- 1Department of Evolutionary and Environmental Biology, University of Haifa, Haifa, Israel
- 2Department of Biology, Ashoka University, Sonipat, India
- 3Department of Biology and Environment, University of Haifa—Oranim, Haifa, Israel
The importance of pollinators as selective agents for many floral traits is well established, but understanding their role in the evolution of complex floral shapes remains challenging. This is because pollinators often need much practice to efficiently handle morphologically complex flowers and extract their food rewards. What induces foragers to persistently visit and pollinate complex flowers despite their initial low profitability? We previously found that naive bumblebees, and unsuccessful feeding attempts of experienced ones, contribute to the pollination of complex flowers. Here we tested a complementary hypothesis, positing that successful foraging on flowers of one complex shape prepares pollinators to visit other species of different complex morphologies. We trained bumblebees to computer-controlled artificial flowers that were either simple, complex or both simple and complex. We then recorded their feeding choices and handling times on a second array of simple and complex flowers that had different shapes and required another handling technique. Bees trained on a single flower type (whether simple or complex) preferred flowers of the same type in the testing array. The foragers’ preferences after training on both flower types depended on the reward schedule during training: when both flower types rewarded equally, simple flowers were preferred at the test phase; when complex flowers provided higher reward during training, they became the preferred flower type during testing. These results suggest that successful foraging on complex flowers, especially when highly rewarding, can indeed induce insect pollinators to attempt additional flower species with other complex shapes.
Introduction
Animal pollinators act as important selective agents that shape many floral traits, including color, scent, symmetry and blooming phenology (Chittka and Raine, 2006; Schiestl and Johnson, 2013). Pollinators are generally assumed to associate specific floral displays with the food rewards (nectar and/or pollen) that they obtain from the flowers. High-rewarding flowers, advertised by preferred display traits, receive better pollination service than flowers that lack them, causing such preferred displays to increase in frequency in plant populations (Caruso et al., 2019).
This straightforward scenario seems insufficient to explain the evolution of flowers with complex morphologies, in which food rewards are hidden in long constrained tubes, tunnels or spurs. Such flowers are common in numerous plant families, for example Fabaceae, Orchidaceae, and Lamiaceae (Krishna and Keasar, 2018). In other plants, the complexity of flower handling involves finding the high-rewarding nectaries out of several available ones (as in some mustards) or sonicating the anthers to access their pollen (as in several Solanaceae species). Some of the existing studies suggest that morphological complexity and pollinator specialization are related. For example, simple dish-shaped flowers are visited by a wider range of pollinator taxa than complex flag-shaped flowers (reviewed in Keasar, 2020). Nectar production often increases with flower complexity, while competition with generalist foragers decreases. Specialization on complex flowers is thus considered a profitable foraging strategy (reviewed in Krishna and Keasar, 2018). Nevertheless, “complexity” is difficult to measure experimentally, therefore empirical assessment of the function relating floral complexity and rewards available to pollinators is scarce.
Inexperienced pollinators often need much time to get at the rewards of complex flowers, and sometimes fail to feed from them altogether. Many repeated attempts are needed to learn efficient motor routines for pollen (Raine and Chittka, 2007) and nectar (Laverty, 1994) extraction. Thus, complex flowers can be highly rewarding for the subset of pollinators that have mastered their handling technique and experience less competition on them than on simple flowers. However, flowers that are difficult to handle are often poor resources for inexperienced individuals. For complex flowers to reproduce and maintain stable populations in plant communities, pollinators need to persist on them despite their initial low profitability. Current models of operant conditioning in invertebrates focus on incremental reinforcement of behaviors that are rewarding in the short-term (Menzel and Benjamin, 2013). However, standard learning theory does not sufficiently address learning of motor tasks that are initially low-rewarding, but become profitable after long practice, such as handling of complex flowers. What behavioral mechanisms might explain the persistence of bees on such tasks?
In a previous study, we presented bumblebees with flowers of three species that varied in morphological complexity and were either intact or experimentally simplified (Krishna and Keasar, 2019). We identified two foraging patterns used by the bees, which could serve the evolutionary interests of complex flowers. First, flower-naive bees mildly but consistently preferred complex flowers to simplified ones. Second, unsuccessful feeding attempts by experienced bees were common, especially to the intact flowers of the most complex species. Such exploratory attempts, although non-rewarding for bees, can nevertheless provide pollination services to plant species with complex flowers.
A complementary mechanism that could potentially further enhance pollinator visits to complex flowers involves previous successful experience with other complex species. Previous studies showed that bees tend to repeat flower-handling motor routines that led them to successful foraging, and that this preference affects their foraging choices (Laverty, 1994; Dukas, 1995). Bumblebees that foraged in mixed patches of two or three flower species were usually flower-constant, i.e., visited a single flower species for many consecutive visits. When switches between species did occur, they were mostly between flowers that required similar handling techniques (Ishii and Kadoya, 2016). Bumblebees that were trained to artificial flowers, accessible either from the bottom or from the top of their corollas, retained the acquired handling method when they could access those flowers by both techniques (Barker et al., 2018). Such “tactic-constancy” was proposed to improve foraging efficiency, since the time required for flower handling decreases as its handling technique becomes routine (Bronstein et al., 2017). Most of these earlier studies have focused on either flower handling behavior and their associated costs or on transfer of motor skills. Here, placing both these together and expanding on these findings, we hypothesized that learning to handle complex flowers may involve motor skills that can be later transferred and applied to other species with different shapes. Such a mechanism would make complex flowers attractive to foragers, after they had previously foraged on other complex species. To test the hypothesis, we trained bumblebees to artificial flowers that were either simple, complex or both simple and complex. We next tested the foragers’ choices in a flower patch with equal numbers of simple and complex morphologies that required a different handling technique from the training flowers. We addressed the following questions: (a) Are the bees’ overall foraging choices, feeding success and foraging costs (handling time) in the experiment’s test phase affected by the training that they had received? (b) How do these change with experience of the bees along the experiment’s test phase?
We hypothesized that the foraging choices would be strongly influenced by the training type. Specifically, we predicted that training to simple flowers would increase the bees’ visit frequencies and feeding success on other simple flowers in the test phase, whereas training to complex flowers would induce more test-phase visits to complex flowers. When trained to both the flower types with equal rewards, we predicted that bees would equally visit both flowers. In the above scenarios, based on previous findings with real (Krishna and Keasar, 2019) and artificial (Barker et al., 2018) flowers, we also expected bees to visit simple artificial flowers increasingly as they gain experience in the testing array, regardless of their training. To explore the interactive effect of food rewards and foraging experience on choices of complex morphologies, we also trained foragers to complex flowers that were paired with higher rewards than simple flowers. We expected that more of these bees would specialize on complex flowers in the test phase than after training on equally rewarding complex and simple flowers.
