- Department of Biology, University of Washington, Seattle, WA, United States
Diversification of the ca. 275,000 extant flowering plant species has been driven in large part by coevolution with animal pollinators. A recurring pattern of pollinator shifts from hummingbird to hawkmoth pollination has characterized plant speciation in many western North American plant taxa, but in the genus Mimulus (monkeyflowers) section Erythranthe the evolution of hawkmoth pollination from hummingbird-pollinated ancestors has not occurred. We manipulated two flower color loci and tested the attractiveness of the resulting four color phenotypes (red, yellow, pink, and white) to naïve hawkmoths (Manduca sexta). Hawkmoths strongly prefer derived colors (yellow, pink, white) over the ancestral red when choosing an initial flower to visit, and generally preferred derived colors when total visits and total visit time were considered, with no hawkmoth preferring ancestral red over derived colors. The simple flower color genetics underlying this innate pollinator preference suggests a potential path for speciation into an unfilled hawkmoth-pollinated niche in Mimulus section Erythranthe, and the deliberate design of a hawkmoth-pollinated flower demonstrates a new, predictive method for studying pollination syndrome evolution.
Introduction
Darwin called the dramatic radiation of the ca. 275,000 flowering plant species “an abominable mystery,” though he recognized the potential role of the strong coevolutionary relationships between plants and their pollinators (Darwin, 1862). It is now clear that animal pollination is responsible for high rates of speciation in the flowering plants (Coyne and Orr, 2004; van der Niet and Johnson, 2012). Shifts between pollinator guilds (e.g., bumblebees, hummingbirds, hawkmoths, bats) often coincide with plant speciation events (Whittall and Hodges, 2007; Forest et al., 2014), and each pollinator guild is attracted by a different suite of floral traits (e.g., color, scent, pattern, shape, nectar reward, anthesis time) collectively known as a pollination syndrome (Fenster et al., 2004). Although some controversy around the validity of these syndromes exists (Ollerton et al., 2009; Wang et al., 2020), evidence suggests they are valid in the broad taxonomic sense (Rosas-Guerrero et al., 2014) as well as in specific taxonomic groups (Murúa and Espíndola, 2014). Extensive work has identified pollination syndromes among various plant families (Fenster et al., 2004), but the detailed genetics of traits involved in pollinator shift-driven plant speciation remain largely unresolved outside of a few key systems (Yuan et al., 2013; Fattorini and Glover, 2020). Have we learned enough about the genetic basis of the origin of flowering plant species to engineer a shift in pollinator guilds? Borrowing from Gould’s metaphor of the “tape of life,” (Gould, 1989) can we anticipate (rather than recapitulate) evolutionary trajectories, and, instead of replaying the tape of life, run the tape in fast forward? Can we predict, and then produce, a pollinator shift into a previously unfilled niche within a specific group, and is this shift genetically simple enough to potentially occur in the wild?
A recurring pattern of pollinator shifts from hummingbird to hawkmoth pollination has characterized plant speciation in many western North American taxa (e.g., Aquilegia, Ipomopsis, and Mimulus section Diplacus: Grant, 1993; Whittall and Hodges, 2007) and more globally (Rosas-Guerrero et al., 2014), but in the genus Mimulus (monkeyflowers) section Erythranthe (sensu Lowry et al., 2019) the evolution of hawkmoth pollination from hummingbird-pollinated ancestors has not occurred. “Hawkmoth flowers” share several characteristics with “hummingbird flowers,” including a large volume of dilute nectar and a long tubular corolla (Martins and Johnson, 2013; Johnson et al., 2016). But most hummingbird flowers are red (Grant, 1966; Rodríguez-Gironés and Santamaría, 2004; Lunau et al., 2011), hence not easily visible to hawkmoths, whose visual sensitivity does not extend into the longer wavelengths (Cutler et al., 1995). Hawkmoth flowers are usually white (or pale) and highly reflective in the visual wavelengths while lacking UV reflection (Grant, 1993; Goyret et al., 2008; Martins and Johnson, 2013; Johnson et al., 2016), adapted for detection by crepuscular and nocturnal hawkmoths.
Our goal is to design and synthesize a new Mimulus species (sensu Duffy et al., 2007; Villa et al., 2019), pollinated by hawkmoths and reproductively isolated from its red-flowered, hummingbird-pollinated ancestor, M. cardinalis. Several traits already present in Mimulus, including nocturnal anthesis, large nectar volume in M. cardinalis, and floral scent, suggest that the evolution of hawkmoth pollination in section Erythranthe should be genetically tractable and require few mutational changes. We set out to determine if the minimal combination of only two flower color changes—loss of anthocyanins and loss of carotenoids, either separately or together, both of which are found in wild M. lewisii and M. cardinalis—(Vickery, 1992; Wu et al., 2013) would be necessary and sufficient to change the behavior of a model hawkmoth, Manduca sexta. Given the extensive existing data on hawkmoth color preferences (White et al., 1994; Kelber, 1997; Goyret et al., 2008; Kuenzinger et al., 2019; and others), largely demonstrating a naïve preference for blue colors but the potential for training to prefer the more common hawkmoth-pollinated white colors (Goyret et al., 2008), we predicted that hawkmoths would prefer flowers with two mutational steps from the “ancestor” (white flowers, with the loss of both anthocyanins and carotenoids) over the ancestral state (red flowers). Moths might also show an intermediate preference for single mutational steps (yellow or pink flowers, with the loss of anthocyanins and carotenoids, respectively).
