- 1Department of Biological Sciences, George Washington University, Washington, DC, United States
- 2Computational Biology Institute, The Milken Institute School of Public Health, The George Washington University, Washington, DC, United States
- 3College of Science and Engineering, James Cook University, Cairns, QLD, Australia
- 4College of Life and Environmental Sciences, University of Exeter, Exeter, United Kingdom
- 5Department of Ecology and Evolutionary Biology, University of California, Irvine, Irvine, CA, United States
- 6Department of Earth System Science, University of California, Irvine, Irvine, CA, United States
- 7Life Sciences Department, The Natural History Museum, London, United Kingdom
Termites are important ecosystem engineers in tropical habitats, with different feeding groups able to decompose wood, grass, litter, and soil organic matter. In most tropical regions, termite abundance and species diversity are assumed to increase with rainfall, with highest levels found in rainforests. However, in the Australian tropics, this pattern is thought to be reversed, with lower species richness and termite abundance found in rainforest than drier habitats. The potential mechanisms underlying this pattern remain unclear. We compared termite assemblages (abundance, activity, diversity, and feeding group composition) across five sites along a precipitation gradient (ranging from ∼800 to 4,000 mm annual rainfall), spanning dry and wet savanna habitats, wet sclerophyll, and lowland and upland rainforests in tropical North Queensland. Moving from dry to wet habitats, we observed dramatic decreases in termite abundance in both mounds and dead wood occupancy, with greater abundance and activity at savanna sites (low precipitation) compared with rainforest or sclerophyll sites (high precipitation). We also observed a turnover in termite species and feeding group diversity across sites that were close together, but in different habitats. Termite species and feeding group richness were highest in savanna sites, with 13 termite species from wood-, litter-, grass-, dung-, and soil-feeding groups, while only five termite species were encountered in rainforest and wet sclerophyll sites—all wood feeders. These results suggest that the Australian termite diversity anomaly may be partly driven by how specific feeding groups colonized habitats across Australia. Consequently, termites in Australian rainforests may be less important in ecosystem processes, such as carbon and nutrient cycling during decomposition, compared with termites in other tropical rainforests.
Introduction
Termites play an important but under-recognized role in the functioning of tropical ecosystems (Bonachela et al., 2015; Ashton et al., 2019; Elizalde et al., 2020). Though best known as pests, fewer than 10% of the 3,000 termite species are categorized as such (Rouland-Lefèvre, 2011), while the majority are integral in carbon and nutrient cycling, breaking down wood, soil, grass and leaf litter. These activities contribute a significant amount of methane (CH4) to the atmosphere, estimated at 1–3% of the global CH4 budget (Nauer et al., 2018). In addition to CH4 release, their nitrogen-fixing activities and redistribution of organic matter, combined with soil movement and compaction through nest-building, provide hotspots of nutrients that support significant biological activity, especially in nutrient deficient or drought affected areas (De Oliveira-Filho, 1992; Jouquet et al., 2011; Ashton et al., 2019). Therefore, understanding termite distributions is key to understanding biogeochemical cycling during decomposition (Sugimoto et al., 2000), especially in the tropics.
In addition to irregular distributions over local space, termite biomass and abundance vary globally (Eggleton et al., 1996). Estimates have been made that termites comprise nearly 10% of animal biomass in the tropics, where they are most diverse and abundant, and are responsible for >55% of decomposition (Bignell, 2006; Jones and Eggleton, 2010; Griffiths et al., 2019). In tropical ecosystems, termite diversity, abundance and biomass increase with rainfall with the greatest values in lowland tropical rainforests (Eggleton, 2000; Bignell, 2006; Davies et al., 2015). For example, in some tropical African rainforests, termites make up 80% of insect biomass, with biomass as high as 300 kg ha–1 and richness as high as 67 species ha–1 (Sugimoto et al., 2000; Davies et al., 2003; Dahlsjö et al., 2014). Estimates for savanna or grassland habitats are <200 kg ha–1 termite biomass with sometimes fewer than eight species ha–1 (Sugimoto et al., 2000; Davies et al., 2015). Although patterns of termite species richness and abundance are affected by many local environmental factors as well as vegetation type, elevation, and latitude, in general, all metrics of termite prevalence in the tropics increase with increasing rainfall.
In contrast, Australia does not appear to follow the patterns observed in other tropical regions, as Australian termite abundance and diversity are thought to be higher in dry tropical ecosystems compared with rainforests, a pattern defined here as the Australian termite diversity anomaly (part of the global termite functional diversity anomaly) (Eggleton, 2000; Davies et al., 2003; Dahlsjö et al., 2014). This anomaly may be due to taxonomic disparities: in Australia, there is a marked deficiency of two of the most abundant and speciose termite feeding groups worldwide—the soil feeders and fungus farmers (Krishna et al., 2013). Whereas, in South/Central America, Africa, and Southeast Asia, soil-feeding and/or fungus-farming termites comprise a large portion of the total termite diversity (Davies et al., 2003), in Australia, these feeding groups are rare (soil feeders) or absent (fungus farmers). Their absence could explain why, despite being sampled extensively, Australian rainforests are very termite species-poor in comparison with other tropical rainforests (Calaby and Gay, 1959; Watson and Gay, 1991). Due to the lack of particular termite feeding groups, there is reason to believe that precipitation plays a different role in shaping termite assemblages in Australia as compared with other tropical regions. Preliminary evidence suggests that although termite activity is impacted by rainfall, it may be in a manner counter to other tropical regions (Cheesman et al., 2017). While we have qualitative descriptions of this anomaly, to date we lack quantitative assessments of how termites change with shifts in rainfall.
In this study, we quantified these responses across a wide rainfall spectrum at a local scale (<100 km) to explore which aspects of termite communities represent the termite diversity anomaly. We surveyed termite assemblages in tropical North Queensland measuring differences in termite abundance, activity, taxonomic richness and feeding group diversity across different habitats and rainfall levels. Under the termite diversity anomaly, we predicted that compared with rainforest, drier savanna sites would have greater termite (i) abundance, (ii) activity in wood pieces, and (iii) species and feeding group density. We explore the changes in termite assemblages across contrasting habitat types and examine the potential causes and consequences of the Australian termite diversity anomaly. We compare our findings with other published studies to contextualize how Australian termites respond to changes in rainfall when compared with other tropical regions.
Materials and Methods
Study Sites
We sampled termites from five sites across a 100 km transect in North Queensland, Australia, with annual rainfall ranging from 800 to 4,260 mm: (1) Pennyweight—dry savanna (Sav1), (2) Station Creek—open Eucalyptus woodland/savanna (Sav2), (3) Mt. Lewis—wet sclerophyll forest (Scl1), (4) Mt. Lewis—upland rainforest (Rft1), and (5) Daintree Rainforest Observatory–lowland rainforest (Rft2) (Figure 1 and Table 1). These sites are connected within continuous forest cover; at increasing distance from the coast, rainfall decreases, and habitats shift. All sites are located on the traditional homeland of the Kuku-Yalangi people. The first four of these sites are situated on Australian Wildlife Conservancy’s Brooklyn Sanctuary1, and the Daintree Rainforest Observatory2 is part of James Cook University. Three of the sites (Sav2, Scl1, and Rft1) change habitat type within 5 km of each other. All sites experience a distinct wet and dry season, with 77% of rainfall occurring between November and April (Cheesman et al., 2017). Because of this seasonality, we conducted termite sampling in both July 2019 (dry season), and December of either 2018 or 2019 (wet season). Three of the sites (Sav1, Sav2, and Scl1) had been burned 6 months to a year prior to surveys, and both of the savanna sites (Sav1 and Sav2) experience low-density feral cattle grazing. Rainfall was estimated using the 20-year trends (2000–2020) of annual rainfall of the closest grid point using SILO LongPaddock gridded data (Jeffrey et al., 2001).
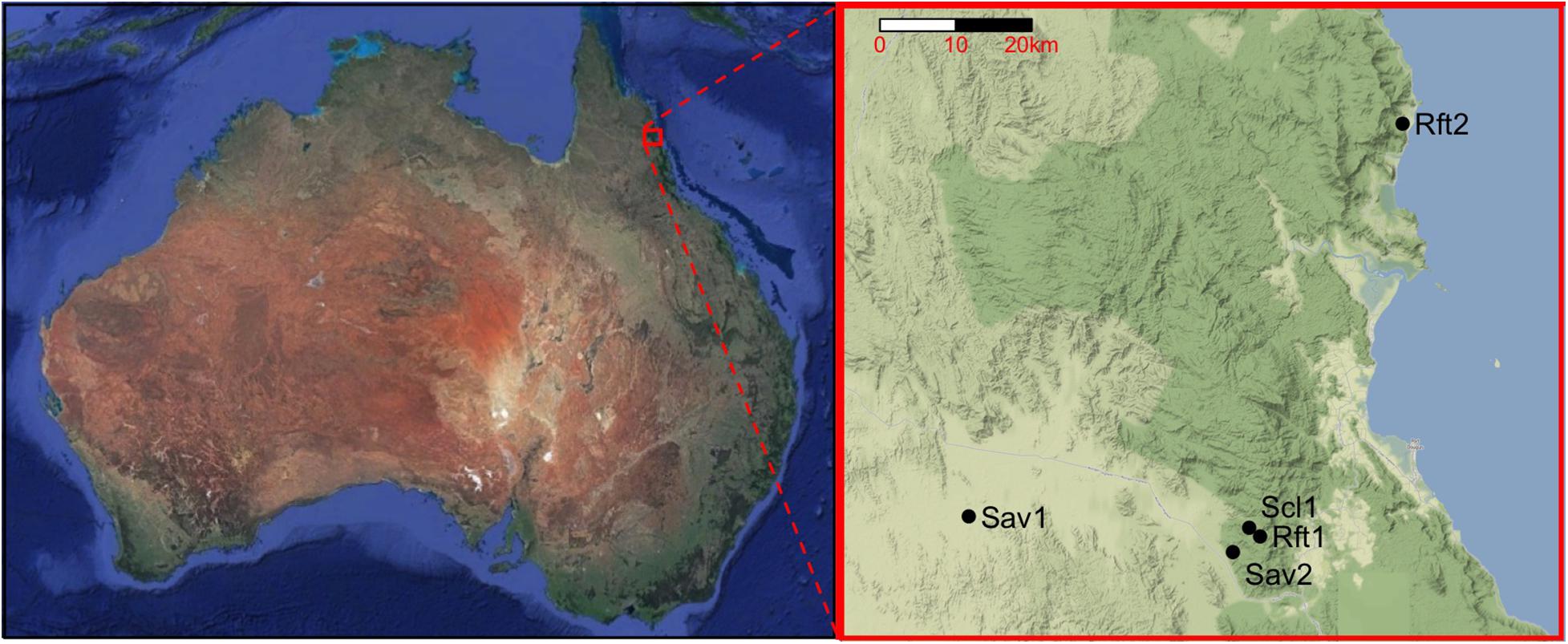
Figure 1. Location of the five sites in the study area in Northern Queensland, Australia: Sav1 (Pennyweight savanna), Sav2 (Station Creek savanna), Scl1 (Mt. Lewis sclerophyll), Rft1 (Mt. Lewis rainforest), and Rft2 (Daintree rainforest). Australia map by Google Earth, earth.google.com/web/. Local map tiles by Stamen Design, under CC BY 3.0. Data by OpenStreetMap, under ODbL.