Materials and Methods
Four Bombus terrestris colonies were obtained from BioBee Ltd. (Sde Eliyahu, Israel). Colonies were housed in a 2.90 × 3.60 m flight room, which was maintained at a temperature range of 26–29°C and a relative humidity range of 40–60%. The room was illuminated by D-65 lights between 0600 and 1,800 h. The colony was placed at one end of the room with a plexiglass corridor attached to its entrance, allowing us to control the exit of foragers from the colony. Pollen was provided directly to the colony every day. Flower-naive bees were pre-trained to a transparent feeder (Petri dish) filled with 30% sucrose solution (w/w) and placed on the experimental table. Foragers were marked with numbered tags after they learnt to visit the feeder regularly. For experiments, we used robotic flowers that were developed in the lab. The automated flower system gave us the flexibility to track the bees’ visit sequences and durations to numerous flowers, and to precisely control the floral rewards.
Artificial Flower System
Thirty-two artificial flowers were used in the experiments. The dimensions and basic design of the flowers are described in detail in Keasar (2000). They contained an infrared (IR) sensor, an electromagnet, a plexiglass reservoir filled with sugar solution, and a plexiglass float with a magnet glued to it. The flowers were computer-controlled by an Arduino Mega 2560, single-board microcontroller platform1, which is based on ATmega2560 processor chip. An external power supply was used to provide direct current to all flowers in the system. The program running on the microcontroller handled the electronics in the system: it read infrared sensors and generated signals to push the electromagnet down and up for refilling (Supplementary Movie 1). The display color of all the flowers used in the experiments was blue, to eliminate potential effects of the bees’ color preferences on their choices. For the same reason, the sugar solution was spiked with the same scent in all flowers. The flowers were placed at least 10 cm apart in both the training and test phases of the experiment (see below). To rule out social learning during the experiment, a single bee foraged on the artificial flowers at a time. After a foraging session, flowers were wiped with ethanol to remove any potential scent marks that might influence the next bee.
Experimental Design
We conducted the experiment in two phases: a training and a test phase. Simple flowers in the training phase had one feeding hole, and complex flowers had three holes, of which only the central one led to reward. This design of the flowers is inspired from some of the flower species (such as mustards, Sinapis spp.) which provide differential rewards in their nectaries and share a common floral morphology. In the test phase, the simple morphology was represented by a flower with single feeding hole accessible through a transparent tunnel. The tunnel was placed such that the morphology was visible through the sides of the tunnel as well as from the top if the bee hovered close to the flowers (Supplementary Figure 1). The complex morphology was represented by a flower with three holes, one of which was a feeding hole, accessible along a similar transparent tunnel as the simple flower. Thus, in the test phase, the bees had to learn a new technique for accessing the flowers that required crawling in the tunnel, and which was different from the handling technique of the training phase. During the training phase, the time taken to access the reward was higher on the complex flowers (28.0 ± 3.7 s) than the simple flowers (15.9 ± 1.8 s, Wilcoxon test: W = 202.5, p = 0.004), suggesting that the complex morphology was indeed the difficult option for the bees to begin with (also see Supplementary Figure 2).
During the training phase, we randomly allocated bees from each of the four colonies to one of four floral arrays: (a) “Simple”: 16 simple flowers (17 bees); (b) “Complex”: 16 complex flowers (15 bees); (c) “SCequal”: equally rewarding simple and complex flowers (50 visits on simple and 50 visits on complex, 20 bees); or (d) “SChigh”: simple and higher-rewarding complex flowers (50 visits on simple and 50 visits on complex, 20 bees). These four floral arrays are referred to as “training types.” Supplementary Table 1 contains further details on the assignment of bees to training types. Each bee was exposed to one training array only. To provide higher reward in the complex flowers in “training type (d),” they were filled with a 50% (w/w) sucrose solution. The simple flowers in this, and all flowers of training types (a–c), dispensed a 40% (w/w) sucrose solution. The training comprised 100 successful (fed) visits on the arrays mentioned above. One half of the bees allocated to (c) and (d) were first trained to simple, then to complex flowers. This was done by concealing the flowers of complex morphology and allowing the bee to complete their 50 visits on simple flowers, and then exposing the complex flowers and concealing the simple ones for 50 more visits. We reversed the training order for the remaining bees. This sequential way of exposing the flower array allowed us to accurately control the amount of experience they have received on each flower type.
In the test phase, bees individually foraged on an array of 16 simple and 16 complex flowers for up to 100 visits (4–5 bouts). All of these flowers rewarded the bees with 1 μl of a 40% sucrose solution on each visit. Bees were allowed to forage freely till they returned to the colony by themselves. Some bees returned to the colony after their first bout and did not attempt to exit again on the same day, thus they completed fewer visits. A scheme of the experimental design is provided in Figure 1.
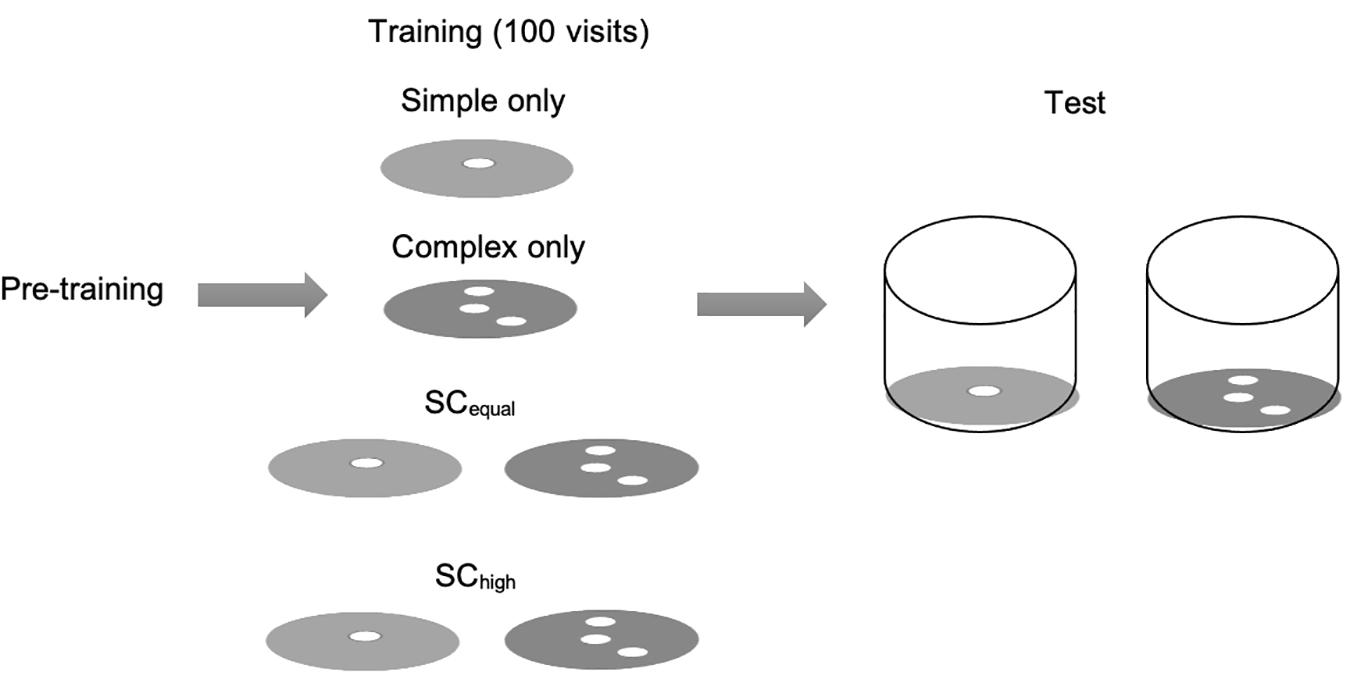
Figure 1. Experimental design and scheme of the artificial flowers. Bees were allocated to one of four training types on artificial flowers with a flat landing surface. In the test phase, all foragers were exposed to a mixture of simple and complex flowers, which could only be accessed by passing through a short tunnel. Floral choices, feeding success and handling times during the test phase were recorded.
