- 1Instituto de Biodiversidad Neotropical, UNT-CONICET, San Miguel de Tucumán, Argentina
- 2Cátedra de Biología General, Facultad de Ciencias Naturales e IML, Universidad Nacional de Tucumán, San Miguel de Tucumán, Argentina
- 3Cátedra de Vertebrados, Facultad de Ciencias Naturales, Universidad Nacional de Salta (UNSa), Salta, Argentina
- 4Cátedra de Biología Animal, Facultad de Ciencias Naturales e IML, Universidad Nacional de Tucumán, San Miguel de Tucumán, Argentina
Geographic Information System (GIS) is a system that captures, stores, manipulates, analyzes, manages, and presents spatial or geographical data. As this technological environment has been created to deal with space problems, it is perfectly adaptable to solve these type of issues in the context of vertebrate comparative morphology. The pectoral and pelvic girdles are key structures that relate the axial skeleton with the limbs in tetrapods. Owed to their importance in locomotion, the morphology, development, and morphogenesis of these structures have been widely studied. The complexity of the structures and tissues implied in the development of the girdles make quantitative approaches extremely difficult. The use of GIS technology provides a visual interpretation of the histological data, a general quantitative assessment of the processes taking place during the ontogeny of any structure, and would allow collecting information about the changes in the surface occupied by the different tissues across the ontogenetic processes of any vertebrate taxa. GIS technology applied to map morphological structures would be a main contribution to the construction of the vertebrate ontologies, as it would facilitate the identification and location of the structures. GIS technology would allow also us to construct a shared database of histological quantitative changes across the ontogeny in any vertebrate. The main objective of this study is to use GIS technology for spatial analysis of histological samples such as these of the pelvic girdle using histological cuts of anurans and chicken, allowing thus to construct a morphoscape, analogous to a landscape. This is the first attempt to apply GIS tools to ontogenetic series to infer biological properties of the spatial analysis in the context of comparative biology. More frequent use of this technology would contribute to obtaining more profitable and biologically informative results.
Introduction
Geographic Information System (GIS) is a system that captures, stores, manipulates, analyzes, manages, and presents spatial or geographical data (Madurika and Hemakumara, 2017). This technology is used to compare layers of different types of data connected by locations in geographic space (Ungar and Williamson, 2000). Many tools of GIS are designed to model the physical surface of the earth (Zuccotti et al., 1998). As this technological environment has been created to deal with space problems, it is perfectly adaptable to solve these type of issues in the context of vertebrate comparative morphology. GIS software has been explored in the context of analyzing topography of the dentition (Zuccotti et al., 1998; Jernvall and Selänne, 1999; Ungar and Williamson, 2000; M’Kirera and Ungar, 2003; Ungar, 2004, 2007; Evans et al., 2007; Plyusnin et al., 2008; Eronen et al., 2009; Wilson et al., 2012; Salazar-Ciudad and Marin-Riera, 2013), spatial analysis of histological traits in human bone (Rose et al., 2012), conodont morphologies (Manship, 2004; Manship et al., 2006; Yacobucci and Manship, 2011; Knauss, 2012, 2013), skeletal structures of echinoderms (Sheffield et al., 2012; Zachos, 2012, 2015), and for morphometric analysis of the ammonoid shells (Knauss and Yacobacci, 2014). Additionally, GIS software is used to contribute to interoperability and data reuse (Fonseca and Egenhofer, 1999; Fonseca and Sheth, 2002), biological data mining (Plyusnin et al., 2008), and additionally could be used in developing terminological standardizations for the anatomy of vertebrates, such as the anatomical ontologies proposed for anurans (Maglia et al., 2007) or any other vertebrate. Techniques of GIS could be also used to find a tissue, helping to find out what exists at a particular location. This location can be described in many ways, using, for example, name or color of a tissue, or even topographic references relative to the area surveyed.
The pectoral and pelvic girdles are key structures that relate the axial skeleton with the limbs in tetrapods. Owed to their importance in locomotion, the anatomy, development and morphogenesis of these structures have been widely studied (Borkhvardt, 1991; Kaplan, 2000; Baleeva, 2001, 2009; Malashichev, 2001; Borkhvardt and Baleeva, 2002; Malashichev et al., 2005, 2008; Ročková and Roček, 2005; Shearman, 2005, 2008; Manzano and Barg, 2005; Pomikal and Streicher, 2010; Robovská-Havelková, 2010; Pomikal et al., 2011; Valasek et al., 2011; Don et al., 2013; Manzano et al., 2013; Nowlan and Sharpe, 2014; Nagashima et al., 2016; Soliz et al., 2018 among many others) mainly from a qualitative perspective. The complexity of the structures and tissues implied in the development of the girdles made quantitatives approximations very limited (but see Roddy et al., 2009; Pomikal and Streicher, 2010; Pomikal et al., 2011).
The main objective of this study is to use GIS technology for spatial analysis of histological samples, taking as examples the pelvic girdle of anurans and chicken, allowing to construct a morphoscape analogous to a landscape. Analyzing and representing pelvic girdle ontogeny with GIS tools provides a frame of reference for normal development and can be used for a visual interpretation of the data, and in a quantification of the growth of any structures or tissues of the vertebrate body. We used these unrelated taxa as examples of the possibilities of this approach, and are sure that more frequent use of this technology would contribute to obtaining more profitable and biologically informative results.
Materials and Methods
Histology
Four specimens of Lysapsus limellum FML 28180 (Fundación Miguel Lillo, Tucumán, Argentina. Stages 37, 40, 44, 46 of Gosner, 1960) and three specimens of Boana riojana FML (Stages 37, 44, 46 of Gosner, 1960) were used in this study. These species were selected because previous work on the girdles by our team (Soliz et al., 2018) provided the necessary histological samples in the required sections. We also use images of four histological cuts of a developing chicken pelvic girdle, Stages 30–35 of Hamburger and Hamilton (1951) from Nowlan and Sharpe (2014). Images of the chicken histological cuts were nicely made available by Dr. Niamh Nowlan (Imperial College, London). We refer to our two species as Anuran Model because differences between them are minor in comparison with those of the Anuran and Chicken Models.
Specimens were dehydrated through a graded ethanol series, cleared in xylene and embedded in Paraplast. Embedded specimens were sectioned in serial and semi-serial sections of 5–10 μm. Sections were then deparaffinized, hydrated, and stained with Hematoxylin–Eosin (H-E). All sections were once again dehydrated, bathed in xylene, and sealed with Canada Balsam under a cover slip. Terminology of girdles tissue and structures follows Baleeva (2001); Shearman (2008), Diogo and Abdala (2010); Manzano et al. (2013), Diogo and Molnar (2014); Soliz et al. (2018).