As a first step, we manipulated two flower color loci in M. cardinalis and tested the attractiveness of the resulting four color phenotypes (red, yellow, pink, white; Figure 1A) to naïve hawkmoths. If we are able to demonstrate a potential pro-hawkmoth change in Mimulus section Erythranthe via color shift, this suggests that a transition into the hawkmoth niche not yet fulfilled in this section might be a potential future evolutionary trajectory in the group resulting in a novel species.
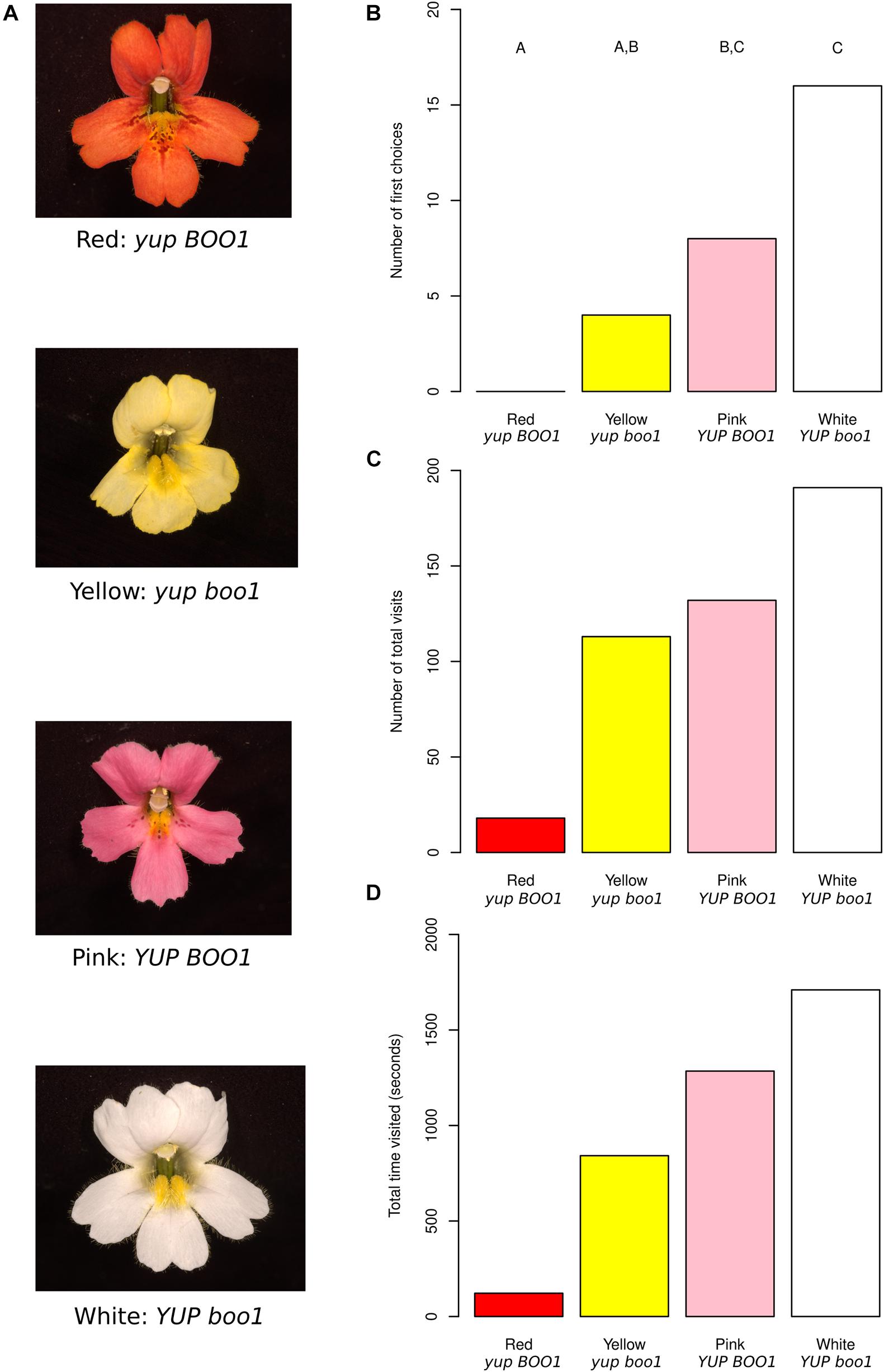
Figure 1. Mimulus color mutants and behavioral results. (A) Red, yellow, pink, and white Mimulus flowers from a single F2 population with their inferred genotypes. (B) First choices to the flowers across all moths, with letters indicating statistically significant differences between colors. (C) Total visits to the flowers across all moths. (D) Total time each flower color was visited across all moths. Data from individual moths were not pooled for statistics for (C,D) and therefore no statistical differences are shown (see section “Methods”).