In the training phase, we counted only successful visits (i.e., visits that involved sucrose feeding). In the test phase, any landing by a bee on a flower tunnel (where the legs have contacted the flower) was regarded as a choice (or visit). Those visits that resulted in successfully accessing the reward were considered as fed visits. Unsuccessful visits included failures to either locate the rewarding hole or to feed from it. The experiments were video recorded, and the amount of time taken to access the reward (henceforth referred to as “handling time”) was extracted from the videos.
Ethical Note
Bees were kept in the colony that they were brought in and maintained under 12 h L: 12 h D with ambient temperature and humidity conditions. Pollen and sucrose were provided ad libitum to the colony without causing any disturbance. Bees were captured while feeding with a sponge-lined cage and handled with care. Identification tags were attached to the thorax with a drop of non-toxic glue and the bees were carefully released after being marked. The colonies were euthanized by placing in the freezer after all the foragers have died naturally and only drones remained in the colony.
Statistical Analysis
All the analyses were conducted using R statistical software (R version 3.3.2). The test-phase choices of bees that had been trained on both flower types (“SCequal” and “SChigh”), were not affected by the flower presentation order during training (Wilcoxon test: “SCequal”: W = 39, p = 0.43 and “SChigh”: W = 54, p = 0.76), therefore the data of all bees from each training type were pooled for analysis.
We tested whether the training type affected the frequency of visits to the complex flowers using generalized linear mixed effects models (binomial GLMM, lme4 package, Bates et al., 2014). The response variable was flower type (complex/simple) and the fixed effects were training type and visit number during the test phase (experience). Bee and colony identities were used as random effects. The significance of each fixed effect in the model was determined using the function “Anova” (car package, Fox et al., 2012). A similar model was run considering only successful visits. Mean run lengths (consecutive visits to a flower type) were computed for each of the bees. We examined, using a linear mixed effects model, whether the training type and the type of flowers visited in the test array (simple and complex) influenced run lengths. We computed a constancy index (Bateman, 1951) for each forager, which is based on the number of transitions between the same flower type and different flower types (complex and simple). The value of this index ranges from −1 (switching flower type after each visit) to 1 (complete constancy). We used a one-way ANOVA to analyse if the constancy index differed between the training types.
To determine whether the time taken to successfully access the reward (log-transformed handling time) from complex and simple flower types depended on training type, experience (visit number) in the test phase, or their interactive effect, we used a linear mixed effects model with bee and colony identity as random effects.
In all the models, when a significant effect of the predictor variable was found, we ran Tukey’s post hoc tests (R package lsmeans, Lenth, 2016) to determine pairwise differences.
Results
Flower-Type Choices
First Visits
0.35 of the bees that were trained on simple flowers, and 0.73 of those trained on complex flowers, made their first visit to a complex flower in the test phase. Complex flowers were chosen on the first visit of the test phase by 0.45 of the foragers that were trained to equally rewarding simple and complex flowers (“SCequal”), and 0.60 of those trained to both flower types with higher reward in complex flowers (“SChigh”). While none of these proportions deviated significantly from 0.5 (binomial tests, p > 0.05 in all tests), a significantly larger proportion of bees trained on complex flowers made their first visit to a complex flower, compared to bees trained on simple flowers (Complex vs. Simple, χ2 = 4.63, df = 1, p = 0.03).
All Visits
The type of training experienced by the bees affected their choices over the whole test phase (GLMM, training: χ2 = 56.94, df = 3, p < 0.0001). In the “Simple” and “Complex” training types, there was a bias toward visiting the flower type that the bees had been trained on. Training on equally rewarding simple and complex flowers (“SCequal”) resulted in choices that were intermediate between “Simple” and “Complex” training types. After training on both flower types with higher rewards in complex flowers (“SChigh”), bees chose complex flowers as often as individuals from the “Complex” training type (Figure 2A). Most of the visits to both simple and complex flowers resulted in sucrose feeding (Figure 2B). The foragers’ experience during the test phase, alone and in interaction with the training type, did not influence their flower-type choices (Training∗ Experience: χ2 = 3.26, df = 3, p = 0.35, Experience: χ2 = 0.03, df = 1, p = 0.84, Figure 3).
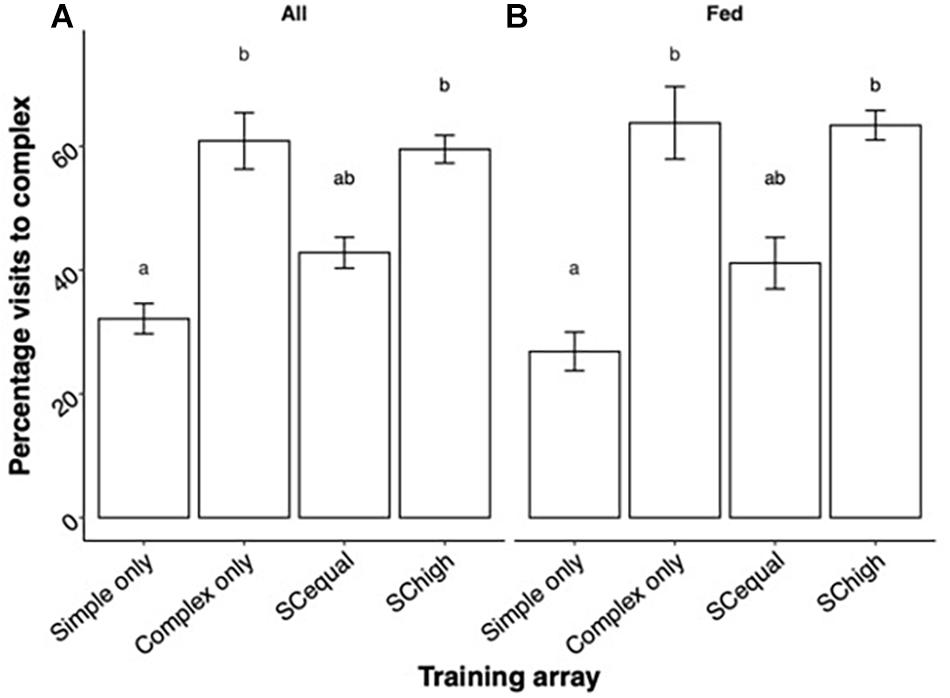
Figure 2. Mean ± SE percent of test-phase visits in complex flowers. (A) Both successful and unsuccessful visits, (B) only successful (fed) visits. Different letters indicate significant differences in Tukey post hoc tests, which are detailed in Supplementary Table 2.