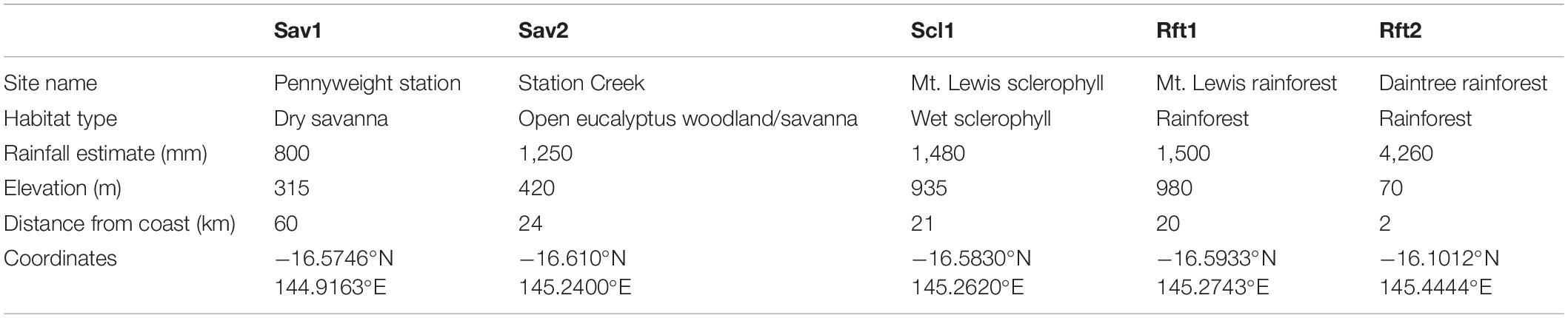
Table 1. Habitat type, rainfall, elevation, distance from coast, and localities for sites surveyed in this study.
Termite Sampling
Routine termite sampling normally includes four microhabitats: visible mounds, dead wood, soil, and arboreal habitats (Jones et al., 2005; Davies et al., 2021), however, since Australia lacks soil-feeding termites (Eggleton, 2000), soil pits seldom have termites in them (Dawes-Gromadzki, 2005). Additionally, methods for sampling arboreal termites are difficult and dangerous (Jones et al., 2005). To maximize termite encounters per effort, in this study, we targeted termite field surveys in termite mounds and dead wood only (Table 2 and Supplementary Table 1).
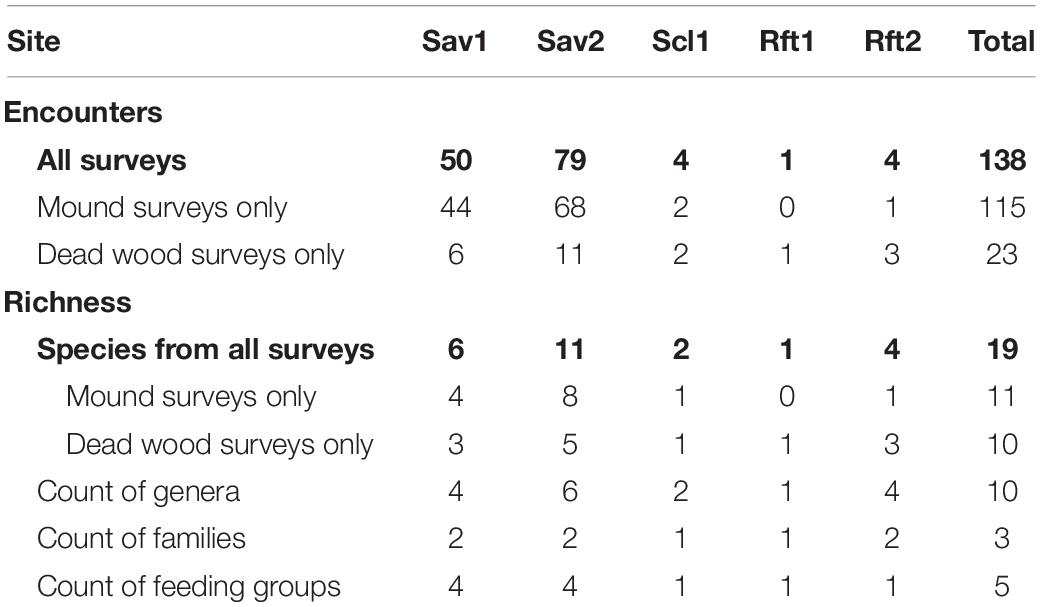
Table 2. Summary of termite encounters, taxonomic richness, and feeding group counts from surveys at Pennyweight dry savanna (Sav1), Station Creek open woodland/savanna (Sav2), Mt. Lewis wet sclerophyll (Scl1), Mt. Lewis rainforest (Rft1), and Daintree rainforest (Rft2).
Abundance: Termite Mound Sampling
Termites build mounds or nests in various shapes using soil mixed with termite saliva, soil and feces, ultimately constructing shelters for termite colonies and food storage space (Jouquet et al., 2016). As recommended for areas with dominant mound-building termites, we conducted mound surveys following Davies et al. (2021). We set up 50 m × 50 m plots at each of our five sites and divided them into 25 subplots (10 m × 10 m) for more accurate mound sampling and an exhaustive search of mounds in the given area. In each subplot, we mapped and recorded all mounds that were on the ground or low on trees as a measure of termite abundance (Figure 2). Each mound was initially measured (maximum height and maximum diameter), subsequently a small portion of mound material was removed to expose the termites. Where possible, we collected two soldiers and five workers from each mound preserved in 96% ethanol. Mound sampling took place in December 2018 (wet season) with the exception of the dry savanna site, Sav1, which was inaccessible at that time, and was sampled in July 2019. Because of the longevity of mounds, we do not expect that this difference in sampling season biased the data. We avoided sampling during the hottest part of the day when termite activity may decline in mounds. We compared the mean and standard deviation of mound abundance between savanna and non-savanna habitat types (wet sclerophyll and rainforest).
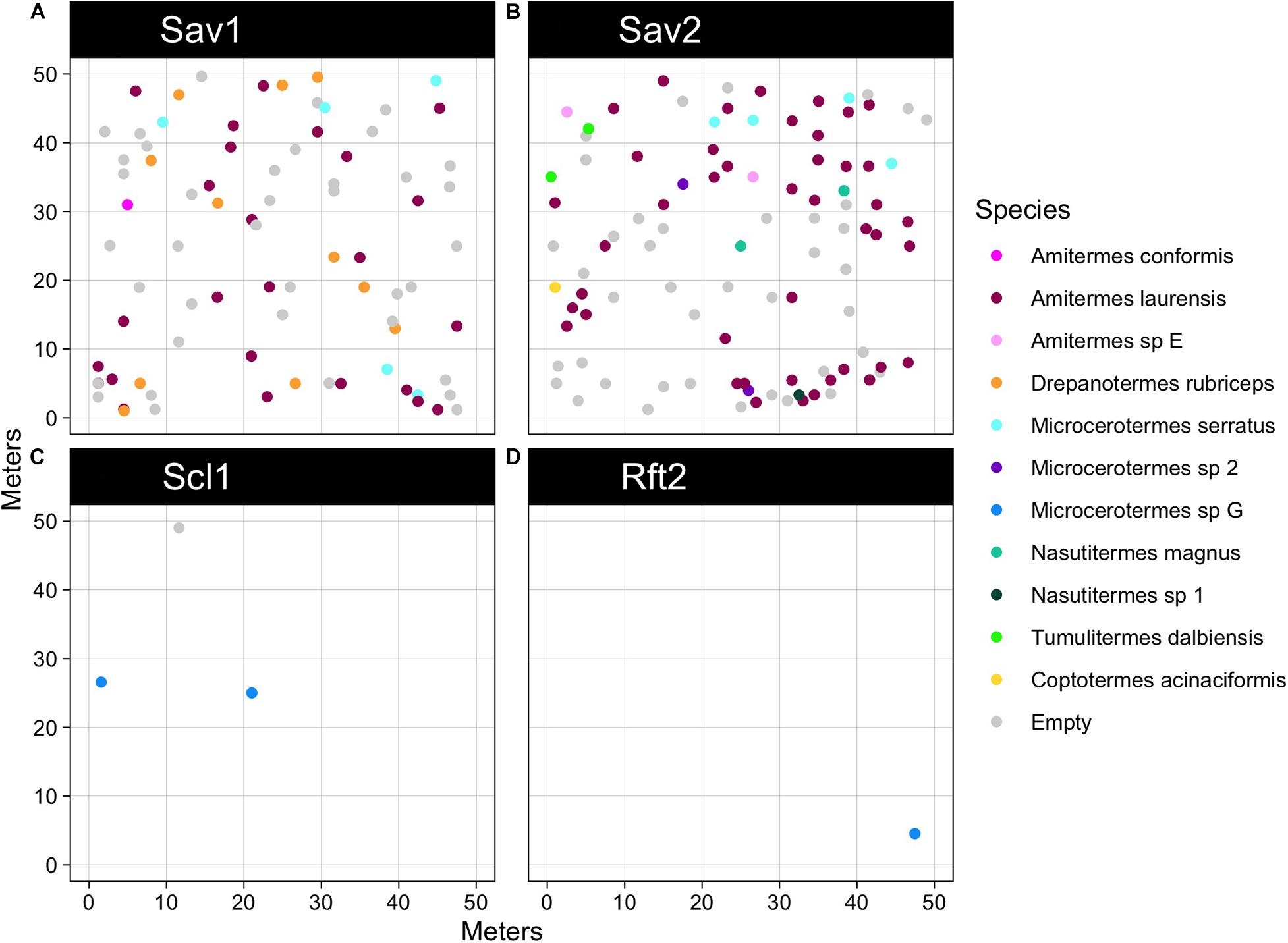
Figure 2. Mound abundance and mapping for each site: (A) Pennyweight savanna (Sav1) n = 80 mounds, (B) Station Creek savanna (Sav2) n = 98 mounds, (C) Mt. Lewis wet sclerophyll (Scl1) n = 3 mounds, (D) Daintree rainforest (Rft2) n = 1 mound. Mt. Lewis rainforest (Rft1) is not shown here because there were no mounds in the plot. Termite mounds colored by species, mapped across sites in quarter hectare plots. Gray points indicate empty mounds where no termites could be collected.
