- Department of General Zoology, Faculty of Biology, University of Duisburg-Essen, Essen, Germany
African mole-rats of the genus Fukomys (Northern common mole-rats) combine a monogamous mating system and pronounced sexual size dimorphism; a pattern highly untypical for mammals. At the same time, they live in cooperatively breeding groups composed of reproductive and non-reproductive members of both sexes. How and to which degree sex and breeding status influence morphofunctional characters in eusocial mole-rats is not well characterized but essential to come to a comprehensive understanding of their peculiar social system. Here, we explore patterns of morphological differentiation in skulls of Ansell’s mole-rats (Fukomys anselli) by means of multivariate analysis of linear skull measurements combined with a 2D shape analysis of cranium and mandible. Compared to females, males display larger skulls relative to body size and show an expansion of the facial portion of the cranium, while reproductive status did not have an effect on any of the traits studied. We also show that species of Fukomys mole-rats display a scaling of relative sexual body size dimorphism in compliance to Rensch’s rule, which is deemed indicative of intense male intrasexual competition. For the bathyergid family as a whole, results of scaling analyses were more ambiguous, but also indicative of Rensch’s rule conformity. In line with genetic field data, our results point to a greater role of male-male conflicts in Fukomys than is traditionally assumed and support the notion that reproductive status does not correlate with morphofunctional segregation in these unusual rodents.
Introduction
African mole-rats (Bathyergidae) are a speciose group of sub-Saharan rodents which are renowned for their superb adaptation to life underground (Gomes Rodrigues et al., 2016). Despite their ecological uniformity and specialization, bathyergids encompass species with strongly contrasting social systems (Figure 1). The genera Bathyergus (dune mole-rats), Georychus (Cape mole-rat), and Heliophobius (silvery mole-rat) lead strictly solitary lives, while the sister genera Cryptomys (Southern common mole-rats) and Fukomys (Northern common mole-rats) as well as the basalmost branching bathyergid genus Heterocephalus (naked mole-rat), live in cooperatively breeding groups. These families typically comprise only a single breeding female in all social genera, while the number of simultaneously active reproductive males varies.
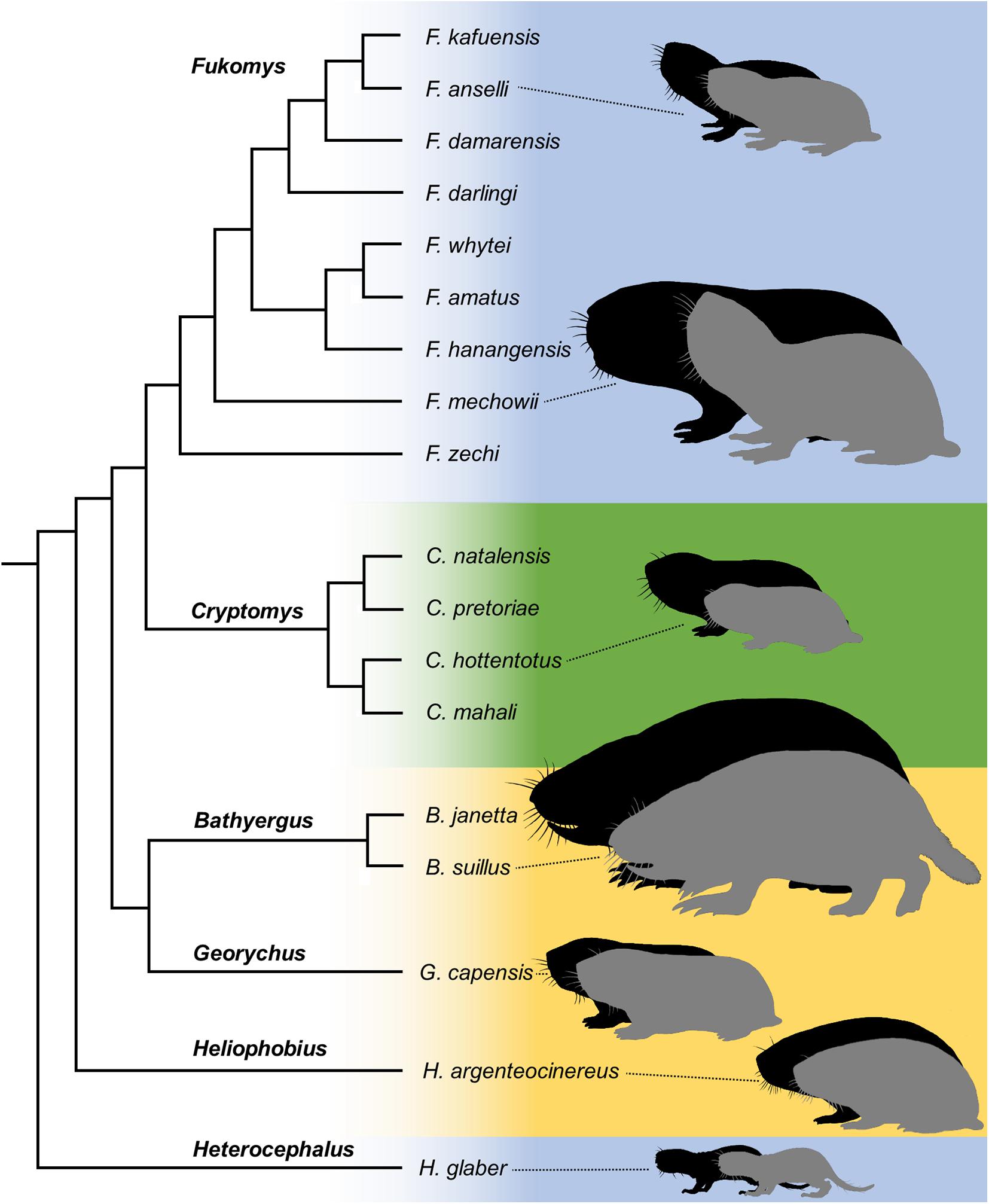
Figure 1. Taxonomy, sexual size dimorphism and social systems of African mole-rats following Visser et al. (2019). Silhouettes indicate variation of body size between species and the degree of dimorphism between the sexes (males = black, females = gray, sexes and species only roughly set to scale for purposes of comprehension). Colors indicate social systems realized in the respective genera: blue – eusocial groups; green – social groups; and yellow – solitary. Silhouettes by Kai R. Caspar.
In Fukomys, the reproductive female is typically monogamous (Burland et al., 2002; Šumbera et al., 2012; Patzenhauerová et al., 2013). When applying the term monogamy, it is important to differentiate between social monogamy (living and raising offspring with a single partner) and genetic monogamy (exclusively mating and producing offspring with such partner). Many socially monogamous mammals are not genetically monogamous (Cohas and Allainé, 2009). However, current evidence suggests that Fukomys indeed exhibits strict social and predominant sexual monogamy, the latter fluctuating in frequency between populations (Burland et al., 2002; Patzenhauerová et al., 2013). While staying faithful to their current mate for prolonged periods (Bappert et al., 2012; Begall et al., 2021), Fukomys may experience serial changes in partners over their lifetime (Burland et al., 2002; Šumbera et al., 2012; Patzenhauerová et al., 2013). Different mating systems are expressed in the other two social bathyergid genera. The breeding female in Heterocephalus families may mate with two (seldom more) males at a time (Braude et al., 2020) and in Cryptomys it often has multiple partners (Bishop et al., 2004). In Heterocephalus as well as in Fukomys, more than six generations of pups overlap in average family groups and the majority of offspring remains with their parents and assist in provisioning their siblings instead of reproducing themselves (Burda et al., 2000; Torrents-Ticó et al., 2018). Because of this high degree of philopatry in combination with partitioning of reproductive labor and cooperative breeding, these genera have at times been characterized as eusocial mammals (Burda et al., 2000). In Cryptomys, levels of philopatry and male reproductive skew are notably lower than in the other social genera (Bishop et al., 2004; Ingram et al., 2004) so that they are generally not considered to be eusocial. Less is known about the mating systems of solitary genera, but morphological, genetic and behavioral evidence suggests that they are either polygynous or promiscuous (Patzenhauerová et al., 2010; Bray et al., 2012; Visser et al., 2017).
In social mole-rats such as Fukomys, non-reproductive individuals are believed to reduce the workload of the breeding female, granting a fitness benefit (Burda et al., 2000). Empirical evidence in support of this assumption has been provided for Fukomys damarensis, the Damaraland mole-rat. In captivity, the fecundity of F. damarensis breeding females as well as the time they spend resting and feeding correlates positively with the number of non-reproductive helpers (Houslay et al., 2020) and in the wild, breeders spend significantly less time foraging than helpers (Francioli et al., 2020). Although at times stated differently, mole-rat helpers do not show developmentally fixed patterns of task specialization (Lacey and Sherman, 1991; Zöttl et al., 2016; Thorley et al., 2018b; Van Daele et al., 2019), meaning that no helper casts dedicated to specific tasks (e.g., foraging, pup raising, and nest defense) exist, as it is the case in many social insects. Rather than that, an individual’s age influences the frequency in which it engages in specific helping behaviors (Zöttl et al., 2016), with differences in the contributions of male and female helpers being negligible (Thorley et al., 2018b).
Due to the differing social and mating systems among bathyergids, it would be predicted that mole-rat genera display varying patterns of sexual size dimorphism (SSD) and sexually selected weaponry directly linked to their mating system (Heske and Ostfeld, 1990; Schulte-Hostedde, 2007). In the solitary genera, one would predict pronounced SSD since access to partners is contested and individual males can gain a reproductive advantage by mating with multiple females via the monopolization of defendable resources. Fitting this assumption, pronounced SSD is found in many solitary subterranean rodents that belong to diverse evolutionary lineages (Daly and Patton, 1986; Mauk et al., 1999; Su et al., 2018). A conjoint prediction would be that males in these species evolve more formidable weapons, in case of mole-rats more powerful and robust jaws and incisors, to solve this task. More subtle or absent sexual dimorphism would be expected in the social genera, particularly so in monogamous Fukomys, where physical breeding competition is low for prolonged periods once a pair-bond is established (Patzenhauerová et al., 2013). Among the few non-bathyergid social subterranean rodents, monogamy and a lack of SSD is for instance evident in the Northern mole vole (Ellobius talpinus; Moshkin et al., 2001). On the other hand, it might be expected that these social genera display differences in functional morphology that relate to reproductive status, for example more strongly developed weaponry in breeders of both sexes to defend their status against challengers (Young and Bennett, 2013). Such hypotheses appear reasonable, since at least female breeders in Fukomys and Heterocephalus show marked changes in their postcranial skeletal anatomy when attaining breeding status (Dengler-Crish and Catania, 2007; Thorley et al., 2018a), indicating a notable degree of developmental plasticity. So far, such differences are not evident in the skulls of female mole-rats (Thorley et al., 2018a) but precise methods such as geometric morphometrics have not yet been employed to differentiate between reproductive status groups and male mole-rats were never studied at all in this respect.
Surprisingly, SSD expression does not appear to correlate with social systems or phylogeny among bathyergids (Burda, 1990, Figure 1). Although these patterns are inconsistent and counterintuitive, the phenomenon received only little scientific attention and is seldom comparatively assessed: The solitary Bathyergus and at least most species of eusocial Fukomys are highly sexually dimorphic in body size with adult males being the larger sex with more massive skulls (Hart et al., 2007; Chimimba et al., 2010; Young and Bennett, 2013). Nevertheless, potential dimorphism in skull shape unrelated to size remains essentially unaddressed in these genera (but see Faulkes et al., 2017 for Fukomys). Within the remaining groups of African mole-rats, available studies suggest that skulls are not sexually dimorphic (Taylor et al., 1985; van Rensburg et al., 2004; Barčíová et al., 2009) and that differences in body size are variably expressed. Solitary Heliophobius are notably dimorphic in mass (Šumbera et al., 2003). For male-biased SSD in social Cryptomys, contradictory results have been published, but studies relying on data from multiple family groups agree that it is indeed present to varying degrees (Spinks et al., 2000; van Rensburg et al., 2004). Finally, eusocial Heterocephalus as well as solitary Georychus are assumed to lack SSD (Brett, 1991; Jarvis and Bennett, 1991; Bennett et al., 2006, see also Thomas et al., 2012).