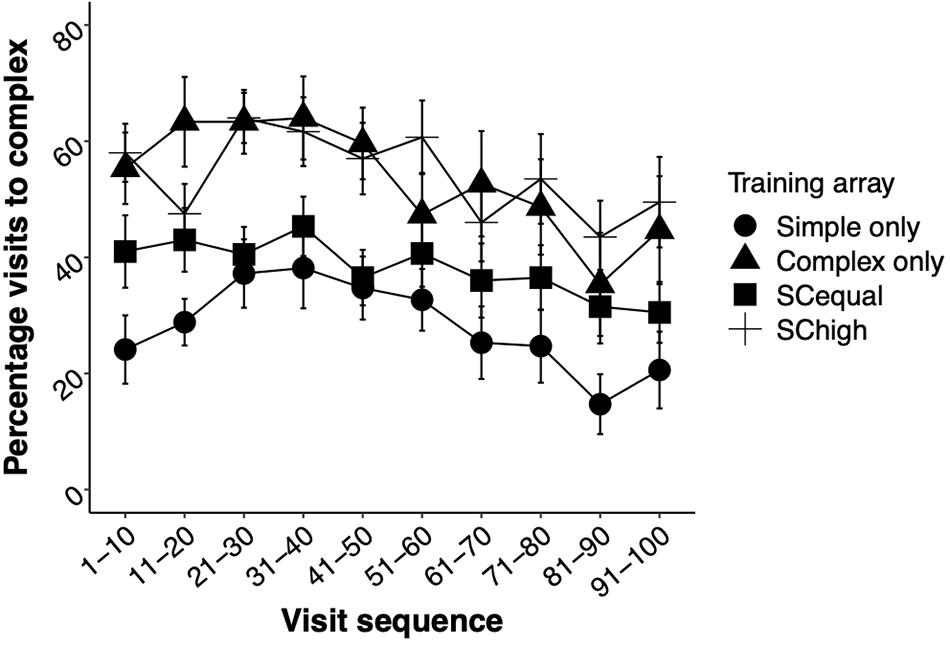
Figure 3. The effects of experience during the test phase on the mean ± SE percentages of complex flowers chosen by the bees. Note that experience (visit number) was analyzed as a continuous variable in the statistical models. In the figure, however, visits are grouped into bins of 10 for graphical clarity.
The bees’ preference for a flower morphology (whether simple or complex) was manifested in longer sequences of consecutive visits (run lengths) to their favored flower type than to the less-preferred type (Figure 4). Thus, training type significantly affected run lengths in the test phase (χ2 = 16.55, df = 3, p < 0.0001). Bees trained to “Simple” and to “SCequal” flowers made shorter runs of visits to complex flowers than bees trained to “Complex” and to “SChigh” flowers (Figure 4).
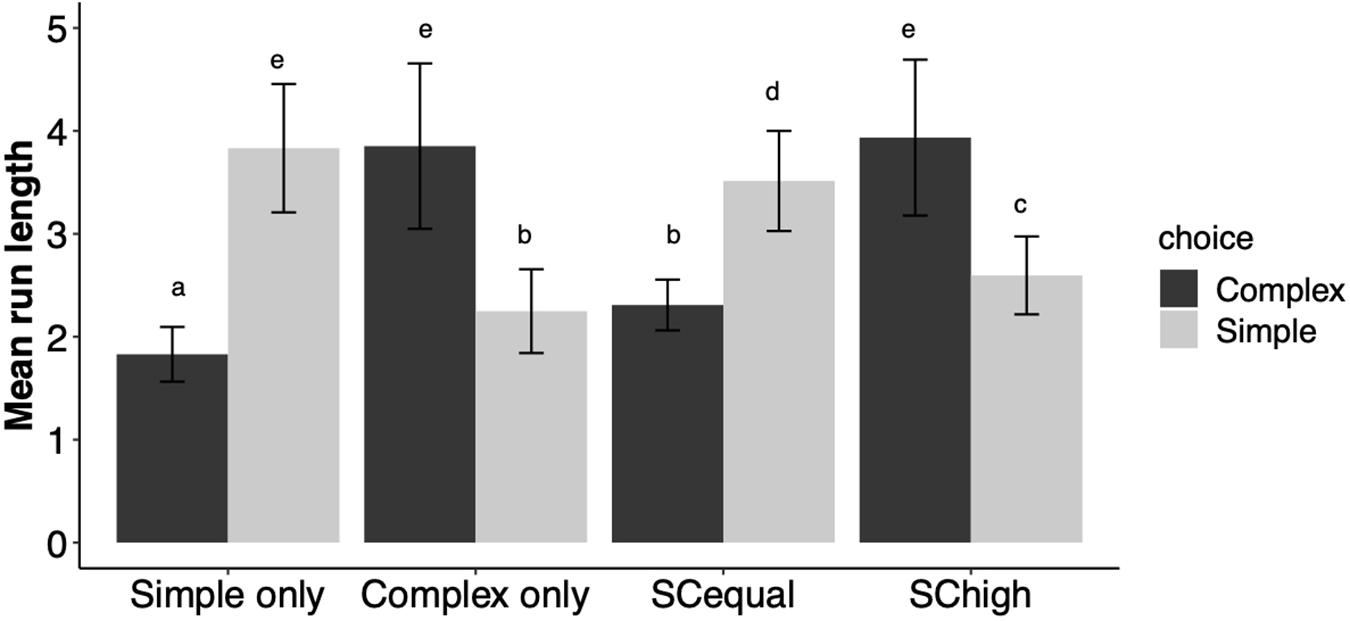
Figure 4. Mean ± SE run lengths (numbers of consecutive visits) to simple and complex flowers during the test phase. Different letters indicate significant differences in Tukey post hoc tests, which are detailed in Supplementary Table 3.