GIS Application
As a way to facilitate the application of this technology, we include a tutorial (Supplementary Material). From more than 100 cuts, a colored section of the same structures from each of the two anuran species, in all four different developmental stages were selected, photographed and digitized, at the same magnification. The original cross-sectional cuts composite image and the SMA.jpg image were imported into a single map in QGIS. To calibrate the measures and enable the accurate use of measuring tools, we built our own reference system. We assign milimeters as the unit of measure. The cuts were scaled 200 μm. In all images the scale was respected. The 200 μm segment of the original cut was rescaled into 0.2 mm. QGIS was used to refer all images to a single reference system. To this end, we used the transverse Mercator reference system. After that, we identified the plane of bilateral symmetry or the sagittal plane, and defined a work area of 1.60 × 0.8 mm, which represents 100% of our area of analysis (Figure 1). After several trials, we found that this size of area allowed us to comfortably follow the anatomical structures through the different ontogenetic stages. New map layers were created in QGIS to represent four cartilaginous structures for the anuran species: pelvic hemigirdle, femur epiphysis and diaphysis, and the lateral articular cartilage; six muscles: ischiotrochantericus D, gracilis major, puboschiofemoralis externus B, pubotibialis A, puboischiofemoralis externus A, puboischiofemoralis internus A-extensor iliotibialis A complex, and two tendons: tendon of the puboschiofemoralis externus B and pubotibialis A. We also identify the connective tissue area that separates both hemigirdles. In regard to the chicken, we identify four cartilaginous structures: ischium, ilium, femur epiphysis and diaphysis; a cavity filled with mesenchimatic tissue (Figure 3). We also identify cell nuclei, the matrix of cartilaginous tissue, dense and lax mesenchimatic tissue and empty areas, which in the Stages 34 and 35 included the acetabular joint cavity. Unsupervised digital classifications of 10× images of the chicken cuts (Nowlan and Sharpe, 2014), another commonly used GIS technique to mark areas, were used to identify the tissues, a procedure commonly used with satellite images. A digital classification of the jpg images of the histological cuts of Gallus gallus was made. Image classification refers to the task of extracting information classes from a multiband raster image, in this case three bands (RGB). The raster resulting from the image classification can be used to create thematic maps. In this case, an almost non-supervised classification (iso clusters) was made. Unsupervised classification searches for spectral classes (or clusters) in a multiband image without the analyst’s intervention. Ten initial classes were defined and from them were recovered those that responded similarly in their RGB combinations (which in GIS would correspond to spectral similarity). Finally, five different types of structures were identified: core, cartilaginous matrix, dense mesenchyme, lax mensenchyme and cavity.
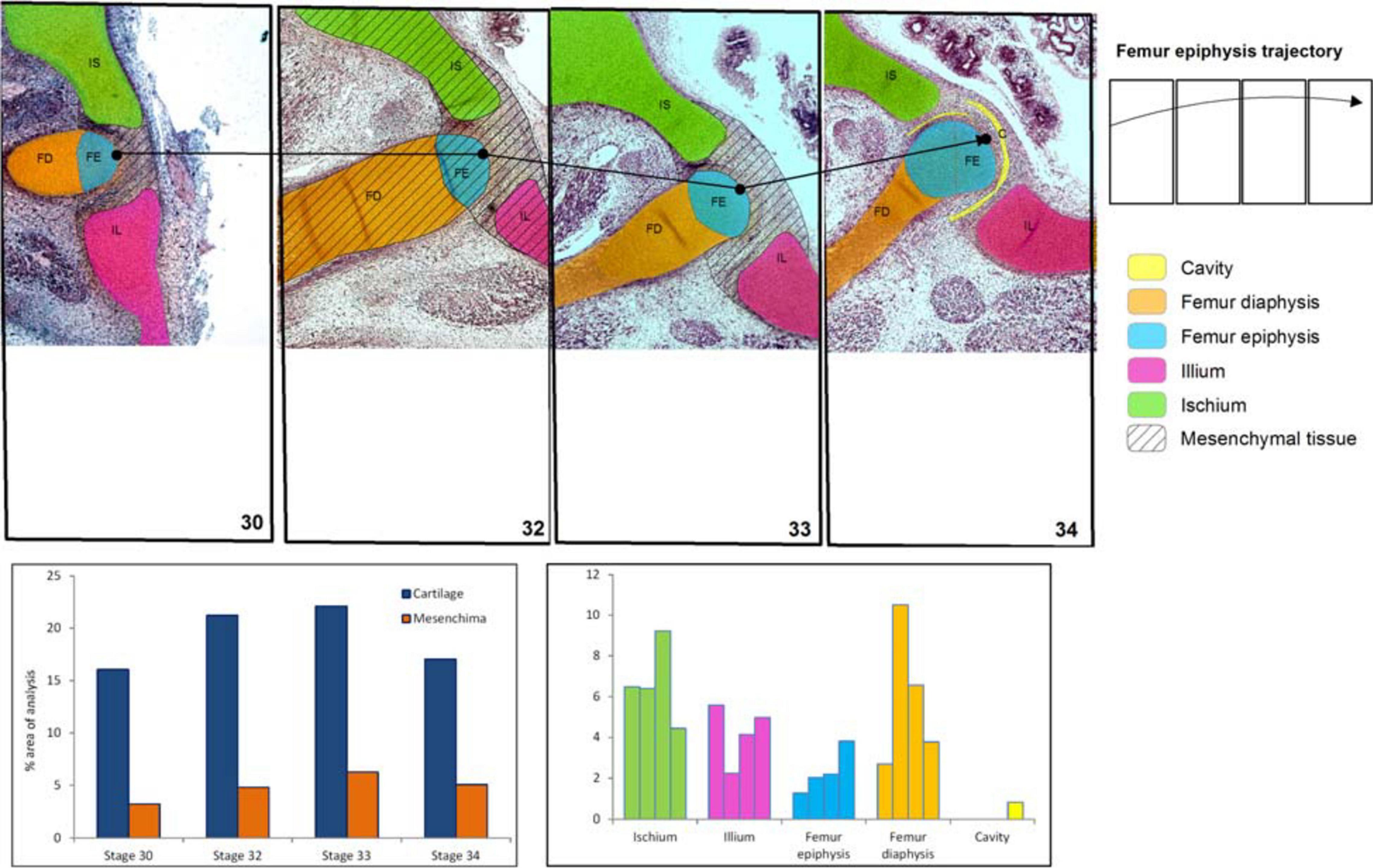
Figure 3. Chicken histological cuts between Stages 30 and 34 HH (Nowlan and Sharpe, 2014) showing the increase in size and change of location of the pelvic girdle tissues and structures. In the frequency histograms the growth of the structures is depicted.