If no clear relationship with sociality is evident, what factors underly the expression of SSD in African mole-rats? In many animal groups, SSD scales with body mass, a phenomenon most prominently described by Rensch’s rule (Abouheif and Fairbairn, 1997). Rensch’s rule posits that among closely related species, SSD grows with increasing general body size when males are the larger sex (Rensch, 1950) and vice versa when the opposite is the case (Rensch, 1960, but see Webb and Freckleton, 2007). However, it is commonly assumed that Rensch’s rule can only be observed among socially polygynous or polygamous species (Dale et al., 2007; Bidau and Martinez, 2016) so that bathyergids and even more so Fukomys species would not be expected to comply to it. In other subterranean rodents studied so far, the polygynous South American tuco-tucos (Ctenomys) and Central Asian zokors (Eospalax and Myospalax), Rensch’s rule is not in effect (Martínez and Bidau, 2016; Su et al., 2018), and notable SSD appears to be rare among small-bodied mammals in general (Lu et al., 2014). Finding Rensch’s rule among bathyergids would be unexpected and could indicate so far unappreciated social dynamics acting across the boundaries of social systems in this group.
In this exploratory study, we focus on patterns of sexual dimorphism and correlates of breeding status in Fukomys anselli, the Ansell’s mole-rat. F. anselli is a typical representative of its genus in displaying the puzzling combination of pronounced SSD in conjunction with prolonged sexual monogamy and cooperative breeding (Burda and Begall, 1998; Patzenhauerová et al., 2013). Besides employing craniometric methods to assess morphological differentiation, we also quantify differences in relative skull size between the sexes. This phenomenon received little study in mammals (but see Young and Bennett, 2013) but is well investigated in squamate reptiles, where it is prominently discussed as an indicator for intrasexual competition (e.g., Baird, 2013). To place sexual dimorphism in the Ansell’s mole-rat into its phylogenetic context, we further compile a dataset on body mass in the sexes of various bathyergid species and test for SSD scaling conforming to Rensch’s rule. By combining these different approaches, we aim to arrive at a comprehensive characterization of sexual dimorphism in cooperatively breeding Fukomys mole-rats, which is a crucial step to understand the interplay between monogamous mating systems and pronounced SSD found in these animals.
Materials and Methods
SSD and the Validity of Rensch’s Rule in the Bathyergidae
We conducted a literature search for information on sex-specific body mass in the Bathyergidae and concluded with a dataset spanning 18 species from all six extant genera, which cover almost the complete spectrum of body sizes found in the family, including size extremes (Table 1). The genus Fukomys is represented by nine species. Species were included when data for at least five specimens per sex and species corresponding to wild adult animals were available. Data from pregnant females were excluded, whenever provided by the respective sources. Following Ingram et al. (2004), we refer to populations of Cryptomys (C. hottentotus, C. mahali, C. natalensis, and C. pretoriae) as full species, since the age of these lineages as well as their genetic divergence exceeds that of many Fukomys populations which are differentiated at the species level.
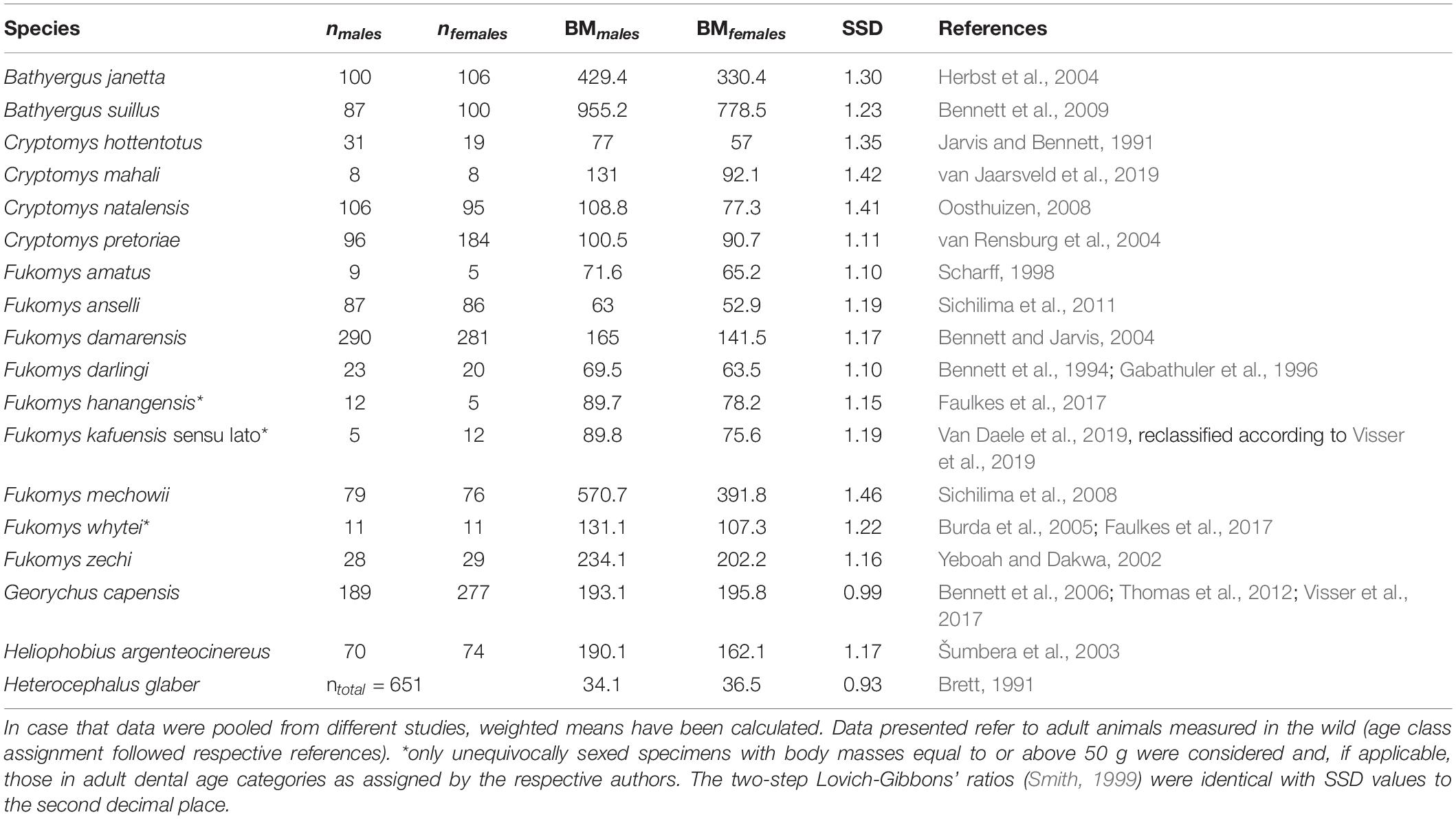
Table 1. Mean body mass (BM, g) and sexual size dimorphism (SSD, male:female) based on BM in African mole-rats.
We tested for the validity of Rensch’s rule in both bathyergids in general and in Fukomys by employing two established methods to investigate SSD scaling. If both were to yield comparable outcomes, the respective results could be assumed to be robust. First, we employed phylogenetic reduced major axis (pRMA) regression, a widely accepted method to study scaling in SSD (Abouheif and Fairbairn, 1997; Fairbairn, 1997; Bidau and Martinez, 2016; Martínez and Bidau, 2016), by utilizing the phytools package in R (Revell, 2012). For such interspecific comparisons, phylogenetic relationship must be accounted for to address the non-independence of species data points due to shared ancestry. Based on the bathyergid phylogeny of Visser et al. (2019; see Figure 1), we calculated pRMA regressions of log10(male body mass) on log10(female body mass) to estimate whether SSD in bathyergids scales with body mass in compliance with Rensch’s rule. The rule is in effect when the coefficient β of said regressions is significantly greater than the expected value 1 (Abouheif and Fairbairn, 1997; Martínez and Bidau, 2016). Clarke’s T statistic with adjusted degrees of freedom was employed to assess the deviation of the slope from the expectation (Bidau and Martinez, 2016).
Second, we calculated phylogenetic linear regression models based on modified Lovich-Gibbons’ ratios (two-step LG ratio) on log10(female body mass) as recommended by Smith (1999). The two-step LG ratio is calculated as follows: If the mean body mass of males is higher than that of females: M/F; if the mean body mass of females is higher than that of males: 2-F/M. Smith (1999) recommends the application of the two-step LG ratio for typical mammalian data sets where male-biased SSD is prevalent. Linear regression models were corrected for phylogeny by using phylogenetic independent contrasts. According to this method, Rensch’s rule is in effect when the coefficient β of the regression line is significantly greater than 0.
Skull Morphometrics of Fukomys anselli
All morphometric data was collected blindly with the researchers taking measurements being unaware of sex and reproductive status of the specimen concerned. All analyses, if not otherwise indicated, were carried out in R Studio for Mac Version 1.3.1093 (RStudio Team, 2020).
Material
Skulls of 40 adult Fukomys anselli, representing ten non-breeders and ten breeders of either sex were extracted from ethanol-fixed or frozen animals from the research collection of the Department of General Zoology, University of Duisburg-Essen (Supplementary Table 1). Respective individuals were kept and often bred in the laboratories of the University but genealogically derive from animals captured close to the type locality of the species in the Lusaka area of Central Zambia. Relying on captive subjects allowed for an unequivocal determination of an individual’s reproductive status, as breeding was closely monitored in the laboratory. Only captive-born individuals that never reproduced where classified as non-breeders, while breeders where required to have produced at least one offspring. All skulls derived from animals that were at least 30 months old at the time of death and therefore fully mature. F. anselli is full grown at an age of approximately 12 months (Burda and Begall, 1998). Mean age for breeders was 111.2 months (=9.3 years; SD: 41.4 months), while mean age for helpers was 57.9 months (=4.8 years; SD: 36.3 months). This stark discrepancy is largely explained by the bimodal aging pattern in the genus Fukomys, with breeders reaching on average two times the age of helpers in captivity (Dammann and Burda, 2006). However, mean ages of males (7.8 years; SD: 49.2 months) and females (6.3 years; SD: 44 months) did not differ significantly (Student’s t-Test, p > 0.2). Each specimen derived from a different litter and for the most part from different families. Only two pairs of different aged siblings were sampled, all of the respective individuals being non-breeders.
Multivariate Skull Morphometrics
A set of 24 linear measurements from cranium and mandible (Table 2) were collected with digital calipers (fixpoint® model 77001). Measurements were taken by a single observer (KRC) to 0.1 mm from either the sagittal plane or the right side of the skull (see Table 2 and Supplementary Table 1) and are visualized in Figure 2. In case relevant structures of the right side of the skull were damaged, measurements were taken from the left one (11 measurements = 1.1% of total measurements). Missing measurements [10 measurements = 1% of total measurements (n = 950), see Supplementary Table 1] were estimated using the LOST package (Arbour and Brown, 2020). Hindfoot length was chosen as a proxy for body size to assess its influence on cranial measurements. Foot length is a common metric in small mammal research that is insensitive to changes in body condition and was measured to the nearest 0.1 mm excluding claws, following Ansell (1965).
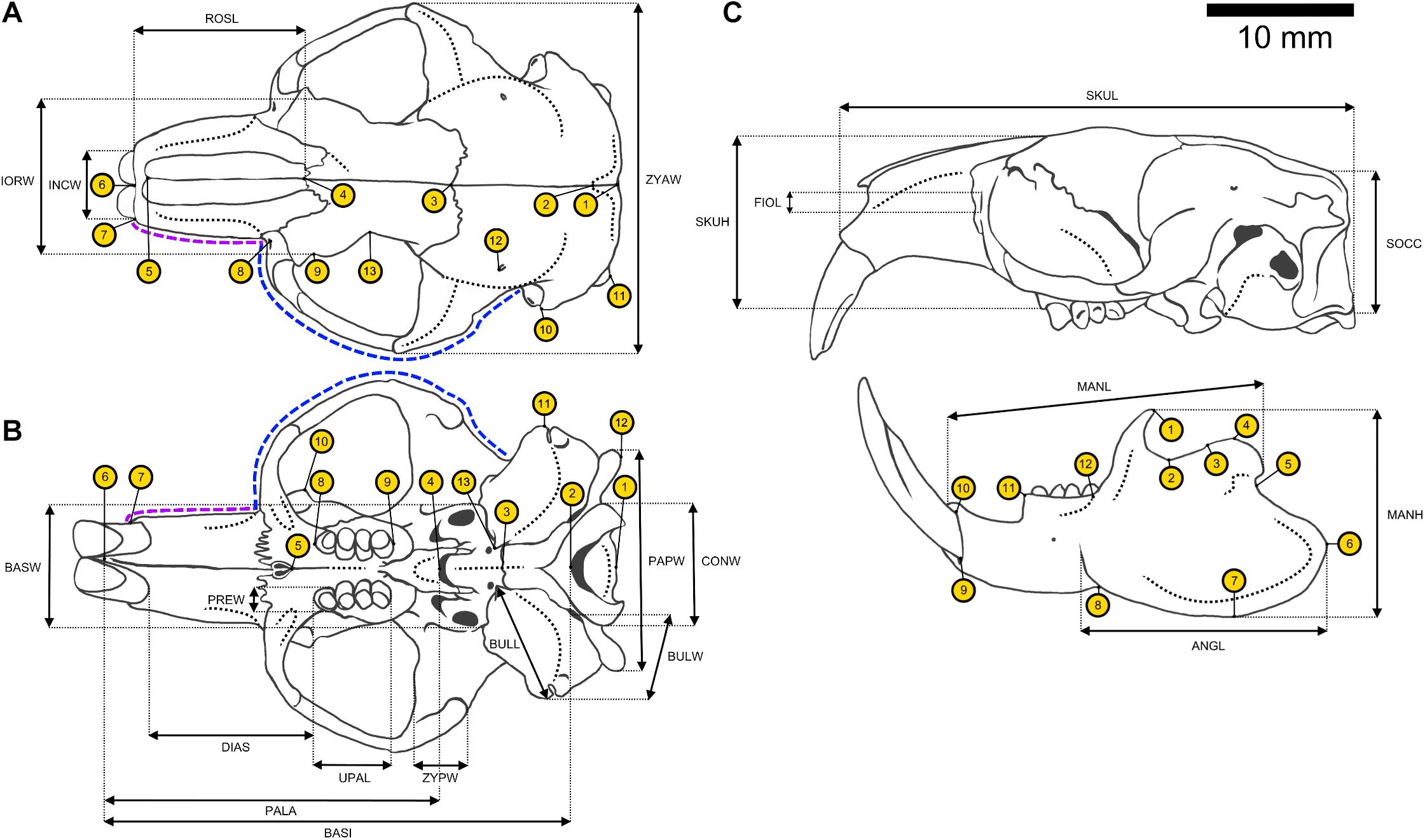
Figure 2. Schematic drawing of a male Ansell’s mole-rat skull with annotated linear measurements and landmarks used in this study. Definitions of landmarks and measurements are provided in Table 2 and Supplementary Table 2. Semilandmarks are indicated as dashed lines. Rostral semilandmarks (purple) encompassed 8 landmark points, while zygomatic semilandmarks (blue) comprised 24. (A) Dorsal perspective of cranium with dorsal landmark set. (B) Ventral perspective of cranium with ventral landmark set. (C) Lateral perspective of cranium and mandible with mandibular landmark set.