We defined a forager as specialized if it made at least 60% of its visits to one of the flower types (the 60% threshold was set arbitrarily). The proportion of specialized bees varied among experimental treatments, being higher in bees trained on simple flowers than in the other experimental treatments (Chi-square test of independence, χ2 = 16.55, df = 6, p < 0.0001). In this training type, all of the specialized bees foraged mostly on simple flowers. After training on complex flowers only, or on flower types with higher reward in complex (“SChigh”), most of the specialization was on the complex type. Training on equally rewarding simple and complex flowers (“SCequal”) resulted in most specializations on the simple morphology (Figure 5). Barring the bees that were trained on only simple flowers, 0.40 of the bees in all the three training types (“Complex” = 0.46, SCequal = 0.65, SChigh = 0.45) did not specialize on either flower morphology, namely directed 0.41–0.59 of their visits to complex flowers.
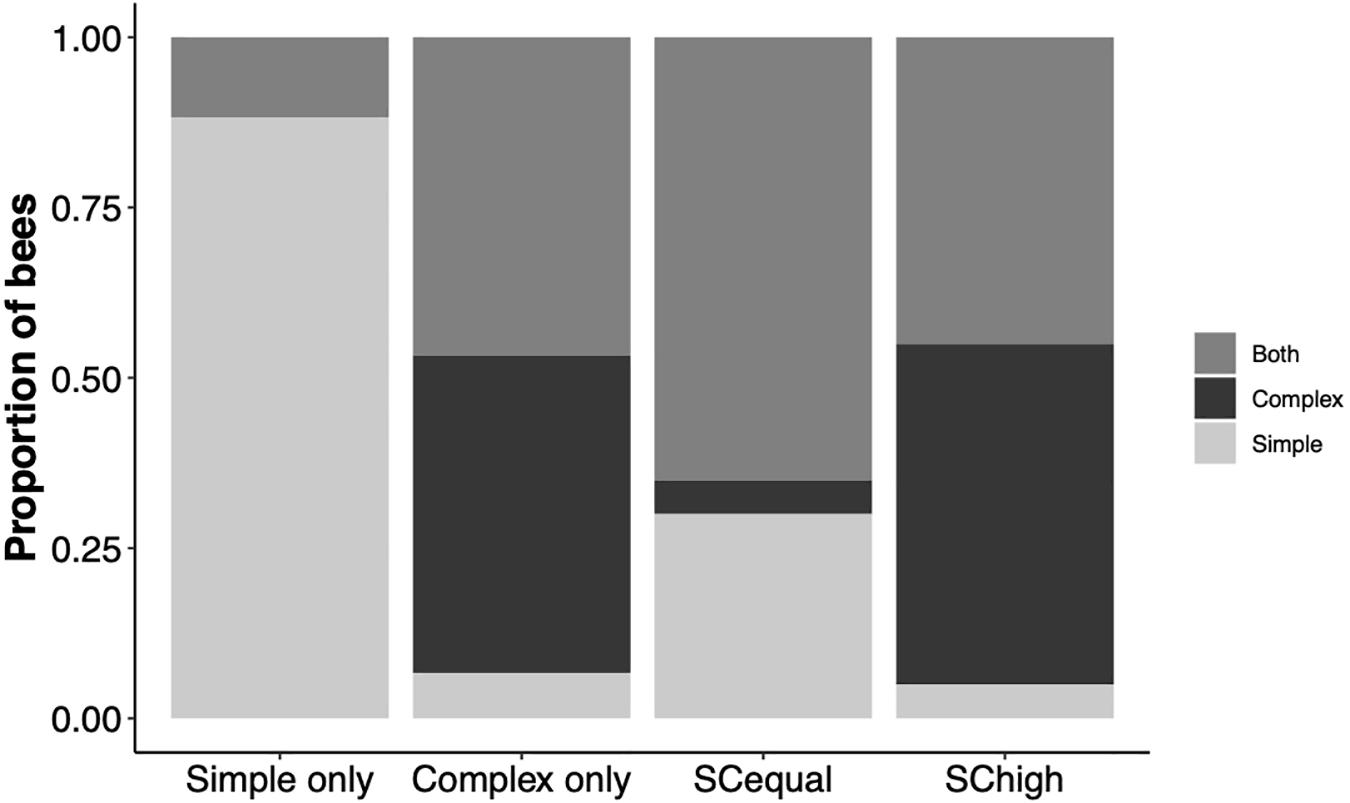
Figure 5. The proportions of bees that specialized on simple flowers, specialized on complex flowers, or showed no flower type specialization during the test phase. Specialized foragers directed > 60% of their visits to one of the flower types.
The mean constancy index of bees trained to better rewards in complex flower types was higher than in the other training types (“SChigh”: 0.31 > “SCequal”: 0.26 > “Simple”: 0.25 > “Complex”: 0.22, Supplementary Figure 3). However, this difference was not statistically significant (>F(3, 68) = 1.05, p = 0.37), as bees within each training type varied widely in the frequencies of transitions between flower types.
Feeding Success
The type of training experienced by the bees influenced their success at accessing the reward during the test phase (GLMM, training: χ2 = 45.83, df = 3, p < 0.0001). Bees that were trained on complex flowers alone, and bees that were trained with higher rewards in complex flowers, had similar success rates, and were more successful at obtaining reward from complex than from simple flowers. The foragers’ experience during the test phase, alone and in interaction with the training type, did not affect their success at flowers (Training∗ Experience: χ2 = 4.05, df = 3, p = 0.25, Experience: χ2 = 2.21, df = 1, p = 0.13, Figure 6 and Supplementary Table 4).
