- 1Laboratório de Mastozoologia, Departamento de Zoologia, Instituto de Biociências, Universidade Federal do Estado do Rio de Janeiro, Rio de Janeiro, Brazil
- 2Programa de Pós-graduação em Biodiversidade e Biologia Evolutiva, Centro de Ciências da Saúde, Universidade Federal do Rio de Janeiro, Rio de Janeiro, Brazil
- 3Programa de Pós-graduação em Ciências Biológicas (Biodiversidade Neotropical), Instituto de Biociências, Universidade Federal do Estado do Rio de Janeiro, Rio de Janeiro, Brazil
The South American native ungulates (SANUs) are usually overlooked in Eutherian phylogenetic studies. In the rare studies where they were included, the diversity of SANUs was underrated, keeping their evolutionary history poorly known. Some authors recognized the SANUs as a monophyletic lineage and formally named it Meridiungulata. Here, we recognized and defined a new supraordinal lineage of Eutheria, the Sudamericungulata, after performing morphological phylogenetic analyses including all lineages of SANUs and Eutheria. The SANUs resulted as non-monophyletic; thus, Meridiungulata is not a natural group; Litopterna and “Didolodontidae” are Panameriungulata and closer to Laurasiatheria than to other “Meridiungulata” (Astrapotheria, Notoungulata, Pyrotheria, and Xenungulata). The other “Meridiungulata” is grouped in the Sudamericungulata, as a new monophyletic lineage of Afrotheria Paenungulata, and shared a common ancestor with Hyracoidea. The divergence between the African and South American lineages is estimated to Early Paleocene, and their interrelationships support the Atlantogea biogeographic model. Shortly afterward, the Sudamericungulata explosively diversified in its four lineages. Confronting the Sudamericungulata evolutionary patterns and the Cenozoic natural events (such as tectonics and climatic and environmental changes, among others) helps to unveil a new chapter in the evolution of Gondwanan Eutheria, as well as the natural history of South America during the Cenozoic.
Introduction
Several molecular phylogenies shook up the Eutheria tree in the last decades, dismissing traditional groups recognized by morphological studies, such as Ungulata, Insectivora, and Anagalida (Murphy et al., 2001; Springer et al., 2003; Gheerbrant et al., 2016). Molecular studies mostly include living mammals and classified extant eutherians into three lineages, each with distinct geographical origins: the Boreoeutheria (Northern Hemisphere), the Xenarthra (South America), and the Afrotheria (Africa). Every eutherian lineage spread its geographical boundaries after its origin. Understanding the phylogenetic and biogeographic affinities among these three lineages still challenges scientists, and although their divergence sequence remains unsettled, it possibly occurred rapidly around 120 Mya (Nishihara et al., 2009). All paired combinations among Xenarthra, Boreoeutheria, and Afrotheria were equally and statistically recovered, including Atlantogenata, a Gondwanan clade comprising South American and African lineages.
Although the extinct South American native ungulates (SANUs: Astrapotheria, Didolodontidae, Litopterna, Notoungulata, Pyrotheria, and Xenungulata) received enthusiastic attention from great naturalists in the past (Darwin, 1845; Owen, 1846, 1853; Ameghino, 1889, 1897; Simpson, 1945, 1948), their phylogenetic relationships—among each other and within Eutheria—as well as their evolutionary history remain insufficiently explored.
While Ameghino (1897) and Stromer (1926) suggested evolutionary affinities between Notoungulata (SANUs) and Hyracoidea, Sinclair (1909) dismissed their closer relationships. Later, Simpson (1945) related SANUs lineages to Paenungulata (Pyrotheria) and Protungulata (Notoungulata and Astrapotheria). Other authors pointed that SANUs constitute a monophyletic group, the Meridiungulata (McKenna, 1975; Kramarz et al., 2017). However, phylogenetic and evolutionary studies including SANUs increased in the past decade. Agnolin and Chimento (2011) suggested a closer relationship between afrotherians and SANUs, nonetheless, without formalizing it in phylogenetic analysis. However, subsequent work rebuts some “homologies” suggested by these authors, such as the astragalar morphology (Billet and Martin, 2011) and the delayed dental eruption (Billet and Martin, 2011; Kramarz and Bond, 2014), weakening the idea of a close affinity between afrotherians and SANUs. Surprisingly, one of these criticizers (Billet) pointed out a rare feature of the eutherians promontorium as similar in the extant hyracoid Procavia (Afrotheria) and the notoungulates (SANU lineage; Billet and De Muizon, 2013). In the meantime, the phylogenetic analysis of O’Leary et al. (2013) included Notoungulata, Litopterna, and Xenungulata taxa in an enormous matrix of morphological traits; the SANUs resulted as non-monophyletic, Litopterna was part of Laurasiatheria, while the others nested within paenungulate Afrotheria.
Since SANUs are only known by extinct taxa and more effective techniques of molecular extraction from fossils are very recent (Rizzi et al., 2012), the molecular phylogenetic studies including SANUs appeared just in the last few years. Buckley (2015) and Welker et al. (2015) found that both Macrauchenia patachonica (Litopterna) and Toxodon platensis (Notoungulata) were part of the Perissodactyla on a proteomics-based analysis and Westbury et al. (2017) recovered M. patachonica as sister to Perissodactyla, but on a mitochondrial genome-based study. Later, Chimento and Agnolin (2020) performed a morphological-based cladistic analysis and corroborated molecular proposals that litopterns and their kin (didolodontids and kollpaniids) were “successive stem clades of crown Perissodactyla.” Very recently, MacPhee et al. (2021) performed a morphological-based phylogenetic analysis recovering litopterns closer to perissodactyls than to other SANUs, i.e., a non-monophyletic SANUs, contrasting with the molecular proposals (Buckley, 2015; Welker et al., 2015). Nevertheless, SANU lineages (Astrapotheria, Didolodontidae, Litopterna, Notoungulata, Pyrotheria, and Xenungulata) were never included altogether in a phylogenetic analysis of eutherians, which is imperative to untangle their evolutionary history as well as their relationships within Eutheria.
Materials and Methods
We selected taxa and characters to compose the phylogenetic matrix (Supplementary Material Sections 2, 3) according to the following criteria: (1) strict use of dental and mandibular features, since those are the most abundant and well-preserved fossil elements, avoiding unnecessary missing data; (2) Paleogene taxa represented lineages, because the closer the taxa are to the origin of the lineage, the less might be the occurrence of homoplasies (Simmons and Geisler, 1998); and (3) the most basal taxa from each lineage, due to the same reason pointed out in the last criterion (Simmons and Geisler, 1998). The dental morphology terminology followed Avilla (2006); Gelfo (2007), Kramarz and Bond (2009), and Billet (2011) (Supplementary Material Section 2).
The matrix resulted in 109 characters and 44 terminals (Supplementary Material Sections 2, 3), and Protungulatum donnae Sloan and Van Valen, 1965 composes the outgroup. The ingroup taxa represent most of the higher-level lineages of Eutheria, excluding Xenarthra and Tubulidentata, since their teeth morphology—when teeth are present—is so simplified that the identification of dental crown features is impossible and/or incomparable to other eutherians. The eutherian lineages included in this analysis are as follows: Euarchontoglires—Rodentia (Tribosphenomys minutus Meng, Wyss, Dawson and Zhai, 1994) and Primates (Purgatorius Van Valen and Sloan, 1965); Laurasiatheria—Cetartiodactyla (Diacodexis Cope, 1882) and Perissodactyla (Cambaytherium thewissi Bajpai, Kapur, Das, Tiwari, Saravanan and Sharma, 2005; Hyrachyus Leidy, 1871; Hyopsodus Leidy, 1870; Phenacodus Cope, 1873); Afrotheria (Abdounodus hamdii Gheerbrant and Sudre, 2001 and Ocepeia daouiensis Gheerbrant and Sudre, 2001)—Macroscelidea (Herodotius pattersoni Simons, Holroyd and Bown, 1991, and Chambius kasserinensis Hartenberger, 1986), Afrosoricida (Widanelfarasia Seiffert and Simons, 1999 and Dilambdogale gheerbranti Seiffert, 2010), Sirenia (Protosiren fraasi Abel, 1904 and Eotheroides Trouessart, 1905), Desmostylia (Behemotops Domning, Ray and McKenna, 1986), Embrithopoda (Palaeoamasia kansui Ozansoy, 1966 and Stylolophus minor Gheerbrant et al., 2018), Proboscidea (Eritherium azzouzorum Gheerbrant, 2009; Daouitherium rebouli Gheerbrant and Sudre, 2002; Phosphatherium esculliei Gheerbrant, Sudre and Cappetta, 1996; Moeritherium trigodon Andrews, 1904; and Numidotherium koholense Mahboubi, Ameur, Crochet and Jaeger, 1986), and Hyracoidea (Seggeurius amourensis Crochet, 1986; Microhyrax lavocati Sudre, 1979; and Titanohyrax andrewsi Matsumoto, 1922); and the Meridiungulata—Didolodontidae [Didolodus multicuspis Ameghino, 1897; Lamegoia conodonta Paula-Couto, 1952; and Paulacoutoia protocenica (Paula-Couto, 1952)], Litopterna [Paranisolambda prodromus (Paula-Couto, 1952) and Protolipterna ellipsoides Cifelli, 1983], Xenungulata (Carodnia vieirai Paula-Couto, 1952), Pyrotheria (Pyrotherium Ameghino, 1888 and Propyrotherium saxeum Ameghino, 1901), Notoungulata (Notostylops Ameghino, 1897 and Henricosbornia Ameghino, 1901), and Astrapotheria [Eoastrapostylops riolorense Soria and Powell, 1981; Maddenia lapidaria Kramarz and Bond, 2009; Parastrapotherium Ameghino, 1895; Astraponotus Ameghino, 1901; Trigonostylops wortmani Ameghino, 1897; Albertogaudrya unica Ameghino, 1901; and Tetragonostylops apthomasi (Price and Paula-Couto, 1950)]. Most of the specimens were analyzed directly by visiting fossil mammalian collections, although some others were studied based on casts, literature, and photographies (Simpson, 1948; Paula-Couto, 1952; Flynn, 1991; Simons et al., 1991; Gingerich et al., 1994; Seiffert and Simons, 2000; Meng and Wyss, 2001; Tabuce et al., 2001, 2007; Kumar et al., 2010; Seiffert, 2010; Kramarz et al., 2011; Kramarz and Bond, 2014; Sandersab et al., 2014; Gheerbrant et al., 2016, 2018; Hellmund, 2016; Erdal et al., 2016; Scott et al., 2016; Tabuce, 2016, 2018).
The phylogenetic analysis was conducted with the free software Tree analysis using New Technology (TNT), version 1.1 (Goloboff et al., 2008). The parsimony analysis was firstly performed by the algorithm “New Technology search,” and all characters are unordered. Secondly, another phylogenetic analysis was performed by the algorithm “New Technology search” with implied weighting.