Methods
Genetic Stocks
The red color of M. cardinalis flowers is produced by the combination of high concentrations of anthocyanin (pink) and carotenoid (yellow) pigments (Hiesey et al., 1971). Mimulus cardinalis Douglas ex Benth. (inbred line CE10, derived by single seed descent from a plant collected along the South Fork of the Tuolumne River, Yosemite, CA) was crossed to Mimulus lewisii Pursh (inbred LF10 line derived in the same way from the same area) homozygous for a recessive EMS-induced mutation at the BOO1 locus (Pince, 2009), producing an anthocyanin-less flower. M. lewisii is homozygous for a dominant suppressor of carotenoid pigmentation (YUP: Bradshaw and Schemske, 2003), and the mutant has the genotype boo1/boo1 YUP/YUP. M. cardinalis is homozygous for the alternative alleles (BOO1/BOO1 yup/yup). A resulting pink-flowered F1 offspring (BOO1/boo1 yup/YUP) was selfed to produce the segregating F2 study population (n = 500). Flowers of four colors were selected (Figure 1A), corresponding to the four combinations of alleles at the two flower color loci: red, similar in color to the “ancestral” M. cardinalis (BOO1 yup); pink (BOO1 YUP); yellow (boo1 yup); and white (boo1 YUP). Three F2 plants of each color were selected based on similarity of flower size, shape including petal reflexing, and nectar volume. Neither M. lewisii nor M. cardinalis reflect in ultraviolet wavelengths (Vickery, 1992; Owen and Bradshaw, 2011), meaning the only visible signals to hawkmoths (whose visual receptors peak at 357, 450, and 520 nm: UV, blue, and green respectively, Cutler et al., 1995) should be in the visible spectrum.
Experimental Animals
Carolina hawkmoths (Manduca sexta) were raised on artificial diet (Bell and Joachim, 1976) under controlled conditions at the University of Washington. As this typical diet is lacking in vitamin A, these moths likely differ in their visual sensitivity compared with wild moths or those reared on a complete diet, which might affect our behavioral results (Goyret et al., 2009). Hawkmoths were eclosed in full artificial lighting and were not fed or light-cycled prior to the experimental runs. Female hawkmoths eclosed four to six days prior to the experiment were used in all experiments, as this is within the approximate range where feeding motivation is highest (Goyret et al., 2007).
Test Chamber Experiments
Hawkmoths were tested in a 1 m × 1 m × 70 cm chamber constructed of black Coroplast (Coroplast, Dallas, TX) with a clear Plexiglas top for observation. The chamber was located within a darkroom illuminated with red safelight; the chamber itself was illuminated with a single blue-white LED emitting 2 lumens mounted on the Plexiglas top. One flower, including pedicel, of each color was mounted at a height of 50 cm on a long side of the symmetrical chamber using matte black tape. Each run was randomized for both flower color at each position and one of three parent plants for each color. As hawkmoths are able to see color in dim starlight (Kelber et al., 2002), the black Coroplast and blue-white LED may not fully reflect the visual environment and background contrast these hawkmoths would encounter in the wild; the darker background may have instead increased contrast between the color morphs and might affect preference (Kuenzinger et al., 2019).
Each hawkmoth was observed until an initial naïve choice—defined as proboscis extension and contact with the floral surface (Raguso and Willis, 2003)—was made. At that point, number of visits and time for each visit were recorded until nectar exhaustion or hawkmoth exhaustion. Nectar exhaustion was defined as a visit of one second or less and hawkmoth exhaustion as the hawkmoth becoming unwilling to fly. At this point the hawkmoth was removed and the flowers replaced before a new hawkmoth was introduced. Each moth was used for only one experiment.
Statistical Analysis
Initial visit data (first choice) were analyzed using a chi-square goodness-of-fit and individual ranking was done with pairwise chi-square tests with a sequential Bonferroni correction (Rice, 1989). Visit profiles were examined to rule out initial preference having an effect on further visits (e.g., moths preferring only their first flower color once they discover it is rewarding). Of 28 moths, 22 visited all three non-red colors, distributed evenly with initial preference (X2 = 1.375, p = 0.503, df = 2); additionally, 17 of the 28 moths visited another color as often as or more than their initial choice.
Chi-square goodness-of-fit tests were considered for total visit numbers and visit time, but a two-way chi-square test on the individual moth data showed that moths differed in their behavior for total visit time (X2 = 1449.638, p = 1.286 × 10–249, df = 81), and a Fisher’s exact test (chosen instead of chi-square due to the expected values violating the chi-square assumptions) showed that moths differed in their behavior for total visit number (p = 5 × 10–4) as well. Therefore, these data were not pooled across moths. Instead, visit time and visit number data from individual moths that made over 20 visits (7 of 28 moths) were analyzed using chi-square goodness-of-fit tests with sequential Bonferroni correction separately for each moth.
