5.6 μl of (–)-β-pinene and 9.09 μl of (E)-β-caryophyllene in 1,000 μl (v/v) of mineral oil. WB2 contained 3.2 μl of (±)-α-pinene, 5.3 μl of (–)-β-pinene, 8.6 μl of (R)-(+)-limonene and 47.6 μl of (E)-β-caryophyllene in 1,000 μl (v/v) of mineral oil. The blends were added to cotton swabs in bags as described and then placed in cross-vane panel traps (SKU AST0030-G, Alpha Scents Inc., USA) coated with fluoropolymer resin (Insect-a-slip Insect Barrier—Fluon, BioQuip products, USA) to increase trapping efficiency (Graham et al., 2010; Graham and Poland, 2012). Pheromone lures (Pest control (India) Pvt. Ltd, India) currently being used in coffee plantations for trapping CWSB were also used. All traps were placed in two locations 100 m apart from each other. Each location contained 7 traps placed 20 m apart (Supplementary Figure 3A) and experiments were repeated for 106 trap days over two CWSB emergence seasons during October-December 2018 and March-May 2019. The seven blends used for the traps were: LB + pheromone, WB1 alone, WB1 + pheromone, WB2 alone, WB2 + pheromone, pheromone alone, and mineral oil (control). We did not test LB without the pheromone because in preliminary tests in the cage setup with the LB alone we did not observe any beetles approaching the traps (beetles released = 55, days observed = 6). The lures were placed in the center of the panel traps (Supplementary Figure 3B) and fresh blends were added every day in the morning before 9:00 am. The collection trap was filled with 30% ethanol to kill and preserve the insects trapped. The collection trap (Supplementary Figure 3C) was emptied twice a week and the CWSB trapped were stored in 50 ml falcon tubes and identified based on morphology as described by Sangamesh (2015). Voucher specimens of CWSB (ID: UASB02023120), Xylotrechus smei (ID:UASB02023121) and Demonax balyi (ID:UASB02023123) have been deposited at the Department of Entomology, UAS, Bangalore.
Statistical Analysis
We used Chi-square analysis for behavior experiments and two-choice bioassays. The choice assays for each experiment were performed for a maximum of 5 days and the number of beetles (N) that made a choice were considered for statistical analysis (n = 18–69). Cohen's w, an effect size index was calculated by dividing the chi-square value (X2) by the number of samples (N) and then taking the square root (Cohen, 1988). Regression lines for EAG were fit using the non-linear regression method (GraphPad Prism Software). The EAG data was tested for homoscedasticity (GraphPad Prism Software) and significance was tested using Mann-Whitney test (GraphPad Prism Software). GraphPad Prism Software (USA) and Inkscape software (Inkscapes's contributors) were used to make the figures. See Supplementary Table 1 for statistical details.
Results
CWSB Approaches Arabica Coffee Plants in Flight and Prefers to Land on the Coffee Leaves
We first looked at whether the beetles exhibited mating-site preference toward arabica coffee plants (Figure 1; see Supplementary Table 1 for all statistical details). We found that 1% mating occurred on arabica coffee plants, 53% on cage nets (Figure 1Ba) and 46% at the release site (Figure 1Ca, N = 72). This indicates that beetles were willing to mate at alternative locations where they find each other (perhaps via pheromone cues; Venkatesha et al., 1995, Veeresh, 1995) and the host plant does not seem to be imperative for mating success. Secondly, we examined how the beetles approached the coffee plants. We found that 95% (Figure 1Cb), N = 37, p < 0.001, chi-square) of the beetles that approached plants flew to the plant rather than walked. This suggested that the cues beetles are using to recognize hosts must be perceived in flight (Figure 1Bb). Thirdly, we examined where the beetles preferred to land on coffee plants. In this case, we found that 97% (Figure 1Cc, N = 38, p < 0.001, chi-square) of the beetles preferred coffee leaves (Figure 1Bc), suggesting that the leaves are important for host selection, even though egg-laying is performed on the stem. We thus followed these experiments with additional choice assays to determine the extent of this preference, if any.
CWSB Prefers Healthy Arabica and Robusta Coffee Plants With Leaves, as Well as Cut Stems of Silver Oak and Nandi Flame
Once we determined how the beetles approached the coffee plants, we then examined the host preference of the beetles toward the two major coffee varieties grown in India —Coffea arabica (arabica) and Coffea canephora (robusta), as well as cut wood of different trees commonly found in the plantations (Figure 2A; see Supplementary Table 1 for statistical details). Arabica exhibits the highest infestation rates, and hence we looked at what type of arabica plant—healthy with leaves, no leaves, infested, or low leaf rust—the beetles preferred. First we found that 78% of the beetles preferred coffee plants with leaves over no leaves (Figure 2Aa; N = 18, p = 0.018, chi-square). This suggests cues from leaves are important for host selection, although we note that some of this difference could be due to the reduced surface area of the defoliated plant. We then found that 70% of the beetles preferred healthy coffee plants over infested coffee plants (Figure 2Ab; N = 23, p = 0.033, chi-square). No preference was observed between higher and lower levels of leaf rust on coffee plants (Figure 2Ac; N = 19, p = 0.491, chi-square). This suggests that the beetles preferred uninfested coffee plants over infested plants, but that leaf rust did not inhibit preference. We also examined host preference between the two varieties of coffee and found that 88% of the beetles preferred robusta over arabica (Figure 2Ad; N = 69, p < 0.001, chi-square). This is notable as historically robusta is considered less attractive than arabica (Venkatesha and Dinesh, 2012).
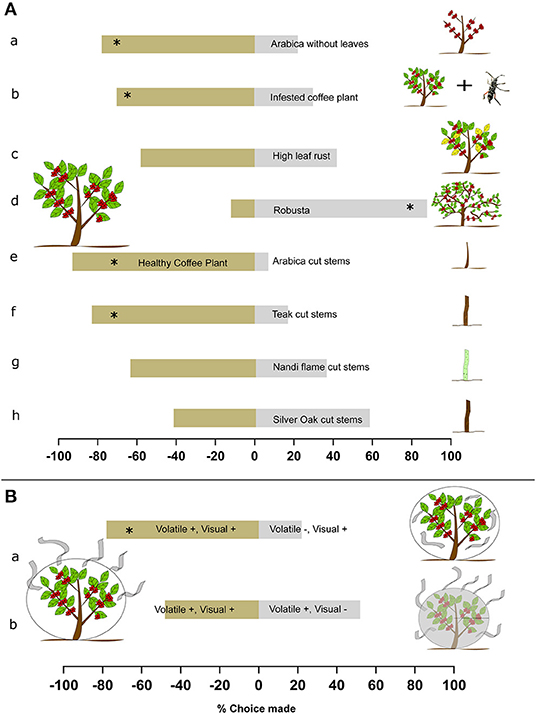
Figure 2. Host preference and cues used by Coffee White Stem Borer (CWSB). (A) Top: CWSB host preference in a 2-choice behavioral bioassay. (a), Healthy coffee plant with leaves vs. healthy coffee plants without leaves (n = 18) (b), Healthy coffee plant vs. infested coffee plant (Coffea arabica) (n = 22) (c), Healthy coffee plant vs. high leaf rust coffee plant (n = 19) (d), Healthy coffee plant vs. healthy robusta (Coffea canephora, n = 69) (e), Healthy coffee plant vs. arabica cut stem (n = 23) (f), Healthy coffee plant vs. teak (Tectona grandis) cut stem (n = 29) (g), Healthy coffee plant vs. nandi flame (Spathodea campanulata) cut stem (n = 25) (h), Healthy coffee plant (Coffea arabica) vs. silver oak (Grevillea robusta) cut stem (n = 26). (B) Cues used by CWSB for host selection. (a), coffee plants (Coffea arabica) with both visual and volatile cues present vs. visual cues present but volatile cues reduced (n = 27). (b), Coffee plants with both visual and volatile cues present vs. volatile cues present but visual cues reduced (n = 21). *p < 0.05, chi-square test. See Supplementary Table 1 for statistical details.