Activity: Dead Wood Sampling
Dead wood serves as both food and shelter for many termites (Korb, 2007). In each 50 m × 50 m plot (described above), we used a line intersect method (Warren and Olsen, 1964; Van Wagner, 1968; Kimber and Eggleton, 2018) to assess termite presence and damage in dead wood as a measure of termite activity. We laid out two 50 m transects in a randomly assigned position across the plots in both the wet season (Dec. 2018 or Dec. 2019) and the dry season (July 2019) for a total of four transects per plot. For each piece of dead wood with a diameter >2 cm that intersected a transect, we measured the length and diameter and used a drywall hammer to break the wood open in three places to search for termites. When one or more termites from the same morphospecies were present in a piece of wood, it was considered a single termite encounter. When more than one termite morphospecies was encountered in the same piece of wood, we considered it a separate encounter. For each termite encounter, we collected five workers and two soldiers into a 2 mL tube of 96% ethanol. We also recorded signs of damage on the wood from termites (piping, runways or termite tunnels) and/or fungus (white rot, fungus fruiting bodies or discoloration). We measured dead wood volume using the method described in Kimber and Eggleton (2018). Termite activity in Australia is influenced by seasonality, with the highest levels of termite activity during the transition period from wet to dry (Dawes-Gromadzki and Spain, 2003; Davies et al., 2012); we sampled dead wood at the end of both the wet and dry seasons to capture the maximum amount of termite activity in dead wood.
Termite activity was defined as (a) average number of termite encounters per 50 m transect (Figure 3), (b) number of termite encounters per dead wood volume, (c) percent of wood pieces with termites present, and (d) percent of dead wood pieces showing termite damage (Table 3). As wood-dwelling microbes, especially fungi, are the main alternate decay agents to termites in these systems, we also compared percentages of wood pieces damaged by fungi across habitat types. To assess the relationship between rainfall and habitat type and counts of termite encounters, wood pieces with termite damage, wood pieces with fungal damage per transect and total wood pieces, we built a generalized linear mixed model with habitat and rainfall as fixed predictors and sites as a random effect. We used a negative binomial distribution to account for zero-inflated variables, and a Poisson distribution for count of wood pieces, which was not zero inflated. We also fitted a generalized linear mixed-effects model to each metric jointly with its respective wood count using habitat and rainfall as fixed predictors and sites as a random effect using a binomial distribution. All statistical analyses were performed using R version 3.6.3 (R Core Team, 2020).
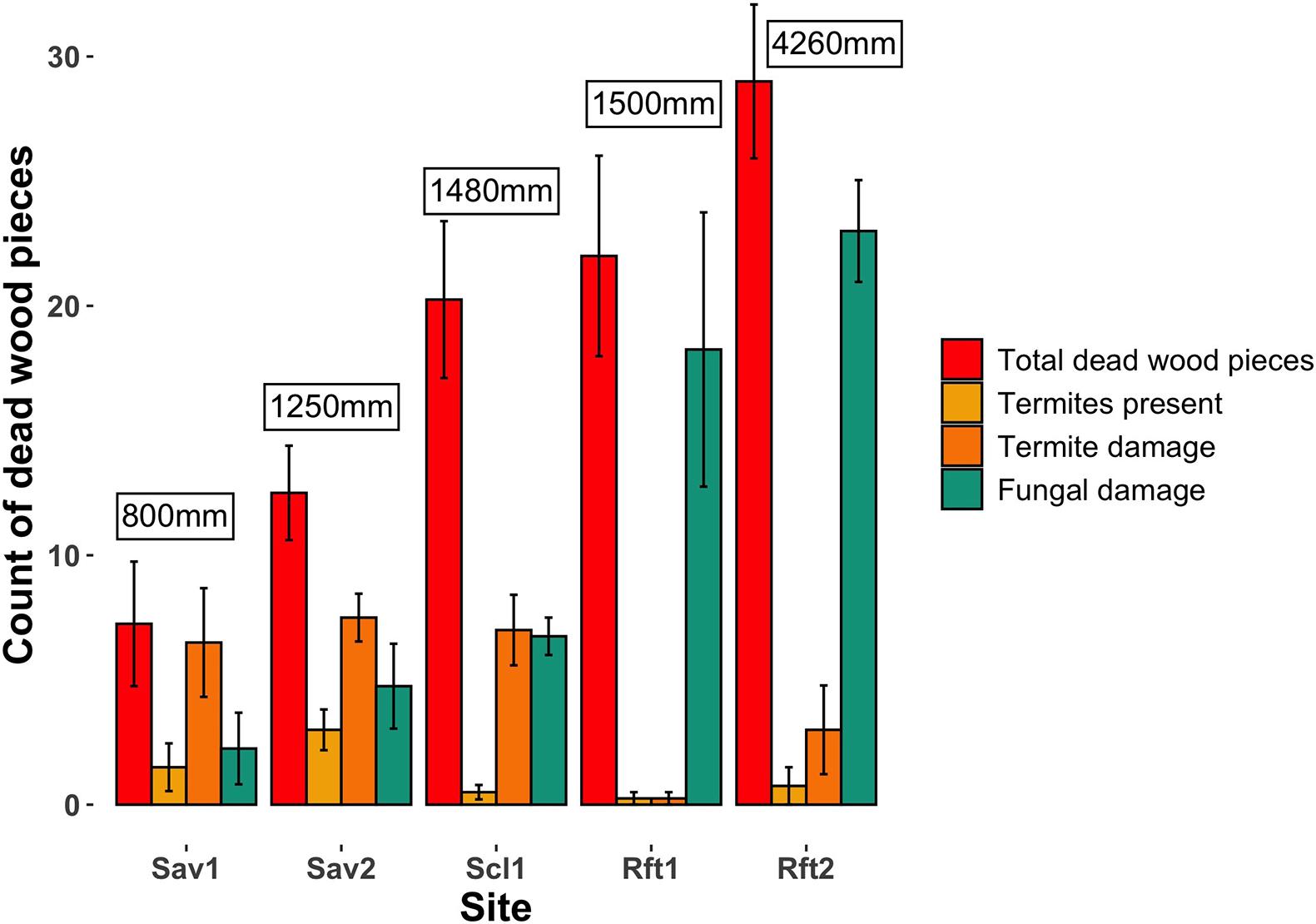
Figure 3. Average count of pieces of dead wood, dead wood with termites present, deadwood with termite damage, and dead wood with fungal damage, encountered across four 50 m transects from savanna (left) to rainforest (right) sites: Sav1 (Pennyweight savanna), Sav2 (Station Creek savanna), Scl1 (Mt. Lewis sclerophyll), Rft1 (Mt. Lewis rainforest), and Rft2 (Daintree rainforest). Error bars show standard error. Measurements in boxes show rainfall at each site.

Table 3. Termite encounters, percent occupancy or damage by termites or fungus, and items/volume of wood per transect from driest to wettest sites: Pennyweight dry savanna (Sav1), Station Creek open woodland/savanna (Sav2), Mt. Lewis wet sclerophyll (Scl1), Mt. Lewis rainforest (Rft1), and Daintree rainforest (Rft2).
In the rainforest and wet sclerophyll sites, we performed additional searching beyond the four dead wood transects because there were very few mounds. We broke open all pieces of dead wood in five 10 × 10 m subplots and recorded the total number of pieces of wood encountered and termite presence/absence. We collected termites found in these wood pieces where present.
Termite Identification
After affirming generic assignment using morphological features of soldiers, we used DNA barcoding for accurate species identification (Davies et al., 2021). For each of the 138 termite collections, we extracted the DNA from one worker using a Qiagen DNeasy Blood and Tissue kit. We amplified the COII gene from each termite using A-tleu and Btlys primers (Inward et al., 2007). Samples were sequenced at Eurofins Genomics. Resulting paired sequence contigs were assembled using Geneious and matched with the closest-related species on NCBI using BLAST (Johnson et al., 2008). If sequences had a unique >97% identity with a sequence from a described species on GenBank, they were assigned to that species. An additional 205 COII sequences from congeneric and Australian termites were downloaded from GenBank (Supplementary Table 2) and aligned with the sequences from this study using MAFFT (Katoh and Standley, 2013). We used IQTree (Nguyen et al., 2015) to reconstruct a maximum likelihood tree of the combined sequences. If a COII sequence from this study fell within a described species clade on the COII tree, we classified it as the given species. Otherwise, we labeled each cluster of our samples as sp, sp 1, sp 2, etc. A few of these clusters matched at high (>98%) sequence similarity with prospective species from a recent genomic study (Bourguignon et al., 2017), and we assigned them accordingly for consistency (Amitermes sp. E, Microcerotermes sp. G, Microcerotermes sp. I). Generated termite COII sequences have been deposited in GenBank under accessions MW772943-MW773117 (Supplementary Table 1).
We also used IQTree to generate a maximum likelihood tree of the COII sequences unique to this study with automatic model selection (GTR + F + G4), 1000 ultrafast bootstraps and an SH-like approximate likelihood ratio test with 1,000 replicates. Species, habitat, and feeding group were mapped onto the tips of the tree using iTOL (Letunic and Bork, 2019) to visualize taxonomic changes across habitat (Supplementary Figure 1).
Termites are on loan from James Cook University and will be lodged at the Natural History Museum, London, United Kingdom.
Taxonomic and Group Diversity
Taxonomic richness was measured at the species, genus, and family level (Table 2). Each species was assigned to one of the following feeding groups: wood-feeder, wood-and-dung feeder, litter-and-dung feeder, litter-and-grass feeder, grass feeder or soil feeder, based on literature from an Australian termite trait database (Cornwell, unpublished). Feeding group richness was measured as the number of feeding groups at each site. We compared termite communities across sites using the Simpson beta-diversity index (Supplementary Table 3) and visualized site differences using non-metric multidimensional scaling (nMDS) based on Bray-Curtis dissimilarity index (Supplementary Figure 2).
