- 1Department of Plant Sciences, University of Cambridge, Cambridge, United Kingdom
- 2NIAB EMR, Kent, United Kingdom
- 3International Centre of Insect Physiology and Ecology, Nairobi, Kenya
- 4Rothamsted Research, Harpenden, United Kingdom
Cucumber mosaic virus (CMV)-infected tomato (Solanum lycopersicum L.) plants emit volatile organic compounds (VOCs) attractive to bumblebees (Bombus terrestris L.), which are important tomato pollinators, but which do not transmit CMV. We investigated if this effect was unique to the tomato-CMV pathosystem. In two bean (Phaseolus vulgaris L.) cultivars, infection with the potyviruses bean common mosaic virus (BCMV) or bean common mosaic necrosis virus (BCMNV), or with the cucumovirus CMV induced quantitative changes in VOC emission detectable by coupled gas chromatography–mass spectrometry. In free-choice olfactometry assays bumblebees showed an innate preference for VOC blends emitted by virus-infected non-flowering bean plants and flowering CMV-infected bean plants, over VOCs emitted by non-infected plants. Bumblebees also preferred VOCs of flowering BCMV-infected plants of the Wairimu cultivar over non-infected plants, but the preference was not significant for BCMV-infected plants of the Dubbele witte cultivar. Bumblebees did not show a significant preference for VOCs from BCMNV-infected flowering bean plants but differential conditioning olfactometric assays showed that bumblebees do perceive differences between VOC blends emitted by flowering BCMNV-infected plants over non-infected plants. These results are consistent with the concept that increased pollinator attraction may be a virus-to-host payback, and show that virus-induced changes in bee-attracting VOC emission is not unique to one virus-host combination.
Introduction
Plants emit volatile organic compounds (VOCs) that function as signals in plant-plant communication, defense, and attraction of pollinators, seed dispersers, and other beneficial organisms (Dudareva and Pichersky, 2008). Insects, and in particular bees, are the most important group of plant pollinators (Knight et al., 2005; Ghazoul, 2006; Klein et al., 2007). With respect to insect-pollinated plants, pollinator visitation (or artificial pollination) is required to obtain maximal seed and fruit production (Klein et al., 2007; Klatt et al., 2014). Bees possess over 130 distinct antennal olfactory receptors and exploit olfactory cues when foraging and pollinating flowers (Chittka and Raine, 2006). The olfactory cues are important in differentiating between plant species, and in signaling the appropriate phenological stage for pollinator visits (Chen et al., 2009; Hossaert-McKey et al., 2010; Song et al., 2014). Indeed, bees exhibit innate preferences for certain floral odors (Raguso, 2008), and VOCs have been shown to act as long-distance flower location signals for pollinators (Kunze and Gumbert, 2001; Burger et al., 2010; Suchet et al., 2011) and can trigger an immediate pollinator decision to land on a flower (Lunau, 1992). However, the complex effects of non-floral VOCs or floral/non-floral VOC combinations on bee foraging and other insect behaviors are still not well understood (Junker et al., 2017), and in particular the influence of microorganisms (including viruses) on plant VOC emission is relatively under-investigated (Beck and Vanette, 2017).
Phaseolus vulgaris L. (common bean) is a plant that benefits from insect pollination with increased seed production (Ibarra-Perez et al., 1999; Kingha et al., 2012; Ramos et al., 2018; Elisante et al., 2020) and is also host to a number of viruses that can reduce seed yield. Bean common mosaic virus (BCMV) and bean common mosaic necrosis virus (BCMNV) are closely related potyviruses that are among the most prevalent viruses infecting legumes and both result in serious crop loss (Morales, 2006). Cucumber mosaic virus (CMV) is a cucumovirus with a very wide host range (Yoon et al., 2019) that causes serious epidemics and losses in cultivated common bean (Morales, 2006; Thompson et al., 2015; Worrall et al., 2015). Aphids transmit CMV, BCMV, and BCMNV, particles of which are carried between hosts on the stylets (probing mouthparts) of these insects, but the viruses are also transmitted efficiently through seed of infected bean plants (Morales, 2006; Worrall et al., 2015; Yoon et al., 2019). Viruses induce changes in plant metabolism that influence insect vector behavior (Carr et al., 2018; Mauck et al., 2016). For example, CMV, BCMV, and BCMNV induce biochemical changes in common bean that alter the feeding behavior of aphid vectors (Wamonje et al., 2020), and CMV induces alterations in the emission of VOCs that attract aphid vectors to infected hosts (Mauck et al., 2010). Such virus-induced changes in VOC emission by infected hosts are thought to contribute to an increase the rate of aphid-mediated virus transmission (Mauck et al., 2016; Carr et al., 2018; Donnelly et al., 2019). Currently, there is a paucity of data about virus-induced changes on other community members, such as pollinators.
There is no evidence that CMV, BCMV, or BCMNV are transmitted by bees, or by other pollinators. Indeed, comparatively few plant viruses are vectored by pollinators (Hull, 2014). It was therefore surprising to discover that bumblebees (Bombus terrestris) could perceive differences in the VOC blends emitted by uninfected and CMV-infected plants of tomato (Solanum lycopersicum), and Arabidopsis thaliana (Groen et al., 2016). In the same study it was also found that bumblebees enhanced seed production by CMV-infected tomato plants to a greater extent than for mock-inoculated plants (Groen et al., 2016). Mathematical modeling indicated that if a pollinator bias in favor of virus-infected plants in wild host populations occurred, it could outweigh the benefits of resistance, and natural selection would instead favor inheritance by plants of alleles for virus susceptibility, rather than those conditioning resistance (Groen et al., 2016). In this study we investigated whether the innate preference of bumblebees for VOC blends emitted by virus-infected plants is peculiar only to the previously investigated tomato-CMV pathosystem, or if this can occur in other virus-host combinations, such as bean plants infected with CMV, BCMV, or BCMNV.
Materials and Methods
Plants and Viruses
Phaseolus vulgaris cv. “Wairimu”/Red Haricot-GLP 585 (Simlaw Seeds, Nairobi, Kenya) and cv. “Dubbele witte” (“Stamslaboon Dubbele Witte”: van Hemert & Co., Tuinzaden.eu, Amsterdam, Netherlands) seeds were germinated and plants grown as previously described (Wamonje et al., 2020). Inocula for BCMV (PV-0915), BCMNV (PV-0413), and CMV (bean isolate PV-0473), obtained from the German Collection of Microorganisms and Cell Cultures, were prepared as previously described (Wamonje et al., 2020). Plants were mechanically inoculated with infected plant sap or mock inoculated with sterile water, and infection was authenticated by double-antibody sandwich enzyme-linked immunosorbent assays (Bio-Reba, Reinach, Switzerland).
Plant-Emitted Volatile Organic Compound Collection and Analysis
Headspace VOCs from flowering plants of the Wairimu variety that had been mock-inoculated or infected with BCMV, BCMNV, or CMV, were analyzed by GC-MS. VOCs were collected from flowering plants between 1 and 2 weeks after the first flowers opened (22–26 days post-inoculation) by dynamic headspace trapping and analyzed by gas chromatography-mass spectrometry (GC-MS) as previously described (Beale et al., 2006; Webster et al., 2008; Groen et al., 2016). Briefly, headspace volatiles were collected from plants over a period of 24 h onto Porapak Q filters [50 mg, 60/80 mesh size, Supelco (Sigma-Aldrich)]. The plants were contained in a 1.0 liter bell jar clamped to two semi-circular metal plates with a hole in the center to accommodate the stem. Charcoal-filtered air was pumped in at the bottom of the container at a rate of 800 ml.min–1 and drawn out through the Porapak Q filter at the top, at a rate of 750 ml.min–1. Leaf fresh weight and dry weight were measured to enable normalization of the volatile abundance. Trapped organic chemicals were eluted from the Porapak Q filter with diethyl ether for analysis by gas chromatography coupled to mass spectrometry (GC-MS). Volatiles were separated on a capillary GC column (ZB-5MSi, Thermo Scientific, United Kingdom). The injection volume (splitless) was 1 μl, the injector temperature was 200°C, and helium was used as the carrier gas at a constant flow rate of 2.6 ml min–1 in an oven maintained at 30°C for 5 min and then programmed at 15°C.min–1 to 230°C. The column was directly coupled to a mass spectrometer (ISQ LT, Thermo Scientific, United Kingdom) with a MS transfer line temperature of 240°C. Ionization was by electron impact with an ion source temperature of 250°C in positive ionization. Mass ions were detected between 30 and 650 m/z. Data were collected using Xcalibur software (Thermo Scientific). VOCs were identified by comparing spectra with those curated by the National Institute of Standards and Technology1 and by comparison with an authentic standard. Abundant VOCs were quantified using standard curves generated with pure benzaldehyde, linalool, ocimene, and pinene, and others quantified as pinene equivalents.