In preliminary field observations, beetles were also observed gathering on freshly uprooted and cut arabica stems and cut wooden poles of various species which were chopped and left in the field for 3–12 days. We thus explored whether cut wood might act as an alternative host to coffee plants, and if this response is species-specific or generic to fresh cut wood as a whole. We tested cut wood of four species: teak, nandi flame, silver oak and arabica coffee (see section Study Area and Plant Species). We found that the beetles did not prefer cut arabica or cut teak stems to a healthy coffee plant. A total of 83% preferred healthy arabica plant over cut arabica stems (Figure 2Ae; N = 23, p = 0.001, Beetles released = 121, chi-square) and 93% of the beetles preferred a healthy coffee plant over cut teak stems (Figure 2Af; N = 29, p < 0.001, chi-square). We note that the increased surface area of the live plant could have contributed to this preference. Nevertheless, for cut nandi flame and silver oak stems, 36% approached nandi flame (Figure 2Ag; N = 25, p = 0.161, chi-square) and 56% approached cut silver oak stems (Figure 2Ah; N = 32, p = 0.479, chi-square) when given a choice against healthy arabica plants. This suggests that cut stems of nandi flame and silver oak have cues that are also attractive to CWSB when compared to coffee plants despite a difference in surface area. However, this preference cannot be extended to cut wood in general, as teak and arabica stems were not preferred against coffee plants.
Plant Volatiles Are Important for Host Plant Selection
We next examined what long-distance cues the beetles use to select host plants (Figure 2B, see Supplementary Table 1 for statistical details). We first tested for the importance of combined olfactory and visual cues by covering one coffee plant with a transparent plastic sheet to permit visual cues but prevent escape of plant volatiles and covering the other coffee plant with a plastic sheet ventilated with several holes to allow volatiles to escape. The two sheets were swapped between the plants every day before the experiment to avoid side bias. Seventy-eight percentage of the beetles preferred coffee plants where both volatiles and visual cues were present (Figure 2Ba; N = 27, p = 0.003, chi-square). This suggests that volatiles released from the plant are important for host location. We next altered the visual cues by covering one of the coffee plants with a black mesh net over the plastic net with holes that obscured reflected light from the plants, whereas the other coffee plant was covered only with the clear plastic sheet with holes, similar to Raguso and Willis (2005). In this experiment, the beetles preferred both plants equally (Figure 2Bb; N = 21, p = 0.241, chi-square) suggesting that visual cues are not of primary importance for host choice.
Attractive Hosts Exhibit Several Common Volatile Compounds Between Species
From our choice assay experiment, we identified both healthy coffee plant leaves and two cut wood species (silver oak and nandi flame) that were attractive to CWSB. We also determined that volatile cues were of primary importance for choosing plants. Hence, we wanted to identify the volatiles released by these attractants. We also examined the volatiles of cut stems of teak and arabica since they were not as attractive as healthy coffee plants. We identified 37 (4 unknown) and 59 compounds (8 unknown) from solvent extracts of arabica (N = 7) and robusta (N = 11) varieties of coffee leaves (Table 1). From SPME of arabica leaves (N = 4), we identified 5 compounds that were also present in the solvent extraction of both arabica and robusta (Table 1, highlighted in gray in Table 1). The five common compounds were (E)-2-hexenal, (Z)-3-hexen-1-ol, (E)-2-hexen-1-ol, (±)-linalool, and methyl salicylate. SPME was performed on cut stems of silver oak, nandi flame, Arabica, and teak. Nine compounds were identified from silver oak (N = 6) and six compounds were identified from nandi flame and teak (N = 4) with (±)-α-pinene, (–)-β-pinene, and (E)-β-caryophyllene consistently present in all cut wood samples. (R)-(+)-Limonene was also present in all cut wood samples, but while in silver oak cut stems it was found in all freshly-cut wood samples (n = 3), this compound was only observed in 20% of the attractive 5 days or older cuts (n = 2/10). Camphene, eucalyptol, (+)-aromadendrene, α-ylangene, γ-terpinene, and β-gurjunene were present only in silver oak. 1-octen-3-ol, 3-octanone, and 3 unknown compounds were present only in nandi flame. (+)-cyclosativene and (E)-α-bergamotene were present only in teak (Table 1).
CWSB Can Detect Several Compounds From Attractive Hosts
GC-EAD experiments were conducted to identify which volatiles were detected by the beetle olfactory system (Figures 3Aa,b). A total of 15 males and 23 females were tested with hexane extracts of coffee leaves and the response of 6 males and 20 females were analyzed. The beetle responded to 18 unique compounds (Supplementary Table 5) from the solvent extracts of arabica and robusta (Table 1). A total of 12 compounds could be identified: (Z)-3-hexen-1-ol, acetophenone, (E)-2-hexen-1-ol, (E)-2-hexenal, n-dodecane, methyl salicylate, tetradec-1-ene, benzyl alcohol, 2,6,11-trimethyldodecane, caffeine, 5-ethyl-2,5-dihydrofuran-2-one, and (E)-3-hexanoic acid (Figure 3B). The female antennae responded to 18 compounds and the male antennae responded to 8 compounds (Supplementary Table 5).