Results
Using naïve captive-bred female hawkmoths (Manduca sexta) in a dimly lit flight chamber with one flower of each color (Supplementary Figure 1), we counted their first choices, the total number of pollinator visits to each flower color, and the time spent on each color (Supplementary Table 1). A total of 28 hawkmoths were observed, resulting in a total of 454 visits with between 3 and 40 visits per moth. First choices differed significantly between color morphs (Figure 1B; n = 28, X2 = 20, df = 3, p = 1.70 × 10–4), with hawkmoths preferring white and pink morphs equally, also visiting yellow morphs equally to pink, and ignoring red morphs. These results indicate that hawkmoths are attracted to flowers with at least one allele substitution step (yellow or pink) from the red flower color characteristic of the ancestral hummingbird-pollinated M. cardinalis.
Since hawkmoths differed in their visitation profiles between moths (see section “Methods”), we present statistics for individual moths instead of pooling them when discussing total visit count (Figure 1C and Supplementary Figure 2A; n = 28) and total visit time (Figure 1D and Supplementary Figure 2B; n = 28). Five out of seven moths making more than 20 visits differed in their visit numbers between color morphs (p < 0.05), while one moth trended toward a difference (p = 0.0534) and one moth showed no difference (p = 0.572). All seven moths making more than 20 visits differed in their visit times to different colors (p < 0.05). Sequential Bonferroni correction for each moth demonstrated some general trends. When total visits were considered, all significant comparisons between red and another color showed higher visitation to the other color (white vs. red: 4 of 5 moths significant; pink vs. red: 3 of 5 moths significant; yellow vs. red: 2 of 5 moths significant). The same was true for total time, i.e., red flowers were visited for a shorter time overall by most moths (white vs. red: 6 of 7 moths significant; pink vs. red: 5 of 7 moths significant; yellow vs. red: 5 of 7 moths significant).
We also considered the transitions between flower morphs in the array (Figure 2). Nearly 20% of transitions were from the white flower back to the white flower, while same-flower transitions were rarer to pink (4.7%) and yellow (5.6%) flowers and absent for red flowers. As suggested by visitation numbers, transitions to red flowers were rare (1.4% from all three other colors). Transition rates were similar in both directions, i.e., transitions from white to pink were similar in frequency (12.7%) to transitions from pink to white (12.0%). As suggested by visit numbers, transitions to/from white and pink were more frequent than transitions to/from white and yellow, with transitions to/from pink and yellow intermediate. When accounting for the position of flowers in the array (Supplementary Figure 3), we see that red flowers were never visited when more than two positions away in the array, suggesting that moths could not see the red flowers very well from a distance, while white flowers were frequently visited when three positions away in the array, suggesting they are more conspicuous at a distance than any of the other colors. Anecdotally, most visits to red flowers seemed to occur after accidental contact between the moth and the flower, while most visits to white, pink, or yellow flowers appeared to be the result of more deliberate navigation, most likely due to the known visual receptor sensitivities of Manduca sexta, peaking in the UV to green range (Cutler et al., 1995) in combination with the higher brightness of the other color morphs. When moths did visit red flowers, however, they did not appear to visit for shorter amounts of time (white: 12.1 s/visit; pink: 9.2 s/visit; yellow: 8.1 s/visit; red: 9.7 s/visit), suggesting that they found red flowers equally rewarding once they were encountered.
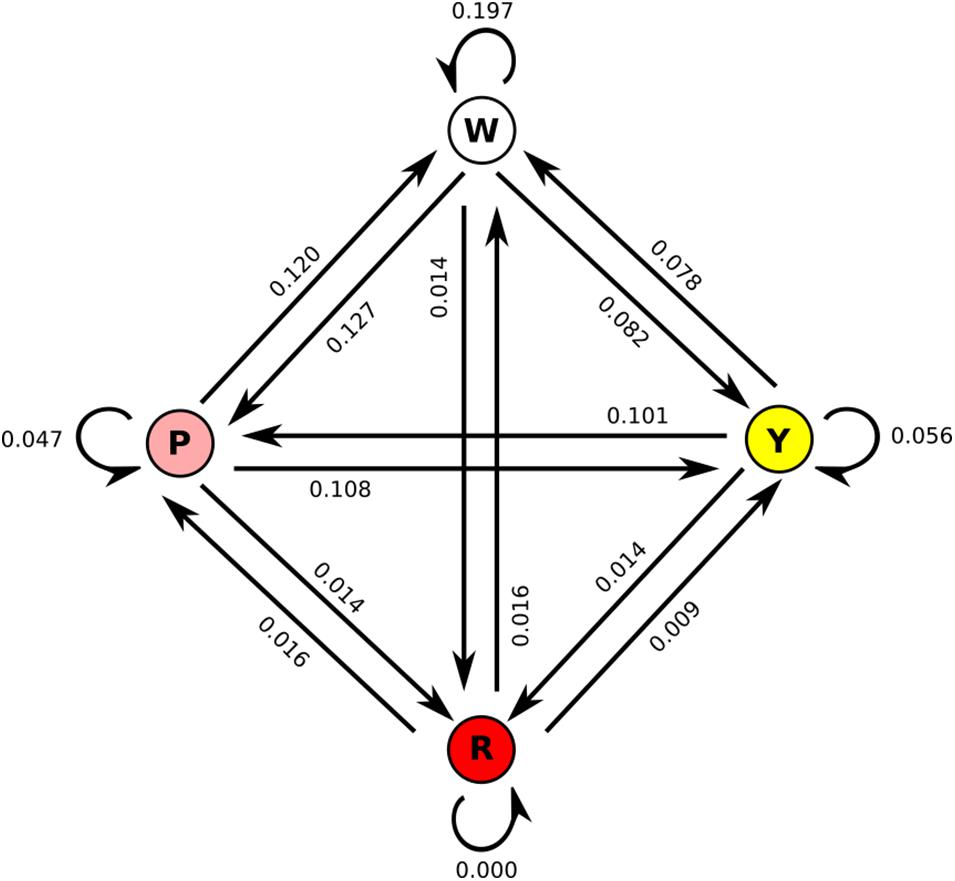
Figure 2. State change diagram for the four floral colors, with values averaged across all moths. All values in the diagram sum to 1, i.e., the numbers are the proportion of all total changes, not of changes to/from each color.