To determine if virus infection causes overall changes in the VOC blends emitted by plants, principal component analysis was performed with MetaboAnalyst 5.0 (Chong et al., 2019) on the quantified VOCs after Pareto scaling. Multivariate analysis of variance (MANOVA), using the Wilks’ lambda test statistic, was carried out with the Real Statistics Resource Pack software, Release 7.6 (Zaiontz, 2020). Significant results from MANOVAs were followed up with univariate one-way ANOVAs to assess which VOC(s) differed between groups using R (RStudio Team, 2020). Significant univariate ANOVAs were then followed up with Tukey’s HSD post hoc test to allow detailed comparisons between individual pairs of groups.
Bumblebee Free-Choice and Differential Conditioning Olfactometry Assays
Buff-tailed bumblebees (Bombus terrestris audax L.) were sourced from Koppert Biological Systems (Berkel en Rodenrijs, Netherlands), or Syngenta-Bioline (Leicester, United Kingdom). Free-choice preference assays were performed to identify any innate preference of bumblebees for VOCs emitted by virus-infected versus mock-inoculated bean plants. Assays were carried out using flight arenas (Whitney et al., 2009) and procedures previously described with 8 cm diameter/15 cm height cylindrical towers enclosing infected or mock-inoculated plants (Groen et al., 2016). To obscure plants, but allow VOC diffusion, towers were roofed with muslin overlaid with plastic mesh, supporting cups containing 30% (w/v) sucrose to incentivize visitation (Supplementary Figure 1). Between experiments, arenas and tower roofs were cleaned with 30% (v/v) ethanol to remove scent marks. Five mock-inoculated and five virus-infected bean plants were concealed under towers randomly placed in the arena. Thirty paint-marked bees were released one at a time (Supplementary Figure 1). The first 10 choices of each bee were recorded either as acceptance or rejection of a tower. A similar choice test was carried out to assess bee preference for volatiles from flowering versus non-flowering plants, except the first 10 choices of 40 bees was recorded.
Free choice assays were analyzed using binomial generalized linear mixed models, fitted using mixed_model in the GLMMadaptive package (Rizopoulos, 2021). The identity of each bee was used as a random effect in fitting the models, allowing differences between bees to be accounted for. Wald tests assessing whether the fixed effect (i.e., the intercept) in the fitted model differed significantly from 0 were used to determine whether bumblebee preference significantly differed from an expected frequency distribution of 50:50 for either mock-inoculated or virus-infected bean plants. To account for multiple comparisons, p-values were subjected to the Holm-Bonferroni correction.
Differential conditioning (“learning curve”) assays determined bumblebees’ ability to discriminate between VOCs emitted by infected and mock-inoculated plants, regardless of instinctive preference (Dyer and Chittka, 2004; Whitney et al., 2009; Groen et al., 2016). Cups on towers concealing five flowering mock-inoculated plants and five flowering BCMNV-infected plants contained 30% (w/v) sucrose “reward” or 0.12% (w/v) quinine hemisulphate solution “punishment,” respectively, and the first 100 choices of each bee were recorded (Groen et al., 2016). Bumblebees cannot distinguish quinine from sucrose except by taste (Whitney et al., 2009). Statistical analysis of data from differential conditioning assays were analyzed using binomial mixed-effects logistic regression (Foster et al., 2014; Groen et al., 2016), again using mixed_model in the GLMMadaptive package (Rizopoulos, 2021). The identity of individual bees controlled a random intercept, allowing differences between bees to be accounted for in the analysis. Whether or not bees “learnt” was tested using a likelihood ratio test comparing the fit of the model in which the probability of selecting the sucrose reward depended on how many choices had been made by an individual bee with a simpler model in which the probability was independent of the choice (but depended on the bee in question).
Results
Viruses Induce Quantitative Changes in Volatile Organic Compounds Emitted by Flowering Bean Plants
Virus infection decreased the quantity of VOCs emitted by approximately half compared with emission levels of uninfected plants (Figure 1A). This might be expected since all three viruses cause stunting (Supplementary Figure 2) and the VOC emission rate at the tissue-mass level for plants infected with BCMV, BCMNV, and CMV was not significantly different from mock-inoculated plants (Figure 1B). Principle component analysis on the emitted VOCs showed that the scores from mock-inoculated plants form a discrete cluster while the scores from the virus-infected plants group together (Figure 2A). The scores and loadings biplot explains which variables have the largest effect on each sample (Figure 2B). (E)-Ocimene, linalool and 4-hexen-1-ol had a strong influence on PC1 (that is mainly responsible for separating the mock and virus-inoculated plant scores), while benzaldehyde had a stronger influence on PC2.
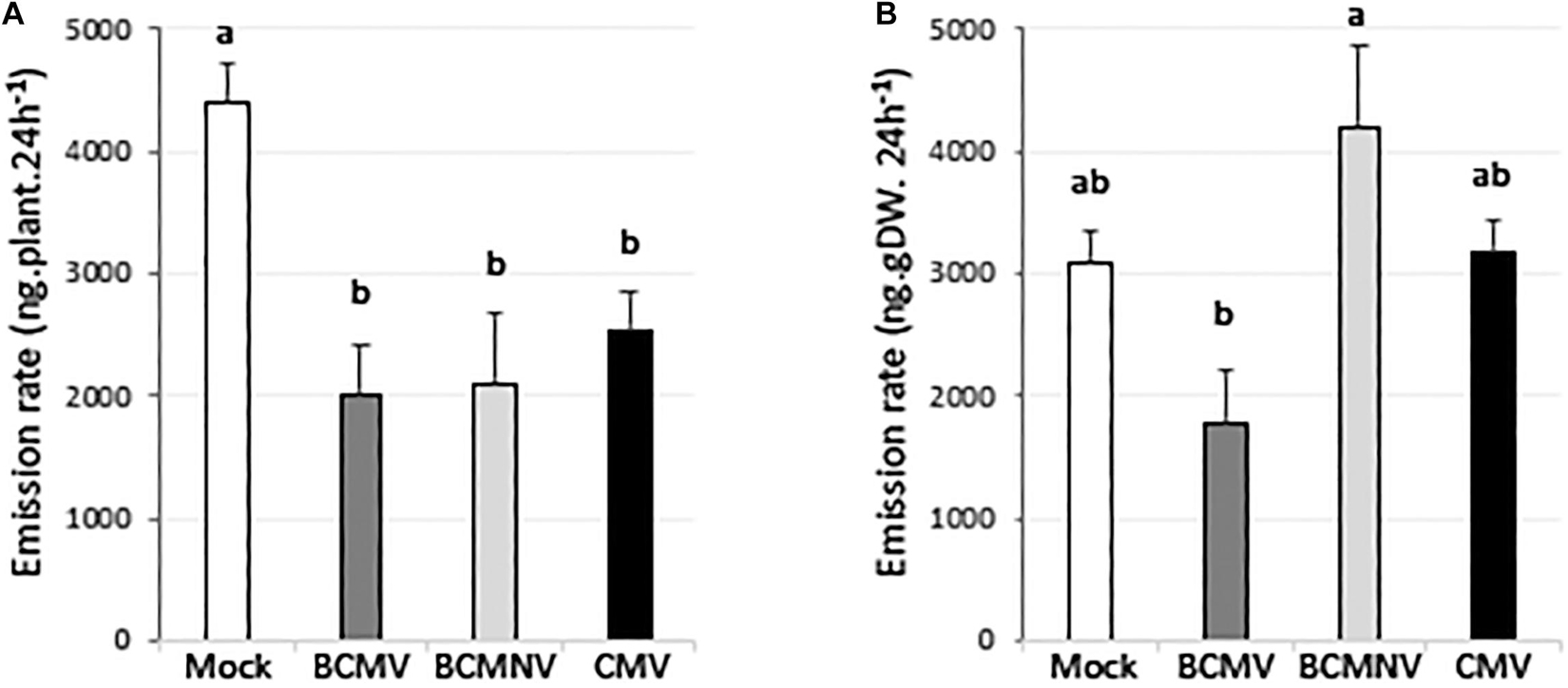
Figure 1. Effects of virus infection on emission of volatile organic compounds from flowering bean plants. (A) Whole plant total emission rate (ng.24h–1) of the 20 most abundant volatiles is lower in virus-infected plants compared to mock-inoculated plants. (B) VOC emission rate (ng.24h–1) per gram dry weight of the combined (20 most abundant) volatiles from virus-infected plants. Mean VOC emission values for combined volatiles are presented (n = 4 plants, or 3 plants in the case of BCMNV-infected plants, per treatment). Error bars represent standard error of the mean. Different letters are assigned to significantly different results calculated with one-way ANOVA and post hoc Tukey HSD testing (p < 0.05). The VOC total emission rate was calculated by summing the emission of the 20 most abundant VOCs for each sample and expressing it per plant (left panel) or per ng.DW (right panel). These VOCs are listed in Supplementary Table 1.