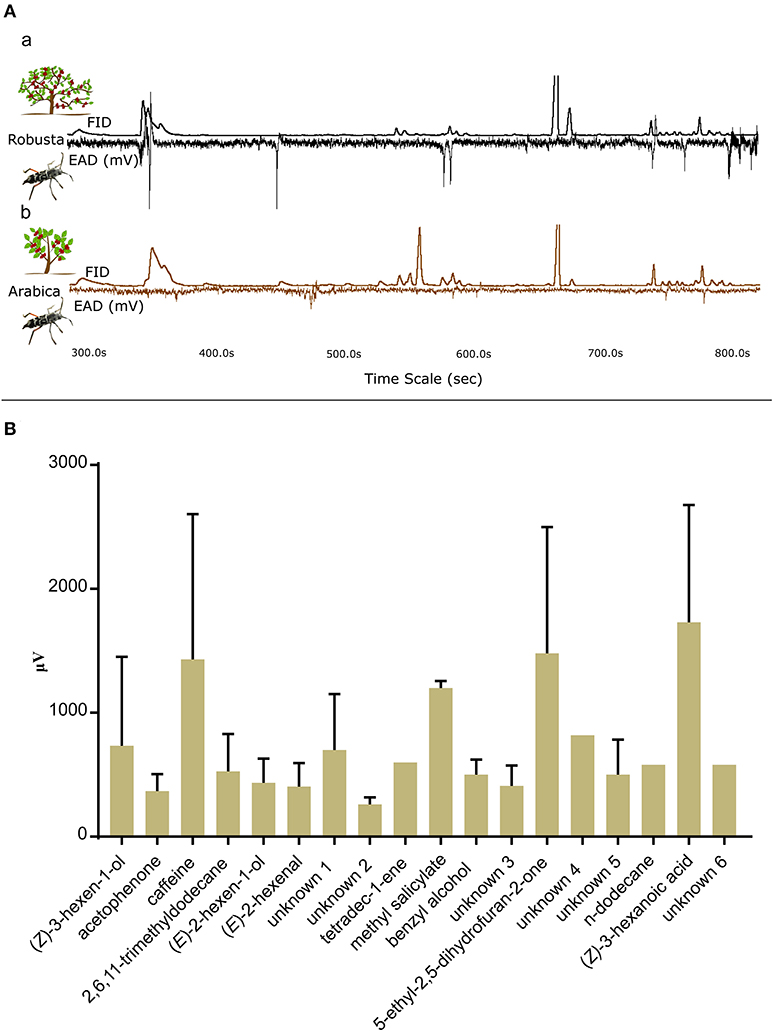
Figure 3. Gas Chromatography—electroantennographic detection (GC-EAD) responses of CWSB. (A) GC-EAD traces with Flame Ionization Detector (FID) peaks and electroantennographic (EAD) responses of CWSB for hexane extracts of robusta (a) and arabica (b). (B) GC-EAD responses of male and female CWSB for different compounds of arabica and robusta hexane leaf extracts identified in Table 1 (n values of male and female for each compound listed in Supplementary Table 5). μV, microVolt; FID, Flame Ionization Detector; EAG, Electroantennography.
CWSB Exhibits Differential Sensitivity Toward Identified Host Volatile Compounds
Following GC-EAD experiments, EAG experiments were performed to assess antennal sensitivity toward the identified chemicals (Figure 4). Eight compounds were selected from coffee leaf extracts to which the beetle gave response during GC-EAD (based on the availability of synthetic standards): (Z)-3-hexen-ol, (E)-2-hexen-al, (E)-2-hexen-ol, acetophenone, n-dodecane, methyl salicylate, benzyl alcohol, and tetradec-1-ene. Two compounds present in SPME analysis of coffee leaves were tested: (±)-linalool and 1-nonanal. An additional six compounds present in cut wood of silver oak, nandi flame and teak, were also tested: (±)-α-pinene, (–)-β-pinene, (E)-β-caryophyllene, γ-terpinene, (R)-(+)-limonene, and (E)-β-ocimene (the last two compounds were only found in fresh stems of silver oak). Male CWSB antennae exhibited significant responses (Mann-Whitney test) as compared to the solvent control for (E)-2-hexenol (N = 5, p = 0.0041) and benzyl alcohol (n = 10, p = 0.0042) at 10−3 v/v. (E)-2-Hexenal (n = 5, p = 0.0159), (±)-linalool (n = 5, p = 0.0317) and acetophenone (n = 5, p = 0.007), elicited responses at a threshold of 10−2 v/v, and (Z)-3-hexenol (n = 17, p = 0.0079), (±)-α-pinene (n = 5, p = 0.01), (–)-β-pinene (n = 5, p = 0.0159), and (E)-β-caryophyllene (n = 5, p = 0.0159) at 10−1 v/v. No significant response from male antennae was observed at any tested concentration for methyl salicylate, 1-nonanal, n-dodecane, γ-terpinene, (R)-(+)-limonene, and tetradec-1-ene (n = 5). Female CWSB antennae responded significantly to seven compounds: (Z)-3-hexenol (n = 11, p = 0.0336) and (±)-linalool (n = 10, p = 0.0003) at 10−3 v/v, and (±)-α-pinene (n = 17, p = 0.0001), (R)-(+)-limonene (n = 8, p = 0.007), (E)-β-ocimene (n = 10, p = 0.0185), (E)-2-hexenol (n = 5, p = 0.0317), and (E)-2-hexenal (n = 5, p = 0.0317) at 10−2 v/v. No significant response from female beetles was observed to any concentration of methyl salicylate, 1-nonanal, n-dodecane, acetophenone, benzyl alcohol, γ-terpinene, (–)-β-pinene, tetradec-1-ene (n = 2, Mann-Whitney test), and (E)-β-caryophyllene (n = 5).
