- 1Centre for Environmental and Climate Science, Lund University, Lund, Sweden
- 2Department of Biology, Lund University, Lund, Sweden
Ecological Focus Areas (EFAs) to benefit biodiversity became mandatory in intensively farmed landscapes after the reform of the European Common Agricultural Policy (CAP) in 2013. The implementation of EFAs as uncropped field margins has been criticized as ineffective but created a window of opportunity to test if augmenting them with annual flower strips can benefit biodiversity. In this study, we investigated if annual flower strips on EFAs benefited functional biodiversity in intensively farmed landscapes. To this end we established eleven annual flower strips with a seed mixture targeted for both natural enemies and pollinators, on areas were farmers had planned for EFAs. We determined effects on aphids and their natural enemies in cereal fields close to six of the flower strips, and for solitary bees and wasp close to and in the surroundings of all eleven flower strips. We found that annual flower strips benefited the abundance of hoverfly larvae and possibly also that of solitary bees. However, there were neither any significant effects on natural enemies (other than hoverfly larvae), nor any difference in natural pest control as shown by lack of differences in aphid numbers and parazitation rates. Abundances of solitary bees and wasps in the surrounding landscapes were unaffected, although there was a tendency for more solitary bee cells closer to the strips. We suggest that the critical issue leading to the mostly negative results is the lack of permanent structures to sustain populations of arthropods that in turn can benefit from annual flower strips. Hence, future agri-environmental policies need to carefully consider if and how annual agri-environmental measures should be implemented in intensively managed agricultural landscapes, e.g., by combining them with more permanent structures.
Introduction
Flower strips are often proposed as a tool to mitigate biodiversity loss in agricultural landscapes (Haaland et al., 2011), especially loss of functional biodiversity such as pollinators (Scheper et al., 2013) and natural enemies of pests (Holland et al., 2016). Supporting biodiversity-related ecosystem services has the potential to increase agricultural production while minimizing negative environmental impacts (Bommarco et al., 2013). Flower strips can be targeted for pollinators (Wood et al., 2015), natural enemies (Tschumi et al., 2015), both pollinators and natural enemies (Campbell et al., 2017; Grab et al., 2018) or serve different purposes (Vickery et al., 2002). Local factors, including plant community composition, size and shape of strips, configuration (e.g., field edge or field interior), can have important positive or negative effects on flower strips’ capacity to support functional biodiversity and ecosystem services in croplands (Haenke et al., 2009; Jönsson et al., 2015; Uyttenbroeck et al., 2015; Wood et al., 2016). However, the consequences of agri-environmental measures such as flower strips, on functional diversity may be context dependent, and related to the agricultural intensity in the surrounding landscape (see e.g., Tscharntke et al., 2005, 2016; Bianchi et al., 2006; Scheper et al., 2013; Grab et al., 2018). Thus, the re-occurring critique toward the European Union’s (EU), Common Agricultural Policy (CAP), that measures need to be targeted (e.g., Smith et al., 2010; Batáry et al., 2015; Pe’er et al., 2019) and based on a landscape perspective (see e.g., Tscharntke et al., 2005; Batary et al., 2011; Kleijn et al., 2011), applies also to flower strips.
In an attempt in the reform 2013 to make the CAP “greener,” and with the objective to safeguard and improve farmland biodiversity in Europe, for many farmers so called Ecological Focus Areas (EFA) became mandatory (through Pillar 1) in 2015 (while the budget for the agri-environmental schemes in Pillar II decreased) (EU, 2013). As one out of three “greening measures” (the others being crop diversification and maintaining permanent grassland at a national level), EFAs were supposed to be simple, generalized, non-contractual, and annual, and can consist of areas such as nitrogen fixing crops and uncropped field margins (EU, 2013). EFAs in the form of uncropped field margins has been criticized as inefficient (Nilsson et al., 2019), but also created a window of opportunity for annual flower strips and are encouraged to be used for such (e.g., by the Swedish Board of Agriculture). The question is what kind of effects that can be expected on farmland biodiversity from these annual strips.
Even though evidence do exist for the potential of flower strips and other non-crop habitat to have positive effects on farmland biodiversity and ecosystem services (Dicks et al., 2014, but see Zamorano et al., 2020), there are many factors that influence the magnitude and direction of these effects (Tscharntke et al., 2016; Karp et al., 2018). Most of the existing knowledge comes from perennial or multi-annual flower strips (see e.g., Jönsson et al., 2015; Wood et al., 2016), whereas little is known about the effects of annual flower strips. Exceptions to this are two studies in Switzerland showing local positive effects of targeted annual flower strips on natural enemies (Tschumi et al., 2015, 2016) and one study in Sweden showing local positive effects on species richness of pollinators (Rundlöf et al., 2018). In the two studies from Switzerland, the landscapes were traditional Swizz agricultural landscapes characterized by a small-scaled mosaic of crop fields. The positive effects in the Swedish study were strongest in the more heterogenous landscapes (Rundlöf et al., 2018).
Given the lack of scientific foundation on the use of annual flower strips on EFAs to benefit functional biodiversity, we in this study aimed to evaluate if they benefited natural enemies and pollinators in the surrounding landscape. Given that EFAs are mandatory mostly in intensively farmed landscapes, and that benefit of functional biodiversity for cash crops is most relevant in these landscapes (Nilsson et al., 2019), we evaluated the effect using experimental implementation of annual flower strips in existing EFAs in replicated simple landscapes. However, to determine if the amount of permanent structures in these landscapes modify responses to annual flower strips, we also analyzed effects of limited variation in the proportion of arable fields in these landscapes. In this way we addressed the following research questions: (I) Do EFAs in the form of annual flower strips have a positive effect on the abundance of natural enemies in adjacent fields? (II) Is there any resulting effect on pest abundance? (III) Do annual flower strips have a positive effect on the reproductive output of adjacently nesting solitary bees and wasps?
Materials and Methods
Experimental Design
The study was conducted as an experimental study in the southernmost part of the county Skåne, in southern Sweden, within the most intensive “production area” (Production area GSS). This is one of the most intensively farmed areas in Sweden, characterized by homogeneous landscapes with large crop fields and specialized high intensity production of cash crops. To select EFAs for experimental implementation of flower strips, we focused on large farms. From a complete list, we randomly contacted farms that were >400 ha in size until we had enough participating farms to create 13 flower strips. The average field size in a radius of 1,000 m around the flower strips were 4.6 ha. The conditions for inclusion were that farms should have EFAs in the form of uncropped field margins as part of their management plan and be prepared to establish flower strips on these (Figure 1). We also made sure that a flower strip could be established without any other flower strips or late mass-flowering crops (e.g., red clover seed production, Trifolium pratense) within 1,000 m. Two farms were sufficiently large to allow the establishment of two and three flower strips, respectively, with sufficient distances between them to assume that they were independent (>10 km). At the rest of the farms, only one flower strip was established on each. To maintain realism, the flower strips were sown where the farmers had already planned for EFAs and the study design was adapted accordingly. All EFAs, and thus the flower strips, were placed on fields with sugar beet, onion and oil seed rape. Because of differences in the assumed scale of effect of flower strips, we studied pollinators and natural enemies in this system using slightly different designs. For natural enemies, we established control sites within the same fields, but at a distance from the flower strips. For pollinators we used transects that extended away from the flower strips, assuming the influence of the flower strips to decline along the transects.
Because the aim of the study was to explore the potential of annual flower strips to enhance natural pest control and conditions for both natural enemies and pollinators, we produced a seed mixture of plant species with the aim to attract both these groups (Table 1). By using a functionally diverse plant species composition the aim was to attract a diversity of nectar and pollen eating organisms (Balzan et al., 2014). The plant species were selected based on existing evidence for plants supporting pollinating insects [bees (Apoidea) and hoverflies (Diptera: Syrphidae)] and key natural enemies of aphids and other pests in cereals [hoverfly larvae, lacewings (Neuroptera: Chrysopidae), ladybirds (Coleoptera: Coccinellidae), as well as parasitic wasps (Hymenoptera)] (Vattala et al., 2006; Lixa et al., 2010; Wäckers and van Rijn, 2012; Tschumi et al., 2015). Since the abundance of ground dwelling predators was expected to benefit from the vegetation cover and refuge area provided by the strips rather than the exact flower composition (Lee et al., 2001; Balzan et al., 2016), we did not tailor the composition of flower strips to them. There is not much information available about specific legumes, but in general they (Fabaceae or Trifolium spp.) are attractive to bees (Carvell et al., 2006; Gardiner et al., 2008) and the three legume species used were suitable also because they flower later than and have different heights compared to Phacelia. The three Trifolium species used in the mixture were suggested by Lindström (2010) and T. resupinatum was together with Phacelia tanacetifolia the most attractive plant species for bees in Eriksson and Rundlöf (2013). For exact quantities of seeds in the seed mixture see Supplementary Table 1.