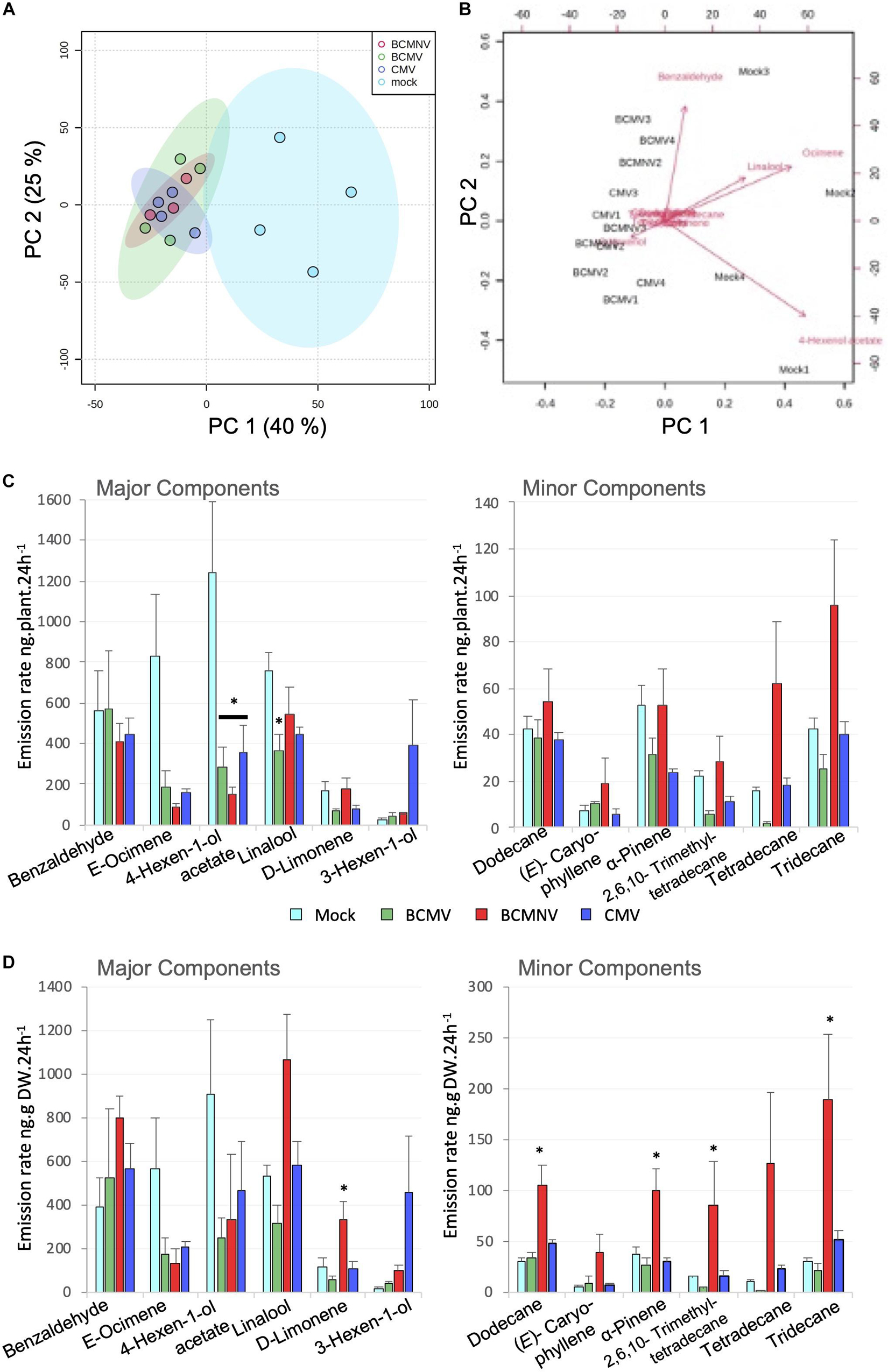
Figure 2. Blend of volatile organic compounds (VOCs) emitted by flowering bean plants infected with different viruses. (A) Score scatter plot from principal component (PC) analysis of quantified VOCs collected by dynamic headspace trapping from bean plants that had been mock-inoculated (light blue), infected with BCMV (green), BCMNV (red) or CMV (dark blue). The percentage of variation of the data explained by PC1 and PC2 is in parentheses (40.1 and 25%, respectively). (B) PCA plot with loadings (biplot) to illustrate the variables that have the largest effect on each sample. The longer the vector, the more influence the variable has on the samples. E-Ocimene and 4-hexen-1-ol have a strong influence on PC1 that largely accounts for the clustering of mock-inoculated plant scores away from the virus-infected plant scores. (C) Whole plant emission rate of VOCs from mock-inoculated, BCMV, BCMNV, and CMV-infected bean plants showing major components (left panel) and minor components (right panel). (D) Emission rate normalized to dry weight of VOCs from mock-inoculated, BCMV, BCMNV and CMV-infected plants expressed showing major components (left panel) and minor components (right panel). Mean VOC emission values for individual volatiles are presented (n = 4 plants per treatment or 3 plants per BCMNV treatment). Error bars represent standard error of the mean. The VOCs of the virus-infected and mock-inoculated plants were statistically different at the whole plant (C: MANOVA p = 0.039), and tissue level (D: MANOVA p = 0.018). Individual VOCs that were different between treatments in ANOVA (significance threshold p = 0.05) were followed up with Tukey’s HSD post hoc test with the significance threshold p < 0.05 (*) indicated.
Benzaldehyde, (E)-ocimene, 4-hexen-1-ol acetate, linalool and D-limonene dominated the VOC blend from the mock-inoculated bean plants (Figure 2C). These VOCs, with the exception of 4-hexen-1-ol acetate, are known to be bee attractants (Granero et al., 2005; Knudsen et al., 2006; Dötterl and Vereecken, 2010; Klatt et al., 2013; Krug et al., 2018). Despite stunting, virus-infected plant benzaldehyde-output was maintained at a level equivalent to uninfected plants (Figure 2C) but (E)-ocimene and 4-hexen-1-ol acetate emissions were, in contrast, disproportionately lower than their decreased tissue mass could account for Figures 2C,D. The lower emission rates of (E)-ocimene and 4-hexen-1-ol acetate were the main cause for the overall decrease in total VOC emission from virus-infected plants (Figure 1) and supports their importance in PCA (Figures 2A,B). BCMNV-infected plants were the most severely stunted (Supplementary Figure 2) and on a tissue per dry weight basis produced greater levels of several VOCs compared to other treatments (Figure 2D: linalool, dodecane, α-pinene, tetradecane, and tridecane). In most cases this resulted in BCMNV-infected whole plants matching or exceeding the VOC output of mock-inoculated plants. Having established that virus infection altered the VOC emissions from bean plants, we went on to test whether this affected bumblebee behavior.