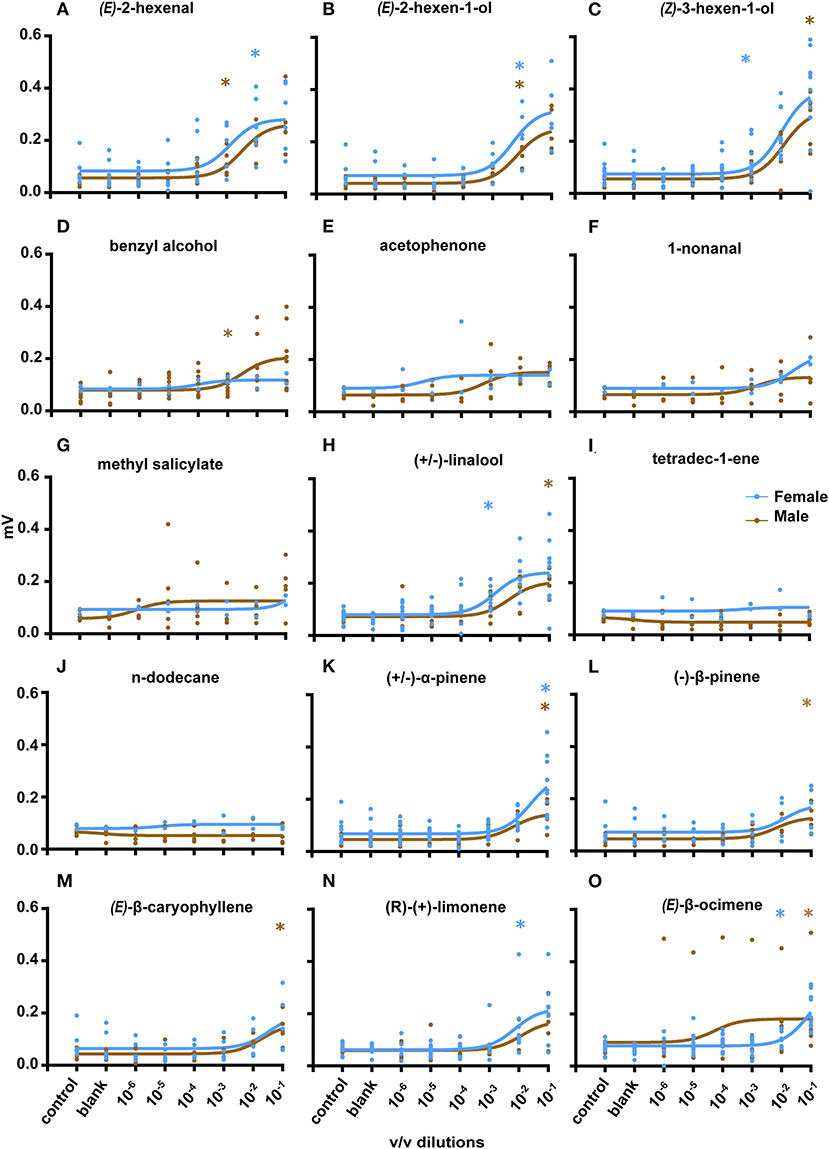
Figure 4. Electroantennogram recordings and concentration response curves. Response of male CWSB (Brown) and female CWSB (Blue) antennae to different coffee leaf volatiles (A–J) and cut stems (K–O). (A) (E)-2-hexenal (male, n = 5, female, n = 5) (B) (E)-2-hexen-1-ol (male, n = 5, female, n = 6) (C) (Z)-3-hexen-1-ol (male, n = 6, female, n = 11) (D) Benzyl alcohol (male, n = 9, female, n = 2) (E) Acetophenone (male, n = 6, female, n = 2) (F) 1-nonanal (male, n = 4, female, n = 2) (G) methyl salicylate (male, n = 5, female, n = 2) (H) (±)-linalool (male, n = 5, female, n = 10) (I) tetradec-1-ene (male, n = 5, female, n = 2) (J) n-dodecane (male, n = 5, female, n = 2) (K) (±)-α-pinene (Male, n = 5, Female, n = 12) (L) (–)-β-pinene (male, n = 5, female, n = 5) (M) (E)-β-caryophyllene (male, n = 5, female, n = 5) (N) (R)-(+)-limonene (male, n = 5, female, n = 8) (O) (E)-β-ocimene (male, n = 6, female, n = 10). *p < 0.05 at first significant test concentration, Mann-Whitney test. Non-linear regression (curve fit, brown, blue) was used for all compounds.
Host Volatile Lures Attracted as Many Beetles as Pheromone Lures in Plantation Trap Analyses
Once the compounds were identified, three types of blends were made based on EAG/EAD active compounds that were also present in leaves or cut wood attractive to CWSB. The first blend was made based on the coffee leaf volatiles and the other two blends were made based on cut wood volatiles of silver oak and nandi flame. The leaf blend (LB) was made using 4 compounds: (Z)-3-hexen-1-ol, (E)-2-hexen-1-ol, (E)-2-hexen-1-al, and (±)-linalool. Cut wood blend 1 (WB1) had 3 compounds [(±)-α-pinene, (–)-β-pinene, (E)-β-caryophyllene] common between nandi flame and silver oak. Cut wood blend 2 (WB2) contained 4 compounds [(±)-α-pinene, (–)-β-pinene, (E)-β-caryophyllene, (R)-(+)-limonene] based on the volatiles of nandi flame and silver oak. (E)-β-ocimene was not included since the compound was present only in young freshly cut silver oak stems and not in 5 day old stems that attracted beetles during preliminary field observations. We trapped three species of longhorn beetles (Figure 5; see Supplementary Table 1 for statistical details): Demonax balyi, Xylotrechus smei, and Xylotrechus quadripes (CWSB). A total of 36 D. balyi, 19 X. smei and 18 X. quadripes were captured over 106 trap days across two seasons during Nov-Dec 2018 and March-May 2019 (Figure 5). Note that while species was noted, sex could not be confidently identified after collection due to rapid tissue degradation. A single CWSB was trapped in control, 5 in pheromone, 5 in WB1, 5 in WB1 + pheromone, 2 in LB + pheromone and no beetles were trapped in WB2 or the WB2 + pheromone lure (Figure 5; n = 18, p = 0.041, chi-square). However, while the Chi-square test indicated there was a treatment effect, i.e., that total CWSB catches per treatment differed from a 1:1:1:1:1:1:1 ratio, due to the low trap catch numbers, further statistical inferences would be unreliable.
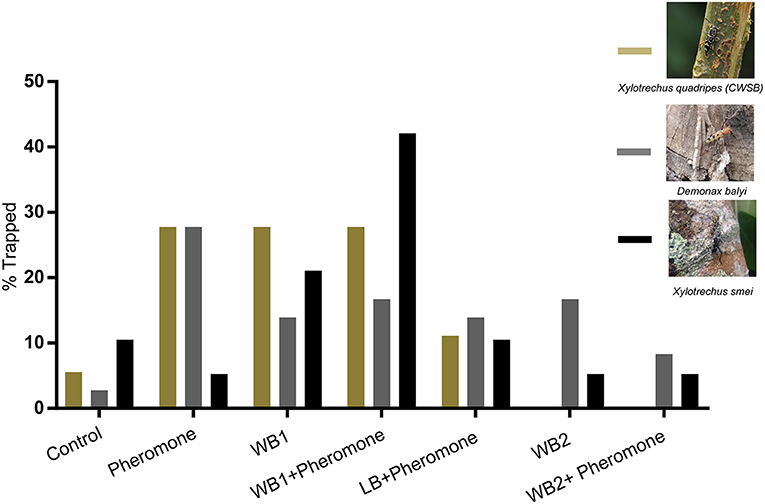
Figure 5. CWSB attraction toward host volatile blends in field conditions. Percentage of longhorn beetles Xylotrechus quadripes (CWSB; n = 18, Olive green), Xylotrechus smei (n = 19, Black), and Demonax balyi (n = 36, Dark gray) trapped in black panel traps with volatiles. See Supplementary Table 1 for statistical details. Wood blend 1 (WB1): blend of (±)-α-pinene, (–)-β-pinene, and (E)-β-caryophyllene. Wood Blend 2 (WB2): blend of (±)-α-pinene, (–)-β-pinene, (E)-β-caryophyllene, and (R)-(+)-limonene. Leaf Blend (LB): blend of (E)-2-hexenal, (E)-2-hexen-1-ol, (Z)-3-hexen-1-ol, and (±)-linalool.