Results
The first phylogenetic analysis yields three equally most parsimonious trees (tree length = 702 steps; Ci = 0.261; and Ri = 0.589), while the second, using implied weightings, results in a single most parsimonious tree (Figure 1; tree length = 716 steps; Ci = 0.256; Ri = 0.578). All traditional lineages are recovered as monophyletic (Springer et al., 2004), except for Tethytheria, Laurasiatheria, and Meridiungulata, the latter embracing two independent lineages. One “Meridiungulata” lineage comprises Didolodontidae and Litopterna, placed outside Afrotheria and closer to Hyopsodus (Figure 1). A paraphyletic Didolodontidae is a successive sister taxa of a monophyletic Litopterna, and this clade is the sister taxon of the Mioclaenidae Hyopsodus (Figures 1, 2; Williamson and Weil, 2011); this clade is the Panameriungulata of de Muizon and Cifelli (2000). The other “Meridiungulata” assembles Astrapotheria, Notoungulata, Pyrotheria, and Xenungulata (Figures 1, 2), and it is positioned among paenungulate afrotherians, closer to Hyracoidea, being supported by seven synapomorphies (Supplementary Material Section 4). Although recognized by previous studies (Soria, 1988; Billet, 2010; MacPhee et al., 2021), this monophyletic group was never formally proposed.
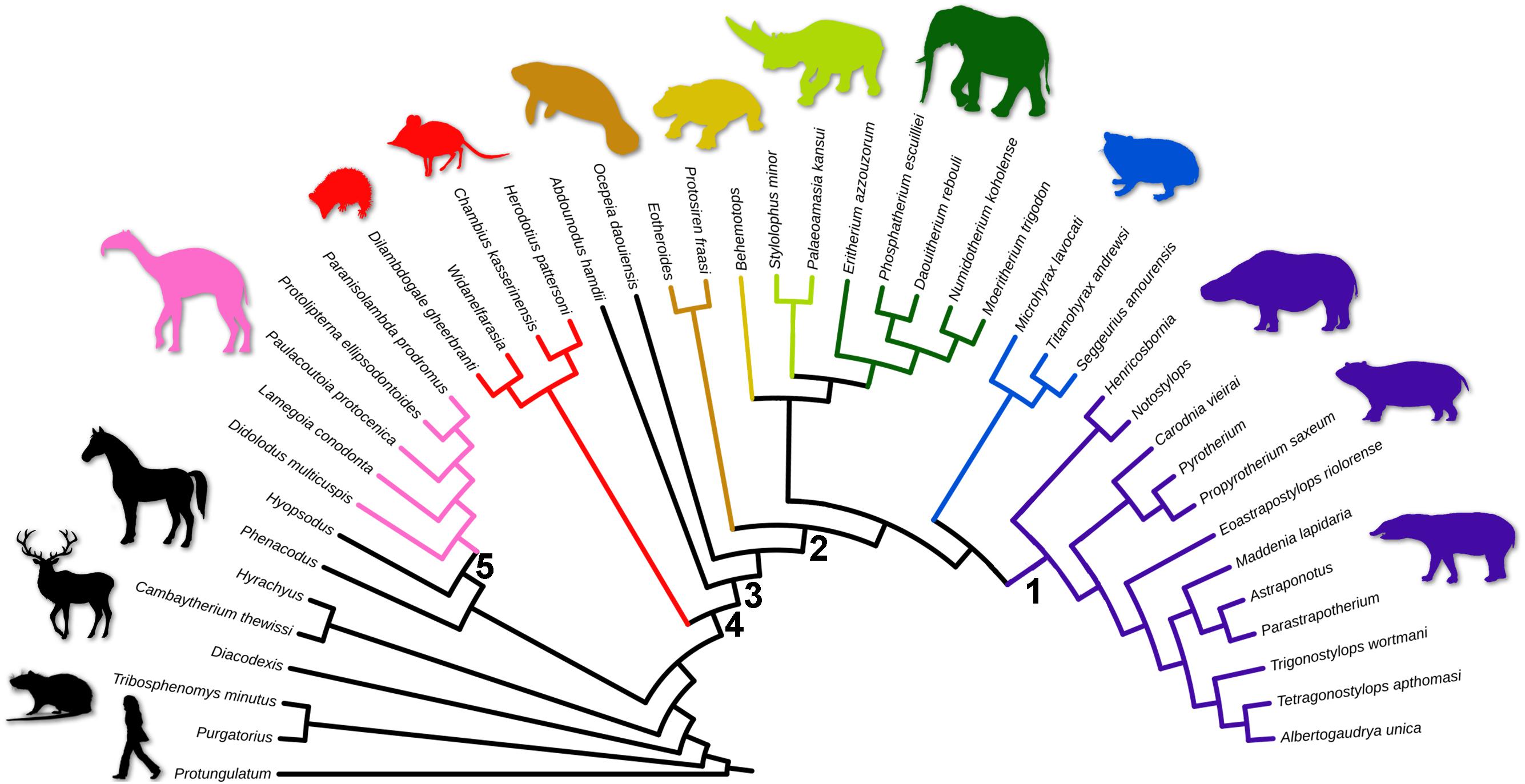
Figure 1. Phylogeny of Sudamericungulata within Eutheria, the single phylogenetic tree using the algorithm “New Technology search” with implied weighting. Colored clades are as follows: Sudamericungulata (purple), Hyracoidea (blue), Proboscidea (dark green), Embrithopoda (light green), Desmostylia (yellow), Sirenia (orange), Afroinsectivora (red), and Litopterna + “Didolodontidae” (pink). Numbered clades are as follows: (1). Sudamericungulata, (2). Paenungulata, (3). Paenungulatomorpha, (4). Afrotheria, and (5). Panameriungulata. The silhouettes of mammals are out of scale.
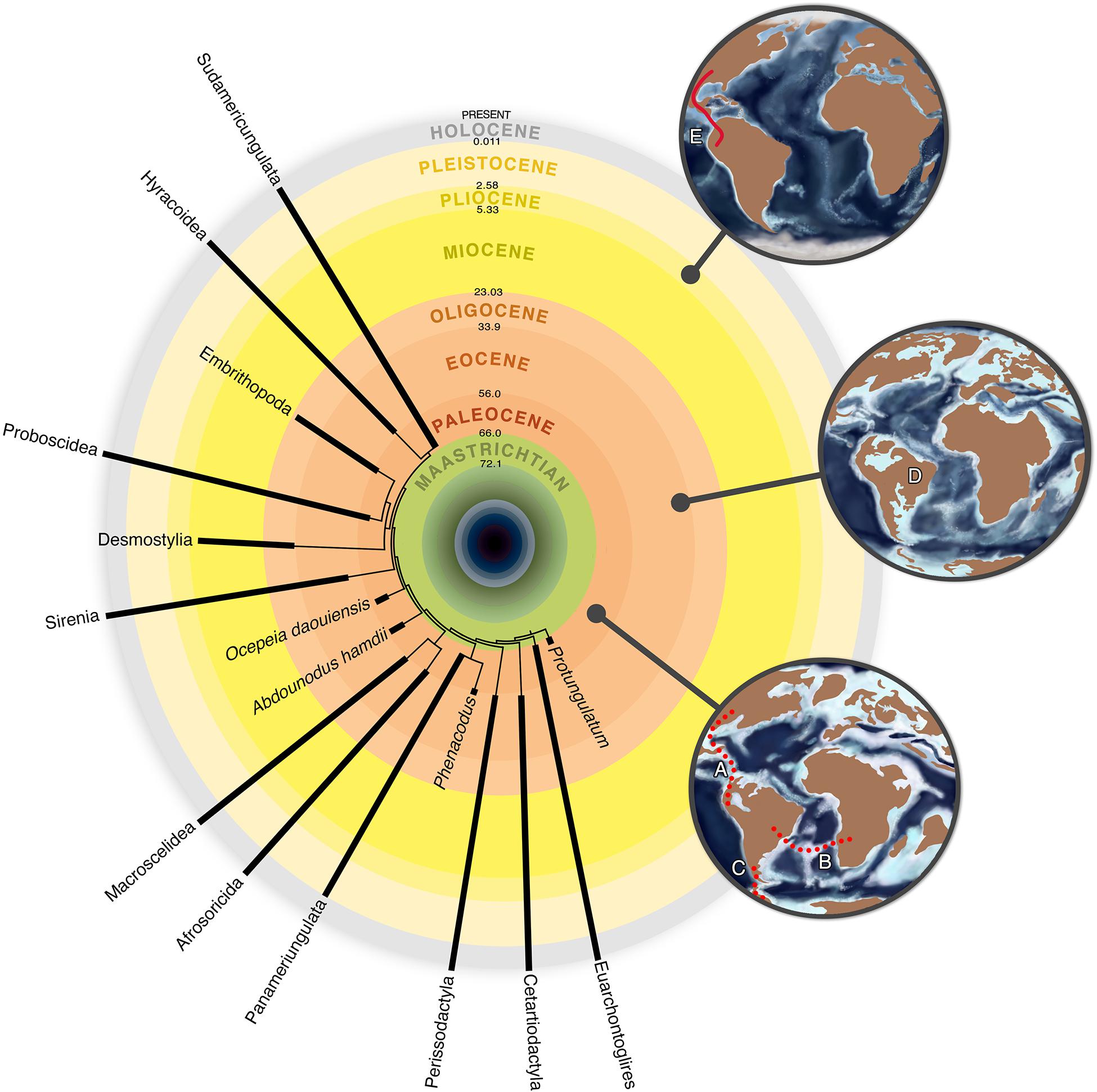
Figure 2. Timescale-calibrated Sudamericungulata relationships within Eutheria, showing the configuration of the continents in three Cenozoic moments. (A) Panamerican route, connecting South America to Central and North America. (B) Atlantogea route, an island chain connecting South America and Africa. (C) “Weddelian Isthmus” route, connecting South America and Antarctica. All routes were intermittent connections. (D) Isolated South America continent. (E) Panamanian Isthmus connecting South America with Central and North America, interrupting the South America geographical isolation.
Furthermore, we performed another analysis using implied weightings but applying “constraint” command on TNT software to force a monophyletic Boreoeutheria (Euarchontoglires and Laurasiatheria), and within it, a subgroup including Didolodontidae, Litopterna, Notoungulata, and Perissodactyla (Cambaytherium and Hyrachyus), to test molecular phylogeny results (Buckley, 2015; Welker et al., 2015; Westbury et al., 2017). This analysis resulted in a single parsimonious tree (tree length = 720 steps; Ci = 0.254; Ri = 0.574), which is four steps longer than our original results.
Systematic Paleontology
Mammalia Linnaeus, 1758
Eutheria Gill, 1872
Afrotheria Stanhope et al., 1998
Paenungulatomorpha Gheerbrant, Filippo and Schmitt, 2016
Paenungulata BR75
Sudamericungulata new taxon
Phylogenetic Definition
The lineage of SANUs, including Astrapotheria, Notoungulata, Pyrotheria, and Xenungulata, more closely related to the afrotherian Hyracoidea than to other afrotherians and eutherian mammals (Figures 1, 2).
Diagnosis
The unique combination of the following 15 features defines Sudamericungulata: metaconid present and equal in size with protoconid on lower p3; protocristid present and high on lower p3 and p4, forming protolophid; reduced trigonid; metacone present, and very close or fused to paracone; high and columnar parastyle present on P4, associated to a fold between paracone and parastyle; trigonid is reduced in relation to talonid; short cristid oblique, connected mesially to valid wall at the base of protoconid, associated to a marked ectoflexid; entoconid and hypoconulid relatively separated, where the latter is more distomedially positioned; preprotocrista present and high on M1 and M2; M1 parastylar lobe anteriorly positioned to paracone; metaconule prominent, mid-way or closer to metacone; M1–M2 metaconular hypocone absent; hypolophid (lophid joining hypoconid to hypoconulid) present; mesostyle absent; and dental substitution delay is absent.