Bumblebees Possess an Innate Preference for Volatile Organic Compounds Emitted by Virus-Infected Bean Plants
In free-choice olfactometry assays bumblebees displayed an innate preference for VOC blends emitted by CMV-infected and BCMV-infected flowering plants of the Wairimu cultivar, as compared to the blend emitted by mock-inoculated plants (Figure 3A). The preferences for VOCs produced by plants infected with CMV or BCMV were statistically significant. However, bees showed no preference for VOC blends emitted by flowering Wairimu plants that had been infected with BCMNV. In another cultivar of bean, Dubbele witte, bees showed a preference for VOC blends emitted by CMV-infected flowering plants but no preference for those emitted by BCMV- or BCMNV-infected plants. When bumblebees were presented with a choice between non-infected and virus-infected non-flowering plants, they preferred the odors from all virus-infected plants over healthy counterparts. This was the case for both cultivars (Figure 3B). Because the bee preference for VOCs released from virus-infected plants was more apparent when plants were not flowering, we also presented bumblebees with feeding towers concealing five non-flowering and five flowering non-infected plants (Figure 3C). Bees did not show a preference between odors from flowering plants over those from non-flowering plants.
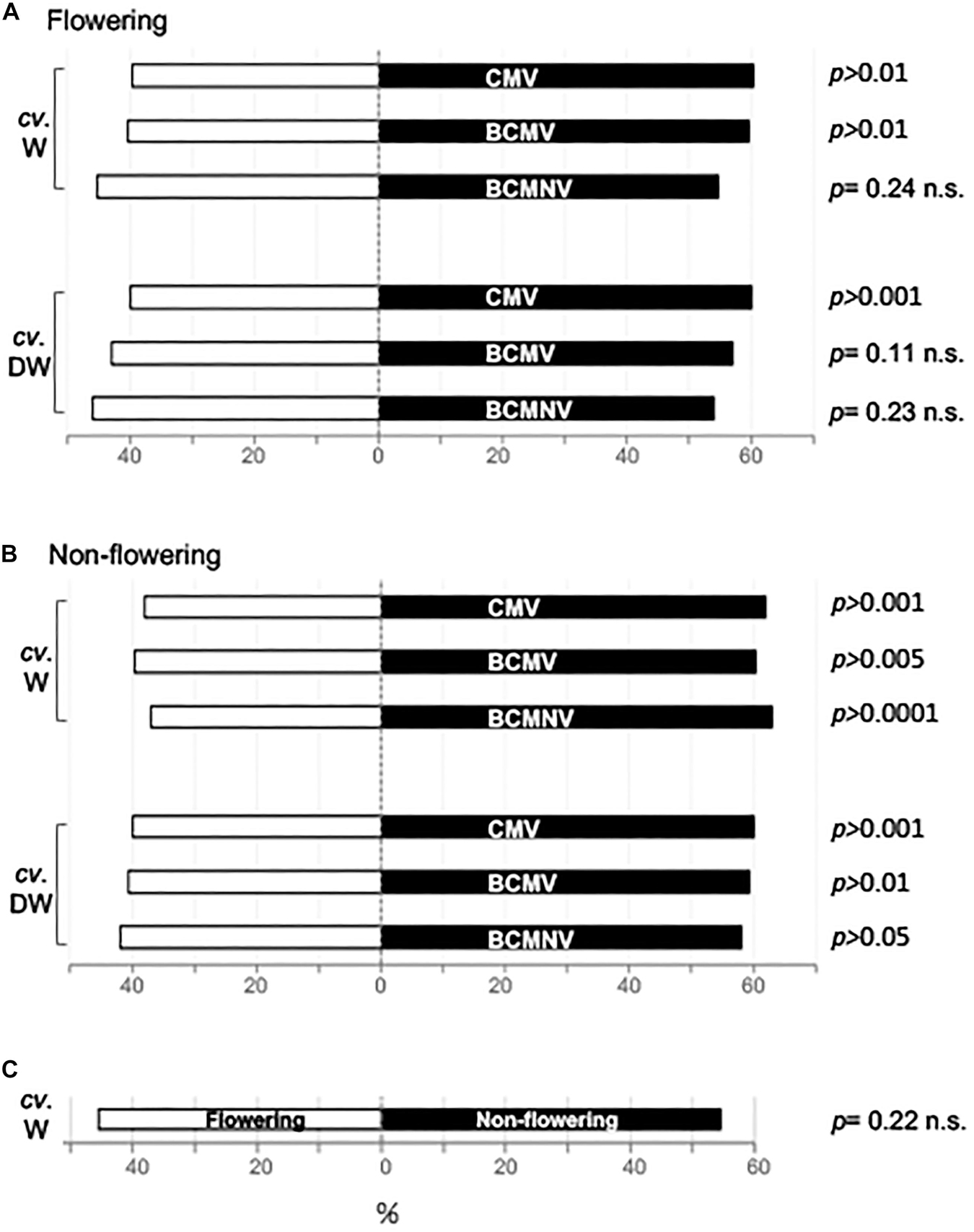
Figure 3. Bumblebees exhibit an innate preference for VOC blends emitted by virus-infected over non-infected bean plants. In free-choice assays, (A) Attraction of bumblebees to VOC blends emitted by flowering virus-infected plants and mock-inoculated plants. (B) Attraction of bumblebees to VOC blends emitted by virus-infected versus mock-inoculated non-flowering plants. (C) Attraction of bumblebees by VOC blends from healthy non-flowering and flowering plants. Plants of two common bean cultivars were used: Wairimu (cv. W) and Dubbele witte (cv. DW). The p-values shown refer to the pooled data of the first 10 choices of 30 (A,B) or 40 (C) naïve bumblebees, and relates to the Wald test of whether the intercept in the fitted binomial generalized linear mixed model was zero, i.e., whether there is any evidence – after controlling for systematic differences between individuals – that bees did anything other than choose at random, with all p-values subjected to a Holm-Bonferroni correction to account for multiple testing.
Bumblebees Perceive Bean Common Mosaic Necrosis Virus-Induced Changes in Volatile Organic Compound Emission by Flowering Plants but Do Not Find Them Intrinsically Attractive or Repellent
Bumblebees did not exhibit a preference for VOCs emitted by BCMNV-infected flowering plants over those emitted by mock-inoculated flowering plants (Figure 3B). This prompted us to investigate whether this was due to an inability of bumblebees to perceive any differences between the VOC blends emitted by BCMNV-infected plants versus non-infected plants, once they begin flowering. We used differential conditioning assays to determine if bumblebees can distinguish between stimuli (in this case VOC blends) by monitoring the insects’ ability to learn to associate one VOC blend with a sucrose reward and the other stimulus with a quinine “punishment.” Bumblebees learned rapidly to associate the reward and punishment with VOCs emitted by mock-inoculated and BCMNV-infected plants, respectively, and did so for flowering plants of both bean cultivars (Figure 4). This indicates that bumblebees can distinguish between VOC blends of BCMNV-infected and mock-inoculated flowering plants (Figure 4), despite not demonstrating a significant preference for either VOC blend in free-choice assays (Figure 3B).