Discussion
Coffee agroforests have a complex structure with understory, mid-level and high-level canopy trees rich in animal diversity (Caudill et al., 2014; Robbins et al., 2015; Karanth et al., 2016; Chang et al., 2018). The use of chemical control to combat CWSB infestation will have an impact on the biodiversity in the ecosystem (Mone et al., 2014). Understanding the behavior of CWSB can help us to identify natural attractants and repellents to monitor, trap or repel the pest and form more ecologically-based management practices that require less manpower and effort while minimizing the use of pesticides. In our study, we found using in-plantation field behavioral assays that CWSB approaches coffee plants directly in flight and lands on coffee leaves rather than the stem where it eventually lays its eggs. Second, we found that CWSB exhibits attraction to healthy arabica and robusta plants as well as cut stems of silver oak and nandi flame. Furthermore, we observed that volatile cues are important for this choice and demonstrate that the olfactory system of CWSB can detect several volatiles identified from these potential hosts. Finally, we found that identified host volatile compounds of cut stems were as attractive as the pheromone lures currently being used to trap and monitor CWSB beetles. Understanding the ecology, behavior, host preference, and chemical ecology of this longhorn beetle could help us in planning better management practices.
In recent years, Indian coffee farmers have shifted from arabica to robusta because CWSB infests arabica severely and does not appear to infest robusta, or perhaps the beetle doesn't survive in robusta (Veeresh, 1995). Further, the shift in cropping from arabica to robusta has substantially altered the coffee agroforest: native, old-growth shade trees have been replaced by monocultures of the exotic Grevillea robusta (silver oak), and remaining native trees are heavily pruned to increase light availability. In our study, we found that CWSB is attracted to both varieties and uses the volatiles released by the host plants to locate them. Furthermore, in our choice assay the beetles preferred to approach robusta over arabica. This first suggests that robusta is attractive to CWSB and is therefore at risk of infestation. Indeed, recent reports have shown evidence of infestation in robusta, although not at levels seen in arabica (Gana, 2016). Hence, a complete shift in cropping from arabica to robusta can potentially face a similar infestation problem unless different management practices are followed. Second, while it should not be a total replacement for arabica, the lower infestation rates of CWSB in robusta (Veeresh, 1995; Venkatesha and Dinesh, 2012), indicate that it could serve a suitable trap host plant if organized strategically such as in a push-pull management system (Khan et al., 2016).
Apart from a preference for arabica and robusta plants, we found that cut wood stems of two species were as attractive as arabica plants despite the difference in surface area between a whole coffee plant and a cut stem. However, when we tested synthetic blends of host volatiles from arabica leaves and cut wood in field conditions along with the pheromone lure currently being used to control CWSB, we trapped very few beetles. This suggests that the pheromone and host volatile traps are not efficient for trapping CWSB in field conditions. Traps for cerambycids in forests have always been a challenge considering the complex system in which they thrive and also because beetles spend most of their time inside tree stems (Haack et al., 2010; Schowalter, 2013; Hanks and Millar, 2016). Pheromone and kairomone traps have been utilized for several years and they have been effective or ineffective depending on the aggregation behavior of the cerambycid species of interest (Allison et al., 2004; Catry et al., 2017; Millar and Hanks, 2017). We note that, while low, the trap catches in our study were similar to previous studies (0.1 beetles/trap day/replicate in our study vs. 0.14 in Hall et al., 2006), and the similarity in trap catches with our host volatile blends also suggests that host volatiles were as effective as the established pheromone lure in trapping CWSB.
Despite the low trap catches, our study indicates the importance of volatile cues for host location in CWSB, and identifies electrophysiologically and behaviorally active volatiles from plants found in the region that could be tested in further studies. Interestingly, these compounds are common volatiles found in a large number of plant species. Several studies have shown that insects have evolved to rely not on single volatiles, but on blends released in specific ratios and mixtures in an “odorscape” particular to their host of interest, for which the insect has evolved a unique sensory repertoire (see Conchou et al., 2019 for a recent review). Here, we show that the olfactory system of CWSB is capable of detecting several of these volatiles. An examination of the volatile profile of attractants in this study (coffee, silver oak and nandi flame) revealed three key compounds [(±)-α-pinene, (-)-β-pinene and (E)-β-caryophyllene, highlighted in light green in Table 1] that were common among them, and were also detected by the beetle antenna in electroantennographic analyses. Even though these three compounds were also present in teak, cut wood from teak was not preferred by the beetles, perhaps due to the presence of other volatile compounds such as (+)-cyclosativene, (E)-α-bergamotene, or others. Further, (R)-(+)-limonene was primarily found in freshly cut silver oak stems and not in the older, attractive stems, and the cut wood blend with this compound added to (WB2) also did not attract any CWSB in our field trapping study.
Understanding attractant and deterrent volatile cues could help us to identify potential host and repellent plants to be intercropped or used as border crops (Wang, 2017) in coffee plantations. The current organization of coffee plantations places coffee plants in rows with intermittent secondary and primary shade trees. We could potentially reorganize the plantation by including alternative host plants as border crops and repellent plants between coffee plants for an effective long-term Push-Pull ecology-based solution with minimized usage of pesticides. Push-Pull technology is an ecology-based integrated pest management solution developed for the agricultural cropping system in Africa (Khan et al., 2016) and has been practiced extensively. Further studies could focus on the CWSB relationship with native host plants that attract CWSB (attractants), natural enemies which kill and deter CWSB (repellents), and, tree diversity and forest edges which reduce CWSB infestation. Our study provides insights on plant species and cues that could be used toward this effort, and we presented these species in our behavioral assays as they are found in the coffee plantation, supporting their use for further analyses. If a method of modifying the agroforests including several parameters such as alternate hosts, attractants and repellents reduces CWSB infestation, this method could be adapted for other agroforestry crops facing similar issues.
Our comprehensive chemo-ecological analysis allowed us to understand the role of chemical cues in CWSB behavior in this unique habitat, and also to develop potential tools for an integrated pest management strategy employing alternate hosts. A method utilizing the properties of the pest's natural behavioral ecology can revolutionize the way insect infestation is managed in agroforests and it will also reduce the amount of insecticides that are being used. This can also encourage the farmers to plant native trees for secondary and primary shade cover aiding the diversity of other animals and plants in agroforests.
Data Availability Statement
The original contributions presented in the study are included in the article/Supplementary Material, further inquiries can be directed to the corresponding author/s.
Author Contributions
SRajus and SO conceived the idea, planned the experiments, analyzed and interpreted the data, and wrote the manuscript. SB conceived the idea, planned the experiments, and performed the behavior and choice-assay experiments. HK performed behavior and choice-assay experiments. SRajus collected volatile samples, performed GC-EAD and EAG, identified the compounds, and carried out all behavior, choice assay, and trap experiments. SRao performed chemical analysis and identification. All authors contributed to the article and approved the submitted version.