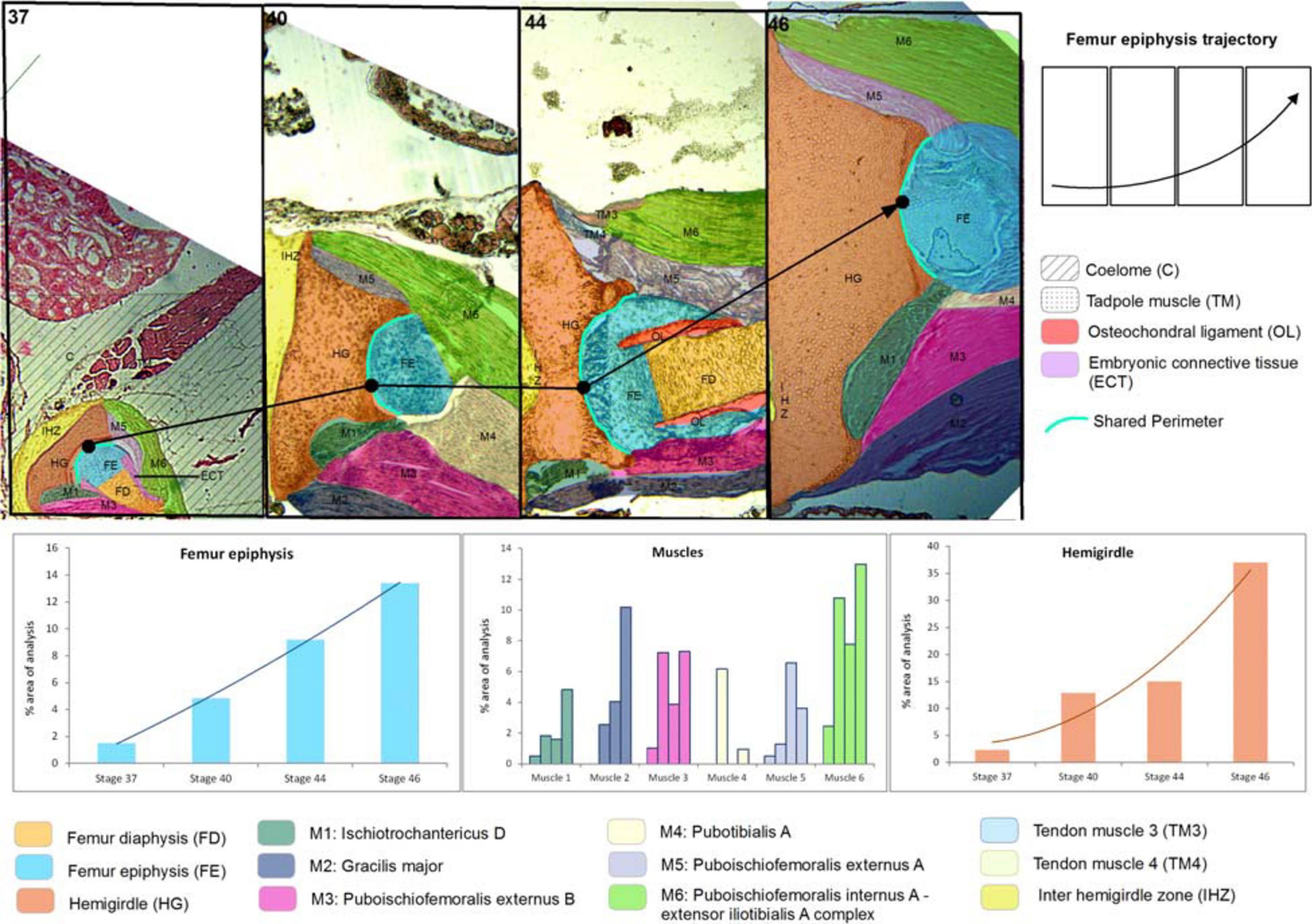
Figure 1. Lisapsus limellum. Selected area and the ontogenetic Stages 37–46 showing the increase in size and change of location of pelvic girdle tissues and structures. In the frequency histograms the growth of the structures is depicted.
These structures and tissues were manually identified and annotated (Figures 1–3), and their scores were recorded in the attribute table for each layer (Figures 1, 3). The attribute table relates any type of data, such as tissue types, and different structures that have been spatially located in the GIS. QGIS was used to create a separate layer containing the images of the histological sections for separate viewing. This was accomplished with the geographical data-base by creating an edited histodatabase. A histodatabase is a data model constructed in standard database formats, allowing for storage of a variety of different layer types within GIS environment. A data repository with all original jpg images, images with reference system, results of digital classifications in raster format and shapefiles used for this work (in a QGIS Project) will be available in a web repository1. After preparation of the appropriate layers, several tools within QGIS were used to identify distributional patterns of histological features. Thus, we obtained, the growth percentages of selected structures and tissues through the ontogenetic stages. We also identify the middle point of the femur epiphysis and follow it in the cuts of all stages of anuran and chicken development, which allowed us to build a trajectory for easy visual recognition of this bone movement thoroughly during development (Figures 1, 3). Additionally, we calculated the shared perimeter between the anatomical structures (Supplementary Material).
Results
Anuran Model
Sequence of the Ontogenetic Stages of the Anuran Pelvic Girdles Structures and Tissues
Lisapsus limellum and Boana riojana
At Stage 37, both species Lisapsus limellum, Figure 1 and Table 1; Boana riojana, Figure 2 and Table 2) present the hemigirdle as a single pre-cartilaginous piece, almost rectangular and at an angle of around 50° with respect to the sagittal plane. This structure occupies 2.4% of the analyzed structures, which are 11.5% of the delimited area in Lisapsus limellum. In Boana riojana the hemigirdle occupies 9.9% of the analyzed structures, which are 61.1% of the delimited area. The space between the sagittal plane is occupied by a loose connective tissue, with abundant ground substance and relatively few fibers and cells, filling the inter hemigirdle zone, 2% of the analyzed area in L. limellum. In B. riojana the central loose connective tissues occupies 23.8% and the typical connective tissue occupies 0.5%, and a part of the tadpole coelome. The acetabular fossa is already observable, housing the pre-cartilaginous femoral head, which occupies the 1.2% of the analyzed area in L. limellum and 7.1% in B. riojana. The femur diaphysis is also observable occupying 1.2% of the analyzed area in L. limellum and 6.9% in B. riojana. Four premyogenic masses corresponding to the ischiotrochantericus D, puboschiofemoralis externus B, puboischiofemoralis externus A, and puboischiofemoralis internus A are visible. All these premuscle or muscle masses occupied the 4.5% of the analyzed area in L. limellum and 12.9% in B. riojana. Considering tissues instead of structures, it could be seen that the cartilaginous tissue occupies 4.7% of the analyzed area, almost the same percentage as the premyogenic masses in L. limellum (4.5%), whereas in B. riojana, cartilage corresponds to 23.9% of the analyzed area and the premyogenic masses to 12.9%. The central loose connective tissue occupies 2% of the analyzed area, whereas the typical connective tissue of the femur epiphysis 0.3% in L. limellum.