Chronostratigraphy and Occurrence
The Sudamericungulata is recorded from early Paleocene (Marshall et al., 1997) to Early–Middle Holocene (Baffa et al., 2000) in South America and Early Eocene in the Antarctic Peninsula (Figures 2, 3; Bond et al., 2011).
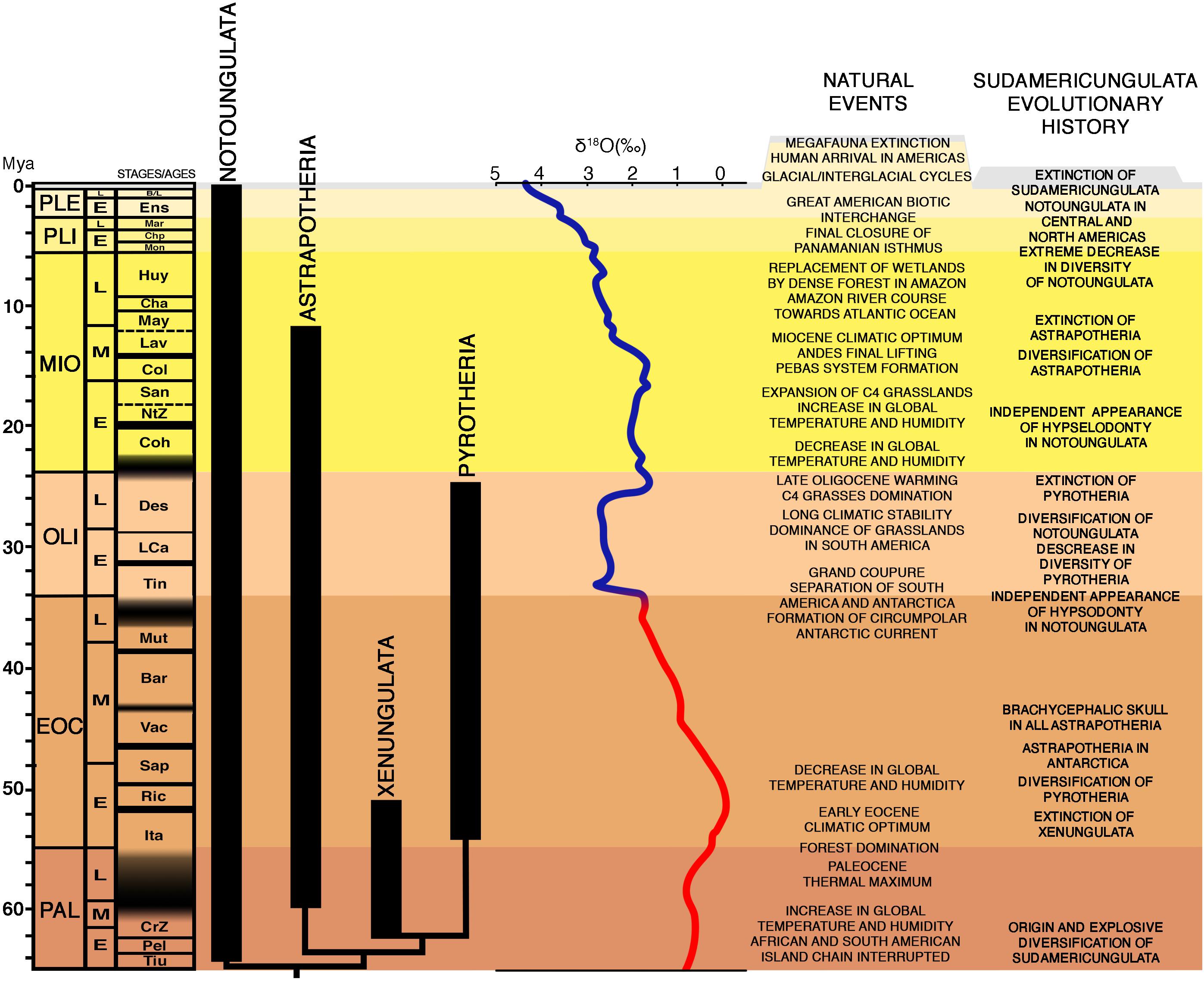
Figure 3. Interrelationships of the Sudamericungulata lineages and its evolutionary history. Phylogeny of Sudamericungulata calibrated by South American Stages/Ages: Tiu (Tiupampan), Pel (Peligran), CrZ (“Carodnia Zone”), Ita (Itaboraian), Ric (Riochican), Sap (“Sapoan”), Vac (Vacan), Bar (Barrancan), Mut (Mustersan), Tin (Tinguirirican), LCa (“La Cantera Fauna”), Des (Deseadan), Coh (Colhuehuapian), NtZ (“Notohippus Zone”), San (Santacrucian), Col (Colloncuran), Lav (Laventan), May (Mayoan), Cha (Chasicoan), Huy (Huayquerian), Mon (Monteherosan), Chp (Chapadmalalan), Mar (Marplatan), Ens (Ensenadan), B (Bonaerian), and L (Lujanian), modified from Croft (2016). The Zachos curve during Cenozoic is represented by the red and blue lines. The red portion represents the “Hot House World” when global temperature and humidity had a high average, while the blue portion represents the “Ice House World,” when global temperature and humidity had a low average (Zachos et al., 2001).
Etymology
Sudamerica is after the South American continent and the term Ungulata is from the Latin ungulatus, meaning “having hooves,” and is related to the ungulate mammals. Thus, Sudamericungulata means ungulates from South America.
Discussion
Phylogenetic Relationships of South American Native Ungulates
For a long time, the SANUs have been overlooked in traditional morphological phylogenies of Eutheria, and when mentioned, they were informally included in the Ungulata (McKenna, 1975). The eutherian tree has been shaken for the last 30 years by a molecular phylogenetic “revolution,” which started with the recognition of a lineage of African endemic mammals (Springer et al., 1997). Later, it was formally proposed as Afrotheria (Stanhope et al., 1998), breaking up the traditional Ungulata, since Afrotheria took four of their lineages (Proboscidea, Sirenia, Hyracoidea, and Tubulidentata; Springer et al., 2004). This “revolution” gradually shaded morphological-based phylogenetic studies that, since then, became rare. Also, extinct taxa were gradually excluded from phylogenies, as for a long time, techniques were unable to extract well-preserved molecular elements from fossils to be included on phylogenies. Under this scenario, the SANUs remained blurred until Agnolin and Chimento (2011) suggest that some of them (Astrapotheria and Notoungulata) might be related to Afrotheria. This study shed light on to SANUs, bringing back the debate on their interrelationships within Eutheria (Billet and Martin, 2011; O’Leary et al., 2013; Kramarz and Bond, 2014; Buckley, 2015; Welker et al., 2015; Westbury et al., 2017; Chimento and Agnolin, 2020; MacPhee et al., 2021). Despite the heated debate, none of these studies included all lineages of SANUs (at least Litopterna, Didolodontidae, Astrapotheria, Notoungulata, Pyrotheria, and Xenungulata) and other Eutheria (at least Afrotheria and Laurasiatheria, the traditional “Ungulata”) in the same phylogenetic analysis. Thus, the main issue about SANUs remained open: their interrelationships among Eutheria. To deal with this issue, we performed here the unique analysis comprising all SANUs and most Eutheria lineages (Euarchontoglires—Rodentia and Primates; Laurasiatheria—Cetartiodactyla and Perissodactyla; Afrotheria—Macroscelidea, Afrosoricida, Sirenia, Desmostylia, Embrithopoda, Proboscidea, and Hyracoidea; Figures 1, 2), and this is also the most representative phylogenetic study of Eutheria to include SANUs. However, to attain this representativeness and to guarantee that we were testing the morphological evolution of characters (testing of homologies), we had to restrict the morphological complexes included in the analyses to the skull and dental characters.
Our phylogenetic result is a very resolved topology (Figures 1, 2), and each resulted clade is well-supported by several synapomorphies (Supplementary Material Section 4), but it presented a low consistency index (under 0.3), which indicates that most of our primary homology proposals are homoplasies indeed. This was expected because most of the taxa included in our matrix were members of the “Ungulata”; traditionally included in this non-monophyletic lineage also exhibits dental and cranial adaptations to herbivory. Furthermore, we also included some taxa that were not part of “Ungulata,” the Rodentia Tribosphenomys minutus and the Primates Purgatorius (Euarchontoglires), but even these show dietary adaptations that include herbivory (Van Valen, 2004; López-Torres et al., 2018). The great diversification of eutherians during the K-Pg transition “produced” ungulate-like mammals in most lineages. This was, most probably, a positive natural selection in response to the domination of luxuriant forests in a warmer and more humid climate world of the Paleogene (Figure 3). Consequently, the high availability of plants promoted eutherian macroevolutionary events from insectivorous to herbivorous alimentary adaptations, i.e., from insectivorous-like to ungulate-like mammals. In this way, we also explain why most of the lineages recognized in our study were defined by ambiguous synapomorphies (Supplementary Material Section 4) because similar evolutionary selective pressures were acting in independent lineages, “producing” ungulate-like (herbivorous) eutherians along the Paleogene. Accordingly, convergent evolution often is considered one of the most powerful lines of evidence for adaptive evolution (Wake, 1991). However, the possibilities of morphological novelties’ appearance are not unlimited (Wake, 1991), and phylogenetic studies have countless cases where similarities often appear independently (convergencies, homoplasies) in lineages within a more comprehensive taxon, such as the ambiguous synapomorphies recovered in our study. Moreover, in cases like the one here for Eutheria, these homologies are at some level (mainly temporal, but also at a taxonomical level) (ambiguous) synapomorphies, being equally important to define lineages in comparison with unambiguous synapomorphies. Thus, our study made great progress in the comprehension of SANUs interrelationships within Eutheria, especially regarding that: (1) Meridiungulata is not monophyletic and (2) Litopterna is monophyletic and sister to a paraphyletic Didolodontidae. The clade formed by Litopterna and “Didolodontidae” is more closely related to Hyopsodus than to other taxa included in this study; and (3) Astrapotheria, Notoungulata, Pyrotheria, and Xenungulata shared a common ancestor, and this clade is part of paenungulate afrotherians (Figures 1, 2).
The validity of Meridiungulata was questioned in the last decades since it was not recovered as a natural group in most phylogenetic studies (Avilla, 2006; Billet, 2010; Agnolin and Chimento, 2011; O’Leary et al., 2013; de Muizon et al., 2015; MacPhee et al., 2021). In fact, in the last years, the sole defenders of a monophyletic Meridiungulata are molecular-based studies (Buckley, 2015; Welker et al., 2015). However, these contributions poorly sampled SANU lineages (only the Litopterna M. patachonica and the Notoungulata T. platensis) to test the monophyly of SANU and even to recognize its interrelationships among Eutheria. Unfortunately, molecular studies still have technical limitations (Rizzi et al., 2012) that hinder the inclusion of all SANU lineages, because no genomic or proteomic fossils older than the latest Early Pleistocene (about one million years before present) were recovered until now (van der Valk et al., 2021), and Astrapotheria, Pyrotheria, Xenungulata, and Didolodontidae were extinct before the early Pliocene (Figure 3). Moreover, Song et al. (2012) published a detailed analysis of the molecular phylogenetic methods available at that moment and present a deep criticism on concatenation phylogenetic analyses, the same method applied by Buckley (2015); Welker et al. (2015), and Westbury et al. (2017). Compared with coalescent phylogenetic analyses, those conducted by Buckley (2015); Welker et al. (2015), and Westbury et al. (2017) are not the most reliable to recover phylogenetic relationships and might result in fake topology (Song et al., 2012). Furthermore, Song et al. (2012) indicated that the lesser the diversity of taxa used, the greater the possibility of resulting in a false topology. Thus, we recognize that these three studies have all the merit and great significance since they were able to recover ancient molecular data on SANU taxa. However, since ancient molecular techniques are still developing, their results, mainly a molecular-based taxonomy, must be carefully assumed.