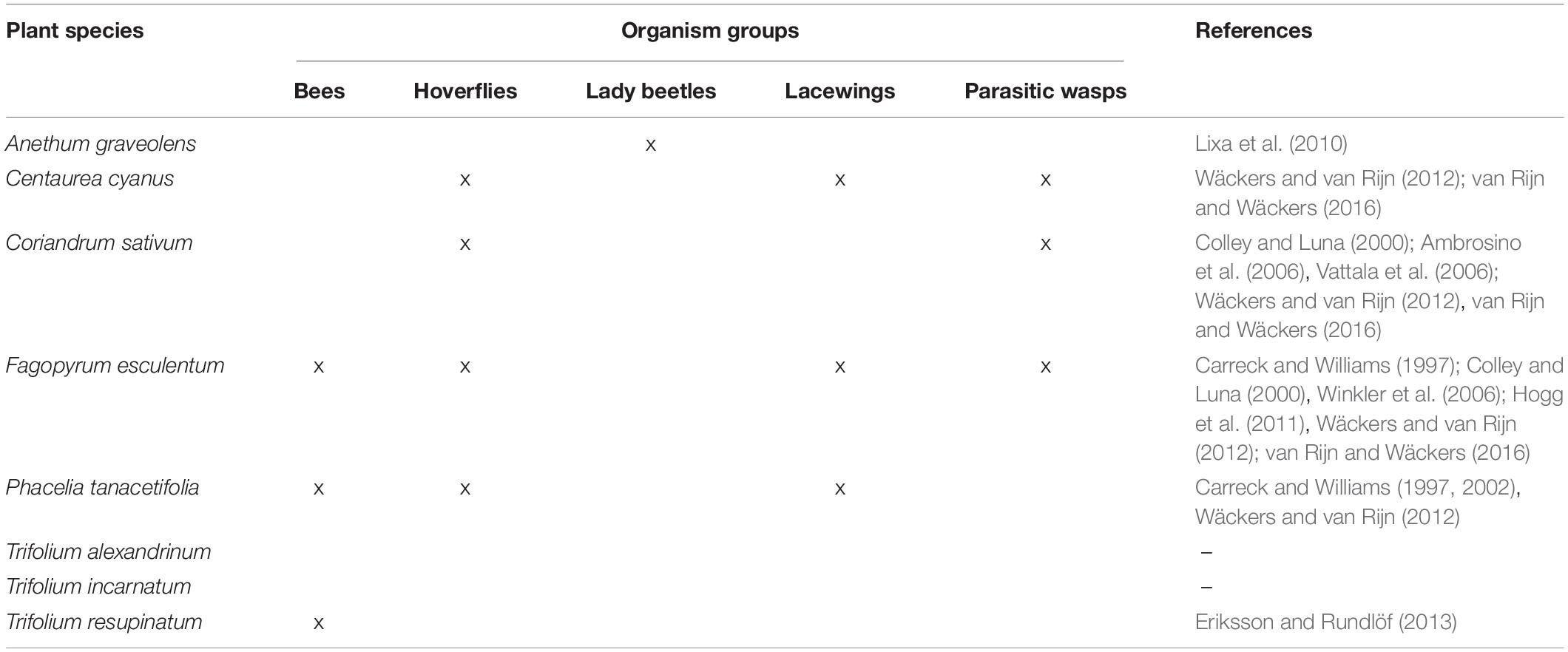
Table 1. Plants in the flower strips and evidence that they attract pollinators and natural enemies (marked with “x”).
Flower strips were sown at the end of April until the beginning of May 2016. The width and length of strips were adapted to the management plans at the different farms, respectively (Supplementary Table 2). In total thirteen flower strips were sown. Two of the strips were later excluded from the study; in one case the flower strip never established probably because of too dry conditions and in the second case the farmer sowed mass flowering crops (field bean and flax seed) next to strips after crop failure in the adjacent cereal fields.
The landscapes within a 1,000 m radius around the flower strips were characterized using digital land-use data from the Integrated Administration and Control System (IACS) provided by the Swedish Board of Agriculture. A radius of 1,000 m was considered appropriate for the type of organisms that were to be investigated (cf. Thies et al., 2003; Greenleaf et al., 2007). To describe the landscape surrounding the flower strips we used the proportions of arable fields, a simple but widely used proxy for land use intensity studies of biodiversity in agricultural landscapes (e.g., Tscharntke et al., 2005; Persson et al., 2010). For the selected flower strip sites, the proportion of agricultural land varied between 75 and 95%. We did not include ley (i.e., grassland on arable land with or without legumes, which usually has a life-length of 2–4 years) when calculating the proportion of arable land, as we considered ley to have the potential to provide resources for all of the studied groups (Weibull et al., 2003; Persson and Smith, 2013; Rusch et al., 2014).
Sampling of Arthropods
Natural Enemies and Pests
We sampled natural enemies and aphids using three different methods: tiller counts, suction sampling and pitfall traps. Parasitoids and predatory larvae were sampled through standardized suction sampling at 5 and 40 m from the field edges, the 5–8 of July. We placed a metal cylinder with a diameter of 30 cm at the ground and sampled within it to make sure that the area sampled always had the same dimensions and were running the suction sampler for 20 × 3 s to cover the whole area. Suction sampling took place in fair weather, i.e., not in strong winds or in temperatures below 15°C, and never directly after rain, to assure dry conditions in the fields. Ground beetles, rove beetles and spiders were sampled using pitfall traps. One pitfall trap was placed in the flower strip/field margin and then at 5, 15, and 40 m from the field edge. The traps were left for 1 week and emptied at three occasions from end of June to mid-July. The traps were moved slightly each time they had been emptied. Aphids, mummies of aphids (parasitized aphids), and hoverfly larvae were sampled from wheat tillers. Along the transects, we randomly picked 25 tillers at each of the distances 5, 15, and 40 m from the field edge, the 23–24 of June. Hoverfly larvae were on top of being included in the group with predatory larvae also assessed separately as they were considered particularly interesting because of the adults flies explicit relationship to flower strips (Haenke et al., 2009).
Six of the EFAs (Supplementary Table 2) were on fields adjacent to cereal fields, allowing us to assess the abundance of natural enemies and aphids. At each site, we established two pairs of transects perpendicular to the flower strip (in one case the transects started at the end of the flower strip and went into two different fields instead) and a semi-natural field border, respectively. By having transects adjacent to flower strips and uncultivated field borders within the same field, we attempted to control for between-field differences in crop (wheat/rye) and management. The control transects were mostly on the opposite side of the field compared to the flower strip transects. Transects started close to the flower strip/uncultivated field border and extended 40 m into the field, such that any part of them where never closer to another transect than 200 m and control transects never closer to flower strips than 200 m. One site only had one transect of each treatment. All sites had all the transects in one or two winter wheat fields, except for one site were one pair of transects (one treatment and one control) were in a winter rye field. The placement of flower strips on existing EFAs determined by farmers’ choice, as part of our strive for policy relevance, led to the presence of an access road, and for three transects a small low-traffic asphalt road, between the flower strips and the focal winter cereal fields. For the study of natural enemies and aphids, the proportion of crop land within 1,000 m from transects, varied between 75 and 95%. By agreement with farmers, pesticide free zones (50 × 20 m) surrounded our transects.
Solitary Bees and Wasps
To assess effects of flower strips on nesting solitary bees and wasps, we used trap nests placed in or in the immediate proximity to all the eleven flower strips and at four distances from them; 200–400 m which could be expected to be in the range of some trap nesting bees and wasps, 600–800 m which could be expected to be out of range for most and, >1,000 m (in one case 966 m) which is outside known foraging range for most trap nesting individuals (Gathmann and Tscharntke, 2002; Holzschuh et al., 2009; Zurbuchen et al., 2010; Hofmann et al., 2020). We placed trap nests in or adjacent to all 11 flower strips, and then in field edges at four different distances (approximately 200–400, 600–800, and >1,000 m from the strip), during three consecutive weeks from June 22nd in 2016 (when at least one of the plant species in a strip was at peak flowering). At one farm, the trap nests at the distance 600–800 m went missing. At each distance, we placed three trap nests. To attract a variety of species, they were of two types: two trap-nests at each distance from the flower strip were filled with reed with a diameter of 2–5 mm to provide nesting sites for small solitary bees and wasps and one trap-nest at each distance was filled with paper tubes with a diameter of 7 and 9 mm to provide nesting sites for intermediate and large solitary bees and wasps. The trap nests were collected in fall, nests and cells counted and the cells identified to taxa or genus. For the trap nests, 51–98% of the surrounding landscape (radius 1,000 m) consisted of arable fields. Since the flower strips differed in size, we also calculated the area flower strip within 1,000 m from each trap nest location. For the trap nests placed within the flower strip or in the immediate proximity this area varied between 509 and 38,310 m2 (mean: 7,865 m2). The trap nests were placed next to commercial bumble bee colonies that were used in another study (Klatt et al., 2020) (see section “Discussion”).
Statistical Analysis
To test the effects of flower strips on natural enemies and aphids in fields adjacent to flower strips, we used generalized linear mixed-effect models (GLMMs) [glmer-function in Package lme4; (Bates et al., 2015)]. Individual models were created for different organism groups, with the response variables: number of aphids, proportion of aphids parasitized (aphids in the form of mummies), number of hoverfly larva, number of parasitic wasps, number of predatory larva (Neuroptera larva, Coccinellidae larva, predatory beetle larva, and hoverfly larva) and number of adult predators (Carabidae, Staphylinidae, and Araneae pooled over three sampling rounds). Poisson error distribution was used for all models except for the proportion of mummies, which was analyzed assuming binomial distribution. All full models included the fixed effects treatment (flower strip or control), distance from field edge and the interaction between these, as well as the covariate proportion arable fields within 1,000 m. Likelihood-ratio tests were used to assess the impact of the fixed effects, following recommendations by Zuur et al. (2009). If the interaction between distance and treatment was not significant, it was dropped from the model and distance and treatment effects were evaluated without the interaction. For models based on data where more than two distances from the field edge had been sampled (pitfall traps and tillers), distance was handled as a continuous variable. In the models based on data from suction sampling, where only data from two distances were available, distance was handled as a categorical variable.