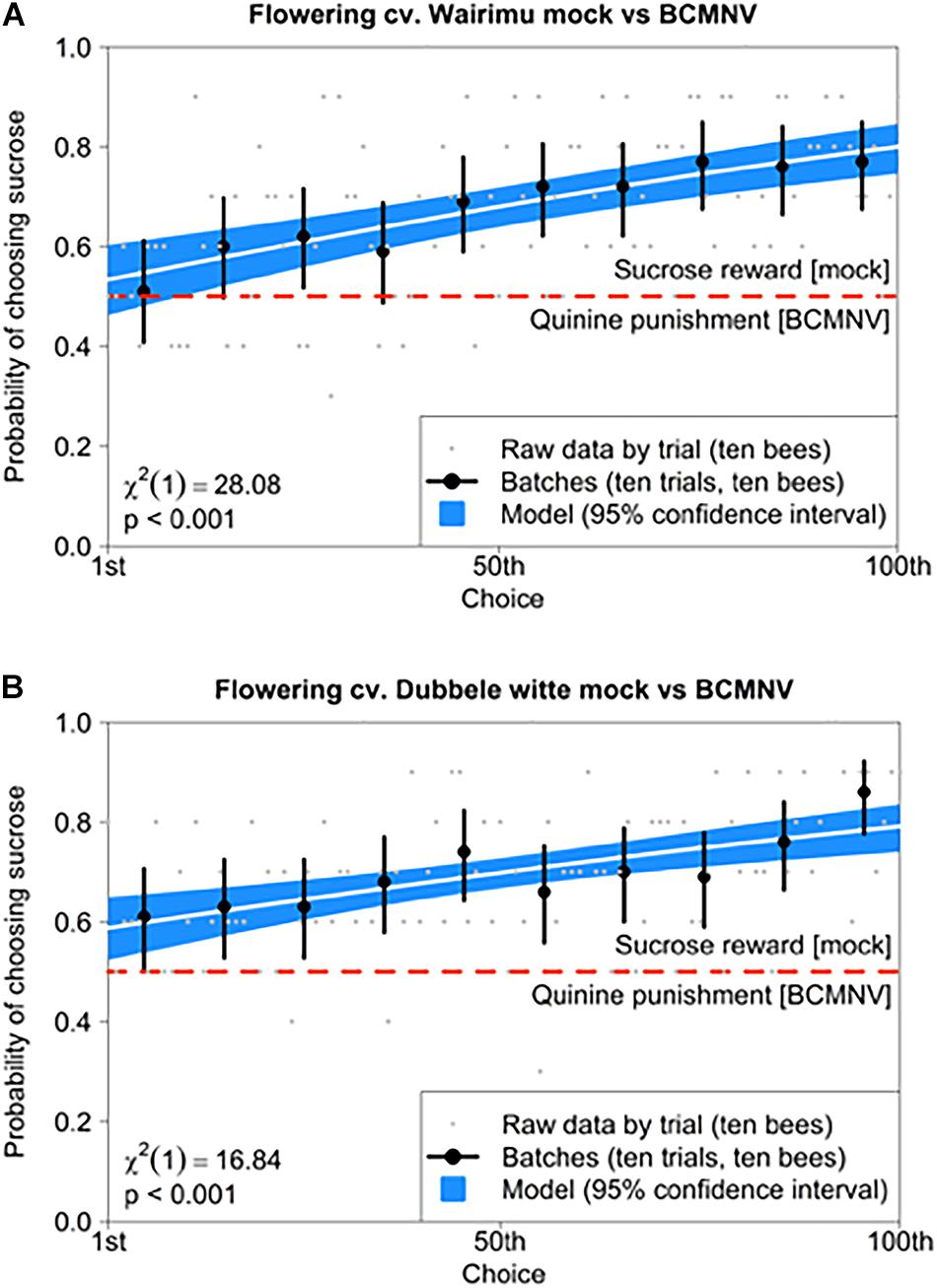
Figure 4. Bumblebees can distinguish between VOC blends emitted by flowering non-infected and BCMNV-infected bean plants despite having no innate preference for either. Using differential conditioning with a sucrose reward and quinine punishment bumblebees learned to distinguish between VOC blends emitted by BCMNV-infected and mock-inoculated flowering common bean. The learning curve indicates the overall ability to distinguish between plant-emitted volatiles analyzed after 100 choices per bee using 10 bees. In flowering cv. Wairimu (A), bumblebees initially chose the sucrose reward at a mean accuracy of 50% in their first 10 choices increasing to about 85% by choices 90 to 100 meaning bumblebees learnt to identify sucrose rewards based on the association with volatiles from flowering mock-inoculated plants. Similar results were obtained with flowering cv. Dubbele witte (B), where bumblebees’ first 10 choices had a mean accuracy of 60% for sucrose increasing to a mean of c. 75% by choices 90 to 100. Data are shown pooled over all bees (n = 10) into successive groups of 10 choices, with error bars showing 95% binomial confidence intervals for the proportion of correct choices. The white curve shows the fitted binomial generalized linear mixed model, with blue borders showing 95% confidence intervals on the fitted response. The χ2 statistic and significance level for the likelihood ratio test assessing whether bees can learn are given at the bottom left of each panel.
Discussion
We found that naïve bumblebees have an innate preference for VOC blends emitted by common bean plants infected with three different viruses over non-infected plants. It was previously established that CMV-infected tomato plants are more attractive to bumblebees than uninfected plants, and benefit more from bumblebee-mediated pollination (Groen et al., 2016). Since CMV is not transmitted by bumblebees, it was hypothesized that this could be a “payback” or compensation to susceptible hosts (Groen et al., 2016). Mathematical modeling further suggested that in wild populations of plants that were dependent upon pollinators for reproductive success, increased pollinator visitation to virus-infected plants might outweigh the benefits of resistance and favor the persistence of alleles for susceptibility in the population (Groen et al., 2016). Our current work shows that virus-induced attraction of bumblebees is not unique or peculiar to the interaction of CMV and tomato, but extends to interactions of CMV and two other viruses (neither of which are pollinator-vectored), the potyviruses BCMV and BCMNV, with common bean. These observations in two different plant species suggest that modification by viruses of the emission of bee-perceivable plant VOCs may have arisen more than once.
Common bean and tomato have very different bumblebee pollination mechanisms. Tomato and several other solanaceous plants exhibit “buzz” or “pepper pot” pollination, in which foraging bumblebees grasp the fused anther cone and vibrate it to release pollen. Excess pollen provides bumblebees with a protein-rich reward but no nectar reward is provided (Glover et al., 2004). In contrast, the papilionate floral morphology of beans and many other legumes requires, almost exclusively, Hymenopteran pollinators with sufficient weight and strength to mechanically “trip” the hull and wing petals to expose the stamens and pistil. This enables bees to carry out pollination, and access nectar (a rich sucrose source) as the predominant reward (Leppik, 1966; Córdoba and Cocucci, 2011; Bailes et al., 2018). CMV is a cucumovirus with a broad host range encompassing many plant families (Yoon et al., 2019). Contrastingly, BCMV and BCMNV are legume pathogens that belong to one of the largest family of plant-infecting viruses, the Potyviridae (Ivanov et al., 2014), and are unrelated to CMV, which lies within the family Bromoviridae (Palukaitis and García-Arenal, 2003). Thus, we have shown that infection by diverse viruses can alter host VOCs to be more attractive to bees, and that the effect occurs in distantly related plants with distinct pollination mechanisms: bean (this study), and tomato (Groen et al., 2016).
Bumblebees are generalist pollinators. This is made possible by their ability to use several sensory channels to perceive multimodal cues for flower detection such as visual and olfactory stimuli (Katzenberger et al., 2013; Glover, 2014; Lawson et al., 2017) coupled to their learning capacity that allows effective association of particular floral features with nectar and pollen rewards (Cnaani et al., 2006; Gomez et al., 2008; Eisenhardt, 2014; Konzmann and Lunau, 2014). In this study, we have discovered an innate preference of bees for odors emitted from virus-infected bean plants in the absence of visual stimuli. Although this is consistent with the idea that viruses may benefit their plant hosts by encouraging pollinator visits, further studies will show how bee foraging behavior is affected by the combination of visual and olfactory cues. In multimodal experiments, Telles et al. (2017) found that flower color had the strongest effect on bumblebee foraging but that familiar floral scents enhanced the ability of bees to locate flowers with a novel color. Virus-infected plants are often stunted making their flowers less conspicuous, and it is conceivable that odor cues may be important to make them more evident to foragers.