The only proposal of the interrelationships of SANU that seems to be a consensus among scientists is a clade composed of Litopterna and Didolodontidae (Cifelli, 1983). However, although de Muizon and Cifelli (2000) also recovered this clade, the Didolodontidae was not monophyletic, positioning as a successive sister taxa at the base of the Litopterna. Furthermore, the clade formed by Litopterna and “Didolodontidae” is more closely related to Mioclanidae, “condylarthrans” from North and South America, and in recognition of this result, de Muizon and Cifelli (2000) raised Panameriungulata. Our study recovered the same Panameriungulata of de Muizon and Cifelli (2000) (Figures 1, 4). However, in the last few years, the Panameriungulata was challenged by results of molecular and morphological phylogenies that found a clade comprising Litopterna and Notoungulata (Buckley, 2015; Welker et al., 2015) or only Litopterna (Westbury et al., 2017) as more closely related to Perissodactyla than to other Eutheria, even to the other SANUs (Chimento and Agnolin, 2020; MacPhee et al., 2021). Despite the particularities of each topology of these contributions, a close relationship between Litopterna and Perissodactyla was proposed as a new rank of Laurasiatheria, the Panperissodactyla.
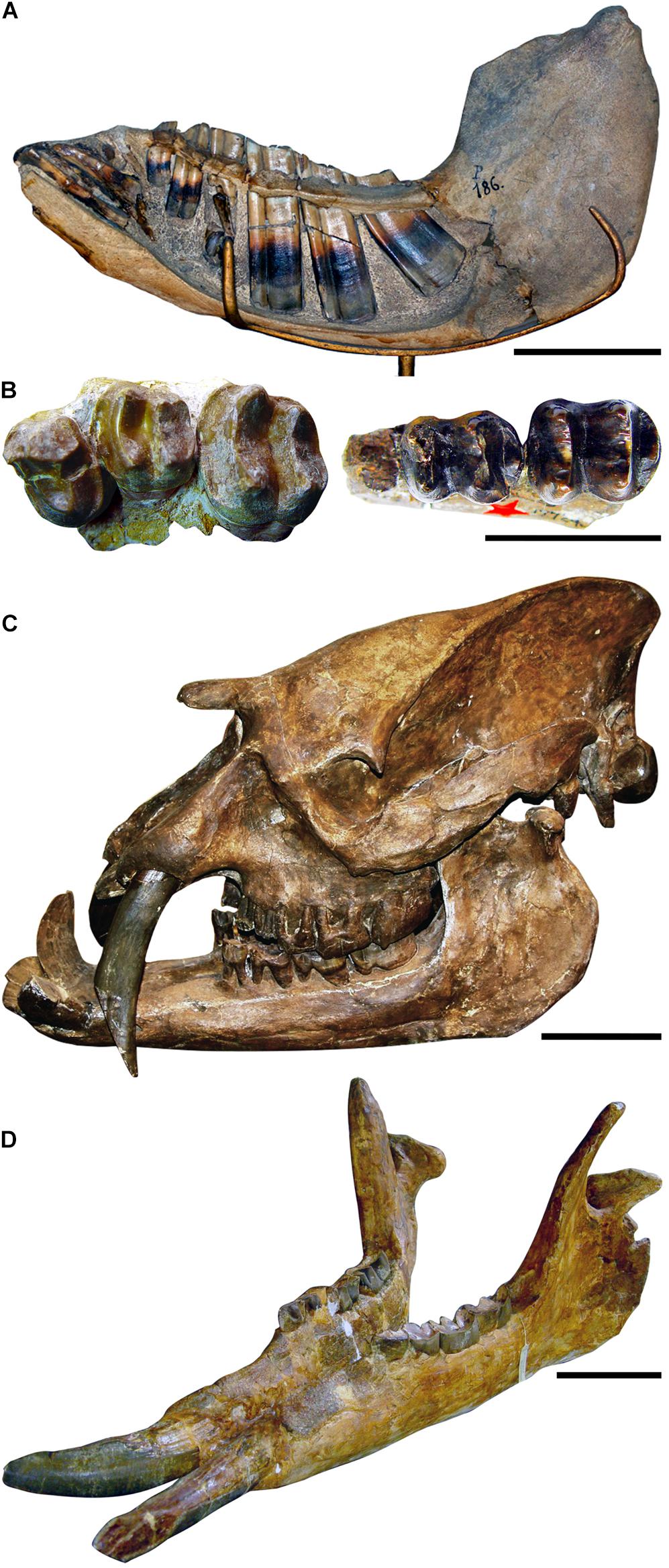
Figure 4. Some evolutionary traits of Sudamericungulata lineages. (A) Left hemimandible of a notoungulate toxodontid showing hypselodonty (ever-growing cheek teeth) in lateral view. Note the teeth with open roots. Photograph courtesy by D. Croft, and reuse permitted under CC BY-NC-SA 2.0. (B) Left P4-M2 of the xenungulate Carodnia vieirai (on the left) and left dp4-m1 of the pyrothere Griphodon peruvianus Anthony, 1924 (on the right) showing the bilophodonty. Photograph courtesy of Carodnia vieirai by L. Bergqvist. (C) Skull of the astrapothere Astrapotherium magnum Owen, 1853, in lateral view. Note the ever-growing tusk-like canines, the absence of upper incisors, the retracted nasal opening, and the reduction of cheek teeth. (D) Mandible of the astrapothere uruguaitheriine Granastrapotherium snorki Johnson and Madden, 1997. Note its gigantic size, the absence of lower incisors, the tusk-like and protruded lower canines, and the extreme reduction of lower cheek teeth. Scale bar in (A,C,D) = 10 cm and in (B) = 5 cm.
Both Panameriungulata and Panperissodactyla propositions present problems, mainly the poor representativeness of Eutheria, compromising the testing of lineages interrelationships. In the original description of Panameriungulata (de Muizon and Cifelli, 2000), the ingroup was composed only by the taxa recognized as Panameriungulata, without any other Eutheria lineage to test its monophyly. Some authors also suggest a closer relationship between kollpaniines and early diverging Notoungulates based on the morphology of tarsal and dental features (Cifelli, 1993; de Muizon and Cifelli, 2000; de Muizon et al., 2019). However, none have tested these proposals in a phylogenetic context, so they are still untested inferences. About the studies that recognized Panperissodactyla, the molecular ones (Buckley, 2015; Welker et al., 2015; Westbury et al., 2017) were already discussed and criticized above, but the morphological studies also present poor eutherian representativeness, hindering comprehensive testing of Eutheria relationships. Surprisingly, Chimento and Agnolin (2020) did not include Astrapotheria, Xenungulata, Pyrotheria, and Notoungulata to test their hypothesis proposed in 2011, regarding the Astrapotheria and Notoungulata as part of Afrotheria. Furthermore, Afrotheria is only represented by Proboscidea and Hyracoidea, and many other lineages are missing.
Despite the eutherian and SANUs representativeness problems in MacPhee et al. (2021), this study recovers Litopterna, Perissodactyla, and Panperissodactyla as paraphyletic. In this way, although it was not our focus to test particularly the phylogenetic position of Litopterna among Eutheria, our study recovers the original Panameriungulata (de Muizon and Cifelli, 2000). However, we believe that to fully test the phylogenetic position of Litopterna and its kin (“Didolodontidae” and Kollpaniinae), the analysis must include other laurasiatherians, such as the Carnivora, Eulipotyphla, and Chiroptera, among others. Nevertheless, we recognize that all late studies, including our own, seem to agree that Litopterna is a lineage of Laurasiatheria.
One of the most important results of our phylogeny is a clade comprising Astrapotheria, Notoungulata, Pyrotheria, and Xenungulata, the Sudamericungulata (Figures 1–3). Some classic studies suggested lineages composed by some of these taxa, such as grouping Notoungulata and Astrapotheria (the Protungulata of Simpson, 1945) and the inclusion of Xenungulata in Pyrotheria and a closer relationship of Notoungulata and Astrapotheria (Van Valen, 1988). However, these relationships were only inferred but not formally tested under phylogenetic analyses. Later, these proposals were reassessed and tested by a phylogenetic approach by Billet (2010, 2011), which recognizes Pyrotheria as part of Notoungulata and this lineage closer to Astrapotheria. Concomitantly, Agnolin and Chimento (2011) also took up the clade comprising Astrapotheria and Notoungulata and related it to Afrotheria. Recently, MacPhee et al. (2021) recognize SANUs as diphyletic and one of these lineages comprises Astrapotheria, Notoungulata, and Pyrotheria (clade C of MacPhee et al., 2021). Although this study lacked the Xenungulata, the clade C resembles our Sudamericungulata (Figures 1–3). However, the study of MacPhee et al. (2021) recognized six unambiguous synapomorphies defining the clade C, but the only true unambiguous synapomorphy, i.e., not shared to other terminal taxa in their matrix, is the presence of Ectoloph (high crest joining paracone and metacone) on upper molars (and premolars) (character 24, state 1). All other five synapomorphies of clade C are ambiguous in their matrix, such as character 23, state 1, and character 48, state 2, which are also present in Tapirus. Moreover, as MacPhee et al. (2021) poorly represented eutherian lineages, some homologies considered by them as unambiguous synapomorphies would also be shared with other eutherian lineages, such as the Afrotheria, not represented in their sample.
Most of the criticism of the literature which addressed SANUs interrelationships demonstrates the importance to include the most representative diversity as possible when testing interrelationships of a high-level taxon. Here, our approach aimed to mitigate the issues presented above in many different SANUs evolutionary hypotheses, moving forward, and oxygenating these discussions, to comprehend Eutheria evolution.
Biogeography of SANUs
Together with marsupials and xenarthrans, the SANUs composed the Archaic South American mammals (Simpson, 1980; Flynn and Wyss, 1988). Although they were included in the faunal stratum 1 of Simpson, possibly originated in this continent during the Early Paleogene (Simpson, 1980), their poorly defined phylogenetic relationships hinder their biogeography comprehension. Two independent biogeographic patterns were recognized in our phylogeny: South America shared a common ancestral area with North America (Figure 2A; Panameriungulata) and, in another moment, with Africa (Figure 2B; Sudamericungulata).
Panameriungulata comprises South and North American ungulates (Cifelli, 1983; de Muizon and Cifelli, 2000; contra Chimento and Agnolin, 2020), suggesting a closer biogeographical relationship among all Americas during the Early Paleocene (Figure 2A). A similar pattern was also recognized for the laurasiatherian Pantodonta (de Muizon et al., 2015) and other Paleocene and Eocene mammals (Gelfo et al., 2009). Furthermore, the oldest Cenozoic mammalian fauna from South America—the Tiupampa Early Paleocene fauna from central Bolivia—relates closely to Puercan Paleocene North American faunas, more than to any other Paleogene South American faunas (Gelfo et al., 2009).