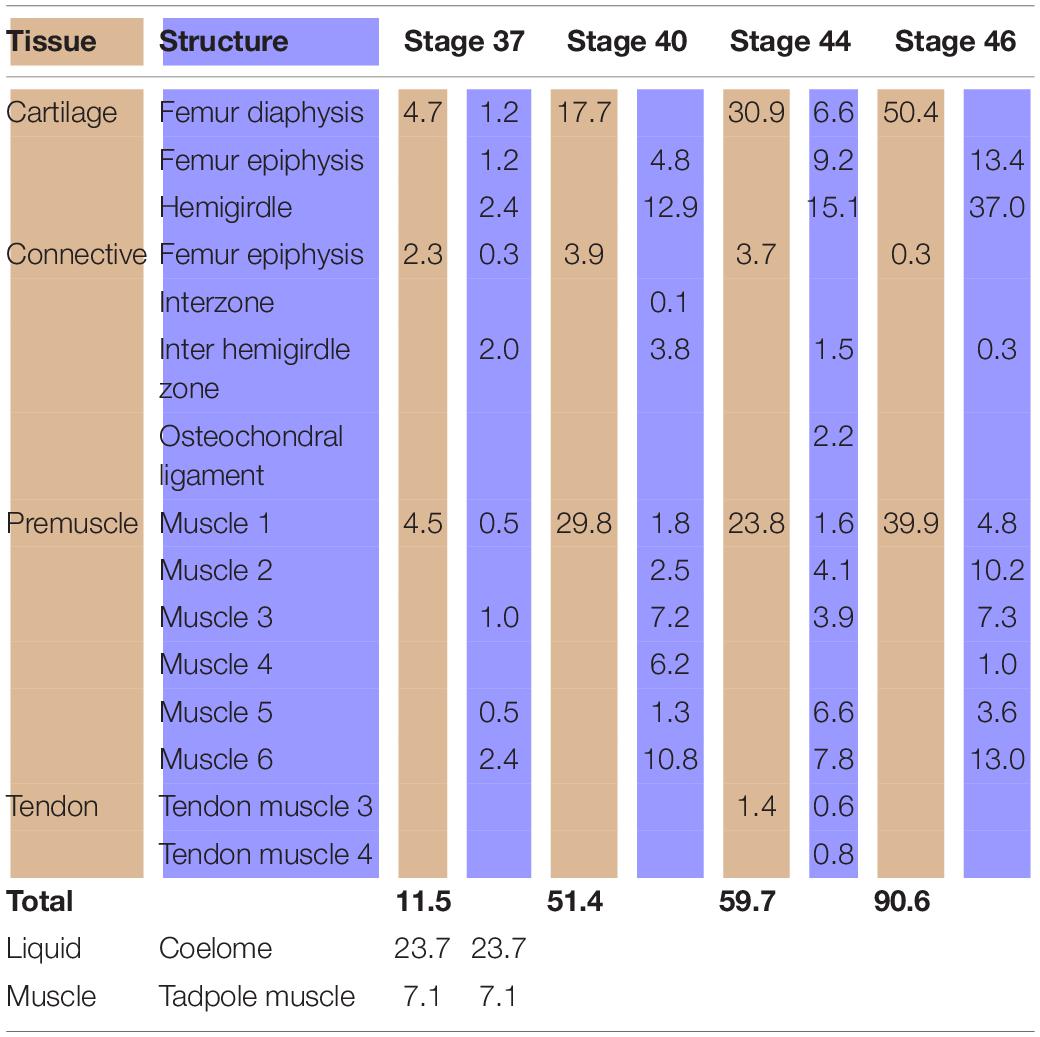
Table 1. Tissues (light brown) and structures (light blue) percentages (%) in relation to the analyzed area (Lisapsus limellum).
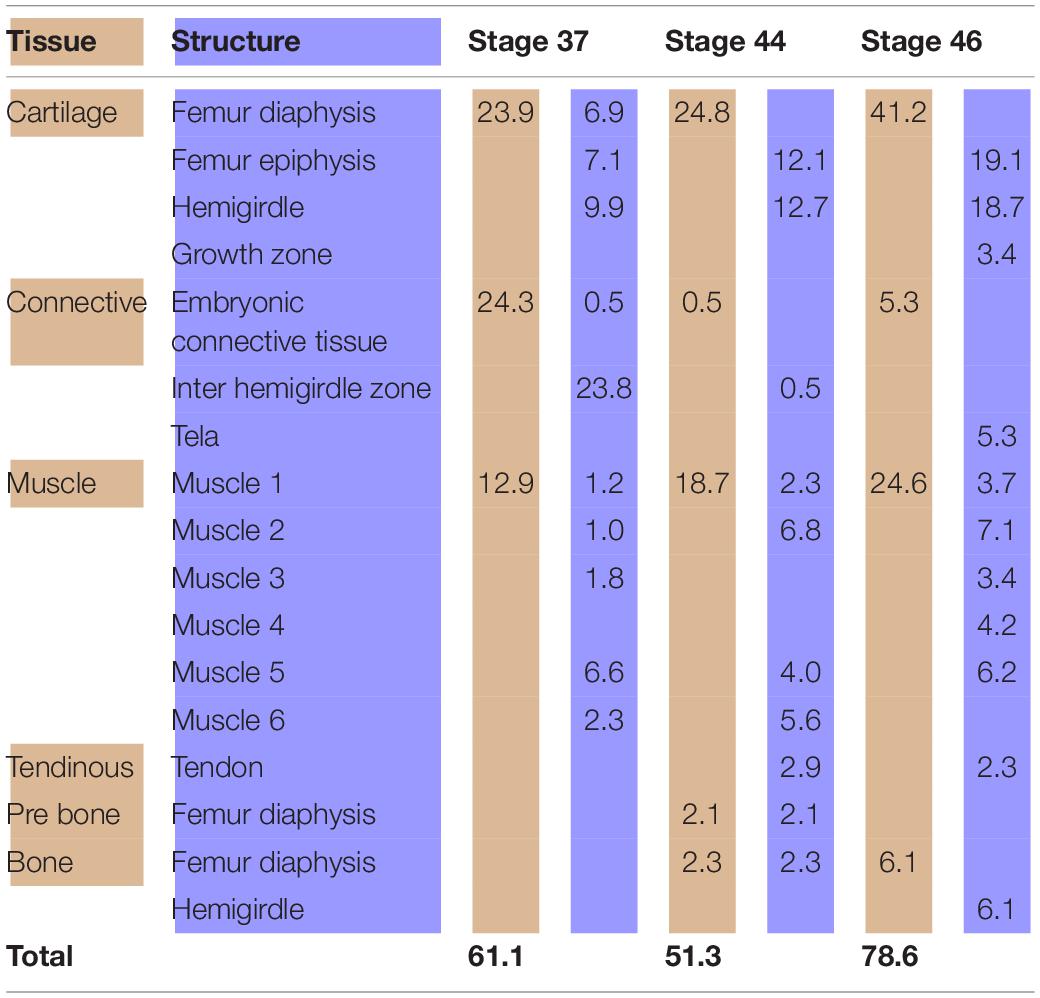
Table 2. Tissues (light brown) and structures (light blue) percentages (%) in relation to the analyzed area (Boana riojana).