In the models based on data from the tillers and pitfall traps, offsets (logged) were included to account for different number of tillers sampled (due to loss of samples) and different number of days pitfall traps had been open (due to destroyed traps and weather conditions). An offset was also included in the model for parasitic wasps to account for different number of transects, since transects in same field were pooled due to low number of parasitic wasps in the samples. An observation level random effect was used if the model showed overdispersion. The random structure followed the study design such that transects were nested within treatment and treatment within site. We used likelihood ratio tests to compare models with a random slope and intercept and with only random intercept, respectively (Zuur et al., 2009). If they did not differ significantly, we assumed no random variation in slopes across position in transects and for simplicity used models with only random intercepts. For the natural enemies, variance components for fields or transects were estimated as zero.
To test the effects of the flower strips on solitary bees and wasps, we used generalized mixed effect models (GLMMs) [glmmTMB-function in Package glmmTMB; (Brooks et al., 2017)]. We were interested in if and how the possibility for reproduction for the solitary bees and wasps, respectively, were affected by the presence of a flower strip. In the analysis, we therefore chose to consider only number of cells and did not analyze the number of nests occupied, because bees and wasps of different species may use different number of tubes to lay their eggs in such that this measure is not necessarily a good estimator of reproductive output. Due to low numbers of cells from individual species or families, we pooled the different taxa into two groups: solitary bees and solitary wasps. Models with number of cells per distance as the response variable were fitted assuming a negative binomial distribution to account for over-dispersion. We used two different models with different fixed effects, the full models included either the fixed effect distance from flower strip or area of flower strip within 1,000 m from the trap nest, as well as the covariate proportion arable fields. The models for bees and wasps included the random intercept site. Adding random slopes to these models, resulted in failure to converge.
We checked that residuals of all models (full models and individual fixed effects) showed homogenous variance and followed the assumed distribution (“simulateResiduals”-function; package DHARMa; Hartig, 2018). GLMM models were also checked for over/under dispersion (“testDispersion”-function; package: DHARMa) (Hartig, 2018) and zero-inflation (“testZeroinflation”-function; package: DHARMa) (Hartig, 2018).
We assed collinearity among explanatory variables using pairwise scatterplots, correlation coefficients and variance inflation factors (VIF), with a threshold of 2 for the VIF (Zuur et al., 2009, 2010). To avoid numerical precision problems, all fixed numerical explanatory variables were standardized (mean of zero and standard deviation of one). All data were analyzed in R 3.6.0 (R Core Team, 2019).
Results
Natural Enemies and Pests in Winter Wheat Fields
Flower strips significantly affected hoverfly larvae abundance (Table 2), which tended to increase with distance to the field edge in the presence of flower strips but decline if a flower strips was lacking. However, this effect was weak (Figure 2). There was also a non-significant tendency that flower strips affected parasitic wasps, with wasp abundance decreasing with distance to the field edge in control sites (Table 2 and Figure 3). Aphids and the proportion mummified aphids were not affected by neither the treatment nor the distance, nor their interaction (Table 2). For the predatory larvae overall, neither the treatment nor the interaction between treatment and distance explained their abundances. However, there were more predatory larvae 40 m from the field edge compared to five m from the field edge independent on the presence of a flower strip (Table 2 and Figure 3). For ground dwelling predators, abundance was not explained by treatment, distance or their interaction (Table 2), they, however, tended to be more abundant further into the fields than to the field edges (Figure 3). The proportion agricultural land did not relate to the abundance of any of the groups (Table 2).
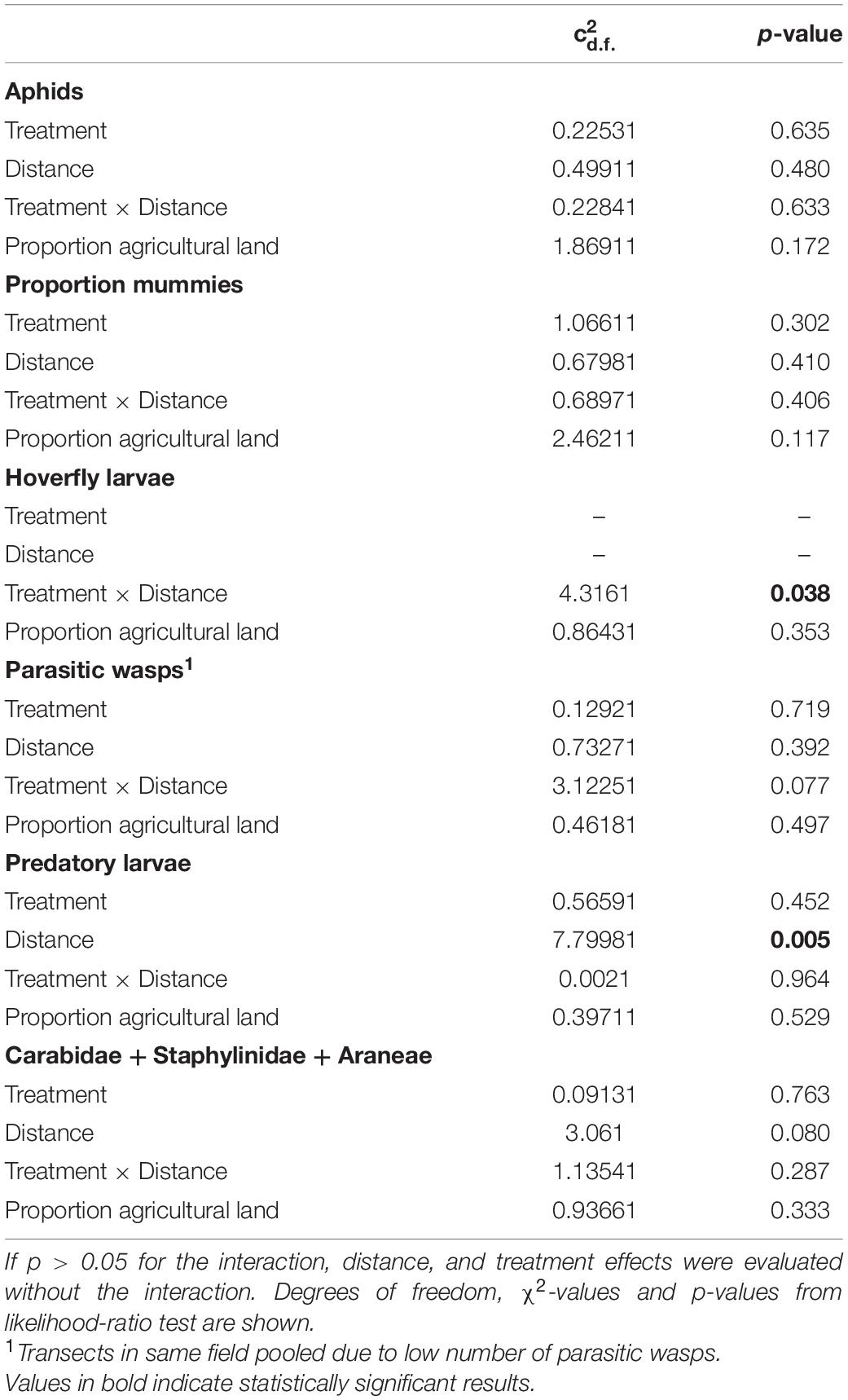
Table 2. Summary of main fixed effects, treatment (flower strip or control, i.e., normal field margin), distance to field edge and their interaction, on abundances of aphids, proportion of mummies, and abundances of the different groups of natural enemies.