A Panamerican terrestrial connection, such as a land bridge (persistent) or an archipelago (intermittent), possibly connected North and South America, at least during the Early to Middle Paleocene (Figure 2A). Subsequently, South America remained isolated from Central-North America, at least, from the Late Paleocene to the earliest Miocene, time interval when no mammalian interchange between North and South America was established. Since then, the earliest evidence of American mammalian interchange is the Platyrrhyni Panamacebus Bloch et al., 2016, member of a South American lineage of primates, from the Early Miocene of Central America (Bloch et al., 2016).
Along the Late Miocene, native North American mammals were recorded in South America (e.g., the procyonid Cyonasua Ameghino, 1885, see Tarquini et al., 2019; and the proboscidean “Amahuacatherium” Romero-Pittman, 1996; Mothé and Avilla, 2015), while native South American mammals reached both North America and the Caribbean by independent routes (xenarthran ground-sloths, Delsuc et al., 2019). However, these records preceded the Great American Biotic Interchange first pulses, which began only after the complete imposition of Panamanian Isthmus, at the latest Pliocene (Figure 2E; Woodburne, 2010). Moreover, the record of some mammals of Holarctic origins in the Miocene of Amazon led to an alternative hypothesis to explain their arrival into South America, across GAARlandia (Agnolin et al., 2019). Nevertheless, both the Miocene age and taxonomic validity of these records had been questioned, and they might represent Pleistocene ordinary taxa (Mothé and Avilla, 2015; Gasparini et al., 2021).
Sudamericungulata supports a closer biogeographic relationship between South America and Africa continents (Figure 2B), which formed Gondwana and have been separating since Early Cretaceous (Aptian-Albian, 120–99.6 Mya; Ezcurra and Agnolin, 2012). The ages of the oldest taxon of Sudamericungulata (a Tiupampan notoungulate; Marshall et al., 1997; de Muizon et al., 2019; see Figures 2, 3) and the oldest taxa of Hyracoidea (an indeterminate taxon possibly from the Late Paleocene of Mali, see O’Leary et al., 2006; and Seggeurius from the Early Eocene of Algeria; Tabuce et al., 2008) suggest that the divergence between these clades probably took place at Early Paleocene (Figures 2, 3), instead of at Early Cretaceous. This pattern fits the Atlantogea biogeographic model: a connection between Africa and South America through an intermittent island chain crossing the South Atlantic sea from Late Cretaceous to Late Eocene (83.5–36.6 Mya; Ezcurra and Agnolin, 2012; Figure 2B).
Sudamericungulata and Panameriungulata were not restricted to South America: the toxodontid notoungulates (a Sudamericungulata) migrated to Central and North America during the Great American Biotic Interchange (Figures 2E, 3; Mixotoxodon Van Frank, 1957, Pleistocene of El Salvador and the United States), while basal astrapotheres (Antarctodon sobrali Bond et al., 2011, a Sudamericungulata) and litopterns (Notiolofos arquinotiensis Bond et al., 2006, a Panameriungulata) were found in shallow marine outcrops of La Meseta Formation, in the Antarctic Peninsula, dating from the late Early Eocene (Figure 3; Woodburne, 2010; Bond et al., 2011; Gelfo et al., 2015). From the Late Cretaceous to the early Late Eocene, the landmasses of Australia, Antarctica, and southernmost South America were associated through the “Weddellian Isthmus” (Woodburne and Zinsmeister, 1984; Reguero et al., 2013), probably forming intermittent connections during this interval (Figure 2C).
The “Weddellian Isthmus” possibly lasted until 41 Mya, when South America became completely isolated (Figures 2D, 3), after the Antarctic Circumpolar Current establishment (Livermore et al., 2004). The imposition of the “Weddellian Isthmus” perhaps allowed the biotic interchange between South America and Oceania, via Antarctica, at the beginning of Cenozoic (Figure 2C). This hypothesis explains the presence of monotremes in South America, the Monotrematum sudamericanum Pascual et al., 1992, from the Early Paleocene (Peligran Stage/Age of Southern Argentina; Pascual et al., 2002) and the origin of Australidelphia marsupials in Oceania, whose divergence estimations indicate an Early Paleocene origin (Meredith et al., 2009). Consequently, by recognizing the Panameriungulata as a laurasiatherian and Sudamericungulata as an Afrotheria clade, all mammalian lineages that survived into the Cenozoic occurred in or, at least, used Antarctica as a dispersion route (Figure 2C) during the Early Paleogene (Monotremata, Marsupialia, Xenarthra, Boreoeutheria, and Afrotheria; see Goin et al., 1999; Pascual et al., 2002; Gelfo et al., 2015).
Therefore, Antarctica was extremely important to mammalian evolution and had a megabiodiversity at that time. Unfortunately, the great diversity of Antarctica got extinct at the end of the Eocene and the beginning of the Oligocene when it became isolated with the formation of the Antarctic Circumpolar Current (Figures 2D, 3). This new oceanic dynamic completely changed the Antarctica environment and climate to a severe polar desert, and the frosted continent drove the global climates from a “Hot House World” to an “Ice House World” (Figure 3; Zachos et al., 2001).
The Evolutionary History of Sudamericungulata
Despite that there is still no consensus for relationships within Paenungulata, there is a general agreement on its monophyly and divergence from other afrotherians between 80 and 62 Mya (Late Cretaceous and earliest Paleocene; Springer et al., 2003; Pardini et al., 2007; Benoit et al., 2015; Figure 2). The Sudamericungulata origin was possibly by vicariance from a common African–South American ancestor shared with Hyracoidea (Figures 2, 3), after one of the intermittences of the South Atlantic transoceanic island chain during the Early Paleocene (Figure 2B, Atlantogea biogeographic model; Ezcurra and Agnolin, 2012).
Sudamericungulata comprises four monophyletic extinct lineages (Astrapotheria, Notoungulata, Pyrotheria, and Xenungulata; Figure 3). Pyrotheria and Xenungulata resulted as sister groups, and Astrapotheria was closer to this clade than to Notoungulata, which was the first group to diverge within Sudamericungulata (Figures 1, 3). The divergence of these four lineages was probably the result of a rapid diversification during the Early Paleocene, matching the explosive model of ordinal diversification for the Eutheria (Springer et al., 2003; Figure 3).
Notoungulata occurred from the early Paleocene of Tiupampan Stage/Age of Bolivia to the Middle Holocene (Paula-Couto, 1952; Marshall et al., 1997; Baffa et al., 2000; Bauzá et al., 2019; de Muizon et al., 2019), being the longer lasting (Figure 3) and, by far, the most diversified lineage among Sudamericungulata [10 families (Billet, 2011) and about a hundred of valid species]. This amazing notoungulate diversity includes from small rodent-like to rhino-sized ungulates. Furthermore, many hypsodont notoungulates occurred sometime between 36 and 32 Mya (Late Eocene–Early Oligocene; Figure 3; Madden, 2014) and may correspond to three or more independent acquisitions (Billet, 2011). Although some authors reject the argument that grasslands were established at that moment in South America, the evolution of hypsodonty in Sudamericungulata was probably a response to the use of more abrasive food frequently found in the South American grasslands. Moreover, after the latest Oligocene, during the Early Miocene, some families independently developed hypselodonty (ever-growing cheek teeth; Figure 4A), probably selected by a dominated C4 grasses scenario (Figure 3). In summary, notoungulates originated as browser forest dwellers and changed to very diversified lineages of openland grazers, an interesting example of the “adapt or die” evolutionary process, but in two subsequent stages (Figure 3): first, it developed hypsodonty; and then, the hypselodonty. However, this diversity inexplicably decreased from Pliocene onward (Figure 3), and only a few species survived into the Pleistocene (Simpson, 1980; Billet, 2011). Although most notoungulates were not restrictly grazers (Croft, 2016), maybe the direct competition with the new Holarctic immigrants (horses, proboscideans, llamas, deers, etc.) for consumption of grasses contributed to most of notoungulates extinction.
Traditionally, notoungulates got extinct together with other megafauna mammals (the native ones and some of the Holarctic immigrants) on the Pleistocene–Holocene transition (Figure 3), and the extinction causes are still incognita. However, T. platensis survived longer into the Middle Holocene, according to some absolute datings (Baffa et al., 2000; Figure 3). There is no known evidence of the ecological interactions of Toxodon Owen, 1837 and ancient humans (including hunting and predation), and consequently, the most probable cause for its extinction might be the loss/reduction of its most frequent habitat, the grassland, by the imposition of evergreen forests. An increase in humidity led to this drastic environmental change during the Holocene Climatic Optimum (Middle Holocene; de Vivo and Carmignotto, 2004).
Pyrotheria and Xenungulata shared the bilophodont dentary condition (Figure 4B) and are sister taxa (Figures 1, 3), indicating that this very particular tooth morphology arose once in the Sudamericungulata. The bilophodonty was selected in the clade Pyrotheria and Xenungulata, probably because it originated and lived in high temperatures and humidity forest-dominant habitats (Figure 3). Most extant bilophodont cheek teeth mammals are forest-dweller browsers (Janis, 2008)—a diet mainly composed of tender leaves and fruits.
Xenungulata had the shorter evolutionary history among Sudamericungulata: recorded from the Early to Middle Paleocene of the “Carodnia Zone” of Patagonia, southern South America, to the Itaboraian Stage/Age (Early Eocene; Gelfo et al., 2008; Figure 3). The fossil record of Pyrotheria indicates its origin into the Early Eocene of northern South America (Salas et al., 2006), but its phylogenetic relationship suggests an earlier origin, from the Early Paleocene (Figure 3). During the moment of the origin of the clade composed by Pyrotheria and Xenungulata, the global average temperatures and humidity were higher than the present levels (Figure 3; Zachos et al., 2001), resulting in a profusion and domination of tropical forests in South America (Croft, 2016). Interestingly, there are no records of Pyrotheria from the Paleocene to the earliest Eocene in Argentinian Patagonia outcrops, which bear several fossiliferous and stratigraphical/temporal continuous paleontological sites. In fact, Pyrotheria records started in the Argentinian Patagonia only after Early–Middle Eocene, when Xenungulata was no longer recorded there. Humidity and temperature increased the domination of forests in South America during the Late Paleocene Thermal Maximum and Early Eocene Climatic Optimum (Figure 3; Zachos et al., 2001), allowing Pyrotheria to expand its southern limits to Patagonia. Since both taxa were bilophodont, brachiodont, and forest-dweller browsers, pyrotheres might have contributed to the extinction of the Xenungulata (Figure 3) by ecological competition.
The disconnection from Antarctica during Late Eocene (Figures 2D, 3) drove South America into cooler conditions, which led habitats to open at the newly isolated continent (Croft, 2016). These climatic conditions became stable all through the Oligocene (Figure 3). However, what was once a great evolutionary novelty (the bilophodonty, Figure 4) for Pyrotheria possibly turned into their curse. First, Pyrotheria decreased its diversity throughout the Oligocene, being only represented by the giant Pyrotherium at Late Oligocene (Deseadan Stage/Age, Figure 4), when grasslands ruled over forests. Later, the humidity and temperature increased once more (Zachos et al., 2001) and the grasslands became dominated by C4 grasses at the latest Oligocene (Figure 3), since these grasses are more adapted to humid and warm environments, with higher luminosity. The more abrasive and harder to digest C4 grasses might have caused a huge problem to brachiodont/bilophodont ungulates during their consumption, being the most possible reason for the extinction of Pyrotheria (Figure 3). Accordingly, different lineages of notoungulates from Late Oligocene and Early Miocene independently developed ever-growing cheek teeth (Figures 3, 4; hypselodonty) (Billet, 2010), being a very efficient tool to oral processing of C4 grasses.