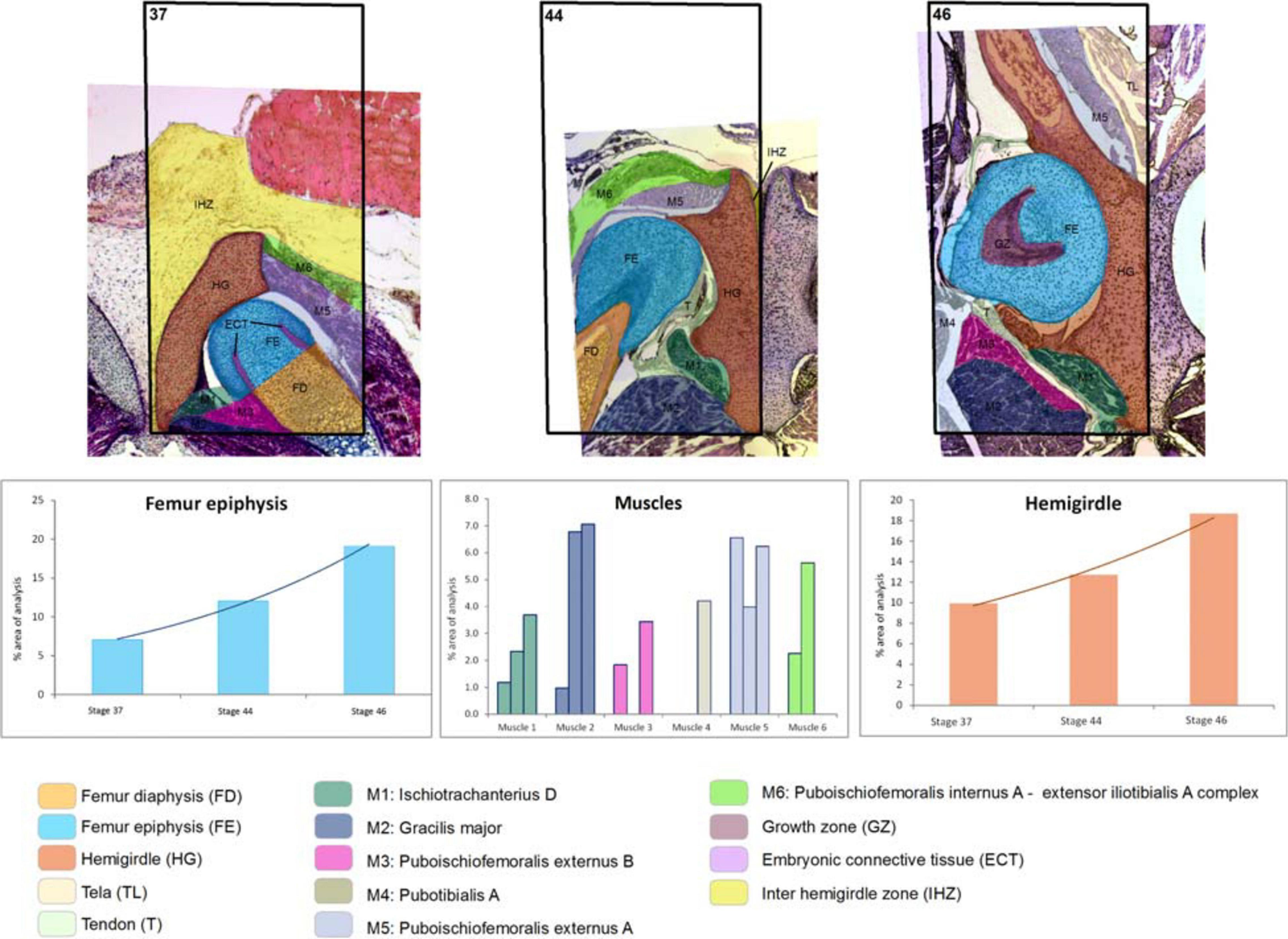
Figure 2. Boana riojana. Selected area and the ontogenetic Stages 37–44–46 showing the increase in size and change of location of the pelvic girdle tissues and structures. Note the embryonic connective tissue restricted to the future osteochondral ligament. In the frequency histograms the growth of the structures is depicted.
In Lisapsus limellum at stage 40 the pre-cartilaginous structures have increased their size. The entire hemigirdle-femur framework is closer to the sagittal plane at an angle of around 30°. The hemigirdle occupies 12.9% of the analyzed area and the femur epiphysis 4.8%. The femur diaphysis is indistinguishable. The space between the hemigirdle and the sagittal plane is entirely occupied by a loose connective tissue (3.8% of the analyzed area). The premyogenic masses also increased their size to occupy 29.8% of the analyzed area. The displacement of the hemigirdle-femur framework allows distinguishing the future gracilis major along with the other five premuscle masses.
Results on the shared perimeters between the structures analyzed in this paper are included in the Supplementary Table 1. The shared perimeter between the femur epiphysis and the hemigirdle is shown in Figure 1.
Lisapsus limellum and Boana riojana Comparison
At Stage 44 the hemigirdle is parallel to the sagittal plane and attached to the other hemigirdle along almost all the medial surface. In Lisapsus limellum the hemigirdle occupies 15.1% of the analyzed area, and in Boana riojana 12.7%. In the center of the attachment region between both hemigirdles is an oval open region filled with the loose connective tissue that is reduced to 1.5% of the analyzed area in Lisapsus limellum. In Boana riojana this connective tissue occupies a long area between the hemigirdles that corresponds to 0.5% of the analyzed area. In the femur epiphysis (9.2%) the lateral articular cartilages are clearly visible, along with the osteochondral ligament (2.2%) in L. limellum. In B. riojana, the femur epiphysis occupies 12.1% of the analyzed area. The osteochondral ligament is not visible in this histological cut. The femur diaphysis is clearly recognizable (6.6%) in L. limellum. In B. riojana it is ossified and occupies 2.3% of the analyzed area. In L. limellum, at Stage 44, the muscles have lengthened compared to the Stage 40, but their covered a smaller area (23.8% vs. 29.8 in Stage 40). In B. riojana, at Stage 44, the muscles have also lengthened but with a greater area than in the Stage 37 (18.7%). In B. riojana tendons are visible at Stage 44, occupying 2.9% of the analyzed area. At Stage 46 both hemigirdles (37%) are completely attached, leaving just vestiges of the loose connective tissue (0.3%) in Lisapsus limellum. In Boana riojana, the hemigirdle is composed by a cartilaginous part (18.7%) and an ossified part (6.1%), so the total hemigirdle occupies 24.8%. The femur epiphysis (13.4% in L. limellum; 19.1% in B. riojana) is totally adjusted to the acetabulum. The femur diaphysis is indistinguishable in L. limellum, and Boana riojana. All muscular masses are composed of mature tissue and occupy 39.9% of the analyzed area in L. limellum and 24.6% in B. riojana. The cartilaginous tissue occupies 50.4% of the analyzed area in L. limellum and 41.2% in B. riojana. In B. riojana the tendons occupy 2.3% of the analyzed area.
Both hemigirdles are moving to the sagittal plane of the body. Growth of cartilaginous structures tend to be proportional through all stages, with the hemigirdle and femur epiphysis being the largest at Stage 46. This pattern of proportional growth is not so obvious in relation to the muscles in L. limellum, which exhibit more variability; in B. riojana the growth of the muscles is as proportional as the cartilaginous structures. In both cases a higher overall growth between the Stages 44 and 46 is noticeable. Tendinous tissue appears late in the ontogeny and remains comparatively small.
Chicken Model
Sequence of the Ontogenetic Stages of the Chicken Pelvic Girdles Tissues and Structures
In the chicken model (Figure 3), at Stage 30, the hemigirdle is composed of two pieces, the ischium and the ilium, separated by mesenchimatic tissue. The hemigirdle is located almost paralell to the sagital plane of the body at Stage 30, and rotates to reach almost 55° at Stage 32. Both hemigirdles are moving away from the sagittal plane of the body. The femur epiphysis and diaphysis are distinguishable. Percentages of the different structures and tissues in relation to the analyzed area are shown in Table 3. Growth of the cartilaginous structures tends to increase to the last stages, with some inconsistencies probably owed to the depth of the histological cuts. The mesenchime of the cavity surrounding the femur epiphysis tend to grow up to the Stage 33 (6.3%), and then shows a soft decrease in Stage 34 (5.1%) owed to the increase of the area occupied by the femur epiphysis (from 1.3% in Stage 30–3.8 in Stage 34).