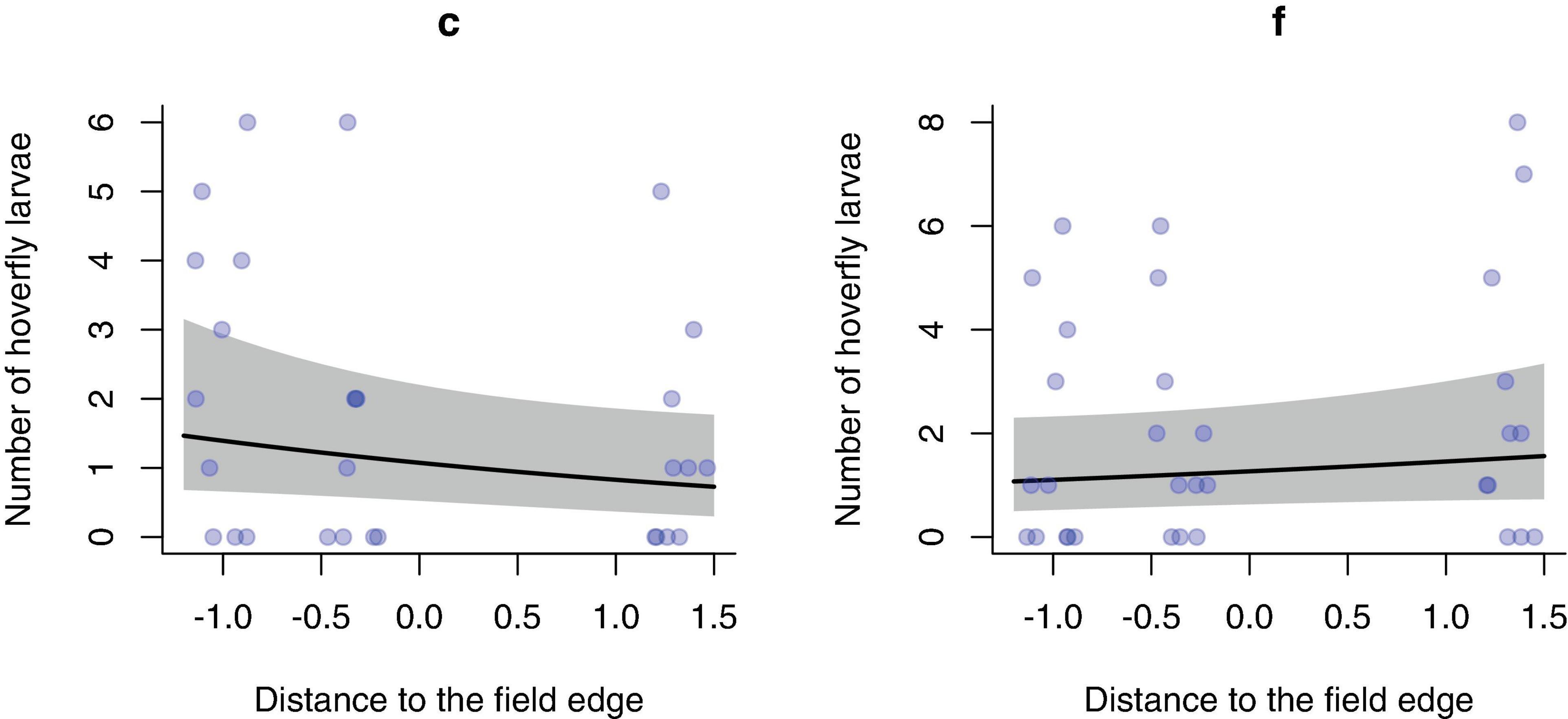
Figure 2. Effects of distance to field edge and treatment for hoverfly larvae. The distances are standardized in the figure, in reality they were 5, 15, and 40 m. There was a weak interactive effect where the number of hoverfly larvae tended to decrease with larger distance from the field edge in control sites (c) and increase in flower strip sites (f).
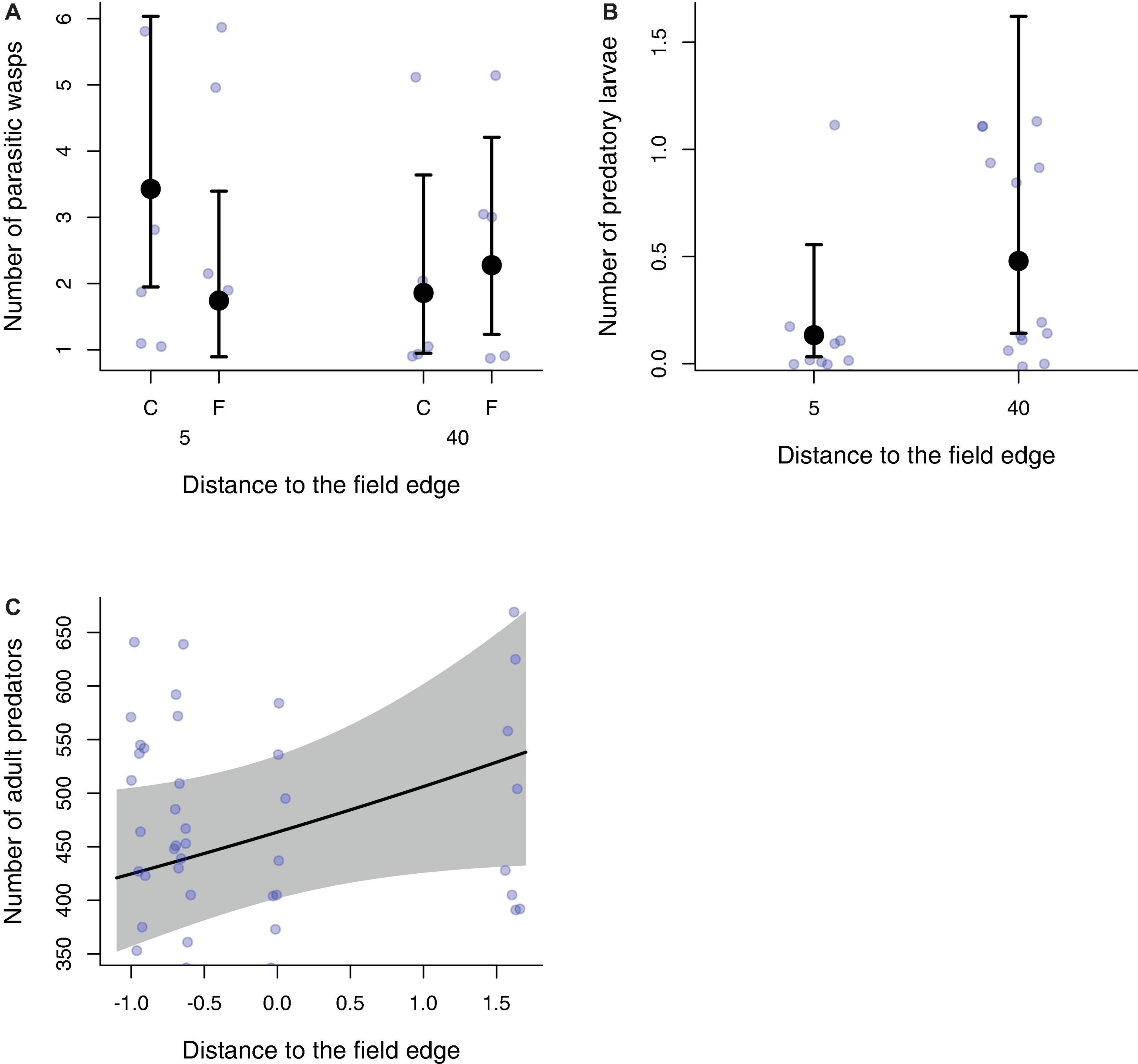
Figure 3. Effects of distance to field edge (m) and treatment. (A) The number of parasitic wasps tended to decrease further from the field edge in control sites (C) but not in flower strip sites (F). (B) The increasing distance from the field edge positively affected the predatory larvae, independently from presence of a flower strip. (C) Adult predators tended to be more abundant further from the field edge, independently from presence of a flower strip. The distances are standardized in the figure, in reality they were 5, 15, and 40 m.
Solitary Bees and Wasps in the Surrounding Landscape
The bee cells in the trap nests mainly came from the families Hylaeus and Megachile, there were only a few Osmia, Chelostoma, and Heriades. There was a non-significant tendency that the number of provisioned cells containing solitary bees was higher closer to the flower strips (Table 3 and Figure 4), but for both solitary bees and wasps the proportion arable fields around the trap nests was the most important explanatory variable (Table 3 and Figure 4), with number of solitary bees and wasp cells decreasing with an increasing proportion of arable fields in the landscape. Exchanging the flower strip categorical variable with the area flower strips within 1,000 m from the strip did not change the results qualitatively; also, here there was only a tendency toward a positive effect (Table 3).
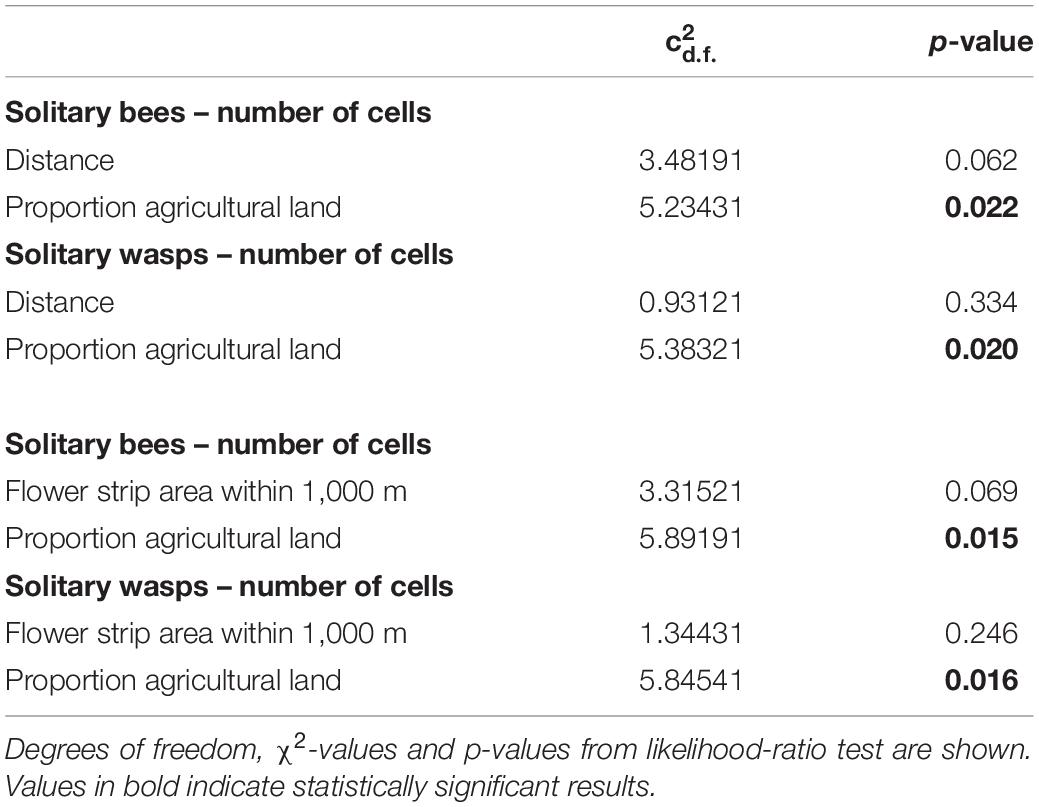
Table 3. Summary of the fixed effects “distance” and “proportion of agricultural land,” as well as “flower strip area within 1,000 m” and “proportion of agricultural land,” on the number of bee and wasp cells in the trap nests.