Measurements were log-transformed and analyzed using principal component analysis (PCA). Subsequently, leave-one-out cross-validated linear discriminant function analysis (LDA) was performed on the principal components (PC) generated by the PCA to estimate how well sexes and breeding status groups could be differentiated based on cranial measurements. One-tailed exact binomial tests were used to check whether correct assignment rates differed significantly from chance level. Suitability of PC covariance for LDA was tested with Box’s M test. The assumption of multivariate normality was tested using the Shapiro–Wilk multivariate normality test. A MANOVA was used to test for the effects of body size, sex, and reproductive status as well as their interaction on skull morphology. Comparisons of each linear measurement between the respective groups of specimens were performed by employing Welch’s two sample t-test for normally distributed measurements and Wilcoxon rank sum test for non-normally distributed ones, respectively, controlling for alpha error accumulation via Bonferroni correction. This resulted in an adjusted significance level of αadjusted = 0.002.
2D Geometric Morphometrics of Cranium and Mandible
Size-independent differences in skull shape were analyzed by employing a 2D landmark approach. Crania were photographed with a Canon EOS 200D reflex digital camera in a standardized fashion from dorsal and ventral perspectives. Mandibles were photographed from a lateral perspective. Specimens were placed on a checkered mat with squares of 1 cm edge length that was used to provide spatial orientation and acted as a size reference. The inclination of the crania was adjusted with a piece of plasticine. The camera focus was adjusted on the molars for both ventral cranium and mandible photos and on the interfrontal suture of the skull roof for pictures taken from the dorsal perspective. Unilateral sets of 13 landmarks for the dorsal and ventral cranium and 12 landmarks for the mandible were selected and analyzed independently from each other (Figure 2; definitions are listed in Supplementary Table 2). Dorsal and ventral sets were amended by two lateral semilandmarks. The first one consisted of 8 semilandmark points and was set along the rostrum from the incisor to the zygomatic arch. The second one comprised 24 landmark points and demarcated the zygomatic arch (Figure 2). Bending energy was used as criterion to optimize landmark positioning during sliding. Landmarks’ digitization and scaling of photographs was achieved in TPSDig2 version 2.31 (Rohlf, 2018) and done by a single researcher for each landmark set (JM: dorsal/ventral; KRC: mandible). The geomorph package (Adams et al., 2020) was employed to analyze shape data. Occasional damage to the coronoid process led to missing landmarks (6 landmark points = 1.25%) in the mandible dataset, which were estimated and replaced by aid of the LOST package (Arbour and Brown, 2020). Landmarks were Procrustes superimposed to allow for subsequent analysis. Allometry was assessed by an ANOVA, regressing shape variables against centroid size, which is a measure of overall size in superimposed landmark datasets. PCA and LDA were employed with procedures analogous to those used in the analysis of linear cranial measurements and served the same purpose. However, the mandible dataset was not normally distributed. Therefore, statistical differences in measurements for sexes and reproductive status groups as well as interaction effects were assessed via MANOVA for dorsal and ventral cranial datasets and via a PERMANOVA for the mandibular one, respectively. Since LDA requires normal distribution of data as well, the randomForest method was used as an alternative classification procedure for mandibular landmark data (Liaw and Wiener, 2002).
Sex Differences in Relative Skull Dimensions
The greatest lengths of the skull (SKUL) and zygomatic arch width (ZYAW) were chosen to represent skull length and width, respectively, while hindfoot length was again used as a proxy for body size. Differences in hindfoot length between sexes and reproductive status groups were assessed in a linear regression model with hindfoot length as the dependent variable and sex and breeding status as predictors. Subsequently, the influence of body size and sex on skull size was tested the same way with either skull length or width as the dependent and hindfoot length as well as sex as predictor variables. Based on results from our previous morphometric analyses, the influence of breeding status on these parameters was not tested. Data were inspected visually for biasing outliers, linearity, normality, and homogeneity of variances in diagnostic plots. The latter two aspects were also assessed through Shapiro–Wilk and Levene tests, respectively, and found to be suitable for linear regression. Values for Cohen’s d were derived from models’ t statistics to provide a measure of effect size.
Results
Occurrence and Scaling of SSD in the Bathyergidae
We found large differences in SSD among bathyergids and within the most speciose genus, Fukomys (Table 1). Lowest SSD values indicating sexual monomorphism were recovered for Georychus and Heterocephalus. All other bathyergids show a male-biased SSD which is pronounced in Fukomys mechowii, the giant mole-rat, and in the small-bodied genus Cryptomys. There was only a modest correlation between log10 female body mass and SSD in the Bathyergidae in general (Pearson correlation: r = 0.3; t = 1.26; and p = 0.23) but a strong one among Fukomys species (Pearson correlation: r = 0.76; t = 3.1; and p = 0.017).
Analyses of body mass data via pRMA models (Table 3 and Figure 3) recovered that bathyergids as a whole show a trend to follow Rensch’s rule of SSD scaling (β = 1.072, p = 0.079) while Fukomys species clearly comply to it (β = 1.102; p = 0.021). For both groups a strong phylogenetic signal associated with SSD was found (λ = 0.999), indicating a tight correlation between the expression of SSD and phylogenetic affiliation in bathyergids. Results of the regression models on two-step LG ratios did only partially align with the ones of the pRMA models. The former found bathyergids to comply to Rensch’s rule (β = 0.2214; p = 0.01) while the data for Fukomys is approaching significance (β = 0.2611; p = 0.05).
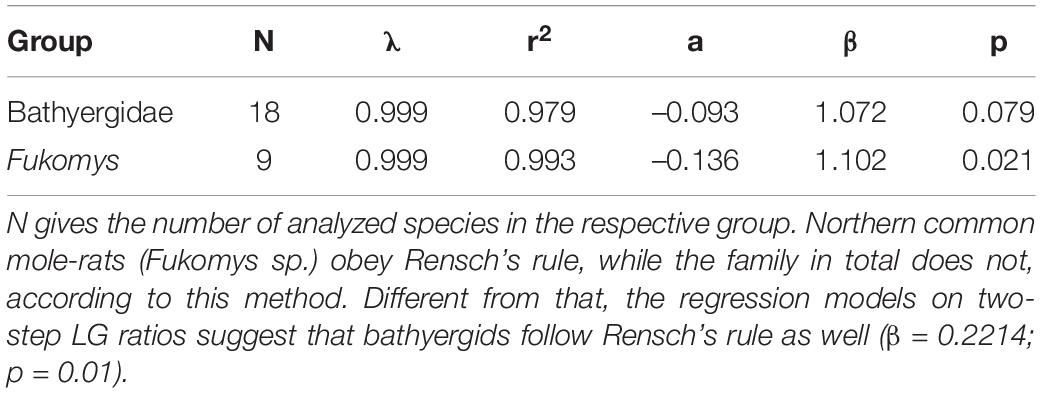
Table 3. Results from phylogenetic major reduced axis models investigating the relationship between male and female body mass in African mole-rats.
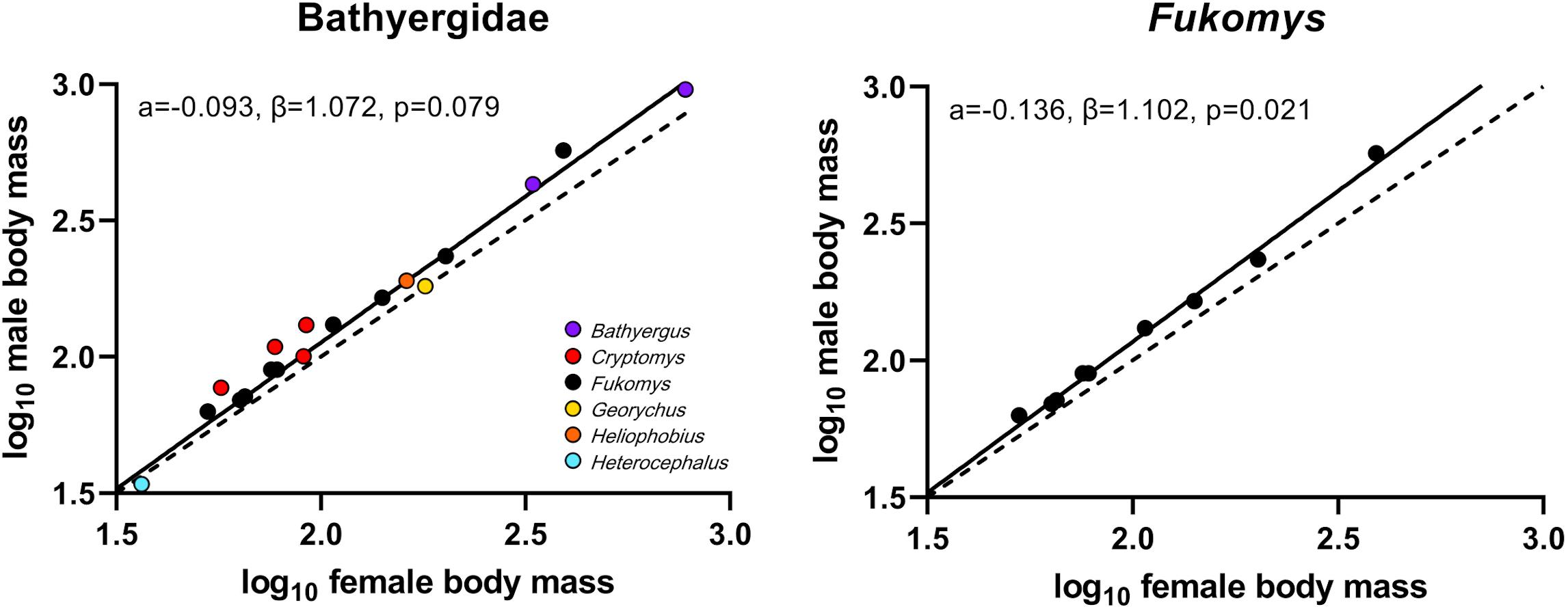
Figure 3. Scaling of sexual size dimorphism in the family Bathyergidae and Fukomys species. The broken line indicates a size ratio of 1.0, equating to isometric scaling. Fukomys follows Rensch’s rule as indicated by a significant deviation of β > 1. At family level, a similar trend could be recovered using phylogenetic major reduced axis models. However, the regression models on two-step LG ratios found that also bathyergids follow Rensch’s rule revealed by a significant deviation of β > 0.