Funding
This work was supported by NCBS-TIFR and funded by a grant from the Coffee Board of India and a SERB Ramanujan Fellowship to SO, including the Department of Atomic Energy, Government of India, under project no. 12-R&D-TFR-5.04-0800 and 12-R&D-TFR-5.04-0900.
Conflict of Interest
The authors declare that the research was conducted in the absence of any commercial or financial relationships that could be construed as a potential conflict of interest.
Acknowledgments
We acknowledge Coffee Research Sub Station (Chettali, India), Central Coffee Research Institute (Balehonnur, India) and Tata Coffee (Coorg, India) for providing insects and field sites for experiments. We thank coffee planters Mr. Umesh and Mr. Murali for providing us with field sites for experiments. We thank Dr. Kurian Raphael and the many scientists from CRSS, Chettali and CCRI, Balehonnur for discussions on CWSB. We thank Pavan Kumar Kaushik at National Center for Biological Sciences (Bangalore, India) for discussions and initial help with EAG analysis, and VS Pragadheesh at CSIR - CIMAP (Bangalore, India) for discussions and initial help with GCMS analysis. We also thank Yeshwanth H.M from department of Entomology, UAS, Bangalore for insect identification.
Supplementary Material
The Supplementary Material for this article can be found online at: https://www.frontiersin.org/articles/10.3389/fevo.2021.607555/full#supplementary-material
Supplementary Figure 1. Temperature, relative humidity, and light intensity of coffee plants. (A) CWSB behavior experiment (n = 27, days = 10). (B) Arabica and robusta choice assay experiment (n = 69, days = 12). (C) High leaf rust and less leaf rust experiment (n = 19, days = 5). (D) Infested vs. healthy arabica experiment (n = 22, days = 6). (E) Arabica with leaves vs. arabica without leaves experiment (n = 18, days = 5). (a) Average temperature and relative humidity of the coffee plants on each day (b) Light Intensity when CWSB approached the plants.
Supplementary Figure 2. Temperature, relative humidity, and light intensity of coffee plants during volatile vs. visual cues experiment. (A) Coffee plants with both visual and volatile cues present vs. visual cues present but volatile cues reduced. (a) Image of the experimental setup in field condition. (b) Light Intensity when CWSB approached the plants. (c) Temperature and relative humidity of the coffee plants on each day (n = 27). (B) Coffee plants with both visual and volatile cues present vs. volatile cues present but visual cues reduced. (a) Image of the experimental setup in field condition. (b) Light intensity when CWSB approached the plants. (c) Temperature and relative humidity of the coffee plants on each day (n = 21).
Supplementary Figure 3. Traps used in field conditions. (A) Image of black panel trap used in the coffee plantation, (B) Cut wood blend (WB1) in a zip pouch bag with cotton swabs. (C) Collection cup with insects trapped.
Supplementary Table 1. List of statistical analyses for behavioral assays and host volatile trap experiments with corresponding figure reference indicating no of days observed, no of beetles tested, n-values (n), Chi-square (X2), degrees of freedom (df) Cohen's w statistics, and significance (*p < 0.05).
Supplementary Table 2. Volatile release rate ratio of leaf blend (LB). Volatile release rate ratio of LB (10−3 v/v) at different hours of field exposure compared with the relative area % of coffee leaf volatiles (arabica, n = 4).
Supplementary Table 3. Volatile release rate ratio of wood blend 1 (WB1). Volatile release rate ratio of WB1 (10−2 v/v) at different hours of field exposure compared with the relative area % of silver oak cut stem volatiles (n = 6).
Supplementary Table 4. Volatile release rate ratio of wood blend 2 (WB2). Volatile release rate ratio of WB2 (10−2 v/v) at different hours of field exposure compared with the relative area % of silver oak cut stem volatiles (n = 6).
Supplementary Table 5. Number of CWSB responses for different compounds during GC-EAD.
References
Allison, J. D., Borden, J. H., and Seybold, S. J. (2004). A review of the chemical ecology of the Cerambycidae (Coleoptera). Chemoecology 14, 123–150. doi: 10.1007/s00049-004-0277-1
Ambinakudige, S., and Sathish, B. N. (2009). Comparing tree diversity and composition in coffee farms and sacred forests in the Western Ghats of India. Biodivers. Conserv. 18, 987–1000. doi: 10.1007/s10531-008-9502-5
Catry, F. X., Branco, M., Sousa, E., Caetano, J., Naves, P., and Nóbrega, F. (2017). Presence and dynamics of ambrosia beetles and other xylophagous insects in a Mediterranean cork oak forest following fire. Forest Ecolo. Manag. 404, 45–54. doi: 10.1016/j.foreco.2017.08.029
Caudill, S. A., Vaast, P., and Husband, T. P. (2014). Assessment of small mammal diversity in coffee agroforestry in the Western Ghats, India. Agrofor. Syst. 88, 173–186. doi: 10.1007/s10457-013-9664-3
Cha, D. H., Olsson, S. B., Yee, W. L., Goughnour, R. B., Hood, G. R., Mattsson, M., et al. (2017). Identification of host fruit volatiles from snowberry (Symphoricarpos albus), attractive to Rhagoletis zephyria flies from the Western United States. J. Chem. Ecol. 43, 188–197. doi: 10.1007/s10886-016-0814-8
Chang, C. H., Karanth, K. K., and Robbins, P. (2018). Birds and beans: comparing avian richness and endemism in arabica and robusta agroforests in India's Western Ghats. Sci. Rep. 8:3143. doi: 10.1038/s41598-018-21401-1
Cohen, J. (1988). Statistical Power Analysis for the Behavioral Sciences. New York, NY: Lawrence Erlbaum Associates. doi: 10.4324/9780203771587
Conchou, L., Lucas, P., Meslin, C., Proffit, M., Staudt, M., and Renou, M. (2019). Insect odorscapes: From plant volatiles to natural olfactory scenes. Front. Physiol. 10:972. doi: 10.3389/fphys.2019.00972
DaMatta, F. M. (2004). Ecophysiological constraints on the production of shaded and unshaded coffee: a review. Field Crops Res. 86, 99–114. doi: 10.1016/j.fcr.2003.09.001
Dastagiri, M. B. (2017). Analysis of economic trends in overseas markets for Indian tea and coffee. Outlook Agr. 46, 44–48. doi: 10.1177/0030727016689736
dosReis, M. R., Fernandes, F. L., Lopes, E. A., Gorri, J. E. R. Z., and Alves, F. M. (2015). “Pesticide Residues in Coffee Agroecosystems”, in Coffee in Health and Disease Prevention, ed. Victor Preedy (Amsterdam, NL: Academic Press), 235–244. doi: 10.1016/B978-0-12-409517-5.00026-7
Gana, S. (2016). White Stem Borer (WSB) in Western Ghats and shifts towards robusta coffee: Evidences from a recent household survey of arabica coffee growers in Kodagu district of Karnataka state. Agric. Situat. India 73, 26–37.