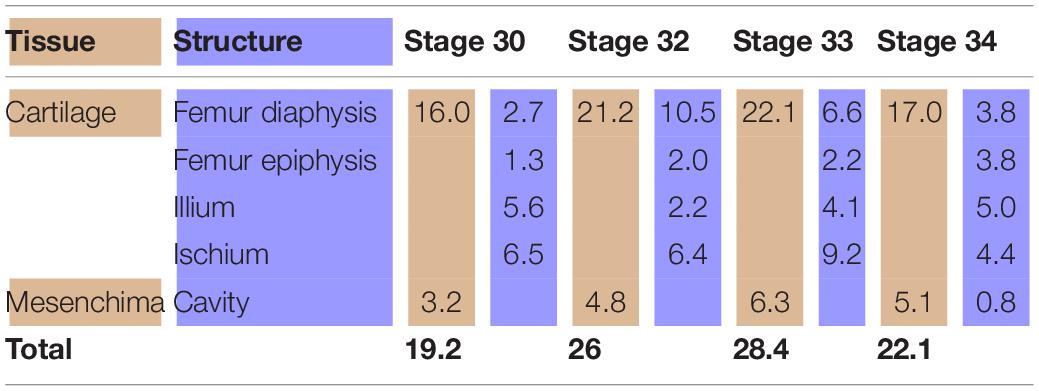
Table 3. Tissues (light brown) and structures (light blue) percentages (%) in relation to the analyzed area (Gallus gallus).
Discussion
Our results show that GIS technology is an effective interface to study spatial problems even to microscopic scales. However, as far as we know, apart from ours there has been only one attempt to apply GIS environment to ontogenetic series to infer biological properties of the spatial analysis in the context of comparative biology (see Zachos, 2015). The application of this technology exhibits some shortcomings more related to the difficulties of obtaining appropriate histological cuts than to the technology itself. Thus, we think that the small area of the tendinous tissue in our results and the decrease of the area occupied by some muscles in later stages compared to earlier ones, can be artifacts owed to the lack of cuts at the same deep. The comparison of samples coming from different species or stages of different sizes would be also a problem. However, in view of analytical benefits the effort to achieve standard samples is worth to carry out. Whenever the focus is put on the analysis of shapes, structures of different sizes can be accommodated within a window of standard size (for instance, the square unit). In spite of these limitations, GIS tools are a powerful technology that could allow discovery of interesting trends in the growth of tissues and structures along all the ontogenetic periods of any living organism.
The use of GIS imaging has facilitated the quantification of several features of pelvic girdle development that could not be possible using whole-mount data and be very difficult with histological data. The major advantage in using GIS is the fast visual perspective of the tissues and structures, as well as the option of a quick collection and catalog of histological and structural data. The possibility to set the same reference area for all specimens allowed us to highlight differences in the trajectories of the hemigirdles between the studied species. Our data clearly demonstrate that in frogs, rotation of the pelvis with respect to the sagittal body plane makes both closer to each other (see also Pomikal et al., 2011), whereas the opposite happens in the chicken (see also Nowlan and Sharpe, 2014). Data on the rotation angles of the hemigirdles in the chicken show that they move away from the sagittal plane of the body. At earlier stages, the angle between the hemigirdle and the sagittal plane of the body is very small, and grows to about 55° in only one stage. In the chicken all the structures occupy only half of the area compared to frogs, and changes in the location of the femur epiphyses are more gradual. The location of the femur epiphyses change more acutely in frogs, from the lower quadrant to the superior one. The more radical growth of the girdle structures and the change in the locations of the femur epiphyses in frogs could be easily related to the dramatic change from an aquatic to a terrestrial habitat, which requires fully functional limbs (Muntz, 1975; Pomikal et al., 2011). It could be also inferred that the opposite direction of the rotation of the femur and girdles in the chicken can be owed to the bipedal position of the hindlimb in birds that requires a more vertical location of this bone. We are aware that these data are visible with histological cuts, however, the use of GIS mapping highlight trends that remain hidden in the complex landscape of serial histological sampling.
Analyses of the anuran pelvic girdle growth during development using GIS tools reveal interesting patterns worth considering. At Stage 37 around 60% of the analyzed area of Lisapsus limellum and 40% of B. riojana are occupied by tissues and structures of the tadpole. The connective tissue that fills the inter hemigirdles zones is more extensive in Boana riojana at Stage 37, 23.9% of the studied area, which corresponds to 61.1% of the total area. At the same stage, in Lisapsus limellum it occupies 2.3%, which corresponds to a 11.5% of the studied area. Remarkably, at Stage 37, in both species the connective tissue of the inter hemigirdle zone is almost the same percentage of the connective tissue in general, 2 of 2.3% in L. limellum (0.3%) and 23.8 of 24.3% in B. riojana (0.5%). Pomikal et al. (2011) reported a highly detailed description of the ilio-sacral connector, a strand of connective tissue linking the ilium and the sacrum in several anuran species. It could be inferred that the big area of connective tissue between the hemigirdles at the earlier stages (IHZ in our Figures 1, 2), which persists as a small region at Stage 46 in Lisapsus limellum and has practically disappeared at Stage 44 in Boana riojana, could also be a hemigirdle connector. As can be observed in the figures, the hemigirdle-connector tissue appears highly different to the surrounding tissues, and-as was demonstrated in Rana temporaria by Pomikal et al. (2011)—growth of the cartilaginous structures is performed at the expense of the connective strand. It would be very interesting to analyze its composition and compare it with the ilio-sacral connector and to the mesenchymal bridge described by Malashichev (2001) that connects the lizard ribs.
The fastest growing of all cartilaginous structures in the anuran model occurs between Stages 44 and 46. It is remarkable and similar to what occurs with muscles. This outcome is surprising considering the sequence proposed by Muntz (1975) in which tadpoles of Stage 42 should have fully functional limbs with their tissues fully differentiated, suggesting that the faster growth should occur to reach that stage. The growth between Stages 44 and 46 of most structures is faster indeed, passing in, e.g., the femur epiphysis of L. limellum from 9.2 to 13.4% and in B. riojana from 12.1 to 19.1% of the total area considered. This fast growth suggests that although functional, tissues and structures in Stage 42 have to reach their most adjusted phenotype to perform the physical activities of the organism.
The images of the chicken development show the gradual change in the femur orientation, which acquires a more vertical position. It is interesting that a previous study of Carrano and Biewener (1999) has showed that an experimental change in the location of the center of mass of a chicken by adding postacetabular weigths, provoked a more horizontal femur orientation. The change in the orientation of the femur through chicken development suggests that the center of mass of the embryo is shifting to a more anterior position. On the contrary, the orientation of the femur in Lisapsus limellum is in the opposite sense, probably indicating a shift of the center of mass to a posterior location. These changes are more clearly perceived and showed because of the GIS technology used here.