Multivariate Skull Morphometrics
Male and female F. anselli were recovered as almost completely separated in the PCA morphospace based on linear cranial measurements, while reproductive groups were not discernible from each other (Figure 4 and Table 4). Further analyses provided results consistent with this finding. MANOVA indicated a strong influence of sex on cranial morphometrics (F = 12.13, p ≪ 0.001) but none of reproductive status (F = 0.63, p = 0.74) or the interaction between both (F = 0.34, p = 0.84). Hindfoot length was a strong general predictor of cranial morphology (F = 7.33, p < 0.001), but not among individuals of the same sex (F = 0.40, p = 0.8), and irrespective of their reproductive status (F = 0.1, p = 0.98). While males (90%) and females (80%) could be reliably classified in the linear measurement LDA (p < 0.01), individuals of the same sex were randomly assigned to reproductive status groups (p > 0.1, Table 5).
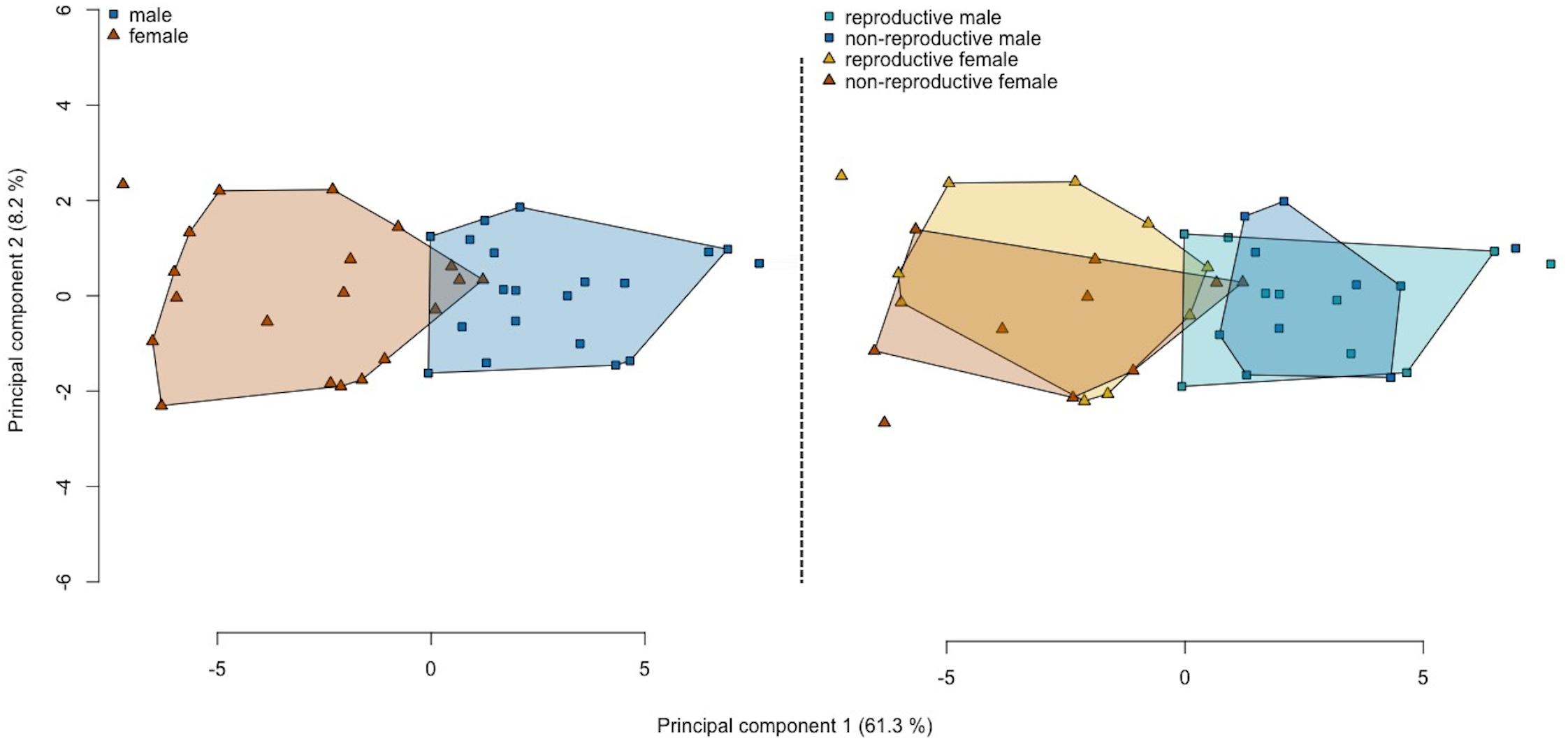
Figure 4. Visualization of PCA results based on linear cranial measurements of F. anselli. Specimens are well separated by sex but not reproductive status.

Table 5. Correct assignment rates (%) of F. anselli skulls based on sex and reproductive status according to discriminant function analyses (based on LDA or in the case of mandibular landmarks on randomForest).
For the linear measurements, PCA computed 24 principal components, the first four of which had eigenvalues > 1 and explained 79.9% of total variance in the sample (Table 4). The most important measurements for group separation in PC1 were indicators of overall skull size, but particularly of its facial portion: mandibular length (MANL: 6.35%), basilar length (BASI: 6.35%), and zygomatic arch width (ZYAW: 6.29%). However, PC1 factor loadings were to a large extent evenly distributed over the measurements, excluding several measurements concerned with dental characters and the skull base, which were of specific relevance to PC2. Width of skull base (BASW: 22.04%), width of premolar (PREW: 21.43%), and upper tooth row length (UPAL: 15.9%) contributed most to PC2 (Table 4).
The comparison of individual measurements between sexes and reproductive groups supported results of other analyses in showing distinct sexual dimorphism and the absence of cranial differences between breeders and non-breeders (Table 5). All but six variables differed significantly between the sexes (padjusted < 0.002). Non-significant variables primarily encompassed measurements relevant to the dentition and skull base. They related to the upper cheek dentition (PREW, UPAL), condyle (CONW) and paroccipital processes (PAPW), infraorbital foramen size (FIOL) and the width of the tympanic bulla (BULW). Differences between breeders and non-breeders of the same sex were consistently non-significant.
Sex Differences in Relative Skull Dimensions
Hindfoot length, approximating body size, was not found to differ intrasexually within reproductive status groups (t = -0.355, p > 0.7, and d = 0.12), but between the sexes (t = 2.626, p = 0.012, and d = 0.88). Skull length as well as width was found to increase proportionally with body size without significant differences among regression slopes between the sexes (skull length: t = 1.543, p = 0.131, and d = 0.51; skull width: t = 1.773, p = 0.085, and d = 0.59; Figure 5). However, relative skull length and width was greater in males compared to females as indicated by significantly different regression constants (skull length: t = 3.849, p < 0.001, and d = 1.27; skull width: t = 6.665, p < 0.001, and d = 2.19). There was a mean sex difference of 1.5 mm (SD: 0.39) in skull length and 2.4 mm (SD: 0.36) in width at equal body size, conforming to 4.4 and 10.1% of mean female skull length and width, respectively.
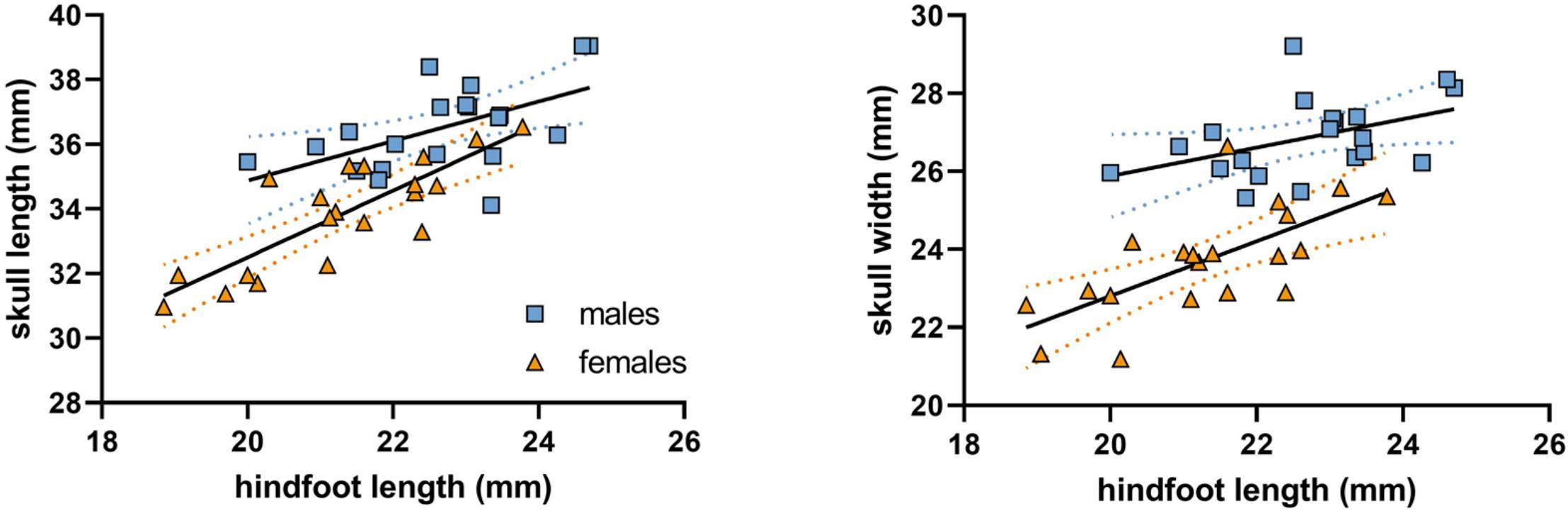
Figure 5. Cranial length and width in Ansell’s mole-rat in relation to body size (approximated by hindfoot length). Dotted lines indicate 95% confidence intervals of sex-specific regression lines.
2D Geometric Morphometrics of Cranium and Mandible
Analyses on the two landmark sets corresponding to dorsal and ventral cranium yielded similar results, while the mandibular dataset deviated from the latter ones in some respects, being less sensitive to influences of sex and skull size. In the dorsal and ventral cranial datasets, the PCA was able to separate specimens well regarding sex but not breeding status. However, the first two principal components encompassed only a fraction of total variance (38.1 and 44.8%, respectively; Figure 6), indicating high overall morphological variability. A similar pattern was recovered for the mandibular dataset, although morphospace overlap between the sexes as well as the variance explained by the first two PCs (49.3%) was greater compared to the other ones (Figure 6, see below). Accordingly, only sex was recovered to significantly influence the shape of the dorsal (F = 6.36, p = 0.001) and ventral cranium (F = 5.32, p = 0.001) as well as mandibular shape (F = 4.82, p = 0.001). Reproductive status or the combined effect of sex and breeding status had no significant effect on these traits (p > 0.1). Shape allometry was detected for the dorsal (F = 3.80, p = 0.001) and ventral (F = 3.17, p = 0.001) cranium, but not for the mandible (F = 1.97, p = 0.06). However, since males had almost consistently larger skulls than females (Figure 6), this result is trivial. No pattern of allometry was found among specimens of the same sex in either landmark dataset (dorsal: F = 0.69, p = 0.25; ventral: F = -0.44, p = 0.66; and mandible: F = 1.1, p = 0.40).