Discussion
When given the choice between four color phenotypes (red, yellow, pink, and white) representing four genotypes at two genetic loci, hawkmoths preferred the “derived” non-red colors for their initial choice, and generally preferred these colors to red (or in a few cases treated them equally) when total visits and total time were considered. Red flowers seemed less conspicuous in the flight chamber than other colors, consistent with the lack of visual sensitivity at these wavelengths (Cutler et al., 1995), while white flowers, with the highest brightness, were frequently visited even when at the opposite end of the array from the previous flower. These results are in agreement with existing data on hawkmoth color preferences (White et al., 1994; Kelber, 1997; Goyret et al., 2008; Kuenzinger et al., 2019; and others), though they may have been influenced by the experimental diet’s deficiency in beta-carotenes (see section “Methods”).
Testing these four flower color phenotypes with naïve hawkmoths in an experimental chamber has established the remarkably simple genetic basis (two mutational steps) of phenotypic change required to initiate a potential pollinator guild shift from hummingbirds to hawkmoths. Observations of pollinator preference and pollen movement in the native environment of M. cardinalis using near-isogenic lines for the YUP and boo1 alleles will be needed for a definitive assessment of reproductive isolation between the hummingbird-pollinated ancestral M. cardinalis and the rationally designed hawkmoth-pollinated derivatives with yellow, pink, or white flowers. Of note, many hummingbird-pollinated flowers are also white (Lunau et al., 2011), and thus our potential pollinator shift would reflect a “pro-hawkmoth” rather than an “anti-hummingbird” trait shift (sensu Castellanos et al., 2004).
Although not measured in this floral study population, floral scent and anthesis time are known to be important characteristics in the hawkmoth pollination syndrome (Faegri and van der Pijl, 1979). Mimulus lewisii and M. cardinalis, the two parent species of our test population, both emit moderate amounts of terpene volatiles (Byers et al., 2014a) that provoke electroantennographic responses in the hawkmoths Hyles lineata (Raguso et al., 1996) and Sphinx perelegans (Raguso and Light, 1998). In addition, Mimulus lewisii demonstrates nocturnal anthesis (Supplementary Video 1). In combination, the scent, nocturnal anthesis, and potential color shift (including a lack of UV reflection, White et al., 1994) would argue that a hawkmoth niche shift is possible in Mimulus section Erythranthe. Although Manduca sexta largely feeds on members of the Solanaceae (the nightshade family), we expect that other local hawkmoths (for which we are here using Manduca as a proxy) would be potential pollinators of these novel color variants should they arise in nature.
The classical approach to understanding plant speciation by pollinator shift is retrospective—sister taxa with different pollinators are analyzed for differences in key floral traits, often with known effects on pollinator preference (e.g., Bradshaw and Schemske, 2003; Streisfeld and Kohn, 2007; Whittall and Hodges, 2007; Byers et al., 2014a; Wessinger et al., 2014), and their underlying alleles (e.g., Schlüter et al., 2011; Hermann et al., 2013; Streisfeld et al., 2013; Byers et al., 2014b) to infer the evolutionary history of divergence from their common ancestor. But perhaps the most stringent test of our understanding of flowering plant diversification is the prospective approach we have used here. Darwin famously predicted that the Malagasy star orchid (Angraecum sesquipedale), which has a white flower and ca. 35cm nectar spur, must be pollinated by a (then-undiscovered) hawkmoth with a ca. 35 cm proboscis (Darwin, 1862). Building on similar predictions, and backed by experimental evidence, we have shown that critical steps toward the origin of a new, human-designed, hawkmoth-pollinated plant species can, likewise, be simple and predicted based upon a fundamental knowledge of pollination syndromes and genetics.
Data Availability Statement
The original contributions presented in the study are included in the article/Supplementary Material, further inquiries can be directed to the corresponding author/s.
Author Contributions
HB conceived the study and provided seed stocks. KB performed experiments and analyzed data and wrote the initial manuscript. Both authors contributed to manuscript editing and designed the study and methodology.