BCMV and CMV induced changes in volatile emission by flowering bean plants that rendered the VOC blend more attractive to bumblebees. Work with yellow-faced bumblebees (Bombus vosnesenskii Radoszkowsk: Byers et al., 2014) and black bean aphids (Aphis fabae Scopoli: Webster et al., 2010) showed that neither are attracted to plants by individual VOCs but rather by specific VOC combinations. Yellow-faced bumblebee workers preferred a combination of limonene, myrcene and (E)-ocimene over any single compound (Byers et al., 2014) while volatiles within a blend that function as host cues to the black bean aphid become non-host cues (or repellent) when presented individually (Webster et al., 2010). Our data on VOC emission from virus-infected bean plants supports the idea that the innate olfactory preferences of insects are driven by VOC blends rather than by single VOCs. Total VOC emission from virus-infected whole plants was roughly half that from healthy plants due in part to the negative effect of virus infection on plant growth, but emission of individual VOCs was also differentially affected at both whole plant and tissue dry weight levels. Two of the most abundant compounds in the VOC blend emitted by flowering bean plants, (E)-ocimene and 4-hexen-1-ol acetate, were significantly lower in all virus-infected plants. Diminishing the proportion of these VOCs in the blend from flowering bean plants is unlikely to account for the innate attraction of bumblebees per se, as the insects found the VOCs from mock-inoculated and BCMNV-infected plants equally attractive in olfactometry tests. The similar trajectories of the loading vectors for the monoterpenes (E)-ocimene and D-linalool (Figure 2) indicate that these VOC outputs are positively correlated and illustrate their closely related biosynthetic pathways derived from geranyl diphosphate (Dudareva and Pichersky, 2000). D-Linalool, which is widespread among floral scents (Knudsen et al., 2006), influences various diurnal (Dötterl and Vereecken, 2010), and nocturnal bees (Krug et al., 2018) and other nocturnal visitors, including moths or bats (Dobson, 2006). However, less D-linalool was emitted by BCMV-infected and CMV-infected plants relative to healthy and BCMNV-infected plants, which is puzzling. However, variation in a few key VOCs in a floral scent blend can profoundly benefit plant competitiveness and reproductive success (Parachnowitsch et al., 2012), which leads us to speculate that (as is the situation for aphid-bean interactions: Webster et al., 2010) it is the blend of VOCs, rather than the amount of specific VOCs, that is most important in driving olfactory attraction.
VOCs emitted by all virus-infected non-flowering plants attracted bumblebees indicating that changes in volatile emission by vegetative tissues provide some olfactory cues in bean-bumblebee interactions. This is consistent with work with CMV-infected tomato plants where bumblebees showed similar innate preferences for flowering and non-flowering plant VOCs (Groen et al., 2016). Although it may seem counterintuitive that bees are attracted to vegetative tissue, Kárpáti et al. (2013) demonstrated that for Manduca sexta, conspecific leaf and flower-derived volatiles combined were more attractive than the flower blend alone from the nectar sources in Nicotiana attenuata and Datura wrightii, suggesting this could be a strategy for foraging insects to optimize food location using odor cues. It is also known that leaf volatiles act in tandem with floral volatiles to attract pollinators from long and short distances. Studies in dwarf palm (Chamaerops humilis) showed that green leaf VOCs attract pollinators over longer distances than floral scents (Caissard et al., 2004; Dufaÿ et al., 2004), and green leaf VOCs may also reinforce floral visual clues (Kunze and Gumbert, 2001; Kulahci et al., 2008). Also, a recent paper has shown that bumblebees can accelerate flowering by interacting with and wounding leaves (Pashalidou et al., 2020). It will be interesting to see if this wounding behavior is influenced by plant VOCs, and if it would be affected by a plant’s infection status, since in nature plants are affected by multiple biotic and abiotic stresses (Gruden et al., 2020).
Finally, revealing further insights into the effects of virus infection on the emission of pollinator-perceivable VOCs by plants may be useful in devising strategies to increase pollination services for crops, and for understanding how pathogens or other stresses may affect pollinated wild plants. Around 87.5% of flowering plants depend upon animal pollinators including many crops (Ollerton et al., 2011), and populations of pollinators, in particular those of bees, are under threat (Potts et al., 2010; Godfray et al., 2015). Studies such as ours on bee-attracting VOCs may aid in improving pollinator service for crops through plant breeding or other methods.
Data Availability Statement
The original contributions presented in the study are included in the article/Supplementary Material, further inquiries can be directed to the corresponding author.
Author Contributions
NM, JCr, AM, FW, and BG conceived the work. NM and JCu collected the data. AM and NC carried out statistical analysis. NM and AM conducted the data analysis. NM, AM, and JCr drafted the manuscript. All authors contributed to manuscript editing and gave final approval for submission.
Funding
This work was supported by the Biotechnology and Biological Sciences Research Council (BBSRC-SCPRID Grant BB/J011762/1 and BBSRC-GCRF Grant BB/P023223/1), the Leverhulme Trust (F/09 741F), Royal Society (FCG\R1\201005), and the Cambridge-Africa ALBORADA Research Fund. FW was supported by a Royal Society-FLAIR Fellowship (FLR\R1\190462). NM was supported by studentships from the Schlumberger Foundation, Cambridge Trust, and Magdalene College Cambridge.
Conflict of Interest
The authors declare that the research was conducted in the absence of any commercial or financial relationships that could be construed as a potential conflict of interest.
Publisher’s Note
All claims expressed in this article are solely those of the authors and do not necessarily represent those of their affiliated organizations, or those of the publisher, the editors and the reviewers. Any product that may be evaluated in this article, or claim that may be made by its manufacturer, is not guaranteed or endorsed by the publisher.
Acknowledgments
We thank Adrienne Pate for expert technical assistance and Sanjie Jiang, and Niels Groen for useful discussions.
Supplementary Material
The Supplementary Material for this article can be found online at: https://www.frontiersin.org/articles/10.3389/fevo.2021.626851/full#supplementary-material
Footnotes
References
Bailes, E. J., Pattrick, J. G., and Glover, B. J. (2018). An analysis of the energetic reward offered by field bean (Vicia faba) flowers: nectar, pollen, and operative force. Ecol. Evol. 8, 3161–3171. doi: 10.1002/ece3.3851
Beale, M. H., Birkett, M. A., Bruce, T. J. A., Chamberlain, K., Field, L. M., Huttly, A. K., et al. (2006). Aphid alarm pheromone produced by transgenic plants affects aphid and parasitoid behavior. Proc. Natl. Acad. Sci. U.S.A. 103, 10509–10513. doi: 10.1073/pnas.0603998103
Beck, J. L., and Vanette, R. L. (2017). Harnessing insect–microbe chemical communications to control insect pests of agricultural systems. J. Agric. Food Chem. 65, 23–28. doi: 10.1021/acs.jafc.6b04298
Burger, H., Dotterl, S., and Ayasse, M. (2010). Host-plant finding and recognition by visual and olfactory floral cues in an oligolectic bee. Funct. Ecol. 24, 1234–1240. doi: 10.1111/j.1365-2435.2010.01744.x
Byers, K. J. P. R., Vela, J. P., Peng, F., Riffell, J. A., and Bradshaw, H. D. (2014). Floral volatile alleles can contribute to pollinator-mediated reproductive isolation in monkeyflowers (Mimulus). Plant J. 80, 1031–1042. doi: 10.1111/tpj.12702
Caissard, J. C., Meekijjironenroj, A., Baudino, S., and Anstett, M. C. (2004). Localization of production and emission of pollinator attractant on whole leaves of Chamaerops humilis (Arecaceae). Am. J. Bot. 91, 1190–1199. doi: 10.3732/ajb.91.8.1190
Carr, J. P., Donnelly, R., Tungadi, T., Murphy, A. M., Jiang, S., Bravo-Cazar, A., et al. (2018). Viral manipulation of plant stress responses and host interactions with insects. Adv. Virus Res. 102, 177–197. doi: 10.1016/bs.aivir.2018.06.004
Chen, C., Song, Q. S., and Proffit, M. (2009). Private channel: a single unusual compound assures specific pollinator attraction in Ficus semicordata. Funct. Ecol. 23, 41–50.
Chittka, L., and Raine, N. E. (2006). Recognition of flowers by pollinators. Curr. Opin. Plant Biol. 9, 428–435. doi: 10.1016/j.pbi.2006.05.002
Chong, J., Wishart, D. S., and Xia, J. (2019). Using metaboanalyst 4.0 for comprehensive and integrative metabolomics data analysis. Curr. Protoc. Bioinformatics 68:e86.
Cnaani, J., Thomson, J. D., and Papaj, D. R. (2006). Flower choice and learning in foraging bumblebees: effects of variation in nectar volume and concentration. Ethology 112, 278–285. doi: 10.1111/j.1439-0310.2006.01174.x
Córdoba, S. A., and Cocucci, A. A. (2011). Flower power: its association with bee power and floral functional morphology in papilionate legumes. Ann. Bot. 108, 919–931. doi: 10.1093/aob/mcr196
Dobson, H. E. M. (2006). “Relationship between floral fragrance composition and type of pollinator,” in Biology of Floral Scent, eds N. Dudareva, E. Pichersky (Boca Raton, FL: CRC Press), 147–198.