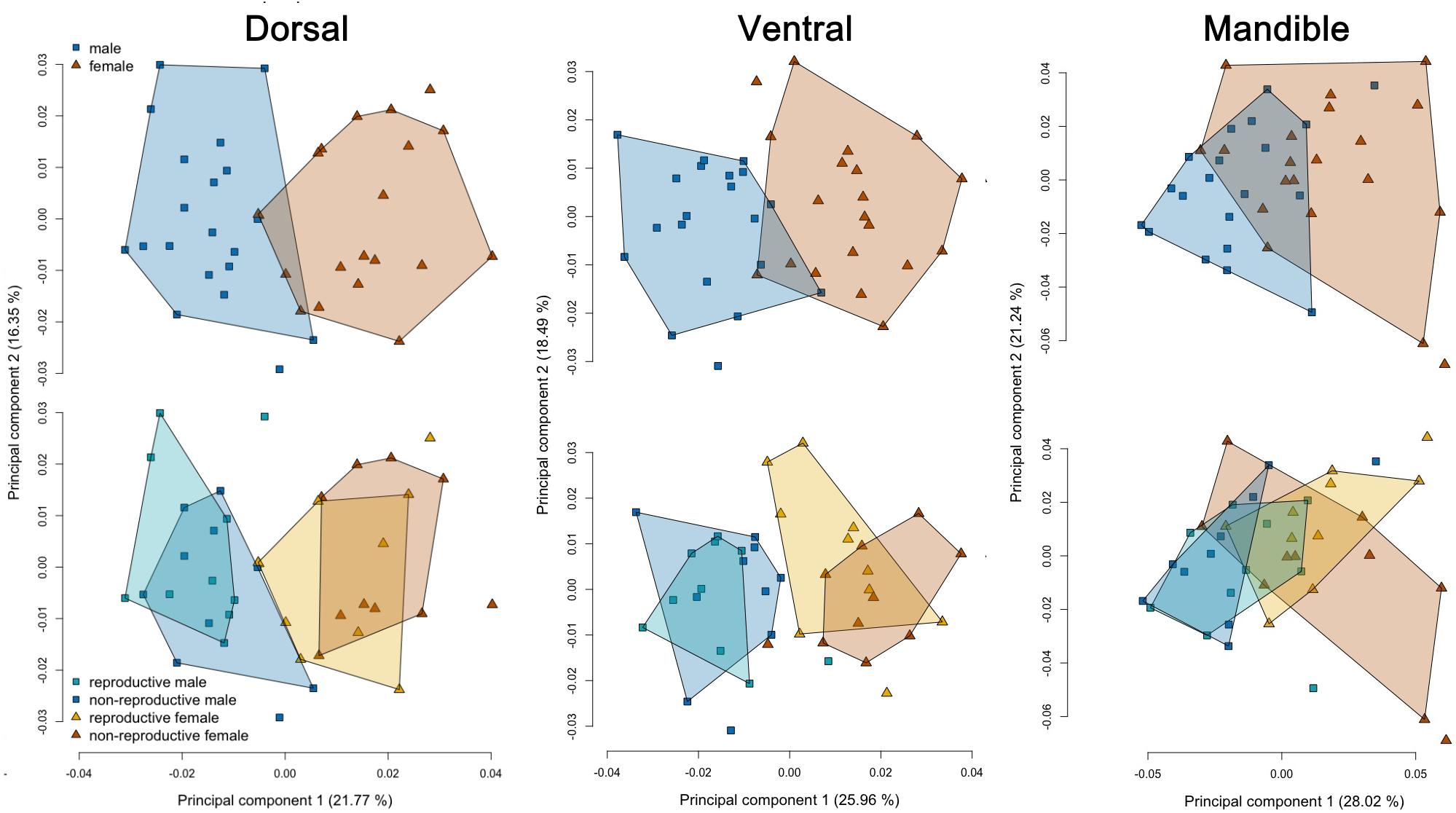
Figure 6. Visualization of PCA-results based on geometric morphometrics of the cranium and mandible in F. anselli. Specimens are well separated by sex in plots based of dorsal and ventral landmarks, while overlap between the sexes is large for the mandibular dataset. No separation of reproductive groups was achieved. Polygons indicate the 95% convex hulls.
Compared to females, the facial portion of the skull in males is enlarged, with longer rostra and widened zygomatic arches, which flare out more anteriorly (Figure 7). When scaled to the same size, the braincase and occipital region of male skulls therefore appear notably compressed compared to those of females. Figure 7 visualizes shape differences between male and female F. anselli in a vector plot, highlighting relevant shape differences. Shape variable contributions and eigenvalues of PCs can be derived from Supplementary Table 3. Male mandibles were found to display a deeper and slightly more elongated processus angularis (landmark 6: 7.8% of PC1 variance, 16.9% of PC2 variance; landmark 7: 41.2% of PC1 variance, 22.7% of PC 2 variance) thicker lower incisors (landmark 9: 23.2% of PC1 variance, 14% of PC2 variance) and a longer, more sickle shaped coronoid process (landmark 1: 14.4% of PC1 variance, 12% of PC2 variance), when compared to females (Figure 7). However, sex differences in the shape of the lower jaw were altogether less pronounced than the ones in cranial shape. This was indicated by a more pronounced morphospace overlap and markedly higher error rates for assignments of individuals to one sex based on mandibular compared to cranial shape (Table 5, see below).
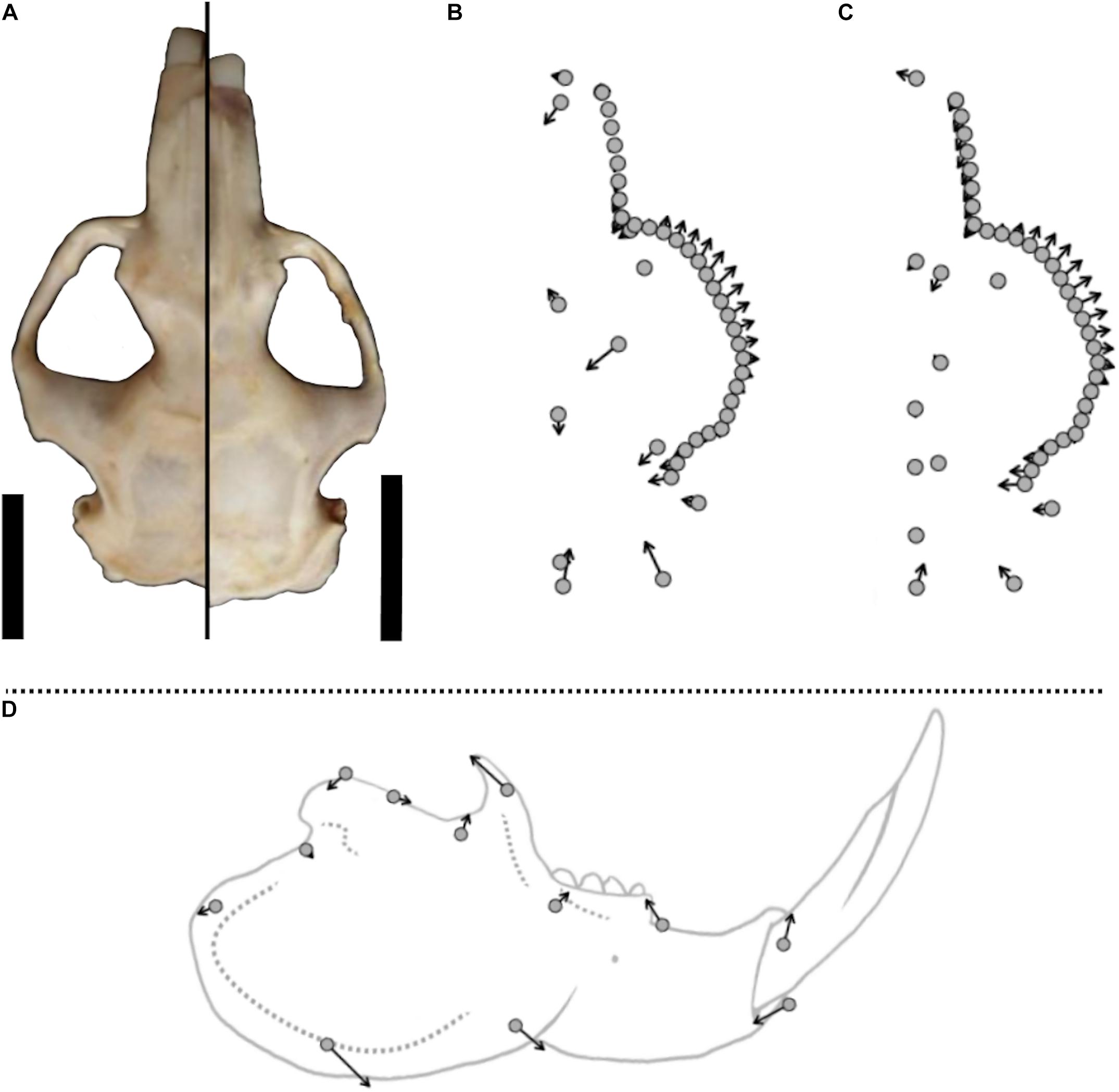
Figure 7. Sex-specific shape differences in the cranium and mandible of F. anselli. Vector plots illustrate mean sex differences with vectors 4x magnified. Points indicate landmarks on female crania, arrows indicate landmark replacements in male crania compared to female ones. (A) Dorsal perspective of a male (left) and female (right) cranium, scaled to the same size and centered on the level of the orbita. Scale bars equal 10 mm and correspond to the specimen’s original size. (B) Vector plot of the dorsal cranium. (C) Vector plot of the ventral cranium. (D) Vector plot of the mandible superimposed on a scheme of a male mandible.
Cranial shape was found to be highly diagnostic for the sexes in F. anselli, but more so for males than for females mirroring the results from linear measurements (Table 5). LDA assigned 90% of males and 85% of females correctly based on dorsal cranial shape, while the ventral dataset allowed to classify 90% of males and 85% of females correctly. In both cases, these proportions differed significantly from random assignments (p ≤ 0.001). The mandibular shape yielded less definite results: 70% of males and 65% of females were assigned to the correct categories, rates that did not significantly deviate from chance levels (p > 0.05). All intrasexual assignments based on reproductive status were randomized (p > 0.1).
Discussion
Occurrence and Scaling of SSD in the Bathyergidae
Regression analyses based on both pRMA and two step LG ratios found Rensch’s rule expressed in the monogamous mole-rat genus Fukomys, while only the latter did so for the bathyergid clade as a whole. The evidence for Fukomys following Rensch’s rule is therefore robust, while its validity for bathyergids might still be questioned. However, although the pRMA regression did not reveal bathyergid SSD scaling significantly deviating from an isometric trajectory, a statistical trend (p < 0.1) emerged. If this is considered together with the positive results from the LG ratio based regression, one could therefore tentatively assume that Rensch’s rule could apply to bathyergids on family level as well. Differences in the clarity of analytical outcomes between the two studied groups (Fukomys versus all bathyergids) might result from more uniform intrasexual selection pressures in Fukomys, when compared to other genera, which encompass a greater diversity of mating systems (compare Bray et al., 2012; Visser et al., 2017; Braude et al., 2020). Still, with the exception of Heterocephalus, aggressive competition between males, deduced either from direct observations (see below for Fukomys and, e.g., Jarvis and Bennett, 1991 for Bathyergus and Oosthuizen, 2008 for Georychus) or from morphological correlates such as sexual dimorphism, is evident throughout the bathyergid radiation. As such, it could underly the prevalence and scaling of SSD in these different taxa.
It is, however, important to point at the limitations of our dataset: Various studies have shown that intraspecific variation in bathyergid SSD (as approximated by body mass) can correlate with geographic provenance (Cryptomys hottentotus – Spinks et al., 2000; Fukomys mechowii — Scharff, 1998) and season of the year (Georychus capensis – Oosthuizen, 2008), factors for which we did not and could not control. Additionally, as we only included adult animals as assigned by the respective studies, we have to expect inconsistencies in the assignment of somatic maturity by the various authors we drew data from (see below). We must therefore consider a certain bias to our dataset. However, since most recovered rates of SSD correspond well to general reporting in the literature, we do not see the validity of our results compromised in that regard. Still, a more comprehensive dataset would have been desirable, especially regarding Fukomys species occurring North to the equator and for unambiguously localized populations of the solitary bathyergid genera, which might encompass significantly more species than currently recognized (Visser et al., 2019). However, biometric information on these mole-rats is currently unavailable.
Smaller-bodied species in our dataset (<150 g) are more variable regarding the expression of dimorphism than larger ones, where marked male-biased SSD is prevalent (Table 1). Among the small species, SSD is particularly pronounced in Cryptomys, in which male reproductive competition can be intense since multiple breeding males are regularly cohabiting a burrow and high incidences of extra-group paternity are common (Bishop et al., 2004). Bathyergids with strongly expressed SSD such as Cryptomys ssp. and F. mechowii are even among the most dimorphic of all rodents (Schulte-Hostedde, 2007), pointing to a crucial biological relevance of SSD in these animals. The latter must be especially emphasized for the genus Fukomys, where SSD scaling unambiguously follows Rensch’s rule.
It appears that if at all, only modest SSD should be considered the ancestral condition in the Bathyergidae. Notable SSD is predominately found in derived bathyergids and is missing in the most basal genus Heterocephalus as well as among the closely allied rodent taxa Thryonomyidae and Petromuridae (Adu et al., 2002; Rathbun and Rathbun, 2006). Still, future studies might try to retrace the evolution of SSD in African hystricognaths in detail to eventually solve this question. Clarifying the matter might be specifically relevant to add to our understanding of the independent evolution of eusociality in Fukomys and Heterocephalus, which apparently derived from ancestors that strongly differed in SSD. In any case, some bathyergid SSD patterns will remain challenging to explain (compare Figure 1). The lack of SSD in Georychus appears to be secondarily acquired and is unexpected in an aggressively territorial solitary species. Not only are other non-social bathyergids, including its sister genus Bathyergus, dimorphic, but well-developed SSD is found in the majority of solitary subterranean rodents (Ctenomyidae – Martínez and Bidau, 2016; Geomyidae – Daly and Patton, 1986; Mauk et al., 1999; and Spalacidae – Su et al., 2018). On the other hand, the presence of pronounced SSD in at least several of the monogamous Fukomys species is surprising: their social system is commonly assumed to result in monomorphic sexes, and compliance to Rensch’s rule could not be expected a priori. Possible explanations for this trait combination based on male-biased dynamic replacement of breeders will be discussed below.