The shared perimeter between the hemigirdle and the femur epiphysis of Lisapsus limellum increases progressively until Stage 44, being shorter at Stage 46. As Figure 1 shows, this shortening could be an artifact, as in the image, muscles cover the terminal prominences of the hemigirdle. The Supplementary Table 1 shows that the longest perimeter of the embryonic tissue present at Stage 37 is shared by the diaphysis of the femur. It could be predicted that this embryonic tissue will differentiate into diaphysis tissue. This type of data will help to uncover other growth patterns of the tissued through the ontogeny.
In this paper we showed that GIS software can be used to document, describe, and compare a variety of different structures and tissues through histological mapping. We thus make a histomorphoscape, in this case applied to girdles development. This method allows the consideration of a new scale at which to evaluate the variability of the histomorphology of a structure (Rose et al., 2012). GIS technology would allow also to construct a shared database of histological quantitative changes across the ontogeny in any vertebrate. These data would be more efficiently recovered and useful for comparisons at a higher scale than with the tools available until now. In this way, any researcher from the field of comparative morphology could achieve a snapshot of possible analyzes involving shapes, measurement of features, and topographic relationships among structures. GIS provides tools that allow a fresh and dynamic new way (Table 4). For example, the function of interpolation could assist in dealing with non-complete samples. It sheds light on the underlying nature of structures otherwise absent, which is intrinsic to many GIS software, but not easily available in other resources from digital image analysis. Sweat streams drained by a rough skin could be analyzed with the same rationale as in a continental watershed, presenting an unprecedented way to study a natural phenomenon.
Data Availability Statement
Publicly available datasets were analyzed in this study. This data can be found here: https://ibn.conicet.gov.ar/recursos/.
Ethics Statement
Ethical review and approval was not required for the animal study because we worked with histological cuts from a collection.
Author Contributions
VA conceived this study and realized drafting of the manuscript. LC applied GIS technology. MS provided the anuran cuts. VA, LC, and DD interpreted the data. All authors critically revised the manuscript.
Funding
PIP CONICET 0389 to VA; PICT 2016-2772 – PICT 2018-00832 to VA.
Conflict of Interest
The authors declare that the research was conducted in the absence of any commercial or financial relationships that could be construed as a potential conflict of interest.
Acknowledgments
We are very grateful to Niamh Nowlan (Department of Bioengineering, Imperial College London) for making the chicken pictures available.
Supplementary Material
The Supplementary Material for this article can be found online at: https://www.frontiersin.org/articles/10.3389/fevo.2021.642255/full#supplementary-material
Footnotes
References
Baleeva, N. (2001). Formation of the scapular part of the pectoral girdle in anuran larvae. Russian J. Herpetol. 8, 195–204.
Baleeva, N. (2009). Formation of the coracoid region of the anuran pectoral girdle. Russ. J. Herpetol. 16, 41–50.
Borkhvardt, V., and Baleeva, N. (2002). Development of the pectoral girdle in larvae of Siberian salamander Salamandrella keyserlingii (Amphibia. Hynobiidae). Russ. J. Herpetol. 9, 177–184.
Borkhvardt, V. G. (1991). Regularities of the development of the cartilaginous elements in vertebrate ontogenesis. Zh. Obshch. Biol. 52, 627–640.
Carrano, M. T., and Biewener, A. A. (1999). Experimental alteration of limb posture in the chicken (Gallus gallus) and its bearing on the use of birds as analogs for dinosaur locomotion. J. Morphol. 240, 237–249. doi: 10.1002/(sici)1097-4687(199906)240:3<237::aid-jmor3>3.0.co;2-n
Diogo, R., and Molnar, J. (2014). Comparative anatomy, evolution, and homologies of tetrapod hindlimb muscles, comparison with forelimb muscles, and deconstruction of the forelimb-hindlimb serial homology hypothesis. Anat. Rec. 297, 1047–1075. doi: 10.1002/ar.22919
Don, E. K., Currie, P. D., and Cole, N. J. (2013). The evolutionary history of the development of the pelvic fin/hindlimb. J. Anat. 222, 114–133. doi: 10.1111/j.1469-7580.2012.01557.x
Eronen, J. T., Evans, A. R., Fortelius, M., and Jernvall, J. (2009). The impact of regional climate on the evolution of mammals: a case study using fossil horses. Evolution 64, 398–408. doi: 10.1111/j.1558-5646.2009.00830.x
Evans, A. R., Wilson, G. P., Fortelius, M., and Jernvall, J. (2007). High-level similarity of dentitions in carnivorans and rodents. Nature 445, 78–81. doi: 10.1038/nature05433
Fonseca, F., and Egenhofer, M. (1999). “Ontology-driven geographic information systems,” in GIS ‘99: Proceedings of the 7th ACM International Symposium on Geographic Information Systems, ed. C. B. Medeiros (New York, NY: Association for Computing Machinery), 14–19.
Fonseca, F., and Sheth, A. (2002). The Geospatial Semantic Web. Available online at: https://corescholar.libraries.wright.edu/knoesis/679
Gosner, K. L. (1960). A simplified table for staging anuran embryos and larvae with notes on identification. Herpetologica 16, 183–190.
Hamburger, V., and Hamilton, H. L. (1951). A series of normal stages in the development of the chick embryo. J. Morphol. 88, 49–92. doi: 10.1002/jmor.1050880104
Jernvall, J., and Selänne, L. (1999). Laser confocal microscopy and geographic information systems in the study of dental morphology. Paleontol. Electronica 2:18.
Kaplan, M. (2000). The pectoral girdles of Rana rugulosa (Ranidae) and Nesomantis thomasseti (Sooglossidae). Herpetologica 56, 188–195.
Knauss, M. J. (2012). Quantifying morphological variability in ammonoids using GIS spatial analyses. Geol. Soc. Am. 44:442.
Knauss, M. J. (2013). Quantifying ornamental variabilityin an ammonoid clade using GIS spatial analyses. Geol. Soc. Am. 45:475.
Knauss, M. J., and Yacobacci, M. M. (2014). Geographic information systems technology as a morphometric tool for quantifying morphological variation in an ammonoid clade. Palaeontol. Electron. 17:19A.
Madurika, H. K. G. M., and Hemakumara, G. P. T. S. (2017). Gis based analysis for suitability location finding in the residential development areas of Greater Matara Region. Int. J. Sci. Technol. Res. 4, 96–105.
Maglia, A. M., Leopold, J. L., Pugener, A., and Gauch, S. (2007). An anatomical ontology for amphibians. Pac. Symp. Biocomput. 12, 367–378.
Malashichev, Y. (2001). Sacrum and pelvic girdle development in lacertidae. Russ. J. Herpetol. 8, 1–16.
Malashichev, Y., Borkhvardt, V., Christ, B., and Scaal, M. (2005). Differential regulation of avian pelvic girdle development by the limb field ectoderm. Anat .Embryol. 210, 187–197. doi: 10.1007/s00429-005-0014-8
Malashichev, Y., Christ, B., and Pröl, F. (2008). Avian pelvis originates from lateral plate mesoderm and its development requires signals from both ectoderm and paraxial mesoderm. Cell. Tissue Res. 331, 595–604. doi: 10.1007/s00441-007-0556-6
Manship, L., Strauss, R. E., and Barrick, J. (2006). “Discrimination of frasnian (Late Devonian) Palmatolepis species using multivariate analysis of platform elements,” in Proceedings of the Programme & Abstracts, First International Conodont Symposium 2006 (ICOS 2006), Leicester, 55.