We fitted multivariate generalized linear models to predict the effect of combined and individual environmental variables (i.e., rainfall, elevation, latitude, distance from the coast) on termite species presence and abundance using R package mvabund (Wang et al., 2012). For the species presence models we used a binomial distribution; for the abundance models we used a Poisson distribution. For abundance and presence data we ran separate single predictive models for all species at all sites, assuming different environmental response for different species (GLM-LASSO, Brown et al., 2014). In these models the species presence or abundance were the response variables, and the environmental variables were predictors. These models apply a lasso penalty which sets non-significant terms to zero [lasso penalty = log(number of sites)].
Results
Through our mound and dead wood surveys, we encountered termites 138 times across our five sites in North Queensland, including specimens from 19 species, ten genera, three families and five feeding groups. The open eucalyptus woodland/savanna site (Sav2) had the highest species richness and number of encounters with 11 species and 79 encounters, followed by the dry savanna (Sav1) with 6 species and 50 encounters. The upland and lowland rainforests (Rft1, Rft2) and the sclerophyll site (Scl1) had fewer encounters and termite species (Table 2).
Mound Abundance
We observed a major reduction in termite mound abundance from savanna to forest habitats (Figure 2). We found that the mean mound abundance for savanna sites (M = 359 mounds ha–1, SD = 55.2) was 68 times greater than the mound abundance for non-savanna sites (M = 5.3 mounds ha–1, SD = 6.1). In the savannas, we found densities of 320 mounds ha–1 (Sav1) and 398 mounds ha–1 (Sav2), compared to 12 mounds ha–1 in the sclerophyll site (Scl1), zero in upland rainforest (Rft1), and four mounds ha–1 in lowland rainforest (Rft2). Most of the mounds sampled in either of the savannas were <1 m tall conical mounds, built by Amitermes laurensis (78/112 or 68% of occupied mounds), but termites from 11 species overall were represented in mounds across sites including Amitermes conformis, Amitermes sp. E, Drepanotermes rubriceps, Microcerotermes serratus, Microcerotermes sp. 2, Microcerotermes sp. G, Nasutitermes magnus and Nasutitermes sp. 1, Tumulitermes dalbiensis, and Coptotermes acinaciformis (Table 4). Of the savanna mounds in Sav1 (n = 80) and Sav2 (n = 98), only 52.5% (n = 44) and 69.4% (n = 68), respectively, were occupied by termites. In Scl1, only two of the three mounds were occupied.
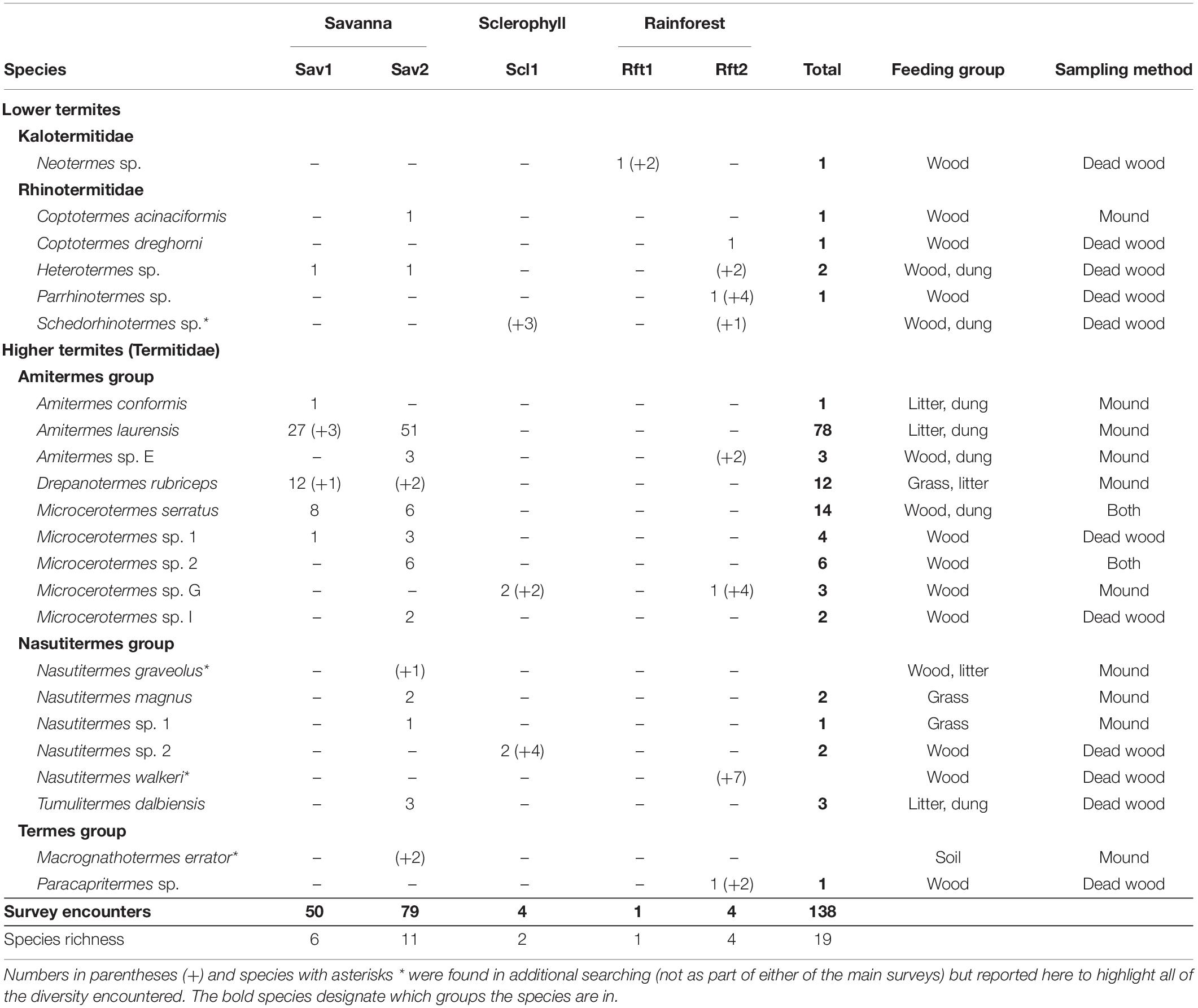
Table 4. Termite species found in five sites in North Queensland [Pennyweight dry savanna (Sav1), Station Creek open woodland/savanna (Sav2), Mt. Lewis wet sclerophyll (Scl1), Mt. Lewis rainforest (Rft1), and Daintree rainforest (Rft2)] with feeding groups and sampling method: Mound, dead wood, or both.
Termite Dead Wood Activity
We encountered the most pieces (M = 29) and highest volume (M = 192.3) of dead wood from transects in our lowland rainforest site Rft2. There was a positive effect (p < 0.05) of rainfall on pieces of wood per transect with fewer pieces of wood per transect in savanna habitats (p < 0.05). However, despite having fewer pieces of wood, we found both a higher number of termites in dead wood per transect (p < 0.05) and a higher proportion of termite encounters per wood count (p < 0.05) in savanna habitats (Table 3). Rainfall had a positive effect (p < 0.05) on the number of pieces of wood damaged by termites, but only after accounting for the negative effect of rainforest habitat on termite damage (p < 0.05). For either of the rainforest sites Rft1 and Rft2, only one of the four transects at each site resulted in termite encounters despite there being 18–36 wood pieces per transect. In savannas, the majority (60–90%) of pieces of dead wood were damaged by termites compared with 34% of wood pieces in the sclerophyll site, and 1 and 10% of the pieces of wood in the two rainforest sites, respectively (Table 3 and Figure 3). Even though we encountered more dead wood and there was more primary productivity in rainforest and sclerophyll transects, wood in savannas had more frequent termite dead wood damage and termites were more abundant. In contrast, fungal damage was higher in the rainforest compared to savannas (p < 0.05). Most (80%) of rainforest dead wood showed signs of fungal damage compared to only 30–40% of savanna and sclerophyll dead wood pieces that were damaged by fungi.
Seasonality only affected termite activity in dead wood in rainforest sites. In rainforests Rft1 and Rft2, we only found termites present or termite damage in dead wood transects at the end of the wet season. In the savanna and sclerophyll sites, seasonality was not related to termite activity. Ten species of termites from 23 total encounters were obtained from dead wood surveys across the five sites.
From additional searching of dead wood (non-survey), there were only four termite encounters in 144 pieces of dead wood in lowland rainforest Rft2 (3%), two termite encounters in 215 pieces of dead wood in upland rainforest Rft1 (1%) and eight encounters in 138 pieces of dead wood in the sclerophyll Scl1 (6%).
Termite Taxonomic Richness
We collected 19 termite species through mound and dead wood surveys, and an additional four species through non-survey searching. This total includes close to half of the known species diversity of the surrounding areas (50–65 species) (Watson and Abbey, 1993; Abensperg-Traun and Steven, 1997)3. While our surveys did not capture all termite diversity in the area (for example, we did not recover Mastotermes darwiniensis in these surveys though it was sighted in Sav1 on a previous trip), we sampled a similar proportion to what Jones and Eggleton (2000) estimate for typical termite surveys in Borneo, Malaysia and Cameroon (31–36% of termite species).
Of the 19 species collected from our mound and dead wood surveys, 14 were higher termites; nine were in the Amitermes group, four were from the Nasutitermes group, and one was from the Termes group. Five were lower termites—four from the family Rhinotermitidae and one from the family Kalotermitidae (Table 4). Surveys from all sites resulted in 10 genera of termites, with the highest generic diversity at Sav2 with 6 genera, and 4 at both Sav1 and Rft2.
The open eucalyptus woodland/savanna site (Sav2) had the most species at any site with 11 total species, with the second highest species richness at the dry savanna (Sav1, 6 species, Table 2). Although we only encountered termites four times in surveys in the Daintree rainforest (Rft2), each encounter was from a different species for four total species. The Sclerophyll site (Scl1) had only two species, and the site with the fewest species was the upland rainforest (Rft1), which had a single individual, Neotermes sp. Only one species of Neotermes is recorded in Australia, Neotermes insularis, but because the match with the N. insularis on GenBank was less than 90%, we expect this is a new species, at least for the continent of Australia.