Garcia, C. A., Bhagwat, S. A., Ghazoul, J., Nath, C. D., Nanaya, K. M., Kushalappa, C. G., et al. (2010). Biodiversity conservation in agricultural landscapes: Challenges and opportunities of coffee agroforests in the Western Ghats, India. Conserv. Biol. 24, 479–488. doi: 10.1111/j.1523-1739.2009.01386.x
Getahun, M. N., Wicher, D., Hansson, B. S., and Olsson, S. B. (2012). Temporal response dynamics of Drosophila olfactory sensory neurons depends on receptor type and response polarity. Front. Cell. Neurosci. 6:54. doi: 10.3389/fncel.2012.00054
Ghaninia, M., Olsson, S. B., and Hansson, B. S. (2014). Physiological organization and topographic mapping of the antennal olfactory sensory neurons in female hawkmoths, Manduca sexta. Chem. Senses. 39, 655–671. doi: 10.1093/chemse/bju037
Graham, E. E., Mitchell, R. F., Reagel, P. F., Barbour, J. D., Millar, J. G., and Hanks, L. M. (2010). Treating panel traps with a fluoropolymer enhances their efficiency in capturing cerambycid beetles. J. Econ. Entomol. 103, 641–647. doi: 10.1603/EC10013
Graham, E. E., and Poland, T. M. (2012). Efficacy of fluon conditioning for capturing cerambycid beetles in different trap designs and persistence on panel traps over time. J. Econ. Entomol. 105, 395–401. doi: 10.1603/EC11432
Haack, R. A., Hérard, F., Sun, J., and Turgeon, J. J. (2010). Managing invasive populations of Asian longhorned beetle and citrus longhorned beetle: a worldwide perspective. Annu. Rev. Entomol. 55, 521–546. doi: 10.1146/annurev-ento-112408-085427
Hall, D. R., Cork, A., Phythian, S. J., Chittamuru, S., Jayarama, B. K., Venkatesha, M. G., et al. (2006). Identification of components of male-produced pheromone of coffee white stemborer, Xylotrechus quadripes. J. Chem. Ecol. 32, 195–219. doi: 10.1007/s10886-006-9360-0
Hanks, L. M., and Millar, J. G. (2016). Sex and aggregation-sex pheromones of cerambycid beetles: Basic science and practical applications. J. Chem. Ecol. 42, 631–654. doi: 10.1007/s10886-016-0733-8
Karanth, K. K., Sankararaman, V., Dalvi, S., Srivathsa, A., Parameshwaran, R., Sharma, S., et al. (2016). Producing diversity: Agroforests sustain avian richness and abundance in India's Western Ghats. Front. Ecol. Evol. 4:111. doi: 10.3389/fevo.2016.00111
Khan, Z., Midega, C. A., Hooper, A., and Pickett, J. (2016). Push-pull: chemical ecology-based integrated pest management technology. J. Chem. Ecol. 42, 689–697. doi: 10.1007/s10886-016-0730-y
Kigathi, R. N., Unsicker, S. B., Reichelt, M., Kesselmeier, J., Gershenzon, J., and Weisser, W. W. (2009). Emission of volatile organic compounds after herbivory from Trifolium pratense (L.) under laboratory and field conditions. J. Chem. Ecol. 35:1335. doi: 10.1007/s10886-009-9716-3
Lee, H. L., and Lee, C. Y. (Eds.). (2007). Building Supply Chain Excellence in Emerging Economies. Vol. 98. Boston, MA: Springer Science & Business Media. doi: 10.1007/978-0-387-38429-0
Manikandan, K. R., Muthuswami, M., Chitra, N., and Ananthan, M. (2019). Management of coffee white stem borer Xylotrechus quadripes (Chevrolat, 1863) (Coleoptera: Cerambycidae) in the Lower Pulney Hills, India. Int. J. Curr. Microbiol. Appl. Sci. 8, 1703–1713. doi: 10.20546/ijcmas.2019.806.203
Mathew, G. (1982). “A survey of beetles damaging commercially important stored timber in Kerala”, in Research Report, Kerala Forest Research Institute No. 10, 93.
Millar, J. G., and Hanks, L. M. (2017). “Chemical Ecology of Cerambycids” in Cerambycidae of the World: Biology and Pest Management, ed. Qiao Wang (Boca Raton, NY: CRC Press), 161–208.
Mone, S., Kusha, K. M., Jathanna, D., Ali, M., and Goel, A. (2014). Comparison of insect biodiversity between organic and conventional plantations in Kodagu, Karnataka, India. J. Threat. Taxa 6, 6186–6194. doi: 10.11609/JoTT.o3778.6186-94
Murphy, S. T., Phiri, N., Sreedharan, K., Kutywayo, D., and Chanika, C. (2008). Integrated Stem Borer Management in Smallholder Coffee Farms in India, Malawi and Zimbabwe. Final Technical Report. Egham: CFC/CABI/ICO/CRS/CB, 220.
Muthuramkumar, S., Ayyappan, N., Parthasarathy, N., Mudappa, D., Raman, T. S., Selwyn, M. A., et al. (2006). Plant community structure in tropical rain forest fragments of the Western Ghats, India. Biotropica 38, 143–160. doi: 10.1111/j.1744-7429.2006.00118.x
Nair, J. V., Shanmugam, P. V., Karpe, S. D., Ramakrishnan, U., and Olsson, S. (2018). An optimized protocol for large-scale in situ sampling and analysis of volatile organic compounds. Ecol. Evol. 8, 5924–5936. doi: 10.1002/ece3.4138
Nath, C. D., Pélissier, R., Ramesh, B. R., and Garcia, C. (2011). Promoting native trees in shade coffee plantations of southern India: Comparison of growth rates with the exotic Grevillea robusta. Agrofor. Syst. 83, 107–119. doi: 10.1007/s10457-011-9401-8
Nordström, K., Dahlbom, J., Pragadheesh, V. S., Ghosh, S., Olsson, A., Dyakova, O., et al. (2017). In situ modeling of multimodal floral cues attracting wild pollinators across environments. Proc. Natl. Acad. Sci. 114, 13218–13223. doi: 10.1073/pnas.1714414114
Olsson, S. B., and Hansson, B. S. (2013). Electroantennogram and single sensillum recording in insect antennae. Methods Mol. Biol. 1068, 157–177. doi: 10.1007/978-1-62703-619-1_11
Prashant. (2014). Studies on chemical ecology and behaviour of coffee white stem borer (CWSB), Xylotrechus quadripes Chevrolat (Coleoptera: Cerambycidae). [Doctoral dissertation]. Bangalore: University of Agricultural Sciences.