Manship, L. L. (2004). Pattern Matching: Classification of Ammonitic Sutures Using GIS. Lubbock, TX: Department of Geosciences.
Manzano, A., Abdala, V., Ponssa, M. L., and Soliz, M. C. (2013). Ontogeny and tissue differentiation of the pelvic girdle and hind limbs of anurans. Acta Zool. 94, 420–436.
Manzano, A., and Barg, M. (2005). The iliosacral articulation in Pseudinae (Anura. Hylidae). Herpetologica 61, 259–267. doi: 10.1655/04-28.1
M’Kirera, F., and Ungar, P. S. (2003). Occlusal relief changes with molar wear in Pan troglodytes troglogytes and Gorilla gorilla gorilla. Amer. J. Primatol. 60, 31–41. doi: 10.1002/ajp.10077
Muntz, L. (1975). Myogenesis in the trunk and leg during development of the tadpole of Xenopus laevis (Daudin 1802). J. Embryol. Exp. Morph. 33, 757–774.
Nagashima, H., Sugahara, F., Watanabe, K., Shibata, M., Chiba, A., and Sato, N. (2016). Developmental origin of the clavicle, and its implications for the evolution of the neck and the paired appendages in vertebrates. J. Anat. 229, 536–548. doi: 10.1111/joa.12502
Nowlan, N. C., and Sharpe, J. (2014). Joint shape morphogenesis precedes cavitation of the developing hip joint. J. Anat. 224, 482–489. doi: 10.1111/joa.12143
Plyusnin, I., Evans, A. R., Karme, A., Gionis, A., and Jernvall, J. (2008). Automated 3D phenotype analysis using data mining. PLoS One 3:e1742. doi: 10.1371/journal.pone.0001742
Pomikal, C., Blumer, R., and Streicher, J. (2011). Four–dimensional analysis of early pelvic girdle development in Rana temporaria. J. Morphol. 272, 287–301. doi: 10.1002/jmor.10913
Pomikal, C., and Streicher, J. (2010). 4D–analysis of early pelvic girdle development in the mouse (Mus musculus). J. Morphol. 271, 116–126. doi: 10.1002/jmor.10785
Robovská-Havelková, P. (2010). How can ontogeny help us to understand the morphology of anuran pectoral girdle? Zoomorphology 129, 121–132. doi: 10.1007/s00435-010-0105-9
Ročková, H., and Roček, Z. (2005). Development of the pelvis and posterior part of the vertebral column in the Anura. J. Anat. 206, 17–35. doi: 10.1111/j.0021-8782.2005.00366.x
Roddy, K. A., Nowlan, N. C., Prendergast, P. J., and Murphy, P. (2009). 3D representation of the developing chick knee joint: a novel approach integrating multiple components. J. Anat. 214, 374–387. doi: 10.1111/j.1469-7580.2008.01040.x
Rose, D. C., Agnew, A., Gocha, T. P., Stout, S. D., and Field, J. S. (2012). Technical note: the use of geographical information systems software for the spatial analysis of bone microstructure. Amer. J. Phys. Anthropol. 148, 648–654. doi: 10.1002/ajpa.22099
Salazar-Ciudad, I., and Marin-Riera, M. (2013). Adaptive dynamics under development-based genotype-phenotype maps. Nature 497, 361–365. doi: 10.1038/nature12142
Shearman, R. (2005). Growth of the pectoral girdle of the leopard frog. Rana pipiens (Anura, Ranidae). J. Morphol. 264, 94–104. doi: 10.1002/jmor.10322
Shearman, R. (2008). Chondrogenesis and ossification of the Lissamphibian pectoral girdle. J. Morphol. 269, 479–495. doi: 10.1002/jmor.10597
Sheffield, S. L., Zachos, L. G., and Lewis, R. D. (2012). A morphometric study of Erisocrinus (Crinoidea) using ArcGIS. Geol. Soc. Am. 44:232.
Soliz, M. C., Ponssa, M. L., and Abdala, V. (2018). Comparative anatomy and development of pectoral and pelvic girdles in hylid anurans. J. Morphol. 279, 904–924. doi: 10.1002/jmor.20820
Ungar, P. (2004). Dental topography and diets of Australopithecus afarensis and early Homo. J. Human Evol. 46, 605–622. doi: 10.1016/j.jhevol.2004.03.004
Ungar, P. (2007). “Dental topograpy and human evolution,” in Dental Perspectives on Human Evolution: State of the Art Research in Dental Paleoanthropology, eds S. E. Bailey and J. J. Hubling (Berlin: Springer Science & Business Media), 411.
Ungar, P., and Williamson, M. (2000). Exploring the effects of tooth wear on functional morphology: a preliminary study using dental topograpic analysis. Palaeontol. Electron. 31:18.
Valasek, P., Theis, S., De Laurier, A., Hinitsf, Y., Lukea, G. L., Ottoa, J., et al. (2011). Cellular and molecular investigations into the development of the pectoral girdle. Devel. Biol. 357, 108–116. doi: 10.1016/j.ydbio.2011.06.031
Wilson, G. P., Evans, A. R., Corfe, I. J., Smits, P. D., Fortelius, M., and Jernvall, J. (2012). Adaptive radiation of multituberculate mammals before the extinction of dinosaurs. Nature 483, 457–460. doi: 10.1038/nature10880
Yacobucci, M. M., and Manship, L. L. (2011). Ammonoid septal formation and suture asymmetry explored with a geographic information systems approach. Palaeontol. Electron. 14:3A.
Zachos, L. G. (2012). Morphometric analysis of fossils using heads-up digitizing and geographic information system (GIS) software. Geol. Soc. Am. 44:18.
Zachos, L. G. (2015). Holistic morphometric analysis of growth of the sand dollar Echinarachnius parma (Echinodermata:Echinoidea:Clypeasteroida). Zootaxa 4052, 151–179. doi: 10.11646/zootaxa.4052.2.1
Keywords: methodological options in anatomy, histology, biological information systems, morphoscape, GIS
Citation: Abdala V, Cristobal L, Solíz MC and Dos Santos DA (2021) Geographical Information System Applied to a Biological System: Pelvic Girdle Ontogeny as a Morphoscape. Front. Ecol. Evol. 9:642255. doi: 10.3389/fevo.2021.642255
Received: 15 December 2020; Accepted: 22 March 2021;
Published: 14 April 2021.
Edited by:
Catherine Anne Boisvert, Curtin University, AustraliaReviewed by:
Rohan Mansuit, UMR 7207 Centre de Recherche sur la Paléobiodiversité et les Paléoenvironnements (CR2P), FranceTerry A. Gates, North Carolina State University, United States
Copyright © 2021 Abdala, Cristobal, Solíz and Dos Santos. This is an open-access article distributed under the terms of the Creative Commons Attribution License (CC BY). The use, distribution or reproduction in other forums is permitted, provided the original author(s) and the copyright owner(s) are credited and that the original publication in this journal is cited, in accordance with accepted academic practice. No use, distribution or reproduction is permitted which does not comply with these terms.
*Correspondence: Virginia Abdala, dmlyZ2luaWFAd2VibWFpbC51bnQuZWR1LmFy; orcid.org/0000-0002-4615-5011