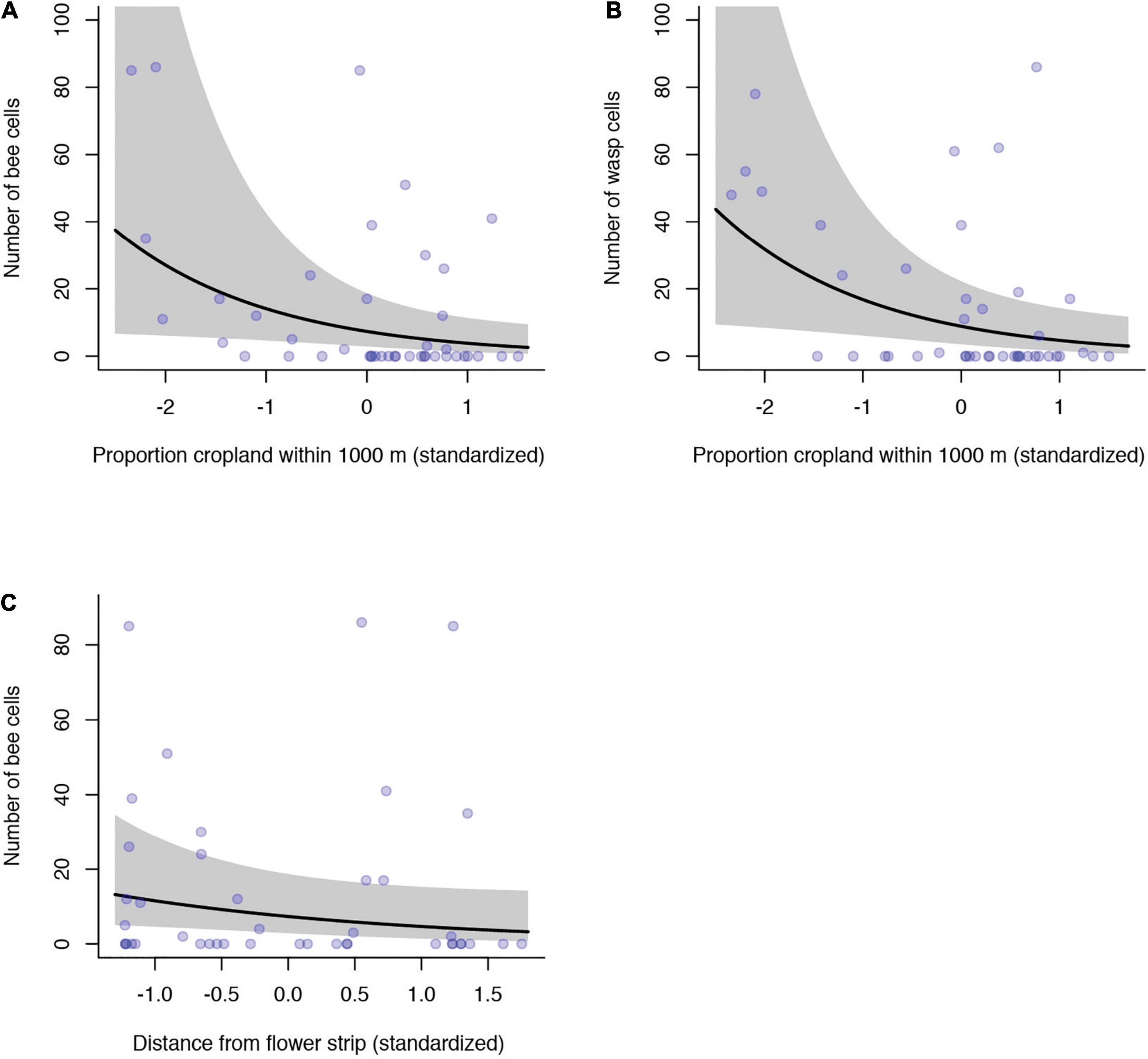
Figure 4. Effect of proportion cropland and distance to flower strip. Increasing proportion cropland negatively influence (A) number of bee cells and (B) number of wasp cells. The increasing distance to flower strips tends to negatively influence (C) number of bee cells.
Discussion
In this study, we demonstrate that annual flower strips can benefit the abundance of hoverfly larvae. However, we could not detect any other significant positive effects on natural enemies and there was no evidence of a difference in natural pest control as shown by lack of flower strip effects on aphid numbers and parazitation rates.
We detected a weak positive effect of flower strips on the abundance of hoverfly larvae, such that they were more abundant further into the field compared to at control sites. This result is consistent with previous studies on the effect of flower strips on hoverflies (Haenke et al., 2009) and may be caused by adult female hoverflies flying further into the fields to lay their eggs when there is a flower strip present to avoid competition from other hoverflies benefiting from local flower availability or that they were less constrained by energy demands due to higher nectar availability and thus able to fly further into the fields. For parasitic wasps, there was a similar but non-significant tendency. Both adult predators (Carabidae, Araneae, and Staphylinidae) and predatory larvae tended to be more abundant further into the fields, but there was no difference between flower strip and control sites. For trap-nesting solitary bees but not for solitary wasps, there was a non-significant tendency that the number of cells was higher closer to flower strips, but not for wasps (in contrast to previous findings; Hoffmann et al., 2018). However, landscape structure best explained the number of cells for both solitary bees and wasps.
We chose to study annual flower strips (Tschumi et al., 2015) rather than multi-annual or perennial flower strips (Jönsson et al., 2015), because our aim was to evaluate the value of using flower strips as EFAs on uncropped field margins in the current CAP policy design. We therefore selected a seed mixture with more or less alien annual plants (flowering in their first year) and adapted the placement and size of the strips in the study to the farmers’ management plans for EFAs, which we did not modify. In addition, we selected to study flower strips in the most intensively farmed landscapes in our region, because it is mostly in these landscapes that EFAs are mandatory for farmers. The weak or lack of effects in our study, can be a result of these specifics of our study design.
Compared to most other similar measures, such as perennial or multi-annual flower strips or buffer strips along water bodies, annual flower strips are agri-environmental schemes with a very short duration (Albrecht et al., 2020). They are generally sown in the springs and then plowed up in the fall the same year. The EFA “uncropped field margins,” evaluated here, only needs to be present until July 31st in the year they are present (in Sweden). The timing of establishment and flowering as well as termination of annual flower strips may thus be important factors determining their potential to impact organisms in the agricultural landscape. In particular, the flower strips may not be available when many species build up their populations early in the spring or during the previous year, when the annual spring sown EFA flower strips are not yet established and even less flowering. For the solitary bees and wasps, it is likely that our spring sown annual flower strip, flowered too late to provide resources during the period when many of the species are active and lay their eggs, which could explain the rather low numbers of cells in the traps. In the trap nests the bee cells mainly came from the families Hylaeus and Megachile, there were only a few Osmia, Chelostoma, and Heriades. For bees like Osmia bicornis, that are active early in the season (Hofmann et al., 2019) and is one of the most common solitary bee species in southern Sweden, the spring sown annual flower strips most likely flowered too late to be able to provide resources for their offspring. Most species from the families Hylaeus and Megachile are active later in the summer (Hofmann et al., 2019) which explains why these were the two most common groups in the trap nests we put out in the end of June- beginning of July. It should be noted that there can have been positive effects of the flower strips that we failed to detect due to timing and/or number of sampling efforts; since the flower strips were annual, we chose to only sample during the year they were present. Future studies should further investigate the effects on functional biodiversity also in the years after an annual flower strip has been in an area (see Blaauw and Isaacs, 2014).
Another key factor determining the effects of an agri-environmental measure such as flower strips, is the characteristics of the landscape in which it is placed (Tscharntke et al., 2016). The availability of semi-natural habitat is in general important for both pollinators and natural enemies (Shackelford et al., 2013; Rusch et al., 2016; Duarte et al., 2018; but see Karp et al., 2018) and a potential reason for why we did not find any effects of our flower strips on abundance of natural enemies and aphids is that potential source populations of natural enemies in our landscapes were too small (Bianchi et al., 2006; Chaplin-Kramer et al., 2011; Rusch et al., 2016). When a flower strip is annual and the duration of the measure is short, it is likely that the surrounding landscape plays an ever bigger role than for perennial or multi-annual strips where a temporal effect may allow populations to build up over time (Korpela et al., 2013; Jönsson et al., 2015; Ganser et al., 2019). If the flower strips in our study had been present for several years it is possible that they could have had a positive effect on, e.g., abundance of pollinators (Jonason et al., 2011; Jönsson et al., 2015). A sufficient amount of perennial habitat will be required in the surrounding landscape to provide founding populations that can take benefit of the resources provided by the annual flower strip (Tschumi et al., 2015; Tscharntke et al., 2016). In a recent study by Pollier et al. (2019), there were positive effects of wildflower strips on natural enemies in and close to the strips in the year of establishment. However, the average field sized reported in that study (0.5–3 ha) suggests that the landscapes were more heterogenous (Martin et al., 2019) than the landscapes of the present study (with an average field size of 4.6 ha). The positive effects we detected here, were among mobile organisms. Hoverflies, although also affected by availability of semi-natural habitats (Schirmel et al., 2018), have a life history (e.g., not being central placed foragers (Covich, 1976) allowing them to take advantage of newly established habitats even when the landscape is fragmented and rather simple, whereas the less mobile organisms showed no response (as they are not able to move from the few and fragmented habitats that can sustain a founder population).