Astrapotheria is a lineage with a long-lasting history; its oldest record is from the early Middle Paleocene (E. riolorense, Rio Loro Formation, northwestern Argentina) and several Laventan Stage/Age (Middle Miocene) outcrops from northern South America marked its last records (Figure 3). Although recent studies recovered Eoastrapostylops outside Astrapotheria (Kramarz et al., 2017; MacPhee et al., 2021), our results support a monophyletic Astrapotheria. Despite the timespan, Astrapotheria matches with Pyrotheria and Xenungulata in having a low diversity, unlikely Notoungulata. Since its early diversification, Astrapotheria had fascinating features, such as the absence of upper incisors and the presence of well-developed tusk-like ever-growing canines (Figure 4C). Two astrapothere lineages were recognized from Early Eocene, and one of these (represented here by Maddenia, Parastraphotherium, and Astraponotus; Figure 1) developed brachycephalic skulls, i.e., short rostrum with the retraction of narials toward the top of the skull (Figure 4C). Subsequently, the brachycephalic skull became the exclusive condition for astrapotheres from the Middle Eocene (Stage/Age Vacan; Kramarz et al., 2019; Figure 3).
In addition, the great diversification of astrapotheres occurred from the Middle Miocene, probably associated with the Miocene Climatic Optimum (Figure 3). This diversification was characterized by the extreme increase in body size, the dentition reduction, the hypsodont cheek teeth, and the retraction of nasal aperture toward the top of the skull (Figure 4). These structures, in addition to those astrapothere original features, resemble the proboscideans, except that the latter have tusk-like incisors, instead of canines. This climatic change was possibly a consequence of the last stages of Andean uplift, the formation of the Pebas System, and the subsequent establishment of the new course of the Amazon River toward the Atlantic Ocean (Figure 3). The Pebas System was a complex of lakes in western Amazon lasting, at least, from the Early (Santacrucian Stage/Age) to Middle (Laventan Stage/Age) Miocene (Wesselingh et al., 2002). The only remaining question is if the Pebas System was connected in its southern limits with the “Paranense sea,” forming a huge continuous wetland from the western Amazon region to Northern Argentinian Patagonia (Cozzuol, 2006; Candela et al., 2012). Accordingly, this wetland would be very similar to the current Brazilian biome Pantanal, an open lowland with innumerous intermittent connected lakes that isolated large “islands” (Cozzuol, 2006). This huge wetland was the home of one of the two lineages of Miocene astrapotheres, the Uruguaytheriinae (Kramarz and Bond, 2009), which were possibly semi-aquatic, giant, grazer beasts, being the most diverse group of Astrapotheria (Kramarz et al., 2011; Vallejo-Pareja et al., 2015). The great diversification of Uruguaytheriinae might be the result of the intermittence of lakes in this wetland during Miocene Climatic Optimum (Figure 3). After this humidity global peak, the entire world experimented with another slow increasing drought, leading to the domination of grasslands from the Late Miocene to Late Pleistocene (from its last million years), except for western Amazon, where the Tropical Amazon Rainforest dominated (Figure 3). Consequently, the gigantic grazers uruguaytheriines (Figure 4D) might have perished in this new Amazon dense environment and got extinct, since there is no record of Astrapotheria after the Middle Miocene (Laventan Stage/Age) (Figure 3).
The recognition of Sudamericungulata and the comprehension of its evolutionary history are mandatory to understand the geological, biological, and biogeographical history of the South American continent itself, as well as the evolution of Eutheria. As previously discussed, Sudamericungulata beheld and sometimes starred the natural events that shaped this continent. Multiple aspects of South American natural history are still poorly known and outdated; however, this contribution sheds light on mammalian evolution in South America.
Data Availability Statement
The original contributions presented in the study are included in the article/Supplementary Material, further inquiries can be directed to the corresponding author/s.
Author Contributions
Both authors listed have made a substantial, direct and intellectual contribution to the work, and approved it for publication.
Funding
The thesis that was the basis for this contribution was funded by doctoral scholarships from Coordenação de Aperfeiçoamento de Pessoal de Nível Superior (CAPES). LA also extends his thanks to the Conselho Nacional de Desenvolvimento Científico e Tecnológico (CNPq) for postdoctoral scholarship 248772/2013-9 and researcher scholarship 307555/2016-0 and to the Fundação Carlos Chagas Filho de Amparo à Pesquisa do Estado do Rio de Janeiro (FAPERJ) researcher scholarship E25/2014-Jovem Cientista do Nosso Estado. DM thanks FAPERJ for the postdoctoral scholarships 202.375/2018 and 202.376/2018. This study was also part of the project entitled “BIOGEORIO: Biodiversidade e Aspectos Biogeográficos Transatlânticos da América do Sul e África durante o Cenozóico” which was funded by the FAPERJ (E_14/2019, Apoio à Grupos Emergentes de Pesquisa no Estado do Rio de Janeiro—2019; under process number: 251221).
Conflict of Interest
The authors declare that the research was conducted in the absence of any commercial or financial relationships that could be construed as a potential conflict of interest.
Acknowledgments
We dedicate this manuscript to our parents and the students who became scientists under our mentorship. We show total appreciation to the curators of the collections visited during this project. This study is part of the Ph.D. thesis of LA, conducted at Programa de Pós-graduação em Zoologia (PPGZOO), in the Museu Nacional, Rio de Janeiro (Brazil), under the mentorship of Ronaldo Fernandes (Museu Nacional), John Flynn (Field Museum of Natural History and American Museum of Natural History), and Lilian Paglarelli Bergqvist (Universidade Federal do Rio de Janeiro). We would like to thank immensely the editor, ME, and the reviewers, FA and MB, for excellent suggestions and corrections that have certainly greatly improved our manuscript since its earliest versions.
Supplementary Material
The Supplementary Material for this article can be found online at: https://www.frontiersin.org/articles/10.3389/fevo.2021.654302/full#supplementary-material
References
Agnolin, F. L., and Chimento, N. R. (2011). Afrotherian affinities for endemic South American “ungulates”. Mamm. Biol. 76, 101–108. doi: 10.1016/j.mambio.2010.12.001
Agnolin, F. L., Chimento, N. R., and Lucero, S. O. (2019). Pre-GABI biotic connections between the Americas: an alternative model to explain the “Less-splendid isolation” of South America. Rev. Geol. Am. Centra 61, 91–106.
Ameghino, F. (1889). Contribución al conocimiento de los mamíferos fósiles de la República Argentina. Actas Acad. Nac. Cienc. Córdoba 6, 1–1027.
Ameghino, F. (1897). Mammifères crétacés de l’Argentine (Deuxième contribution à la connaissance de la faune mammalogique des couches à Pyrotherium). Bol. Inst. Geog. Argent. 18, 406–521.
Avilla, L. S. (2006). Sistemática de Astrapotheria, Pyrotheria e Xenungulata (Mammalia: Ungulata: Uintatheriamorpha). Doctoral Thesis. Brazil: Universidade Federal do Rio de Janeiro.
Baffa, O., Antonio-Brunetti, A., Karmann, I., and Dias-Neto, C. M. (2000). ESR dating of a toxodon tooth from a Brazilian karstic cave. Appl. Radiat. Isot. 52, 1345–1349. doi: 10.1016/s0969-8043(00)00093-2
Bauzá, N., Gelfo, J. N., and López, G. M. (2019). Early steps in the radiation of notoungulate mammals in southern South America: a new henricosborniid from the eocene of patagonia. Acta Palaeon. Pol. 64, 597–603.
Benoit, J., Crochet, J., Mahboubi, M., Jaeger, J., Bensalah, M., Adaci, M., et al. (2015). New material of Seggeurius amourensis (Paenungulata, Hyracoidea), including a partial skull with intact basicranium. J. Vertebr. Paleontol. 36:e1034358. doi: 10.1080/02724634.2015.1034358
Billet, G. (2010). New observations on the skull of Pyrotherium (Pyrotheria, Mammalia) and new phylogenetic hypotheses on South American ungulates. J. Mammal. Evol. 17, 21–59. doi: 10.1007/s10914-009-9123-0
Billet, G. (2011). Phylogeny of the Notoungulata (Mammalia) based on cranial and dental characters. J. Syst. Palaeo. 9, 481–497. doi: 10.1080/14772019.2010.528456
Billet, G., and De Muizon, C. (2013). External and internal anatomy of a petrosal from the late Paleocene of Itaboraí, Brazil, referred to Notoungulata (Placentalia). J. Vertebr. Paleontol. 33, 455–469. doi: 10.1080/02724634.2013.722153
Billet, G., and Martin, T. (2011). No evidence for an afrotherian-like delayed dental eruption in South American notoungulates. Sci. Nat. 98, 509–517. doi: 10.1007/s00114-011-0795-y
Bloch, J. I., Woodruff, E. D., Wood, A. R., Rincon, A. F., Harrington, A. R., Morgan, G. M., et al. (2016). First North American fossil monkey and early Miocene tropical biotic interchange. Nature 533, 243–246.
Bond, M., Kramarz, A., MacPhee, R. D. E., and Reguero, M. (2011). A new astrapothere (Mammalia, Meridiungulata) from La Meseta Formation, Seymour (Marambio) Island, and a reassessment of previous records of Antarctic astrapotheres. Am. Mus. Novit. 3718, 1–16. doi: 10.1206/3718.2
Buckley, M. (2015). Ancient collagen reveals evolutionary history of the endemic South American ‘ungulates’. Proc. R. Soc. B. 282:20142671. doi: 10.1098/rspb.2014.2671
Candela, A. M., Bonini, R. A., and Noriega, J. I. (2012). First continental vertebrates from the marine Paraná Formation (Late Miocene, Mesopotamia, Argentina): chronology, biogeography, and paleoenvironments. Geobios 45, 515–526. doi: 10.1016/j.geobios.2012.05.003
Chimento, N. R., and Agnolin, F. L. (2020). Phylogenetic tree of Litopterna and Perissodactyla indicates a complex early history of hoofed mammals. Sci. Rep. 10:13280.
Cifelli, R. L. (1983). The origin and affinities of the South American condylarthra and early tertiary Litopterna (Mammalia). Am. Mus. Novit. 2772, 1–49.
Cifelli, R. L. (1993). “The phylogeny of the native South American ungulates,” in Mammal Phylogeny, eds F. S. Szalay, M. J. Novacek, and M. C. McKenna (New York, NY: Springer-Verlag), 195–216.
Cozzuol, M. A. (2006). The Acre vertebrate fauna: age, diversity, and geography. J. S. Am. Earth Sci. 21, 185–203. doi: 10.1016/j.jsames.2006.03.005
Croft, D. A. (2016). Horned Armadillos and Rafting Monkeys: the Fascinating Fossil Mammals of South America (Life of the Past). Bloomington: Indiana University Press.