Additional (non-survey) searching (Table 4 in parentheses) recovered four additional species for a total of 23 species throughout all 5 sites, reinforcing that our surveys uncovered a majority, but not all of the species present in these sites. Additional termite species collected were Amitermes sp. E, Heterotermes sp., Nasutitermes walkeri, and Schedorhinotermes sp. from Rft2, Schedorhinotermes sp. from Scl1, and Drepanotermes rubriceps, Macrognathotermes errator, and Nasutitermes graveolus from Sav2.
Out of the 183 COII sequences from our samples, only 114 (<65%) matched to a described species on GenBank with more than 97% identity, suggesting the need for more barcode sequencing and associated termite taxonomic work in this region.
Taxonomic Turnover Across Sites and Habitat Types
Although the dry savanna (Sav1) and open woodland/savanna (Sav2) shared four species and the wet sclerophyll (Scl1) and lowland rainforest (Rft2) shared one species, there was a complete turnover in species among the sites of different habitat types that were within 5 km of each other (Sav2, Scl1, and Rft1) (Figure 1, Supplementary Figure 2, and Supplementary Table 3). Four species were shared between savanna sites: Microcerotermes serratus, Microcerotermes sp. 1, Heterotermes sp., and Amitermes laurensis. One species, Microcerotermes sp. G was shared between Rft2 and Scl1, and is a close sister group to Microcerotermes serratus, shared between Sav1 and Sav2.
We observed a compositional difference in the termites across the habitat types. Of the five rainforest species encountered through surveys, three of them were lower termites. Two of the thirteen savanna species were lower termites (Table 4 and Supplementary Figure 1). Species of genera Microcerotermes, Nasutitermes, and Coptotermes were found across savanna and rainforest sites.
With the additional non-survey collections included, Rft2 shares two species with Sav2 and two species with Scl1, and Sav2 and Sav1 share five species.
Termite Diversity and Abundance in Response to Environmental Variables
We found evidence for a significant effect of environmental variables on termite species presence (p = 0.005). Both habitat type (p = 0.009) and elevation (p = 0.005) are significantly associated with termite local species diversity. Rainfall (p = 0.008), habitat type (p = 0.001) and distance from the coast (p = 0.007) are significantly associated with termite species turnover across communities, but the effect of rainfall and distance from coast on local species richness is statistically unclear (p > 0.05). There was also no clear effect of latitude on local species diversity or species turnover (p > 0.05).
Our GLM-LASSO model predicts that within our sites, species differ in their response to environmental variables in both presence and abundance (Supplementary Figures 3A,B).
Feeding Group Richness
Of the 138 termite encounters from this study, 22 were wood-feeders, 19 were wood-and-dung feeders, 3 were grass feeders, 12 were grass-and-litter feeders, and 82 were litter-and-dung feeders (Table 4). The savanna habitats had all five feeding groups. In the rainforest and sclerophyll sites, we found only wood-feeders. None of the 19 termite species encountered through surveys in this study were soil feeders or fungus-farming termites. However, one species of soil-feeding termite, Macrognathotermes errator, was encountered in additional non-survey collections in Sav2.
Discussion
Through this study, we quantified differences in termite abundance, activity, taxonomic richness, and feeding group diversity across habitat types and rainfall levels in North Queensland, Australia as a documentation of the termite diversity anomaly. Our results confirm quantitatively and more comprehensively the earlier findings that in Australia, termite mound abundance, dead wood activity, and species and feeding group richness is greater in savanna and limited in rainforest sites, contrasting with patterns seen in many other tropical regions (Bignell, 2006). Additionally, we found a complete turnover of observed species across habitat types despite sites being within only a few kilometers of each other. Finally, we found differences in feeding group distribution with rainforests supporting only wood-feeding termites, while savannas had wood, litter, dung, grass and soil feeders. Below, we contextualize the results of our study as we examine the relationship between termite diversity and rainfall globally and inspect the patterns that comprise the Australian anomaly. We discuss potential mechanisms for the Australian termite diversity anomaly and how these patterns in turn affect decomposition in the Australian tropics. The Australian termite diversity anomaly combined with local environmental factors affect termite distributions at a local and continent-wide scale in at least four aspects.
Patterns of Termite Assemblages in Australia
Australian Termite Species Richness Is Not Shaped by Rainfall
Similar to earlier work showing a positive correlation between generic richness and net primary productivity across three continents (Eggleton et al., 1994), our analysis of termite species richness patterns from surveys with methods similar to ours shows that globally, there is a significant positive correlation between termite species richness and rainfall (Figure 4 and Supplementary Table 4). However, our surveys in tropical Australia (Figure 4, circled triangles) show that while rainfall can have a positive effect on incidences of termite damage in wood within a habitat type, overall it is not associated with local termite diversity. The overall pattern of increased termite species richness with rainfall is driven by large, significant relationships seen in surveys in Africa and Central/South America, while in Australia, Madagascar, and Southeast Asia, these two variables are unrelated. This suggests that the termite diversity anomaly could apply to other continents, and perhaps there is a range of responses across continents of how tightly termite diversity is tied to rainfall. The anomaly is most extreme in Australia; sites in other tropical rainforests had 10–20 times the species richness of the wettest rainforest site from our study (Constantino, 1992; Eggleton et al., 1995; Gathorne-Hardy et al., 2001), and across habitat types, Australian termite species richness is lower than on other continents. This indicates that Australian termite richness is not shaped by rainfall in the same way it is on other continents, or at least not to the same degree. Further investigation is needed to gauge to what extent the anomaly shapes relationships between termite species and rainfall on other continents.
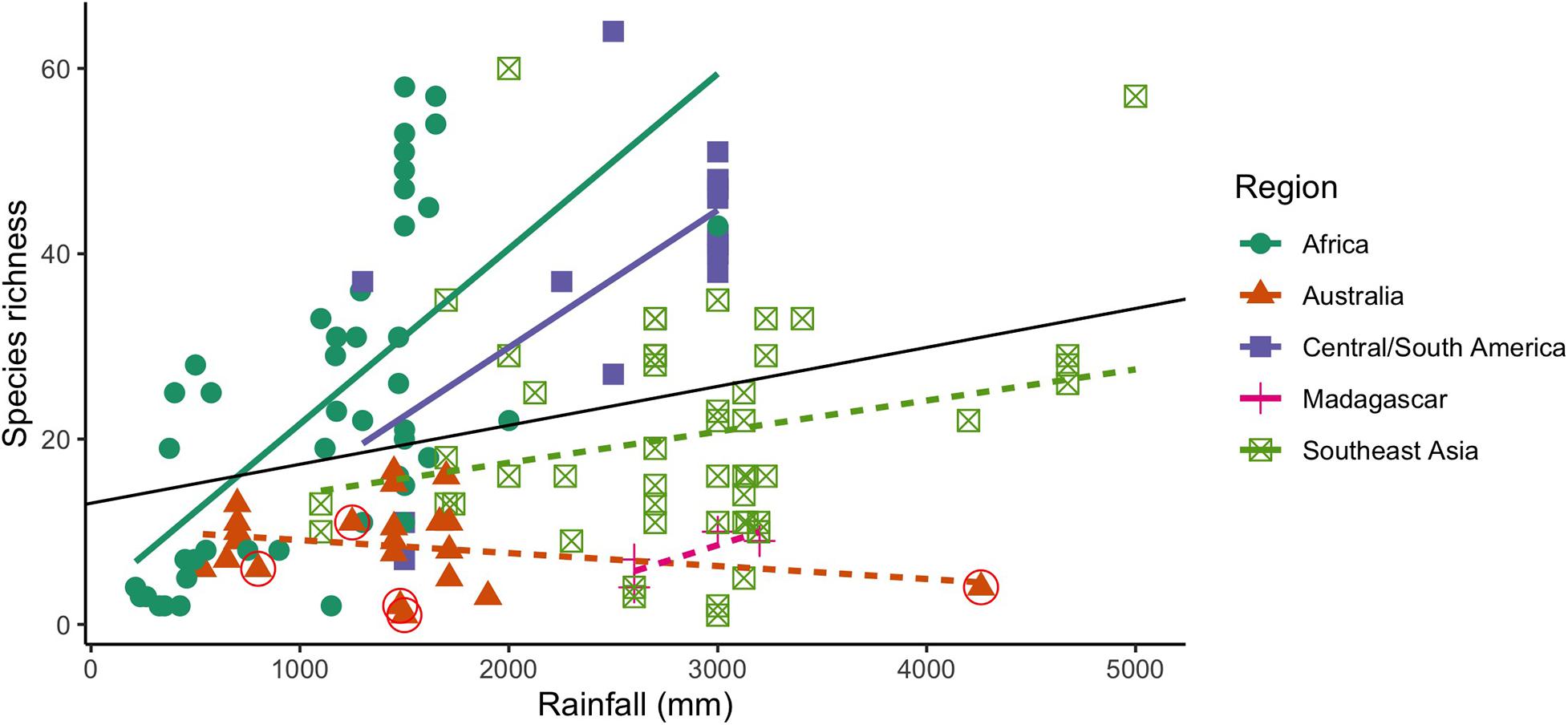
Figure 4. Relationship between termite species richness and rainfall from 143 tropical sites from 31 studies of surveys in 5 different regions (Supplementary Table 4) (Sands, 1965; Mathews, 1977; Wood, 1977; Abe and Matsumoto, 1979; Collins, 1980; Buxton, 1981; Wood et al., 1982; Braithwaite et al., 1988; Constantino, 1992; Holt et al., 1996; Gathorne-Hardy et al., 2001; Davies et al., 2003; Dawes-Gromadzki and Spain, 2003; Dawes-Gromadzki, 2005, 2008; Jamali et al., 2011; Davies et al., 2015; Houston et al., 2015). Lines indicate best linear model fit: Africa (slope: 0.019, R2adj: 0.396, p < 0.001), Central/South America (slope: 0.015, R2adj: 0.413, p: 0.002), Southeast Asia (slope: 0.0036, R2adj: 0.0311, p: 0.11), Madagascar (slope: 0.0070, R2adj: 0.46, p: 0.20), Australia (slope: −0.0014, R2adj: −0.0186, p: 0.28). Black line shows linear regression for all sites and rainfalls (slope: 0.0042, R2adj: 0.0751, p < 0.001). Dashed lines are not significant. Circles indicate sites from the current study.