We did not find an effect of the surrounding landscape on the abundance of natural enemies or the abundance and parasitation rate of aphids. We suggest that the variation in landscape complexity (75–95% arable land) was such that founding populations were consistently too low for annual flower strips to affect their populations (Tscharntke et al., 2007), whereas Tschumi et al. (2015) also did not find any effect of landscape complexity and suggested this was because landscapes were always very complex. The trap nests in our study were placed in landscapes with a broader range of landscape complexity (51–98% agricultural arable fields within 1,000 m) than the sampling of natural enemies was and for both solitary bees and wasps, there was a negative relationship between the number of cells and the proportion of arable fields around the trap nests. Since bumble bees utilize landscapes at larger spatial scales than solitary bees, landscape effects could theoretically have been mediated by interspecific competition, but we did not find any such competition in another study explicitly focusing on this (Johanna Yourstone, personal communication). We are also aware that the proxy we use to describe the landscape is coarse and acknowledge that there may be important aspects of landscape structure with consequences for natural enemies and pollinators that we did not capture.
An important characteristic of our study was that we used EFAs as planned by farmers independent of our study, but then made farmers experimentally implement flower strips on them. However, by strictly adapting our design to the farmers choices of placement of EFAs, resulted in only six of the EFAs (i.e., flower strips) being located on a field adjacent to a cereal field, reducing the statistical power of the study on natural enemies and pests. In addition, because farmers chose to create the flower strips on sugar beet, oil seed rape and onion fields, the flower strips and the focal fields were separated by a small road, which might have been enough to hamper spill-over from the flower strips, since such effects often are restricted to only a few meters from the flower strips (Pollier et al., 2019). Furthermore, while studying potential effects of the flower strips on natural enemies and aphids we used existing field edges as controls, which differed in appearance and structure, from broad with ample vegetation to narrow with less vegetation, potentially creating a noise hiding any effect of flower strips in the paired design. In addition, except for a small area around transects, pesticides were freely applied on fields, which may have reduced populations able to take advantage from flower strips. In fact, pesticides may even drift from the field into the transects (Ricci et al., 2019). However, regardless of these limitations, we found little evidence that EFAs with annual flower strips as planned by farmers enhance populations or ecosystem services provided by pollinators or natural enemies in intensive agricultural landscapes.
Annual flower strips have become increasingly common in agricultural landscapes. They are agri-environmental measures with potential to increase farmland biodiversity and ecosystem services (Tschumi et al., 2015), as well as being flexible and relatively easy to implement for farmers (Nilsson et al., 2019). Nevertheless, the results from our study highlights that their potential for promoting functional biodiversity such as pollinators and natural enemies, especially in simple landscapes, is limited but also needs to be studied more carefully. For overwintering arthropods annual flower strips, plowed up in the fall, may even act as ecological traps (Ganser et al., 2019). As other studies before, we stress, the importance of basing conservation and agri-environmental measures on scientific evidence (see e.g., Dicks et al., 2014). In intensively managed and ecologically simple agricultural landscapes, where perennial habitat is scarce, the annual flower strips would most likely have a better effect if combined with more permanent agri-environmental measures (Krimmer et al., 2019). This type of management arrangement is something that future research needs to study more specifically. We chose to study annual flower strips because of the annual character of the EFAs and their potential to be more appealing to farmers than permanent measures, though, for long-term positive effects on biodiversity in farmland, networks of perennial or at least multi-annual flower strips (Korpela et al., 2013; Jönsson et al., 2015; Ganser et al., 2019; Albrecht et al., 2020) and semi natural habitats such as semi-natural grasslands (Ekroos et al., 2013) have considerably greater potential.
Data Availability Statement
The raw data supporting the conclusions of this article will be made available by the authors, without undue reservation.
Author Contributions
HS received the funding. LN, BK, and HS designed the study. LN and BK did fieldwork and data collection. LN wrote the manuscript with input from HS and BK. All authors conducted the statistical analysis.
Funding
This work was funded by grants from the Swedish Research Council FORMAS grant number: 2014-01603 and Region Skåne to HS grant number: 1202836.
Conflict of Interest
The authors declare that the research was conducted in the absence of any commercial or financial relationships that could be construed as a potential conflict of interest.
Acknowledgments
We thank farmers and land managers for letting us work on their land and Hushållningssällskapet for collaboration on flower strip establishment, and M. Holmberg at Olssons frö for advice on the flower strip seed mixture. We are very grateful to A. Andersson, F. Arvidsson, A. Berg, A. Björk, A. Brosset, and N. Michon for field and lab assistance. Thanks to K. Birkhofer and M. Tschumi for advice on study design, M. Tschumi for valuable discussions about results, and J. Ekroos, R. Carrié, and O. Olsson for rewarding discussions on the analysis. We also thank A. Jönsson for establishing contacts with farmers and N. Boke Olén, Y. Clough, and P. Olsson for help with spatial data. The research presented in this article is a contribution from the strategic research area BECC (Biodiversity and Ecosystems in a Changing Climate).
Supplementary Material
The Supplementary Material for this article can be found online at: https://www.frontiersin.org/articles/10.3389/fevo.2021.629124/full#supplementary-material
References
Albrecht, M., Kleijn, D., Williams, N. M., Tschumi, M., Blaauw, B. R., Bommarco, R., et al. (2020). The effectiveness of flower strips and hedgerows on pest control, pollination services and crop yield: a quantitative synthesis. Ecol. Lett. 23, 1488–1498. doi: 10.1111/ele.13576
Ambrosino, M. D., Luna, J. M., Jepson, P. C., and Wratten, S. D. (2006). Relative frequencies of visits to selected insectary plants by predatory hoverflies (Diptera: Syrphidae), other beneficial insects, and herbivores. Environ. Entomol. 35, 394–400. doi: 10.1603/0046-225x-35.2.394
Balzan, M. V., Bocci, G., and Moonen, A.-C. (2016). Utilisation of plant functional diversity in wildflower strips for the delivery of multiple agroecosystem services. Entomol. Exp. Appl. 158, 304–319. doi: 10.1111/eea.12403
Balzan, M. V., Bocci, G., and Moonen, A. C. (2014). Augmenting flower trait diversity in wildflower strips to optimise the conservation of arthropod functional groups for multiple agroecosystem services. J. Insect Conserv. 18, 713–728. doi: 10.1007/s10841-014-9680-2
Batary, P., Andras, B., Kleijn, D., and Tscharntke, T. (2011). Landscape-moderated biodiversity effects of agri-environmental management: a meta-analysis. Proc. R. Soc. B Biol. Sci. 278, 1894–1902. doi: 10.1098/rspb.2010.1923
Batáry, P., Dicks, L. V., Kleijn, D., and Sutherland, W. J. (2015). The role of agri-environment schemes in conservation and environmental management. Conserv. Biol. 29, 1006–1016. doi: 10.1111/cobi.12536
Bates, D., Maechler, M., Bolker, B., and Walker, S. (2015). Fitting linear mixed-effects models using lme4. J. Stat. Softw.67, 1–48.
Bianchi, F., Booij, C. J. H., and Tscharntke, T. (2006). Sustainable pest regulation in agricultural landscapes: a review on landscape composition, biodiversity and natural pest control. Proc. R. Soc. B Biol. Sci. 273, 1715–1727. doi: 10.1098/rspb.2006.3530
Blaauw, B. R., and Isaacs, R. (2014). Flower plantings increase wild bee abundance and the pollination services provided to a pollination-dependent crop. J. Appl. Ecol. 51, 890–898. doi: 10.1111/1365-2664.12257
Bommarco, R., Kleijn, D., and Potts, S. G. (2013). Ecological intensification: harnessing ecosystem services for food security. Trends Ecol Evol. 28, 230–238. doi: 10.1016/j.tree.2012.10.012
Brooks, M. E., Kristensen, K., van Benthem, K. J., Magnusson, A., Berg, C. W., Nielsen, A., et al. (2017). glmmTMB balances speed and flexibility among packages for zero-inflated generalized linear mixed modeling. R J. 9, 378–400. doi: 10.32614/rj-2017-066
Campbell, A. J., Wilby, A., Sutton, P., and Wackers, F. (2017). Getting more power from your flowers: multi-functional flower strips enhance pollinators and pest control agents in apple orchards. Insects 8:101. doi: 10.3390/insects8030101
Carreck, N. L., and Williams, I. H. (1997). Observations on two commercial flower mixtures as food sources for beneficial insects in the UK. J. Agric. Sci. 128, 397–403. doi: 10.1017/s0021859697004279
Carreck, N. L., and Williams, I. H. (2002). Food for insect pollinators on farmland: insect visits to flowers of annual seed mixtures. J. Insect Conserv. 6, 13–23.