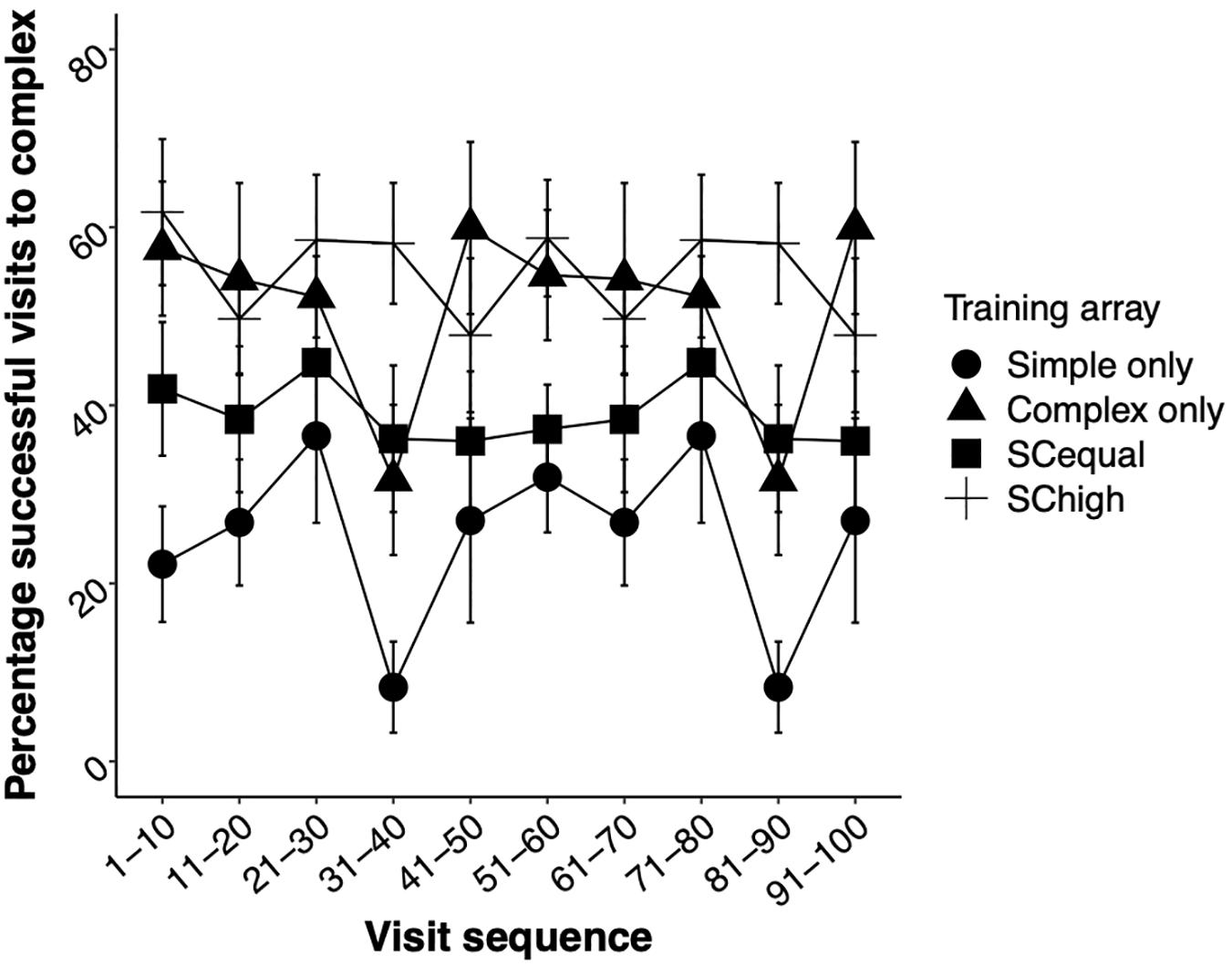
Figure 6. The effects of experience during the test phase on the mean ± SE percentages of successful (fed) visits to complex flowers. As in Figure 3, experience (visit number) was analyzed as a continuous variable in the statistical models but is plotted here in groups of 10 visits for visual clarity.
Handling Time
The time needed to handle the flowers and access the reward successfully declined with experience during the test phase (Experience: χ2 = 577.23.15, df = 1, p < 0.0001, Figure 7). There were no significant differences between handling times of complex and simple flowers and they were similar across the four training types (Supplementary Table 5).
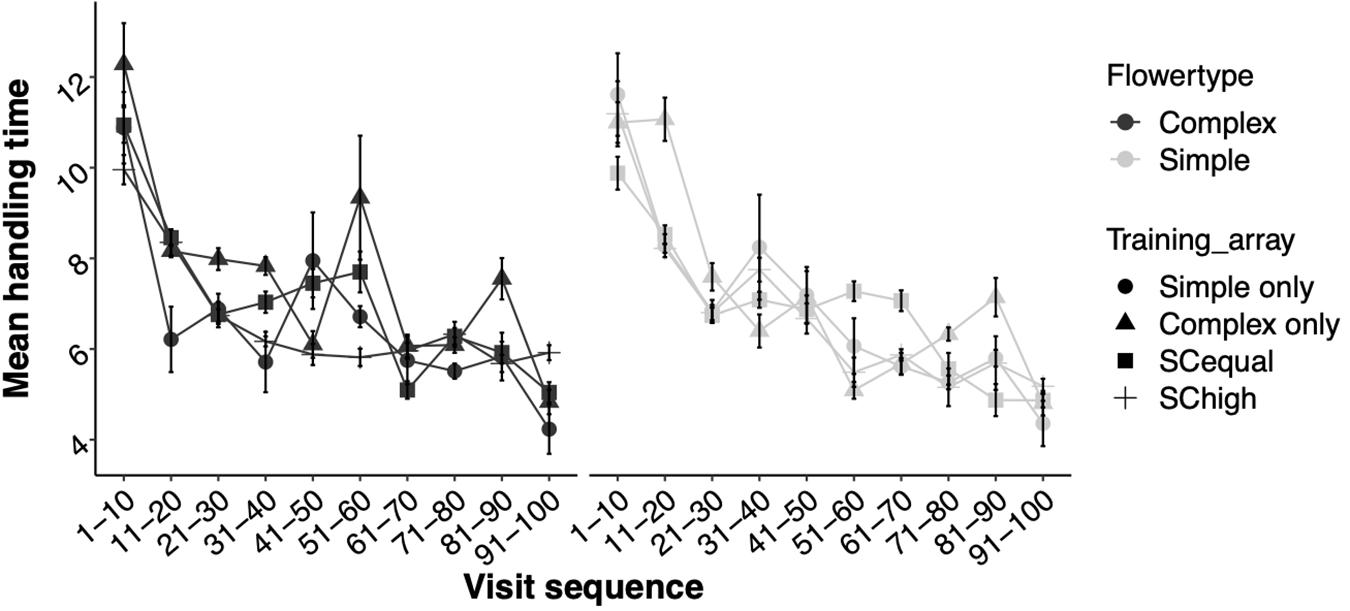
Figure 7. Mean ± SE handling times (in seconds) of complex (black) and simple (gray) flowers throughout the experiment’s test phase. Although aggregated here into 10-visit bins for visual clarity, the handling time of each visit was treated as a data point in the statistical analysis.
Discussion
Training to a single flower-handling method (using either a single hole, or one of three holes, to access a nectar reward) induced bees to apply it to a new foraging task during the test phase of our experiment. Thus, bees trained to complex flowers chose other complex flowers in the test phase more often than bees trained to simple flowers. In bees that were trained to both flower types, the food rewards during training affected the bee’s later choices. Increasing the relative profitability of complex flowers in the training phase caused bees to choose complex flowers more often when tested. These results are in line with our first prediction, that a successful foraging experience on one complex flower species would increase the bees’ preference for other complex species. Moreover, the significance of such foraging experience is also evident from the absence of difference in the proportion of unsuccessful and successful visits to the flowers in the test phase.