Darwin, C. (1845). Journal of Researches into the Natural History and Geology of the Countries Visited During the Voyage of H.M.S. Beagle Round the World. London: John Murray.
de Muizon, C., and Cifelli, R. L. (2000). The “condylarths” (archaic Ungulata, Mammalia) from the early Palaeocene of Tiupampa (Bolivia): implications on the origin of the South American ungulates. Geodiversitas 22, 47–150.
de Muizon, C., Billet, G., and Ladevèze, S. (2019). New remains of kollpaniine “condylarths” (Panameriungulata) from the early Palaeocene of Bolivia shed light on hypocone origins and molar proportions among ungulate-like placentals. Geodiversitas 41, 841–874. doi: 10.5252/geodiversitas2019v41a25
de Muizon, C., Billet, G., Argot, C., Ladevèze, S., and Goussard, F. (2015). Alcidedorbignya inopinata, a basal pantodont (Placentalia, Mammalia) from the early Palaeocene of Bolivia: anatomy, phylogeny and palaeobiology. Geodiversitas 37, 397–634. doi: 10.5252/g2015n4a1
de Vivo, M., and Carmignotto, A. P. (2004). Holocene vegetation change and the mammal faunas of South America and Africa. J. Biogeogr. 31, 943–957. doi: 10.1111/j.1365-2699.2004.01068.x
Delsuc, F., Kuch, M., Gibb, G. C., Karpinski, E., Hackenberger, D., Szpak, P., et al. (2019). Ancient mitogenomes reveal the evolutionary history and biogeography of sloths. Curr. Biol. 29, 2031–2042.e6.
Erdal, O., Antoine, P., and Sen, S. (2016). New material of Palaeoamasia kansui (Embrithopoda, Mammalia) from the Eocene of Turkey and a phylogenetic analysis of Embrithopoda at the species level. Palaeontology 59, 631–655. doi: 10.1111/pala.12247
Ezcurra, M. D., and Agnolin, F. L. (2012). A new global palaeobiogeographical model for the late mesozoic and early tertiary. Syst. Biol. 61, 553–566. doi: 10.1093/sysbio/syr115
Flynn, J. J. (1991). Hyopsodus (Mammalia) from the Tepee trail formation (Eocene), Northwestern Wyoming. Am. Mus. Novit. 3007, 1–19.
Flynn, J. J., and Wyss, A. R. (1988). Recent advances in South American mammalian paleontology. Trends Ecol. Evol. 13, 449–454. doi: 10.1016/s0169-5347(98)01457-8
Gasparini, G. M., Parisi-Dutra, R., Perini, F. A., Croft, D. A., Cozzuol, M. A., Missagia, R. V., et al. (2021). On the supposed presence of miocene tayassuidae and dromomerycinae (Mammalia, Cetartiodactyla) in South America. Bull. Am. Mus. Nat. Hist. 3968, 1–27.
Gelfo, J. N. (2007). The ‘Condylarth’ Raulvaccia peligrensis (Mammalia: Didolodontidae) from the Paleocene of Patagonia, Argentina. J. Vertebr. Paleontol. 27, 651–660. doi: 10.1671/0272-4634(2007)27[651:tcrpmd]2.0.co;2
Gelfo, J. N., Goin, F. J., Woodburne, M. O., and Muizon, C. (2009). Biochronological relationships of the earliest South American Paleogene Mammalian Faunas. Palaeontology 52, 251–269. doi: 10.1111/j.1475-4983.2008.00835.x
Gelfo, J. N., Lopes, G. M., and Bond, M. (2008). A New Xenungulata (Mammalia) from the Paleocene of Patagonia. Argentina. J. Paleontol. 82, 329–335. doi: 10.1666/06-099.1
Gelfo, J. N., Mörs, T., Lorente, M., Lopez, G. M., and Reguero, M. (2015). The oldest mammals from Antarctica, early Eocene of the La Meseta formation, Seymour Island. Palaeontology 58, 101–110.
Gheerbrant, E., Filippo, A., and Schmitt, A. (2016). Convergence of Afrotherian and Laurasiatherian ungulate-like mammals: first morphological evidence from the Paleocene of Morocco. PLoS One 11:e0157556. doi: 10.1371/journal.pone.0157556
Gheerbrant, E., Schmitt, A., and Kocsis, L. (2018). Early African fossils elucidate the origin of Embrithopod mammals. Curr. Biol. 28, 2167–2173. doi: 10.1016/j.cub.2018.05.032
Gingerich, P. D., Domning, D. P., Blane, C. E., and Uhen, M. D. (1994). Cranial morphology of Protosiren fraasi (Mammalia, Sirenia) from the middle Eocene of Egypt: a new study using computed tomography. Contrib. Mus. Paleontol. Univ. Mich. 29, 41–67.
Goin, F. J., Case, J. A., Woodburne, M. O., Vizcaino, S. F., and Reguero, M. A. (1999). New discoveries of “Opposum-like” Marsupials from Antarctica (Seymour Island, Medial Eocene). J. Mammal. Evol. 6, 335–365.
Goloboff, P. A., Farris, J. S., and Nixon, K. C. (2008). TNT, a free program for phylogenetic analysis. Cladistics 24, 1–13.
Hellmund, M. (2016). Tooth emergence and replacement in the European Hyrachyus minimus (Fischer, 1829) (Mammalia, Perissodactyla) from the Geiseltal Fossillagerstätte - a further example for ‘Schultz’s rule’ in ungulates. Neues Jahrb. Geol. Palaontol. Abh. 282, 157–180. doi: 10.1127/njgpa/2016/0611
Janis, C. (2008). “An evolutionary history of browsing and grazing ungulates,” in The Ecology of Browsing and Grazing, eds I. J. Gordon and H. H. T. Prins (Berlin: Springer).
Kramarz, A. G., and Bond, M. (2009). A new Oligocene astrapothere (Mammalia, Meridiungulata) from Patagonia and a new appraisal of astrapothere phylogeny. J. Syst. Palaeontol. 7, 117–128. doi: 10.1017/s147720190800268x
Kramarz, A. G., and Bond, M. (2014). Reconstruction of the dentition of Propyrotherium saxeum Ameghino, 1901 (Mammalia, Pyrotheria): taxonomic and phylogenetic implications. J. Vertebr. Paleontol. 34, 434–443. doi: 10.1080/02724634.2013.799070
Kramarz, A. G., Bond, M., and Carlini, A. A. (2019). Astrapotheres from Cañadón Vaca, middle Eocene of central Patagonia. New insights on diversity, anatomy, and early evolution of Astrapotheria. Palaeo. Elec. 22.2.52A, 1–22. doi: 10.26879/986
Kramarz, A. G., Bond, M., and Forasiepi, A. M. (2011). New remains of Astraponotus (Mammalia, Astrapotheria) and considerations on Astrapothere cranial evolution. Palaeontol. Zeitschrift 85, 185–200. doi: 10.1007/s12542-010-0087-4
Kramarz, A. G., Bond, M., and Rougier, G. W. (2017). Re-description of the auditory region of the putative basal Astrapothere (Mammalia) Eoastrapostylops riolorense Soria and Powell, 1981. Syst. Phylogenet. Considerations Ann. Carnegie Mus. 84, 95–164. doi: 10.2992/007.084.0204
Kumar, K., Rose, K. D., Rana, R. S., Singh, L., Smith, T., and Sahni, A. (2010). Early eocene artiodactyls (Mammalia) from Western India. J. Vertebr. Paleontol. 30, 1245–1274. doi: 10.1080/02724634.2010.483605
Livermore, R., Eagles, G., Morris, P., and Maldonado, A. (2004). Shackleton fracture zone: no barrier to early circumpolar ocean circulation. Geology 32, 797–800. doi: 10.1130/g20537.1
López-Torres, S., Selig, K. R., Prufrock, K. A., Lin, D., and Silcox, M. T. (2018). Dental topographic analysis of paromomyid (Plesiadapiformes, Primates) cheek teeth: more than 15 million years of changing surfaces and shifting ecologies. Hist. Biol. 30, 76–88. doi: 10.1080/08912963.2017.1289378
MacPhee, R. D. E., Del Pino, S. H., Kramarz, A., Forasiepi, A. M., Bond, M., and Sulser, B. (2021). Cranial morphology and phylogenetic relationships of Trigonostylops wortmani, an eocene South American native ungulate. Bull. Am. Mus. Nat. Hist. 449, 1–183. doi: 10.11646/zootaxa.1213.1.1
Madden, R. H. (2014). Hypsodonty in Mammals: Evolution, Geomorphology, and the Role of Earth Surface Processes. Cambridge: Cambridge University Press.
Marshall, L. G., Sempere, T., and Butler, R. F. (1997). Chronostratigraphy of the mammal-bearing Paleocene of South America. J. South Am. Earth Sci. 10, 49–70. doi: 10.1016/s0895-9811(97)00005-9
McKenna, M. C. (1975). “Toward a phylogenetic classification of the mammalia,” in Phylogeny of the Primates: a Multidisciplinary Approach, eds W. P. Luckett and F. S. Szalay (New York, NY: Plenum Press).
Meng, J., and Wyss, A. R. (2001). The morphology of Tribosphenomys (Rodentiaformes, Mammalia): phylogenetic implications for basal glires. J. Mammal. Evol. 8, 1–71. doi: 10.1206/0003-0090(2003)275<0001:toormg>2.0.co;2
Meredith, R. W., Krajewski, C., Westerman, M., and Springer, M. S. (2009). Relationships and divergence times among the orders and families of Marsupialia. papers on geology, vertebrate paleontology, and biostratigraphy in honor of michael O. Woodburne. Mus. North. Ariz. Bull. 65, 383–406.
Mothé, D., and Avilla, L. S. (2015). Mythbusting evolutionary issues on South American gomphotheriidae (Mammalia: Proboscidea). Quat. Sci. Rev. 110, 23–35. doi: 10.1016/j.quascirev.2014.12.013
Murphy, W. J., Eizirik, E., O’Brien, S. J., Madsen, O., Scally, M., Douady, C. J., et al. (2001). Resolution of the early placental mammal radiation using Bayesian phylogenetics. Science 294, 2348–2351. doi: 10.1126/science.1067179
Nishihara, H., Maruyama, S., and Okada, N. (2009). Retroposon analysis and recent geological data suggest near-simultaneous divergence of the three superorders of mammals. PNAS 106, 5235–5240. doi: 10.1073/pnas.0809297106
O’Leary, M. A., Bloch, J. I., Flynn, J. J., Gaudin, T. J., Giallombardo, A., Giannini, N. P., et al. (2013). The placental mammal ancestor and the Post-K-Pg radiation of placentals. Science 339, 662–667. doi: 10.1126/science.1229237
O’Leary, M. A., Roberts, E. M., Bouare, M., Sissoko, F., and Tapanila, L. (2006). Malian Paenungulata (Mammalian: Placentalia): new African afrotheres from the early Eocene. J. Vertebr. Paleontol. 26, 981–988. doi: 10.1671/0272-4634(2006)26[981:mpmpna]2.0.co;2
Owen, R. (1846). “Notices of some fossil Mammalia of South America,” in Proceedings of the Report of the 16th meeting of the British Association for the Advancements of Science, (London: British Science Association).