Effects of Breeding Status on Skull Morphology in F. anselli
None of our analyses recovered significant differences between breeding and non-breeding mole-rats of the same sex, while sexual dimorphism was found to be pronounced within both groups. An important caveat to our analysis is the limited sample size of only ten individuals per status group and sex, especially in light of the high morphological variability we recover. However, since none of the different datasets we compared indicated even a trend of morphological segregation, we are reasonably confident that our findings reflect the actual conditions. Additionally, although our study is the first to explicitly address potential differences in skull morphology between mole-rats of different reproductive status, previous publications have already alluded to the lack of status-dependent cranial differentiation in Fukomys (Thorley et al., 2018a). Still, there surely is the possibility that future studies relying on more refined methods, such as 3D geometric morphometrics, may show detectable differences between mole-rat status groups. Nevertheless, even if such disparities would eventually be demonstrated, respective traits will be far more subtle than general sex-specific skull characteristics.
The uniformity of cranial characters contrasts with the pronounced differences in postcranial skeletal anatomy of breeding and non-breeding females in this genus (Thorley et al., 2018a) and points to similar functional demands to the skull among reproductive and non-reproductive mole-rats. Although there is evidence that non-reproductive Fukomys mole-rats are generally more active (Šklíba et al., 2016; Van Daele et al., 2019; Francioli et al., 2020; Houslay et al., 2020), both breeders and helpers engage in the same set of tasks, resulting in quantitative rather than qualitative differences in behavior, which could explain the lack of cranial divergence between them. Our results suggest that breeders do not develop more formidable weaponry or experience further somatic growth (safe for already documented allometric changes in breeding females’ postcranium – Thorley et al., 2018a) after acquiring their status. Still, having more powerful jaws or greater body size might enhance the chances of becoming a breeder in the wild (compare Young and Bennett, 2013).
Field studies reported reproductives (excluding pregnant females) to be significantly heavier than helpers in F. anselli (Sichilima et al., 2011). de Bruin et al. (2012) even describe breeders (males: 81.45 ± 13.71 g, n = 18; females: 63.87 ± 11.39 g, n = 19) to be on average roughly two times as heavy as helpers (males: 39.80 ± 18.97 g, n = 49; females: 33.47 ± 11.78 g, n = 64). However, there are several problems with status assignment in these studies. First, the authors assume a very early onset of maturity, classifying individuals ≥35 g as adults, whereas laboratory results suggest that this species reaches adulthood at approximately two times this body mass (Burda and Begall, 1998). We consider this difference to be too extreme to simply derive from the likely higher growth rates in provisioned captive animals. The assumption of such an early onset of maturity will strongly skew the results toward lower weights in non-reproductive animals. This way, a certain fraction of immature individuals will be counted as adult helpers since diagnostic traits of breeders are missing. Such practices will also bias estimations of SSD (see above). Unfortunately, precise aging of mole-rats in the wild is not possible offhand. Second, typically employed phenotypical criteria to assign breeding status in wild F. anselli males (and some congeneric species), such as testes size estimated by palpation or perioral secretions, are ambiguous. The latter frequently occur in non-reproductive males, at least in captivity (pers. obs.) and apart from the difficulty of assessing testes size by touch, contradicting findings have been published on testes volume differences between reproductive groups (de Bruin et al., 2012; Garcia Montero et al., 2016). Therefore, the reliability of available field data on weight distributions, especially for males can be questioned.
In case significant intrasexual differences in both sexes are confirmed, it needs to be clarified whether wild F. anselli might attain breeding status because of elevated body mass or develop it after succeeding to do so. Since body size is typically only approximated by mass in field studies, findings of intrasexual size differences might simply result from the allocation of limited resources in combination with lower activity levels of breeders in the wild (compare Francioli et al., 2020). In provisioned captive families, no such deviation in body mass is apparent (pers. obs., see Thorley et al., 2018a for F. damarensis) and even the opposite pattern might occur (Schielke et al., 2017). In any case, data on captive animals from this study and others demonstrate that attaining permanent breeding status per se does not go along with an isometric increase in body size (which would affect both hindfoot and cranial measurements, see Figure 5) or body mass in this species.
On a different note, our findings of uniform skull morphology in F. anselli status groups are relevant to Fukomys taxonomy, indicating that missing information about a specimen’s reproductive history does not bias outcomes of anatomical studies.
Effects of Sex on Skull Morphology in F. anselli
In contrast to reproductive status, sex importantly influences both skull size and shape in F. anselli. In fact, F. anselli was also recovered as one of the more dimorphic small (<200 g) Fukomys species in regard to body mass, with males being roughly 20% heavier than females in the wild (Sichilima et al., 2011, but see above for problems with this estimate), with data from captive animals indicating even 40% higher body mass in males (Burda and Begall, 1998). Detected cranial difference are profound and not only point to a larger relative skull size in males but also to greater robustness of the male jaw apparatus. In light of this, it is surprising that a previous landmark analysis of the dorsal and ventral skull in sexually dimorphic F. anselli, F. hanangensis and F. whytei (compare Table 1) found no sex specific shape patterns (Faulkes et al., 2017). However, this study included immature specimens, which could have led to biased results.
Sex-specific cranial differences in F. anselli emerge due to hypertrophy of the facial portion of the skull in males (compare Figure 7), while several measurements of the skull base vary little between the sexes (Table 4). When scaled to the same size, males display wider as well as thicker zygomatic arches and larger angular processes than females which permit the development of a more voluminous musculus masseter, the most important masticatory muscle (Cox et al., 2020). Shape analysis also revealed a higher coronoid process in male mandibles, which together with more pronounced sagittal crests in males (pers. obs.) indicates a more strongly developed musculus temporalis, another jaw adductor (Cox et al., 2020). We therefore predict higher bite forces in male F. anselli compared to females relative to body mass (but see Van Daele et al., 2019 reporting equal bite forces in a small sample of a similar-sized Fukomys species). Apart from that, males can be expected to display a wider gape, since their rostra and mandibles are more elongated than the ones of females (Table 4). Still, there appears to be no noteworthy sex difference in cheek dentition, indicating that masticatory demands do not drive the sex-specific differentiation in the facial skeleton. The incisors on the other hand, which represent the most important weapons of African mole-rats, are hypertrophied in males, indicating adaptation to combat (Young and Bennett, 2013). This sex-specific trait was not only recovered herein but has already been noticed by Burda (1990) for F. anselli and by Young and Bennett (2013) for F. damarensis.
Sexual size dimorphism in relative skull size has been seldomly studied in mammals, but its occurrence was reported for F. damarensis (Young and Bennett, 2013). Our results agree with the respective study, in which head width (cranium and adhering soft tissues) in anesthetized F. damarensis was measured and males were found to have significantly wider heads than females relative to their body size. Unfortunately, head dimensions were only approximated by that single measure and raw data were not communicated, so that no further comparison with our findings on F. anselli can be drawn.
Clues to what underlies relative skull size SSD in mole-rats might derive from comparisons with reptiles, since the phenomenon has been intensively researched in lizards, subterranean amphisbaenians, and snakes, where exaggerated relative male head size is a common trait (Shine, 1991; Gienger and Beck, 2007; Martín et al., 2012; Baird, 2013). In fact, squamate reptiles and mole-rats differ little in that jaw and gape dimensions rather than other physical characteristics can decide conflicts with conspecifics. Just as male-biased body mass SSD in general, it is commonly assumed that enlarged heads in reptiles are intrasexually selected traits (Baird, 2013). Alternatively, ecological niche divergence between sexes could underly this anatomical difference (Shine, 1991, but see Baird, 2013), a factor also hypothesized to explain SSD in some monogamous mammals (e.g., Hillis and Mallory, 1996). However, the peculiar lifestyle and foraging behavior of mole-rats argues against ecological influences on dimorphic head dimensions and again points to males combating competitors. There is also no evidence that male and female Fukomys differ in distances or mode of dispersal (above ground or underground) when leaving their natal family (Finn, 2017), so that an influence of this factor can also be ruled out (compare Young and Bennett, 2013). Instead, all the cranial traits discussed beforehand as well as the pattern of SSD scaling in Fukomys indicate a pronounced role of male-male combat in the social life of this genus. But how does that comply with the monogamous mating strategy of these mole-rats?
Socioecological Implications of Sexual Dimorphism in Fukomys Mole-Rats
Mammals exhibiting social and/or sexual monogamy are not expected to show pronounced SSD (compare Bidau and Martinez, 2016), so that the patterns found in Fukomys are surprising. Field studies employing microsatellite data demonstrated that reproductive skew in wild Fukomys is indeed extremely high. In F. anselli, only a single resident breeder of each sex is present and juveniles are sired almost exclusively by the established breeding male (nfamilies = 13; 96.4% – Patzenhauerová et al., 2013). At the same time, the number of immigrants into established colonies is very small (nnon–breeders = 85; 3.5%) but it is yet unclear whether these data are representative for Fukomys in general. In F. damarensis, a similar field study found the number of immigrants to be higher, at 7.5%, and the rate of extra-pair paternity was increased compared to F. anselli [up to 16% (31.9% if two outlier families are included); Patzenhauerová et al., 2013 referring to Burland et al., 2002]. These results do not challenge the assumption that both social and sexual monogamy is predominant in Fukomys but suggest that the latter may become compromised under specific circumstances.
However, although mating partners stay mostly faithful to each other and do so over long periods of time, once formed pairs can still be disrupted. This process appears to be mediated by male intrusion into established families. Shorter tenure length in reproductive males compared to females has been demonstrated by several genetic and capture-recapture studies (Young and Bennett, 2013, see below), indicating sex-biased social dynamics compliant with asymmetric reproductive competition and male-male combat. Genetic relatedness levels in males compared to females in wild F. damarensis groups also suggest that males do more frequently invade foreign groups and take over a breeding position (Burland et al., 2002). In case a breeder succession happens, or when a foreign male joins a group which lost its male breeder, a “patchwork family” with offspring of mixed descent can be established. Breeding male turnovers by intruders appear not to be rare (but see Torrents-Ticó et al., 2018), as all parentage studies on wild Fukomys found evidence for it in at least one family group analyzed (F. anselli – Patzenhauerová et al., 2013; F. damarensis – Burland et al., 2002; and F. mechowii – Šumbera et al., 2012).
Established wild Fukomys groups therefore exhibit high reproductive skew, experience at least some degree of immigration pressure and can tolerate the replacement of male breeders. The extreme, cooperatively enacted xenophoby found in laboratory-housed Fukomys appears to be an artifact of captivity (compare Bishop et al., 2004 for a similar pattern in Cryptomys). We suggest that the increased rate of male compared to female breeder turnover in the wild might be an unappreciated indication for stronger male intrasexual competition in Fukomys with major implications for SSD. Given the degree of SSD in Fukomys and the cranial adaptations described herein, it is likely that these replacements follow violent attacks. Still, such interactions are not documented so far. The reproductive benefit of securing a reproductive female, a burrow system and even a number of helpers for potentially several years must be extreme and might explain why SSD in Fukomys is more strongly expressed than in many polygynous or polygamous rodents, were mating associations are shorter-lived.
But there are several caveats to this hypothesis. First, it must be explained why such reproductive competition would occur in Fukomys and not in other cooperatively breeding rodents with slow life histories that occupy self-constructed defendable home ranges, such as mole-voles (Ellobius spp.), beavers (Castor spp.) and most importantly, Heterocephalus. It could also be argued that low documented rates of male group takeovers (Torrents-Ticó et al., 2018) could not create a sufficient selective pressure to explain the observed sexual dimorphism. Besides, faster male turnovers might not be provoked by intrasexual combat but by males facing higher mortality risks, for example increased predation pressure. For this, however, one would expect differences in the activity and ranging patterns of males and females, which is so far not apparent in wild Fukomys (F. damarensis – Francioli et al., 2020). A final potential caveat is the lack of intraspecific combat adaptations in Fukomys that are found in other bathyergids: Even large-bodied Fukomys lack defensive dermal shields, a trait found in the dune mole-rats of the genus Bathyergus (Jarvis and Bennett, 1991), which might be expected to evolve convergently in both genera in case males commonly experience violent encounters with competitors.
Conclusion
Our study provides a comprehensive description of the well-developed sexual dimorphism in the skull of F. anselli, which points to a significant role of male competition in the social life of mole-rats belonging to this cooperatively-breeding genus. Fukomys might best be characterized as serially monogamous rodent genus, in which males stay faithful to their partners for prolonged time but have to regularly engage in conflicts with same-sex rivals to secure their mate. That assumption is further supported by the recovery of SSD scaling conforming to Rensch’s rule among Fukomys species, indicating violent monopolization of breeding females by males. At the same time, we show that no morphological differentiation in the cranium of breeders and non-breeders exists, indicating a lack of morpho-functional caste specialization beyond characters relevant to reproduction. The currently available data can only be considered a starting point regarding efforts to understand the evolutionary pressures influencing sexual dimorphism in social mole-rats. Future studies might address the following questions to characterize the evolutionary pressures behind these morphological findings: How frequent are attempts to take over groups by foreign males in the wild and how do invaded family groups react? Why do helpers accept unrelated male breeders within the “patchwork family” scheme? To which degree do Fukomys species differ in respect to this behavior and how do they compare to Cryptomys and Heterocephalus? What factors underly the varying expression of SSD in the three solitary bathyergid genera?