At a local scale, other environmental factors influenced species richness in our sites more than rainfall. Both habitat type and elevation contributed significantly to local species diversity. Savanna sites had higher species richness than any of the other sites. The two sites with the lowest species diversity (Scl1 and Rft1) were 500–900 m higher elevation than any of the other sites; termite species richness on other continents is known to drop with even a 100 m increase in elevation (Gathorne-Hardy et al., 2001). Disturbance may have also played a role in species richness at our sites. For example, we encountered fewer than half as many species in dry savanna Sav1 as open woodland/savanna Sav2, which differed only 450 mm in rainfall, but Sav1 had more frequent cattle visitation and fire frequency. Termite species richness tends to be lower in heavily grazed areas (Holt et al., 1996), and we noticed several termite mounds had been toppled, potentially by cows. Although latitude is a good predictor of termite richness on other continents, Australia is known to differ in this regard (Abensperg-Traun and Steven, 1997), and our analysis also showed no significant effect of latitude on either alpha or beta species diversity. Our sites however, showed very little variation in latitude. Additionally, distance from the coast and rainfall contributed significantly to beta diversity. Our predictions show that species respond differentially to environmental variables implying that some species respond strongly to rainfall, but most of the species in our sites are better predicted by elevation, habitat type and disturbance.
Mound Abundance and Termite Activity in Dead Wood Are Greatest in Savanna Sites
Termite activity and mound abundance levels from our sites were within the known range of other savanna sites in North Queensland and Northern Territory (Holt et al., 1996; Dawes-Gromadzki and Spain, 2003; Dawes-Gromadzki, 2008). Termite mound densities were much higher in savannas than forested areas, with Amitermes laurensis responsible for much of this mound-building activity. This litter-and-dung-feeding termite builds the smallest and most fragile of the termite mounds we encountered. The high frequency of A. laurensis mounds could be due to a lower building cost than mounds that are sturdier and denser, or multiple mounds may be from the same termite colony. With an almost endless amount of grass and its corresponding litter in these savannas, mound-building species like A. laurensis, N. magnus, and D. rubriceps have become dominant, feeding on these substrates. Although there is also litter in rainforests, it may be that the type of litter prohibits these species from inhabiting rainforest habitats as well. Mound building may also be contingent on the makeup of the soil. In savannas in Australia, there is a negative relationship between soil fertility and the number of termite mounds (Ratcliffe et al., 1952; Goodland, 1965), which suggests that Australian mound-building termites have adapted to poor soil conditions.
It is remarkable that there are few termites in dead wood in the rainforest sites, especially in comparison with rainforests in other tropical regions. In rainforest dead wood surveys in Malaysia, termites are typically found in ∼20% of wood pieces (Kimber and Eggleton, 2018), but we found termites in fewer than 3% of wood pieces in North Queensland rainforests. Additionally, in our rainforest sites, we found that over 80% of pieces of dead wood had been damaged by wood-dwelling fungi, compared with less than 40% of dead wood in savanna sites. These results suggest that dead wood decomposition in Australian rainforests is especially dependent on fungal decomposers (Cheesman et al., 2017). It is unclear whether any of this pattern is driven by direct competitive interactions between termites and fungi. Viana-Junior et al. (2018) suggest that wood-inhabiting fungi are potential facilitators of fungi, but other studies (Um et al., 2013) say the opposite. Further testing through exclusion experiments is needed to determine whether fungi and termites regulate one another’s access to dead wood, and if Australian fungi contribute to the termite diversity anomaly.
High Turnover of Species Across Habitat Types
The high level of species turnover across different habitat types that are very close together (Sav2, Scl1, Rft2, Figure 1, Supplementary Figure 2, and Supplementary Table 3) suggests that habitat type is an important determinant of termite assemblage composition in this region of Australia. This habitat-related pattern may be a byproduct of how termite species sorted as they arrived to and radiated across Australia. Many Australian termites descended from the dry-adapted termites that first diversified in Australia (Arab et al., 2017), and there may not have been time for these lineages to invade rainforests. The rainforest termites may be from other lineages that drifted over at a different time. If these contrasting habitats were filled by lineages adapted to contrasting habitats, the shift between rainforest and savanna would form a strong barrier to dispersal for the termites.
Feeding Groups Are More Diverse in Savannas Than Rainforests
Our fourth key observation about the termite diversity anomaly is that habitat had strong consequences for the diversity of feeding groups. The rainforest termites in this study were limited not only in species richness and total abundance, but also in feeding groups. All of the termites found in our rainforest surveys were wood feeders, despite the presence of abundant litter, as well as some grass and dung. This finding is consistent with other Australian monsoonal rainforests in Darwin where only wood feeders were detected (Dawes-Gromadzki, 2005) and could be connected with the limited number of higher termites in rainforest sites, as most lower termites are wood feeders. The savanna termites were from a diversity of feeding groups, mainly dominated by litter-and-dung or grass-and-litter feeders, but also characterized by wood feeders, grass feeders, wood-and-litter, wood-and-dung, and soil feeders from both higher and lower termites. Similar to our rainforest finding for wood that despite numerous wood pieces present, few termites were found, we also noted high amounts of rainforest litter, but there were no litter-feeding termites present. This result suggests either that Australian termites are not targeting rainforest leaf litter or that amount of substrate is not the limiting factor for termites in rainforests.
Potential Mechanisms of the Australian Termite Diversity Anomaly
Without true soil-feeding termites or fungus farming termites, the termite communities in Australia are quite different from those on other continents. Australian termite communities lack three major clades of higher termites—Apicotermitinae, which are soil-feeding termites in the Neotropics and Afrotropics; Cubitermitinae, which are common soil-feeding termites in African tropics; and Macrotermitinae, which are fungus-farming termites in Africa and Southeast Asia (Braithwaite et al., 1988; Davies et al., 2003). The Nutrient-Poverty/Intense-Fire theory claims that most of the anomalous features of Australian flora and fauna are evolutionary consequences of adapting to low nutrient availability and frequent fire (Orians and Milewski, 2007). Australian soils are poor in P, Zn, I, Co, Mg, and Se, and do not receive nutrients in aerosols because of Australia’s distance from other continents (Braithwaite, 1990; Orians and Milewski, 2007). To explain the lack of fungus-growing termites, the Megacatalyst Theory (Milewski and Diamond, 2000), states that these species are unable to live in Australia because they are dependent on I, Co, and Se in soils for their growth and rapid reproduction. Additionally, studies from Malayan rainforests show a positive relationship between termite richness and soil nutrient content (Salick and Tho, 1984), suggesting that termites, especially those that are feeding on soil, would be unable to survive in Australia’s nutrient-poor soil. Of note, Braithwaite et al. (1988) found that in Darwin, Australia, termite richness was greatest in areas with poorer-quality soils, which may indicate that the Australian termites are particularly adapted for nutrient-poor soil conditions, albeit not as soil feeders.
It seems likely that at the core of the Australian termite diversity anomaly is a selection process influenced by the biogeographical history of termites in Australia. The first termites likely evolved at the end of the breakup of Pangea and at the beginning of the breakup of Gondwana ∼140 Ma (Bourguignon et al., 2014). When Termitidae, or the higher termites (comprising the bulk of termite diversity worldwide), evolved in Africa ∼50 Ma, rainforests were important for their diversification and cladogenesis (Eggleton, 2000). However, when closed canopy forests first evolved in other tropical regions, Australia was still at a very southern latitude, meaning the termites that evolved in these rainforests may have been unable to raft to or survive in Australia (Davies et al., 2003). Australia has been colonized by higher termites at least five times over the past 20 M years, many of these dispersal events happening when the arid biome was expanding in Australia, which made it possible for dry-adapted higher termites to diversify and take over ecosystems (Eggleton, 2000; Bourguignon et al., 2017). Most of these dispersals have happened through rafting across the ocean on pieces of wood (Bourguignon et al., 2016). Soil feeders and fungus farmers, which make up >50% of all termite species worldwide, do not produce supplementary reproductives that can raft in wood. Thus, Australia’s termite assemblage is made up mostly of nutrient-poor or dry adapted higher termites (Abensperg-Traun and Steven, 1997), which are mostly wood, grass, litter, and dung feeders.
Effect of Australian Termite Diversity Anomaly on Carbon and Nutrients
Only by gaining better quantitative estimates of termite assemblages can scientists uncover the impact of termites on ecosystem processes and predict how they shape carbon and nutrient turnover. These findings have important implications for modeling the breakdown of carbon and nutrients in tropical systems. As termites tunnel and build mounds, they increase soil bioturbation and nutrient levels, the effects of which can last for decades (Beaudrot et al., 2011). Termites differ from fungi in the speed at which they break down wood and the pathways by which they release carbon from wood (e.g., termites release both CO2 and CH4 as compared with fungi that just release CO2). In some tropical rainforests, termites are responsible for 58–64% of decomposition (Griffiths et al., 2019), but our observations suggest that a lack of termite activity in Australian rainforests could slow down dead wood turnover as compared with other tropical rainforests in the world.
Knowledge of the diversity, abundance, activity, and feeding group composition of termites is critical in modeling carbon cycling and storage. For example, fungus farming termites have the capacity to break down lignin. Without lignin decomposition, termites will instead translocate lignin from termite decayed wood into nests, mounds, and soils. Therefore, feeding groups, or in this case the lack of certain feeding groups, has implications for the way that carbon is stored or released into the surrounding environment. The Australian termite diversity anomaly is an important key to understanding the varying effects of termite assemblages on ecosystem services and carbon cycling around the globe.
Based on our study, greater quantification of termite responses to shifts in rainfall is warranted in other parts of the world. As we document biogeographic shifts in relationships between termites and environmental gradients, these differences need to be incorporated into how we model carbon and nutrient cycling. Our work suggests that such cycling is likely very different in Australia than in other tropical regions, with termites playing a smaller role as decomposers in rainforests in Australia compared with tropical rainforests in Africa and South America (Cheesman et al., 2017). As relationships between rainfall/habitat type and termite assemblages in Australia and elsewhere are clarified, we can better understand how termites are shaping ecosystems globally and incorporate them more accurately into our models of carbon turnover.
Data Availability Statement
The datasets presented in this study can be found in online repositories. The names of the repository/repositories and accession number(s) can be found in the article/Supplementary Material.
Author Contributions
RC, AZ, SA, and PE conceived of the project design. RC, AZ, PE, HF-M, LC, AC, and AY carried out the experiment. RC performed the analysis with help from HF-M, AY, and AZ. RC took lead in writing the manuscript. PE provided the conceptual background. AZ supervised the work. All authors contributed to the article and approved the submitted version.