We further predicted that the bees’ training-phase experience would affect their choices primarily at the start of the test phase. This prediction derived from our previous experiment with real flowers, where bees gradually reduced their attempts to feed from complex flowers when simplified and equally rewarding complex flowers were available (Krishna and Keasar, 2019). Contrary to this expectation, the bees’ experience in the test phase did not affect their overall flower choices (Figure 3) or feeding success (Figure 6) in the present study. We also found that bees handled simple and complex flowers equally quickly during the test phase (Supplementary Table 5). It thus seems that our complex and simple artificial flowers became similarly profitable to foragers by the end of the training phase, since they provided identical rewards and required similar handling times. Hence no benefit, in terms of foraging success, would have been gained from changing flower choices over the course of the test phase. Furthermore, in our test phase, we considered a bee to visit or make a choice only if it landed. Previous studies have demonstrated that bees sample and discriminate floral features from close distances, thereby making some of the foraging decisions without probing the flowers (Dukas, 2001; Ma et al., 2016). Such rejections without landing possibly explain how bees that had been trained to complex flowers, and that received higher rewards on them, chose complex flowers from the very beginning of the test phase. The similar profitability of both flower types in the test phase differs from our earlier observations on complex real flowers, which required longer handling times than simple ones and offered lower feeding success, even for experienced bees (Krishna and Keasar, 2019). On the other hand, in a previous experiment with simple and complex artificial flowers, experienced bees attained similar handling times in both flower types (Muth et al., 2015).
Our experiment also allows unplanned comparisons between bees trained to a single motor task (“Simple” and “Complex”) and identically rewarded bees exposed to two tasks at training (“SCequal”). Bumblebees readily learnt to perform one of two motor routines (turning either right or left in a Y-maze) in response to a color cue, and retained such learnt associations for several days (Chittka and Thomson, 1997; Chittka, 1998). However, they were slower and more error-prone than bees trained to one of these tasks only. In our experiment, on the other hand, handling times were similar across all training types (Figure 7). The bees’ feeding success in “SCequal” also resembled the average feeding success of “Simple” and “Complex” training types (Figure 2B). Thus, we found no reduction in foraging efficiency in bees that learnt two motor tasks. Possibly, our learning tasks were easier than those posed by Chittka and Thomson (1997) and Chittka (1998), allowing the bees in our study to reach high foraging proficiency even after training on two routines. Note also that the design of our experiment confounds the number of training tasks (one vs. two) with the length of training (100 to a single flower type vs. 50 visits to each of the two flower types).
Barker et al. (2018) trained bumblebees to either a simple (“secondary robbing”) or a more complex (“legitimate”) nectar extraction technique from artificial flowers, and then exposed them to similar flowers that could be exploited by both techniques. As in our experiment, the bees initially preferred the technique to which they had been trained. Our study extends these findings by showing that practice with the complex technique induces preference for a complex flower type that the foragers had not encountered before. These findings are in line with extensive previous demonstrations of bees’ ability to generalize a learnt preference to perceptually similar stimuli (Gerber et al., 1996; Vieira et al., 2018). Most studies on generalization and transfer in bees have focused on learning of olfactory and visual signals in classical conditioning experiments. Our work adds evidence for transfer learning of a complex motor task.
Natural plant communities in bloom comprise multiple species, which vary in flower shape, handling difficulty and food rewards. The frequency of species with complex flowers correlates with the composition of the pollinator community, increasing in habitats that are rich in specialized pollinators such as birds, large bees and lepidopterans. Such habitats include islands, alpine areas and forest canopies (Keasar, 2020). Pollinating insects distribute among the available flower species proportionately to their detectability, accessibility, abundance and profitability (Junker et al., 2013). Yet, how this distribution is achieved in practice, and which behavioral mechanisms shape pairwise species interactions in pollination networks, is not well known (Olito and Fox, 2015). Our experiment suggests that foragers that had learnt to handle a complex flower species may remain specialized to complex floral morphologies in new foraging situations. Similarly, bumblebees showed high constancy to their flower-handling tactics (legitimate visits in some plant species, nectar-robbing in others) in a natural montane plant community (Lichtenberg et al., 2020). Such transfer of preferences could help explain how plants with complex flowers persist in diverse plant communities, even though they have low profitability for naïve pollinators. Likewise, in plant species with poricidal anthers bees have to learn to efficiently buzz in order to extract pollen and they extend this buzzing to species with non-poricidal anthers, such as Pedicularis spp. (Buchmann, 1985). Such a “carry over” phenomenon (Buchmann, 1983) is likely to benefit flowers with non-poricidal anthers that release pollen in response to the buzzing, if they co-occur with species containing poricidal anthers. In our study, the training arrays resembled some common field conditions, such as in a seasonally flowering community where complex flowered species are the first to bloom and communities where complex flowered species provide greater rewards compared to co-flowering simple flowered species (bumblebees are known to frequently sample other species as part of their minoring). Such design allowed us to better understand possible ways by which repeated visits and thereby successful pollination is achieved in complex flowered species. Information on the frequencies of complex flowered species and on their spatial and temporal distributions in communities will help us comprehend handling strategies used by bees at the individual as well as the colony level.
From the pollinators’ point of view, extrapolating from prior experience in complex floral environments is a key skill that can be put to use to improve foraging efficiency. The transfer of preferences documented in our experiment may result in some within-colony foraging specialization under field conditions, with part of the workers visiting mainly simple flowers and others preferring complex ones. This may be an efficient colony-level foraging strategy, as it allows the colony to utilize a wide range of flower species, while enabling some workers to specialize on the high-risk-high-reward complex species. How naïve and experienced workers from a colony evaluate and utilize social cues while handling such complex flowers is intriguing, but this question was beyond the scope of the present experiment. Whether and how colony-level specializations in fact occur in social pollinators is a promising direction for further study.
Data Availability Statement
The datasets presented in this study can be found in online repositories. The names of the repository/repositories and accession number(s) can be found below: https://tamarkeasarlab.weebly.com/data-sets.html.
Author Contributions
TK conceived the study. TK and SK designed the experiments. SK conducted the experiments and statistical analysis. Both authors were involved in writing and editing the manuscript drafts.
Funding
This work was supported by grant #250/16 from the Israel Science Foundation.
Conflict of Interest
The authors declare that the research was conducted in the absence of any commercial or financial relationships that could be construed as a potential conflict of interest.
The reviewer NI declared a past co-authorship with one of the authors SK to the handling editor.
Acknowledgments
We are extremely thankful to Yehuda Agus for the development of artificial flower system used in the study.
Supplementary Material
The Supplementary Material for this article can be found online at: https://www.frontiersin.org/articles/10.3389/fevo.2021.655086/full#supplementary-material
Footnotes
References
Barker, J. L., Dornhaus, A., Bronstein, J. L., and Muth, F. (2018). Learning about larceny: experience can bias bumble bees to rob nectar. Behav. Ecol. Sociobiol. 72:68. doi: 10.1007/s00265-018-2478-6
Bateman, A. J. (1951). The taxonomic discrimination of bees. Heredity 5, 271–278. doi: 10.1038/hdy.1951.24
Bates, D., Mächler, M., Bolker, B., and Walker, S. (2014). Fitting linear mixed-effects models using lme4. J. Statist. Softw. arXiv 1406:5823.