Solving these questions will importantly contribute to fully unravel the remarkable diversity of social patterns found within the Bathyergidae and the factors that shaped it.
Data Availability Statement
The original contributions presented in the study are included in the article/Supplementary Material; further inquiries can be directed to the corresponding author/s.
Author Contributions
KRC conceived the study and its methodology with input from SB. KRC and SB prepared the skulls, took linear measurements, and prepared the figures. JM photographed the skulls and compiled landmark datasets of the dorsal and ventral skull. KRC collected landmark data on the mandibles. SB and JM analyzed the data with input from KRC. All authors interpreted the findings. KRC wrote the first draft, which was reviewed by SB and JM. All authors contributed to the article and approved the submitted version.
Funding
KRC was supported by a Ph.D. fellowship of the German National Academic Foundation (Studienstiftung des deutschen Volkes e.V.). We acknowledge support by the Open Access Publication Fund of the University of Duisburg-Essen.
Conflict of Interest
The authors declare that the research was conducted in the absence of any commercial or financial relationships that could be construed as a potential conflict of interest.
Acknowledgments
We thank Irina Ruf for inviting us to contribute our manuscript to this special issue and two reviewers for their insightful comments on earlier versions of this manuscript. Martin Schnorr is acknowledged for assisting the preparation of the skulls used in this study.
Supplementary Material
The Supplementary Material for this article can be found online at: https://www.frontiersin.org/articles/10.3389/fevo.2021.638754/full#supplementary-material
Supplementary Table 1 | Linear skull measurements and hindfoot lengths of Fukomys anselli specimens used in this study. Yellow mark: Measurement was taken from the left side of the skull. Red mark: Measurement was estimated using the LOST package (Arbour and Brown, 2020).
Supplementary Table 2 | Overview and definitions of point landmarks used to characterize skull traits in Fukomys anselli.
Supplementary Table 3 | Variable contributions (%) and eigenvalues of principal components computed for the shape of the dorsal and ventral cranium as well as for the mandible of Fukomys anselli.
References
Abouheif, E., and Fairbairn, D. J. (1997). A comparative analysis of allometry for sexual size dimorphism: assessing Rensch’s rule. Am. Nat. 149, 540–562. doi: 10.1086/286004
Adams, D. C., Collyer, M., and Kaliontzopoulou, A. (2020). Geomorph: Software for Geometric Morphometric Analyses. R package version 3.2.1.
Adu, E. K., Wallace, P. A., and Ocloo, T. O. (2002). Efficacy of sex determination in the greater cane rat, Thryonomys swinderianus, Temminck. Trop. Anim. Health Product. 34, 27–33.
Ansell, W. F. H. (1965). Standardisation of field data on mammals. Zool. Afr. 1, 97–113. doi: 10.1080/00445096.1965.11447303
Arbour, J., and Brown, C. (2020). LOST: Missing Morphometric Data Simulation and Estimation. R package version 2.0.2.
Baird, T. A. (2013). “Lizards and other reptiles as model systems for the study of contest behaviour,” in Animal Contests, eds C. W. Hardy and M. Briffa (Cambridge: Cambridge University Press), 258–286. doi: 10.1017/cbo9781139051248.014
Bappert, M.-T., Burda, H., and Begall, S. (2012). To mate or not to mate? Mate preference and fidelity in monogamous Ansell’s mole-rats, Fukomys anselli, Bathyergidae. Folia Zool. 61, 71–83. doi: 10.25225/fozo.v61.i1.a11.2012
Barčíová, L., Šumbera, R., and Burda, H. (2009). Variation in the digging apparatus of the subterranean silvery mole-rat, Heliophobius argenteocinereus (Rodentia, Bathyergidae): the role of ecology and geography. Biol. J. Linn. Soc. 97, 822–831. doi: 10.1111/j.1095-8312.2009.01228.x
Begall, S., Nappe, R., Hohrenk, L., Schmidt, T. C., Burda, H., et al. (2021). Life expectancy, family constellation, and stress in giant mole-rats (Fukomys mechowii). Philos. Trans. R. Soc. B 376:20200207. doi: 10.1098/rstb.2020.0207
Bennett, N. C., Faulkes, C. G., Hart, L., and Jarvis, J. U. M. (2009). Bathyergus suillus. Mamm. Spec. 2009, 1–7. doi: 10.1644/828.1
Bennett, N. C., and Jarvis, J. U. M. (2004). Cryptomys damarensis. Mammal. Spec. 2004, 1–5. doi: 10.1644/756
Bennett, N. C., Jarvis, J. U. M., and Cotterill, F. P. D. (1994). The colony structure and reproductive biology of the afrotropical Mashona mole-rat, Cryptomys darlingi. J. Zool. 234, 477–487. doi: 10.1111/j.1469-7998.1994.tb04861.x
Bidau, C. J., and Martinez, P. A. (2016). Sexual size dimorphism and Rensch’s rule in Canidae. Biol. J. Linn. Soc. 119, 816–830. doi: 10.1111/bij.12848
Bishop, J. M., Jarvis, J. U. M., Spinks, A. C., Bennett, N. C., and O’Ryan, C. (2004). Molecular insight into patterns of colony composition and paternity in the common mole-rat Cryptomys hottentotus hottentotus. Mol. Ecol. 13, 1217–1229. doi: 10.1111/j.1365-294x.2004.02131.x
Braude, S., Hess, J., and Ingram, C. (2020). Inter-colony invasion between wild naked mole-rat colonies. J. Zool. 313:12834.
Bray, T. C., Bloomer, P., O’Riain, M. J., and Bennett, N. C. (2012). How attractive is the girl next door? An assessment of spatial mate acquisition and paternity in the solitary Cape dune mole-rat, Bathyergus suillus. PLoS One 7:e39866. doi: 10.1371/journal.pone.0039866
Brett, R. A. (1991). “The population structure of naked mole-rat colonies,” in The Biology of the Naked Mole-Rat, eds P. W. Sherman, J. U. M. Jarvis, and R. D. Alexander (New Jersey: Princeton Univ. Press), 97–136. doi: 10.1515/9781400887132-007
Burda, H. (1990). Constraints of pregnancy and evolution of sociality in mole-rats with special reference to reproductive and social patterns in Cryptomys hottentotus (Bathyergidae, Rodentia). J. Zool. Syst. Evol. Res. 28, 26–39. doi: 10.1111/j.1439-0469.1990.tb00362.x
Burda, H., and Begall, S. (1998). Reproductive characteristics and growth in the eusocial Zambian common mole-rat (Cryptomys sp., Bathyergidae). Z. Säugetierkunde 63, 297–306.
Burda, H., Honeycutt, R. L., Begall, S., Locker-Grütjen, O., and Scharff, A. (2000). Are naked and common mole-rats eusocial and if so, why? Behav. Ecol. Sociobiol. 47, 293–303. doi: 10.1007/s002650050669
Burda, H., Šumbera, R., Chitaukali, W. N., and Dryden, G. L. (2005). Taxonomic status and remarks on ecology of the Malawian mole-rat Cryptomys whytei (Rodentia, Bathyergidae). Acta Theriol. 50, 529–536. doi: 10.1007/bf03192646
Burland, T. M., Bennett, N. C., Jarvis, J. U. M., and Faulkes, C. G. (2002). Eusociality in African mole-rats: new insights from patterns of genetic relatedness in the Damaraland mole-rat (Cryptomys damarensis). Proc. R. Soc. Lond. Ser. B Biol. Sci. 269, 1025–1030. doi: 10.1098/rspb.2002.1978
Chimimba, C. T., Sichilima, A. M., Faulkes, C. G., and Bennett, N. C. (2010). Ontogenetic variation and craniometric sexual dimorphism in the social giant mole-rat, Fukomys mechowii (Rodentia: Bathyergidae), from Zambia. Afr. Zool. 45, 160–176. doi: 10.3377/004.045.0218
Cohas, A., and Allainé, D. (2009). Social structure influences extra-pair paternity in socially monogamous mammals. Biol. Lett. 5, 313–316. doi: 10.1098/rsbl.2008.0760
Cox, P. G., Faulkes, C. G., and Bennett, N. C. (2020). Masticatory musculature of the African mole-rats (Rodentia: Bathyergidae). PeerJ 8:e8847. doi: 10.7717/peerj.8847
Dale, J., Dunn, P. O., Figuerola, J., Lislevand, T., Székely, T., and Whittingham, L. A. (2007). Sexual selection explains Rensch’s rule of allometry for sexual size dimorphism. Proc. R. Soc. B Biol. Sci. 274, 2971–2979. doi: 10.1098/rspb.2007.1043
Daly, J. C., and Patton, J. L. (1986). Growth, reproduction, and sexual dimorphism in Thomomys bottae pocket gophers. J. Mammal. 67, 256–265. doi: 10.2307/1380878
Dammann, P., and Burda, H. (2006). Sexual activity and reproduction delay ageing in a mammal. Curr. Biol. 16, R117–R118.
de Bruin, P. R., Viljoen, H., Sichilima, A. M., and Bennett, N. C. (2012). Socially induced infertility in Ansell’s mole-rat: are there depressed hormone levels in non-reproductive males and females? J. Zool. 286, 15–21. doi: 10.1111/j.1469-7998.2011.00841.x
Dengler-Crish, C. M., and Catania, K. C. (2007). Phenotypic plasticity in female naked mole-rats after removal from reproductive suppression. J. Exp. Biol. 210:4351. doi: 10.1242/jeb.009399
Fairbairn, D. J. (1997). Allometry for sexual size dimorphism: pattern and process in the coevolution of body size in males and females. Annu. Rev. Ecol. Syst. 28, 659–687. doi: 10.1146/annurev.ecolsys.28.1.659
Faulkes, C. G., Mgode, G. F., Archer, E. K., and Bennett, N. C. (2017). Relic populations of Fukomys mole-rats in Tanzania: description of two new species F. livingstoni sp. nov. and F. hanangensis sp. nov. PeerJ 5:e3214. doi: 10.7717/peerj.3214
Finn, K. T. (2017). Density-Dependent Effects on Body Size, Philopatry, and Dispersal in the Damaraland Mole-Rat (Fukomys damarensis). M.Sc. thesis. Grahamstown: Rhodes University.
Francioli, Y., Thorley, J., Finn, K., Clutton-Brock, T., and Zöttl, M. (2020). Breeders are less active foragers than non-breeders in wild Damaraland mole-rats. Biol. Lett. 16:20200475. doi: 10.1098/rsbl.2020.0475
Gabathuler, U., Bennett, N. C., and Jarvis, J. U. M. (1996). The social structure and dominance hierarchy of the Mashona mole-rat, Cryptomys darlingi (Rodentia: Bathyergidae) from Zimbabwe. J. Zool. 240, 221–231. doi: 10.1111/j.1469-7998.1996.tb05281.x
Garcia Montero, A., Vole, C., Burda, H., Malkemper, E. P., Holtze, S., Morhart, M., et al. (2016). Non-breeding eusocial mole-rats produce viable sperm—spermiogram and functional testicular morphology of Fukomys anselli. PLoS One 11:e0150112. doi: 10.1371/journal.pone.0150112
Gienger, C. M., and Beck, D. D. (2007). Heads or tails? Sexual dimorphism in helodermatid lizards. Can. J. Zool. 85, 92–98. doi: 10.1139/z06-198
Gomes Rodrigues, H., Šumbera, R., and Hautier, L. (2016). Life in burrows channelled the morphological evolution of the skull in rodents: the case of African mole-Rats (Bathyergidae, Rodentia). J. Mamm. Evol. 23, 175–189. doi: 10.1007/s10914-015-9305-x
Hart, L., Chimimba, C. T., Jarvis, J. U. M., O’Riain, J., and Bennett, N. C. (2007). Craniometric sexual dimorphism and age variation in the South African Cape dune mole-rat (Bathyergus suillus). J. Mammal. 88, 657–666. doi: 10.1644/06-mamm-a-058r1.1
Herbst, M., Jarvis, J. U. M., and Bennett, N. C. (2004). A field assessment of reproductive seasonality in the threatened wild Namaqua dune mole-rat (Bathyergus janetta). J. Zool. 263, 259–268. doi: 10.1017/s0952836904005114
Heske, E. J., and Ostfeld, R. S. (1990). Sexual dimorphism in size, relative size of testes, and mating systems in North American voles. J. Mammal. 71, 510–519. doi: 10.2307/1381789
Hillis, T. L., and Mallory, F. F. (1996). Sexual dimorphism in wolves (Canis lupus) of the Keewatin District, Northwest Territories, Canada. Can. J. Zool. 74, 721–725. doi: 10.1139/z96-081
Houslay, T. M., Vullioud, P., Zöttl, M., and Clutton-Brock, T. H. (2020). Benefits of cooperation in captive Damaraland mole-rats. Behav. Ecol. 31, 711–718. doi: 10.1093/beheco/araa015
Ingram, C. M., Burda, H., and Honeycutt, R. L. (2004). Molecular phylogenetics and taxonomy of the African mole-rats, genus Cryptomys and the new genus Coetomys Gray, 1864. Mol. Phylogenet. Evol. 31, 997–1014. doi: 10.1016/j.ympev.2003.11.004
Jarvis, J. U. M., and Bennett, N. C. (1991). “Ecology and behaviour of the family Bathyergidae,” in The Biology of the Naked Mole-Rat, eds P. W. Sherman, J. U. M. Jarvis, and R. D. Alexander (New Jersey: Princeton Univ. Press), 66–96. doi: 10.1515/9781400887132-006
Lacey, E. A., and Sherman, P. W. (1991). “Social organization of naked-mole rat colonies: evidence for the division of labor,” in The Biology of the Naked Mole-Rat, eds P. W. Sherman, J. U. M. Jarvis, and R. D. Alexander (New Jersey: Princeton University Press), 275–336. doi: 10.1515/9781400887132-013
Lu, D., Zhou, C. Q., and Liao, W. B. (2014). Sexual size dimorphism lacking in small mammals. N. W. J. Zool. 10, 53–59.