Funding
Funding for this work was provided through National Science Foundation Grant DEB: 1655759; “Collaborative Research: NSFDEB-NERC: Tropical deadwood carbon fluxes: Improving carbon models by incorporating termites and microbes” to AZ, SA, and PE), through an NSF Graduate Research Fellowship No. 2019282279, and through a Mortensen grant from George Washington University Department of Biological Sciences.
Conflict of Interest
The authors declare that the research was conducted in the absence of any commercial or financial relationships that could be construed as a potential conflict of interest.
Acknowledgments
We are thankful for the logistical support provided by staff of James Cook University’s Daintree Rainforest Observatory. We thank the Australian Wildlife Conservation for allowing us to collect on their property and protecting our field sites from burning. We also thank Basil Byrne, Alexander Simpson, Baptiste Wijas, and Donna Davis for assistance in the field, and Nathan Lo for consultation on analysis and setup. We also thank two reviewers for their helpful comments.
Supplementary Material
The Supplementary Material for this article can be found online at: https://www.frontiersin.org/articles/10.3389/fevo.2021.657444/full#supplementary-material
Supplementary Figure 1 | Maximum likelihood tree of termite COII genes from termites in this study with sites and feeding groups.
Supplementary Figure 2 | Non-metric multidimensional scaling (NMDS) analysis representing similarity of termite species across sites.
Supplementary Figure 3 | Heat map from predictive model assuming different environmental response for different species (GLM-LASSO, Brown et al., 2014) showing association between (A) species presence/absence and (B) species abundance as responses and standardized environmental variables as predictors.
Supplementary Table 1 | Termite samples collected, their sites, collection method, and GenBank accession numbers.
Supplementary Table 2 | Accession numbers from 205 termite COII sequences from GenBank used for tree-building and termite species identification.
Supplementary Table 3 | Dissimilarity matrix of termite species across sites based on Simpson beta-diversity index.
Supplementary Table 4 | Compiled sources of termite surveys with region, site name, average annual rainfall, habitat type, and number of species (N).
Footnotes
- ^ https://www.australianwildlife.org/where-we-work/brooklyn/
- ^ https://www.jcu.edu.au/daintree
- ^ GBIF.org
References
Abe, T., and Matsumoto, T. (1979). Studies on the distribution and ecological role of termites in a lowland rain forest of west Malaysia:(3) Distribution and abundance of termites in Pasoh Forest Reserve. Jap. J. Ecol. 29, 337–351.
Abensperg-Traun, M., and Steven, D. (1997). Latitudinal gradients in the species richness of Australian termites (Isoptera). Aust. J. Ecol. 22, 471–477. doi: 10.1111/j.1442-9993.1997.tb00698.x
Arab, D. A., Namyatova, A., Evans, T. A., Cameron, S. L., Yeates, D. K., Ho, S. Y. W., et al. (2017). Parallel evolution of mound-building and grass-feeding in Australian nasute termites. Biol. Lett. 13:20160665. doi: 10.1098/rsbl.2016.0665
Ashton, L. A., Griffiths, H. M., Parr, C. L., Evans, T. A., Didham, R. K., Hasan, F., et al. (2019). Termites mitigate the effects of drought in tropical rainforest. Science 177, 174–177. doi: 10.1126/science.aau9565
Beaudrot, L., Du, Y., Rahman Kassim, A., Rejmánek, M., and Harrison, R. D. (2011). Do epigeal termite mounds increase the diversity of plant habitats in a tropical rain forest in peninsular Malaysia? PLoS One 6:e19777. doi: 10.1371/journal.pone.0019777
Bignell, D. E. (2006). “Termites as soil engineers and soil processors,” in Intestinal Microorganisms of Termites and Other Invertebrates, eds H. König and A. Varma (Berlin: Springer Berlin Heidelberg), 183–220. doi: 10.1007/3-540-28185-1_8
Bonachela, J. A., Pringle, R. M., Sheffer, E., Coverdale, T. C., Guyton, J. A., Caylor, K. K., et al. (2015). Termite mounds can increase the robustness of dryland ecosystems to climatic change. Science 347, 651–655. doi: 10.1126/science.1261487
Bourguignon, T., Lo, N., Cameron, S. L., Sobotn, J., Hayashi, Y., Shigenobu, S., et al. (2014). The evolutionary history of termites as inferred from 66 mitochondrial genomes. Mol. Biol. Evol. 32, 406–421. doi: 10.1093/molbev/msu308
Bourguignon, T., Lo, N., Jan, S., Sillam-dusses, D., Roisin, Y., and Evans, T. A. (2016). Oceanic dispersal, vicariance and human introduction shaped the modern distribution of the termites Reticulitermes, Heterotermes and Coptotermes. Proc. R. Soc. B Biol. Sci. 283, 1–9. doi: 10.1098/rspb.2016.0179
Bourguignon, T., Lo, N., Šobotník, J., Ho, S. Y. W., Iqbal, N., Coissac, E., et al. (2017). Mitochondrial phylogenomics resolves the global spread of higher termites, ecosystem engineers of the tropics. Mol. Biol. Evol. 34, 589–597. doi: 10.1093/molbev/msw253
Braithwaite, R. W. (1990). Australia’s unique biota: implications for ecological processes. J. Biogeogr. 17, 347–354. doi: 10.2307/2845364
Braithwaite, R. W., Miller, L., and Wood, J. T. (1988). The structure of termite communities in the Australian tropics. Aust. Ecol. 13, 375–391. doi: 10.1111/j.1442-9993.1988.tb00986.x
Brown, A. M., Warton, D. I., Andrew, N. R., Binns, M., Cassis, G., and Gibb, H. (2014). The fourth-corner solution – using predictive models to understand how species traits interact with the environment. Methods Ecol. Evol. 5, 344–352.
Buxton, A. R. D. (1981). Changes in the composition and activities of termite communities in relation to changing rainfall. Oecologia 51, 371–378.
Calaby, J. H., and Gay, F. J. (1959). “Aspects of the distribution and ecology of australian termites,” in Biogeography and Ecology in Australia, eds A. Keast, R. L. Crocker, and C. S. Christian (Dordrecht: Springer Netherlands), 211–223. doi: 10.1007/978-94-017-6295-3_12
Cheesman, A. W., Cernusak, L. A., and Zanne, A. E. (2017). Relative roles of termites and saprotrophic microbes as drivers of wood decay: a wood block test. Aust. Ecol. 43, 257–267. doi: 10.1111/aec.12561
Collins, N. M. (1980). The distribution of soil macrofauna on the west ridge of Gunung (Mount) Mulu. Sarawak. Oecologia 44, 263–275. doi: 10.1007/BF00572689
Constantino, R. (1992). Abundance and diversity of termites (Insecta: Isoptera) in two sites of primary rain forest in brazilian amazonia. Biotropica 24, 420–430. doi: 10.2307/2388613
Dahlsjö, C. A. L., Parr, C. L., Malhi, Y., Rahman, H., Meir, P., Jones, D. T., et al. (2014). First comparison of quantitative estimates of termite biomass and abundance reveals strong intercontinental differences. J. Trop. Ecol. 30, 143–152. doi: 10.1017/S0266467413000898
Davies, A. B., Eggleton, P., van Rensburg, B. J., and Parr, C. L. (2012). The pyrodiversity–biodiversity hypothesis: a test with savanna termite assemblages. J Appl Ecol. 49, 422–430. doi: 10.1111/j.1365-2664.2012.02107.x
Davies, A. B., Eggleton, P., van Rensburg, B. J., and Parr, C. L. (2015). Seasonal activity patterns of African savanna termites vary across a rainfall gradient. Insectes Soc. 62, 157–165. doi: 10.1007/s00040-014-0386-y
Davies, R. G., Eggleton, P., Jones, D. T., Gathorne-Hardy, F. J., and Hernández, L. M. (2003). Evolution of termite functional diversity: analysis and synthesis of local ecological and regional influences on local species richness. J. Biogeogr. 30, 847–877. doi: 10.1046/j.1365-2699.2003.00883.x
Davies, A. B., Parr, C. L., and Eggleton, P. (2021). A global review of termite sampling methods. Insectes Sociaux 68, 1–12. doi: 10.1007/s00040-020-00797-y
Dawes-Gromadzki, T. Z. (2005). The termite (Isoptera) fauna of a monsoonal rainforest near Darwin, northern Australia. Aust. J. Entomol. 44, 152–157. doi: 10.1111/j.1440-6055.2005.00452.x
Dawes-Gromadzki, T. Z. (2008). Abundance and diversity of termites in a savanna woodland reserve in tropical Australia. Aust. J. Entomol. 47, 307–314. doi: 10.1111/j.1440-6055.2008.00662.x
Dawes-Gromadzki, T. Z., and Spain, A. (2003). Seasonal patterns in the activity and species richness of surface-foraging termites (Isoptera) at paper baits in a tropical Australian Savanna. J. Trop. Ecol. 19, 449–456. doi: 10.1017/S0266467403003481
De Oliveira-Filho, A. T. (1992). Floodplain “murundus” of Central Brazil: evidence for the Termite-Origin hypothesis. J. Trop. Ecol. 8, 1–19. doi: 10.1017/S0266467400006027
Eggleton, P. (2000). “Global Patterns of Termite Diversity,” in Termites: Evolution, Sociality, Symbioses, Ecology, eds T. Abe, D. E. Bignell, and M. Higashi (Dordrecht: Springer Netherlands), 25–51. doi: 10.1007/978-94-017-3223-9_2
Eggleton, P., Bignell, D. E., Sands, W. A., Mawdsley, N. A., Lawton, J. H., Wood, T. G., et al. (1996). The diversity, abundance and biomass of termites under differing levels of disturbance in the mbalmayo forest reserve, southern cameroon. Philos. Trans. R. Soc. Lond. B Biol. Sci. 351, 51–68. doi: 10.1098/rstb.1996.0004
Eggleton, P., Bignell, D. E., Sands, W. A., Waite, B., Wood, T. G., and Lawton, J. H. (1995). The species richness of termites (Isoptera) under differing levels of forest disturbance in the Mbalmayo Forest Reserve. Southern Cameroon. J. Trop. Ecol. 11, 85–98.