Owen, R. (1853). Description of some species of the extinct genus Nesodon, with remarks on the primary group (Toxodontia) of hoofed quadrupeds, to which that genus is referable. Philos. Trans. R. Soc. 143, 291–310. doi: 10.1098/rstl.1853.0011
Pardini, A. T., O’Brien, P. C. M., Fu, B., Bonde, R. K., Elder, F. F. B., Ferguson-Smith, M. A., et al. (2007). Chromosome painting among Proboscidea, Hyracoidea and Sirenia: support for Paenungulata (Afrotheria, Mammalia) but not Tethytheria. Proc. R. Soc. 274, 1333–1340. doi: 10.1098/rspb.2007.0088
Pascual, R., Goin, F. J., Balarino, L., and Udrizar-Sauthier, D. E. (2002). New data on the Paleocene monotreme Monotrematum sudamericanum, and the convergent evolution of triangulate molars. Acta Palaeon. Pol. 47, 487–492.
Paula-Couto, C. (1952). Fossil mammals from the beginning of the Cenozoic in Brazil. Bull. Am. Mus. Nat. Hist. 99, 355–394.
Reguero, M., Goin, F. J., Acosta-Hospitaleche, C., Dutra, T., and Marenssi, S. (2013). Late Cretaceous/Paleogene West Antarctica Terrestrial Biota and its Intercontinental Affinities. Heidelberg: Springer.
Rizzi, E., Lari, M., Gigli, E., De Bellis, G., and Caramelli, D. (2012). Ancient DNA studies: new perspectives on old samples. Genet. Sel. Evol. 44, 1–19. doi: 10.1007/978-981-15-1614-6_11-1
Salas, R., Sánchez, J., and Chacaltana, C. (2006). A new pre-deseadan pyrothere (Mammalia) from northern Peru and the wear facets of molariform teeth of Pyrotheria. J. Vertebr. Paleontol. 26, 760–769. doi: 10.1671/0272-4634(2006)26[760:anppmf]2.0.co;2
Sandersab, W. J., Nemecc, W., Aldinuccid, M., Janbue, N. E., and Ghinassif, M. (2014). Latest evidence of Palaeoamasia (Mammalia, Embrithopoda) in Turkish Anatolia. J. Vertebr. Paleontol. 34, 1155–1164. doi: 10.1080/02724634.2014.850430
Scott, C. S., Fox, R. C., and Redman, C. M. (2016). A new species of the basal plesiadapiform Purgatorius (Mammalia, Primates) from the early Paleocene Ravenscrag Formation, Cypress Hills southwest Saskatchewan, Canada: further taxonomic and dietary diversity in the earliest primates. Can. J. Earth Sci. 53, 343–354. doi: 10.1139/cjes-2015-0238
Seiffert, E. R. (2010). The oldest and youngest records of afrosoricid placentals from the Fayum depression of northern Egypt. Acta Palaeon. Pol. 55, 599–616. doi: 10.4202/app.2010.0023
Seiffert, E. R., and Simons, E. L. (2000). Widanelfarasia, a diminutive placental from the Late Eocene of Egypt. PNAS 97, 2646–2651. doi: 10.1073/pnas.040549797
Simmons, N. B., and Geisler, J. H. (1998). Phylogenetic relationships of Icaronycteris, Archaeonycteris, Hassianycteris, and Palaeochiropteryx to extant bat lineages, with comments on the evolution of echolocation and foraging strategies in Microchiroptera. Bull. Am. Mus. Nat. Hist. 235, 1–181.
Simons, E. L., Holroyd, P. A., and Bown, T. M. (1991). Early Tertiary elephant-shrews from Egypt and the origin of the Macroscelidea. Proc. Natl. Acad. Sci. U. S. A. 88, 9734–9737. doi: 10.1073/pnas.88.21.9734
Simpson, G. G. (1945). The principles of classification and a classification of mammals. Bull. Am. Mus. Nat. Hist. 85, 1–350.
Simpson, G. G. (1948). The beginning of the age of mammals in South America. Bull. Am. Mus. Nat. Hist. 91, 1–232. doi: 10.1201/9781003079200-1
Simpson, G. G. (1980). Splendid Isolation: the Curious History of South American Mammals. New Haven: Yale University Press.
Sinclair, W. J. (1909). “Typotheria of the Santa Cruz beds,” in Reports of the Princeton University Expedition to Patagonia 1896–1899, ed. W. B. Scott (USA: Creative Media Partners).
Song, S., Liub, L., Edwards, S. V., and Wub, S. (2012). Resolving conflict in eutherian mammal phylogeny using phylogenomics and the multispecies coalescent model. Proc. Natl. Acad. Sci. U. S. A. 109, 14942–14947. doi: 10.1073/pnas.1211733109
Soria, M. F. (1988). Estudios sobre los Astrapotheria (Mammalia) del Paleoceno y Eoceno. parte II: Filogenia, origen y relaciones. Ameghiniana 25, 47–59.
Springer, M. S., Cleven, G. C., Madsen, O., de Jong, W. W., Waddell, V. G., Amrine, H. M., et al. (1997). Endemic African mammals shake the phylogenetic tree. Nature 388, 61–64. doi: 10.1038/40386
Springer, M. S., Murphy, W. J., Eizirik, E., and O’Brien, S. J. (2003). Placental mammal diversification and the Cretaceous-Tertiary boundary. Proc. Natl Acad. Sci. U. S. A. 100, 1056–1061. doi: 10.1073/pnas.0334222100
Springer, M. S., Stanhope, M. J., Madsen, O., and de Jong, W. W. (2004). Molecules consolidate the placental mammal tree. Trends Ecol. Evol. 19, 430–438. doi: 10.1016/j.tree.2004.05.006
Stanhope, M. J., Waddell, V. G., Madsen, O., de Jong, W., Hedges, S. B., Clevem, G. C., et al. (1998). Molecular evidence for multiple origins of Insectivora and for a new order of endemic African insectivore mammals. Proc. Natl. Acad. Sci. U. S. A. 95, 9967–9972. doi: 10.1073/pnas.95.17.9967
Stromer, E. (1926). “Reste land-und süsswasser-bewohnender Wirbeltiere aus den Diamantfelden Deutsch-Südwestafrikas,” in Die Diamantenwüste Südwestafrikas, ed. E. Kaiser (Berlin: D. Reimer), 107–153.
Tabuce, R. (2016). A mandible of the hyracoid mammal Titanohyrax andrewsi in the collections of the Muséum National d’Histoire Naturelle, Paris (France) with a reassessment of the species. Palaeovertebrata 40, 1–12. doi: 10.11646/zootaxa.635.1.1
Tabuce, R. (2018). New remains of Chambius kasserinensis from the Eocene of Tunisia and evaluation of proposed affinities for Macroscelidea (Mammalia, Afrotheria). Hist. Biol. 30, 251–266. doi: 10.1080/08912963.2017.1297433
Tabuce, R., Asher, R. J., and Lehmann, L. (2008). Afrotherian mammals: a review of current data. Mammalia 72, 2–14.
Tabuce, R., Mahboubi, M., and Sudre, J. (2001). Reassessment of the algerian Eocene hyracoid Microhyrax. consequences on the early diversity and basal phylogeny of the order Hyracoidea (Mammalia). Eclogae Geol. Helv. 94, 537–545.
Tabuce, R., Marivaux, L., Adaci, M., Bensalah, M., Hartenberger, J., Mahboubi, M., et al. (2007). Early Tertiary mammals from North Africa reinforce the molecular Afrotheria clade. Proc. R. Soc. 274, 1159–1166. doi: 10.1098/rspb.2006.0229
Tarquini, J., Morgan, C. C., Toledo, N., and Soibelzon, L. H. (2019). Comparative osteology and functional morphology of the forelimb of Cyonasua (Mammalia, Procyonidae), the first South American carnivoran. J. Morphol. 280, 446–470. doi: 10.1002/jmor.20956
Vallejo-Pareja, M. C., Carrillo, J. D., Moreno-Bernal, J. W., Pardo-Jaramillo, M., Rodriguez-Gonzalez, D. F., and Muñoz-Duran, J. (2015). Hilarcotherium castanedaii, gen. et sp. nov. a new Miocene astrapothere (Mammalia, Astrapotheriidae) from Upper Magdalena Valley, Colombia. J. Vertebr. Paleontol. 35:e903960. doi: 10.1080/02724634.2014.903960
van der Valk, T., Pečnerová, P., Díez-del-Molino, D., Bergström, A., Oppenheimer, J., Hartmann, S., et al. (2021). Million-year-old DNA sheds light on the genomic history of mammoths. Nature 591, 265–269. doi: 10.1038/s41586-021-03224-9
Van Valen, L. (1988). Paleocene dinosaurs or Cretaceous ungulates in South America? Evol. Monog. 10, 1–79.
Van Valen, L. (2004). Adaptation and the origin of rodents. Bull. Am. Mus. Nat. Hist. 285, 110–119. doi: 10.1206/0003-0090(2004)285<0110:c>2.0.co;2
Wake, D. B. (1991). Homoplasy: the result of natural selection, or evidence of design limitations? Am. Nat. 138, 543–567. doi: 10.1086/285234
Welker, F., Collins, M. J., Thomas, J. A., Wadsley, M., Brace, S., Cappellini, E., et al. (2015). Ancient proteins resolve the evolutionary history of Darwin’s South American ungulates. Nature 522, 81–84. doi: 10.1038/nature14249
Wesselingh, F. P., Räsänen, M. E., Irion, G., Vonhof, H. B., Kaandorp, R., Renema, W., et al. (2002). Lake Pebas: a palaeoecological reconstruction of a Miocene, long-lived lake complex in western Amazonia. Cainozoic Res. 1, 35–81.
Westbury, M., Baleka, S., Barlow, A., Hartmann, S., Paijmans, J. L. A., Kramarz, A., et al. (2017). A mitogenomic timetree for Darwin’s enigmatic South American mammal Macrauchenia patachonica. Nat. Commun. 8:15951.
Williamson, T. E., and Weil, A. (2011). A new Puercan (early Paleocene) hyopsodontid “condylarth” from New Mexico. Acta Palaeon. Pol. 56, 247–255. doi: 10.4202/app.2009.0147
Woodburne, M. O. (2010). The Great American Biotic Interchange: dispersals, tectonics, climate, sea level and holding pens. J. Mammal. Evol. 17, 245–264.
Woodburne, M. O., and Zinsmeister, W. J. (1984). The first land mammal from Antarctica and its biogeographic implications. J. Paleontol. 54, 913–948.
Keywords: Atlantogea, Eutheria, evolution, Mammalia, Meridiungulata, Paenungulata, Paleogene, phylogeny
Citation: Avilla LS and Mothé D (2021) Out of Africa: A New Afrotheria Lineage Rises From Extinct South American Mammals. Front. Ecol. Evol. 9:654302. doi: 10.3389/fevo.2021.654302
Received: 15 January 2021; Accepted: 07 June 2021;
Published: 05 July 2021.
Edited by:
Martin Daniel Ezcurra, Museo Argentino de Ciencias Naturales Bernardino Rivadavia, ArgentinaReviewed by:
Federico Agnolin, Museo Argentino de Ciencias Naturales Bernardino Rivadavia, ArgentinaMariano Bond, Universidad Nacional de La Plata, Argentina
Copyright © 2021 Avilla and Mothé. This is an open-access article distributed under the terms of the Creative Commons Attribution License (CC BY). The use, distribution or reproduction in other forums is permitted, provided the original author(s) and the copyright owner(s) are credited and that the original publication in this journal is cited, in accordance with accepted academic practice. No use, distribution or reproduction is permitted which does not comply with these terms.
*Correspondence: Leonardo S. Avilla, bGVvbmFyZG8uYXZpbGxhQGdtYWlsLmNvbQ==
†These authors have contributed equally to this work and share first authorship