Conflict of Interest
The authors declare that the research was conducted in the absence of any commercial or financial relationships that could be construed as a potential conflict of interest.
Acknowledgments
We are indebted to T. Daniel, A. Mountcastle, D. Haak, T. Schuldiner-Harpaz, C. Miller, and K. Brandt for advice and assistance. Greenhouse and darkroom resources for this work were provided by the University of Washington Department of Biology and hawkmoths were kindly provided by T. Daniel. The manuscript has also benefited from feedback by KL, JG, and MK. This manuscript has previously appeared online at bioRxiv (Byers and Bradshaw, 2017).
Supplementary Material
The Supplementary Material for this article can be found online at: https://www.frontiersin.org/articles/10.3389/fevo.2021.658710/full#supplementary-material
Supplementary Figure 1 | Carolina hawkmoth (Manduca sexta) feeding from white Mimulus mutant in flight chamber.
Supplementary Figure 2 | Visit and visitation time data for individual moths. Individuals are presented in order of experiments, which were randomized, and the order is the same for parts (A,B). (A) Total number of visits broken down by floral color for each moth (from bottom to top of each bar: white, pink, yellow, red). (B) Total visit time broken down by floral color for each moth [as in (A)].
Supplementary Figure 3 | Moth transitions to flowers of each color based on distance flown to that flower. “Transition index” is the ratio of the percentage of time moths flew to a given color to the percentage of time that color was n positions away from the origin color, where a higher transition index indicates a larger percentage of the time that a given color was flown to.
Supplementary Table 1 | Experimental design and raw data from hawkmoth visitation experiments.
Supplementary Video 1 | Mimulus lewisii flowers open during nighttime, with full anthesis present at approximately 02:00 a.m.
References
Bell, R. A., and Joachim, F. G. (1976). Techniques for rearing laboratory colonies of tobacco hornworms and pink bollworms. Ann. Ent. Soc. Amer. 69, 365–373. doi: 10.1093/aesa/69.2.365
Bradshaw, H. D. Jr., and Schemske, D. W. (2003). Allele substitution at a flower colour locus produces a pollinator shift in monkeyflowers. Nature 426, 176–178. doi: 10.1038/nature02106
Byers, K. J. R. P., and Bradshaw, H. D. Jr. (2017). Rational design of a novel pollinator interaction. bioRxiv [preprint] doi: 10.1101/2020.06.26.173286
Byers, K. J. R. P., Bradshaw, H. D. Jr., and Riffell, J. A. (2014a). Three floral volatiles contribute to differential pollinator attraction in monkeyflowers (Mimulus). J. Exp. Biol. 217, 614–623. doi: 10.1242/jeb.092213
Byers, K. J. R. P., Vela, J. P., Peng, F., Riffell, J. A., and Bradshaw, H. D. Jr. (2014b). Floral volatile alleles can contribute to pollinator-mediated reproductive isolation in monkeyflowers (Mimulus). Plant J. 80, 1031–1042. doi: 10.1111/tpj.12702
Castellanos, M. C., Wilson, P., and Thomson, J. D. (2004). ‘Anti-bee’ and ‘pro-bird’ changes during the evolution of hummingbird pollination in Penstemon flowers. J. Evol. Biol. 17, 876–885. doi: 10.1111/j.1420-9101.2004.00729.x
Cutler, D. E., Bennett, R. R., Stevenson, R. D., and White, R. H. (1995). Feeding behavior in the nocturnal moth Manduca sexta is mediated mainly by blue receptors, but where are they located in the retina? J. Exp. Biol. 198, 1909–1917.
Darwin, C. R. (1862). On the Various Contrivances by Which British and Foreign Orchids are Fertilized by Insects, and on the Good Effects of Intercrossing. London: J. Murray.
Duffy, S., Burch, C. L., and Turner, P. E. (2007). Evolution of host specificity drives reproductive isolation among RNA viruses. Evolution 61, 2614–2622. doi: 10.1111/j.1558-5646.2007.00226.x
Fattorini, R., and Glover, B. J. (2020). Molecular mechanisms of pollination biology. Annu. Rev. Plant Biol. 71, 487–515. doi: 10.1146/annurev-arplant-081519-040003
Fenster, C. B., Armbruster, W. S., Wilson, P., Dudash, M. R., and Thomson, J. D. (2004). Pollination syndromes and floral specialization. Annu. Rev. Ecol. Evol. Syst. 35, 375–403. doi: 10.1146/annurev.ecolsys.34.011802.132347
Forest, F., Goldblatt, P., Manning, J. C., Baker, D., Colville, J. F., Devey, D. S., et al. (2014). Pollinator shifts as triggers of speciation in painted petal irises (Lapeirousia: iridaceae). Ann. Bot. 113, 357–371. doi: 10.1093/aob/mct248
Gould, S. J. (1989). Wonderful Life: The Burgess Shale and the Nature of History. New York, NY: W.W. Norton and Company.