Eggleton, P., Williams, P. H., and Gaston, K. J. (1994). Explaining global termite diversity: productivity or history? Biodiv. Conserv. 3, 318–330. doi: 10.1007/BF00056505
Elizalde, L., Arbetman, M., Arnan, X., Eggleton, P., Leal, I. R., Lescano, M. N., et al. (2020). The ecosystem services provided by social insects: traits, management tools and knowledge gaps. Biol. Rev. Camb. Philos. Soc. 95, 1418–1441. doi: 10.1111/brv.12616
Gathorne-Hardy, F., Syaukani, and Eggleton, P. (2001). The effects of altitude and rainfall on the composition of the termites (Isoptera) of the Leuser Ecosystem (Sumatra. Indonesia). J. Trop. Ecol. 17, 379–393. doi: 10.1017/s0266467401001262
Goodland, R. J. A. (1965). On Termitaria in a savanna ecosystem. Can. J. Zool. 43, 641–650. doi: 10.1139/z65-065
Griffiths, H. M., Ashton, L. A., Evans, T. A., Parr, C. L., and Eggleton, P. (2019). Termites can decompose more than half of deadwood in tropical rainforest. Curr. Biol. 29, 118–119. doi: 10.1016/j.cub.2019.01.012
Holt, J. A., Bristow, K. L., and Mcivor, J. G. (1996). The effects of grazing pressure on soil animals and hydraulic properties of two soils in semi-arid tropical Queensland. Soil Res. 34, 69–79. doi: 10.1071/sr9960069
Houston, W. A., Wormington, K. R., and Black, R. L. (2015). Termite (Isoptera) diversity of riparian forests, adjacent woodlands and cleared pastures in tropical eastern Australia. Aust. Entomol. 54, 221–230. doi: 10.1111/aen.12115
Inward, D. J. G., Vogler, A. P., and Eggleton, P. (2007). A comprehensive phylogenetic analysis of termites (Isoptera) illuminates key aspects of their evolutionary biology. Mol. Phylogenet. Evol. 44, 953–967. doi: 10.1016/j.ympev.2007.05.014
Jamali, H., Livesley, S. J., Grover, S. P., Dawes, T. Z., Hutley, L. B., Cook, G. D., et al. (2011). The importance of termites to the CH4 balance of a tropical savanna woodland of Northern Australia. Ecosystems 14, 698–709. doi: 10.1007/s10021-011-9439-5
Jeffrey, S. J., Carter, J. O., Moodie, K. B., and Beswick, A. R. (2001). Using spatial interpolation to construct a comprehensive archive of Australian climate data. Environ. Model. Softw. 1, 309–330. doi: 10.1016/S1364-8152(01)00008-1
Johnson, M., Zaretskaya, I., Raytselis, Y., Merezhuk, Y., McGinnis, S., and Madden, T. L. (2008). NCBI BLAST: a better web interface. Nucleic Acids Res. 36, W5–W9. doi: 10.1093/nar/gkn201
Jones, D. T., and Eggleton, P. (2000). Sampling termite assemblages in tropical forests: testing a rapid biodiversity assessment protocol. J. Appl. Ecol. 37, 191–203. doi: 10.1046/j.1365-2664.2000.00464.x
Jones, D. T., and Eggleton, P. (2010). “Global biogeography of termites: a compilation of sources,” in Biology of Termites: A Modern Synthesis, eds D. Bignell, Y. Roisin, and N. Lo (Dordrecht: Springer), 477–498. doi: 10.1007/978-90-481-3977-4_17
Jones, D. T., Verkerk, R. H. J., and Eggleton, P. (2005). “Methods for sampling termites,” in Insect Sampling in Forest Ecosystems, ed. S. R. Leather (Hoboken, NJ: John Wiley & Sons), 221–253.
Jouquet, P., Bottinelli, N., Shanbhag, R. R., Bourguignon, T., Traoré, S., and Abbasi, S. A. (2016). Termites: the neglected soil engineers of tropical soils. Soil Sci. 181, 157–165. doi: 10.1097/SS.0000000000000119
Jouquet, P., Traoré, S., Choosai, C., Hartmann, C., and Bignell, D. (2011). Influence of termites on ecosystem functioning. Ecosystem services provided by termites. Eur. J. Soil Biol. 47, 215–222. doi: 10.1016/j.ejsobi.2011.05.005
Katoh, K., and Standley, D. M. (2013). MAFFT multiple sequence alignment software version 7: improvements in performance and usability. Mol. Biol. Evol. 30, 772–780. doi: 10.1093/molbev/mst010
Kimber, A., and Eggleton, P. (2018). Strong but taxon-specific responses of termites and wood-nesting ants to forest regeneration in Borneo. Biotropica 50, 266–273. doi: 10.1111/btp.12517
Krishna, K., Grimaldi, D. A., Krishna, V., and Engel, M. S. (2013). Treatise on the Isoptera of the World. Bull. Am. Mus. Nat. Hist. 1, 977–1992.
Letunic, I., and Bork, P. (2019). Interactive Tree Of Life (iTOL) v4: recent updates and new developments. Nucleic Acids Res. 47, W256–W259. doi: 10.1093/nar/gkz239
Mathews, A. G. A. (1977). Studies on Termites From the Mato Grosso state. Brazil: Academia Brasileira de Ciências Rio de Janeiro.
Milewski, A. V., and Diamond, R. E. (2000). Why are very large herbivores absent from Australia? A new theory of micronutrients. J. Biogeogr. 27, 957–978. doi: 10.1046/j.1365-2699.2000.00436.x
Nauer, P. A., Hutley, L. B., and Arndt, S. K. (2018). Termite mounds mitigate half of termite methane emissions. Proc. Natl. Acad. Sci. U.S.A. 115, 1–6. doi: 10.1073/pnas.1809790115
Nguyen, L.-T., Schmidt, H. A., von Haeseler, A., and Minh, B. Q. (2015). IQ-TREE: a fast and effective stochastic algorithm for estimating maximum-likelihood phylogenies. Mol. Biol. Evol. 32, 268–274. doi: 10.1093/molbev/msu300
Orians, G. H., and Milewski, A. V. (2007). Ecology of Australia: the effects of nutrient-poor soils and intense fires. Biol. Rev. Camb. Philos. Soc. 82, 393–423. doi: 10.1111/j.1469-185X.2007.00017.x
R Core Team (2020). R: A Language and Environment for Statistical Computing. Vienna: R Foundation for Statistical Computing,.
Ratcliffe, F. N., Gay, F. J., and Greaves, T. (1952). Australian Termites. The Biology, Recognition, and Economic Importance of the Common Species. Melbourne: Commonwealth Scientific and Industrial Research Organization.
Rouland-Lefèvre, C. (2011). “Termites as pests of agriculture,” in Biology of Termites: A Modern Synthesis, eds D. E. Bignell, Y. Roisin, and N. Lo (Dordrecht: Springer Netherlands), 499–517. doi: 10.1007/978-90-481-3977-4_18
Salick, J., and Tho, Y. P. (1984). An analysis of termite faunae in malayan rainforests. J. Appl. Ecol. 21, 547–561. doi: 10.2307/2403428
Sands, W. A. (1965). Termite distribution in man-modified habitats in West Africa, with special reference to species segregation in the genus Trinervitermes (Isoptera, Termitidae, Nasutitermitinae). J. Anim. Ecol. 34, 557–571. doi: 10.2307/2449
Sugimoto, A., Bignell, D. E., and MacDonald, J. A. (2000). “Global impact of termites on the carbon cycle and atmospheric trace gases,” in Termites: Evolution, Sociality, Symbioses, Ecology, eds T. Abe, D. E. Bignell, and M. Higashi (Dordrecht: Springer), 409–435. doi: 10.1007/978-94-017-3223-9_19
Um, S., Fraimout, A., Sapountzis, P., Oh, D. C., and Poulsen, M. (2013). The fungus-growing termite Macrotermes natalensis harbors bacillaene-producing Bacillus sp. that inhibit potentially antagonistic fungi. Sci. Rep. 3:3250. doi: 10.1038/srep03250
Van Wagner, C. E. (1968). The line intersect method in forest fuel sampling. For. Sci. 14, 20–26. doi: 10.1093/forestscience/14.1.20
Viana-Junior, A. B., Côrtes, M. O., Cornelissen, T. G., and de Siqueira Neves, F. (2018). Interactions between wood-inhabiting fungi and termites: a meta-analytical review. Arthropod Plant Interact. 12, 229–235. doi: 10.1007/s11829-017-9570-0
Wang, Y. I., Naumann, U., Wright, S. T., and Warton, D. I. (2012). mvabund–an R package for model-based analysis of multivariate abundance data. Methods Ecol. Evol. 3, 471–474.
Warren, W. G., and Olsen, P. F. (1964). A line intersect technique for assessing logging waste. For. Sci. 10, 267–276. doi: 10.1093/forestscience/10.3.267
Watson, J. A. L., and Abbey, H. M. (1993). Atlas of Australian Termites. Collingwood: Csiro Publishing.
Watson, J. A. L., and Gay, F. J. (1991). “Isoptera (termites),” in The Insects of Australia, 2nd Edn, ed. CSIRO (Carlton, VIC: Melbourne University Press), 330–347.
Wood, T. G. (1977). Population of termites (Isoptera) in natural and agricultural ecosystems in southern Guinea savanna near Mokwa, Nigeria. Geo-Eco-Trop 1, 139–148.
Keywords: Isoptera, community assembly, ecosystem engineers, Blattodea, termite community assembly, carbon cycle, Australian tropical forest, savanna
Citation: Clement RA, Flores-Moreno H, Cernusak LA, Cheesman AW, Yatsko AR, Allison SD, Eggleton P and Zanne AE (2021) Assessing the Australian Termite Diversity Anomaly: How Habitat and Rainfall Affect Termite Assemblages. Front. Ecol. Evol. 9:657444. doi: 10.3389/fevo.2021.657444
Received: 22 January 2021; Accepted: 29 March 2021;
Published: 23 April 2021.
Edited by:
Solange Del Carmen Issa Ponce, Simón Bolívar University, VenezuelaReviewed by:
Olga Patricia Pinzon-Florian, Universidad Distrital Francisco Jose de Caldas, ColombiaArleu Barbosa Viana-Junior, Museu Paraense Emílio Goeldi, Brazil
Copyright © 2021 Clement, Flores-Moreno, Cernusak, Cheesman, Yatsko, Allison, Eggleton and Zanne. This is an open-access article distributed under the terms of the Creative Commons Attribution License (CC BY). The use, distribution or reproduction in other forums is permitted, provided the original author(s) and the copyright owner(s) are credited and that the original publication in this journal is cited, in accordance with accepted academic practice. No use, distribution or reproduction is permitted which does not comply with these terms.
*Correspondence: Rebecca A. Clement, cmViZWNsZW1AZ21haWwuY29t
†These authors have contributed equally to this work and share senior authorship