Carvell, C., Roy, D. B., Smart, S. M., Pywell, R. F., Preston, C. D., and Goulson, D. (2006). Declines in forage availability for bumblebees at a national scale. Biol. Conserv. 132, 481–489. doi: 10.1016/j.biocon.2006.05.008
Chaplin-Kramer, R., O’Rourke, M. E., Blitzer, E. J., and Kremen, C. (2011). A meta-analysis of crop pest and natural enemy response to landscape complexity. Ecol. Lett. 14, 922–932. doi: 10.1111/j.1461-0248.2011.01642.x
Colley, M. R., and Luna, J. M. (2000). Relative attractiveness of potential beneficial insectary plants to aphidophagous hoverflies (Diptera : Syrphidae). Environ. Entomol. 29, 1054–1059. doi: 10.1603/0046-225x-29.5.1054
Covich, A. P. (1976). Analyzing shapes of foraging areas: some ecological and economic theories. Annu. Rev. Ecol. Syst. 7, 235–257. doi: 10.1146/annurev.es.07.110176.001315
Dicks, L. V., Hodge, I., Randall, N. P., Scharlemann, J. P. W., Siriwardena, G. M., Smith, H. G., et al. (2014). A transparent process for “Evidence-Informed” policy making. Conserv. Lett. 7, 119–125. doi: 10.1111/conl.12046
Duarte, G. T., Santos, P. M., Cornelissen, T. G., Ribeiro, M. C., and Paglia, A. P. (2018). The effects of landscape patterns on ecosystem services: meta-analyses of landscape services. Landsc. Ecol. 33, 1247–1257. doi: 10.1007/s10980-018-0673-5
Ekroos, J., Rundlöf, M., and Smith, H. G. (2013). Trait-dependent responses of flower-visiting insects to distance to semi-natural grasslands and landscape heterogeneity. Landsc. Ecol. 28, 1283–1292. doi: 10.1007/s10980-013-9864-2
Eriksson, S., and Rundlöf, M. (2013). Pollinatörer i Insådda Ettåriga Blomremsor - en Fältundersökning av Förekomsten av Blombesökande Insekter i Insådda Blommande Remsor i tre Slättbygdsomr{åden i Sverige 2011-12. Uppsala: Hushållningssällskapet.
EU (2013). Regulation (EU) No 1307/2013 of the European Parliment and of the Council of 17 December 2013. Brussels: EU.
Ganser, D., Knop, E., and Albrecht, M. (2019). Sown wildflower strips as overwintering habitat for arthropods: effective measure or ecological trap? Agric. Ecosyst. Environ. 275, 123–131. doi: 10.1016/j.agee.2019.02.010
Gardiner, T., Edwards, M., and Hill, J. (2008). Establishment of clover-rich field margins as a forage resource for bumblebees Bombus spp. on Romney Marsh, Kent, England. Conserv. Evid. 5, 51–57.
Gathmann, A., and Tscharntke, T. (2002). Foraging ranges of solitary bees. J. Anim. Ecol. 71, 757–764. doi: 10.1046/j.1365-2656.2002.00641.x
Grab, H., Poveda, K., Danforth, B., and Loeb, G. (2018). Landscape context shifts the balance of costs and benefits from wildflower borders on multiple ecosystem services. Proc. R. Soc. B Biol. Sci. 285:20181102. doi: 10.1098/rspb.2018.1102
Greenleaf, S. S., Williams, N. M., Winfree, R., and Kremen, C. (2007). Bee foraging ranges and their relationship to body size. Oecologia 153, 589–596. doi: 10.1007/s00442-007-0752-9
Haaland, C., Naisbit, R. E., and Bersier, L.-F. (2011). Sown wildflower strips for insect conservation: a review. Insect Conserv. Divers. 4, 60–80. doi: 10.1111/j.1752-4598.2010.00098.x
Haenke, S., Scheid, B., Schaefer, M., and Tscharntke, T. (2009). Increasing syrphid fly diversity and density in sown flower strips within simple vs. complex landscapes. J. Appl. Ecol.46, 1106–1114. doi: 10.1111/j.1365-2664.2009.01685.x
Hartig, F. (2018). DHARMa: Residual Diagnostics for Hierarchical (Multi-Level / Mixed) Regression Models. R package version 0.2.0. Available online at: http://florianhartig.github.io/DHARMa/ (accessed October 23, 2018).
Hoffmann, U. S., Jauker, F., Lanzen, J., Warzecha, D., Wolters, V., and Diekötter, T. (2018). Prey-dependent benefits of sown wildflower strips on solitary wasps in agroecosystems. Insect Conserv. Divers. 11, 42–49. doi: 10.1111/icad.12270
Hofmann, M. M., Zohner, C. M., and Renner, S. S. (2019). Narrow habitat breadth and late-summer emergence increases extinction vulnerability in Central European bees. Proc. R. Soc. B Biol. Sci. 286:20190316. doi: 10.1098/rspb.2019.0316
Hofmann, M. M., Fleischmann, A., and Renner, S. S. (2020). Foraging distances in six species of solitary bees with body lengths of 6 to 15 mm, inferred from individual tagging, suggest 150 m-rule-of-thumb for flower strip distances. J. Hymenoptera Res. 77, 105–117. doi: 10.3897/jhr.77.51182
Hogg, B. N., Bugg, R. L., and Daane, K. M. (2011). Attractiveness of common insectary and harvestable floral resources to beneficial insects. Biol. Control 56, 76–84. doi: 10.1016/j.biocontrol.2010.09.007
Holland, J. M., Bianchi, F. J., Entling, M. H., Moonen, A.-C., Smith, B. M., and Jeanneret, P. (2016). Structure, function and management of semi-natural habitats for conservation biological control: a review of European studies. Pest Manage. Sci. 72, 1638–1651. doi: 10.1002/ps.4318
Holzschuh, A., Steffan-Dewenter, I., and Tscharntke, T. (2009). Grass strip corridors in agricultural landscapes enhance nest-site colonization by solitary wasps. Ecol. Appl. 19, 123–132. doi: 10.1890/08-0384.1
Jonason, D., Andersson, G. K. S., Öckinger, E., Rundlöf, M., Smith, H. G., and Bengtsson, J. (2011). Assessing the effect of the time since transition to organic farming on plants and butterflies. J. Appl. Ecol. 48, 543–550. doi: 10.1111/j.1365-2664.2011.01989.x
Jönsson, A. M., Ekroos, J., Dänhardt, J., Andersson, G. K., Olsson, O., and Smith, H. G. (2015). Sown flower strips in southern Sweden increase abundances of wild bees and hoverflies in the wider landscape. Biol. Conserv. 184, 51–58. doi: 10.1016/j.biocon.2014.12.027
Karp, D. S., Chaplin-Kramer, R., Meehan, T. D., Martin, E. A., DeClerck, F., Grab, H., et al. (2018). Crop pests and predators exhibit inconsistent responses to surrounding landscape composition. Proc. Natl. Acad. Sci. U.S.A. 115, E7863–E7870.
Klatt, B. K., Nilsson, L., and Smith, H. G. (2020). Annual flowers strips benefit bumble bee colony growth and reproduction. Biol. Conserv. 252:108814. doi: 10.1016/j.biocon.2020.108814
Kleijn, D., Rundlöf, M., Scheper, J., Smith, H. G., and Tscharntke, T. (2011). Does conservation on farmland contribute to halting the biodiversity decline? Trends Ecol. Evol. 26, 474–481. doi: 10.1016/j.tree.2011.05.009
Korpela, E.-L., Hyvönen, T., Lindgren, S., and Kuussaari, M. (2013). Can pollination services, species diversity and conservation be simultaneously promoted by sown wildflower strips on farmland? Agric. Ecosyst. Environ. 179, 18–24. doi: 10.1016/j.agee.2013.07.001
Krimmer, E., Martin, E. A., Krauss, J., Holzschuh, A., and Steffan-Dewenter, I. (2019). Size, age and surrounding semi-natural habitats modulate the effectiveness of flower-rich agri-environment schemes to promote pollinator visitation in crop fields. Agric. Ecosyst. Environ. 284:106590. doi: 10.1016/j.agee.2019.106590
Lee, J. C., Menalled, F. D., and Landis, D. A. (2001). Refuge habitats modify impact of insecticide disturbance on carabid beetle communities. J. Appl. Ecol. 38, 472–483. doi: 10.1046/j.1365-2664.2001.00602.x
Lindström, S. (2010). Fröblandningar för den Biologiska Mångfalden på Slätten. Kristianstad: Hushållningssällskapet.
Lixa, A. T., Campos, J. M., Resende, A. L. S., Silva, J. C., Almeida, M., and Aguiar-Menezes, E. L. (2010). Diversity of Coccinellidae (Coleoptera) using aromatic plants (Apiaceae) as survival and reproduction sites in agroecological system. Neotrop. Entomol. 39, 354–359.
Martin, E. A., Dainese, M., Clough, Y., Báldi, A., Bommarco, R., Gagic, V., et al. (2019). The interplay of landscape composition and configuration: new pathways to manage functional biodiversity and agroecosystem services across Europe. Ecol. Lett. 22, 1083–1094.