Donnelly, R., Cunniffe, N. J., Carr, J. P., and Gilligan, C. A. (2019). Pathogenic modification of plants enhances long−distance dispersal of nonpersistently transmitted viruses to new hosts. Ecology 100:e02725.
Dötterl, S., and Vereecken, N. J. (2010). The chemical ecology and evolution of bee-flower interactions: a review and perspectives. Can. J. Zool. 88, 668–697. doi: 10.1139/Z10-031
Dudareva, N., and Pichersky, E. (2000). Biochemical and molecular genetic aspects of floral scents. Plant Physiol. 122, 627–634. doi: 10.1104/pp.122.3.627
Dudareva, N., and Pichersky, E. (2008). Metabolic engineering of plant volatiles. Curr. Opin. Biotech. 19, 181–189. doi: 10.1016/j.copbio.2008.02.011
Dufaÿ, M., Hossaert-McKey, M., and Anstett, M. C. (2004). Temporal and sexual variation of leaf-produced pollinator-attracting odours in the dwarf palm [published correction appears in Oecologia. 2004 Jul;140(2):379]. Oecologia 139, 392–398.
Dyer, A. G., and Chittka, L. (2004). Fine colour discrimination requires differential conditioning in bumblebees. Naturwissenschaften 91, 224–227. doi: 10.1007/s00114-004-0508-x
Eisenhardt, D. (2014). Molecular mechanisms underlying formation of long-term reward memories and extinction memories in the honeybee (Apis mellifera). Learn. Mem. 21, 534–542. doi: 10.1101/lm.033118.113
Elisante, F., Ndakidemi, P., Arnold, S. E. J., Belmain, S. R., Gurr, G. M., Darbyshire, I., et al. (2020). Insect pollination is important in a smallholder bean farming system. PeerJ 8:e10102. doi: 10.7717/peerj.10102
Foster, J. J., Sharkey, C. R., Gaworska, A. W. A., Roberts, N. W., Whitney, H. M., and Partridge, J. C. (2014). Bumblebees learn polarization patterns. Curr. Biol. 24, 1415–1420. doi: 10.1016/j.cub.2014.05.007
Ghazoul, J. (2006). Floral diversity and the facilitation of pollination. J. Ecol. 94, 295–304. doi: 10.1111/j.1365-2745.2006.01098.x
Glover, B. J. (2014). Understanding Flowers and Flowering: An Integrated Approach, 2nd Edn. Oxford: Oxford University Press.
Glover, B. J., Bunnewell, S., and Martin, C. (2004). Convergent evolution within the genus Solanum: the specialised anther cone develops through alternative pathways. Gene 331, 1–7. doi: 10.1016/j.gene.2004.01.027
Godfray, H. C. J., Blacquière, T., Field, L. M., Hails, R. S., Potts, S. G., Raine, N. E., et al. (2015). A restatement of recent advances in the natural science evidence base concerning neonicotinoid insecticides and insect pollinators. Proc. R. Soc. B 282:20151821. doi: 10.1098/rspb.2015.1821
Gomez, J. M., Bosch, J., Perfectti, F., Fernández, J., Abdelaziz, M., and Camacho, J. (2008). Association between floral traits and rewards in Erysimum mediohispanicum (Brassicaceae). Ann. Bot. 101, 1413–1420. doi: 10.1093/aob/mcn053
Granero, A. M., Sanz, J. M., Gonzalez, F. J. E., Vidal, J. L. M., Dornhaus, A., Ghani, J., et al. (2005). Chemical compounds of the foraging recruitment pheromone in bumblebees. Naturwissenschaften 92, 371–374. doi: 10.1007/s00114-005-0002-0
Groen, S. C., Westwood, J. H., Jiang, S., Murphy, A. M., Davey, M. P., Bruce, T. J. A., et al. (2016). Virus infection of plants alters pollinator preference: a payback mechanism for susceptible hosts? PLoS Pathog. 12:e1005790. doi: 10.1371/journal.ppat.1005790
Gruden, K., Lidoy, J., Petek, M., Podpe, V., Flors, V., Papadopoulou, K. K., et al. (2020). Menage a trois: unraveling the mechanisms regulating plant-microbe-arthropod interactions. Trends Plant Sci. 25, 1215–1226. doi: 10.1016/j.tplants.2020.07.008
Hossaert-McKey, M., Soler, C., Schatz, B., and Proffit, M. (2010). Floral scents: their roles in nursery pollination mutualisms. Chemoecology 20, 75–88. doi: 10.1007/s00049-010-0043-5
Ibarra-Perez, F. J., Barnhart, D., Ehdaie, B., Knio, K. M., and Waines, J. G. (1999). Effects of insect tripping on seed yield of common beans. Crop Sci. 39, 428–433. doi: 10.2135/cropsci1999.0011183x0039000200022x
Ivanov, K. I., Eskelin, K., Löhmus, A., and Mäkinen, K. (2014). Molecular and cellular mechanisms underlying potyvirus infection. J. Gen. Virol. 95, 1415–1429. doi: 10.1099/vir.0.064220-0
Junker, J. J., Kuppler, J., Amo, L., Blande, J. D., Borges, R. M., van Dam, N. M., et al. (2017). Covariation and phenotypic integration in chemical communication displays: biosynthetic constraints and eco-evolutionary implications. New Phytol. 220, 739–749. doi: 10.1111/nph.14505
Kárpáti, Z., Knaden, M., Reinecke, A., and Hansson, B. S. (2013). Intraspecific combinations of flower and leaf volatiles act together in attracting hawkmoth pollinators. PLoS One 8:e72805. doi: 10.1371/journal.pone.0072805
Katzenberger, T. D., Lunau, K., and Junker, R. R. (2013). Salience of multimodal flower cues controls bumblebee’s initial responses and learning behaviour. Behav. Ecol. Sociobiol. 67, 1587–1599. doi: 10.1007/s00265-013-1570-1
Kingha, B. M. T., Fohouo, F.-N. T., Ngakou, A., and Brückner, D. (2012). Foraging and pollination activities of Xylocopa olivacea (Hymenoptera, Apidae) on Phaseolus vulgaris (Fabaceae) flowers at Dang (Ngaoundere-Cameroon). J. Agric. Ext. Rural Dev. 4, 330–339.
Klatt, B. K., Burmeister, C., Westphal, C., Tscharntke, T., and von Fragstein, M. (2013). Flower volatiles, crop varieties and bee responses. PLoS One 8:e72724. doi: 10.1371/journal.pone.0072724
Klatt, B. K., Holzschuh, A., Westphal, C., Clough, Y., Smit, I., Pawelzik, E., et al. (2014). Bee pollination improves crop quality, shelf life and commercial value. Proc. Roy. Soc. B. 281:20132440. doi: 10.1098/rspb.2013.2440
Klein, A. M., Vaissiere, B. E., Cane, J. H., Steffan-Dewenter, I., Cunningham, S. A., Claire Kremen, C., et al. (2007). Importance of pollinators in changing landscapes for world crops. Proc. R. Soc. B 274, 303–313. doi: 10.1098/rspb.2006.3721
Knight, T. M., Steets, J. A., Vamosi, J. C., Mazer, S. J., Burd, M., Campbell, D. R., et al. (2005). Pollen limitation of plant reproduction: pattern and process. Ann. Rev. Ecol. Evol. Syst. 36, 467–497. doi: 10.1146/annurev.ecolsys.36.102403.115320
Knudsen, J. T., Eriksson, R., Gershenzon, J., and Ståhl, B. (2006). Diversity and distribution of floral scent. Bot. Rev. 72, 1–120. doi: 10.1663/0006-8101(2006)72[1:dadofs]2.0.co;2
Konzmann, S., and Lunau, K. (2014). Divergent rules for pollen and nectar foraging bumblebees – a laboratory study with artificial flowers offering diluted nectar substitute and pollen surrogate. PLoS One 9:e91900. doi: 10.1371/journal.pone.0091900
Krug, C., Cordeiro, G. D., Schäffler, I., Silva, C. I., Oliveira, R., Schlindwein, C., et al. (2018). Nocturnal bee pollinators are attracted to Guarana flowers by their scents. Front. Plant Sci. 9:1072. doi: 10.3389/fpls.2018.01072
Kulahci, I. G., Dornhaus, A., and Papaj, D. R. (2008). Multimodal signals enhance decision making in foraging bumble-bees. Proc. R. Soc. B 275, 797–802. doi: 10.1098/rspb.2007.1176
Kunze, J., and Gumbert, A. (2001). The combined effect of color and odor on flower choice behavior of bumblebees in flower mimicry systems. Behav. Ecol. 12, 447–456. doi: 10.1093/beheco/12.4.447
Lawson, D. A., Whitney, H. M., and Rands, S. A. (2017). Nectar discovery speeds and multimodal displays: assessing nectar search times in bees with radiating and non-radiating guides. Evol. Ecol. 31, 899–912. doi: 10.1007/s10682-017-9916-1
Leppik, E. E. (1966). Floral evolution and pollination in the Leguminosae. Ann. Bot. Fenn. 3, 299–308.