Bronstein, J. L., Barker, J. L., Lichtenberg, E. M., Richardson, L. L., and Irwin, R. E. (2017). The behavioral ecology of nectar robbing: why be tactic constant? Curr. Opin. Insect Sci. 21, 14–18. doi: 10.1016/j.cois.2017.05.013
Buchmann, S. L. (1983). Buzz pollination in angiosperms. In C. E. Jones and R. J. Little (eds.), Handbook of Experimental Pollination Biology. (New York: Van Nostrand Reinhold).
Buchmann, S. L. (1985). Bees use vibration to aid pollen collection from non-poricidal flowers. J. Kansas Entomol. Soc. 58, 517–525.
Caruso, C. M., Eisen, K. E., Martin, R. A., and Sletvold, N. (2019). A meta-analysis of the agents of selection on floral traits. Evolution 73, 4–14. doi: 10.1111/evo.13639
Chittka, L. (1998). Sensorimotor learning in bumblebees: long-term retention and reversal training. J. Exp. Biol. 201, 515–524.
Chittka, L., and Raine, N. E. (2006). Recognition of flowers by pollinators. Curr. Opin. Plant Biol. 9, 428–435. doi: 10.1016/j.pbi.2006.05.002
Chittka, L., and Thomson, J. D. (1997). Sensori-motor learning and its relevance for task specialization in bumble bees. Behav. Ecol. Sociobiol. 41, 385–398. doi: 10.1007/s002650050400
Dukas, R. (1995). Transfer and interference in bumblebee learning. Anim. Behav. 49, 1481–1490. doi: 10.1016/0003-3472(95)90069-1
Dukas, R. (2001). Effects of perceived danger on flower choice by bees. Ecology Lett. 4, 327–333. doi: 10.1046/j.1461-0248.2001.00228.x
Fox, J., Weisberg, S., Adler, D., Bates, D., Baud-Bovy, G., Ellison, S., et al. (2012). Package ‘car’. (Vienna: R Foundation for Statistical Computing).
Gerber, B., Geberzahn, N., Hellstern, F., Klein, J., Kowalksy, O., and Wüstenberg, D., et al., (1996). Honey bees transfer olfactory memories established during flower visits to a proboscis extension paradigm in the laboratory. Anim. Behav. 52, 1079–1085. doi: 10.1006/anbe.1996.0255
Ishii, H. S., and Kadoya, E. Z. (2016). Legitimate visitors and nectar robbers on Trifolium pratense showed contrasting flower fidelity versus co-flowering plant species: could motor learning be a major determinant of flower constancy by bumble bees? Behav. Ecol. Sociobiol. 70, 377–386. doi: 10.1007/s00265-016-2057-7
Junker, R. R., Blüthgen, N., Brehm, T., Binkenstein, J., Paulus, J., Martin Schaefer, H., et al. (2013). Specialization on traits as basis for the niche-breadth of flower visitors and as structuring mechanism of ecological networks. Funct. Ecol. 27, 329–341. doi: 10.1111/1365-2435.12005
Keasar, T. (2000). The spatial distribution of nonrewarding artificial flowers affects pollinator attraction. Anim. Behav. 60, 639–646. doi: 10.1006/anbe.2000.1484
Keasar, T. (2020). Patterns of flower complexity in plant communities. Annu. Plant Rev. 3, 643–660. doi: 10.1002/9781119312994.apr0757
Krishna, S., and Keasar, T. (2018). Morphological complexity as a floral signal: from perception by insect pollinators to co-evolutionary implications. Int. J. Mol. Sci. 19:1681. doi: 10.3390/ijms19061681
Krishna, S., and Keasar, T. (2019). Bumblebees forage on flowers of increasingly complex morphologies despite low success. Anim. Behav. 155, 119–130. doi: 10.1016/j.anbehav.2019.06.028
Laverty, T. M. (1994). Bumble bee learning and flower morphology. Anim. Behav. 47, 531–545. doi: 10.1006/anbe.1994.1077
Lichtenberg, E. M., Richman, S. K., Irwin, R. E., and Bronstein, J. L. (2020). Competition for nectar resources does not affect bee foraging tactic constancy. Ecol. Entomol. 45, 904–909. doi: 10.1111/een.12866
Ma, X., Shi, J., Bänziger, H., Sun, Y., Guo, Y., and Liu, Z., et al., (2016). The functional significance of complex floral colour pattern in a food-deceptive orchid. Funct. Ecol. 30, 721–732. doi: 10.1111/1365-2435.12571
Muth, F., Keasar, T., and Dornhaus, A. (2015). Trading off short-term costs for long-term gains: how do bumblebees decide to learn morphologically complex flowers? Anim. Behav. 101, 191–199. doi: 10.1016/j.anbehav.2014.12.024
Olito, C., and Fox, J. W. (2015). Species traits and abundances predict metrics of plant–pollinator network structure, but not pairwise interactions. Oikos 124, 428–436. doi: 10.1111/oik.01439
Raine, N. E., and Chittka, L. (2007). Pollen foraging: learning a complex motor skill by bumblebees (Bombus terrestris). Naturwissenschaften 94, 459–464. doi: 10.1007/s00114-006-0184-0
Schiestl, F. P., and Johnson, S. D. (2013). Pollinator-mediated evolution of floral signals. Trends Ecol. Evol. 28, 307–315. doi: 10.1016/j.tree.2013.01.019
Keywords: Bombus terrestris, flower handling, flower morphology, generalization, learning, pollination
Citation: Krishna S and Keasar T (2021) Generalization of Foraging Experience Biases Bees Toward Flowers With Complex Morphologies. Front. Ecol. Evol. 9:655086. doi: 10.3389/fevo.2021.655086
Received: 20 January 2021; Accepted: 21 April 2021;
Published: 13 May 2021.
Edited by:
Eirik Søvik, Volda University College, NorwayReviewed by:
Aurore Avargues-Weber, UMR 5169 Centre de Recherches sur la Cognition Animale (CRCA), FranceNatalie Hempel de Ibarra, University of Exeter, United Kingdom
Copyright © 2021 Krishna and Keasar. This is an open-access article distributed under the terms of the Creative Commons Attribution License (CC BY). The use, distribution or reproduction in other forums is permitted, provided the original author(s) and the copyright owner(s) are credited and that the original publication in this journal is cited, in accordance with accepted academic practice. No use, distribution or reproduction is permitted which does not comply with these terms.
*Correspondence: Tamar Keasar, tkeasar@research.haifa.ac.il