Martín, J., Polo-Cavia, N., Gonzalo, A., López, P., and Civantos, E. (2012). Sexual dimorphism in the North African amphisbaenian Trogonophis wiegmanni. J. Herpetol. 46, 338–341. doi: 10.1670/10-286
Martínez, P. A., and Bidau, C. J. (2016). A re-assessment of Rensch’s rule in tuco-tucos (Rodentia: Ctenomyidae: Ctenomys) using a phylogenetic approach. Mamm. Biol. 81, 66–72. doi: 10.1016/j.mambio.2014.11.008
Mauk, C. L., Houck, M. A., and Bradley, R. D. (1999). Morphometric analysis of seven species of pocket gophers (Geomys). J. Mammal. 80, 499–511. doi: 10.2307/1383296
Moshkin, M. P., Novikov, E. A., and Petrovski, D. V. (2001). Seasonal changes of thermoregulation in the mole vole Ellobius talpinus. Physiol. Biochem. Zool. 74, 869–875. doi: 10.1086/324750
Oosthuizen, M. K. (2008). The Reproductive Biology of the Solitary Cape Mole-Rat, Georychus capensis and the Social Natal mole-rat, Cryptomys hottentotus Natalensis (Rodentia : Bathyergidae). Ph.D. thesis. Pretoria: University of Pretoria.
Patzenhauerová, H., Bryja, J., and Šumbera, R. (2010). Kinship structure and mating system in a solitary subterranean rodent, the silvery mole-rat. Behav. Ecol. Sociobiol. 64, 757–767. doi: 10.1007/s00265-009-0893-4
Patzenhauerová, H., Šklíba, J., Bryja, J., and Šumbera, R. (2013). Parentage analysis of Ansell’s mole-rat family groups indicates a high reproductive skew despite relatively relaxed ecological constraints on dispersal. Mol. Ecol. 22, 4988–5000. doi: 10.1111/mec.12434
Rathbun, G. B., and Rathbun, C. D. (2006). Social monogamy in the noki or dassie-rat (Petromus typicus) in Namibia. Mamm. Biol. 71, 203–213. doi: 10.1016/j.mambio.2006.01.008
Rensch, B. (1950). Die Abhängigkeit der relativen Sexualdifferenz von der Körpergröße. Bonner Zool. Beiträge 1, 58–69.
Revell, L. J. (2012). phytools: an R package for phylogenetic comparative biology (and other things). Methods Ecol. Evol. 3, 217–223. doi: 10.1111/j.2041-210x.2011.00169.x
Rohlf, F. J. (2018). tpsDig2, Digitize Landmarks and Outlines, Version 2.31. New York, NY: Department of Ecology and Evolution, State University of New York.
Scharff, A. (1998). Systematik und Verhaltensökologie sambischer Sandgräber (Bathyergidae, Rodentia). Ph.D. thesis. Duisburg: University of Essen.
Schielke, C. K. M., Burda, H., Henning, Y., Okrouhlík, J., and Begall, S. (2017). Higher resting metabolic rate in long-lived breeding Ansell’s mole-rats (Fukomys anselli). Front. Zool. 14:45. doi: 10.1186/s12983-017-0229-6
Schulte-Hostedde, A. (2007). “Chapter 10 Sexual size dimorphism in rodents,” in Rodent Societies: An Ecological and Evolutionary Perspective, eds J. O. Wolff and P. W. Sherman (Chicago, IL: The University of Chicago Press), 115–128.
Shine, R. (1991). Intersexual dietary divergence and the evolution of sexual dimorphism in snakes. Am. Nat. 138, 103–122. doi: 10.1086/285207
Sichilima, A. M., Bennett, N. C., and Faulkes, C. G. (2011). Field evidence for colony size and aseasonality of breeding and in Ansell’s mole-rat, Fukomys anselli (Rodentia: Bathyergidae). Afr. Zool. 46, 334–339. doi: 10.1080/15627020.2011.11407506
Sichilima, A. M., Faulkes, C. G., and Bennett, N. C. (2008). Field evidence for aseasonality of reproduction and colony size in the Afrotropical giant mole-rat Fukomys mechowii (Rodentia: Bathyergidae). Afr. Zool. 43, 144–149. doi: 10.3377/1562-7020-43.2.144
Šklíba, J., Lövy, M., Burda, H., and Šumbera, R. (2016). Variability of space-use patterns in a free living eusocial rodent, Ansell’s mole-rat indicates age-based rather than caste polyethism. Sci. Rep. 6:37497.
Smith, R. J. (1999). Statistics on sexual size dimorphism. J. Hum. Evol. 36, 423–459. doi: 10.1006/jhev.1998.0281
Spinks, A. C., Bennett, N. C., and Jarvis, J. U. M. (2000). A comparison of the ecology of two populations of the common mole-rat, Cryptomys hottentotus hottentotus: the effect of aridity on food, foraging and body mass. Oecologia 125, 341–349. doi: 10.1007/s004420000460
Su, J., Hegab, I. M., Ji, W., and Nan, Z. (2018). Function-related drivers of skull morphometric variation and sexual size dimorphism in a subterranean rodent, plateau zokor (Eospalax baileyi). Ecol. Evol. 8, 4631–4643. doi: 10.1002/ece3.3986
Šumbera, R., Burda, H., and Chitaukali, W. N. (2003). Reproductive biology of a solitary subterranean bathyergid rodent, the silvery mole-rat (Heliophobius argenteocinereus). J. Mammal. 84, 278–287. doi: 10.1644/1545-1542(2003)084<0278:rboass>2.0.co;2
Šumbera, R., Mazoch, V., Patzenhauerová, H., Lövy, M., Šklíba, J., Bryja, J., et al. (2012). Burrow architecture, family composition and habitat characteristics of the largest social African mole-rat: the giant mole-rat constructs really giant burrow systems. Acta Theriol. 57, 121–130. doi: 10.1007/s13364-011-0059-4
Taylor, P. J., Jarvis, J. U. M., Crowe, T. M., and Davies, K. C. (1985). Age determination in the Cape molerat Georychus capensis. S. Afr. J. Zool. 20, 261–267.
Thomas, H. G., Bateman, P. W., Scantlebury, M., and Bennett, N. C. (2012). Season but not sex influences burrow length and complexity in the non-sexually dimorphic solitary Cape mole-rat (Rodentia: Bathyergidae). J. Zool. 288, 214–221. doi: 10.1111/j.1469-7998.2012.00944.x
Thorley, J., Katlein, N., Goddard, K., Zöttl, M., and Clutton-Brock, T. (2018a). Reproduction triggers adaptive increases in body size in female mole-rats. Proc. R. Soc. B Biol. Sci. 285:20180897. doi: 10.1098/rspb.2018.0897
Thorley, J., Mendonça, R., Vullioud, P., Torrents-Ticó, M., Zöttl, M., Gaynor, D., et al. (2018b). No task specialization among helpers in Damaraland mole-rats. Anim. Behav. 143, 9–24. doi: 10.1016/j.anbehav.2018.07.004
Torrents-Ticó, M., Bennett, N. C., Jarvis, J. U. M., and Zöttl, M. (2018). Sex differences in timing and context of dispersal in Damaraland mole-rats (Fukomys damarensis). J. Zool. 306, 252–257. doi: 10.1111/jzo.12602
Van Daele, P. A., Blonde, P., Stjernstedt, R., and Adriaens, D. (2013). A new species of African mole-rat (Fukomys, Bathyergidae, Rodentia) from the Zaire-Zambezi watershed. Zootaxa 3636, 171–189. doi: 10.11646/zootaxa.3636.1.7
Van Daele, P. A. A. G., Desmet, N., Šumbera, R., and Adriaens, D. (2019). Work behaviour and biting performance in the cooperative breeding Micklem’s mole-rat Fukomys micklemi (Bathyergidae, Rodentia). Mamm. Biol. 95, 69–76. doi: 10.1016/j.mambio.2019.02.002
van Jaarsveld, B., Bennett, N. C., Hart, D. W., and Oosthuizen, M. K. (2019). Locomotor activity and body temperature rhythms in the Mahali mole-rat (C. h. mahali): the effect of light and ambient temperature variations. J. Therm. Biol. 79, 24–32. doi: 10.1016/j.jtherbio.2018.11.013
van Rensburg, L. J., Chimimba, C. T., van der Merwe, M., Schoeman, A. S., and Bennett, N. C. (2004). Relative age and reproductive status in Cryptomys hottentotus pretoriae (Rodentia: Bathyergidae) from South Africa. J. Mammal. 85, 1225–1232. doi: 10.1644/ber-113.1
Visser, J. H., Bennett, N. C., and Jansen van Vuuren, B. (2017). Distributional range, ecology, and mating system of the Cape mole-rat (Georychus capensis) family Bathyergidae. Can. J. Zool. 95, 713–726. doi: 10.1139/cjz-2017-0016
Visser, J. H., Bennett, N. C., and Jansen van Vuuren, B. (2019). Phylogeny and biogeography of the African Bathyergidae: a review of patterns and processes. PeerJ 7:e7730. doi: 10.7717/peerj.7730
Webb, T. J., and Freckleton, R. P. (2007). Only half right: species with female-biased sexual size dimorphism consistently break Rensch’s rule. PLoS One 2:e897. doi: 10.1371/journal.pone.0000897
Yeboah, S., and Dakwa, K. B. (2002). Colony and social structure of the Ghana mole-rat (Cryptomys zechi, Matchie) (Rodentia: Bathyergidae). J. Zool. 256, 85–91. doi: 10.1017/s0952836902000110
Young, A. J., and Bennett, N. C. (2013). Intra-sexual selection in cooperative mammals and birds: why are females not bigger and better armed? Philos. Trans. R. Soc. B Biol. Sci. 368:20130075. doi: 10.1098/rstb.2013.0075
Keywords: Bathyergidae, Rensch’s rule, shape analysis, osteology, geometric morphometrics
Citation: Caspar KR, Müller J and Begall S (2021) Effects of Sex and Breeding Status on Skull Morphology in Cooperatively Breeding Ansell’s Mole-Rats and an Appraisal of Sexual Dimorphism in the Bathyergidae. Front. Ecol. Evol. 9:638754. doi: 10.3389/fevo.2021.638754
Received: 08 December 2020; Accepted: 11 May 2021;
Published: 24 June 2021.
Edited by:
Lucja A. Fostowicz-Frelik, Institute of Paleobiology, Polish Academy of Sciences, PolandReviewed by:
Daniel Frynta, Charles University, CzechiaWieslaw Bogdanowicz, Museum and Institute of Zoology, Polish Academy of Sciences, Poland
Copyright © 2021 Caspar, Müller and Begall. This is an open-access article distributed under the terms of the Creative Commons Attribution License (CC BY). The use, distribution or reproduction in other forums is permitted, provided the original author(s) and the copyright owner(s) are credited and that the original publication in this journal is cited, in accordance with accepted academic practice. No use, distribution or reproduction is permitted which does not comply with these terms.
*Correspondence: Kai R. Caspar, a2FpLmNhc3BhckB1bmktZHVlLmRl; Sabine Begall, c2FiaW5lLmJlZ2FsbEB1bmktZHVlLmRl