Lunau, K. (1992). Innate recognition of flowers by bumblebees: orientation of antennae to visual stamen signals. Can. J. Zool. 70, 2139–2144. doi: 10.1139/z92-288
Mauck, K. E., De Moraes, C. M., and Mescher, M. C. (2010). Deceptive chemical signals induced by a plant virus attract insect vectors to inferior hosts. Proc. Natl. Acad. Sci. U.S.A. 107, 3600–3605. doi: 10.1073/pnas.0907191107
Mauck, K. E., Moraes, C. M., and Mescher, M. C. (2016). Effects of pathogens on sensory-mediated interactions between plants and insect vectors. Curr. Opin. Plant Biol. 32, 53–61. doi: 10.1016/j.pbi.2016.06.012
Morales, F. J. (2006). “Common beans,” in Natural Resistance Mechanisms of Plants to Viruses, eds G. Loebenstein and J. P. Carr (The Netherlands: Springer), 367–382. doi: 10.1007/1-4020-3780-5_16
Ollerton, J., Winfree, R., and Tarrant, S. (2011). How many flowering plants are pollinated by animals? Oikos 120, 321–326. doi: 10.1111/j.1600-0706.2010.18644.x
Palukaitis, P., and García-Arenal, F. (2003). Cucumoviruses. Adv. Virus Res. 62, 241–323. doi: 10.1016/s0065-3527(03)62005-1
Parachnowitsch, A. L., Raguso, R. A., and Kessler, A. (2012). Phenotypic selection to increase floral scent emission, but not flower size or colour in bee-pollinated Penstemon digitalis. N. Phytol. 195, 667–675. doi: 10.1111/j.1469-8137.2012.04188.x
Pashalidou, F. G., Lambert, H., Peybernes, T., Mescher, M. C., and De Moraes, C. M. (2020). Bumble bees damage plant leaves and accelerate flower production when pollen is scarce. Science 368, 881–884.
Potts, S. G., Biesmeijer, J. C., Kremen, C., Neumann, P., Schweiger, O., and Kunin, W. E. (2010). Global pollinator declines: trends, impacts and drivers. Trends Ecol. Evol. 25, 345–353. doi: 10.1016/j.tree.2010.01.007
Raguso, R. A. (2008). Wake up and smell the roses: the ecology and evolution of floral scent. Ann. Rev. Ecol. Evol. Syst. 39, 549–569. doi: 10.1146/annurev.ecolsys.38.091206.095601
Ramos, D. L., Bustamante, M. M. C., Silva, F. D., and Carvalheiro, L. G. (2018). Crop fertilization affects pollination service provision – common bean as a case study. PLoS One 13:e0204460. doi: 10.1371/journal.pone.0204460
Rizopoulos, D. (2021). GLMMadaptive: Generalized Linear Mixed Models using Adaptive Gaussian Quadrature. R Package Version 0.8-0. Available online at: https://CRAN.R-project.org/package=GLMMadaptive (accessed August 5, 2021).
Song, B., Chen, G., and Stöcklin, J. (2014). A new pollinating seed-consuming mutualism between Rheum nobile and a fly fungus gnat, Bradysia sp., involving pollinator attraction by a specific floral compound. N. Phytol. 203, 1109–1118. doi: 10.1111/nph.12856
Suchet, C., Dormont, L., Schatz, B., Giurfa, M., Simon, V., Raynaud, C., et al. (2011). Floral scent variation in two Antirrhinum majus subspecies influences the choice of naive bumblebees. Behav. Ecol. Sociobiol. 65, 1015–1027. doi: 10.1007/s00265-010-1106-x
Telles, F. J., Corcobado, G., Trillo, A., and Rodríguez-Gironés, M. A. (2017). Multimodal cues provide redundant information for bumblebees when the stimulus is visually salient, but facilitate red target detection in a naturalistic background. PLoS One 12:e0184760. doi: 10.1371/journal.pone.0184760
Thompson, J. R., Langenhan, J. L., Fuchs, M., and Perry, K. L. (2015). Genotyping of Cucumber mosaic virus isolates in western New York State during epidemic years: characterization of an emergent plant virus population. Virus Res. 210, 169–177. doi: 10.1016/j.virusres.2015.07.028
Wamonje, F. O., Donnelly, R., Tungadi, T. D., Murphy, A. M., Pate, A. E., Woodcock, C., et al. (2020). Different plant viruses induce changes in feeding behavior of specialist and generalist aphids on common bean that are likely to enhance virus transmission. Front. Plant Sci. 10:1811. doi: 10.3389/fpls.2019.01811
Webster, B., Bruce, T., Dufour, S., Birkemeyer, C., Birkett, M., Hardie, J., et al. (2008). Identification of volatile compounds used in host location by the black bean aphid, Aphis fabae. J. Chem. Ecol. 34, 1153–1161. doi: 10.1007/s10886-008-9510-7
Webster, B., Bruce, T., Pickett, J., and Hardie, J. (2010). Volatiles functioning as host cues in a blend become nonhost cues when presented alone to the black bean aphid. Anim. Behav. 79, 451–457. doi: 10.1016/j.anbehav.2009.11.028
Whitney, H. M., Chittka, L., Bruce, T. J. A., and Glover, B. J. (2009). Conical epidermal cells allow bees to grip flowers and increase foraging efficiency. Curr. Biol. 19, 948–953. doi: 10.1016/j.cub.2009.04.051
Worrall, E. A., Wamonje, F. O., Mukeshimana, G., Harvey, J. J. W., Carr, J. P., and Mitter, N. (2015). Bean common mosaic virus and bean common mosaic necrosis virus: relationships, biology, and prospects for control. Adv. Virus Res. 93, 1–46. doi: 10.1016/bs.aivir.2015.04.002
Yoon, J.-Y., Palukaitis, P., and Choi, S.-K. (2019). “Host range,” in Cucumber Mosaic Virus, eds P. Palukaitis and F. García-Arenal (St Paul, MN: American Phytopathological Society), 15–18.
Zaiontz, C. (2020). Real Statistics Resource Pack Software (Release 7.6) [Computer Software]. Available online at: https://www.real-statistics.com/ (accessed August 5, 2021).
Keywords: bumblebee, cucumovirus, innate preference, pollination, potyvirus, semiochemical, trade-off
Citation: Mhlanga NM, Murphy AM, Wamonje FO, Cunniffe NJ, Caulfield JC, Glover BJ and Carr JP (2021) An Innate Preference of Bumblebees for Volatile Organic Compounds Emitted by Phaseolus vulgaris Plants Infected With Three Different Viruses. Front. Ecol. Evol. 9:626851. doi: 10.3389/fevo.2021.626851
Received: 10 November 2020; Accepted: 31 August 2021;
Published: 20 September 2021.
Edited by:
Dani Lucas-Barbosa, University of Zurich, SwitzerlandReviewed by:
Aphrodite Kantsa, ETH Zürich, SwitzerlandQuint Rusman, University of Zurich, Switzerland
Copyright © 2021 Mhlanga, Murphy, Wamonje, Cunniffe, Caulfield, Glover and Carr. This is an open-access article distributed under the terms of the Creative Commons Attribution License (CC BY). The use, distribution or reproduction in other forums is permitted, provided the original author(s) and the copyright owner(s) are credited and that the original publication in this journal is cited, in accordance with accepted academic practice. No use, distribution or reproduction is permitted which does not comply with these terms.
*Correspondence: John P. Carr, anBjMTAwNUBjYW0uYWMudWs=