Raguso, R. A., and Willis, M. A. (2005). Synergy between visual and olfactory cues in nectar feeding by wild hawkmoths, Manduca sexta. Anim. Behav. 69, 407–418. doi: 10.1016/j.anbehav.2004.04.015
Rhainds, M., Lan, C. C., King, S., Gries, R., Mo, L. Z., and Gries, G. (2001a). Pheromone communication and mating behaviour of coffee white stem borer, Xylotrechus quadripes Chevrolat (Coleoptera: Cerambycidae). Appl. Entomol. Zool. 36, 299–309. doi: 10.1303/aez.2001.299
Rhainds, M., Warndorff, M., Chiew, P. K., Lan, C. C., and Gries, G. (2001b). Stimuli increasing oviposition by female coffee white stem borer (Coleoptera: Cerambycidae). Can. Entomol. 133, 409–412. doi: 10.4039/Ent133409-3
Robbins, P., Chhatre, A., and Karanth, K. (2015). Political ecology of commodity agroforests and tropical biodiversity. Conserv. Lett. 8, 77–85. doi: 10.1111/conl.12169
Robert, A. H. (2017). “Cerambycid Pests in Forest and Urban Trees,” in Cerambycidae of the World: Biology and Pest Management, ed. Qiao Wang (Boca Raton, NY: CRC Press), 305–320.
Sangamesh, R. H. (2015). Cerambycidae fauna in plantation and fruit crop ecosystem of Western Ghats in Karnataka. M.Sc thesis, University of Agricultural and Horticultural Sciences, Shivamogga.
Santosh, P., Sreenath, H. L., Kumar, P. K. V., Seetharama, H. G., and Jayarama. (2011). New record of coffee white stem borer Xylotrechus quadripes Chevrolat on Psilanthus bengalensis in India. J. Plant. Crops, 396–398.
Schowalter, T. D. (2013). “Ecology and Management of Bark Beetles”, in International Symposium on Forest Health Management, Taiwan: Taiwan Forest Research Institute, 167–187.
Seetharama, H. G., Vasudev, V., Kumar, P. K. V., and Sreedharan, K. (2004). Behaviour of coffee white stem borer beetle in the field. J. Coffee Res. 32, 55–64.
Seetharama, H. G., Vasudev, V., Kumar, P. K. V., and Sreedharan, K. (2005). Biology of coffee white stem borer Xylotrechus quadripes Chev. (Coleoptera: Cerambycidae). J. Coffee Res. 33, 98–107.
Shylesha, A. N. (1992). Studies on bioecology and management of white stem borer of coffee Xylotrechus quadripes chev.(cerambycidae: coleoptera). Doctoral dissertation, University of Agricultural Sciences, Bangalore.
Shylesha, A. N., and Veeresh, G. K. (1995). Incidence of coffee white stemborer Xylotrechus quadripes Cheve. in major coffee growing tracts of Karnataka. J. Hill Res. 8, 239–241.
Suresh Kumar, V. B., Sambamurthy Reddy, A. G., and Ramachandran, M. (1992). Parkia roxburghii G. Don.-a leguminous shade tree for coffee. Indian Coffee 56, 3–9.
Tait, C., Batra, S., Ramaswamy, S. S., Feder, J. L., and Olsson, S. B. (2016). Sensory specificity and speciation: a potential neuronal pathway for host fruit odour discrimination in Rhagoletis pomonella. Pro. R. Soc. B. 283:1845. doi: 10.1098/rspb.2016.2101
The Coffee Board of India (2019). Export Info. Abstract on Both Indian & Re–exported Coffee. Available online at: https://www.indiacoffee.org/ExportInfo_Archives.aspx (accessed August 16, 2020).
The Coffee Board of India (2020). Database on Coffee, January 2020. Available online at: https://www.indiacoffee.org/database-coffee.html (accessed August 16, 2020).
Tscharntke, T., Clough, Y., Bhagwat, S. A., Buchori, D., Faust, H., Hertel, D., et al. (2011). Multifunctional shade-tree management in tropical agroforestry landscapes - a review. J. Appl. Ecol. 48, 619–629. doi: 10.1111/j.1365-2664.2010.01939.x
Veeresh, G. K. (1995). Bioecology and Management of Coffee White Stem Borer: Xylotrechus quadripes (Chev). Bangalore: University of Agricultural Sciences, GKVK.
Venkatesha, M. G. (2010). “Sustainable coffee cultivation in India: challenges and management”, in Proceedings of the 16th Asian Agricultural Symposium and 1st International Symposium on Agricultural Technology (Bangkok: King Mongkut's Institute of Technology Ladkrabang), 492–495.
Venkatesha, M. G., and Dinesh, A. S. (2012). The coffee white stemborer Xylotrechus quadripes (Coleoptera: Cerambycidae): Bioecology, status and management. Int. J. Trop. Insect Sci. 32, 177–188. doi: 10.1017/S1742758412000331
Venkatesha, M. G., Krishnamoorthy Bhat, P., and Seetharama, H. G. (1995). Courtship behaviour of the coffee white stem borer, Xylotrechus quadripes (Chevr.) (Coleoptera: Cerambycidae). J. Coffee Res. 25, 88–93.
Venkatesha, M. G., and Seetharama, H. G. (1999a). Incidence of coffee white stem borer, Xylotrechus quadripes (Chevr) (Coleoptera: Cerambycidae) on teak, Tectona grandis L. Pest Manag. Horti. Ecosys. 5, 142–144.
Venkatesha, M. G., and Seetharama, H. G. (1999b). Sexing of adults of coffee white stem borer, Xylotrechus quadripes (Chevr.) (Coleoptera: Cerambycidae). Entomon 24, 275–280.
Keywords: chemical ecology, electrophysiology, agriculture, phytochemistry, cerambycidae, kairomones
Citation: Rajus S, Bhagavan SG, Kharva H, Rao S and Olsson SB (2021) Behavioral Ecology of the Coffee White Stem Borer: Toward Ecology-Based Pest Management of India's Coffee Plantations. Front. Ecol. Evol. 9:607555. doi: 10.3389/fevo.2021.607555
Received: 17 September 2020; Accepted: 08 January 2021;
Published: 09 February 2021.
Edited by:
William Benjamin Walker III, United States Department of Agriculture (USDA-ARS), United StatesReviewed by:
Jin Zhang, Max Planck Institute for Chemical Ecology, GermanyJonathan David Sweeney, Canadian Forest Service, Canada
Copyright © 2021 Rajus, Bhagavan, Kharva, Rao and Olsson. This is an open-access article distributed under the terms of the Creative Commons Attribution License (CC BY). The use, distribution or reproduction in other forums is permitted, provided the original author(s) and the copyright owner(s) are credited and that the original publication in this journal is cited, in accordance with accepted academic practice. No use, distribution or reproduction is permitted which does not comply with these terms.
*Correspondence: Shannon B. Olsson, c2hhbm5vbkBuaWNlLm5jYnMucmVzLmlu