Goyret, J., Kelber, A., Pfaff, M., and Raguso, R. A. (2009). Flexible responses to visual and olfactory stimuli by foraging Manduca sexta: larval nutrition affects adult behaviour. Proc. Royal Soc. B: Biol. Sci. 276, 2739–2745. doi: 10.1098/rspb.2009.0456
Goyret, J., Markwell, P. M., and Raguso, R. A. (2007). The effect of decoupling olfactory and visual stimuli on the foraging behavior of Manduca sexta. J. Exp. Biol. 210, 1398–1405. doi: 10.1242/jeb.02752
Goyret, J., Pfaff, M., Raguso, R. A., and Kelber, A. (2008). Why do Manduca sexta feed from white flowers? Innate and learnt colour preferences in a hawkmoth. Naturwissenschaften 95, 569–576. doi: 10.1007/s00114-008-0350-7
Grant, K. (1966). A hypothesis concerning the prevalence of red coloration in California hummingbird flowers. Am. Nat. 100, 85–97.
Grant, V. (1993). Origin of floral isolation between ornithophilous and sphingophilous plant species. Proc. Natl. Acad. Sci. 90, 7729–7733. doi: 10.1073/pnas.90.16.7729
Hermann, K., Klahre, U., Moser, M., Sheehan, H., Mandel, T., and Kuhlemeier, C. (2013). Tight genetic linkage of prezygotic barrier loci creates a multifunctional speciation island in Petunia. Curr. Biol. 23, 873–877. doi: 10.1016/j.cub.2013.03.069
Hiesey, W. M., Nobs, M. A., and Bjorkman, O. (1971). Experimental Studies on the Nature of Species. V. Biosystematics, Genetics, and Physiological Ecology of the Erythranthe Section of Mimulus. Washington, DC: Carnegie Institute of Washington.
Johnson, S. D., Moré, M., Amorim, F. W., Haber, W. A., Frankie, G. W., Stanley, D. A., et al. (2016). The long and the short of it: a global analysis of hawkmoth pollination niches and interaction networks. Func. Ecol. 31, 101–115. doi: 10.1111/1365-2435.12753
Kelber, A. (1997). Innate preferences for flower features in the hawkmoth Macroglossum stellatarum. J. Exp. Biol. 200, 827–836.
Kelber, A., Balkenius, A., and Warrant, E. J. (2002). Scotopic colour vision in nocturnal hawkmoths. Nature 419, 922–925. doi: 10.1038/nature01065
Kuenzinger, W., Kelber, A., Weesner, J., Travis, J., Raguso, R. A., and Goyret, J. (2019). Innate colour preferences of a hawkmoth depend on visual context. Biol. Lett. 15:20180886. doi: 10.1098/rsbl.2018.0886
Lowry, D. B., Sobel, J. M., Angert, A. L., Ashman, T.-L., Baker, R. L., Blackman, B. K., et al. (2019). The case for the continued use of the name Mimulus for all monkeyflowers. Taxon 68, 617–623. doi: 10.1002/tax.12122
Lunau, K., Papiorek, S., Eltz, T., and Sazima, M. (2011). Avoidance of achromatic colours by bees provides a private niche for hummingbirds. J. Exp. Biol. 214, 1607–1612. doi: 10.1242/jeb.052688
Martins, D. J., and Johnson, S. D. (2013). Interactions between hawkmoths and flowering plants in East Africa: polyphagy and evolutionary specialization in an ecological context. Biol. J. Linn. Soc. 110, 199–213. doi: 10.1111/bij.12107
Murúa, M., and Espíndola, A. (2014). Pollination syndromes in a specialized plant-pollinator interaction: does floral morphology predict pollinators in Calceolaria? Plant Biol. 17, 551–557. doi: 10.1111/plb.12225
Ollerton, J., Alarcón, R., Waser, N. M., Price, M. V., Watts, S., Cranmer, L., et al. (2009). A global test of the pollination syndrome hypothesis. Ann. Bot. 103, 1471–1480. doi: 10.1093/aob/mcp031
Owen, C. R., and Bradshaw, H. D. (2011). Induced mutations affecting pollinator choice in Mimulus lewisii (Phrymaceae). Arth.-Plant Interact. 5, 235–244. doi: 10.1007/s11829-011-9133-8
Pince, C. R. (2009). Evolution and Genetics of Floral Form in Mimulus. Ph.D. thesis, University of Washington, Seattle, WA.
Raguso, R. A., and Light, D. M. (1998). Electroantennogram responses of male Sphinx perelegans hawkmoths to floral and ‘green-leaf volatiles’. Entomol. Exp. Et Appl. 86, 287–293. doi: 10.1046/j.1570-7458.1998.00291.x
Raguso, R. A., Light, D. M., and Pichersky, E. (1996). Electroantennogram responses of Hyles lineata (Sphingidae: lepidoptera) to volatile compounds from Clarkia breweri (Onagraceae) and other moth-pollinated flowers. J. Chem. Ecol. 22, 1735–1766. doi: 10.1007/BF02028502
Raguso, R. A., and Willis, M. A. (2003). “Hawkmoth pollination in Arizona’s Sonoran Desert: behavioral responses to floral traits,” in Butterflies: Ecology and Evolution Taking Flight, eds C. Boggs, W. B. Watt, and P. R. Ehrlich (Chicago, IL: University of Chicago Press), 43–66.