Nilsson, L., Clough, Y., Smith, H. G., Alkan Olsson, J., Brady, M. V., Hristov, J., et al. (2019). A suboptimal array of options erodes the value of CAP ecological focus areas. Land Use Policy 85, 407–418. doi: 10.1016/j.landusepol.2019.04.005
Pe’er, G., Zinngrebe, Y., Moreira, F., Sirami, C., Schindler, S., Müller, R., et al. (2019). A greener path for the EU common agricultural policy. Science 365, 449–451. doi: 10.1126/science.aax3146
Persson, A. S., Olsson, O., Rundlöf, M., and Smith, H. G. (2010). Land use intensity and landscape complexity—analysis of landscape characteristics in an agricultural region in Southern Sweden. Agric. Ecosyst. Environ. 136, 169–176. doi: 10.1016/j.agee.2009.12.018
Persson, A. S., and Smith, H. G. (2013). Seasonal persistence of bumblebee populations is affected by landscape context. Agric. Ecosyst. Environ. 165, 201–209. doi: 10.1016/j.agee.2012.12.008
Pollier, A., Tricault, Y., Plantegenest, M., and Bischoff, A. (2019). Sowing of margin strips rich in floral resources improves herbivore control in adjacent crop fields. Agric. Forest Entomol. 21, 119–129. doi: 10.1111/afe.12318
R Core Team (2019). R: A Language and Environment for Statistical Computing. Vienna: R Foundation for Statistical Computing.
Ricci, B., Lavigne, C., Alignier, A., Aviron, S., Biju-Duval, L., Bouvier, J. C., et al. (2019). Local pesticide use intensity conditions landscape effects on biological pest control. Proc. R. Soc. B Biol. Sci. 286:20182898. doi: 10.1098/rspb.2018.2898
Rundlöf, M., Lundin, O., and Bommarco, R. (2018). Annual flower strips support pollinators and potentially enhance red clover seed yield. Ecol. Evol. 8, 7974–7985. doi: 10.1002/ece3.4330
Rusch, A., Birkhofer, K., Bommarco, R., Smith, H. G., and Ekbom, B. (2014). Management intensity at field and landscape levels affects the structure of generalist predator communities. Oecologia 175, 971–983. doi: 10.1007/s00442-014-2949-z
Rusch, A., Chaplin-Kramer, R., Gardiner, M. M., Hawro, V., Holland, J., Landis, D., et al. (2016). Agricultural landscape simplification reduces natural pest control: a quantitative synthesis. Agric. Ecosyst. Environ. 221, 198–204. doi: 10.1016/j.agee.2016.01.039
Scheper, J., Holzschuh, A., Kuussaari, M., Potts, S. G., Rundlöf, M., Smith, H. G., et al. (2013). Environmental factors driving the effectiveness of European agri-environmental measures in mitigating pollinator loss– a meta-analysis. Ecol. Lett. 16, 912–920. doi: 10.1111/ele.12128
Schirmel, J., Albrecht, M., Bauer, P.-M., Sutter, L., Pfister, S. C., and Entling, M. H. (2018). Landscape complexity promotes hoverflies across different types of semi-natural habitats in farmland. J. Appl. Ecol. 55, 1747–1758. doi: 10.1111/1365-2664.13095
Shackelford, G., Steward, P. R., Benton, T. G., Kunin, W. E., Potts, S. G., Biesmeijer, J. C., et al. (2013). Comparison of pollinators and natural enemies: a meta-analysis of landscape and local effects on abundance and richness in crops. Biol. Rev. 88, 1002–1021. doi: 10.1111/brv.12040
Smith, H. G., Ockinger, E., and Rundlöf, M. (2010). Biodiversity and the landscape ecology of agri-environment schemes. Asp. Appl. Biol. 100, 225–232.
Thies, C., Steffan-Dewenter, I., and Tscharntke, T. (2003). Effects of landscape context on herbivory and parasitism at different spatial scales. Oikos 101, 18–25. doi: 10.1034/j.1600-0706.2003.12567.x
Tscharntke, T., Bommarco, R., Clough, Y., Crist, T. O., Kleijn, D., Rand, T. A., et al. (2007). Conservation biological control and enemy diversity on a landscape scale. Biol. Control 43, 294–309. doi: 10.1016/j.biocontrol.2007.08.006
Tscharntke, T., Karp, D. S., Chaplin-Kramer, R., Batary, P., DeClerck, F., Gratton, C., et al. (2016). When natural habitat fails to enhance biological pest control–five hypotheses. Biol. Conserv. 204, 449–458. doi: 10.1016/j.biocon.2016.10.001
Tscharntke, T., Klein, A. M., Kruess, A., Steffan-Dewenter, I., and Thies, C. (2005). Landscape perspectives on agricultural intensification and biodiversity–ecosystem service management. Ecol. Lett. 8, 857–874. doi: 10.1111/j.1461-0248.2005.00782.x
Tschumi, M., Albrecht, M., Collatz, J., Dubsky, V., Entling, M. H., Najar-Rodriguez, A. J., et al. (2016). Tailored flower strips promote natural enemy biodiversity and pest control in potato crops. J. Appl. Ecol. 53, 1169–1176. doi: 10.1111/1365-2664.12653
Tschumi, M., Albrecht, M., Entling, M. H., and Jacot, K. (2015). High effectiveness of tailored flower strips in reducing pests and crop plant damage. Proc. R. Soc. B Biol. Sci. 282, 189–196.
Uyttenbroeck, R., Hatt, S., Piqueray, J., Paul, A., Bodson, B., Francis, F., et al. (2015). Creating perennial flower strips: think functional! Agric. Agric. Sci. Procedia 6, 95–101. doi: 10.1016/j.aaspro.2015.08.044
van Rijn, P. C. J., and Wäckers, F. L. (2016). Nectar accessibility determines fitness, flower choice and abundance of hoverflies that provide natural pest control. J. Appl. Ecol. 53, 925–933. doi: 10.1111/1365-2664.12605
Vattala, H. D., Wratten, S. D., Phillips, C. B., and Wackers, F. L. (2006). The influence of flower morphology and nectar quality on the longevity of a parasitoid biological control agent. Biol. Control 39, 179–185. doi: 10.1016/j.biocontrol.2006.06.003
Vickery, J., Carter, N., and Fuller, R. J. (2002). The potential value of managed cereal field margins as foraging habitats for farmland birds in the UK. Agric. Ecosyst. Environ. 89, 41–52. doi: 10.1016/s0167-8809(01)00317-6
Weibull, A. C., Östman, O., and Granqvist, A. (2003). Species richness in agroecosystems: the effect of landscape, habitat and farm management. Biodivers. Conserv. 12, 1335–1355.
Winkler, K., Wackers, F., Bukovinszkine-Kiss, G., and van Lenteren, J. (2006). Sugar resources are vital for Diadegma semiclausum fecundity under field conditions. Basic Appl. Ecol. 7, 133–140. doi: 10.1016/j.baae.2005.06.001
Wood, T. J., Holland, J. M., and Goulson, D. (2016). Providing foraging resources for solitary bees on farmland: current schemes for pollinators benefit a limited suite of species. J. Appl. Ecol. 121, 479–494.
Wood, T. J., Holland, J. M., Hughes, W. O., and Goulson, D. (2015). Targeted agri-environment schemes significantly improve the population size of common farmland bumblebee species. Mol. Ecol. 24, 1668–1680. doi: 10.1111/mec.13144
Wäckers, F. L., and van Rijn, P. C. J. (2012). “Pick and mix: selecting flowering plants to meet the requirements of target biological control insects,” in Biodiversity and Insect Pests: Key Issues for Sustainable Management, eds G. M. Gurr, S. D. Wratten, W. E. Snyder, and D. M. Y. Read (Chichester, UK: John Wiley & Sons, Ltd.), 139–165. doi: 10.1002/9781118231838.ch9
Zamorano, J., Bartomeus, I., Grez, A. A., and Garibaldi, L. A. (2020). Field margin floral enhancements increase pollinator diversity at the field edge but show no consistent spillover into the crop field: a meta-analysis. Insect Conserv. Divers. 13, 519–531. doi: 10.1111/icad.12454
Zurbuchen, A., Landert, L., Klaiber, J., Müller, A., Hein, S., and Dorn, S. (2010). Maximum foraging ranges in solitary bees: only few individuals have the capability to cover long foraging distances. Biol. Conserv. 143, 669–676. doi: 10.1016/j.biocon.2009.12.003
Zuur, A. F., Ieno, E. N., and Elphick, C. S. (2010). A protocol for data exploration to avoid common statistical problems. Methods Ecol. Evol. 1, 3–14. doi: 10.1111/j.2041-210x.2009.00001.x
Keywords: annual flower strips, EFA, CAP, agri-environmental measures, functional biodiversity
Citation: Nilsson L, Klatt BK and Smith HG (2021) Effects of Flower-Enriched Ecological Focus Areas on Functional Diversity Across Scales. Front. Ecol. Evol. 9:629124. doi: 10.3389/fevo.2021.629124
Received: 13 November 2020; Accepted: 21 April 2021;
Published: 25 May 2021.
Edited by:
Juliana Hipólito, National Institute of Amazonian Research (INPA), BrazilReviewed by:
Alistair John Campbell, Embrapa Amazônia Oriental, BrazilOrianne Rollin, University of Lisbon, Portugal
Copyright © 2021 Nilsson, Klatt and Smith. This is an open-access article distributed under the terms of the Creative Commons Attribution License (CC BY). The use, distribution or reproduction in other forums is permitted, provided the original author(s) and the copyright owner(s) are credited and that the original publication in this journal is cited, in accordance with accepted academic practice. No use, distribution or reproduction is permitted which does not comply with these terms.
*Correspondence: Lovisa Nilsson, bG92aXNhLm5pbHNzb25AY2VjLmx1LnNl