Rice, W. R. (1989). Analyzing tables of statistical tests. Evolution 43, 223–225. doi: 10.2307/2409177
Rodríguez-Gironés, M. A., and Santamaría, L. (2004). Why are so many bird flowers red? PLoS Biol. 2:e350. doi: 10.1371/journal.pbio.0020350
Rosas-Guerrero, V., Aguilar, R., Martén-Rodríguez, S., Ashworth, L., Lopezaraiza-Mikel, M., Bastida, J. M., et al. (2014). A quantitative review of pollination syndromes: do floral traits predict effective pollinators? Ecol. Lett. 17, 388–400. doi: 10.1111/ele.12224
Schlüter, P. M., Xu, S., Gagliardini, V., Whittle, E., Shanklin, J., Grossniklaus, U., et al. (2011). Stearoyl-acyl carrier protein desaturases are associated with floral isolation in sexually deceptive orchids. Proc. Natl. Acad. Sci. U.S.A. 108, 5696–5701. doi: 10.1073/pnas.1013313108
Streisfeld, M. A., and Kohn, J. R. (2007). Environment and pollinator-mediated selection on parapatric floral races of Mimulus aurantiacus. J. Evo. Bio. 20, 122–132. doi: 10.1111/j.1420-9101.2006.01216.x
Streisfeld, M. A., Young, W. N., and Sobel, J. M. (2013). Divergent selection drives genetic differentiation in an R2R3-MYB transcription factor that contributes to incipient speciation in Mimulus aurantiacus. PLoS Genet. 9:e1003385. doi: 10.1371/journal.pgen.1003385
van der Niet, T., and Johnson, S. D. (2012). Phylogenetic evidence for pollinator-driven diversification of angiosperms. Trends Ecol. Evol. 27, 353–361. doi: 10.1016/j.tree.2012.02.002
Vickery, R. K. Jr. (1992). Pollinator preferences for yellow, orange, and red flowers of Mimulus verbenaceus and M. cardinalis. Great Basin Nat. 52, 145–148.
Villa, S. M., Altuna, J. C., Ruff, J. S., Beach, A. B., Mulvey, L. I., and Poole, E. J. (2019). Rapid experimental evolution of reproductive isolation from a single natural population. Proc. Natl. Acad. Sci. U.S.A. 116, 13440–13445. doi: 10.1073/pnas.1901247116
Wang, X., Wen, M., Qian, X., Pei, N., and Zhang, D. (2020). Plants are visited by more pollinator species than pollination syndromes predicted in an oceanic island community. Sci. Rep. 10:13918. doi: 10.1038/s41598-020-70954-7
Wessinger, C. A., Hileman, L. C., and Rausher, M. D. (2014). Identification of major quantitative trait loci underlying floral pollination syndrome divergence in Penstemon. Phil. Trans. Roy. Soc. B. 369:20130349. doi: 10.1098/rstb.2013.0349
White, R. H., Stevenson, R. D., Bennett, R. R., and Cutler, D. E. (1994). Wavelength discrimination and the role of ultraviolet vision in the feeding behavior of hawkmoths. Biotropica 26, 427–435. doi: 10.2307/2389237
Whittall, J. B., and Hodges, S. A. (2007). Pollinator shifts drive increasingly long nectar spurs in columbine flowers. Nature 447, 706–709. doi: 10.1038/nature05857
Wu, C. A., Streisfeld, M. A., Nutter, L. I., and Cross, K. A. (2013). The genetic basis of a rare flower color polymorphism in Mimulus lewisii provides insight into the repeatability of evolution. PLoS One 8:e81173. doi: 10.1371/journal.pone.0081173
Keywords: Mimulus, floral color, Manduca sexta, experimental evolution, pollination, reproductive isolation, speciation
Citation: Byers KJRP and Bradshaw HD Jr (2021) Rational Design of a Novel Hawkmoth Pollinator Interaction in Mimulus Section Erythranthe. Front. Ecol. Evol. 9:658710. doi: 10.3389/fevo.2021.658710
Received: 26 January 2021; Accepted: 11 March 2021;
Published: 29 March 2021.
Edited by:
Johannes Spaethe, Julius Maximilian University of Würzburg, GermanyReviewed by:
Klaus Lunau, Heinrich Heine University of Düsseldorf, GermanyMatthew Koski, Clemson University, United States
Joaquín Goyret, University of Tennessee at Martin, United States
Copyright © 2021 Byers and Bradshaw. This is an open-access article distributed under the terms of the Creative Commons Attribution License (CC BY). The use, distribution or reproduction in other forums is permitted, provided the original author(s) and the copyright owner(s) are credited and that the original publication in this journal is cited, in accordance with accepted academic practice. No use, distribution or reproduction is permitted which does not comply with these terms.
*Correspondence: Kelsey J. R. P. Byers, a2Vsc2V5LmJ5ZXJzQGppYy5hYy51aw==
†Present address: Kelsey J. R. P. Byers, John Innes Centre, Norwich, United Kingdom